the Creative Commons Attribution 4.0 License.
the Creative Commons Attribution 4.0 License.
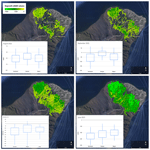
Remote sensing reveals fire-driven enhancement of a C4 invasive alien grass on a small Mediterranean volcanic island
Riccardo Guarino
Daniele Cerra
Renzo Zaia
Alessandro Chiarucci
Pietro Lo Cascio
Duccio Rocchini
Piero Zannini
Salvatore Pasta
The severity and the extent of a large fire event that occurred on the small volcanic island of Stromboli (Aeolian archipelago, Italy) on 25–26 May 2022 were evaluated through remotely sensed data to assess the short-term effect of fire on local plant communities. For this purpose, the differenced normalized burned index (dNBR) was also used to quantify the extent of early-stage vegetation recovery dominated by Saccharum biflorum Forssk. (Poaceae), a rhizomatous C4 perennial grass of Paleotropical origin. The burned area was estimated to have an extension of 337.83 ha, corresponding to 27.7 % of the island surface and to 49.8 % of Stromboli's vegetated area. On the one hand, this event considerably damaged the native plant communities, hosting many species of high biogeographic interest. On the other hand, Saccharum biflorum clearly benefited from fire. In fact, this species showed a very high vegetative performance after burning, being able to exert unchallenged dominance in the early stages of the postfire succession. Our results confirm the complex and probably synergic impact of different human disturbances (repeated fires and the introduction of invasive alien plants) on the natural ecosystems of small volcanic islands.
- Article
(5787 KB) - Full-text XML
-
Supplement
(21066 KB) - BibTeX
- EndNote
Wildfires are a main disturbance factor affecting the Mediterranean terrestrial ecosystems, whose vegetation patterns are largely influenced by interactions with fire. Fire frequency and severity delineate landscape attributes (Pausas, 2006; Jouffroy-Bapicot et al., 2021), affect the structure and composition of the vegetation (Trabaud, 1994), and regulate speed and direction of ecological succession dynamics (Canelles et al., 2019). Also, fire causes sudden variations in the carbon and energy balance of ecosystems (Novara et al., 2013; Harris et al., 2016; Pausas and Millán, 2019) and in the soil microbial activity and functional diversity of the microbiome (Velasco et al., 2009; Goberna et al., 2012).
At the onset of human civilizations, Mediterranean landscapes were deeply modified by anthropogenic fires that were used to expand the open-canopy space available for human activities and facilitate a wide array of foraging activities (Pausas and Keeley, 2009). Throughout human history, demographic fluctuations, innovations, and cultural exchanges have always been accompanied by changes in land use and, thus, in fire regimes and the amount and patchiness of fuel (Guyette et al., 2002; Driscoll et al., 2021).
After the mid-twentieth century, land abandonment associated with an increase in woody cover and the buildup of fuels (Mantero et al., 2020) chiefly contributed to the increased fire hazard in the Mediterranean region (Le Houérou, 1993; Salis et al., 2022). Despite the occurrence of some natural factors favoring fires, most of them are ignited by humans through carelessness or voluntary action. Given that vegetation burning is strongly related to plant water content (Bond and Wilgen, 1996), fires happen mostly during the warmest and driest months, i.e., during the Mediterranean summer (Bergmeier et al., 2021). Climate change scenarios indicate rising temperatures and decreasing amounts of precipitation, resulting in longer summer aridity, soil water shortages, and increasing fire risk (Moriondo et al., 2006; Lozano et al., 2017; IPCC, 2021), despite the fact that lower productivity may limit fuel availability (Baudena et al., 2020).
Nevertheless, typical Mediterranean shrublands are highly resilient to relatively frequent, high-intensity fires, but changes in the fire regime may make these communities susceptible to compositional changes, potentially followed by alien plant invasions (Keely and Brennan, 2012; Vallejo et al., 2012). The positive feedback between invasive species and fire can be a major cause of unidirectional change in invaded ecosystems (Brooks et al., 2004), and invasive species able to sustain increased fire frequency and intensity may generate favorable conditions for their self-perpetuation (Pauchard et al., 2008).
