the Creative Commons Attribution 4.0 License.
the Creative Commons Attribution 4.0 License.
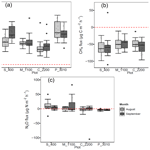
Ideas and perspectives: patterns of soil CO2, CH4, and N2O fluxes along an altitudinal gradient – a pilot study from an Ecuadorian neotropical montane forest
Paula Alejandra Lamprea Pineda
Marijn Bauters
Hans Verbeeck
Selene Baez
Matti Barthel
Samuel Bodé
Pascal Boeckx
Tropical forest soils are an important source and sink of greenhouse gases (GHGs), with tropical montane forests, in particular, having been poorly studied. The understanding of this ecosystem function is of vital importance for future climate change research. In this study, we explored soil fluxes of carbon dioxide (CO2), methane (CH4), and nitrous oxide (N2O) in four tropical forest sites located on the western flanks of the Andes in northern Ecuador. The measurements were carried out during the dry season from August to September 2018 and along an altitudinal gradient from 400 to 3010 m a.s.l. (above sea level). During this short-term campaign, our measurements showed (1) an unusual but marked increase in CO2 emissions at high altitude, possibly linked to changes in soil pH and/or root biomass, (2) a consistent atmospheric CH4 sink over all altitudes with high temporal and spatial variability, and (3) a transition from a net N2O source to sink along the altitudinal gradient. Our results provide arguments and insights for future and more detailed studies on tropical montane forests. Furthermore, they stress the relevance of using altitudinal transects as a biogeochemical open-air laboratory with a steep in situ environmental gradient over a limited spatial distance. Although short-term studies of temporal variations can improve our understanding of the mechanisms behind the production and consumption of soil GHGs, the inclusion of more rigorous sampling for forest management events, forest rotation cycles, soil type, hydrological conditions and drainage status, ground vegetation composition and cover, soil microclimate, and temporal (seasonality) and spatial (topographic positions) variability is needed in order to obtain more reliable estimates of the CO2, CH4, and N2O source/sink strength of tropical montane forests.
- Article
(417 KB) - Full-text XML
-
Supplement
(428 KB) - BibTeX
- EndNote
Soils play a vital role in the global greenhouse gas (GHG) budget. Tropical forest soils, in particular, represent a net sink of carbon (C) (Pan et al., 2011), but at the same time, they are the largest natural source of N2O, with an estimated contribution of 14 %–23 % to the annual global N2O budget (Werner et al., 2007). In general, soil CO2 is produced mainly by root respiration, microbial respiration, litter decomposition, and the oxidation of soil organic matter (Dalal and Allen, 2008). CH4 is consumed by methanotrophic bacteria (Jang et al., 2006); however, forest soils prone to inundation emit CH4 by methanogenic microorganisms (Archaea domain). N2O is emitted through denitrification or a number of alternative pathways (e.g., nitrification, nitrifier denitrification, chemodenitrification, etc.; Butterbach-Bahl et al., 2013; van Cleemput, 1998; Clough et al., 2017) but can also be consumed during complete denitrification (Butterbach-Bahl et al., 2013). Overall, tropical forest soils emit on average 12.1 t CO2-C ha−1 yr−1 (heterotrophic and autotrophic respiration), slightly less than the net primary productivity (NPP) (12.5 t CO2-C ha−1 yr−1); i.e., the net C sink (belowground and aboveground) of tropical forests is ∼ 0.4 t CO2-C ha−1 yr−1 (Dalal and Allen, 2008; Grace et al., 2006). Under aerobic conditions, CH4 fluxes vary from −0.7 to −30.0 kg CH4-C ha−1 yr−1, with an average consumption of −3.0 kg CH4-C ha−1 yr−1, while the mean rate of N2O emissions from tropical forest soils is 3.03 ± 0.52 kg N2O-N ha−1 yr−1 (Dalal and Allen, 2008), i.e., 2–3 times higher than the mean N2O emissions from temperate forest soils (1.0 ± 0.36 kg N2O-N ha−1 yr−1; Chapui-Lardy et al., 2007; Van Groenigen et al., 2015).
The understanding of the mechanisms and processes underlying GHG flux variability has greatly improved over the last decades (Butterbach-Bahl et al., 2013; Heil et al., 2016; Müller et al., 2015; Sousa Neto et al., 2011; Su et al., 2019; Teh et al., 2014). However, there is still (1) considerable uncertainty about the overall balances of many ecosystems (Castaldi et al., 2013; Heil et al., 2014; Kim et al., 2016b; Pan et al., 2011; Purbopuspito et al., 2006), (2) a strong imbalance in field observations, skewed towards the Northern Hemisphere (Jones et al., 2016; Montzka et al., 2011), and (3) a bias towards the quantification of emissions in lowland forests within the tropics (Müller et al., 2015; Purbopuspito et al., 2006; Wolf et al., 2011). For instance, based on a compilation made of CO2, CH4, and N2O fluxes in South America (Table S1) from 1983 to 2019, there have only been six studies carried out on tropical montane forests (i.e., > 2000 m a.s.l., above sea level), while they represent more than 11 % of the world's tropical forests (Müller et al., 2015; Teh et al., 2014). In fact, Teh et al. (2014) and Spahni et al. (2011) have argued that tropical upland soils are one potentially important source of CH4 and N2O that has been overlooked in both bottom-up and top-down emission inventories; their sink/source strength might be comparable to or greater than their lowland counterparts and, therefore, quantitatively important in regional and global GHG budgets.
To further improve our understanding of the role of tropical forest ecosystems in the global GHG balance, environmental gradients (altitudinal, latitudinal, etc.) can offer great opportunities to study the influence of abiotic factors on biogeochemical processes under field conditions (Bauters et al., 2017; Jobbágy and Jackson, 2000; Kahmen et al., 2011; Laughlin and Abella, 2007), which complements the knowledge on short-term responses from experimental approaches. In the case of altitudinal gradients, these responses are driven by abiotic variables that covary with elevation, which, amongst others, creates a distinctly strong climate gradient over a short spatial distance (Bubb et al., 2004; Killeen et al., 2007; Körner, 2007; Myers et al., 2000).
