the Creative Commons Attribution 4.0 License.
the Creative Commons Attribution 4.0 License.
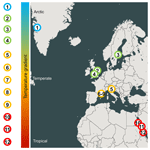
Ideas and perspectives: The fluctuating nature of oxygen shapes the ecology of aquatic habitats and their biogeochemical cycles – the aquatic oxyscape
Sylvain Rigaud
Giovanna Guadagnin
Alberto Barausse
Ramona Marasco
Daniele Daffonchio
Julie Régis
Louison Huchet
Capucine Camin
Laura Pettit
Cristina Vina-Herbon
Oxygen availability is a pivotal factor for ecosystem functioning and the resistance of organisms to the effect of climate change in aquatic habitats. Although extensive work has been done to assess the effect of oxygen on marine and freshwater biota, many studies have not captured the ecological importance of oxygen variations. Overlooking the fluctuating nature of oxygen may cause potential biases in the design and implementation of management policies for aquatic habitats. Conceptual perspectives on the dynamic nature of oxygen fluctuations have been raised in the scientific community in order to enhance the understanding of the effect of oxygen on the physiology and the ecology of aquatic species as well as the biogeochemical functioning of their ecosystems. A growing number of empirical work has been outlining a novel conceptual framework that considers the magnitude of oxygen fluctuation as a key variable that explains adaptation to stress conditions. Oxygen in productive aquatic habitats shows large fluctuations at the diel scale, exposing aquatic species to conditions ranging from extreme supersaturation to anoxia. Recent research has indicated that such a fluctuation tunes the physiological plasticity of the animal in response to thermal stresses. In this paper, we provide compelling evidence based on current research that the fluctuating oxygen landscape, here defined as “oxyscape”, has an important role in aquatic animal physiology and adaptation as well as the ecosystem biogeochemistry. We propose that the oxyscape should be considered in the modelling and managing policies of aquatic ecosystems.
- Article
(3531 KB) - Full-text XML
-
Supplement
(24393 KB) - BibTeX
- EndNote
The actual assembly of organism communities in a given habitat is determined by the specific environmental conditions that select specific sets of species. Climate anomalies and changes raise concerns over ecosystem stability and habitat preservation, with consequences ranging from species substitution to species extinction (Antão et al., 2020). To model and predict such effects on community composition and stability, it is necessary to learn about the limits of the environmental conditions that challenge the different species (Bennett et al., 2021). However, in many cases, such conditions are resumed into averaged parameters that do not capture the whole range to which organisms are exposed to (Bernhardt et al., 2020).
In aquatic environments, chemical and physical parameters vary greatly at different timescales and spatial scales, following cyclical fluctuation or stochastic variations (Shaw et al., 2013; Kerrison et al., 2011; Vargas et al., 2017, 2022; Manzello, 2010; Challener et al., 2016; Duarte et al., 2013). The lack of details on such variations in time and space challenges our understanding of how the species adapt their physiology, limiting our estimation of their resilience to ongoing environmental changes (Booth et al., 2023a). The perception of such a knowledge gap is progressively moving the research interest on species' eco-physiological response to chemical and physical parameters, such as temperature, salinity, or pH, and the associated biogeochemical cycles toward more accurate assessments of their natural variability (Antão et al., 2020; Bernhardt et al., 2020). Increasing efforts are now devoted to upgrading eco-physiological approaches to integrate the relevant environmental background (Giomi et al., 2019; Marasco et al., 2023; Bitter et al., 2018, 2021; Pörtner et al., 2023).
Oxygen variability exerts a great effect on aquatic life yet remains underestimated. Most studies dealing with oxygen variation have addressed the decline of mean oxygen availability in oceans (Breitburg et al., 2018) and the occurrence and repercussions of events of environmental hypoxia (Diaz and Rosenberg, 2008; Bickler and Buck, 2007). How short-term (i.e. daily) oxygen variations shape aquatic life and biogeochemical processes remains largely overlooked. These variations are generally of greater amplitudes and more regular than seasonal ones (Limburg et al., 2020; Bates et al., 2018). We refer to deviation from the saturation of oxygen concentrations as the ratio between the actual concentration and the saturation concentration of oxygen that would be observed in water under equilibrium conditions between air and water. The fine measure of the range of variation in aquatic oxygen and its effect on organisms and biogeochemical cycles has the potential to elucidate unpredicted mechanisms of resilience and tolerance to ongoing climate change.
A gradual decline in the total oxygen content of about 2 % since the second half of the 20th century has recently been reported, with a further reduction of up to 7 % predicted for the following century (Schmidtko et al., 2017; Breitburg et al., 2018). In parallel, open-ocean oxygen minimum zones (OMZs) have largely expanded (Stramma et al., 2010) together with the increasing occurrence of severe hypoxia events whose predictability has decreased as their occurrence spreads across all oceans (Diaz and Rosenberg, 2008). The main cause of deoxygenation is attributed to global warming, and deoxygenation is exacerbated by the discharge of nutrients and pollutants (Ito et al., 2016). The topography of water basins also affects the incidence of hypoxia events, thereby influencing the rate of mixing of oxygenated layers and the resident time of water bodies (Breitburg and Grégoire, 2018; Laffoley and Baxter, 2019). For example, in the Baltic Sea, the geomorphology of the coastline can explain up to 80 % of the hypoxic phenomena (Virtanen et al., 2019).
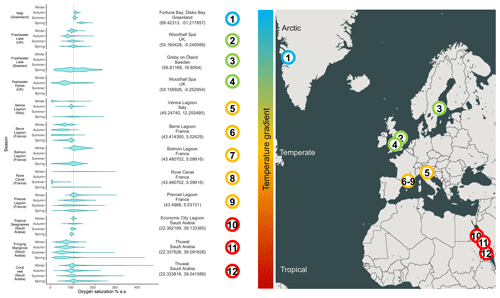
Figure 1Range of oxygen saturations in several productive aquatic habitats. The dashed line represents 100 % oxygen saturation. The violin plots report the densities of the frequencies of oxygen saturation measured at each ecosystem across different seasons (based on data availability). The data source is specified for each environment in the supplementary dataset for Figs. 1 and 2.
The gradual decrease in total oxygen in oceans has been reported in several studies that analyse long temporal trends (Keeling et al., 2010; Schmidtko et al., 2017; Breitburg et al., 2018) and in reports at the global geographical scale (Laffoley and Baxter, 2016). However, unexpected oxygen profiles have also been reported in several specific habitats and at different timescales, suggesting that different abiotic and biotic drivers modulate oxygen dynamics (Craig and Hayward, 1983). For instance, the alternation of oxygen biological production and consumption determines a massive fluctuation in oxygen availability in highly productive aquatic habitats, such as mangrove forests, salt marshes, coral reefs (Giomi et al., 2019; Fusi et al., 2021; Booth et al., 2021), kelp forests (Krause-Jensen et al., 2016), plankton blooms in pelagic systems (Riser and Johnson, 2008; Benoiston et al., 2017), freshwater lakes (Andersen et al., 2017), or even agricultural drainage channels (Booth et al., 2023a). The seasonal variation in diel oxygen fluctuations is increasingly reported at all latitudes and habitats, and it occurs in a vast range of water bodies independently of the scale, the hydrological features, and the local biotic components (Fig. 1). For example, fluctuations of 150 % in spring and 30 % in winter occur in the Venice lagoon (Fig. 1, Location 5), and even more pronounced fluctuations occur in mangroves, corals, and seagrasses (Fig. 1, Locations 10–12; Giomi et al., 2019). The magnitude of oxygen fluctuations is site-specific and depends, among other factors, on the solar radiation and water temperature and their effect on primary producers' photosynthetic and respiration activity, the cycles of water column stratification and mixing, and the nutrient loads. Spatial differences in oxygen saturation also occur between surface and bottom water masses because of isolation driven by water stratification, the decrease in light penetration and the coupling with increased oxygen demand close to the sediments at the bottom of the water column (Fig. 2). In summer, enhanced benthic respiration is determined by a higher water temperature, which decreases oxygen solubility and enhances oxygen demand. Lower vertical mixing, higher water residence time and higher turbidity due to a higher concentration of organic matter further decrease oxygen concentrations, causing hypoxia in the deep water layers (Fig. 2; Talke et al., 2009; Schmidt et al., 2019). Conversely, dissolved oxygen increases in the euphotic part of the water column because of the enrichment of photosynthetic communities (Spietz et al., 2015).
