the Creative Commons Attribution 4.0 License.
the Creative Commons Attribution 4.0 License.
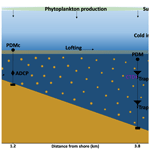
Influence of a small submarine canyon on biogenic matter export flux in the lower St. Lawrence Estuary, eastern Canada
Michel Gosselin
Catherine Lalande
Alexandre Normandeau
Jean-Carlos Montero-Serrano
Khouloud Baccara
Daniel Bourgault
Owen Sherwood
Submarine canyons enhance shelf–slope sediment exchange and influence hydrodynamic processes, with consequences for biogeochemical cycles. This work documents variations in the vertical export of biogenic matter on the northern shore of the lower St. Lawrence Estuary (LSLE, Quebec, eastern Canada), which is characterized by the presence of an active submarine canyon system. A total of three moorings were deployed from November 2020 to September 2021. One nearshore mooring (PDMc) was deployed in the main axis of the Pointe-des-Monts (PDM) canyon system and was equipped with an acoustic Doppler current profiler (ADCP), and two moorings equipped with sediment traps were deployed in the distal PDM canyon system (PDM-154, PDM-224) and offshore Baie-Comeau (BC-133). The ADCP data revealed the occurrence of a minor sediment remobilization event (December 2020) and a small turbidity current (February 2021) in the canyon. Concurrent elevated fluxes of total particulate matter, particulate organic carbon, particulate nitrogen, and chloropigments showed that these events left a signature in sediment traps PDM-154 and PDM-224 located > 2.6 km further offshore by enhancing lateral dispersion of resuspended sediments. The composition of diatom and dinoflagellate assemblages was similar in the canyon system and offshore BC, but the diatom bloom occurred 2 weeks earlier (in mid-April) at the PDM site. A bloom of the potentially toxic diatom Pseudo-nitzschia seriata was also observed during the second half of September 2021 at the BC site. Annual diatom and dinoflagellate fluxes were almost 2 times lower at the PDM site than at the BC site, possibly due to differences in riverine input and the structure of the water column, as well as increased sediment input and resuspension at the PDM site, leading to limited light availability. This study notably helps identify the relationship between near-bed canyon processes and biogenic matter export in the water column, thereby directly influencing the ecosystem offshore PDM. The study period further covered an anomalously nearly ice-free winter, and thus, in the context of climate change, it provides valuable insight into future trends of biogenic matter export in the LSLE.
- Article
(8179 KB) - Full-text XML
- BibTeX
- EndNote
Submarine canyons are erosional features that cut into the seabed of continental margins and slopes. Canyons are preferential particle transport routes from the coast to deep depositional sinks, leading to important sites of sediment accumulation on submarine fans (Covault, 2011). Owing to their bathymetric complexity, large canyon systems are known to significantly influence hydrodynamic processes and induce shelf–slope exchanges of water and matter through turbidity currents, funneling of internal tides and waves, and enhanced downwelling and upwelling currents, thereby playing a key role in biogeochemical cycling of elements and supporting biodiversity hotspots (Fernandez-Arcaya et al., 2017; Paull et al., 2018; Santora et al., 2018, and references therein).
The St. Lawrence Estuary (Quebec, eastern Canada), one of the largest and deepest estuaries in the world, supports several ecosystem services, such as biodiversity, tourism, and fisheries. Located at the transition between the lower St. Lawrence Estuary (LSLE) and the Gulf of St. Lawrence, the region of Pointe-des-Monts (PDM; Fig. 1) hosts three small submarine canyons with lengths up to 3.5 km, widths of 100–300 m, and depths down to 300 m (Normandeau et al., 2015). These canyons are sediment-starved, meaning they have limited sediment input at their heads. Turbidity currents thus remobilize small volumes of sediment stored on the shelf and previously deposited deglacial and post-glacial sediment within the canyons. The processes leading to the formation of the canyons and storm-induced turbidity currents have been the subjects of previous studies (Normandeau et al., 2014, 2015, 2020, 2022). However, despite the presence of the nearby Saguenay–St. Lawrence Marine Park, a National Marine Conservation Area (Fig. 1c), it is currently unknown whether the canyon's sedimentary and hydrodynamic processes influence regional primary production and biogenic matter export. Furthermore, while it is relatively well known that the complex hydrodynamics of large submarine canyons increase the occurrence of biodiversity hotspots (Fernandez-Arcaya et al., 2017; Paull et al., 2018; Santora et al., 2018), the same concept is not established for smaller systems, such as at PDM.
To address this gap, the present study investigates the seasonal and spatial variability in the magnitude and composition of biogenic matter export on the north shore of the LSLE using in situ measurements obtained from November 2020 to September 2021. Two sediment traps were strategically deployed at two depths over the submarine fan at the base of the PDM canyon system to characterize the biogenic matter sinking from the euphotic zone and resuspended from the seafloor (Fig. 1d, e). To identify canyon-specific processes, a sediment trap was also deployed offshore Baie-Comeau (BC), a site not affected by canyon-related sediment remobilization events, to contrast with biogenic matter export in the LSLE (Fig. 1c). At PDM, the time-series biogenic matter fluxes were further compared to data obtained with a down-looking acoustic Doppler current profiler (ADCP) installed in the axis of the main canyon (Fig. 1d, e). The ADCP also recorded data from November 2020 to September 2021 to determine if turbidity currents and other sediment remobilization events within the canyon influenced biogenic matter fluxes and phytoplankton assemblages over the submarine fan located > 2.6 km further offshore. Finally, sinking particles were examined for the presence of potentially harmful species of diatoms and dinoflagellates that may pose a threat to the LSLE ecosystem.
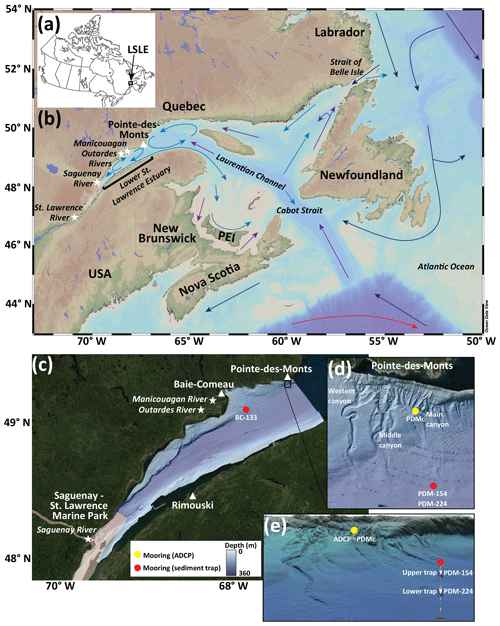
Figure 1(a) Location of the lower St. Lawrence Estuary (LSLE), eastern Canada. (b) Map of eastern Canada highlighting the main patterns of surface (light blue) and deep-water circulation (purple), which is a combination of the Labrador Current (dark blue) and the Gulf Stream (red). Circulation patterns are adapted from Weise et al. (2002), Bernier et al. (2018), and Ryan et al. (2019). White stars represent the outlets of the major freshwater sources to the lower St. Lawrence Estuary and the white triangle highlights Pointe-des-Monts. (c–e) The Lower St. Lawrence Estuary with locations of moorings equipped with sediment traps (red) and an acoustic Doppler current profiler (yellow). The location of the Saguenay–St. Lawrence Marine Park is highlighted in light pink. (d–e) Pointe-des-Monts submarine canyon system.
The LSLE is characterized by a seasonally stratified three-layer water column that exhibits large spatial and temporal variability (Galbraith et al., 2022). The low-salinity surface mixed layer (25 to 32 psu) flows seaward into the Atlantic Ocean, with the main sources of freshwater being the Saguenay, Outardes, and Manicouagan rivers located on the north shore, in addition to the St. Lawrence River (Fig. 1). The cold intermediate layer (−1 to 2 ∘C, 31.5 to 33 psu) predominantly flows landward on the north shore and seaward on the south shore, with an overall net landward transport (Mucci et al., 2011). In the fall and winter, atmospheric cooling and intense vertical mixing, due to wind-driven mixing and brine rejection, cause the surface layer to gradually become cooler and deeper until it merges with the cold intermediate layer (Gilbert and Pettigrew, 1997). The warmer deep layer (2 to 6 ∘C, > 33 psu) is a mixture of waters from the Labrador Current and the North Atlantic entering the Gulf of St. Lawrence via Cabot Strait (Dickie and Trites, 1983). The Labrador Current originates in the Arctic and contributes cold, fresh, oxygen-rich waters, while the North Atlantic waters, originating from the Gulf Stream, are warm, saline, and oxygen-poor waters (Gilbert et al., 2005). The deep layer flows landward through the Laurentian Channel, a 1240 km glaciated trough which extends from the edge of the eastern Canadian continental shelf to the mouth of the Saguenay River, where a sill causes the seabed to rise suddenly and leads to upwelling of bottom waters (Ingram, 1983).
