the Creative Commons Attribution 4.0 License.
the Creative Commons Attribution 4.0 License.
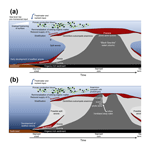
Biomarker evidence for the occurrence of anaerobic ammonium oxidation in the eastern Mediterranean Sea during Quaternary and Pliocene sapropel formation
Darci Rush
Helen M. Talbot
Marcel T. J. van der Meer
Ellen C. Hopmans
Ben Douglas
Jaap S. Sinninghe Damsté
The eastern Mediterranean Sea sedimentary record is characterised by intervals of organic-rich sapropel sediments, indicating periods of severe anoxia triggered by astronomical forcing. It has been hypothesised that nitrogen fixation was crucial in injecting the Mediterranean Sea with bioavailable nitrogen (N) during sapropel events. However, the evolution of the N biogeochemical cycle of sapropels is poorly understood. For example, the role of the complementary removal reactions like anaerobic ammonium oxidation (anammox) has not been investigated because the traditional lipid biomarkers for anammox, ladderane fatty acids, are not stable over long periods in the sedimentary record. Using an alternative lipid biomarker for anammox, bacteriohopanetetrol stereoisomer (BHT isomer), we present here for the first time N removal throughout the progression, e.g. formation, propagation, and termination, of basin-wide anoxic events. BHT isomer and ladderanes were analysed in sapropel records taken from three eastern Mediterranean sediment cores, spanning S1 to Pliocene sapropels. Ladderanes were rapidly degraded in sediments, as recently as the S5 sapropel. BHT isomer, however, was present in all sapropel sediments, as far back as the Pliocene, and clearly showed the response of anammox bacteria to marine water column redox shifts in high-resolution records. Two different N removal scenarios were observed in Mediterranean sapropels. During S5, anammox experienced Black Sea-type water column conditions, with the peak of BHT isomer coinciding with the core of the sapropel. Under the alternative scenario observed in the Pliocene sapropel, the anammox biomarker peaked at onset and termination of said sapropel, which may indicate sulfide inhibition of anammox during the core of sapropel deposition. This study shows the use of BHT isomer as a biomarker for anammox in the marine sediment record and highlights its potential in reconstructing anammox during past anoxic events that are too old for ladderanes to be applied, e.g. the history of oxygen minimum zone expansion and oceanic anoxic events.
- Article
(4146 KB) - Full-text XML
-
Supplement
(853 KB) - BibTeX
- EndNote
The typical hemipelagic, carbonate-rich, organic-carbon-poor sediment record of the eastern Mediterranean Sea is periodically interspersed with dark, organic-rich layers, known as sapropels. Sapropels typically have total organic carbon (TOC) content of >2 %, a striking contrast to non-sapropel TOC-lean sediments in the area, with TOC contents of generally 0.2 %–0.6 % (Cramp and O'Sullivan, 1999; Möbius et al., 2010). Evidence of Mediterranean sapropels can be found as far back 13.5 Ma in the sedimentary record. These features are the result of changes in astronomical forcing (Rossignol-Strick, 1983). Briefly, at maximum insolation, a wetter, localised monsoonal climate caused an increased discharge of freshwater into the eastern Mediterranean mainly from the African continent. This brought terrestrial nutrients into the oligotrophic eastern basin, while at the same time forming a layer of lower salinity water at the surface of the Mediterranean, inhibiting ventilation of deeper waters (for recent review see Rohling et al., 2015). The consequences of these climate-induced changes were (1) an increase in primary productivity followed by remineralisation and increased oxygen consumption in the underlying waters and (2) reduced resupply of oxygen to bottom waters leading to a ventilation crisis in the Mediterranean. Combined, this led to the total depletion of oxygen (anoxia) (Sinninghe Damsté and Hopmans, 2008) and raised levels of hydrogen sulfide (euxinia) during the most intense sapropel events (Menzel et al., 2002). The depletion of oxygen is believed to have started first in the pore and bottom waters and progressively shoaled over hundreds of years until the Mediterranean was characterised by photic zone anoxia/euxinia. There is some dispute over whether high-TOC values observed in sapropel sediments are primarily due to enhanced productivity, better preservation under anoxic conditions, or a combination of both.
The degree of oxygen depletion and presence of euxinic conditions for individual sapropels can vary according to the strength of astronomical forcing. A recent sapropel, S5 (121–128.5 ka), is the most well-developed Late Quaternary sapropel, characterised by high TOC content (ca. 7 %–8 %, max. 12 %), low bioturbation, and evidence for photic zone euxinia (Marino et al., 2007; Rohling et al., 2006; Struck et al., 2001). In comparison, however, certain Pliocene sapropels have been shown to contain much more elevated TOC content, of up to 30 % (Nijenhuis and de Lange, 2000), suggesting that sapropels from these periods are more developed. Spatial variation also occurs during sapropel formation, with TOC-rich horizons more commonly forming in the east of the basin, but with oxygen depletion not necessarily being stronger in the east (cf. Menzel et al., 2002).
The reorganisation of nutrient cycles, e.g. the phosphorus (P) cycle (Slomp et al., 2004) and the nitrogen (N) cycle (Calvert et al., 1992; Higgins et al., 2010), can impact the production and preservation of organic matter during the formation of Mediterranean sapropels. It has been shown that the anoxic water column during sapropel deposition caused enhanced regeneration of sedimentary P (Slomp et al., 2002). If sporadic vertical mixing then brought P to the photic zone, this would have further offset the Redfield ratio. The input of terrestrial N was likely insufficient to balance the enhanced sedimentary P remineralisation that occurred in the newly anoxic water column. This would have shifted phytoplankton communities towards diazotrophy (Higgins et al., 2010).
It appears that, under anoxic water column conditions in the Mediterranean, N might already have been a limiting nutrient. However, N can also be removed from the marine system via denitrification and anaerobic ammonium oxidation (anammox) (Ward, 2013). Anammox is the oxidation of ammonium using nitrite as the electron acceptor to produce N2 and is performed by anaerobic, sulfide-sensitive (Jensen et al., 2008), chemolithoautotrophic bacteria (Strous et al., 1999). Anammox has been observed in the water columns of modern oxygen minimum zones (Hamersley et al., 2007; Pitcher et al., 2011; Rush et al., 2012b) and euxinic basins (Jensen et al., 2008; Kuypers et al., 2003; Wakeham et al., 2012). The anammox process is also proposed to have been an important N-cycling process during Cretaceous oceanic anoxic events (Kuypers et al., 2004), removing bioavailable N for primary production and forcing a shift in the phytoplankton community to nitrogen-fixing organisms. However, whether anammox is a positive or negative feedback to anoxia during sapropel formation is poorly understood. For instance, is the removal of N from the system a way to quench primary productivity, the main source of the organic matter that is remineralised and consuming oxygen? Or does anammox simply contribute to the continuous removal of N, much in the same way it does in modern euxinic basins like the Cariaco Basin and the Black Sea? Studying the occurrence of anammox during the propagation of sapropels might help clarify the role anammox plays in maintaining anoxic conditions.
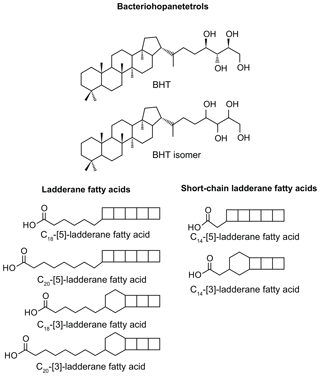
Figure 1Structures of anammox biomarker lipids used in this study. Bacteriohopanetetrol (BHT); bacteriohopanetetrol stereoisomer (BHT isomer), unknown stereochemistry; ladderane fatty acids with 3 or 5 cyclobutane moieties and 18 or 20 carbon atoms; short-chain ladderane fatty acids with 3 or 5 cyclobutane moieties and 14 carbon atoms.