2.1 The oxygen variation in the daily lives of aquatic organisms
Marked oxygen variations occur at a diel scale, driven by temperature and light diel cycles as well as the balance between photosynthesis and respiration (Winter et al., 2019). The amplitude of diel oxygen variation can exceed the average seasonal variation, thus being variable with a higher explanatory power for the physiology of aquatic life (Giomi et al., 2019). While water temperature is only linked to meteorological changes, the oxygen concentration in productive aquatic environments is also regulated by the biota component (Chapman, 2021). The community composition of primary producers (Power and Cardinale, 2009), rainfall level (Mallin et al., 1993), nutrients' runoff (Kinney and Roman, 1998), biotic networks (Graham et al., 2018; Breen and Mann, 1976) and bacterial community dynamics (Guo et al., 2022) govern, at a small scale, high spatial and temporal changes in the concentrations of dissolved oxygen. The interaction of all these factors strongly affects dissolved oxygen fluctuations beyond the levels that can be predicted from just the meteorological trends.
Oxygen availability plays an important role in the physiology of aquatic species (Pörtner, 2010; Hochachka, 1991; Stillman and Somero, 2000). In the design of experiments on the physiology of aquatic animals, oxygen is frequently treated as a fixed factor and maintained around the water saturation. However, organisms inhabiting aquatic environments experience ample diel oxygen fluctuations and are adapted to endure or respond to the experienced variation (Blewett et al., 2022; Morash et al., 2018). Oxygen may be systematically depleted at night and fully recovered during the day, changes that certainly have consequences for the tolerance to acute stressors such as hypoxia and warming. Depending on the intensity of the photosynthetic activity, dissolved oxygen concentrations can reach levels far above the physical saturation (Giomi et al., 2019; McArley et al., 2020). Under such hyperoxic conditions, aquatic organisms experience oxygen availability well beyond their physiological needs, but the effects on their physiology and life history are largely unknown.
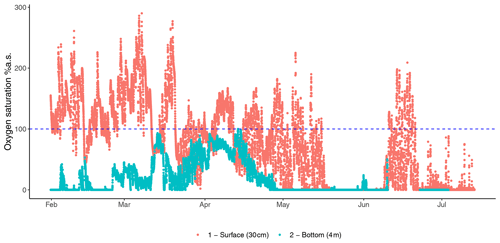
Figure 2Fluctuations in oxygen saturation measured at the bottom (4 m) and surface (30 cm) oxygen saturation of the Rove canal in France highlight the contrast between the two depths of the eutrophicated coastal environment from the winter to summer.
Hyperoxia benefits the metabolic performance of fishes and invertebrates, especially under acute warming that may be very intense in tidal ponds or shallow coastal habitats (Fusi et al., 2021; Booth et al., 2021; Giomi et al., 2019; McArley et al., 2020; Booth et al., 2023a). It extends the upper thermal tolerance of aquatic animals during acute events of daily warming and their survival of anomalous heat waves (McArley et al., 2022). Within the current trend of climate change, the beneficial effect of hyperoxia against extreme heating events can have underestimated positive results on the survival of the animals. For instance, an important consequence of exposure to the hyperoxic conditions experienced during the daytime under high heating regimes is the enhanced capability to extract oxygen from the poorly oxygenated water during the night and endure hypoxic or even anoxic periods (Giomi et al., 2019; Booth et al., 2021).
Oxygen fluctuations are also appreciated as a factor contributing to the structuring of organismal networks and to the synchronisation of life history dynamics such as spawning or larval recruitment (Garzke et al., 2019; Viaroli and Christian, 2004).
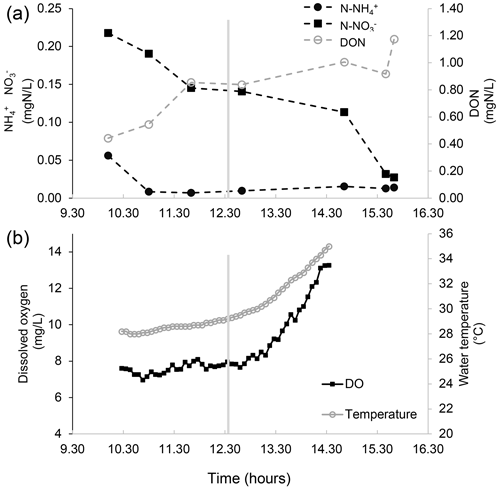
Figure 3Nutrient concentration, dissolved oxygen, and water temperature measurements in the water of a salt marsh of the northern Venice Lagoon (Italy) on 13 August 2015. (a) The concentration of different nitrogen forms in the marsh water (notice the two different vertical axes) over time in the middle of the day. Measurements are made in the water entering the marsh (flood phase, left of the vertical grey line, which indicates the tidal peak) and in the water exiting from the salt marsh (ebb phase, right of the vertical grey line). After some time spent in the marsh, which is flooded by tides twice per day, water becomes depleted in inorganic nutrients, presumably due to the uptake by primary producers, exchanges with the sediments, and (in the case of ammonium) nitrification. Concurrently, water is enriched with dissolved organic nitrogen related to the intense biological activity within the marsh. (b) Dissolved oxygen and the temperature of the water entering the marsh (flood phase, left of the vertical grey line, which indicates the tidal peak) and the water exiting from the salt marsh (ebb phase, right of the vertical grey line) over time in the middle of the day. Notably, in the biologically productive habitat of the marsh, the increase in dissolved oxygen in the water is paralleled by an increase in water temperature.