Sea ice typically begins to form along the north shore of the LSLE in December. However, by mid-December 2020, sea surface temperatures were above average, delaying the onset of sea ice formation by several weeks (Fig. 2). Sea ice cover has been declining since 1990, with record low sea ice conditions observed in 2010 followed by 2021, and both years were classified as nearly ice-free (Galbraith et al., 2022). Thus, in the context of climate warming, high-resolution data collection during these anomalous years can provide valuable insight into future trends in the LSLE system
3.1 CTD-rosette and moorings
Water column profiles of temperature, salinity, in vivo chlorophyll fluorescence, and photosynthetically active radiation were obtained on board the research vessel Coriolis II at the BC and PDM mooring sites before mooring deployment on 15 October 2020. Data acquisition was done using a rosette sampler equipped with a conductivity–temperature–depth (CTD) probe (Sea-Bird Electronic SBE 911plus), an in situ fluorometer (Wet Labs ECO-AFL/FL), and a Chelsea scalar quantum irradiance sensor. The euphotic zone was determined based on the depth where the photosynthetically active radiation (PAR) value was 1 % of its surface value. The depths of the surface mixed layer and cold intermediate layer were estimated based on the vertical gradient in temperature and salinity.
A total of three moorings were deployed in October 2020 and retrieved in October 2021. Two moorings were equipped with sediment traps (Technicap PPS 6/2; aspect ratio: 6.25; opening area: 0.5 m2) deployed at 133 m at the Baie-Comeau site (BC-133) and at 154 and 224 m at the Pointe-des-Monts site (PDM-154 and PDM-224; Fig. 1, Table 1). Traps BC-133 and PDM-224 were positioned 67 and 58 m above the seafloor, respectively, above the estimated benthic nepheloid layer in the LSLE (i.e., 250–310 m of water depth; Bourgault et al., 2014; Casse et al., 2019). The sediment traps consisted of a funnel connected to a carousel holding with 24 sample cups (525 mL) programmed to rotate approximately every 2 weeks over an annual cycle with increased resolution in April. Prior to deployment, seawater was sequentially filtered through a 3 µm A/D glass fiber filter (Pall Corporation) and a 0.2 µm GTTP membrane filter to prepare the preservative solution for the sample cups. The cups were then filled with filtered seawater adjusted to a salinity > 33 psu with NaCl, fixed with 37 % formaldehyde (final concentration 5 % w/v formalin), and buffered with borax to preserve samples during deployment and after recovery (Hargrave et al., 2002). The last sample cup of each annual cycle was excluded from the analyses due to a technical error resulting in the cups remaining open upon recovery. After retrieval, all sample cups were kept at 4 ∘C in the dark until biogenic matter analysis and cell identification. CTD and CT sensors attached to traps BC-133 and PDM-154 recorded water temperature and salinity at 15 min intervals from November 2020 to September 2021.
A third mooring was deployed in the axis of the main PDM canyon (PDMc) at 183 m water depth (Fig. 1; Table 1). The PDMc mooring was equipped with a down-looking ADCP deployed at 156 m that recorded velocity, acoustic backscatter, and pressure once every 20 s with a vertical bin size of 1 m. The ADCP recorded all pings ensemble averaging, and the acoustic beams were at a 20∘ angle respective to a vertical line. The ADCP data were analyzed in conjunction with bathymetric data to monitor the occurrence of turbidity currents, smaller sediment remobilization events and hydrodynamic processes. To assess the relation between sediment remobilization and sinking particles, the average acoustic backscatter (dB) and the maximum daily average acoustic backscatter were determined for each corresponding sediment trap sampling period.
3.2 Total particulate matter and geochemical signature
Prior to subsampling for all laboratory analyses, each sample cup was gently mixed for 100 s to ensure homogeneity before subsamples were collected using a pipette. For total particulate matter (TPM) measurements, triplicates of 1 to 3 mL were filtered onto 21 mm Whatman GF/F filters (nominal porosity of 0.7 µm) pre-combusted at 450 ∘C for 5 h and pre-weighed with a Mettler Toledo MX5 Microbalance. The filters were rinsed with 5 mL of Nanopure water to eliminate salts. The filters were dried at 60 ∘C for 36 to 48 h and reweighed with the microbalance. Inorganic carbon was then removed by placing the filters in a desiccator saturated with HCl fumes overnight. For particulate organic carbon (POC) and particulate nitrogen (PN) determination, the filters were pelletized in tin capsules, combustion was performed with an Elementar PYRO Elemental Analyzer, and detection was done with a Thermo Scientific DeltaPlus XP continuous-flow isotope ratio mass spectrometer (ISMER-UQAR). Acetanilide (B2000, Elemental Microanalysis) was used as a calibration standard for the analysis. Instrument blanks (empty tin capsules) were performed during calibration to stabilize and establish a baseline for the instrument. Analytical detection limits were 0.1 mg C and 0.02 mg N for POC and PN, respectively. Subsamples of each sample cup were also analyzed for total organic carbon (TOC) and total nitrogen (TN), allowing for the calculation of TOC:TN mass ratios, as well as the carbon (δ13C) and nitrogen (δ15N) isotopic signatures of the sinking particles. Each subsample was centrifuged at 3000 rpm, decanted, resuspended, and rinsed with distilled water three times. Centrifuge cycles began at 12 min and increased by 4 min intervals with each subsequent rinse. Subsamples were stored overnight at −80 ∘C and subsequently freeze-dried for 2 to 3 d. The particles were re-homogenized using an agate mortar and pestle. Subsamples used for TOC and δ13C were acidified using sequential aliquots of 1 M HCl, administered in scintillation vials, until no reaction was visible. Samples were dried at 60 ∘C before being weighed into tin capsules and loaded into a Costech 4010 elemental analyzer coupled to a Delta V Plus – Conflo IV isotope ratio mass spectrometer (UNB).
3.3 Chloropigments
Chlorophyll a (chl a) is an index of the phytoplankton biomass and can be used as an indicator of the freshness of algal inputs in sediments (Boon and Duineveld, 1996). Pheopigments are chlorophyll degradation products resulting from cell senescence and zooplankton grazing (Mantoura and Llewellyn, 1983; Villanueva and Hastings, 2000). Thus, chloropigments (chl a + pheopigments) are a reliable proxy for surface primary productivity. For chl a and pheopigment measurements, subsamples of 0.25 or 0.5 mL were collected using a pipette and filtered onto 25 mm Whatman GF/F filters (nominal porosity of 0.7 µm). The pigments retained on the filters were extracted in 10 mL of 90 % acetone for 18 to 24 h at 4 ∘C in the dark. The supernatant was then transferred to a borosilicate tube for fluorescence measurements with a Turner Designs 10-AU fluorometer calibrated using chl a extract from Anacystis nidulans (Sigma-Aldrich; ISMER-UQAR). Prior to pheopigment measurements, three drops of 5 % v/v HCl were added to the supernatant (Parsons et al., 1984).
3.4 Phytoplankton
For each sample, an aliquot of 80 to 150 µL was diluted with 10 mL of a preservative solution in a settling chamber and left to settle evenly over a counting chamber for a minimum of 6 h. The preservative solution consisted of 1 L of artificial seawater (30 psu) mixed with 20 mL of 37 % weight/volume (w/v) formaldehyde. Phytoplankton were identified and counted using an inverted microscope (Olympus CKX53; UNB). A survey of the entire counting chamber was first conducted at 100× magnification to identify any large phytoplankton that could be missed during the subsequent three transects at 400× magnification for the phytoplankton enumeration. Taxonomic identifications of diatoms and dinoflagellates were completed following Vinyard (1979), Hasle and Syvertsen (1997), Steidinger and Jangen (1997), Bérard-Therriault et al. (1999), and Round et al. (1990).
Daily fluxes (m−2 d−1) of TPM, POC, PN, chloropigments, diatoms, and dinoflagellates were calculated based on the collecting area of the trap and the sampling period and were subsequently integrated to annual fluxes (m−2 yr−1).
4.1 Structure of the water column
During the deployment of the mooring on 15 October 2020, the euphotic zone comprised the upper 10 m, the surface mixed layer extended down to 12 m, and the cold intermediate layer extended down to 65 m at the BC site (Fig. 3). At the PDM site, the euphotic zone comprised the upper 13 m, which extended below the surface mixed layer at 10 m, and a thicker cold intermediate layer extended down to 108 m. A subsurface fluorescence maximum was observed at 7 and 13.5 m at the BC site and at 5 m with a lower value at the PDM site.