The presence of anammox in water column and sediments is usually inferred from biomarker evidence of ladderane fatty acids. Ladderane lipids contain concatenated cyclobutane rings (Fig. 1) and are synthesised exclusively by anammox bacteria (Sinninghe Damsté et al., 2002). However, ladderanes are labile lipids and are known to be susceptible to diagenetic modification in the sediment record (Rush et al., 2012a; Jaeschke et al., 2008). An alternative biomarker for anammox bacteria in paleo-records has recently been proposed to be bacteriohopanetetrol isomer (BHT isomer; Fig. 1), a much less common stereoisomer of the ubiquitous BHT. Both BHT and BHT isomer are synthesised by marine anammox bacteria (Ca. Scalindua sp.) in roughly equal amounts (Rush et al., 2014b). Notably, the synthesis of BHT isomer has also been seen in a few other non-anammox, non-marine bacteria (van Winden et al., 2012; Rosa-Putra et al., 2001; Peiseler and Rohmer, 1992), and, therefore, some care should be taken when applying this lipid as a biomarker for anammox. However, anammox is the only known marine source of BHT isomer, and BHT isomer has been shown to correlate with ladderanes (Rush et al., 2014b) and metagenomic evidence for anammox bacteria (Matys et al., 2017) in modern oxygen-deficient marine settings.
Anammox bacteria use the carbon assimilation pathway acetyl coenzyme A (Strous et al., 2006). This pathway has been shown to result in the production of severely depleted ladderane fatty acids, observed in both cultures and in the Black Sea water column (δ13C ∼ −45 ‰; Schouten et al., 2004). In cultures, a C30 hopene also had similar isotopically depleted values as the ladderane fatty acids. Isotopically depleted BHT isomer (δ13C value of 51 ‰) was detected in a singular Pliocene sapropel sample in the Ionian Basin of the eastern Mediterranean (Ocean Drilling Program, ODP, Leg 160, Site 964) (Hemingway et al., 2018). In the same sample, BHT was 21 ‰ more enriched than BHT isomer. These results indicate that BHT isomer observed in a Mediterranean sapropel was derived from anammox bacteria.
Three Mediterranean sapropel records were analysed for ladderanes and/or BHT isomer. Here, for the first time, we report the presence of anammox in high-resolution Mediterranean sapropel records. We assess the periodic formation of anoxia in the paleo-record of a constrained basin and discuss its potential impact on N cycling.
2.1 Sapropel cores
2.1.1 Recent S1–S5 sapropels (Aegean Sea)
Core LC21 was collected at 1522 m water depth in the Aegean Sea (34∘40′ N, 26∘35′ E; Fig. 2) by the R/V Marion Dufresne in 1995. The split cores have been stored in the British Ocean Sediment Core Research Facility (BOSCORF) in Southampton, UK, and were subsampled in 2014 for BHT analyses. A total of 19 sediments were collected from sapropels S1, S3, S4, and S5, with a background sediment sample from outside each sapropel (taken from sections either before or after the sapropel event). Sediments were freeze-dried and stored at −20 ∘C until extraction for ladderanes and BHT isomer.
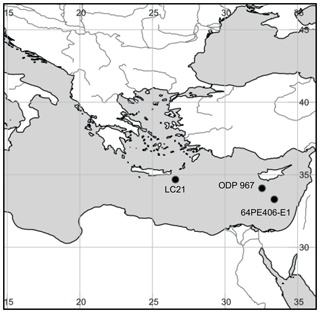
Figure 2Map of the eastern Mediterranean showing the locations of sapropel cores used in this study. LC21: low-resolution S1, S2, S3, and S5 sapropels from the Aegean Sea; 64PE406: high-resolution S5 sapropel from the Levantine Basin; ODP 967: high-resolution Pliocene sapropel from the Levantine Basin. Map created with SimpleMappr: David P. Shorthouse, 2010. SimpleMappr is an online tool to produce publication-quality point maps.
2.1.2 High-resolution S5 sapropel (Levantine Basin)
An S5 sapropel (core 64PE406-E1) was sampled in relatively high resolution (1 cm slices) from a piston core taken at a water depth of 1760 m in the eastern basin (Station 1; 33∘18′ N, 33∘24′ E; Fig. 2) aboard the R/V Pelagia in January 2016. The core was opened and slices were immediately transferred to geochemical bags and stored at −40 ∘C until sediments were freeze-dried in preparation for ladderanes and BHT isomer lipid extractions, as well as bulk TOC and isotopic analyses.
2.1.3 High-resolution Pliocene sapropel (Levantine Basin)
Site 967 of ODP Leg 160 was located at a water depth of 2560 m, south of Cyprus on the lower northern slope of Eratosthenes Seamount, in the eastern Levantine Basin (34∘04′ N, 32∘33′ E; Fig. 2). A total of 33 1 cm slices were selected from Hole B, Core 9, Sect. 6. These were from 40 to 87 cm within the core section, corresponding to depths of 79.70–80.16 m below sea floor (mbsf). This sample set included sediments from above, within, and below the sapropel horizon S65 (Grant et al., 2017), which was characterised by dark-coloured sediment. ODP Leg 160 shipboard biostratigraphic studies (Emeis and Party, 1996) and subsequent astrochronologies were used to tune the age model (Grant et al., 2017) that indicated the sediment for this core is of Pliocene age, 2.67 Ma. Sediment was freeze-dried and prepared for lipid extraction and TOC measurements.
2.2 TOC content
Around 0.1 g of freeze-dried sediments from LC21 and ODP 967 were weighed individually into a porous crucible. HCl (1 mL, 4 mM) was added to remove any inorganic carbon from the sediment. After HCl was drained, samples were neutralised with deionised water and were dried at 65 ∘C. TOC content of each sample was obtained by means of non-dispersive infrared spectrometry using a LECO CS230 analyser. A standard (Chinese stream sediment, NCS DC 73307; LGC, Teddington, UK) was analysed after every 10 samples to check accuracy. TOC content of the 64PE406-E1 sediments was determined by a Thermo Scientific Flash 2000 elemental analyser coupled to a Thermo Scientific Delta V isotope ratio monitoring mass spectrometer (EA-irMS) via a Conflo IV.
2.3 Bulk isotope measurements
Freeze-dried 64PE406-E1 sediments were analysed to determine both bulk δ15N and bulk δ13C values. For carbon isotope analysis, the sediment was first decalcified using a 2N HCl solution for approximately 18 h. The sediment was rinsed three times using double-distilled water and then freeze-dried again. δ15NTOC and δ13CTOC were measured using a Thermo Scientific EA-irMS (see above). The 15NTOC and 13CTOC are expressed relative to air and the Vienna Pee Dee Belemnite (VPDB) standard, respectively, and the isotope analysis precision was 0.2 ‰. For nitrogen isotope analysis, acetanilide, urea, and casein with predetermined isotope values were used as reference material; for carbon analysis benzoic acid and acetanilide were used.