Small islands are particularly vulnerable to biological invasions (Bellard et al., 2016) due to the combined effects of the reduced species pool and the competitive traits of invasive species. This process has been reported for Mediterranean islands (Celesti-Grapow et al., 2016; Fois et al., 2020), particularly in the case of volcanic islands with ongoing or recent volcanic activity (Karadimou et al., 2015; Pasta et al., 2017).
The island of Stromboli (northeast Sicily) represents an ideal case study for interactions between fires and invasive species because it has the lowest number of species, as expected within the archipelago species–area relationship among the seven largest islands of the Aeolian archipelago (Chiarucci et al., 2021).
This study uses remotely sensed data to analyze the postfire damage to local vegetation through the application of a spectrally sensitive index, i.e., the differenced normalized burned index (dNBR), which has also been used to quantify the extent of the subsequent early-stage vegetation recovery, dominated by Saccharum biflorum, in order to highlight the ecological behavior of this invasive alien species and its fire-driven ability to colonize new spaces.
2.1 Study area
The island of Stromboli, 12.6 km2, represents the northeastern end of the Aeolian archipelago, in the southeastern Tyrrhenian Sea, which is in the Mediterranean biogeographical region (Cervellini et al., 2020). Stromboli is the summit of the youngest and most active of the Aeolian islands; its subaerial activity began around 85 ka (Francalanci et al., 2013), and the emerged part consists of a single cone that rises up to 926 m above sea level (m a.s.l.). The island has quite a regular slope averaging 28° and two large horseshoe-shaped flank collapses named “Sciara del Fuoco” on the northwestern flank and “Rina Grande” on the southeastern flank. Our study area covers a surface of ca 3.4 km2, between 50 and 530 m a.s.l., on the northern and eastern sides of Stromboli and can be roughly divided into two sectors. The northern sector is bounded by the “Fili del Fuoco” ridge, which overlooks Sciara del Fuoco, to the west and by the Vallonazzo valley to the east; the eastern sector is bounded by the Vallonazzo valley to the northwest and by the Rina Grande depression to the southeast (Fig. 1). Both sectors are characterized by medium to gentle slopes, with 80 % of the area sloping less than 30° (Fornaciai et al., 2010).
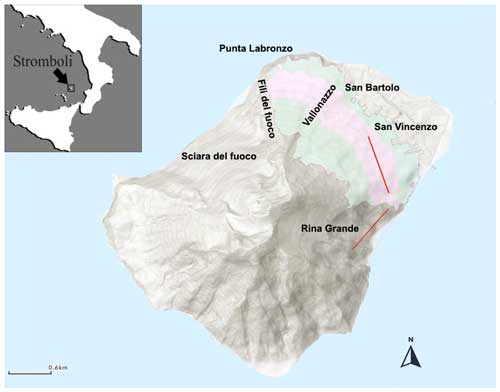
Figure 1Map of the study area (light green) with the place names mentioned in the text. The pink color indicates the area where the vegetation plots for validation were sampled. The red lines identify the two transects along which the stem density of Saccharum was measured.
The climate of Stromboli is typically Mediterranean. At 4 m a.s.l., the average yearly temperature is 18.2 °C, with a mean temperature of 12.3 °C in the coldest month (January) and 26.0 °C in the warmest month (August). The annual rainfall averages 570 mm, while the relative humidity is 75.0 % in winter and 60.8 % in summer. Based on the WorldClim interpolated maps (Hijmans et al., 2005) and on the Rivas-Martínez bioclimates classification (2004), the study area is characterized by an upper thermo-Mediterranean thermotype and a dry to sub-humid ombrotype (Bazan et al., 2015).
The study area was dominated by a typical Mediterranean rockrose garrigue (Cistus creticus subsp. eriocephalus; C. monspeliensis; and C. salviifolius) with scattered patches of maquis including Genista tyrrhena, Spartium junceum, Olea europaea, Erica arborea, and Pistacia lentiscus (Richter, 1984; Cavallaro et al., 2009). The former cultivated land and the volcanic ash deposits were colonized by Saccharum biflorum, while small Quercus ilex stands were occasionally found along the impluvium lines. Equally rare and scattered were the patches dominated by Euphorbia dendroides, limited to the rocky outcrops, especially along the south-facing rim of Vallonazzo valley (Ferro and Furnari, 1968; Richter and Lingenhöhl, 2002). The highest and southernmost end of the study area included part of the local population of Cytisus aeolicus, a narrow-ranging endemic broom growing only on the islands of Vulcano, Alicudi, and Stromboli (Zaia et al., 2020).