At BC-133, water temperatures were highest (> 5 ∘C) in March and lowest (< 1 ∘C) in May 2021, while salinity ranged from 32.0 to 34.3 psu (Fig. 4). At PDM-154, water was generally warmer, frequently reaching temperatures > 5 ∘C and never decreasing < 1 ∘C, while salinity was in the same range as at BC-133 (Fig. 5). These data confirm that all three sediment traps were located within the deep layer of the water column. We note that since the sediment traps BC-133 and PDM-154 were moored within the deep layer, the recorded fluctuations in temperature and salinity shown in Figs. 4–5 may be due to (1) a change in the contribution from the Labrador Current (lower temperature and salinity) and North Atlantic waters (higher temperature and salinity) or (2) vertical mixing of the cold intermediate layer (lower temperature and salinity) with the deep layer (higher temperature and salinity). However, the data that we collected as part of this study are not sufficient to determine the cause for the changes in the properties of the water mass.
4.2 Magnitude and composition of particle fluxes
At BC-133, daily TPM fluxes remained lower than 1.1 g m−2 d−1 from November 2020 to September 2021 (Fig. 4). Peak chloropigment fluxes (1.6 mg m−2 d−1) were recorded in early May 2021, concurrent with an increase in TPM, POC, and PN fluxes. Peak POC and PN fluxes were observed in early August 2021 (106.5 and 16.5 mg m−2 d−1, respectively). Chloropigment, POC, and PN fluxes remained consistently low from mid-November 2020 until April 2021. POC:PN mass ratios peaked (11.7) in February 2021, bulk sediment TOC:TN mass ratios peaked (11.3) in November 2020, δ13C values peaked (−21.0 ‰) in August 2021, and δ15N values peaked (9.8 ‰) in November 2020 and March 2021. Sinking particles collected at BC-133 showed relatively high POC:PN and TOC:TN mass ratios and low δ13C values from November 2020 to April 2021, while the period from May to September 2021 was characterized by relatively low POC:PN and TOC:TN mass ratios and high δ13C values, whereas δ15N values did not reflect a seasonal trend (Fig. 4).
At the PDM site, peak TPM fluxes occurred during the first half of February 2021 at both depths (6.5 and 7.9 g m−2 d−1 at PDM-154 and PDM-224, respectively; Fig. 5). These peak values were up to 7 times higher than the maximum value recorded at BC-133 (1.1 g m−2 d−1; Fig. 4). Fluxes of chloropigments (1.1 mg m−2 d−1) were highest at both PDM-154 and PDM-224 in early November 2020. Overall, fluxes of chloropigments exhibited similar patterns at both depths, with higher flux values at PDM-224. Temporal variations in chloropigment and TPM fluxes were decoupled during April and May 2021 at the PDM site, contrasting with the concurrent peaks at BC-133 in early May 2021. Unlike at BC-133, POC and PN fluxes were higher from November 2020 to February 2021 at both PDM-154 and PDM-224. Peaks in POC and PN fluxes were recorded in November 2020 and February 2021 at PDM-154, whereas values were significantly greater in November and December 2020 at PDM-224. Compared to BC-133, the POC:PN mass ratio peaks were the same or higher (12.8 and 11.6 in PDM-154 and PDM-224, respectively) in February 2021. TOC:TN mass ratio peaks were lower (9.5 in November 2020 at PDM-154 and 8.0 in February 2021 at PDM-224) than the peak measured at BC-133. The δ13C peak values were lower (−21.8 ‰ at PDM-154 and PDM-224) and peaked in August 2021, while δ15N peak values were higher (10.3 ‰ and 10.8 ‰ at PDM-154 and PDM-224, respectively) and peaked in July 2021. A similar TOC:TN mass ratios against δ13C pattern to BC-133 was observed at PDM but with less variation between the November–April and May–September periods (Fig. 6). The seasonal pattern was less distinctive at PDM-224, where average δ13C and low TOC:TN values were maintained during the majority of the sampling period.
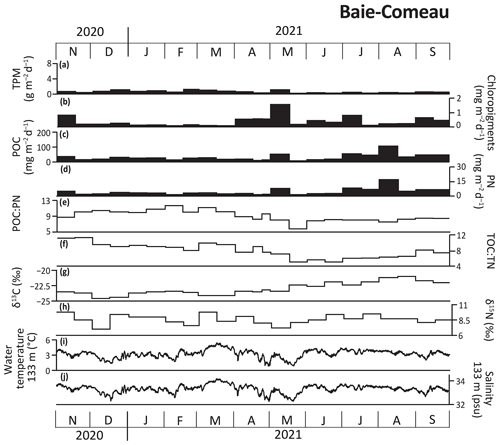
Figure 4Data from the Baie-Comeau (BC-133) mooring site from November 2020 to September 2021. (a) Total particulate matter (TPM); (b) chloropigments; (c) particulate organic carbon (POC) and (d) particulate nitrogen (PN) fluxes; (e) POC:PN and (f) TOC:TN mass ratios; and (g) carbon (δ13C) and (h) nitrogen (δ15N) isotopic values of the sinking particles, (i) water temperature, and (j) salinity.
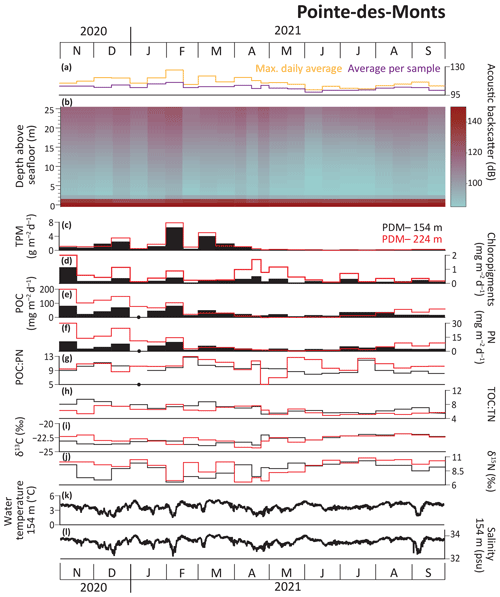
Figure 5Data from the PDMc and PDM mooring sites from November 2020 to September 2021. (a) Maximum daily average and average backscatter per sampling period and (b) average backscatter per 1 m depth from ADCP. (c) Total particulate matter (TPM), (d) chloropigment, (e) particulate organic carbon (POC), and (f) particulate nitrogen (PN) fluxes. (g) POC:PN and (h) TOC:TN mass ratios. (i) Carbon (δ13C) and (j) nitrogen (δ15N) isotopic values of the sinking particles, (k) water temperature, and (l) salinity. Black dots indicate that there are no POC or PN data for PDM-154 for early January 2021.
4.3 Magnitude and composition of phytoplankton fluxes
At BC-133, diatom fluxes were 2 orders of magnitude higher than dinoflagellate fluxes (Figs. 7 and 8). Fluxes of centric diatoms were always higher than pennate diatoms, except during late September 2021, when fluxes of the potentially harmful pennate diatom Pseudo-nitzschia seriata were elevated (Fig. 7). In accordance with chloropigment fluxes, peaks in diatom (∼ 87×106 frustules per square meter per day), dinoflagellate theca ( thecae per square meter per day) and dinoflagellate cyst ( cysts per square meter per day) fluxes were observed in early May 2021. The pennate diatom Pseudo-nitzschia spp. and the centric diatoms Chaetoceros spp., Skeletonema spp., and Thalassiosira spp. dominated the diatom assemblages. Relative contributions of dinoflagellate thecae and cysts varied throughout the year, with a large contribution of the genus Protoperidinium spp. (∼ 14×106 thecae per square meter per day) in early May (Fig. 8). Dinophysis acuminata and D. norvegica, two dinoflagellate species known for producing okadaic acid group toxins associated with diarrhetic shellfish poisoning (Bates et al., 2020), were observed in June and August 2021 at BC-133. See Appendix A for the complete list of diatom and dinoflagellate taxa observed and photo plates showing representative specimens.
At PDM-154, the peak in diatom fluxes (∼ 73×106 frustules per square meter per day) was observed in mid-April 2021, while the peak in dinoflagellate fluxes (∼ 15×104 thecae per square meter per day) occurred in early July 2021 (Figs. 7 and 8). From December 2020 to early April 2021, pennate diatom fluxes were often similar or higher than centric diatom fluxes, while centric taxa dominated the diatom fluxes during November 2020 and from late April to September 2021. All the dominant diatom taxa showed lower fluxes at PDM-154 compared to BC-133 (Fig. 7), and none of the maximum fluxes for the dominant diatoms were synchronous between the two sites. At PDM-154, dinoflagellates were rarely collected before May 2021, apart from Dinophysis spp., which were present in early December 2020. However, we note that the dinoflagellate cyst Brigantedinium spp. was observed in December, February, and March 2021.