2.4 Lipid extractions
2.4.1 Bligh and Dyer lipid extractions
Freeze-dried sediments from LC21 (Aegean Sea; S1–S5) and ODP 967 (Levantine Basin; Pliocene) were extracted at Newcastle University using a modified Bligh and Dyer extraction (BDE) method (Bligh and Dyer, 1959; Cooke et al., 2008). Briefly, freeze-dried material was extracted in a 10 : 5 : 4 () mixture of MeOH : chloroform : H2O in a Teflon tube, sonicated for 15 min at 40 ∘C, and centrifuged for 10 min. After the supernatant was transferred to a second tube, the residue was re-extracted two more times. The chloroform in the supernatant was separated and collected from the aqueous phase by making the H2O : MeOH ratio 1 : 1 (v:v). This procedure was repeated for the subsequent extractions. The collected BDE was dried by rotary evaporation in a round-bottom flask. Lipid extraction on the high-resolution S5 sapropel (64PE406-E1; Levantine Basin) was performed at NIOZ, where the extraction protocol was similar but instead used MeOH : dichloromethane (DCM) : phosphate buffer in the solvent mixtures (see Rush et al., 2012a). All BDEs were analysed for BHT isomer, where C16 platelet-activating factor (PAF) standard (1-O-hexadecyl-2-acetyl-sn-glycero-3-phosphocholine) was added as an internal standard. Aliquots from the 64PE406-E1 BDEs were taken for ladderane extractions.
2.4.2 Ladderane fatty acid extractions
Freeze-dried sediments of LC21 were also ultrasonically extracted three times using a DCM/methanol mixture (2 : 1 v∕v). Extracts of LC21 sediments were combined and dried using rotary evaporation yielding the total lipid extract (TLE), and residues were reserved for direct saponification. The LC21 TLEs, residues, and the aliquots of the 64PE406-E1 BDEs were saponified by refluxing with aqueous KOH (in 96 % MeOH) for 1 h. Fatty acids were obtained by acidifying the saponified samples to a pH of 3 with 1N HCl in MeOH and extracted using DCM. The fatty acids were converted to their corresponding fatty acid methyl esters (FAMEs) by methylation with diazomethane. N2 was not used to aid evaporation of solvents after derivatisation as this practice was found to significantly decrease the yield of volatile short-chain ladderane fatty acids (Rush et al., 2012a). Instead solvents were air-dried. Polyunsaturated fatty acids (PUFAs) were removed by eluting the sample over a small AgNO3 (5 %) impregnated silica column with DCM. Fatty acid fractions were stored at 4 ∘C until analysis.
2.5 Lipid analyses
2.5.1 Analysis of derivatised BHT isomer (Newcastle University)
A known amount of internal standard (5α-pregnane-3β, 20β-diol) was added to aliquots of LC21 and ODP 967 for BHT isomer analysis. Samples were acetylated in 0.5 mL of a 1 : 1 (v:v) mixture of pyridine and acetic anhydride at 50 ∘C for 1 h and then overnight at room temperature. Solvent was dried on a 50 ∘C heating block under a stream of N2. Samples were dissolved in MeOH : propan-2-ol (3 : 2; v:v) and filtered on 0.2 µm PTFE filters.
BHT isomer was analysed by high-performance liquid chromatography coupled to positive ion atmospheric pressure chemical ionisation mass spectrometry (HPLC/APCI-MS), using a data-dependent (3 events) scan mode on a system equipped with an ion trap MS (Talbot et al., 2007; van Winden et al., 2012). Semi-quantification of BHT isomer was achieved at Newcastle University using a BHT standard gifted by Michel Rohmer.
2.5.2 Analysis of non-derivatised BHT isomer (NIOZ)
BHT isomer of the high-resolution S5 sapropel (64PE406-E1) was measured on non-derivatised aliquots of BDEs using an ultra-high-performance liquid chromatography (UHPLC)-Q Exactive Orbitrap MS with electrospray ionisation (Thermo Fischer Scientific, Waltham, MA), using a method for analysis of intact polar lipids according to Wörmer et al. (2013). Briefly, separation was achieved on an Acquity BEH C18 column (Waters, 2.1×150 mm, 1.7 µm) maintained at 30 ∘C, using (a) MeOH ∕ H2O ∕ formic acid ∕ 14.8 M (85 : 15 : 0.12 : 0.04 []) and (b) IPA ∕ MeOH ∕ formic acid ∕ 14.8 M (50 : 50 : 0.12 : 0.04 []) as eluent. The elution program was 95 % A for 3 min, a linear gradient to 40 % A at 12 min, and then to 0 % A at 50 min, which was maintained until 80 min. The flow rate was 0.2 mL min−1. Positive ion electrospray ionisation settings were capillary temperature, 300∘C; sheath gas (N2) pressure, 40 arbitrary units (AU); auxiliary gas (N2) pressure, 10 AU; spray voltage, 4.5 kV; probe heater temperature, 50 ∘C; S-lens 70 V. Target lipids were analysed with a mass range of m∕z 350–2000 (resolution 70 000 ppm at m∕z 200), followed by data-dependent tandem MS2 with parameters as described by Besseling et al. (2018). The combined extracted ion currents (within 3 ppm) of the protonated, ammoniated, and sodiated adducts (m∕z 547.472 + 564.499 + 569.454, respectively) were used to integrate BHT isomer. The relative abundance of peak area does not necessarily reflect the actual relative abundance of the different compounds; however, this method allows for comparison between the samples analysed in this study. BHT and BHT isomer were baseline separated, and the MS2 spectra of BHT and its isomer (Fig. S1) were comparable to spectra of non-derivatised BHT published by Talbot et al. (2016b). MS performance was continuously monitored, and matrix effects were assessed using the PAF standard. Peak areas were corrected accordingly. However, as no commercially available authentic standards were available for non-derivatised BHPs, semi-quantitative BHT isomer abundance is reported as the integrated peak area response (response unit, ru) for the Levantine S5 (64PE406-E1) record. Although quantification in not possible, this method does allow for comparison of BHT isomer abundances between samples as response factors should be identical across the S5 sample set.
2.5.3 Analysis of ladderane fatty acids
Methylated fatty acid fractions were dissolved in acetone; filtered through 0.45 µm, 4 mm diameter PTFE filters; and analysed by high-performance liquid chromatography coupled to positive ion atmospheric pressure chemical ionisation tandem mass spectrometry (HPLC/APCI-MS/MS) in selective reaction monitoring mode to detect the four ladderane fatty acids and two short-chain ladderane fatty acids (Hopmans et al., 2006; modified by Rush et al., 2011). Ladderanes were quantified using external calibration curves of three standards of isolated methylated ladderane fatty acids (C14–[3]–ladderane fatty acid, C20–[3]–ladderane fatty acid, and C20–[5]–ladderane fatty acid) (Hopmans et al., 2006; Rush et al., 2011; Rattray et al., 2008).
To test the hypotheses that (1) anaerobic ammonium oxidation occurred in the water column during Mediterranean sapropel events and (2) BHT isomer could be used as a biomarker for anammox during these events, a suite of Quaternary and Pliocene sapropels were examined.
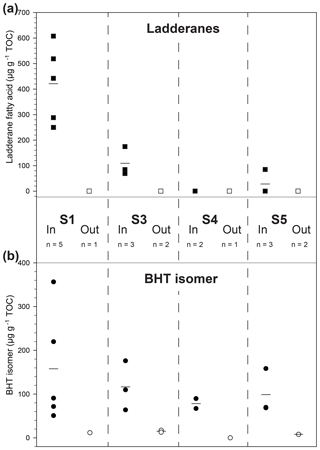
Figure 3Scattered distribution of (a) ladderane fatty acid concentration (squares) and (b) BHT isomer concentration (circles) in four recent sapropels (S1–S5; 7–125 ka) from the Aegean Sea (R/V Marion Dufresne LC21). Filled symbols denote samples taken within a sapropel sediment and open symbols from outside. Lines are the mean markers when data points are not equal.