3.1 Biogeochemical cycles in the pelagic compartment in relation to oxygen fluctuation at the daily scale
In productive coastal ecosystems, the biogeochemical cycles of key chemical elements are strongly interconnected with oxygen dynamics. Nitrogen and phosphorus are typically key elements which limit primary production in shallow coastal marine ecosystems, whose emissions from river basins (or other sources, such as previously enriched sediments) can lead to eutrophication with detrimental effects on biodiversity and human welfare (Palmeri et al., 2013). The biogeochemical cycles of nitrogen and phosphorus are composed of multiple physical, chemical, and biological processes, of which some are accelerated by oxygen availability, such as nitrification and mineralisation, while others are enhanced by its absence, such as denitrification and P release from sediments. The regular daily alternation in contrasting conditions of below- and above-saturation dissolved oxygen concentrations in the water column, particularly in the euphotic zone, promotes changes in the relative abundance of different nutrient forms (Fig. 3). This becomes clear when looking, for example, at the nitrogen cycle in the water column and its complex relationship with oxygen availability: the presence of dissolved oxygen speeds up the mineralisation of organic nitrogen to ammonium as well as nitrification, i.e. the microbial oxidation of ammonium into nitrites and then nitrates. The absence of oxygen makes denitrification possible; i.e. the reduction of nitrates into inert gaseous nitrogen is promoted by facultative aerobic bacteria that, in the absence of anoxia, will prefer oxygen to nitrates as an electron acceptor (Palmeri et al., 2013). Ammonium, nitrites, and nitrates are important nitrogen forms that are bioavailable to primary producers, and the oscillating oxygen conditions in the water column at the diel scales can promote their removal via the nitrification–denitrification chain. High oxygen concentrations are also associated with high photosynthetic activity (e.g. planktonic) and, therefore, with the fast removal of dissolved inorganic nutrients from water through uptake by primary producers (Caron, 1994). The picture is made more complex by the typically non-linear dependence of biogeochemical reactions on oxygen concentration (e.g. nitrification and denitrification) and on temperature (in the case of most biogeochemical processes), which can both change enormously throughout the day, especially in shallow productive ecosystems, altering reaction rates at sub-daily scales in a way which cannot be appreciated if only the daily mean in dissolved oxygen is considered, but which needs to be quantified if we are to predict future biogeochemical cycling mechanistically under climate change (Caballero-Alfonso et al., 2015). A further source of complexity is given by the feedback between abiotic and biotic components of pelagic ecosystems. The growth of primary producers, both microscopic and macroscopic, is on the one hand affected by the presence of bioavailable nutrient forms, and on the other hand their biomass can reduce light availability by increasing shading or turbidity and limiting light penetration and, so, oxygen production and warming in deeper waters. These examples highlight the importance of higher-frequency measurements of oxygen fluctuations; nutrient concentrations; and microbial community abundance, composition, and activity in the water column at sub-daily scales to get a better, more quantitative grasp of biogeochemical cycling in coastal water bodies (Meire et al., 2013). A general framework to assess the short-term (hourly) oxygen fluctuations in relation to the movement and related physical properties of water masses, i.e. of processes such as stratification, residence time, and thermohaline flows, in addition to other processes (photosynthesis, respiration, solubility changes, etc.) is important to future oxygen modelling.
3.2 Biogeochemical cycles in the benthic compartment in relation to oxygen fluctuation at the daily scale
The intertwined oxygen and nutrient dynamics in the water column cannot be understood, at least in relatively shallow aquatic ecosystems, without looking at the interactions between the pelagic and the benthic compartment. This takes place through the vertical transport of matter (settling, resuspension, convection, upwelling currents, etc.) and is also affected by stratification, gradient-driven diffusive fluxes, and organism movements and vertical migrations.
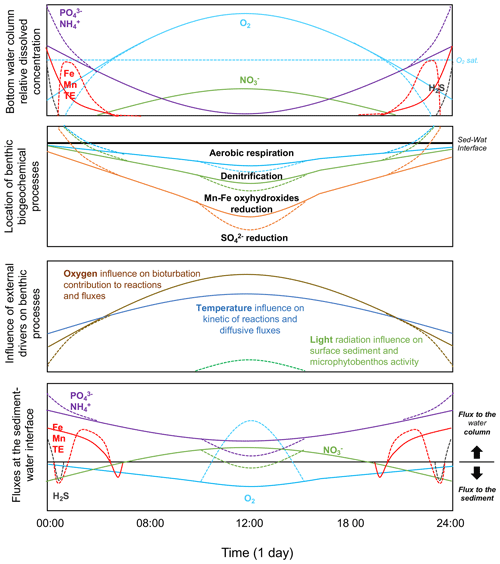
Figure 4Scheme of the benthic biogeochemical cycle in relation to diel oxygen fluctuation. Relative evolution of the bottom water chemical composition and evolution of the location of the most important biogeochemical processes in the benthic compartment. Relative influence of external drivers on benthic processes. Relative evolution of resulting flux direction and intensity. Note that the graphs are not drawn to scale. Although concentrations of and and of Mn and Fe may behave differently, they are represented as similar in this figure for simplification.
When temperature and oxygen concentration in the water column fluctuate at short daily timescales, yet with a very large amplitude (from anoxia/hypoxia to hyperoxia), the impact on biogeochemical cycles and chemical transfers is mostly unknown. Based on the trends observed at seasonal “steady-state” conditions, a conceptual model on the location of the major benthic processes in the sediment and the resulting fluxes at the sediment–water interface within the diurnal temporal scale can be drawn (Fig. 4).
As oxygen penetration depth and concentration in sediment are known to evolve rapidly in response to the oxygen concentration in the overlying water (Glud, 2008), the importance of the aerobic process in surface sediment is also expected to fluctuate at the diurnal timescale, with a higher contribution during the day than during the night. The fast kinetics of the reductive dissolution/oxidative precipitation of Mn and Fe oxyhydroxides and the redox cycle of S chemical species (i.e. H2S/) suggests that those chemical species should evolve with a similar trend over daily timescales, with a reasonable short-term (minutes–hours) delay (Rigaud et al., 2018). Consequently, we expect a vertical fluctuation of those biogeochemical redox processes in the sediment and related fluxes under diel oxygen fluctuation (Fig. 4). The low oxygen concentration at night should induce a lower oxygen penetration depth and an upward distribution of , Mn and Fe oxyhydroxides, and reductions. If the oxygen concentration is low enough, the reductive dissolution of Mn and Fe oxyhydroxides may occur directly at the sediment–water interface, inducing their release into the water column with other chemical species associated with these phases (i.e. and most trace elements). In the specific case of anoxia, the sulfate-reduction process may occur at the sediment surface, releasing H2S into the water column. It is expected that in such a condition, the release of dissolved metals (Fe, Mn, and trace elements), which also present a rapid kinetic for metal sulfide formation in the presence of S(−II), can be reduced and likely reverted (Fig. 4). In contrast, the increase in oxygen concentration during the day promotes oxygen penetration into the sediment and the oxidation of reduced species accumulated during the night, such as dissolved Mn, Fe, , and eventually H2S, preventing their release from the sediment. The reconstitution of the Mn/Fe oxyhydroxide reservoir in surface sediment favours the trapping of and trace elements associated with Fe and Mn cycles. The oxidation of induces the formation of and that may be released from the sediment.
In shallow coastal areas, the light radiation may also reach the sediment surface, allowing the photosynthetic activity of the microphytobenthos to occur in surface sediment (Fig. 4). The local source of oxygen at the sediment surface occurs because photosynthesis strongly enhances the biogeochemical processes described above (Denis et al., 2012; Rigaud et al., 2018). The oxygen released into the water column enhances the water hyperoxia and consumes nutrients and CO2 during photosynthesis. This creates a very peculiar situation that modifies the direction and intensity of chemical fluxes at the sediment–water interface and the chemical composition of water and surface sediment in coastal areas at the diurnal scale. For those rapid redox-sensitive chemical species, processes and fluxes are thus expected to evolve, between such extreme night–day fluctuations, with a transient response related to the reaction kinetics and transport within the sediment surface.