On 25–26 May 2022, due to recklessness during the filming of a television drama, a fire broke out in the upper outskirts of the village of San Vincenzo and, fuelled by a strong Sirocco wind, burned the whole of our study area. While Saccharum stands were entirely burned, very few small patches of garrigue and Quercus ilex stands escaped the fire.
2.2 Target species
By far the most common invasive alien species on Stromboli is Saccharum biflorum Forssk. (=S. spontaneum L. subsp. aegyptiacum (Willd.) Hack.; henceforth: Saccharum), a vigorously growing rhizomatous grass of Palaeotropical origin (Amalra and Balasundaram, 2006) with culms of 1.5–2.5 m and flowering stems up to 3 m high. Its rhizomes can be up to 6 m long, with nodes every 10–15 cm from which the culms and fascicled roots branch off (Sect. S1, Fig. S1 in the Supplement). This species has a C4 metabolism and thrives in sandy and silty, often alluvial, soils (Pignatti et al., 2017–2019).
As for the Aeolian archipelago, Saccharum was introduced in the nineteenth century as a windbreak. Gussone (1832) recorded its occurrence (despite wrongly identifying it as Saccharum ravennae L.) on the islands of Stromboli, Panarea, Lipari, and Vulcano as “cultivated hedges in vineyards”. Saccharum then spread on former cultivations and in abandoned terraced fields and wherever there was an accumulation of volcanic ash, as noticed by Ferro and Furnari (1968; our translation):
a large part of the northeastern slope of the island, the very slope that Lojacono (1878) travelled through `vineyards that produce beautiful wines', is covered by dense, almost-monophytic Saccharum vegetation, from sea level up to the upper limit of the ancient crops (…). This slope could have been colonized in a different way by native floristic elements, but it is difficult to make predictions on the final outcome of the competition, given the compactness of the Saccharum rhizomatous apparatus.
However, photos published by Ferro and Furnari (1968) give the impression that Saccharum from 50 years ago was more widespread than nowadays. Besides cultivation abandonment, the establishment of this plant is favored by fire, as observed by Richter (1984). Local elder people recall a major spread of Saccharum soon after the fire, which was caused by the intense eruption in 1930 and the subsequent abandonment of a large portion of the cultivated terraces along the eastern slopes of the island (Richter and Lingenhöhl, 2002). Another large fire event, ignited at the Punta Labronzo landfill site in 1978, promoted the recovery of Saccharum all over the eastern slopes above Punta Labronzo. In following years, the spread of this species was somewhat reduced by the development of native shrubland and garrigue, which, until recently, were the most widespread vegetation types in the study area.
2.3 Satellite imagery processing
To infer the extent of fire damage to the vegetation and the postfire surface of the resprouted Saccharum patches, we used optical satellite images acquired by the spaceborne Sentinel-2 sensor, which is a multispectral mission launched in the framework of the European Space Agency (ESA) Copernicus program (Drusch et al., 2012).
Sentinel-2 globally measures the backscattered solar radiation from ground targets with a temporal resolution of around 5 d across 13 spectral bands with different ground sampling distances (GSDs) varying from 10 to 60 m. In this work, we employed the four bands at a 10 m GSD, namely in the visible range (blue, green, and red) and near-infrared (NIR) range. We relied on band 12 in the shortwave infrared (SWIR) at a 20 m GSD to detect burned areas. Additionally, spectral bands 5, 6, 7, 8a, and 11, all at a 20 m GSD, were used for the supervised classification of different vegetation types. All other bands at a 60 m GSD were not used in this analysis. The products used were at processing level 2A, which provides date that are radiometrically corrected, georeferenced, orthorectified, atmospherically corrected, and converted to the bottom-of-atmosphere reflectance. The choice of using reflectance rather than radiance products is motivated by the following reasons: (1) the overall brightness differences in different images due to different acquisition conditions are reduced in the level 2A products, and (2) the quantities estimated from single images through spectral indices are more meaningful when applied to data in reflectance.
The data selection and processing were carried out on Google Earth Engine (GEE; Amani et al., 2020), which is at the same time a multi-petabyte repository of georeferenced and harmonized Earth observation raster, vector, and tabular datasets, which include the whole Sentinel-2 archive.