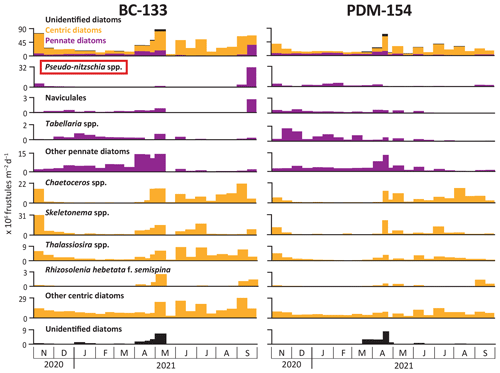
Figure 7Fluxes of dominant diatoms measured at Baie-Comeau (BC-133) and Pointe-des-Monts (PDM-154) from November 2020 to September 2021. The top panel indicates total diatom fluxes, distinguishing unidentified, centric, and pennate diatom groupings. The red box indicates a potentially harmful taxon. Several genera were grouped together in the order Naviculales (Diploneis spp., Gyrosigma/Pleurosigma spp., Navicula spp., and cf. Plagiotropis spp.).
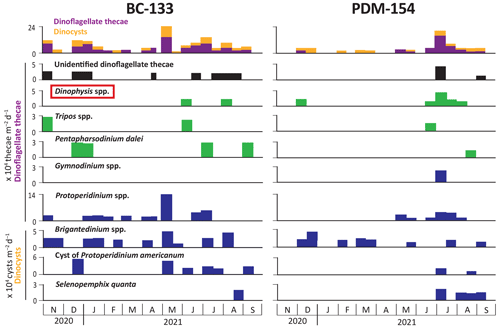
Figure 8Fluxes of dinoflagellate thecae and dinoflagellate cysts (dinocysts) measured at Baie-Comeau (BC-133) and Pointe-des-Monts (PDM-154) from November 2020 to September 2021. The top panel indicates total dinoflagellate fluxes, distinguishing thecae and cysts. The red box indicates a potentially harmful taxon. Green indicates autotrophic taxa, and blue indicates heterotrophic taxa.
4.4 Annual fluxes
Annual fluxes of chloropigments, diatoms, and dinoflagellates were almost 2 times higher at BC-133 than at PDM-154 (Table 2). In contrast, annual fluxes of TPM, POC, and PN were highest at PDM-224, where the TPM flux value was more than 2 times higher than at BC-133.
4.5 Backscatter data in the main Pointe-des-Monts canyon
The average backscatter measured at PDMc ranged from 98.7 to 110.8 dB, with a peak during the first half of February 2021 (Fig. 5a). This peak was associated with a small turbidity current that occurred on 3 February 2021 (Appendix B, Fig. B1). The maximum daily average of backscatter for each trap sampling period ranged from 101.7 to 126.2 dB, with the highest value recorded concurrently with enhanced TPM and POC at both PDM-154 and PDM-224 (Fig. 5a, c, e). A smaller resuspension event was identified on 22–23 December 2020 based on a sharp increase in backscatter near the seafloor (Fig. 5b), which also occurred concurrently with enhanced TPM and POC fluxes. TPM fluxes at both depths were positively correlated with the average backscatter (R2=0.456 for PDM-154 and 0.586 for PDM-224; p<0.01) and maximum daily average backscatter (R2=0.652 for PDM-154 and 0.751 for PDM-224; p<0.001).
5.1 Canyon-related sediment resuspension events
Normandeau et al. (2020) determined that turbidity currents in the PDM canyon system are associated with sustained (> 7 h) windstorms (> 60 km h−1) that create large waves which remobilize sediment on the shelf at low tide. Based on repeat bathymetric data, they estimated that the upper region of the main canyon, the most active part of the system, experiences a minimum of one turbidity current every 2–3 years that is large enough to cause migration of crescentic bedforms in the canyon. However, recent ultra-high-resolution surveys suggest that smaller turbidity currents likely occur more frequently but are not strong enough to erode the seafloor and are thus not observed through bathymetric data alone (Normandeau et al., 2022). Continuous measurements of the hydrodynamics in the main axis of the canyon during the present study identified one small turbidity current on 3 February 2021 based on a combination of high backscatter values and a sharp increase in down-canyon water velocity. This event concurred with the highest daily wind gusts recorded for that year (> 90 km h−1; Government of Canada Past Weather and Climate Historical Data). Increased backscatter (i.e., sediment resuspension) from complex hydrodynamic processes (e.g., turbidity currents, upwelling and downwelling currents, internal tides, internal waves) in the main canyon was associated with increased TPM fluxes at both PDM-154 and PDM-224. The small turbidity current event on 3 February 2021 showed some lofting (i.e., heavy sediments sink but lighter sediments rise in the water column), which may have been sustained by a strong pycnocline (Fig. 9). Thus, we propose that the vertical structure of the water column may have contributed to enhancing lateral dispersion of resuspended sediments, which may enhance the regional impact of canyon sediment processes. Our data suggest that despite the relatively small size of the PDM canyon system and relative weakness of the recorded turbidity current, it nonetheless influenced the vertical fluxes of particles at a lateral distance of > 2.6 km within the deep water column layer. This is supported by the annual TPM flux being more than 2 times higher at PDM-154 than BC-133, with the maximum daily TPM flux being 6 times higher during early February 2021. Thus, our data suggest that larger turbidity currents, which are estimated to occur every 2–3 years (Normandeau et al., 2020), may have relatively severe and widespread impacts on the PDM region.
A smaller resuspension event was identified on 22–23 December 2020 based on a sharp increase in backscatter near the seafloor but lacked the sharp increase in down-canyon water velocity required of a turbidity current. This event was not correlated with significant wind gusts (< 33 km h−1; Government of Canada Past Weather and Climate Historical Data). During this smaller resuspension event, TPM fluxes increased at the PDM site, although values were lower than those associated with the turbidity current.
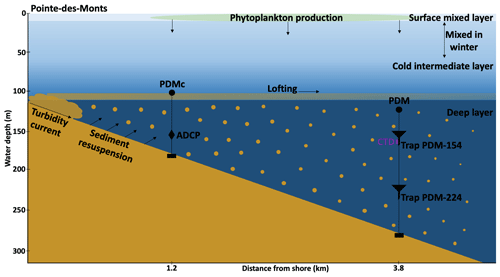
Figure 9Schematic showing a turbidity current event with sediment resuspension and lofting contributing to increased biogenic matter in the sediment traps.
POC:PN ratios are used as an indicator of nutrient limitation or relative diagenetic state (i.e., “chemical freshness”) of marine particulate organic matter, with values around 6 to 8 being representative of labile organic matter, and degraded organic matter typically yielding values > 10 (e.g., Redfield, 1958; Walker et al., 2016; Fox and Walker, 2022). In the present study, the highest POC:PN mass ratio value (12.8), recorded at PDM-154 during the second half of February 2021, likely indicates increased contributions of comparatively more degraded particulate matter resulting from resuspension of relatively old material from the seafloor. Additionally, enhanced POC and PN fluxes observed during both remobilization events were concurrent with small increases in the chloropigment fluxes, which were mostly comprised of degradation-indicative pheopigments. Thus, we infer that canyon-related resuspension events had an impact on biogeochemical cycling in the deep layer, remobilizing and advecting particulate organic matter in the water column during winter when supplies to benthic communities are typically limited.
5.2 Sources of biogenic matter
Discrimination between autochthonous and allochthonous contributions to organic matter can be done using POC:PN mass ratios and bulk sediment δ13C signatures. Marine sources are typically characterized by POC:PN mass ratios of 4 to 10 and δ13C of −20 ‰ to −24 ‰, while terrestrial sources have POC:PN mass ratios of 20 to 100 and a δ13C signature around −27 ‰ because the majority of photosynthetic plants found along the coasts of the LSLE produces organic matter using a C3 Calvin pathway (Meyers, 1994; Macdonald et al., 2004). In the present study, POC:PN mass ratios (5.1 to 12.8) and measured δ13C values (−24.6 to −21.0 ‰) indicate that the sinking particles collected at both sites were of predominantly marine origin with some terrestrial influence. Sinking particles collected at BC-133 were likely influenced by the Manicouagan River discharge, which transports terrestrial material into the LSLE (Therriault and Levasseur, 1985). The terrestrial influence appeared relatively high from November 2020 to April 2021 at BC-133, as indicated by high bulk sediment TOC:TN mass ratios and low δ13C values (Fig. 6), as well as high POC:PN mass ratios. This is likely due to the four hydroelectric dams located in the Manicouagan River basin, which retain large reservoirs of water from the spring runoff and then release during the fall and winter when electricity demands increase (Lavoie et al., 2017). In contrast, the PDM site does not receive inputs of matter from a local freshwater system but instead from resuspended sediments from the seafloor (e.g., Normandeau et al., 2020).