For organic matter (OM) mineralisation processes, the effects of short-term oxygen fluctuation are more challenging to predict. It will also be dependent on the capacity of heterotrophs to react/adapt to the changing oxygen concentrations and eventually shift between different metabolic processes (for microorganisms where variable metabolic pathways coexist) or to activate/cease the process in relation to physicochemical conditions and oxidant availability (for microorganisms with specialised metabolisms). Consequently, the dynamic response of the microbial community to short-term oxygen and chemical fluctuations needs to be investigated in more detail in relation to microbial communities' resilience and adaptation capacity (see Sect. 5).
In productive coastal areas, the benthic organisms, including macro- and meiofaunal species, adapt to wide and rapid daily oxygen variation by modifying their behaviour. The bioturbating activity may be favoured during conditions of high oxygen concentration and lowered during conditions of low oxygen availability. We expect that during the night when oxygen is deficient, the benthic organism activity is reduced, inducing a decrease in the sediment reworking intensity. This is accompanied by a reduction in the biologically mediated solute fluxes at the sediment–water interface and oxygen penetration depth in the sediment. In contrast, during oxygen supersaturation occurring in the day, bioturbation is intensified and may induce a short-term change in the contribution of biologically mediated reactions and transfers. Here too, a delay between the oxygen fluctuation in the water column and the biogeochemical response of the sediment, related to the biological activity, would depend on site characteristics (oxygen concentration, a range of fluctuation, bioturbating species). For instance, in areas where anoxia events are recurrent, the absence of macrofauna prevents bioturbation and its influence on the benthic biogeochemistry (Nilsson and Rosenberg, 1997).
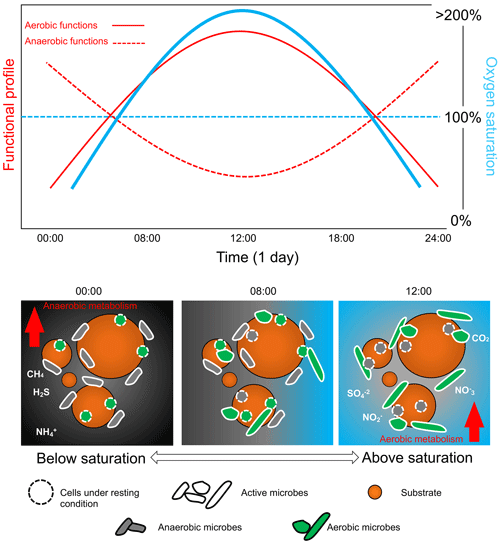
Figure 5Daily bacterial community cycle scheme in relation to diel oxygen fluctuation. Relative shift in the microbial community controlled by the oxygen availability that controls the ratio between aerobic and anaerobic taxa and their relative function. Oxygen fluctuation is important in order to determine the diel cycling of the bacterial communities that, in turn, affect the biogeochemistry and the overall functioning of the aquatic ecosystems. Note that the graphs are not drawn to scale.
In response to a large amplitude and diel oxygen fluctuations, the sediment's biogeochemical cycles are also expected to fluctuate (Fig. 4). However, as the resulting benthic biogeochemistry cycles are associated with the dynamics of three interdependent compartments (i.e. geochemical composition, microbial communities, bioturbating-faunal communities), each presenting its kinetics and responses to oxygen fluctuations, we expect the sediment to be permanently maintained under unsteady-state conditions. This makes it difficult to quantitatively predict the resulting OM mineralisation rates, the proportion of involved processes, and the chemical transfers at the sediment–water interface.
The recent development of tools which can measure the chemical composition and sediment–water fluxes over the short-term scale (i.e. microsensors, eddy covariance technic, gradient-flux method), in addition to benthic microbial and macrofaunal activity (e.g. improved sediment profile imaging), may be beneficial to producing experimental data that will help to fill these gaps in understanding. At the same time, specific efforts should be focused on assessing the isolated response to those three different compartments. Those data should then be implemented to appropriately calibrate non-steady-state coupled pelagic–benthic biogeochemical models, which can predict the resulting biogeochemical functioning of productive coastal ecosystems and, thus, predict their fate under climatic changes.
Oxygen is a two-faced element that acts as the terminal electron acceptor in aerobic respiration (by far the most efficient energy metabolism) and as an element of toxicity because the reduction in O2 molecules partly results in reactive oxygen species. For this reason, oxygen remains a strong evolutionary force dominating functional interactions and the spatial structure of many microbial communities (Fenchel and Finlay, 2008). Oxygen availability determines microbial metabolism, and many studies have been carried out to elucidate the different kinds of communities and metabolisms occurring under anaerobic or aerobic conditions (Sandrin et al., 2009). Marine microorganisms in productive coastal environments also experience wide oxygen diel fluctuations, especially at the boundary layer between sediment/primary producers and water (Pacherres et al., 2022).
Assessing the full environmental oxygen variability in aquatic habitats using high-resolution temporal and spatial scales relevant to microorganisms can reveal in detail the complexity of the patterns of community dynamics and diversity in such oxygen-variable environments (Berg et al., 2022). For example, oxygen largely varies during the day in tropical clear shallow waters because of the photosynthetic activity of seagrasses, corals, and mangroves (Giomi et al., 2019). Therefore, the microbial communities in these ecosystems can experience environmental conditions ranging from hyperoxia to anoxia (see the oxygen profile in Fig. 2). These fluctuations imply that the microbial communities have to shift their composition and function according to the oxygen availability (Fenchel and Finlay, 2008; Booth et al., 2023b, 2019). Microorganisms generally have a short generation time (and high turnover) that favour timely adaptation to new conditions in a changing environment (Steiner et al., 2019). During low oxygen availability at nighttime, bacterial communities will shift their composition and function toward anaerobic function (Fig. 5). End products of anaerobic metabolisms such as ammonium, sulfur, and methane are consumed when oxygen becomes available again during the daylight and photosynthesis recovery, re-establishing aerobic respiration as the central metabolism of the microbial communities (Fenchel and Finlay, 2008). For example, in the Bohai Sea (China), under an oxygen concentration lower than 4.2 mg L−1, the microbial communities were dominated by bacteria belonging to the group of the Anaerolineaceae (Guo et al., 2022). In Chilean coast ecosystems, the composition of the bacterial communities is regulated by the seasonality of the upwelling waters, with the partitioning of community composition driven by dissolved oxygen. Bacteroidetes, SAR11, SAR86, and Alphaproteobacteria dominated in waters containing dissolved oxygen concentrations higher than 70 µM. In contrast, taxonomic groups such as Arctic96BD-19, SUP05, SAR324, and Desulfobacterales were observed at dissolved oxygen concentrations below 70 µM to undetectable levels (Aldunate et al., 2018). The continuous variation in oxygen concentration enhances the diel cycling of nutrients in productive coastal environments, highlighting the importance of assessing the ecologically relevant oxygen fluctuations in order to determine the effective microbial functionality of the marine coastal ecosystem (Trowbridge et al., 2017).
Incorporating methods to assess the ecologically relevant variability in oxygen availability, intensity, and synchronicity in future experimental designs will generate a more accurate prediction of the response of bacterial communities, and therefore their potential for biogeochemical cycling, to climate change, better informing the development of management strategies to mitigate detrimental stressor impacts on ecosystems effectively. The current challenge in microbial ecology is to understand the widespread temporal and spatial environmental oxygen variability and to switch from the “mean condition paradigm” often used for investigations of microbial ecological processes to a dynamic model able to capture the microbial changing and functional potential under oxygen-fluctuating conditions (Fusi et al., 2022).