To quantify the damage caused by the abovementioned fire event to the vegetation, different Sentinel-2 scenes acquired in a relatively short time span were aggregated. An image composite of the island before the event was derived by considering eight acquisition dates with cloud cover below 5 % acquired before the fire event from 15 April to 22 May 2022 and considering the median reflectance for each image element. This allows the removal of abnormal values due to specific atmospheric conditions inducing errors into the reflectance estimation process, undetected clouds, and cloud shadows in the scene. The postfire reflectance was estimated by applying the same processing to six acquisition dates after the event, from 26 May to 15 June 2022. The two image composites are reported in Fig. 2. Therein, pre- and post-event true-color images obtained from Sentinel-2 bands in the visible range (namely bands 4, 3, and 2) can be visually assessed, with damage caused by the fire in the northeastern part of the island already evident in this band combination.
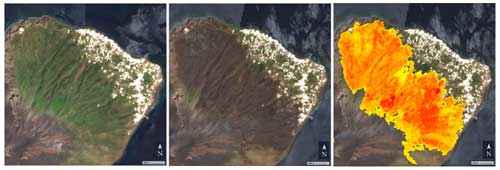
Figure 2From left to right: Sentinel-2 image before the fire event (composite of acquisitions in the time period of 22 April–22 May 2022); Sentinel-2 image after the fire event (composite of acquisitions in the time period of 25 May–15 June 2022); dNBR-inferred burned area (yellow: low-severity damage, orange: middle-severity damage, and red: high-severity damage) overlaid on the middle image composite.
In order to estimate vegetation loss and total burned area, we derived the normalized burn ratio (NBR), defined for a multispectral image x, as
where NIR and SWIR indicate reflectance in the near-infrared and shortwave infrared, represented for Sentinel-2 by bands 8 and 12, respectively. The NBR is a commonly used index to detect burned area and burn severity (Key and Benson, 2006) and is particularly sensitive to the changes in the amount of live green vegetation, moisture content, and some soil conditions, which may occur after a fire (Lentile et al., 2006).
Change detection relying on spectral indices from multitemporal pre-fire and postfire images can be used to estimate vegetation loss or recovery. Relying on the availability of multitemporal images, we used the differenced NBR(dNBR) since it performs well in capturing the spatial severity within fire perimeters (Picotte and Robertson, 2010; Soverel et al., 2010).
The dNBR related to pre- and post-event images, respectively acquired at time t and acquired at time t1, is the delta of the two measurements:
This equation has been used to estimate both fire severity and vegetation recovery after the fire event: a negative dNBR is correlated with recovery after fires, while a positive one indicates damage, with the severity proportional to the dNBR value.
We first estimated the area affected by fire immediately after the event by computing the dNBR for the whole island. The affected area was derived by applying the damage classes defined in Key and Benson (2006). In particular, the value of the dNBR in the middle of the range related to low-severity damage (0.0-0.27) and approximated to the second decimal place, specifically 0.19, was selected and assessed using expert knowledge in order to exclude false positives from the estimation and perform further analysis only on relevant image elements, considering as damaged all image elements with the dNBR above this threshold (Fig. 2). This was necessary as using the value of 0.1 was raising false alarms, most notably within urban areas.
To check whether the severity of the damage was related to geomorphological features, rather than to different vegetation units, the correlation between results of the dNBR and a digital elevation model (DEM) was evaluated. The normalized difference vegetation index (NDVI; Gandhi et al., 2015) was also applied in order to estimate the loss in live green vegetation, and its correlation with dNBR values was checked (Sect. S2).
Finally, in order to evaluate the quality of our results, we computed a new dNBR between the pre-event image and a mosaic of Sentinel-2 acquisitions from the time range of 15–17 August 2022. The burned area detected in such a way was compared with high-resolution images acquired by a drone professional flying a DJI Phantom 3 drone on 17 August 2022, i.e., around 3 months after the fire event and 5 d after the first intense rainstorm. Drone images were merged and georeferenced through the software Agisoft Photoscan Professional (version 1.2.6). These images have a 10 cm GSD and were mosaicked over the northeastern part of the island, covering the inhabited area of San Bartolo and San Vincenzo. The drone images did not cover the higher elevations of our study area, closer to the volcano's vents, or the northernmost part, near Punta Labronzo (Fig. 4).