Bulk sediment δ15N provides valuable information regarding nutrient sources, nitrogen utilization, and trophic alteration. Phytoplankton selectively uptake and assimilate light nitrate (14NO or ammonia (14NH3) when available and must incorporate increasingly more 15NO as nitrogen concentrations become depleted in surface waters (Altabet and Francois, 1994; Waser et al., 1998; Altabet and Francois, 2001, and references therein; Minagwa et al., 2001). Thus, the δ15N signature of sinking particles collected in the traps would be expected to increase during and following the main bloom period (Wada and Hattori, 1978; Altabet et al., 1991; Nakatsuka et al., 1992; Sigman et al., 1999; Altabet and Francois, 2001). In both PDM traps, we observed variable δ15N values until the spring bloom in April 2021, followed by high values until September 2021, but we note no correlation to other primary production proxies (i.e., fluxes of chloropigments, diatoms, and dinoflagellates). This may be because the bulk samples contained all nitrogen forms, including nitrogen-bearing lithics (Kienast et al., 2005) and organic matter sorbed onto resuspended fine clays and silts (Mayer, 1994), which undergo selective removal of nitrogen-containing compounds during degradation and early diagenesis (Close, 2019). This is particularly relevant for sinking particles collected at the BC and PDM sites, where PN fluxes comprise only a small proportion (< 1 %) of the TPM fluxes. We therefore propose that bulk δ15N analysis of the organic-only fraction would be more informative for studies along the north shore of the LSLE.
5.3 Phytoplankton dynamics
The sinking rate of biogenic matter is highly variable depending on shape, size, density, aggregation, remineralization, etc. For example, sinking rates of individual diatoms range from 0.1 to 10 m d−1 (Smayda, 1970), while aggregates can sink at rates of 88 to 569 m d−1 (Iversen et al., 2010). Sinking biogenic matter generally forms aggregates (“marine snow”) to facilitate vertical fluxes of pelagic particles; therefore, we can assume that the sinking particles obtained in the sediment traps are representative of their respective sampling time periods.
At both BC-133 and PDM-154, relatively high diatom fluxes were sustained for 7 months, during November 2020 and from April to September 2021, exhibiting a long production season for the region (Therriault and Levasseur, 1985; Blais et al., 2021; Genin et al., 2021). Diatom fluxes were unusually high during November 2020, particularly at BC-133, which may be attributed to riverine and coastal nutrient input. Importantly, the 2020–2021 winter season experienced anomalous nearly ice-free conditions with above-average sea surface temperatures delaying sea ice formation (Galbraith et al., 2022), which may have played a key role in the elevated diatom fluxes in November 2020. The predicted climate-induced decline in sea ice cover will likely have important implications on primary production, including a lengthening of the production season. In addition, reduced sea ice cover exposes the coastal area near PDM to increased potential for sediment remobilization events during winter (Ruest et al., 2016), highlighting the necessity of understanding how these events influence regional primary production. Thus, in the context of climate warming, this study provides valuable insight into future trends in the LSLE, which could have impacts on regional St. Lawrence ecosystems. We note that since the present study covers an anomalous period in terms of sea ice, we cannot definitively determine if the long diatom production season observed here was typical of the study sites or if it was lengthened due to nearly ice-free winter conditions. Thus, we recommend continued monitoring to gather more information in consecutive years under a wider range of environmental conditions.
Primary producer assemblages in the LSLE have been reported to be dominated by pennate diatoms in the spring and centric diatoms in the early summer, followed by dinoflagellates (Levasseur et al., 1984; Therriault and Levasseur, 1985; Roy et al., 1996; Romero et al., 2000). Here, diatom fluxes suggest that the spring diatom bloom occurred earlier at PDM-154 (mid-April 2021) than at BC-133 (early May 2021). Both sites displayed several subsequent peaks throughout the summer and fall months, but those observed at BC-133 were notably of higher magnitude (Fig. 7). The similar compositional assemblages but distinct magnitude and timing of diatom fluxes at the two sites likely reflect differential nutrient inputs. One could hypothesize that canyon-related hydrodynamics would stimulate phytoplankton production at the PDM site because past studies have shown that the complex hydrodynamics of large submarine canyons increase the occurrence of biodiversity hotspots (Fernandez-Arcaya et al., 2017, and references therein; Paull et al., 2018; Santora et al., 2018). However, phytoplankton fluxes do not provide evidence that this applies at PDM, a relatively small and shallow submarine canyon system located within a nutrient-rich estuarine system. Instead, annual fluxes of diatoms and dinoflagellates were lower at PDM-154 compared to BC-133. At the PDM site, in addition to the absence of direct riverine input and differences in the structure of the water column, increased sediment input from the coast and seafloor remobilization by canyon processes may have hindered primary production by limiting light availability, which is the most important variable controlling phytoplankton growth in the LSLE (Therriault and Levasseur, 1985). As seen with the small turbidity current on 3 February 2021, a strong pycnocline between the cold intermediate layer and the deep layer can enhance sediment lofting and allow resuspended sediments to stay in the water column for a longer time and over a larger region before settling on the seafloor. However, our data do not permit us to evaluate if lofted sediments also reached the surface layer where they would have decreased primary production. Additionally, turbidity currents and other sediment remobilization events appear to be more frequent from late fall through winter, therefore we cannot evaluate if sediment lofting would play a key role in this system throughout the entire year, particularly from late spring through early fall, when primary production is greatest.
5.4 Potentially harmful phytoplankton taxa
At BC-133, large fluxes of the pennate diatom Pseudo-nitzschia seriata were observed (∼ 32×106 frustules per square meter per day) during the second half of September 2021, suggesting the occurrence of an autumn bloom. This species produces domoic acid associated with amnesic shellfish poisoning, which has led to mortality of humans, marine mammals, fish, and birds worldwide but has not yet been fatal in Canadian waters (Bates et al., 2020, and references therein). No concerning ecological effects were reported at that time (e.g., high toxin levels, mortalities), and the bloom was relatively constrained spatially as it was not observed as far east as PDM-154. Concentrations greater than 5000 cells per liter of P. seriata are generally considered harmful algal blooms, although toxin production is variable and is not always correlated to cell abundance but is instead dependent on several factors such as salinity, temperature, pH, and nutrient limitations (e.g., Boivin-Rioux et al., 2022). The dinoflagellates Dinophysis acuminata and D. norvegica, which produce okadaic acid group toxins associated with diarrhetic shellfish poisoning, a non-deadly but severe gastrointestinal illness, were also present in low abundances in sinking particles collected at both sites. Our data do not show evidence of the potential role of canyon processes for the inoculation of harmful algal blooms in the water column during the 2020–2021 deployment period. However, a more detailed analysis focused on harmful algae would be required to quantify this relationship.
5.5 Flux comparison with previous sediment trap studies
TPM fluxes previously measured in the LSLE during May and July (1.8 to 11.3 g m−2 d−1; 150 m trap depth, 350 m water column depth; Colombo et al., 1996), were much higher than those measured in this study for the same spring and summer months (0.1 to 1.0 g m−2 d−1). However, total mass fluxes from an annual cycle in the Gulf of Maine (max. 0.12 g m−2 d−1 in May; 150 m trap depth, 280 m water column depth; Pilskaln et al., 2014) and from several drifting sediment trap deployments in the Gulf of St. Lawrence (max. 1.6 g m−2 d−1 in June; 50 and 150 m trap depths; Romero et al., 2000) were significantly lower than TPM fluxes measured at both sites in our study. Annual chloropigment (70 to 120 mg m−2 yr−1) and POC (11 to 19 g m−2 yr−1) fluxes measured here were much greater than those measured near Cabot Strait (35.9 mg m−2 yr−1 and 1.1 g m−2 yr−1, respectively; 100 m trap depth, 461 m water column depth; Genin et al., 2021), suggesting enhanced biological productivity in the estuarine system compared to the seaward entrance of the Gulf of St. Lawrence. These variations in flux measurements could be attributed to many factors, including variations in environmental conditions, local productivity, sediment trap deployment, and laboratory methods.
Time series biogenic matter fluxes and ADCP data indicate that the PDM submarine canyon system experienced a minor sediment remobilization event on 22–23 December 2020 and a small turbidity current on 3 February 2021. Both events led to enhanced particle fluxes over the submarine fan > 2.6 km further offshore. We suggest that larger turbidity currents, which are estimated to occur every 2–3 years, potentially have widespread impacts on fluxes of biogenic matter in the PDM region. The events recorded here influenced biogeochemical cycling in the deep layer by remobilizing and advecting biogenic matter, as reflected by concurrent elevated POC, PN, and chloropigment fluxes. Sinking particles collected at the base of the submarine canyon (PDM-154) and outside the canyon system (BC-133) had similar compositional assemblages of diatoms and dinoflagellates. However, our data show that annual phytoplankton fluxes were lower in the canyon compared with background LSLE values as recorded at Baie-Comeau. This may be attributed to differences in riverine input and the structure of the water column, in addition to increased sediment input and resuspension at the PDM site, collectively contributing to limited light availability, the most important variable controlling primary production in the LSLE. We also show that the potentially harmful pennate diatom Pseudo-nitzschia seriata and dinoflagellates Dinophysis acuminata and D. norvegica were present at both sites. The 2020–2021 winter season experienced anomalous nearly ice-free conditions, which may have implications on the length of the diatom production season and likelihood of remobilization events in the PDM canyon. Thus, in the context of climate warming, this study provides valuable insight into future trends of biogenic matter export in the LSLE, which could have regional ecological impacts. We thereby recommend continued monitoring of the PDM canyons, using ADCPs and sediment traps, to gather more information in consecutive years under a wider range of environmental conditions.