Oxygen production is considered an ecosystem service (https://cices.eu/, last access: 2 June 2023) because of the benefits humans receive, but, to date, there has been limited inclusion of the impacts of deoxygenation or oxygen fluctuation in marine environmental policies. There are only a few areas whose oxygen dynamics have been extensively monitored, like North America (i.e. Chesapeake Bay, Gulf of Mexico, Long Island Sound), and have been included in management plans for environmental protections (e.g. https://coastalscience.noaa.gov/project/operational-gulf-of-mexico-hypoxia-monitoring/, last access: 2 June 2023). However, globally, there has been a lack of oxygen dynamics' integration into the computation of biodiversity indicators that assess aquatic communities (Breitburg et al., 2019; Chen et al., 2022), and in particular the oxygen dynamic at a daily scale remains largely neglected in marine assessment and management. Policies on pollution or nutrient control have had a positive effect on the oxygen level in ocean water; however, there is still a clear missing link on specific policies to monitor and manage daily oxygen variability. While attention has been given to ocean warming and acidification, daily oxygen dynamics have been overlooked in assessing marine habitat or species' sensitivity to climate changes and anthropogenic disturbances. Including ecologically relevant variations in oxygen availability into aquatic biodiversity indicators, in addition to continuous and high-temporal-resolution oxygen and chemical concentration measurements in the sediment and water column, offers a great opportunity to refine and produce more robust predictors that will be able to better disentangle the response of aquatic ecosystems to climate change and anthropogenic disturbances (Dafforn et al., 2012).
Current hypoxic thresholds for aquatic ecosystem management are generally calculated on averages and minimum oxygen concentrations in water with the risk of overlooking the effect of the oxygen fluctuation, which is more relevant for communities' physiology (Tomasetti and Gobler, 2020). Enhancing monitoring programmes by capturing the daily oxygen fluctuation will allow for the detection of when nighttime low-oxygen content is followed by a daytime recovery period, often reaching oxygen supersaturation. This alternation allows the aquatic communities to sustain their homeostasis in a fluctuating environment. Therefore, proper monitoring can inform correct management to conserve, protect, and restore coastal water mosaic patterns of primary producers to ensure the ecologically relevant fluctuations of dissolved oxygen.
In this paper, based on recent research, we provide evidence that fluctuating oxygen creates a spatial and temporal heterogeneous aquatic oxygen landscape that we define here as oxyscape. The oxyscape – having an important role in aquatic animal physiology and adaptation as well as the ecosystem biogeochemistry – should be considered in the modelling and managing policies of aquatic ecosystems in order to capture the ecologically relevant oxygen fluctuation. Understanding the oxyscape can help us to reconsider the dynamics of many productive aquatic ecosystems, and we, therefore, call on ecologists to rethink their models and experiments in light of this. This would increase the understanding of variations in and resilience of communities to changes in environmental conditions at the scales of space and time relevant to individual components.
All the data are available as supplementary material.
The supplement related to this article is available online at: https://doi.org/10.5194/bg-20-3509-2023-supplement.
MF and FG conceived the study and wrote Sects. 1 to 3. AB, GG, SR, and FG wrote Sect. 4.1 and 4.2 and provided data for the Mediterranean sites. MF, RM, and DD wrote Sect. 5 and provided data for the Red Sea. MF, LP, and CVH wrote Sect. 6. All the authors discussed and reviewed the final version of the paper.
The contact author has declared that none of the authors has any competing interests.
Publisher's note: Copernicus Publications remains neutral with regard to jurisdictional claims in published maps and institutional affiliations.
This article is part of the special issue “Low-oxygen environments and deoxygenation in open and coastal marine waters”. It is a result of the 53rd International Colloquium on Ocean Dynamics (3rd GO2NE Oxygen Conference), Liége, Belgium, 16–20 May 2022.
We are very grateful to the LabEx DRIIHM French programme Investissements d'Avenir (ANR-11-LABX-0010), which is managed by the French ANR, under the Human-Environment Observatory Mediterranean coast (OHM Littoral Meíditerraneíen), for its funding that allowed us to collect data from the Prevost lagoon. We also thank the Excellence Initiative of Aix-Marseille University – A*MIDEX, a French Investissements d'Avenir programme (reference number ANR-11-IDEX-0001-02); the PACA region and the CNRS-INSU-EC2CO programme (PREDHYP-O2 programme) for its funding that allowed us to collect data from the Berre lagoon; and the Occitanie region and the University of Nîmes for the funding of Julie Reígis' PhD thesis. We also thank all the members who allowed us to collect data from the coastal Mediterranean lagoons in France (syndicat mixte GIPREB, Mairie de Chateauneuf-les-Martigues, Compagnons de Maguelone). The PREDHYPO programme has received funding from the Excellence Initiative of Aix-Marseille University – A*MIDEX, a French Investissements d'Avenir programme (reference number ANR-11-IDEX-0001-02), the PACA region, and the CNRS-INSU-EC2CO programme (PREDHYP-O2 programme).
This research has been supported by the King Abdullah University of Science and Technology (grant nos. REI/1/4483-01-01 and CRG-7-3739). Daniele Daffonchio was financially supported by the King Abdullah University of Science and Technology (KAUST) through the Circular Carbon Economy initiative (grant no. REI/1/4483-01-01). Daniele Daffonchio was also supported by a Competitive Research Grant for “the role of the bacterial symbiome at the gill–water (air) interface in the evolution toward territorialisation (microlanding)” (no. CRG-7-3739; 1 April 2019 to 31 March 2022).
This paper was edited by Kenneth Rose and reviewed by two anonymous referees.
Aldunate, M., De la Iglesia, R., Bertagnolli, A. D., and Ulloa, O.: Oxygen modulates bacterial community composition in the coastal upwelling waters off central Chile, Deep-Res. Pt. II, 156, 68–79, https://doi.org/10.1016/j.dsr2.2018.02.001, 2018.
Andersen, M. R., Kragh, T., and Sand-Jensen, K.: Extreme diel dissolved oxygen and carbon cycles in shallow vegetated lakes, P. Roy. Soc. B-Biol. Sci., 284, 20171427, https://doi.org/10.1098/rspb.2017.1427, 2017.
Antão, L. H., Bates, A. E., Blowes, S. A., Waldock, C., Supp, S. R., Magurran, A. E., Dornelas, M., and Schipper, A. M.: Temperature-related biodiversity change across temperate marine and terrestrial systems, Nat. Ecol. Evol., 4, 927–933, https://doi.org/10.1038/s41559-020-1185-7, 2020.
Bates, A. E., Helmuth, B., Burrows, M. T., Duncan, M. I., Garrabou, J., Guy-Haim, T., Lima, F., Queiros, A. M., Seabra, R., Marsh, R., Belmaker, J., Bensoussan, N., Dong, Y., Mazaris, A. D., Smale, D., Wahl, M., and Rilov, G.: Biologists ignore ocean weather at their peril, Nature, 560, 299–301, 2018.
Bennett, J. M., Sunday, J., Calosi, P., Villalobos, F., Martínez, B., Molina-Venegas, R., Araújo, M. B., Algar, A. C., Clusella-Trullas, S., Hawkins, B. A., Keith, S. A., Kühn, I., Rahbek, C., Rodríguez, L., Singer, A., Morales-Castilla, I., and Olalla-Tárraga, M. Á.: The evolution of critical thermal limits of life on Earth, Nat. Commun., 12, 1–9, https://doi.org/10.1038/s41467-021-21263-8, 2021.