2.4 Vegetation recovery assessment
The mentioned image composite of Stromboli, derived from eight acquisitions from April–May 2022, was also used to map the structural types of the vegetation affected by the fire through supervised classification based on spectral information. Three vegetation classes have been defined: maquis, garrigue, and Saccharum. The “maquis” class groups tall woody vegetation patches, namely (1) shrublands including Genista tyrrhena, Spartium junceum, Erica arborea, and Pistacia lentiscus; (2) abandoned olive groves invaded by Cytisus infestus and C. laniger; (3) Quercus ilex groves; (4) Euphorbia dendroides shrublands; and (5) Cytisus aeolicus shrublands. The “garrigue” class refers to vegetation patches with dwarf shrubs, subshrubs, and bunchgrasses, including (1) dwarf shrublands dominated by Cistus sp. pl.; (2) herbaceous–chamaephytic vegetation dominated by Cymbopogon hirtus, Oloptum miliaceum, Centranthus ruber, Jacobaea maritima subsp. bicolor, and Scrophularia canina; and (3) small impluvia colonized by Rubus ulmifolius and Pteridium aquilinum. Finally, the vegetation patches dominated by Saccharum were attributed to the “Saccharum” class, easily recognized by its typical yellowish-green color and remarkable structural homogeneity, given by one single species covering well over 80 % of the soil. These patches have two different textures: a smoother texture where Saccharum has invaded abandoned vineyards and a more granular texture where Saccharum has invaded former fig tree plantations, as happened in the upper part of our study area.
For each of the three classes described above, 10 patches of 50 pixels each were selected by experts to constitute the training dataset, and 150 random points equally split among the three classes constituted the validation dataset. The area where damage occurred was fed to a support vector machine (SVM) classifier (Hearst et al., 1998), as implemented in the libsvm routine in GEE using a linear kernel and setting the cost C to 1. The input parameters were all Sentinel-2 spectral bands, namely 2 to 8, 8a, 11, and 12, that had a ground sampling distance of 10 or 20 m. The results of the classification algorithm (Fig. 3) were evaluated through visual analysis by experts and numerically validated using the validation dataset, yielding an overall accuracy higher than 90 %.
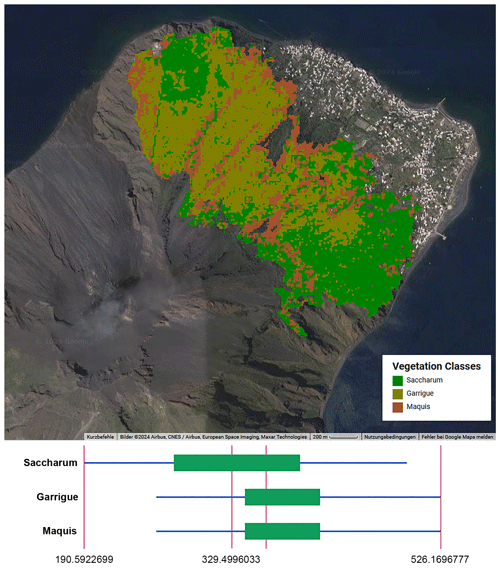
Figure 3The top panel shows a supervised classification of vegetation classes in the study area, overlaid on a Google Earth base map (© Google Earth 2024, CNES/Airbus, European Space Imaging, Maxar Technologies). The bottom panel shows a box plot showing the distribution of dNBR values per vegetation class, evaluated on the image composites from acquisitions in the periods of 15 April–22 May and 26 May–15 June 2022. Boxes and whiskers correspond to 1 and 2 standard deviations, accounting for 68 % and 95 % of the processed values, respectively. The fire that occurred in garrigue and maquis was estimated to be the most severe.
To check variations in the distribution of burn severity levels, and to evaluate the short-term response after a fire among different vegetation types, the pixel values of the dNBR were randomly sampled at 50 random points for each of the three vegetation classes described above. Levene's test was used to assess the homogeneity of variance followed by the nonparametric Kruskal–Wallis test, using chi-square distribution (right-tailed) and Dunn's post hoc comparison to reject the null hypothesis.
To evaluate the short-term vegetation response after a fire, the composite images of Sentinel-2 acquisitions from the following time ranges were analyzed: 15–17 August 2022, 14–26 September 2022, 22–28 October 2022, and 10 May–15 June 2023.