Moreover, since altitudinal gradients reflect long-term adaptations based on a broad range of factors, they provide valuable insights into the influence that climate change may have on ecosystem processes (Malhi et al., 2010). There is indeed a growing concern regarding the sensitivity of tropical forests to climate change mainly because species in the tropics have evolved with narrow thermal tolerances compared to their temperate counterparts; this makes them particularly vulnerable to changes in global climate (Fadrique et al., 2018; Perez et al., 2016). Therefore, the effects of global warming are expected to be severe in the tropics, and the understanding and integration of the magnitude of their feedbacks in the Earth system are important to come up with appropriate forest management options to mitigate climate change (Bonan, 2008; Li et al., 2020).
To address these knowledge gaps, we present a pilot study of the soil–atmosphere exchange of CO2, CH4, and N2O along an altitudinal gradient in a neotropical montane forest located on the western flanks of the Andes in northern Ecuador. The sampling campaign took place from 6 August to 28 September 2018. Four study sites (Fig. S1) were selected: Río Silanche at 400 m a.s.l (hereinafter: S_400), Milpe at 1100 m a.s.l. (hereinafter: M_1100), El Cedral at 2200 m a.s.l. (hereinafter: C_2200), and Peribuela at 3010 m a.s.l. (hereinafter: P_3010). Gas samples were taken using a static flux chamber method once per day per stratum over 2 weeks. Samples of soil were collected once during the whole field campaign for the analysis of bulk density (ρb), pH, nitrate (NO) and ammonium (NH) content, C and nitrogen (N) concentrations, stable N isotope signatures (δ15N), and soil texture. Additionally, soil moisture (expressed as water-filled pore space, WFPS) and soil temperature were measured daily. Specifically, we aimed to determine the magnitude of the soil–atmosphere exchange of CO2, CH4, and N2O during the dry season. By working along this altitudinal gradient, we wanted to explore the potential effect of altitude on the GHG fluxes of the forest soils. Findings from this research could provide insights for future and more detailed studies on tropical montane forests.
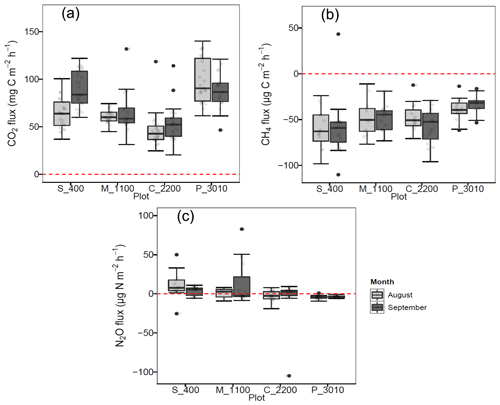
Figure 1(a) Soil CO2 (mg C m−2 h−1), (b) CH4 (µg C m−2 h−1), and (c) N2O (µg C m−2 h−1) fluxes per month at Río Silanche (400 m a.s.l.; S_400), Milpe (1100 m a.s.l.; M_1100), El Cedral (2200 m a.s.l.; C_2200), and Peribuela (3010 m a.s.l.; P_3010). Light gray boxplots indicate the fluxes in August 2018, whereas dark gray boxplots indicate the fluxes in September 2018. Light gray dots in each boxplot represent the measurements taken each day and black dots the outliers of the respective site. The dotted red line across the boxes indicates zero net flux.
Table 1Average measurements plus or minus the standard deviations (SDs) of soil CO2, CH4, and N2O fluxes at Río Silanche (400 m a.s.l.; S_400), Milpe (1100 m a.s.l.; M_1100), El Cedral (2200 m a.s.l.; C_2200), and Peribuela (3010 m a.s.l.; P_3010) per month.
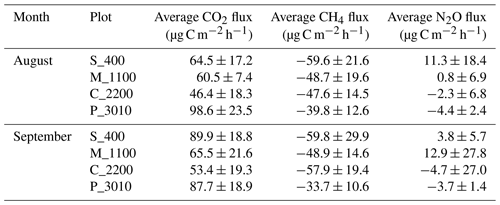
Note: flux values represent the mean of five chambers per site and measurement week using four-point time series and considering the constraint set to evaluate linearity in each measurement cycle (R2>0.65).
Across our study sites, P_3010 (the highest stratum) exhibited the highest soil CO2 emissions (Fig. 1a and Table 1) probably due to the dominant role of soil pH and shifts in C allocation patterns. The highest soil pH in water (pHwater) was observed at this site (Table 2), and under acid conditions, Sitaula et al. (1995) and Persson and Wiren (1989) reported a decrease in CO2 emissions with decreasing pHwater. On the other hand, although not measured or estimated in this study, an increase in fine root biomass is expected in tropical mountain forests compared to lowland forests due to imbalances or limitations in resource (water and/or nutrients) availability at higher altitudes (Bauters et al., 2017; Leuschner et al., 2007). Therefore, the observed increase in CO2 emissions at P_3010 might be further driven by an increase in root biomass as the latter has been shown to be positively correlated with soil respiration (Han et al., 2007; Luo and Zhou, 2006a; Reth et al., 2005; Silver et al., 2005).