Benoiston, A. S., Ibarbalz, F. M., Bittner, L., Guidi, L., Jahn, O., Dutkiewicz, S., and Bowler, C.: The evolution of diatoms and their biogeochemical functions, Philos. T. R. Soc. B, 372, https://doi.org/10.1098/rstb.2016.0397, 2017.
Berg, P., Huettel, M., Glud, R. N., Reimers, C. E., and Attard, K. M.: Aquatic Eddy Covariance: The Method and Its Contributions to Defining Oxygen and Carbon Fluxes in Marine Environments, Annu. Rev. Mar. Sci., 14, 431–455, https://doi.org/10.1146/annurev-marine-042121-012329, 2022.
Bernhardt, J. R., O'Connor, M. I., Sunday, J. M., and Gonzalez, A.: Life in fluctuating environments, Philos. T. Roy. Soc. B., 375, 20190454, https://doi.org/10.1098/rstb.2019.0454, 2020.
Bickler, P. E. and Buck, L. T.: Hypoxia tolerance in reptiles, amphibians, and fishes: Life with variable oxygen availability, Annu. Rev. Physiol., 69, 145–170, https://doi.org/10.1146/annurev.physiol.69.031905.162529, 2007.
Bitter, M. C., Wong, J. M., Dam, H. G., Donelan, S. C., Kenkel, C. D., Komoroske, L. M., Nickols, K. J., Rivest, E. B., Salinas, S., Burgess, S. C., and Lotterhos, K. E.: Fluctuating selection and global change: A synthesis and review on disentangling the roles of climate amplitude, predictability and novelty, P. Roy. Soc. B-Biol. Sci, 288, https://doi.org/10.1098/rspb.2021.0727, 2021.
Blewett, T. A., Binning, S. A., Weinrauch, A. M., Ivy, C. M., Rossi, G. S., Borowiec, B. G., Lau, G. Y., Overduin, S. L., Aragao, I., and Norin, T.: Physiological and behavioural strategies of aquatic animals living in fluctuating environments, J. Exp. Biol., 225, jeb242503, https://doi.org/10.1242/jeb.242503, 2022.
Booth, J., Fusi, M., Giomi, F., Chapman, E., Diele, K., and McQuaid, C.: Diel oxygen fluctuation drives the thermal response and metabolic performance of coastal marine ectotherms, P. Roy. Soc. B-Biol. Sci, 288, 20211141, 2021.
Booth, J. M., Fusi, M., Marasco, R., Mbobo, T., and Daffonchio, D.: Fiddler crab bioturbation determines consistent changes in bacterial communities across contrasting environmental conditions, Sci. Rep.-UK, 9, 1–14, https://doi.org/10.1038/s41598-019-40315-0, 2019.
Booth, J. M., Giomi, F., Daffonchio, D., Mcquaid, C. D., and Fusi, M.: Disturbance of primary producer communities disrupts the thermal limits of the associated aquatic fauna, Sci. Total Environ., 872, 162135, https://doi.org/10.1016/j.scitotenv.2023.162135, 2023a.
Booth, J. M., Fusi, M., Marasco, R., and Daffonchio, D.: The microbial landscape in bioturbated mangrove sediment: A resource for promoting nature-based solutions for mangroves, Microb. Biotechnol., in press, https://doi.org/10.1111/1751-7915.14273, 2023b.
Breen, P. A. and Mann, K. H.: Changing lobster abundance and the destruction of kelp beds by sea urchins, Mar. Biol., 34, 137–142, https://doi.org/10.1007/BF00390755, 1976.
Breitburg, D., Grégoire, M., and Isensee, K.: The ocean is losing its breath, UNESCO, Paris, France, 11, 1–43, 2018.
Breitburg, D., Levin, L. A., Oschlies, A., Grégoire, M., Chavez, F. P., Conley, D. J., Garçon, V., Gilbert, D., Gutiérrez, D., Isensee, K., Jacinto, G. S., Limburg, K. E., Montes, I., Naqvi, S. W. A., Pitcher, G. C., Rabalais, N. N., Roman, M. R., Rose, K. A., Seibel, B. A., Telszewski, M., Yasuhara, M., and Zhang, J.: Declining oxygen in the global ocean and coastal waters, Science, 359, eaam7240, https://doi.org/10.1126/science.aam7240, 2018.
Breitburg, D. L., Conley, D., Isensee, K., Levin, L. A., Limburg, K. E., and Williamson, P.: What can we do? Adaptation and solutions to declining ocean oxygen, in: Ocean deoxygenation everyone's Probl. Causes, impacts, consequences Solut., edited by: Laffoley, D. and Baxter, J. M., IUCN, Gland Switzerland, 545–562, 2019.
Caballero-Alfonso, A. M., Carstensen, J., and Conley, D. J.: Biogeochemical and environmental drivers of coastal hypoxia, J. Marine Syst., 141, 190–199, https://doi.org/10.1016/j.jmarsys.2014.04.008, 2015.
Caron, D. A.: Inorganic nutrients, bacteria, and the microbial loop, Microb. Ecol. Ecol., 28, 295–298, https://doi.org/10.1007/BF00166820, 1994.
Challener, R. C., Robbins, L. L., and Mcclintock, J. B.: Variability of the carbonate chemistry in a shallow, seagrass-dominated ecosystem: Implications for ocean acidification experiments, Mar. Freshwater Res., 67, 163–172, https://doi.org/10.1071/MF14219, 2016.
Chapman, D. (Ed.): Water Quality Assessments – A Guide to Use of Biota, Sediments and Water in Environmental Monitoring, Second Edn., E&FN Spon, London, 0419216006, 2021.
Chen, H., Costanza, R., and Kubiszewski, I.: Legitimacy and limitations of valuing the oxygen production of ecosystems, Ecosyst. Serv., 58, 101485, https://doi.org/10.1016/j.ecoser.2022.101485, 2022.
Craig, H. and Hayward, T.: Oxygen Supersaturation in the Ocean: Biological Versus Physical Contributons, Science, 735, 1983–1986, 1983.
Dafforn, K. A., Simpson, S. L., Kelaher, B. P., Clark, G. F., Komyakova, V., Wong, C. K. C., and Johnston, E. L.: The challenge of choosing environmental indicators of anthropogenic impacts in estuaries, Environ. Pollut., 163, 207–217, https://doi.org/10.1016/j.envpol.2011.12.029, 2012.
Denis, L., Gevaert, F., and Spilmont, N.: Microphytobenthic production estimated by in situ oxygen microprofiling: Short-term dynamics and carbon budget implications, J. Soils Sediments, 12, 1517–1529, https://doi.org/10.1007/s11368-012-0588-8, 2012.
Diaz, R. J. and Rosenberg, R.: Spreading dead zones and consequences for marine ecosystems, Science, 321, 926–929, https://doi.org/10.1126/science.1156401, 2008.
Duarte, C. M., Losada, I. J., Hendriks, I. E., Mazarrasa, I., and Marbà, N.: The role of coastal plant communities for climate change mitigation and adaptation, Nat. Clim. Change, 3, 961–968, https://doi.org/10.1038/nclimate1970, 2013.
Fenchel, T. and Finlay, B.: Oxygen and the spatial structure of microbial communities, Biol. Rev., 83, 553–569, https://doi.org/10.1111/j.1469-185X.2008.00054.x, 2008.