3.1 Anammox lipids in S1–S5 sapropels from the Aegean Sea
Sapropels spanning four of the most recent five events in the Aegean Sea were sampled from core LC21 from the Aegean Sea and analysed for anammox biomarkers (Fig. 3a). Ladderane fatty acids (i.e. C18–[3]–ladderane fatty acid, and C18–[5]–ladderane fatty acid, C20–[3]–ladderane fatty acid, and C20–[5]–ladderane fatty acid; Fig. 1), the traditional biomarkers for anammox bacteria (Jaeschke et al., 2009; Rush et al., 2012a; Sinninghe Damsté et al., 2002), were found in the most recent sapropel (290–610 ng g−1 TOC; in S1, ∼ 7 ka; Fig. 3a) in abundances comparable to those found in sediments of the Peru Margin and Arabian Sea (Rush et al., 2012a). Conversely, ladderanes were not detected in the sediment sampled directly below this sapropel layer (out S1, Fig. 3a), indicating anammox was an important process during S1 deposition, but likely not before the onset of sapropel deposition. Ladderane concentration progressively decreased with increasing age of the deeper sapropels: 80–170 ng g−1 TOC in S3 (∼ 85 ka); not detected in S4 (∼ 100 ka); and 0–90 ng g−1 TOC in S5 (∼ 125 ka). It is worth noting that two of the three sediments from within S5 did not contain detectable ladderanes. This demonstrates the previously described sensitivity of ladderane lipids to diagenesis (Rush et al., 2012a; Jaeschke et al., 2008) and highlights their potential weakness as a biomarker proxy for past anammox bacteria in ancient sediments. Residues of TLEs were also saponified for ladderane analysis, as these have previously been shown to extend the detection of anammox in older sediments by releasing more matrix-bound ladderanes (Rush et al., 2012a). However, this did not show any difference in the presence of anammox (i.e. there was no detection of ladderanes in residues in which the original TLEs did not contain these biomarkers). The non-detection of ladderanes in most of the S5 samples is particularly surprising as this is the most intense of the Late Quaternary sapropels (Struck et al., 2001), having been described as analogous to the modern-day Black Sea (Menzel et al., 2006). Since anammox is currently present and actively removing N in the cline of a strong redox gradient (redoxcline) of the Black Sea (Jensen et al., 2008; Kuypers et al., 2003), it was expected that anammox behaved similarly in the nitrogen cycle of the eastern Mediterranean during deposition of the S5 sapropel. Given that the oldest detection of ladderanes comes from a slightly older record in the Arabian Sea (Jaeschke et al., 2009), it is unclear why ladderane detection in S5 is sporadic. Perhaps degradation is responsible for the rapid removal of ladderanes from the system during deposition, or the low resolution in the S5 record made these specific sediment depths not ideal targets for anammox activity.
Bacteriohopanetetrol isomer (BHT isomer; Fig. 1) has recently been proposed to be an alternative biomarker for anammox bacteria in paleo-records (Rush et al., 2014b). Our analysis of non-derivatised BHT isomer was based on the previously published method analysing intact polar lipids via reverse phase liquid chromatography (Wörmer et al., 2013) and achieved better separation of BHT isomer from BHT compared to the acetylated LC–MS (liquid chromatography–mass spectrometry) method (Rush et al., 2014b; Fig. S1).The concentration of BHT isomer in the Aegean Sea sapropels showed a similar trend to ladderanes in the shallow sediment layers (Fig. 3b): the concentration was high in S1 (71–360 µg g−1 TOC) and low in the underlying sediment (12 µg g−1 TOC; out S1), in good agreement with the ladderane data. In contrast, however, BHT isomer was detected in all deeper sapropels at higher concentrations (64–180 µg g−1 TOC in S3; 67–90 µg g−1 TOC in S4; and 68–160 µg g−1 TOC in S5) than the ladderanes. Sediments from outside the sapropel had relatively low, but measurable, BHT isomer concentration (8–17 µg g−1 TOC). As BHT isomer was detected in all sapropels, including the oldest S5 sediments, it appears that the rapid removal of ladderanes from the system is due to degradation during deposition. These results clearly demonstrate the utility of BHT isomer as a biomarker for anammox in paleo-records compared to the more labile ladderane lipids. A hemipelagic, light, non-sapropel sediment sampled between S3 and S4 contained neither ladderanes nor BHT isomer (Fig. 3; out S4), indicating a period where anammox was likely not active in the Mediterranean nitrogen cycle. Furthermore, the detection of BHT isomer in the non-sapropel sediments underlying S1 and S5 and overlying S3 shows that this lipid is a better biomarker than ladderanes for recording trace amounts of anammox throughout the history of the Mediterranean system, especially in sediment deposited under oxic (bottom) water conditions.
3.2 High-resolution evidence shows anammox responds to marine redox shifts in S5 sapropel record
To further investigate the occurrence of anammox during sapropel deposition, we analysed in high resolution the well-developed S5 (TOC content up to 12 %; Fig. 4) recovered from the Levantine Basin in the eastern Mediterranean during a cruise of the R/V Pelagia in 2016 (64PE406-E1; Fig. 2). X-ray fluorescence scanning of this core showed no peak in Mn ∕ Ti in the top of the sapropel, indicating this S5 record does not contain the burndown effect of oxygen diffusing downward post-deposition (Dirksen et al., 2019). This was corroborated by the Ba ∕ Ti record, used as a proxy for paleo-productivity, which followed the same trend as organic carbon throughout this sapropel. Thus, it was expected that ladderane fatty acids would be preserved in the high-TOC sediments of this S5 record. However, in line with the earlier results of ladderane analyses for S5 in the Aegean Sea record, the results from the Levantine Basin were inconclusive. Ladderanes were detected in all, except two, of the thirty sapropel samples but were at the detection limit (i.e. peak area of 3× background), preventing interpretation of the ladderane profile in S5. The cause of low ladderane concentration even in sediments with high TOC may be due to unknown degradation in Mediterranean sapropel sediments, and future work should include anoxic degradation experiments with anammox biomass to elucidate potential mechanisms.
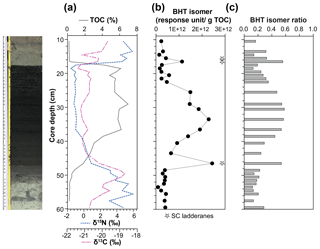
Figure 4(a) Total organic carbon (TOC) content, isotope values of bulk nitrogen (δ15N) and carbon (δ13C), (b) BHT isomer concentration (circles) and presence of short-chain (SC) ladderane fatty acids (stars), and (c) BHT isomer ratio through a high-resolution S5 sapropel record from site 64PE406 (R/V Pelagia) in the Levantine Basin. The sapropel is indicated by the darker sediment. Core photo provided by Rick Hennekam.
The BHT isomer does not appear to have been affected by degradation in the same way as ladderane lipids; it was above detection limit in all S5 sediments (Fig. 4b). The concentration of BHT isomer increased progressively by a factor of 10 from the onset of S5 until the core of the sapropel event (from average pre-sapropel value 2.69×1011 ru g−1 TOC to 2.28×1012 ru g−1 TOC at 33–34 cm core depth; Fig. 4) and then waned until the termination. This indicates that anammox was an important process during the formation of S5, actively removing nitrogen from the marine system. Photic zone euxinia has been observed in cores from the western part of the eastern basin during S5 formation by the identification of isorenieratene (Marino et al., 2007; Rohling et al., 2006). Isorenieratene is a biomarker lipid for the brown strains of the photosynthetic, green sulfur bacteria (Chlorobiaceae). These organisms require the unique conditions of light, albeit at relatively low intensity, and euxinic waters, as they are very sensitive to the presence of molecular oxygen (Overmann et al., 1992). Although anammox bacteria are inhibited by the presence of free sulfide, they likely thrived at the redoxcline during deposition of S5 (Fig. 5a). This is the case, for instance, in the modern Black Sea: at 90 m water depth, where oxygen and sulfide concentrations are both low and nitrite and ammonium are readily available, the presence and activity of anammox have been confirmed via rate measurements and ladderane biomarker observations (Kuypers et al., 2003; Jensen et al., 2008).