Table A1Diatom taxa identified in the moored sediment traps at Baie-Comeau (BC-133) and Pointe-des-Monts (PDM-154). P indicates present at the study site.
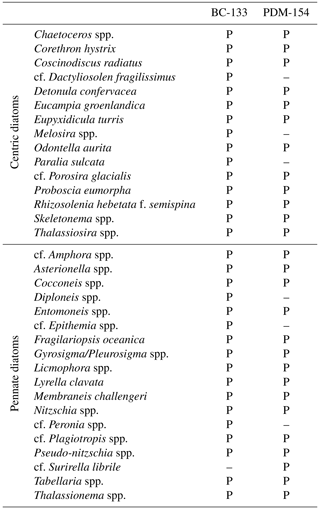
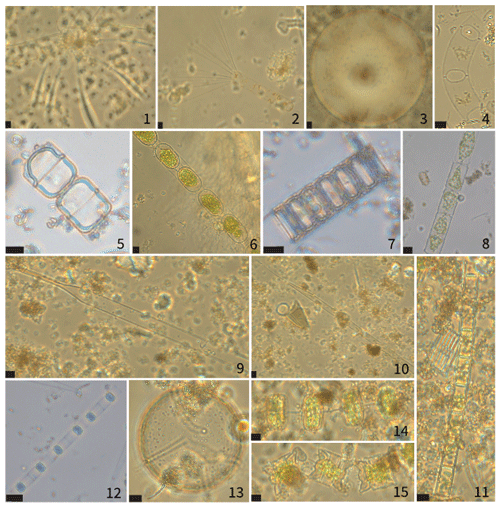
Figure A1Micrographs of representative centric diatoms identified in sediment traps BC-133 and PDM-154. 1. Chaetoceros atlanticus. 2. Corethron hystrix. 3. Coscinodiscus radiatus. 4. Eucampia groenlandica. 5. Melosira spp. 6. Eupyxidicula turris. 7. Paralia sulcata. 8. cf. Dactyliosolen fragilissimus. 9. Proboscia eumorpha. 10. Rhizosolenia hebetata f. semispina. 11. Detonula confervacea. 12. Skeletonema costatum. 13. cf. Porosira glacialis. 14. Thalassiosira cf. gravida. 15. Odontella aurita. All scale bars are 10 µm.
Table A2Dinoflagellate taxa identified in the moored sediment traps at Baie-Comeau (BC-133) and Pointe-des-Monts (PDM-154 m). P indicates present at the study site. Brackets highlight thecae or cysts that were not present, but the corresponding theca or cyst was present.
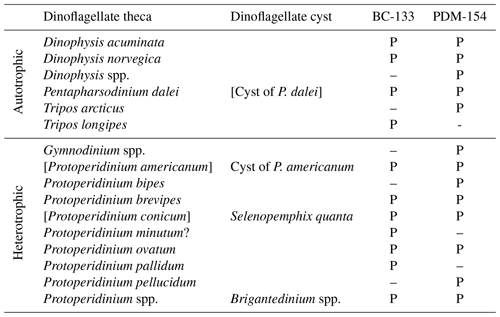
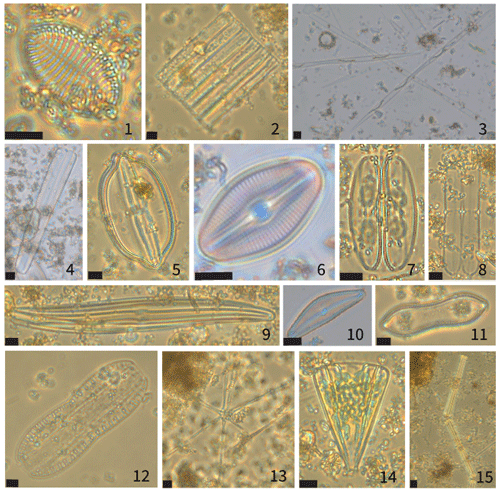
Figure A2Micrographs of representative pennate diatoms identified in sediment traps BC-133 and PDM-154. 1. Cocconeis spp. 2. Nitzschia spp. 3. Pseudo-nitzschia seriata. 4. cf. Peronia spp. 5. Lyrella clavata. 6. Diploneis spp. 7. Membraneis challengeri. 8. cf. Plagiotropis spp. 9. Gyrosigma/Pleurosigma spp. 10. cf. Epithemia spp. 11. cf. Surirella librile. 12. Entomoneis spp. 13. Asterionella spp. 14. Licmophora spp. 15. Tabellaria spp. All scale bars are 10 µm.
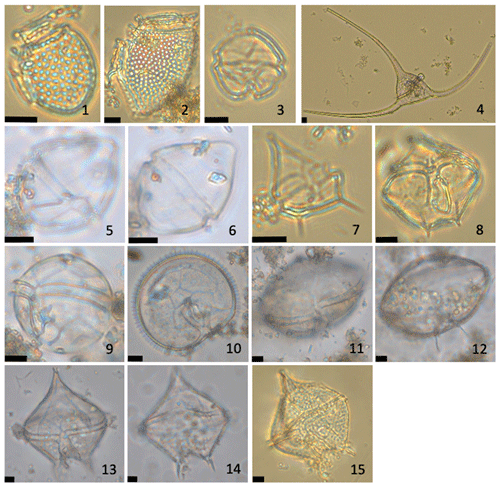
Figure A3Micrographs of representative dinoflagellate thecae identified in sediment traps BC-133 and PDM-154. 1. Dinophysis acuminata. 2. Dinophysis norvegica. 3. cf. Gymnodinium spp. 4. Tripos arcticus. 5–6. Pentapharsodinium dalei. 7. Protoperidinium bipes. 8. Protoperidinium brevipes. 9. Protoperidinium minutum? 10–12. Protoperidinium ovatum. 13–14. Protoperidinium pallidum. 15. Protoperidinium pellucidum. All scale bars are 10 µm.
Observations can be obtained at https://doi.org/10.26071/ogsl-9b07076f-e47b (Sharpe et al., 2023).
This project was designed by AL, AN, and JCMS, and AL funded the study. HS conducted the analyses and interpretation of the results, prepared the figures, and wrote the original draft of the manuscript with supervision and training from AL, MG, CL, AN, and OS. KB and DB provided the ADCP data. All co-authors contributed to the methodology design, interpretation of the data, and reviewed and approved the manuscript.
The contact author has declared that none of the authors has any competing interests.
Publisher's note: Copernicus Publications remains neutral with regard to jurisdictional claims made in the text, published maps, institutional affiliations, or any other geographical representation in this paper. While Copernicus Publications makes every effort to include appropriate place names, the final responsibility lies with the authors.
This study received financial support from the Marine Environmental Observation, Prediction, and Response Network (MEOPAR) and Réseau Québec Maritime (RQM). We also acknowledge the support of the Natural Sciences and Engineering Research Council of Canada (NSERC-DG 2018-03984 to Audrey Limoges and CGS-M to Hannah Sharpe). We thank the participants and crew of the COR2007 and COR2110 expeditions on the R/V Coriolis II, with special thanks to Christian Boutot for the design, deployment, and retrieval of the moorings. We thank Isabelle Tremblay from MEOPAR and Erwann Fraboulet from RQM for their logistical help throughout this project. We thank Kyung-Hoon Shin and an anonymous reviewer for comments and suggestions which improved the quality of the manuscript.
This research has been supported by the Natural Sciences and Engineering Research Council of Canada (grant nos. NSERC-DG 2018-03984 and NSERC CGS-M) and the Marine Environmental Observation Prediction and Response Network (grant no. RQM-MEOPAR 01).
This paper was edited by Ji-Hyung Park and reviewed by Kyung-Hoon Shin and one anonymous referee.
Altabet, M. A., Deuser, W. G., Honjo, S., and Stinen, C.: Seasonal and depth-related changes in the source of sinking particles in the North Atlantic, Nature, 354, 136–139, https://doi.org/10.1038/354136a0, 1991.
Altabet, M. A. and Francois, R.: Sedimentary nitrogen isotopic ratio as a recorder for surface ocean nitrate utilization, Global Biogeochem. Cy., 8, 10–35, https://doi.org/10.1029/93GB03396, 1994.
Altabet, M. A. and Francois, R.: Nitrogen isotope biogeochemistry of the Antarctic Polar Frontal Zone at 170∘ W, Deep Sea Res. Part II, 48, 4247–4273, https://doi.org/10.1016/S0967-0645(01)00088-1, 2001.