Fusi, M., Daffonchio, D., Booth, J., and Giomi, F.: Dissolved Oxygen in Heterogeneous Environments Dictates the Metabolic Rate and Thermal Sensitivity of a Tropical Aquatic Crab, Front. Mar. Sci., 8, 1–9, https://doi.org/10.3389/fmars.2021.767471, 2021.
Fusi, M., Marasco, R., Ramond, J., Barausse, A., and Baldanzi, S.: Editorial: Fluctuating Habitats: Ecological Relevance of Environmental Variability and Predictability on Species, Communities, and Ecosystems, Front. Ecol. Evol., 10, 1–4, https://doi.org/10.3389/fevo.2022.907622, 2022.
Garzke, J., Connor, S. J., Sommer, U., and O'connor, M. I.: Trophic interactions modify the temperature dependence of community biomass and ecosystem function, PLOS Biol., 17, 1–27, https://doi.org/10.1371/journal.pbio.2006806, 2019.
Giomi, F., Barausse, A., Duarte, C. M., Booth, J., Agusti, S., Saderne, V., Anton, A., Daffonchio, D., and Fusi, M.: Oxygen supersaturation protects coastal marine fauna from ocean warming, Sci. Adv., 5, 1–8, https://doi.org/10.1126/sciadv.aax1814, 2019.
Glud, R. N.: Oxygen dynamics of marine sediments, Mar. Biol. Res., 4, 243–289, https://doi.org/10.1080/17451000801888726, 2008.
Graham, N. A. J., Wilson, S. K., Carr, P., Hoey, A. S., Jennings, S., and MacNeil, M. A.: Seabirds enhance coral reef productivity and functioning in the absence of invasive rats, Nature, 559, 250–253, https://doi.org/10.1038/s41586-018-0202-3, 2018.
Guo, X., Song, G., Li, Y., Zhao, L., and Wang, J.: Switch of Bacteria Community Under Oxygen Depletion in Sediment of Bohai Sea, Front. Mar. Sci., 9, 1–13, https://doi.org/10.3389/fmars.2022.833513, 2022.
Hochachka, P. W.: Temperature: the ectothermy option, in: Phylogenetic and biochemical perspectives: Biochemistry and molecular biology of fishes, 1, vol. 1, edited by: Hochachka, P. W. and Mommsen, T. P., Elsevier, Amsterdam, https://doi.org/10.1016/B978-0-444-89124-2.50016-6, 313–322, 1991.
Ito, T., Nenes, A., Johnson, M. S., Meskhidze, N., and Deutsch, C.: Acceleration of oxygen decline in the tropical Pacific over the past decades by aerosol pollutants, Nat. Geosci., 9, 443–447, https://doi.org/10.1038/ngeo2717, 2016.
Keeling, R. F., Körtzinger, A., and Gruber, N.: Ocean deoxygenation in a warming world, Annu. Rev. Mar. Sci., 2, 199–229, https://doi.org/10.1146/annurev.marine.010908.163855, 2010.
Kerrison, P., Hall-Spencer, J. M., Suggett, D. J., Hepburn, L. J., and Steinke, M.: Assessment of pH variability at a coastal CO2 vent for ocean acidification studies, Estuar. Coast. Shelf S., 94, 129–137, https://doi.org/10.1016/j.ecss.2011.05.025, 2011.
Kinney, E. H. and Roman, C. T.: Response of primary producers to nutrient enrichment in a shallow estuary, Mar. Ecol. Prog. Ser., 163, 89–98, https://doi.org/10.3354/meps163089, 1998.
Krause-Jensen, D., Marba, N., Sanz-Martin, M., Hendriks, I. E., Thyrring, J., Carstensen, J., Sejr, M. K., and Duarte, C. M.: Long photoperiods sustain high pH in Arctic kelp forests, Sci. Adv., 2, e1501938–e1501938, https://doi.org/10.1126/sciadv.1501938, 2016.
Laffoley, D. and Baxter, J. M.: Explaining Ocean Warming: Causes, scale, effects and consequences, IUCN, Gland Switzerland, 458 pp., https://doi.org/10.2305/IUCN.CH.2016.08.en, 2016.
Laffoley, D. D. A. and Baxter, J. M.: Ocean Deoxygenation: Everyone's Problem-Causes, Impacts, Consequences and Solutions, IUCN, Gland Switzerland, 2019.
Limburg, K. E., Breitburg, D., Swaney, D. P., and Jacinto, G.: Ocean Deoxygenation: A Primer, One Earth, 2, 24–29, https://doi.org/10.1016/j.oneear.2020.01.001, 2020.
Mallin, M. A., Paerl, H. W., Rudek, J., and Bates, P. W.: Regulation of estuarine primary production by watershed rainfall and river flow, Mar. Ecol. Prog. Ser., 93, 199–203, https://doi.org/10.3354/meps093199, 1993.
Manzello, D. P.: Ocean acidification hot spots: Spatiotemporal dynamics of the seawater CO2 system of eastern Pacific coral reefs, Limnol. Oceanogr., 55, 239–248, https://doi.org/10.4319/lo.2010.55.1.0239, 2010.
Marasco, R., Fusi, M., Coscolín, C., Barozzi, A., Almendral, D., Bargiela, R., Gohlke, C., Christopher, P., Dittrich, J., Gohlke, H., Matesanz, R., Sanchez-carrillo, S., Mapelli, F., Chernikova, T. N., Golyshin, P. N., Ferrer, M., and Daffonchio, D.: Enzyme adaptation to habitat thermal legacy microbiomes, Nat. Commun., in press, https://doi.org/10.1038/s41467-023-36610-0, 2023.
McArley, T. J., Sandblom, E., and Herbert, N. A.: Fish and hyperoxia—From cardiorespiratory and biochemical adjustments to aquaculture and ecophysiology implications, Fish Fish., 1–32, https://doi.org/10.1111/faf.12522, 2020.
McArley, T. J., Morgenroth, D., Zena, L. A., Ekström, A. T., and Sandblom, E.: Experimental hyperoxia (O2 supersaturation) reveals a gill diffusion limitation of maximum aerobic performance in fish, Biol. Lett.-UK, 18, 20220401, https://doi.org/10.1098/rsbl.2022.0401, 2022.
Meire, L., Soetaert, K. E. R., and Meysman, F. J. R.: Impact of global change on coastal oxygen dynamics and risk of hypoxia, Biogeosciences, 10, 2633–2653, https://doi.org/10.5194/bg-10-2633-2013, 2013.
Morash, A. J., Neufeld, C., MacCormack, T. J., and Currie, S: The importance of incorporating natural thermal variation when evaluating physiological performance in wild species, J. Exp. Biol., 221, 1–10, https://doi.org/10.1242/JEB.164673, 2018.
Nilsson, H. C. and Rosenberg, R.: Benthic habitat quality assessment of an oxygen stressed fjord by surface and sediment profile images, J. Marine Syst., 11, 249–264, https://doi.org/10.1016/S0924-7963(96)00111-X, 1997.
Pacherres, C. O., Ahmerkamp, S., Koren, K., Richter, C., and Holtappels, M.: Ciliary flows in corals ventilate target areas of high photosynthetic oxygen production, Curr. Biol., 32, 4150–4158, https://doi.org/10.1016/j.cub.2022.07.071, 2022.
Palmeri, L., Barausse, A., and Jorgensen, S. E. (Eds.): Ecological processes handbook, CRC Press, Boca Raton FL, ISBN 9780429097997, 375 pp., 2013.