Table 2Physicochemical soil properties of the study areas Río Silanche (400 m a.s.l.; S_400), Milpe (1100 m a.s.l.; M_1100), El Cedral (2200 m a.s.l.; C_2200), and Peribuela (3010 m a.s.l.; P_3010) at 5 and 20 cm depth, including mean values plus or minus the standard deviations (SDs) of bulk density (ρb), porosity, pH in water (pHwater) and KCl suspension (pHKCl), nitrate (NO) and ammonium (NH) concentrations, bulk nitrogen (N) and carbon (C) content, carbon-to-nitrogen ratio (C∕N), and δ15N signatures from samples of soil taken in August. Similar lowercase letters in superscript and next to some values within one row and per depth (5 and 20 cm) indicate no significant difference at P<0.05 between sites (S_400, M_1100, C_2200, and P_3010).
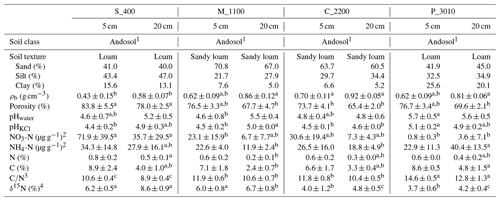
Note: mean values plus or minus the SD were calculated from soil samples taken adjacent to each soil chamber (n=5) except for soil texture for which composites for each site at 5 and 20 cm depth were made from the soil samples taken from each chamber. 1 Commonly known as Andisol in the United States Department of Agriculture (USDA) soil taxonomy. 2 Expressed per gram of dry soil. 3 Calculated by dividing C (%) by N (%) for each soil sample. 4 Expressed relative to the international standard AIR.
In contrast to P_3010, the low CO2 emissions observed at C_2200 could be attributed to (1) the lower WFPS (Fig. S3), (2) the lower contents of C and N (Table 2), or (3) the higher bulk density (Table 2). The lowest soil water content was observed at this site in August at 5 cm depth, and exactly in this month, the lowest emissions of CO2 were obtained. The low contents of C and N exhibited in C_2200 (indeed, the lowest from all the sites) could also have hampered the CO2 emissions (Dalal and Allen, 2008; Luo and Zhou, 2006a; Oertel et al., 2016). Additionally, this site had the highest soil bulk density (i.e., lowest porosity), which could have led to a decrease in soil respiration either by a physical impediment for root growth or by a decrease in soil aeration for microbial activities (Dilustro et al., 2005; Luo and Zhou, 2006a, b).
All sites acted as net sinks for CH4 (Fig. 1b and Table 1) (i.e., uptake of atmospheric CH4 by soils). During the entire field campaign (10 d), only one chamber at one site (S_400) and on a specific date (08/09/2018) acted as a net source of CH4 (43.2 µg CH4-C m−2 h−1). However, there were no statistical differences between months, and all sites exhibited indeed a high temporal and spatial variability.
Only S_400 and M_1100 (both months) (i.e., plots located at the lower locations) acted as net sources of N2O (Fig. 1c and Table 1), whereas the plots located at the highest stratum (P_3010 & C_2200) showed a general net N2O consumption during August and September.
The N2O emissions obtained at the lowest strata corroborate the literature data on lowland tropical forests (Butterbach-Bahl et al., 2004, 2013; Koehler et al., 2009) and could be mainly attributed to the soil water content, temperature, and N availability observed at these sites (Figs. S2 and S3 and Table 2). Firstly, N2O emissions in tropical forest soils are predominantly governed by WFPS which influences microbial activity, soil aeration, and thus the diffusion of N2O out of the soil (Davidson et al., 2006; Werner et al., 2007). Secondly, an increase in temperature leads to an increase in soil respiration and thus to a depletion of oxygen concentrations, which is indeed a major driver in N2O emissions. In fact, rising temperatures lead to a positive feedback in microbial metabolism, in which the stimulation of mineralization and nitrification processes induces an increase in the availability of substrates for denitrification and thus to an increase in N2O emissions (Butterbach-Bahl et al., 2013; Sousa Neto et al., 2011). Finally, the dependency of N2O emissions on WFPS and temperature is affected by substrate availability (NO). High contents of NO give an indication of an open or “leaky” N cycle with higher rates of mineralization, nitrification, and thus N2O emissions (Davidson et al., 2006). Moreover, NO is normally preferred as an electron acceptor over N2O, and it can also inhibit the rate of N2O consumption to N2 (Dalal and Allen, 2008).
In contrast to the low elevation sites where net N2O emissions were observed, P_3010 and C_2200 (Fig. 1c and Table 1) presented net consumption (negative values, i.e., fluxes from the atmosphere to the soil). From 35 valid measurements, only one resulted in net emission at P_3010 (range: −9.3 to 0.95 µg N2O-N m−2 h−1), whereas from 36 valid measurements, 19 resulted in net emissions at C_2200 (range: −104.9 to 9.3 µg N2O-N m−2 h−1). Net N2O consumption is often related to N-limited ecosystems, and it is presumably the cause in our case. At low NO concentrations, atmospheric and/or soil gaseous N2O may be the only electron acceptor left for denitrification (Chapui-Lardy et al., 2007; Goossens et al., 2001). P_3010 had the lowest content of NO along with the lowest soil δ15N (Table 2), which clearly reflects the shift towards a more closed N cycle at higher elevations (Bauters et al., 2017; Gerschlauer et al., 2019). In fact, studies performed by Teh et al. (2014) and Müller et al. (2015) in the southern Peruvian and Ecuadorian Andes, respectively, related the decrease in N2O emissions and thus the potential for N2 production in soils at high elevations to differences in NO availability. Moreover, Wolf et al. (2011) and Martinson et al. (2013) have indicated that N availability was (1) a dominant control on N2O fluxes and (2) inversely proportional to altitude. In addition, the low N2O fluxes could also be supported by the high content of clay (Table 2) and CO2 emissions (Fig. 1a) (i.e., development of microsites for N2O reduction) along with the low soil water content (% of WFPS) (Fig. S3) (i.e., better diffusion of atmospheric N2O into the soil) and higher soil pH value (Table 2) (i.e., less severe inhibition of the nitrous oxide reductase) observed at P_3010 (Chapui-Lardy et al., 2007).