Bates, S. S., Beach, D. G., Comeau, L. A., Haigh, N., Lewis, N. I., Locke, A., Martin, J. L., McCarron, P., McKenzie, C. H., Michel, C., Miles, C. O., Poulin, M., Quilliam, M. A., Rourke, W. A., Scarratt, M. G., Starr, M., and Wells, T.: Marine Harmful Algal Blooms and Phycotoxins of Concern to Canada, Canadian Technical Report of Fisheries and Aquatic Sciences, Open File 3384, 322 pp., ISBN 978-0-660-35266-4, 2020.
Bérard-Therriault, L., Poulin, M., and Bossé, L.: Guide d'identification du phytoplancton marin de l'estuaire et du golfe du Saint-Laurent: Incluant également certains protozoaires, Publication spéciale canadienne des sciences halieutiques et aquatiques, 128, 387 p., ISBN 0-660-96057-5, 1999.
Bernier, R. Y., Jamieson, R. E., and Moore, A. M.: State of the Atlantic Ocean Synthesis Report, Canadian Technical Report of Fisheries and Aquatic Science, Open File 3167, iii + 149 p., ISBN 978-0-660-09252-2, 2018.
Blais, M., Galbraith, P. S., Plourde, S., Devred, E., Clay, S., Lehoux, C., and Devine, L.: Chemical and Biological Oceanographic Conditions in the Estuary and Gulf of St. Lawrence during 2020, Canadian Science Advisory Secretariat Research Document No. 2021/060, iv + 67 p., ISBN 978-0-660-40307-6, 2021.
Boivin-Rioux, A., Starr, M., Chassé, J., Scarratt, M., Long, Z., and Lavoie, D.: Harmful algae and climate change on the Canadian East Coast: Exploring occurrence predications of Dinophysis acuminata, D. norvegica, and Pseudo-nitzschia seriata, Harmful Algae, 112, 102183, https://doi.org/10.1016/j.hal.2022.102183, 2022.
Boon, A. R. and Duineveld, G. C. A.: Phytopigments and fatty acids as molecular markers for the quality of near-bottom particulate organic matter in the North Sea, Neth. J. Sea Res., 35, 279–291, https://doi.org/10.1016/S0077-7579(96)90084-8, 1996.
Bourgault, D., Morsilli, M., Richards, C., Neumeier, U., and Kelley, D. E.: Sediment resuspension and nepheloid layers induced by long internal solitary waves shoaling orthogonally on uniform slopes, Cont. Shelf Res., 72, 21–33, https://doi.org/10.1016/j.csr.2013.10.019, 2014.
Casse, M., Montero-Serrano, J.-C., St-Onge, G., and Poirier, A.: REE distribution and Nd isotope composition of estuarine waters and bulk sediment leachates tracing lithogenic inputs in eastern Canada, Mar. Chem., 211, 117–130, https://doi.org/10.1016/j.marchem.2019.03.012, 2019.
Close, H. G.: Compound-specific isotope geochemistry in the ocean, Annu. Rev. Mar. Sci., 11, 27–56, https://doi.org/10.1146/annurev-marine-121916-063634, 2019.
Colombo, J. C., Silverberg, N., and Gearing, J. N.L Biogeochemistry of organic matter in the Laurentian Trough, I. Composition and vertical fluxes of rapidly settling particles, Mar. Chem., 51, 277–293, https://doi.org/10.1016/0304-4203(95)00059-3, 1996.
Covault, J. A.: Submarine fans and canyon-channel systems: A review of processes, products and models, Nature Education Knowledge, 3, 4, https://www.nature.com/scitable/knowledge/library/submarine-fans-and-canyon-channel-systems-a-24178428/, 2011.
Dickie, L. M. and Trites, R. W.: The Gulf of St. Lawrence, in: Estuaries and Enclosed Seas, edited by: Ketchum, B. H., Elsevier., Amsterdam, 403 pp., ISBN 0-444-41921-7, 1983.
Fernandez-Arcaya, U., Ramirez-Llodra, E., Aguzzi, J., Allcock, A. L., Davies, J. S., Dissanayake, A., Harris, P., Howell, K., Huvenne, V. A. I., Macmillan-Lawler, M., Martín, J., Menot, L., Nizinski, M., Puig, P., Rowden, A. A., Sanchez, F., and Van den Beld, I. M. J.: Ecological role of submarine canyons and need for canyon conservation: A review, Front. Mar. Sci., 4, 5, https://doi.org/10.3389/fmars.2017.00005, 2017.
Fox, A. and Walker, B. D.: Sources and cycling of particulate organic matter in Baffin Bay: A multi-isotope δ13C, δ15N, and Δ14C approach, Front. Mar. Sci., 9, 846025, https://doi.org/10.3389/fmars.2022.846025, 2022.
Galbraith, P. S., Chassé, J., Dumas, J., Shaw, J.-L., Caverhill, C., Lefaivre, D., and Lafleur, C.: Physical Oceanographic Conditions in the Gulf of St. Lawrence during 2021, Canadian Science Advisory Secretariat Research Document No. 2022/034, iv + 83 p., ISBN 978-0-660-44402-4, 2022.
Genin, F., Lalande, C., Galbraith, P. S., Larouche, P., Ferreyra, G. A., and Gosselin, M.: Annual cycle of biogenic carbon export in the Gulf of St. Lawrence, Cont. Shelf Res., 221, 104418, https://doi.org/10.1016/j.csr.2021.104418, 2021.
Gilbert, D. and Pettigrew, B.: Interannual variability (1948–1994) of the CIL core temperature in the Gulf of St. Lawrence, Can. J. Fish. Aqua. Sci., 54, 57–67, https://doi.org/10.1139/f96-160, 1997.
Gilbert, D., Sundby, B., Gobeil, C., Mucci, A., and Tremblay, G.-H.: A seventy-two-year record of diminishing deep-water oxygen in the St. Lawrence estuary: The northwest Atlantic connection, Limnol. Oceanogr., 50, 1654–1666, https://doi.org/10.4319/lo.2005.50.5.1654, 2005.
Hargrave, B. T., Walsh, I. D., and Murray, D. W.: Seasonal and spatial patterns in mass and organic matter sedimentation in the North Water, Deep Sea Res. Part II, 49, 5227–5244, https://doi.org/10.1016/S0967-0645(02)00187-X, 2002.
Hasle, G. R. and Syvertsen, E. E.: Marine Diatoms, in: Identifying Marine Phytoplankton, edited by: Tomas, C. R., Academic Press, 5–385, ISBN 978-0-12-693018-4, 1997.
Ingram, R. G.: Vertical mixing at the head of the Laurentian Channel, Estuarine, Coas. Shelf Sci., 16, 333–338, https://doi.org/10.1016/0272-7714(83)90150-6, 1983.
Iversen, M. H., Norwald, N., Ploug, H., Jackson, G. A., and Fischer, G.: High resolution profiles of vertical particulate organic matter export off Cape Blanc, Mauritania: Degradation processes and ballasting effects, Deep-Sea Res. Part I, 57, 771–784, https://doi.org/10.1016/j.dsr.2010.03.007, 2010.
Kienast, M., Higginson, M. J., Mollenhauer, G., Eglinton, T. I., Chen, M.-T., and Calvert, S. E. L.: On the sedimentological origin of down-core variations of bulk sedimentary nitrogen isotope ratios, Paleoceanography, 20, PA2009, https://doi.org/10.1029/2004PA001081, 2005.
Lavoie, D., Gilson, G., Chassé, J., Lambert, N., Brunelle, C. B., Starr, M., Plourde, S., Brickman, D., and Maps, F.: Impacts of freshwater flow regulation of Quebec's large rivers on the physical environment and krill transport in the Gulf of St. Lawrence and on the Scotian Shelf, Canadian Technical Report of Hydrography and Ocean Sciences No. 318, xii + 101 p., ISBN 978-0-660-08480-0, 2017.
Levasseur, M., Therriault, J.-C., and Legendre, L.: Hierarchical control of phytoplankton succession by physical factors, Mar. Ecol. Progress. Ser., 19, 211–222, https://doi.org/10.3354/meps019211, 1984.
Macdonald, R. W., Naudi, S. W., and Yunker, M. B.: The Beaufort Sea: Distribution, sources, fluxes and burial of organic carbon, in: The organic carbon cycle in the Arctic Ocean, edited by: Stein, R. and Macdonald, R. W., Springer, 177–192, ISBN 978-3540011538, 2004.
Mantoura, R. F. C. and Llewellyn, C. A.: The rapid determination of algal chlorophyll and carotenoid pigments and their breakdown products in natural waters by reverse-phase high-performance liquid chromatography, Anal. Chim. Acta, 151, 297–314, https://doi.org/10.1016/S0003-2670(00)80092-6, 1983.