Pörtner, H.-O.: Oxygen- and capacity-limitation of thermal tolerance: a matrix for integrating climate-related stressor effects in marine ecosystems., J. Exp. Biol., 213, 881–893, 2010.
Pörtner, H.-O., Scholes, R. J., Arneth, A., Barnes, D. K. A., Burrows, M. T., Diamond, S. E., Duarte, C. M., Kiessling, W., Leadley, P., Managi, S., McElwee, P., Midgley, G., Ngo, H. T., Obura, D., Pascual, U., Sankaran, M., Shin, Y. J., and Val, A. L.: Overcoming the coupled climate and biodiversity crises and their societal impacts, Science, 380, eabl4881, https://doi.org/10.1126/science.abl4881, 2023.
Power, L. D. and Cardinale, B. J.: Species richness enhances both algal biomass and rates of oxygen production in aquatic microcosms, Oikos, 118, 1703–1711, https://doi.org/10.1111/j.1600-0706.2009.17585.x, 2009.
Rigaud, S., Deflandre, B., Maire, O., Bernard, G., Duchêne, J. C., Poirier, D., and Anschutz, P.: Transient biogeochemistry in intertidal sediments: New insights from tidal pools in Zostera noltei meadows of Arcachon Bay (France), Mar. Chem., 200, 1–13, https://doi.org/10.1016/j.marchem.2018.02.002, 2018.
Riser, S. C. and Johnson, K. S.: Net production of oxygen in the subtropical ocean, Nature, 451, 323–325, https://doi.org/10.1038/nature06441, 2008.
Sandrin, T. R., Dowd, S. E., Herman, D. C., and Maier, R. M.: Aquatic environments, in: Environment Microbiology, edited by: Maier, R. M., Pepper, I. L., and Gerba, C. P., Elsevier, 103–122, 2009.
Schmidt, S., Diallo, I. I., Derriennic, H., Fallou, H., and Lepage, M.: Exploring the susceptibility of turbid estuaries to hypoxia as a prerequisite to designing a pertinent monitoring strategy of dissolved oxygen, Front. Mar. Sci., 6, 1–8, https://doi.org/10.3389/fmars.2019.00352, 2019.
Schmidtko, S., Stramma, L., and Visbeck, M.: Decline in global oceanic oxygen content during the past five decades, Nature, 542, 335–339, https://doi.org/10.1038/nature21399, 2017.
Shaw, E. C., Munday, P. L., and McNeil, B. I.: The role of CO2 variability and exposure time for biological impacts of ocean acidification, Geophys. Res. Lett., 40, 4685–4688, https://doi.org/10.1002/grl.50883, 2013.
Spietz, R. L., Williams, C. M., Rocap, G., and Horner-Devine, M. C.: A dissolved oxygen threshold for shifts in bacterial community structure in a seasonally hypoxic estuary, PLOS ONE, 10, 1–18, https://doi.org/10.1371/journal.pone.0135731, 2015.
Steiner, P. A., De Corte, D., Geijo, J., Mena, C., Yokokawa, T., Rattei, T., Herndl, G. J., and Sintes, E.: Highly variable mRNA half-life time within marine bacterial taxa and functional genes, Environ. Microbiol., 21, 3873–3884, https://doi.org/10.1111/1462-2920.14737, 2019.
Stillman, J. H. and Somero, G. N.: A comparative analysis of the upper thermal tolerance limits of eastern Pacific porcelain crabs, genus Petrolisthes: influences of latitude, vertical zonation, acclimation, and phylogeny, Physiol. Biochem. Zool., 73, 200–208, https://doi.org/10.1086/316738, 2000.
Stramma, L., Schmidtko, S., Levin, L. A., and Johnson, G. C.: Ocean oxygen minima expansions and their biological impacts, Deep-Sea Res. Pt. I, 57, 587–595, https://doi.org/10.1016/j.dsr.2010.01.005, 2010.
Talke, S. A., De Swart, H. E., and De Jonge, V. N.: An idealized model and systematic process study of oxygen depletion in highly turbid estuaries, Estuar. Coast., 32, 602–620, https://doi.org/10.1007/s12237-009-9171-y, 2009.
Tomasetti, S. J. and Gobler, C. J.: Dissolved oxygen and pH criteria leave fisheries at risk, Science, 368, 372–373, https://doi.org/10.1126/science.aba4896, 2020.
Trowbridge, C. D., Davenport, J., Cottrell, D. M., Harman, L., Plowman, C. Q., Little, C., and McAllen, R.: Extreme oxygen dynamics in shallow water of a fully marine Irish sea lough, Reg. Stud. Mar. Sci., 11, 9–16, https://doi.org/10.1016/j.rsma.2017.01.008, 2017.
Vargas, C. A., Lagos, N. A., Lardies, M. A., Duarte, C., Manríquez, P. H., Aguilera, V. M., Broitman, B., Widdicombe, S., and Dupont, S.: Species-specific responses to ocean acidification should account for local adaptation and adaptive plasticity, Nat. Ecol. Evol., 1, 1–7, https://doi.org/10.1038/s41559-017-0084, 2017.
Vargas, C. A., Cuevas, L. A., Broitman, B. R., San Martin, V. A., Lagos, N. A., Gaitán-Espitia, J. D., and Dupont, S.: Upper environmental pCO2 drives sensitivity to ocean acidification in marine invertebrates, Nat. Clim. Change, 12, 200–207, https://doi.org/10.1038/s41558-021-01269-2, 2022.
Viaroli, P. and Christian, R. R.: Description of trophic status, hyperautotrophy and dystrophy of a coastal lagoon through a potential oxygen production and consumption index – TOSI: Trophic Oxygen Status Index, Ecol. Indic., 3, 237–250, https://doi.org/10.1016/j.ecolind.2003.11.001, 2004.
Virtanen, E. A., Norkko, A., Nyström Sandman, A., and Viitasalo, M.: Identifying areas prone to coastal hypoxia – the role of topography, Biogeosciences, 16, 3183–3195, https://doi.org/10.5194/bg-16-3183-2019, 2019.
Winter, G., Hetzel, Y., Huang, P., Hipsey, M. R., Mulligan, R. P., and Hansen, J.: Coastal processes, extreme events and forecasting, in: Marine Extremes, edited by: Techera, E. and Winter, G., London ISBN 9780429491023, 17, 2019.
- Abstract
- Introduction to environmental dissolved oxygen variability and its ecological relevance
- Oxygen trends and stochastic variations
- The role of oxygen fluctuation in coastal biogeochemical cycles
- The interrelationship of oxygen fluctuation and aquatic microbial communities
- Implications for marine assessment and management
- Data availability
- Author contributions
- Competing interests
- Disclaimer
- Special issue statement
- Acknowledgements
- Financial support
- Review statement
- References
- Supplement
oxyscape, and we addressed the importance of considering the oxyscape in the modelling and managing of aquatic ecosystems.
- Abstract
- Introduction to environmental dissolved oxygen variability and its ecological relevance
- Oxygen trends and stochastic variations
- The role of oxygen fluctuation in coastal biogeochemical cycles
- The interrelationship of oxygen fluctuation and aquatic microbial communities
- Implications for marine assessment and management
- Data availability
- Author contributions
- Competing interests
- Disclaimer
- Special issue statement
- Acknowledgements
- Financial support
- Review statement
- References
- Supplement