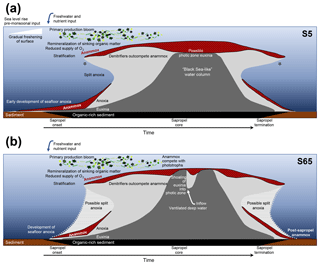
Figure 5Hypothesised temporal evolution of anammox in the Levantine Basin water column during sapropel formations. (a) Scenario of S5; (b) scenario of Pliocene S65. Depth not to scale. Proposed niches for anammox bacteria are shaded in dotted red. Light grey area represents water column anoxia; dark grey is euxinia. Stars denote periods when short-chain ladderanes were formed by β oxidation in the oxic water column. Figure should be used as a guide for the text.
There are two considerable peaks in BHT isomer that fall outside of the S5 trend (Fig. 4b), occurring at the onset (2.43×1012 ru g−1 TOC; 46–47 cm core depth) and termination (1.12×1012 ru g−1 TOC; 16–17 cm core depth) of the sapropel. Sea level rise and gradual freshening of the Mediterranean are believed to have caused a stepwise removal of oxygen and subsequent slow build-up of anoxia ca. 3 kyr before the (massive) freshwater discharge from the African continent instigated the real onset of S5 (Schmiedl et al., 2003). The intense anammox peak pre-sapropel formation could be a response to this marine redox shift (Fig. 5a). Anammox would have thrived, consuming the residual low levels of ammonium and nitrite in an anoxic Mediterranean water column. Then, once monsoonal discharge brought in the initial pulse of nutrients from the Nile, the slow-growing anammox bacterial population would have been rapidly outcompeted by heterotrophic denitrifiers consuming sinking organic carbon being produced in the overlying oxic waters. As S5 progressed and N supply became scarcer, anammox would have repopulated the niche of redoxcline N remover at core sapropel conditions. The peak of BHT isomer observed at S5 termination (Fig. 4) shows that the conditions were again favourable for anammox to thrive. However, this may have occurred at the anoxic sediment–water interface rather than in the water column, where low concentrations of nitrite and ammonium could have persisted from the degradation of organic matter settling on the seafloor after the reoxidation of the water column. The BHT isomer ratio (BHT isomer/total BHT; Sáenz et al., 2011) normalises the contribution of the anammox biomarker to other potential sources of BHT. The ratio in the S5 record (Fig. 4c) showed the same trend as BHT isomer concentration in the sapropel (e.g. the ratio was highest during the core sapropel, 0.58 at 30–32 cm, and showed distinct peaks at its onset and termination). The slight decrease in BHT isomer ratio before and after the sapropel event is likely due to an increased production of BHT by other bacterial sources rather than a change in the BHT isomer producer.
Short-chain (SC) ladderane fatty acids (i.e. C14–[3]–ladderane fatty acid and C14–[5]–ladderane fatty acid; Fig. 1) are oxic biodegradation products of ladderane fatty acids (Rush et al., 2011) and are used to infer exposure of ladderane lipids to oxic conditions either pre- or post-deposition. SC ladderane fatty acids were only detected in three of the S5 sediments (Fig. 4b), specifically at sapropel onset (46–47 cm core depth) and termination (15–16 and 16–17 cm core depth). This implies that, during sapropel maximum, anammox was thriving at the Mediterranean redoxcline. Anammox detritus would then have sunk through an anoxic (euxinic) Black Sea-type water column, unexposed to oxygen and the effects of β oxidation that produces SC ladderane fatty acids (Rush et al., 2011). This has been seen in the modern Cariaco Basin, where ladderanes are observed, but SC ladderanes are absent (Rush et al., 2012a). The presence of SC ladderanes at the onset and termination, yet absence in the core S5 record, could also corroborate the concept of “split-anoxia” (as proposed for S1 by Bianchi et al., 2006), which hypothesises for the first 100 to 1000+ years of sapropel formation euxinia was present as a mid-depth oxygen minimum zone rather than a continuation from the seafloor. During these periods where the water column was not fully euxinic, ladderanes would have been oxidised to SC ladderanes in the underlying waters, which would have contained a certain amount of available oxygen. Alternatively, as productivity waned, sedimentation rates would have decreased in the Levantine Basin. Lower sedimentation rates at the onset and termination of S5 would suggest a longer residence time of ladderanes in sediment that would periodically be exposed to (sub)oxic bottom water conditions. Oxic water inflow of pore waters would have stimulated the β oxidation responsible for SC ladderane formation (Rush et al., 2011). It is worth noting that, in the low-resolution Aegean Sea sample set (LC21), all samples from S1 and S3 that contained ladderanes also contained a high concentration of SC-ladderane fatty acids, whereas the singular S5 sediment did not contain SC ladderanes. This would appear to indicate that the Aegean water column during S1 and S3 deposition was not fully euxinic and that S5 in the Aegean mirrored the euxinic Levantine Basin.
Nitrogen isotope ratio (δ15N) values of bulk nitrogen in S5 sediment show a strong shift towards low values within the sapropel (Fig. 4a), a feature seen in most sapropels (Calvert et al., 1992; Sachs and Repeta, 1999; Struck et al., 2001; Higgins et al., 2010; Möbius et al., 2010). This could potentially be explained by either enhanced diazotrophic N2 fixation because N was limited in the system (Möbius et al., 2010) or the preferential uptake and burial of 14N when nitrate is present in excess and primary producers have the opportunity to fractionate maximally (Calvert et al., 1992). As a biomarker for N removal from the system was not available, previous work has only been able to approach this conundrum with evidence for N fixation processes. Using isotopic evidence of diazotrophic phytoplankton, Sachs and Repeta (1999) and Higgins et al. (2010) argue that Mediterranean surface water was nitrogen-limited during sapropel events. Here, for the first time, we present evidence of N loss in a Mediterranean sapropel using BHT isomer as an anammox biomarker. The fact that BHT isomer concentration increases towards the core of S5 appears to suggest that N species were not limited and rather that freshwater run-off could be resupplying these nutrients to microorganisms in the water column and enhancing the pool of N. However, anammox thrive at the redoxclines of modern oxygen minimum zones (Pitcher et al., 2011; Rush et al., 2012b) and euxinic basins (Wakeham et al., 2012; Kuypers et al., 2003), where pulses of fresh N species do not necessarily reach. At the S5 Black Sea-type redoxcline, anammox did not need a riverine supply of N but could have instead been sustained by the advection of N from deeper waters (Rohling et al., 2006) or by N remineralised from the sinking pool of (diazotrophic) organic matter from above. Thus, BHT isomer results show that N removal by anammox was at its highest flux during core S5 sapropel conditions and that the anammox process appears to play an integral role in N cycling during sapropel events.