It is important to mention that the region where these measurements were taken is characterized by a marked seasonality in rainfall. We measured at the end of the dry season; thus, it is expected that there will be fluctuations in net fluxes (sources vs sinks) depending on the season. Moreover, although our limited dataset did not allow us to corroborate the main drivers that controlled these fluxes, daily measurements like those carried out here reflect the importance of evaluating short-term variations. As such, the net N2O consumption with increasing altitude might be overlooked in an annual analysis, but it is equally important to (1) understand the mechanisms behind the production and consumption of N2O and (2) have reliable estimates of the N2O source/sink strength of tropical forests for regional and even global GHG budgets. Moreover, in order to corroborate the net consumption observed at high altitudes and improve the understanding of N2O dynamics in terrestrial ecosystems, disentangling gross N2O production and consumption at field scale is needed. Although the most commonly used method to measure N2O fluxes via static chambers only allows the quantification of net fluxes, stable isotope techniques would greatly contribute to our mechanistic understanding of gross fluxes. For instance, enrichment and natural abundance approaches (18O, 15N) can be used to identify and estimate the contribution of different microbial processes to N2O production/consumption (Butterbach-Bahl et al., 2013; Yu et al., 2020). Nevertheless, (1) the coupling of isotope techniques with molecular analyses of functional genes is paramount to fully understand the complexity of the microbial processes present, and (2) the improvement of measuring techniques for N2O reduction is needed to close N ecosystem balances (Butterbach-Bahl et al., 2013; Chapui-Lardy et al., 2007). In fact, microbial composition and diversity, as well as the presence or absence of important genes (e.g., N2O reductase nosZ I and nosZ II; Van Groenigen et al., 2015b) can help to detect N2O consumption. Similarly, analytical techniques such as Raman gas spectroscopy could be used to detect and quantify N2 fluxes from denitrification (Frosch et al., 2016), which is indeed a novel and simple approach compared to previously widely used techniques that may have led to underestimations (Fang et al., 2015).
GHG fluxes from tropical montane forests in South America are particularly scarce with limited spatial coverage and seasonal fluctuation in rainfall but important for consideration in future field measurements and modeling research. Overall, we found an unusual but marked increase in CO2 emissions at the highest altitude, which is probably explained by soil pH and root biomass even though the latter was not measured or estimated. Our CH4 uptake fluxes exhibited a high temporal and spatial variability but reiterate the role of humid tropical forest soils as CH4 sinks. Contrary to the net N2O emissions observed in the lowest strata, the net consumption at higher elevations seems to be quite unique, and it might reflect the shift towards a more closed N cycle at higher altitudes that was reported previously in tropical regions. Our results highlight the importance of short-term variations in N2O fluxes, but it calls for more and broader studies especially in tropical montane forests, including the impact of spatial and temporal variability, forest management events and forest rotation cycles, ground vegetation composition and cover, and soil microclimate and hydrological conditions, as well as the implementation of isotope techniques, the coupling of microbial analysis with N2O fluxes, and the response of tropical forests to current and future changes in N content.
In terms of spatial variation, GHG fluxes may vary between lower slope, mid-slope, and/or ridge (see Table S1) (Courtois et al., 2018; Teh et al., 2014; Wolf et al., 2011, 2012). Fluctuations of net fluxes can be observed depending on the season and the transition between them (see Table S1) (Butterbach-Bahl et al., 2013; Kim et al., 2016a). Management events (e.g., thinning, clear cutting, fertilization, draining improvements) and/or forest composition and growth stage (e.g., young vs mature forest) may influence, e.g., forest vegetation, soil characteristics, hydrology, and nutrient management among others and ultimately lead to changes in soil GHG fluxes (Barrena et al., 2013; Jauhiainen et al., 2019; Kim et al., 2016a). Moreover, soil hydrology (runoff, evapotranspiration, soil moisture, etc.) may affect biogeochemical cycles (Kim et al., 2016a). Microbial composition and diversity could be a key to understand the variability in N2O fluxes (Butterbach-Bahl et al., 2013). Changes in N content – due to, e.g., urban development and increasing use of agricultural land – could cause shifts in soil N cycling and thus CO2, CH4, and N2O fluxes (Koehler et al., 2012). Besides this, the effects of climate change on tropical regions (e.g., increases in temperature and CO2 concentrations, as well as changes in rainfall patterns and drought events) may also affect soil GHG fluxes. Therefore, a strategic plan must be implemented. Long-term data of at least 1 or 2 hydrological years is needed, with sampling intervals covering seasonal fluctuations and being appropriate for the type of land (i.e., spatial variability across different topographical positions). The effect of N content and climate change in tropical forests could be evaluated using laboratory (e.g., incubations under controlled conditions) and/or field experiments (e.g., see Koehler et al., 2009, 2012; Hall and Matson, 1999; Martinson et al., 2013) with the use of altitudinal gradients like biogeochemical open-air laboratories. Finally, although sampling conditions in tropical montane regions can be challenging, (1) establishing networks and collaborations with local communities (i.e., citizen science) could contribute not only in terms of data acquisition but also in the development of local knowledge (e.g., how climate and land use change might affect ecosystems and people), and (2) modeling approaches for C and N biogeochemistry in forest ecosystems (e.g., Forest-DeNitrification-DeComposition, DNDC, model; GRAMP, 2013) could help to upscale fluxes from site to regional level. Nevertheless, the cooperation and contribution between field researchers and scientific organizations, e.g., in South America and around the world, as well as the capacity building in the respective countries, are crucial for improving our understanding of soil GHG fluxes from tropical regions and paramount for getting tangible datasets of remote regions such as montane forests.
The data used in this study have been published in Zenodo and are available under: https://doi.org/10.5281/zenodo.4412827 (last access: 3 Januray 2021, Lamprea Pineda et al., 2021).