Mayer, L. M.: Surface area control of organic carbon accumulation in continental shelf sediments, Geochim. Cosmochim. Acta, 58, 1271–1284, https://doi.org/10.1016/0016-7037(94)90381-6, 1994.
Meyers, P. A.: Preservation of elemental and isotopic source identification of sedimentary organic matter, Chem. Geol., 114, 289–302. https://doi.org/10.1016/0009-2541(94)90059-0, 1994.
Minagwa, M., Ohashia, M., Kuramoto, T., and Noda, N.: δ15N of PON and nitrate as a clue to the origin and transformation of nitrogen in the subarctic North Pacific and its marginal sea, J. Oceanogr., 57, 285–301, https://doi.org/10.1023/A:1012430512137, 2001.
Mucci, A., Starr, M., Gilbert, D., and Sundby, B.: Acidification of Lower St. Lawrence Estuary bottom waters, Atmos.-Ocean, 49, 206–218, https://doi.org/10.1080/07055900.2011.599265, 2011.
Nakatsuka, T., Handa, N., Wada, E., and Wong, C. S.: The dynamic changes of stable isotopic ratios of carbon and nitrogen in suspended and sedimented particulate organic matter during a phytoplankton bloom, J. Mar. Res., 50, 267–296, 1992.
Normandeau, A., Lajeunesse, P., St-Onge, G., Bourgault, D., Drouin, S. S.-O., Senneville, S., and Bélanger, S.: Morphodynamics in sediment-starved inner-shelf submarine canyons (Lower St. Lawrence Estuary, Eastern Canada), Mar. Geol., 357, 243–255, https://doi.org/10.1016/j.margeo.2014.08.012, 2014.
Normandeau, A., Lajeunesse, P., and St-Onge, G.: Submarine canyons and channels in the Lower St. Lawrence Estuary (Eastern Canada): Morphology, classification and recent sediment dynamics, Geomorphology, 241, 1–18, https://doi.org/10.1016/j.geomorph.2015.03.023, 2015.
Normandeau, A., Bourgault, D., Neumeier, U., Lajeunesse, P., St-Onge, G., Gostiaux, L., and Chavanne, C.: Storm-induced turbidity currents on a sediment-starved shelf: Insight from direct monitoring and repeat seabed mapping of upslope migrating bedforms, Sedimentology, 67, 1045–1068, https://doi.org/10.1111/sed.12673, 2020.
Normandeau, A., Lajeunesse, P., Ghienne, J. F., and Dietrich, P.: Detailed seafloor imagery of turbidity current bedforms reveals new insight into fine-scale near-bed processes, Geophys. Rese. Lett., 49, e2021GL097389, https://doi.org/10.1029/2021GL097389, 2022.
Parsons, T. R., Maita, Y., and Lalli, C. M.: A Manual of Chemical and Biological Methods for Seawater Analysis, Pergamon Press, xii + 173 p., https://doi.org/10.1016/C2009-0-07774-5, 1984.
Paull, C. K., Talling, P. J., Maier, K. L., Parsons, D., Xu, J., Caress, D. W., Gwiazda, R., Lundsten, E. M., Anderson, K., Barry, J. P., Chaffey, M., O'Reilly, T., Rosenberger, K. J., Gales, J. A., Kieft, B., McGann, M., Simmons, S. M., McCann, M., Sumner, E. J., Clare, M. A.., and Cartigny, M. J.: Powerful turbidity currents driven by dense basal layers, Nat. Commun., 9, 4114, https://doi.org/10.1038/s41467-018-06254-6, 2018.
Pilskaln, C. H., Anderson, D. M., McGillicuddy, D. J., Keafer, B. A., Hayashi, K., and Norton, K.: Spatial and temporal variability of Alexandrium cyst fluxes in the Gulf of Maine: Relationship to seasonal particle export and resuspension, Deep Sea Res. Part II, 103, 40–54. https://doi.org/10.1016/j.dsr2.2012.11.001, 2014.
Redfield, A. C.: The biological control of chemical factors in the environment, Am. Sci., 46, 205–221, 1958.
Romero, N., Silverberg, N., Roy, S., and Lovejoy, C.: Sediment trap observations from the Gulf of St. Lawrence and the continental margin of eastern Canada, Deep Sea Res. Part II, 47, 545–583, https://doi.org/10.1016/S0967-0645(99)00118-6, 2000.
Round, F. E., Crawford, R. M., and Mann, D. G.: The Diatoms: Biology and Morphology of the Genera, Cambridge University Press, x + 747 p., ISBN 0521363187, 1990.
Roy, S., Chanut, J., Gosselin, M., and Sime-Ngando, T.: Characterization of phytoplankton communities in the lower St. Lawrence Estuary using HPLC-detected pigments and cell microscopy, Mar. Ecol.Progress. Ser., 142, 55–73, https://doi.org/10.3354/meps142055, 1996.
Ruest, B., Neumeier, U., Dumont, D., Bismuth, E., Senneville, S., and Caveen, J.: Recent wave climate and expected future changes in seasonally ice-infested waters of the Gulf of St. Lawrence, Canada, Clim. Dynam., 46, 449–466, https://doi.org/10.1007/s00382-015-2592-3, 2016.
Ryan, S. A., Wohlgeschaffen, G., Jahan, N., Niu, H., Ortmann, A. C., Brown, T. N., King, T. L., and Clyburne, J.: State of Knowledge on Fate and Behaviour of Ship-Source Petroleum Spills: Volume 4, St. Lawrence Seaway, Montreal to Anticosti, Québec, Canadian Manuscript Report of Fisheries and Aquatic Sciences Technical Report No. 3176, viii + 42 p., ISBN 978-0-660-30896-8, 2019.
Santora, J. A., Zeno, R., Dorman, J. G., and Sydeman, W. J.: Submarine canyons represent an essential habitat network for krill hotspots in a Large Marine Ecosystem, Sci. Rep., 8, 7579, https://doi.org/10.1038/s41598-018-25742-9, 2018.
Sharpe, H., Gosselin, M., Lalande, C., Normandeau, A., Montero-Serrano, J.-C., Baccara, K., Bourgault, D., Sherwood, O., and Limoges, A.: Time-series biogenic matter export fluxes offshore Pointe-des-Monts and Baie-Comeau, SLGO/OGSL [data set], https://doi.org/10.26071/ogsl-9b07076f-e47b, 2023.
Sigman, D. M., Altabet, M. A., McCorkle, D. C., Francois, R., and Fisher, G.: The δ15N of nitrate in the Southern Ocean: II. Consumption of nitrate in surface waters, Global Biogeochem. Cy., 13, 1149–1166, https://doi.org/10.1006/rwos.2001.0172, 1999.
Smayda, T.: The suspension and sinking of phytoplankton in the sea, Oceanogr. Mar. Bull., 8, 353–414, 1970.
Steidinger, K. A. and Jangen, K.: Dinoflagellates, in: Identifying Marine Phytoplankton, edited by: Tomas, C. R., Academic Press, London, 387–584, ISBN 978-0-12-693018-4, 1997.
Therriault, J. C. and Levasseur, M.: Control of phytoplankton production in the Lower St. Lawrence Estuary: Light and freshwater runoff, Naturaliste Canadien, 112, 77–96, 1985.
Villanueva, J. and Hastings, D. W.: A century-scale record of the preservation of chlorophyll and its transformation products in anoxic sediments, Geochim. Cosmochim. Acta, 64, 2281–2294, https://doi.org/10.1016/S0016-7037(99)00428-7, 2000.
Vinyard, W., C.: Diatoms of North America, Mas River Press, Inc., 120 pp., ISBN 0-916422-15-1, 1979.
Wada, E. and Hattori, A.: Nitrogen isotope effects in the assimilation of inorganic nitrogenous compounds by marine diatoms, Geomicrobiol. J., 1, 85–101, https://doi.org/10.1080/01490457809377725, 1978.
Walker, B. D., Beaupré, S. R., Guilderson, T. P., McCarthy, M. D., and Druffel, E. R. M.: Pacific carbon cycling constrained by organic matter size, age and composition relationships, Nat. Geosci., 9, 888–891, https://doi.org/10.1038/ngeo2830, 2016.
Waser, N. A. D., Turpin, D. H., Harrison, P. J., Nielson, B., and Calvert, S. E.: Nitrogen isotope fractionation during the uptake and assimilation of nitrate, nitrite, and urea by a marine diatom, Limnol. Oceanogr., 43, 215–224, https://doi.org/10.4319/lo.1998.43.2.0215, 1998.
Weise, A. M., Levasseur, M., Saucier, F. J., Senneville, S., Bonneau, E., Roy, S., Sauvé, G., Michaud, S., and Fauchot, J.: The link between precipitation, river runoff, and blooms of the toxic dinoflagellate Alexandrium tamarense in the St. Lawrence, Can. J. Fish. Aqua. Sci., 59, 464–473, https://doi.org/10.1139/f02-024, 2002.