3.3 Anammox distribution varies between sapropel formations: evidence from a Pliocene sapropel event
To confirm that anaerobic ammonium oxidation has occurred throughout the history of anoxia in the Mediterranean Basin, not only in the most recent Quaternary sapropels, BHT isomer concentration was analysed across a high-resolution Pliocene sapropel (ODP Leg 160, Site 967; Fig. 2). The Ba record of this sapropel shows the same trend with depth as TOC, indicating no significant burndown of organic matter after its deposition (Grant et al., 2017). BHT isomer is present throughout this older record (Fig. 6b), and as the BHT isomer ratio (BHT isomer ∕ total BHT) is consistently elevated (average 0.48; Fig. 6c), anammox is the likely source in the entirety of the record. Much like the trend seen in the S5 Levantine sapropel, sapropel S65 showed two distinct peaks in BHT isomer concentration at its onset (110–240 µg g−1 TOC; 69–73 cm core depth) and termination (640– 1100 µg g−1 TOC; 54–59 cm core depth). However, BHT isomer concentration displayed a distribution different to that of the S5 record during the core Pliocene sapropel event (Fig. 6b). BHT isomer concentration was low, likely representing unfavourable conditions for anammox during this sapropel. Isorenieratene has been detected in the Pliocene record of Site 967, albeit in a different sapropel event (Menzel et al., 2002). It is possible that euxinia shoaled further into the photic zone during this Pliocene sapropel, forcing anammox at the redoxcline to compete for N with phytoplankton (Fig. 5b). Anammox would have therefore only thrived during the build-up and termination periods when photic zone euxinia would have been deeper/less intense. Nevertheless, this hypothesis should be confirmed through future analysis of photic zone euxinia biomarkers (e.g. isorenieratene). There was a spike in BHT isomer concentration mid-sapropel that coincided shortly after with a decrease in TOC (65–67 cm core depth; Fig. 6a). Mid-sapropel breaks have been reported elsewhere, as repopulation events of benthic fauna (e.g. Rohling et al., 1993), and could be due to inflow of freshly ventilated deep water. Re-ventilation would have directly stimulated anammox bacteria that were inhibited by euxinia, whereas there may have been a slight delay on the effect of decreasing TOC (Fig. 5b). The concentration of BHT isomer was still high after sapropel deposition (∼ 250 µg g−1 TOC; < 40 cm core depth), relative to that pre-sapropel. This may indicate that the anammox process remained an important N process in the Mediterranean after bottom water anoxia waned.
Combined, the high-resolution results from the S5 and Pliocene sapropels indicate that the functioning of anammox is not always the same during periods of Mediterranean anoxia. This demonstrates that the response of the N cycle to anoxic conditions can vary drastically from one sapropel event to the next.
BHT isomer, a lipid synthesised by marine anaerobic ammonium-oxidising (anammox) bacteria, was detected at high concentration in all Mediterranean sapropel sediments. This study highlights the potential of BHT isomer as a biomarker for anammox during past periods of basin-wide anoxia. It is also apparent that the response of anammox to shifts in redox conditions during anoxia is not consistent between sapropel events. The anammox peak in S5 occurred during core sapropel conditions, whereas anammox responded in an opposite trend in the Pliocene sapropel record.
Investigating the variability of anammox in these sapropel events may enhance our understanding of N cycling during other periods of intense organic matter deposition in the past. Sapropel features have been found in the sediment records of different marginal seas (e.g. Japan Sea, Red Sea; Emeis et al., 1996). The restricted paleogeography during oceanic anoxic events is also thought to have contributed to the propagation of anoxia in the Cretaceous and Jurassic. BHT isomer can possibly be used to explore the role anammox may have played in these basin anoxic events. The residence time of BHT isomer in marine sediment records likely does not extend beyond the Early Cretaceous (van Dongen et al., 2006; Talbot et al., 2016a). However, BHT isomer can be applied to the Paleocene–Eocene Thermal Maximum (PETM; 55 Ma). Thermally stable lipid products of anammox biomass (Rush et al., 2014a) could serve as alternative biomarkers for anammox in more mature sediments from the Cretaceous and Jurassic. Furthermore, investigating the compound-specific isotope values of BHT isomer in a marine sample set will strengthen the use of BHT isomer as a biomarker for anammox.
These data have been deposited with the open-access library PANGAEA and can be accessed at https://doi.org/10.1594/PANGAEA.898591 (Rush et al., 2019).
The supplement related to this article is available online at: https://doi.org/10.5194/bg-16-2467-2019-supplement.
DR designed the project; BD and DR performed the measurements; and DR, HMT, MTJvM, and JSSD contributed to the analysis of the results and the writing of the manuscript.
The authors declare that they have no conflict of interest.
Guy Rothwell is thanked for his help collecting LC21 samples from the BOSCORF repository. We thank the Captain and crew of the R/V Pelagia for the collection of the sapropel S5 (cruise 64PE406). Pieter Dirksen subsampled the S5 record. Denise van der Slikke-Dorhout and Çağlar Yildiz are acknowledged for extracting the S5 record. We are grateful to the Ocean Drilling Program (ODP) for the samples used in this study as well as to the ODP Core Repository (Bremen, Germany) where Luke Handley and Thomas Wagner were involved in the collection of ODP Leg 160 sapropel sequences. Eelco Rohling is thanked for initial discussion about sapropel sampling, Rick Hennekam is thanked for fruitful discussions of XRF data in 64PE406-E1, and Lucas Lourens is thanked for Pliocene sapropel discussions. Michel Rohmer is thanked for gifting the original BHT standard to Helen Talbot at Newcastle.
This work was supported by the Natural Environment Research Council (NERC) project ANAMMARKS (NE/N011112/1) awarded to Darci Rush. This work was also supported by funding from the Netherlands Earth System Science Center (NESSC) through a gravitation grant (NWO 024.002.001) from the Dutch Ministry for Education, Culture and Science to Jaap S. Sinninghe Damsté. NERC (grant number NE/E017088/1) and the European Research Council (ERC) (Starting Grant no. 258734 awarded to Helen M. Talbot for project AMOPROX) are gratefully acknowledged for partially funding this research.
This paper was edited by S. Wajih A. Naqvi and reviewed by Cecile Blanchet and one anonymous referee.
Besseling, M. A., Hopmans, E. C., Boschman, R. C., Sinninghe Damsté, J. S., and Villanueva, L.: Benthic archaea as potential sources of tetraether membrane lipids in sediments across an oxygen minimum zone, Biogeosciences, 15, 4047–4064, https://doi.org/10.5194/bg-15-4047-2018, 2018.
Bianchi, D., Zavatarelli, M., Pinardi, N., Capozzi, R., Capotondi, L., Corselli, C., and Masina, S.: Simulations of ecosystem response during the sapropel S1 deposition event, Palaeogeogr. Palaeocl., 235, 265–287, 2006.
Bligh, E. G. and Dyer, W. J.: A rapid method of total lipid extraction and purification, Can. J. Biochem. Phys., 37, 911–917, 1959.
Calvert, S. E., Nielsen, B., and Fontugne, M. R.: Evidence from nitrogen isotope ratios for enhanced productivity during formation of eastern Mediterranean sapropels, Nature, 359, 223–225, 1992.
Cooke, M. P., Talbot, H. M., and Farrimond, P.: Bacterial populations recorded in bacteriohopanepolyol distributions in soils from Northern England, Org. Geochem., 39, 1347–1358, 2008.
Cramp, A. and O'Sullivan, G.: Neogene sapropels in the Mediterranean: a review, Mar. Geol., 153, 11–28, 1999.
Dirksen, J. P., Hennekam, R., Geerken, E., and Reichart, G.-J.: A novel approach using time-depth distortions to assess multicentennial variability in deep-sea oxygen deficiency in the Eastern Mediterranean Sea during sapropel S5, Palaeogeogr. Palaeocl., https://doi.org/10.1029/2018PA003458, online first, 2019.
Emeis, K.-C. and Party, S. S.: Paleoceanography and sapropel introduction, in: Proceedings of the Ocean Drilling Program, Initial Reports, 160th edn., edited by: Robertson, A. H. F., and Richter, C., 21–28, 1996.
Grant, K. M., Rohling, E. J., Westerhold, T., Zabel, M., Heslop, D., Konijnendijk, T., and Lourens, L.: A 3 million year index for North African humidity/aridity and the implication of potential pan-African Humid periods, Quaternary Sci. Rev. 171, 100–118, https://doi.org/10.1016/j.quascirev.2017.07.005, 2017.