The supplement related to this article is available online at: https://doi.org/10.5194/bg-18-413-2021-supplement.
MarB, HV, SeB, and PB developed the project. PALP and MarB carried out the fieldwork and analyzed the data. MatB and SaB provided technical and analytical support in analyzing the gas and soil samples. All authors contributed to the ideas presented and edited the paper.
The authors declare that they have no conflict of interest.
We thank Mindo Cloud Forest Foundation, El Cedral Ecolodge, and the Escuela Politécnica Nacional del Ecuador for the logistic support in Ecuador.
This research has been supported by Ghent University and the VLIR-UOS South Initiative COFOREC (EC2018SIN223A103) and COFOREC II (EC2020SIN279A103). Matti Barthel was supported through ETH Zurich core funding provided to Johan Six, and Marijn Bauters is funded as a postdoctoral fellow of the Research Foundation – Flanders (FWO).
This paper was edited by Kees Jan van Groenigen and reviewed by Klaus Butterbach-Bahl and two anonymous referees.
Barrena, I., Menéndez, S., Duñabeitia, M., Merino, P., Florian Stange, C., Spott, O., González-Murua, C., and Estavillo, J. M.: Greenhouse gas fluxes (CO2, N2O and CH4) from forest soils in the Basque Country: Comparison of different tree species and growth stages, For. Ecol. Manage., 310, 600–611, https://doi.org/10.1016/j.foreco.2013.08.065, 2013.
Bauters, M., Verbeeck, H., Demol, M., Bruneel, S., Taveirne, C., Van der Heyden, D., Cizungu, L., and Boeckx, P.: Parallel functional and stoichiometric trait shifts in South American and African forest communities with elevation, Biogeosciences, 14, 5313–5321, https://doi.org/10.5194/bg-14-5313-2017, 2017.
Bonan, G. B.: Forests and climate change: forcings, feedbacks, and the climate benefits of forests, Science, 320, 1444–1449, https://doi.org/10.1126/science.1155121, 2008.
Bubb, P., May, I., Miles, L., and Sayer, J.: Cloud Forest Agenda, in United Nations Environment Programme-World Conservation Monitoring Centre, Cambridge, UK, availabe at: http://www.unep-wcmc.org/index.html?http://www.unep-wcmc.org/resources/publications/UNEP_WCMC_bio_series/20.html (last access: 22 March 2020), 2004.
Butterbach-Bahl, K., Kock, M., Willibald, G., Hewett, B., Buhagiar, S., Papen, H., and Kiese, R.: Temporal variations of fluxes of NO, NO2, N2O, CO2, and CH4 in a tropical rain forest ecosystem, Global Biogeochem. Cy., 18, 1–11, https://doi.org/10.1029/2004GB002243, 2004.
Butterbach-Bahl, K., Baggs, E. M., Dannenmann, M., Kiese, R., and Zechmeister-Boltenstern, S.: Nitrous oxide emissions from soils: how well do we understand the processes and their controls?, Phil. Trans. R. Soc. B., 368, 1–20, 2013.
Castaldi, S., Bertolini, T., Valente, A., Chiti, T., and Valentini, R.: Nitrous oxide emissions from soil of an African rain forest in Ghana, Biogeosciences, 10, 4179–4187, https://doi.org/10.5194/bg-10-4179-2013, 2013.
Chapui-Lardy, L., Wrage, N., Metay, A., Chotte, J.-L., and Bernoux, M.: Soils, a sink for N2O? A review, Glob. Change Biol., 13, 1–17, https://doi.org/10.1111/j.1365-2486.2006.01280.x, 2007.
Clough, T. J., Lanigan, G. J., de Klein, C. A. M., Sainur Samar, M., Morales, S. E., Rex, D., Bakken, L. R., Johns, C., Condron, L. M., Grant, J., and Richards, K. G.: Influence of soil moisture on codenitrification fluxes from a urea-affected pasture soil, Sci. Rep.-UK, 7, 1–12, https://doi.org/10.1038/s41598-017-02278-y, 2017.
Courtois, E. A., Stahl, C., Van den Berge, J., Bréchet, L., Van Langenhove, L., Richter, A., Urbina, I., Soong, J. L., Peñuelas, J., and Janssens, I. A.: Spatial variation of soil CO2, CH4 and N2O fluxes across topographical positions in tropical forests of the Guiana Shield, Ecosystems, 21, 1445–1458, https://doi.org/10.1007/s10021-018-0232-6, 2018.
Dalal, R. C. and Allen, D. E.: TURNER REVIEW No. 18 Greenhouse gas fluxes from natural ecosystems, Aust. J. Bot., 56, 369–407, https://doi.org/10.1071/BT07128, 2008.
Davidson, E. A., Keller, M., Erickson, H. E., Verchot, L. V., and Veldkamp, E.: Testing a conceptual model of soil emissions of nitrous and nitric oxides, Bioscience, 50, 667–680, https://doi.org/10.1641/0006-3568(2000)050[0667:tacmos]2.0.co;2, 2006.
Dilustro, J. J., Collins, B., Duncan, L., and Crawford, C.: Moisture and soil texture effects on soil CO2 efflux components in southeastern mixed pine forests, For. Ecol. Manage., 204, 85–95, https://doi.org/10.1016/j.foreco.2004.09.001, 2005.
Fadrique, B., Báez, S., Duque, Á., Malizia, A., Blundo, C., Carilla, J., Osinaga-Acosta, O., Malizia, L., Silman, M., Farfán-Ríos, W., Malhi, Y., Young, K. R., Cuesta C. F., Homeier, J., Peralvo, M., Pinto, E., Jadan, O., Aguirre, N., Aguirre, Z., and Feeley, K. J.: Widespread but heterogeneous responses of Andean forests to climate change, Nature, 564, 207–212, https://doi.org/10.1038/s41586-018-0715-9, 2018.
Fang, Y., Koba, K., Makabe, A., Takahashi, C., Zhu, W., Hayashi, T., Hokari, A. A., Urakawa, R., Bai, E., Houlton, B. Z., Xi, D., Zhang, S., Matsushita, K., Tu, Y., Liu, D., Zhu, F., Wang, Z., Zhou, G., Chen, D., Makita, T., Toda, H., Liu, X., Chen, Q., Zhang, D., Li, Y., and Yoh, M.: Microbial denitrification dominates nitrate losses from forest ecosystems, P. Natl. Acad. Sci., 112, 1470–1474, https://doi.org/10.1073/pnas.1416776112, 2015.
Frosch, T., Popp, J., Trumbore, S. E., Jochum, T., and Fastnacht, A.: Direct Raman Spectroscopic Measurements of Biological Nitrogen Fixation under Natural Conditions: An Analytical Approach for Studying Nitrogenase Activity, Anal. Chem., 89, 1117–1122, https://doi.org/10.1021/acs.analchem.6b03101, 2016.
Gerschlauer, F., Saiz, G., Schellenberger Costa, D., Kleyer, M., Dannenmann, M., and Kiese, R.: Stable carbon and nitrogen isotopic composition of leaves, litter, and soils of various ecosystems along an elevational and land-use gradient at Mount Kilimanjaro, Tanzania, Biogeosciences, 16, 409–424, https://doi.org/10.5194/bg-16-409-2019, 2019.
Goossens, A., De Visscher, A., Boeckx, P., and Van Cleemput, O.: Two-year field study on the emission of N2O from coarse and middle-textured Belgian soils with different land use, Nutr. Cycl. Agroecosystems, 60, 23–34, https://doi.org/10.1023/A:1012695731469, 2001.
Grace, J., José, J. S., Meir, P., Miranda, H. S., and Montes, R. A.: Productivity and carbon fluxes of tropical savannas, J. Biogeogr., 33, 387–400, https://doi.org/10.1111/j.1365-2699.2005.01448.x, 2006.
GRAMP: Forest-DNDC, About For., available at: http://gramp.ags.io/models/1 (last access: 18 July 2020), 2013.
Hall, S. J. and Matson, P. A.: Nitrogen oxide emissions after nitrogen additions in tropical forests, Lett. Nat., 400, 152–155, https://doi.org/10.1038/22094, 1999.
Han, G., Zhou, G., Xu, Z., Yang, Y., Liu, J., and Shi, K.: Biotic and abiotic factors controlling the spatial and temporal variation of soil respiration in an agricultural ecosystem, Soil Biol. Biochem., 39, 418–425, https://doi.org/10.1016/j.soilbio.2006.08.009, 2007.
Heil, J., Wolf, B., Brüggemann, N., Emmenegger, L., Tuzson, B., Vereecken, H., and Mohn, J.: Site-specific 15N isotopic signatures of abiotically produced N2O, Geochim. Cosmochim. Ac., 139, 72–82, https://doi.org/10.1016/j.gca.2014.04.037, 2014.
Heil, J., Vereecken, H., and Brüggemann, N.: A review of chemical reactions of nitrification intermediates and their role in nitrogen cycling and nitrogen trace gas formation in soil, Eur. J. Soil Sci., 67, 23–39, https://doi.org/10.1111/ejss.12306, 2016.
Jang, I., Lee, S., Hong, J. H., and Kang, H.: Methane oxidation rates in forest soils and their controlling variables: A review and a case study in Korea, Ecol. Res., 21, 849–854, https://doi.org/10.1007/s11284-006-0041-9, 2006.
Jauhiainen, J., Alm, J., Bjarnadottir, B., Callesen, I., Christiansen, J. R., Clarke, N., Dalsgaard, L., He, H., Jordan, S., Kazanavičiūtė, V., Klemedtsson, L., Lauren, A., Lazdins, A., Lehtonen, A., Lohila, A., Lupikis, A., Mander, Ü., Minkkinen, K., Kasimir, Å., Olsson, M., Ojanen, P., Óskarsson, H., Sigurdsson, B. D., Søgaard, G., Soosaar, K., Vesterdal, L., and Laiho, R.: Reviews and syntheses: Greenhouse gas exchange data from drained organic forest soils – a review of current approaches and recommendations for future research, Biogeosciences, 16, 4687–4703, https://doi.org/10.5194/bg-16-4687-2019, 2019.
Jobbágy, E. G. and Jackson, R. B.: The vertical distribution of soil organic carbon and its relation to climate and vegetation, Ecol. Appl., 10, 423, https://doi.org/10.2307/2641104, 2000.
Jones, S. P., Diem, T., Huaraca Quispe, L. P., Cahuana, A. J., Reay, D. S., Meir, P., and Teh, Y. A.: Drivers of atmospheric methane uptake by montane forest soils in the southern Peruvian Andes, Biogeosciences, 13, 4151–4165, https://doi.org/10.5194/bg-13-4151-2016, 2016.
Kahmen, A., Sachse, D., Arndt, S. K., Tu, K. P., Farrington, H., Vitousek, P. M., and Dawsona, T. E.: Cellulose δ18O is an index of leaf-to-air vapor pressure difference (VPD) in tropical plants, P. Natl. Acad. Sci., 108, 1981–1986, https://doi.org/10.1073/pnas.1018906108, 2011.
Killeen, T. J., Douglas, M., Consiglio, T., Jørgensen, P. M., and Mejia, J.: Dry spots and wet spots in the Andean hotspot, J. Biogeogr., 34, 1357–1373, https://doi.org/10.1111/j.1365-2699.2006.01682.x, 2007.