Hamersley, M. R., Lavik, G., Woebken, D., Rattray, J. E., Lam, P., Hopmans, E. C., Sinninghe Damsté, J. S., Krüger, S., Graco, M., Gutiérrez, D., and Kuypers, M. M. M.: Anaerobic ammonium oxidation in the Peruvian oxygen minimum zone, Limnol. Oceanogr., 52, 923–933, 2007.
Hemingway, J. D., Kusch, S., Walter, S. R. S., Polik, C. A., Elling, F. J., and Pearson, A.: A novel method to measure the C-13 composition of intact bacteriohopanepolyols, Org. Geochem., 123, 144–147, https://doi.org/10.1016/j.orggeochem.2018.07.002, 2018.
Higgins, M. B., Robinson, R. S., Carter, S. J., and Pearson, A.: Evidence from chlorin nitrogen isotopes for alternating nutrient regimes in the Eastern Mediterranean Sea, Earth Planet. Sc. Lett., 290, 102–107, 2010.
Hopmans, E. C., Kienhuis, M. V. M., Rattray, J. E., Jaeschke, A., Schouten, S., and Sinninghe Damsté, J. S.: Improved analysis of ladderane lipids in biomass and sediments using high-performance liquid chromatography/atmospheric pressure chemical ionization tandem mass spectrometry, Rapid Commun. Mass Sp., 20, 2099–2103, 2006.
Jaeschke, A., Lewan, M. D., Hopmans, E. C., Schouten, S., and Sinninghe Damsté, J. S.: Thermal stability of ladderane lipids as determined by hydrous pyrolysis, Org. Geochem., 39, 1735–1741, 2008.
Jaeschke, A., Ziegler, M., Hopmans, E. C., Reichart, G.-J., Lourens, L. J., Schouten, S., and Sinninghe Damsté, J. S.: Molecular fossil evidence for anaerobic ammonium oxidation in the Arabian Sea over the last glacial cycle, Paleoceanography, 24, PA2202, https://doi.org/10.1029/2008PA001712, 2009.
Jensen, M. M., Kuypers, M. M. M., Lavik, G., and Thamdrup, B.: Rates and regulation of anaerobic ammonium oxidation and denitrification in the Black Sea, Limnol. Oceanogr., 53, 23–36, https://doi.org/10.4319/lo.2008.53.1.0023, 2008.
Kuypers, M. M. M., Sliekers, A. O., Lavik, G., Schmid, M., Jørgensen, B. B., Kuenen, J. G., Sinninghe Damsté, J. S., Strous, M., and Jetten, M. S. M.: Anaerobic ammonium oxidation by anammox bacteria in the Black Sea, Nature, 422, 608–611, 2003.
Kuypers, M. M. M., van Breugel, Y., Schouten, S., Erba, E., and Sinninghe Damsté, J. S.: N2-fixing cyanobacteria supplied nutrient N for Cretaceous oceanic anoxic events, Geology, 32, 853–856, 2004.
Marino, G., Rohling, E. J., Rijpstra, W. I. C., Sangiorgi, F., Schouten, S., and Sinninghe Damsté, J. S.: Aegean Sea as driver of hydrographic and ecological changes in the eastern Mediterranean, Geology, 35, 675–678, 2007.
Matys, E. D., Sepulveda, J., Pantoja, S., Lange, C. B., Caniupan, M., Lamy, F., and Summons, R. E.: Bacteriohopanepolyols along redox gradients in the Humboldt Current System off northern Chile, Geobiology, 15, 844–857, https://doi.org/10.1111/gbi.12250, 2017.
Menzel, D., Hopmans, E. C., van Bergen, P. F., de Leeuw, J. W., and Sinninghe Damsté, J. S.: Development of photic zone euxinia in the eastern Mediterranean Basin during deposition of Pliocene sapropels, Mar. Geol., 189, 215–226, 2002.
Menzel, D., Hopmans, E. C., Schouten, S., and Sinninghe Damsté, J. S.: Membrane tetraether lipids of planktonic Crenarchaeota in Pliocene sapropels of the eastern Mediterranean Sea, Palaeogeogr. Palaeocl., 239, 1–15, 2006.
Möbius, J., Lahajnar, N., and Emeis, K.-C.: Diagenetic control of nitrogen isotope ratios in Holocene sapropels and recent sediments from the Eastern Mediterranean Sea, Biogeosciences, 7, 3901–3914, https://doi.org/10.5194/bg-7-3901-2010, 2010.
Nijenhuis, I. A. and de Lange, G. J.: Geochemical constraints on Pliocene sapropel formation in the eastern Mediterranean, Mar. Geol., 163, 41–63, 2000.
Overmann, J., Cypionka, H., and Pfennig, N.: An extremely low-light-adapted phototrophic sulfur bacterium from the Black Sea, Limnol. Oceanogr., 37, 150–155, 1992.
Peiseler, B. and Rohmer, M.: Prokaryotic Triterpenoids of the Hopane Series – Bacteriohopanetetrols of New Side-Chain Configuration from Acetobacter Species, J. Chem. Res., 9, 298–299, 1992.
Pitcher, A., Villanueva, L., Hopmans, E. C., Schouten, S., and Sinninghe Damsté, J. S.: Niche segregation of ammonia-oxidizing archaea and anammox bacteria in the Arabian Sea oxygen minimum zone, ISME Journal, 5, 1896–1904, 2011.
Rattray, J. E., van de Vossenberg, J., Hopmans, E. C., Kartal, B., van Niftrik, L., Rijpstra, W. I. C., Strous, M., Jetten, M. S. M., Schouten, S., and Sinninghe Damsté, J. S.: Ladderane lipid distribution in four genera of anammox bacteria, Arch. Microbiol., 190, 51–66, 2008.
Rohling, E. J., Destigter, H. C., Vergnaudgrazzini, C., and Zaalberg, R.: Temporary repopulation by low-oxygen tolerant benthic foraminifera within an Upper Pliocene sapropel: Evidence for the role of oxygen depletion in the formation of sapropels, Mar. Micropaleontol., 22, 207–219, https://doi.org/10.1016/0377-8398(93)90044-x, 1993.
Rohling, E. J., Hopmans, E. C., and Sinninghe Damsté, J. S.: Water column dynamics during the last interglacial anoxic event in the Mediterranean (sapropel S5), Paleoceanography, 21, PA2018, https://doi.org/10.1029/2005PA001237, 2006.
Rohling, E. J., Marino, G., and Grant, K. M.: Mediterranean climate and oceanography, and the periodic development of anoxic events (sapropels), Earth-Sci. Rev., 143, 62–97, 2015.
Rosa-Putra, S., Nalin, R., Domenach, A. M., and Rohmer, M.: Novel hopanoids from Frankia spp. and related soil bacteria – Squalene cyclization and significance of geological biomarkers revisited, Eur. J. Biochem., 268, 4300–4306, 2001.
Rossignol-Strick, M.: African monsoons, an immediate climate response to orbital insolation, Nature, 304, 46–49, 1983.
Rush, D., Jaeschke, A., Hopmans, E. C., Geenevasen, J. A. J., Schouten, S., and Sinninghe Damsté, J. S.: Short chain ladderanes: Oxic biodegradation products of anammox lipids, Geochim. Cosmochim. Ac., 75, 1662–1671, 2011.
Rush, D., Hopmans, E. C., Wakeham, S. G., Schouten, S., and Sinninghe Damsté, J. S.: Occurrence and distribution of ladderane oxidation products in different oceanic regimes, Biogeosciences, 9, 2407–2418, https://doi.org/10.5194/bg-9-2407-2012, 2012a.