Kim, D.-G., Thomas, A. D., Pelster, D., Rosenstock, T. S., and Sanz-Cobena, A.: Greenhouse gas emissions from natural ecosystems and agricultural lands in sub-Saharan Africa: synthesis of available data and suggestions for further research, Biogeosciences, 13, 4789–4809, https://doi.org/10.5194/bg-13-4789-2016, 2016a.
Kim, D.-G., Thomas, A. D., Pelster, D., Rosenstock, T. S., and Sanz-Cobena, A.: Greenhouse gas emissions from natural ecosystems and agricultural lands in sub-Saharan Africa: synthesis of available data and suggestions for further research, Biogeosciences, 13, 4789–4809, https://doi.org/10.5194/bg-13-4789-2016, 2016b.
Koehler, B., Corre, M. D., Veldkamp, E., Wullaert, H., and Wright, S. J.: Immediate and long-term nitrogen oxide emissions from tropical forest soils exposed to elevated nitrogen input, Glob. Change Biol., 15, 2049–2066, https://doi.org/10.1111/j.1365-2486.2008.01826.x, 2009.
Koehler, B., Corre, M. D., Steger, K., Well, R., Zehe, E., Sueta, J. P., and Veldkamp, E.: An in-depth look into a tropical lowland forest soil: Nitrogen-addition effects on the contents of N2O, CO2 and CH4 and N2O isotopic signatures down to 2-m depth, Biogeochemistry, 111, 695–713, https://doi.org/10.1007/s10533-012-9780-6, 2012.
Körner, C.: The use of “altitude” in ecological research, Trends Ecol. Evol., 22, 569–574, https://doi.org/10.1016/j.tree.2007.09.006, 2007.
Lamprea Pineda, P. A., Bauters, M., Verbeeck, H., Baez, S., Barthel, M., Bodé, S., and Boeckx, P.: Dataset: Ideas and perspectives: patterns of soil CO2, CH4, and N2O fluxes along an altitudinal gradient – a pilot study from an Ecuadorian neotropical montane forest [Data set], available at: http://doi.org/10.5281/zenodo.4412827, Zenodo, last access: 3 Januray 2021.
Laughlin, D. C. and Abella, S. R.: Abiotic and biotic factors explain independent gradients of plant community composition in ponderosa pine forests, Ecol. Modell., 205, 231–240, https://doi.org/10.1016/j.ecolmodel.2007.02.018, 2007.
Leuschner, C., Moser, G., Bertsch, C., Röderstein, M., and Hertel, D.: Large altitudinal increase in tree root/shoot ratio in tropical mountain forests of Ecuador, Basic Appl. Ecol., 8, 219–230, https://doi.org/10.1016/j.baae.2006.02.004, 2007.
Li, L., Zheng, Z., Wang, W., Biederman, J. A., Xu, X., Ran, Q., Qian, R., Xu, C., Zhang, B., Wang, F., Zhou, S., Cui, L., Che, R., Hao, Y., Cui, X., Xu, Z., and Wang, Y.: Terrestrial N2O emissions and related functional genes under climate change: A global meta-analysis, Glob. Change Biol., 26, 931–943, https://doi.org/10.1111/gcb.14847, 2020.
Luo, Y. and Zhou, X.: Controlling Factors, in: Soil Respiration and the Environment, edited by: Luo, Y. and Zhou, X., Elsevier, San Diego, USA, 79–105, 2006a.
Luo, Y. and Zhou, X.: Responses to disturbances, in: Soil Respiration and the Environment, edited by: Luo, Y. and Zhou, X., Elsevier, San Diego, USA, 133–158, 2006b.
Malhi, Y., Silman, M., Salinas, N., Bush, M., Meir, P., and Saatchi, S.: Introduction: Elevation gradients in the tropics: laboratories for ecosystem ecology and global change research, Glob. Change Biol., 16, 3171–3175, https://doi.org/10.1111/j.1365-2486.2010.02323.x, 2010.
Martinson, G. O., Corre, M. D., and Veldkamp, E.: Responses of nitrous oxide fluxes and soil nitrogen cycling to nutrient additions in montane forests along an elevation gradient in southern Ecuador, Biogeochemistry, 112, 625–636, https://doi.org/10.1007/s10533-012-9753-9, 2013.
Montzka, S. A., Dlugokencky, E. J., and Butler, J. H.: Non-CO2 greenhouse gases and climate change, Nature, 476, 43–50, https://doi.org/10.1038/nature10322, 2011.
Müller, A. K., Matson, A. L., Corre, M. D., and Veldkamp, E.: Soil N2O fluxes along an elevation gradient of tropical montane forests under experimental nitrogen and phosphorus addition, Front. Earth Sci., 3, 1–12, https://doi.org/10.3389/feart.2015.00066, 2015.
Myers, N., Mittermeier, R. A., Mittermeier, C. G., da Fonseca, G. A. B., and Kent, J.: Biodiversity hotspots for conservation priorities, Nature, 43, 853–858, https://doi.org/10.1080/21564574.1998.9650003, 2000.
Oertel, C., Matschullat, J., Zurba, K., Zimmermann, F., and Erasmi, S.: Greenhouse Gas Emissions From Soil – A review, Chem. Erde, 76, 327–352, https://doi.org/10.1016/j.chemer.2016.04.002, 2016.
Pan, Y., Birdsey, R. A., Fang, J., Houghton, R., Kauppi, P. E., Kurz, W. A., Philips, O. L., Shvidenko, A., Lwis, S. L., Canadell, J. G., Ciais, P., Jackson, R. B., Pacala, S. W., McGuire, D. A., Piao, S., Rautiainen, A., Sitch, S., and Hayes, D.: A large and persistent carbon sink in the world's forests, Science, 333, 988–992, https://doi.org/10.1126/science.1201609, 2011.
Perez, T. M., Stroud, J. T., and Feeley, K. J.: Thermal trouble in the tropics, Science, 351, 1392–1393, https://doi.org/10.1126/science.aaf3343, 2016.
Persson, T. and Wiren, A.: Microbial activity in forest soils in relation to acid/base and carbon/nitrogen status, in: Air Pollution as Stress Factor in Nordic Forests, edited by: Braekke, F.H., Bjor, K., and Halvorsen, B., Norwegian Institute for Forest Research, Aas., Norway, 83–95, 1989.
Purbopuspito, J., Veldkamp, E., Brumme, R., and Murdiyarso, D.: Trace gas fluxes and nitrogen cycling along an elevation sequence of tropical montane forests in Central Sulawesi, Indonesia, Global Biogeochem. Cy., 20, 11, https://doi.org/10.1029/2005GB002516, 2006.
Reth, S., Reichstein, M., and Falge, E.: The effect of soil water content, soil temperature, soil pH-value and the root mass on soil CO2 efflux – a modified model, Plant Soil, 268, 21–33, https://doi.org/10.1007/s11104-005-0175-5, 2005.
Silver, W. L., Thompson, A. W., McGroddy, M. E., Varner, R. K., Dias, J. D., Silva, H., Crill, P. M., and Keller, M.: Fine root dynamics and trace gas fluxes in two lowland tropical forest soils, Glob. Change Biol., 11, 290–306, https://doi.org/10.1111/j.1365-2486.2005.00903.x, 2005.
Sitaula, B. K., Bakken, L. R., and Abrahamsen, G.: N-fertilization and soil acidification effects on N2O and CO2 emission from temperate pine forest soil, Soil Biol. Biochem., 27, 1401–1408, https://doi.org/10.1016/0038-0717(95)00078-S, 1995.
Sousa Neto, E., Carmo, J. B., Keller, M., Martins, S. C., Alves, L. F., Vieira, S. A., Piccolo, M. C., Camargo, P., Couto, H. T. Z., Joly, C. A., and Martinelli, L. A.: Soil-atmosphere exchange of nitrous oxide, methane and carbon dioxide in a gradient of elevation in the coastal Brazilian Atlantic forest, Biogeosciences, 8, 733–742, https://doi.org/10.5194/bg-8-733-2011, 2011.
Spahni, R., Wania, R., Neef, L., van Weele, M., Pison, I., Bousquet, P., Frankenberg, C., Foster, P. N., Joos, F., Prentice, I. C., and van Velthoven, P.: Constraining global methane emissions and uptake by ecosystems, Biogeosciences, 8, 1643–1665, https://doi.org/10.5194/bg-8-1643-2011, 2011.
Su, Q., Domingo-Félez, C., Jensen, M. M., and Smets, B. F.: Abiotic nitrous oxide (N2O) production is strongly pH dependent, but contributes little to overall N2O emissions in biological nitrogen removal systems, Environ. Sci. Technol., 53, 3508–3516, https://doi.org/10.1021/acs.est.8b06193, 2019.
Teh, Y. A., Diem, T., Jones, S., Huaraca Quispe, L. P., Baggs, E., Morley, N., Richards, M., Smith, P., and Meir, P.: Methane and nitrous oxide fluxes across an elevation gradient in the tropical Peruvian Andes, Biogeosciences, 11, 2325–2339, https://doi.org/10.5194/bg-11-2325-2014, 2014.
van Cleemput, O.: Subsoils: chemo- and biological denitrification, N2O and N2 emissions, Nutr. Cycl. Agroecosystems, 52, 187–194, https://doi.org/10.1023/a:1009728125678, 1998.
van Groenigen, J. W., Huygens, D., Boeckx, P., Kuyper, Th. W., Lubbers, I. M., Rütting, T., and Groffman, P. M.: The soil N cycle: new insights and key challenges, SOIL, 1, 235–256, https://doi.org/10.5194/soil-1-235-2015, 2015b.
Werner, C., Kiese, R., and Butterbach-Bahl, K.: Soil-atmosphere exchange of N2O, CH4, and CO2 and controlling environmental factors for tropical rain forest sites in western Kenya, J. Geophys. Res., 112, D03308, https://doi.org/10.1029/2006JD007388, 2007.
Wolf, K., Veldkamp, E., Homeier, J., and Martinson, G. O.: Nitrogen availability links forest productivity, soil nitrous oxide and nitric oxide fluxes of a tropical montane forest in southern Ecuador, Global Biogeochem. Cy., 25, 1–12, https://doi.org/10.1029/2010GB003876, 2011.
Wolf, K., Flessa, H., and Veldkamp, E.: Atmospheric methane uptake by tropical montane forest soils and the contribution of organic layers, Biogeochemistry, 111, 469–483, https://doi.org/10.1007/s10533-011-9681-0, 2012.
Yu, L., Harris, E., Lewicka-Szczebak, D., Barthel, M., Blomberg, M. R. A., Harris, S. J., Johnson, M. S., Lehmann, M. F., Liisberg, J., Müller, C., Ostrom, N. E., Six, J., Toyoda, S., Yoshida, N., and Mohn, J.: What can we learn from N2O isotope data? – Analytics, processes and modelling, Rapid Commun. Mass Spectrom., 34, 1–14, https://doi.org/10.1002/rcm.8858, 2020.
- Abstract
- The importance of tropical forests for greenhouse gas budgets
- Altitudinal gradients as a biogeochemical open-air laboratory
- What did we see in Ecuador?
- Conclusions and future directions
- Data availability
- Author contributions
- Competing interests
- Acknowledgements
- Financial support
- Review statement
- References
- Supplement
- Abstract
- The importance of tropical forests for greenhouse gas budgets
- Altitudinal gradients as a biogeochemical open-air laboratory
- What did we see in Ecuador?
- Conclusions and future directions
- Data availability
- Author contributions
- Competing interests
- Acknowledgements
- Financial support
- Review statement
- References
- Supplement