Rush, D., Wakeham, S. G., Hopmans, E. C., Schouten, S., and Sinninghe Damsté, J. S.: Biomarker evidence for anammox in the oxygen minimum zone of the Eastern Tropical North Pacific, Org. Geochem., 53, 80–87, 2012b.
Rush, D., Jaeschke, A., Geenevasen, J. A., Tegelaar, E., Pureveen, J., Lewan, M. D., Schouten, S., and Sinninghe Damsté, J. S.: Generation of unusual branched long chain alkanes from hydrous pyrolysis of anammox bacterial biomass, Org. Geochem., 76, 136–145, 2014a.
Rush, D., Sinninghe Damsté, J. S., Poulton, S. W., Thamdrup, B., Garside, A., Gonzalez, J. A., Schouten, S., Jetten, M. S., and Talbot, H. M.: Anaerobic ammonium-oxidising bacteria: A biological source of the bacteriohopanetetrol stereoisomer in marine sediments, Geochim. Cosmochim. Ac., 140, 50–64, 2014b.
Rush, D., Talbot, H. M., van der Meer, M. T. J., Hopmans, E. C., Douglas, B., Sinninghe D., Jaap, S.: Biomarker in the eastern Mediterranean Sea during Quaternary and Pliocene sapropel formation, PANGAEA, https://doi.org/10.1594/PANGAEA.898591, 2019.
Sachs, J. P. and Repeta, D. J.: Oligotrophy and nitrogen fixation during eastern Mediterranean sapropel events, Science, 286, 2485–2488, 1999.
Sáenz, J. P, Wakeham, S. G., Eglinton, T. I., Summons, R. E.: New constraints on the provenance of hopanoids in the marine geologic record: Bacteriohopanepolyols in marine suboxic and anoxic environments, Org. Geochem., 42, 1351–1362, 2011.
Schmiedl, G., Mitschele, A., Beck, S., Emeis, K.-C., Hemleben, C., Schulz, H., Sperling, M., and Weldeab, S.: Benthic foraminiferal record of ecosystem variability in the eastern Mediterranean Sea during times of sapropel S5 and S6 deposition, Palaeogeogr. Palaeocl., 190, 139–164, 2003.
Schouten, S., Strous, M., Kuypers, M. M. M., Rijpstra, W. I. C., Baas, M., Schubert, C. J., Jetten, M. S. M., and Sinninghe Damsté, J. S.: Stable carbon isotopic fractionations associated with inorganic carbon fixation by anaerobic ammonium-oxidizing bacteria, Appl. Environ. Microbiol., 70, 3785–3788, 2004.
Sinninghe Damsté, J. S. and Hopmans, E. C.: Does fossil pigment and DNA data from Mediterranean sediments invalidate the use of green sulfur bacterial pigments and their diagenetic derivatives as proxies for the assessment of past photic zone euxinia?, Environ. Microbiol., 10, 1392–1399, 2008.
Sinninghe Damsté, J. S., Strous, M., Rijpstra, W. I. C., Hopmans, E. C., Geenevasen, J. A. J., van Duin, A. C. T., van Niftrik, L. A., and Jetten, M. S. M.: Linearly concatenated cyclobutane lipids form a dense bacterial membrane, Nature, 419, 708–712, 2002.
Slomp, C. P., Thomson, J., and de Lange, G. J.: Enhanced regeneration of phosphorus during formation of the most recent eastern Mediterranean sapropel (S1), Geochim. Cosmochim. Ac., 66, 1171–1184, 2002.
Slomp, C. P., Thomson, J., and de Lange, G. J.: Controls on phosphorus regeneration and burial during formation of eastern Mediterranean sapropels, Mar. Geol., 203, 141–159, 2004.
Strous, M., Fuerst, J. A., Kramer, E. H. M., Logemann, S., Muyzer, G., van de Pas-Schoonen, K. T., Webb, R., Kuenen, J. G., and Jetten, M. S. M.: Missing lithotroph identified as new planctomycete, Nature, 400, 446–449, 1999.
Strous, M., Pelletier, E., Mangenot, S., Rattei, T., Lehner, A., Taylor, M. W., Horn, M., Daims, H., Bartol-Mavel, D., Wincker, P., Barbe, V., Fonknechten, N., Vallenet, D., Segurens, B., Schenowitz-Truong, C., Médigue, C., Collingro, A., Snel, B., Dutilh, B. E., Op den Camp, H. J. M., van der Drift, C., Cirpus, I., van de Pas-Schoonen, K. T., Harhangi, H. R., van Niftrik, L., Schmid, M., Keltjens, J., van de Vossenberg, J., Kartal, B., Meier, H., Frishman, D., Huynen, M. A., Mewes, H.-W., Weissenbach, J., Jetten, M. S. M., Wagner, M., and le Paslier, D.: Deciphering the evolution and metabolism of an anammox bacterium from a community genome, Nature, 440, 790–794, 2006.
Struck, U., Emeis, K. C., Voss, M., Krom, M. D., and Rau, G. H.: Biological productivity during sapropel S5 formation in the Eastern Mediterranean Sea: Evidence from stable isotopes of nitrogen and carbon, Geochim. Cosmochim. Ac., 65, 3249–3266, 2001.
Talbot, H. M., Rohmer, M., and Farrimond, P.: Rapid structural elucidation of composite bacterial hopanoids by atmospheric pressure chemical ionisation liquid chromatography/ion trap mass spectrometry, Rapid Commun. Mass Sp., 21, 880–892, 2007.
Talbot, H. M., McClymont, E. L., Inglis, G. N., Evershed, R. P., and Pancost, R. D.: Origin and preservation of bacteriohopanepolyol signatures in Sphagnum peat from Bissendorfer Moor (Germany), Org. Geochem., 97, 95–110, https://doi.org/10.1016/j.orggeochem.2016.04.011, 2016a.
Talbot, H. M., Sidgwick, F. R., Bischoff, J., Osborne, K. A., Rush, D., Sherry, A., and Spencer-Jones, C. L.: Analysis of non-derivatised bacteriohopanepolyols by ultrahigh-performance liquid chromatography/tandem mass spectrometry, Rapid Commun. Mass Sp., 30, 2087–2098, https://doi.org/10.1002/rcm.7696, 2016b.
van Dongen, B. E., Talbot, H. M., Schouten, S., Pearson, P. N., and Pancost, R. D.: Well preserved Palaeogene and Cretaceous biomarkers from the Kilwa area, Tanzania, Org. Geochem., 37, 539–557, 2006.
van Winden, J. F., Talbot, H. M., Kip, N., Reichart, G.-J., Pol, A., McNamara, N. P., Jetten, M. S. M., Op den Camp, H. J. M., and Sinninghe Damsté, J. S.: Bacteriohopanepolyol signatures as markers for methanotrophic bacteria in peat moss, Geochim. Cosmochim. Ac., 77, 52–61, 2012.
Wakeham, S. G., Turich, C., Schubotz, F., Podlaska, A., Li, X. N., Varela, R., Astor, Y., Sáenz, J. P., Rush, D., Sinninghe Damsté, J. S., Summons, R. E., Scranton, M. I., Taylor, G. T., and Hinrichs, K.-U.: Biomarkers, chemistry and microbiology show chemoautotrophy in a multilayer chemocline in the Cariaco Basin, Deep-Sea Res. Pt. I, 63, 133–156, 2012.
Ward, B. B.: How Nitrogen Is Lost, Science, 341, 352–353, https://doi.org/10.1126/science.1240314, 2013.
Wörmer, L., Lipp, J. S., Schroder, J. M., and Hinrichs, K. U.: Application of two new LC-ESI-MS methods for improved detection of intact polar lipids (IPLs) in environmental samples, Org. Geochemistry, 59, 10–21, https://doi.org/10.1016/j.orggeochem.2013.03.004, 2013.