On-site surveys were carried out on 15–19 September 2022, 7–9 March 2023, and 9–12 September 2023 in order to validate the remotely sensed data and to sample vegetation plots in the burned area. The vegetation was sampled in 38 plots, 10 m2 each, and randomly selected along a belt between 180 and 220 m a.s.l. (Fig. 1). To optimize the sampling effort, the location of the sampling sites deviated little from the paths that run along the volcano's flank above the villages of San Vincenzo and San Bartolo. The only rules adopted were that the plots should have been at least 50 m apart in order to avoid spatial autocorrelation and that each of the abovementioned three vegetation classes should have been represented by at least 10 plots. Vegetation data were collected using a modified Braun-Blanquet (1964) approach by visually estimating the cover–abundance in percentage values and by measuring the mean and maximum height (in cm) of each species.
In order to collect useful information to better understand the interaction between Saccharum and fire, a comparative evaluation of stem density per square meter in burned vs. unburned patches was carried out in the field on 18 September 2022. Sampling plots 1 × 1 m were located every 100 m along two almost-contiguous transects, which were 900 m long, 10 inside the burned area, above the village of San Vincenzo, and 10 outside the burned area, in the bottom part of Rina Grande (Fig. 1). In each plot, the number of stems of Saccharum was counted and the average and maximum height was recorded. In the unburned patches, the relative percentage of dry stems compared to green stems was also assessed in order to showcase the ease of fire ignition due to the abundant presence of dry biomass, consisting mainly of the flowering stems of Saccharum which, once faded, dry out completely but remain standing as they are supported by the green stems which have not yet flowered.
The application of the dNBR yielded a severity map showing the difference between pre-fire and postfire acquisitions. The burned area was quantified in 337.83 ha, corresponding to 27.7 % of the island surface (Fig. 2). Concerning the burn severity (Keeley, 2009), 75.15 ha showed a low severity level, 218.37 ha showed an intermediate severity level, and 44.31 ha showed a high severity level. The Kruskal–Wallis H test indicated a significant difference in the distribution of severity levels among vegetation classes (χ2(2)=8.56, p=0.013), where the burned garrigue and maquis suffered higher-severity damage than Saccharum (Fig. 3).
We found no correlation between the dNBR and neither the elevation nor the slope (therefore not reported here). NDVI values were strongly correlated with dNBR values (Pearson correlation of 0.97; see Sect. S2). However, NDVI showed some noise in the estimation of vegetation loss and false positives scattered across the inhabited area. Therefore, these results are not reported further in this paper, despite the NDVI having a true resolution of 10 m in Sentinel-2 products, while NBR employs the SWIR band, which is originally at a 20 m GSD and therefore interpolated.
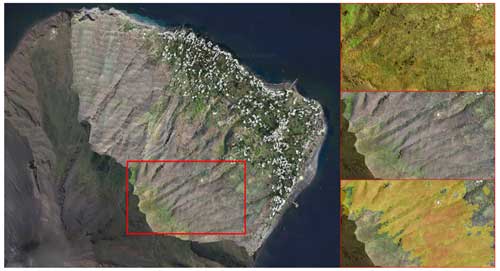
Figure 4The left panel shows a high-resolution drone image, overlaid on a high-resolution image from a Google Earth base map, acquired on 17 August 2022 to assess the quality of the information derived from the dNBR analysis. The top-right panel shows pre-fire detail from a Google Earth base map. The middle-right panel shows postfire detail from a drone image. The bottom-right panel shows the same detail with overlaid thresholded dNBR values higher than 0.19 (using pre-fire and the August 2022 scene), and it is semitransparent for visual comparison (yellow: low-severity damage, orange: middle-severity damage, and red: high-severity damage). Credits of the drone images: Antonio Zimbone. Credits for Google base map: © Google Earth 2024, CNES/Airbus, European Space Imaging, Maxar Technologies.
Considering the limitations imposed on spatial resolution by the satellite-derived damage evaluation, the burned area detected by the dNBR from the mosaic of Sentinel-2 acquisitions in the time range of 15–17 August 2022 well matched the burned area observable in the drone image acquired on 17 August, with humanmade structures and even single trees that were spared by the fire correctly regarded as undamaged in the dNBR estimation (Fig. 4). At the same time, partially burned vegetated areas were correctly included in dNBR results because even if they did not burn completely, a steep decrease in the red edge portion of the spectrum around 700 nm revealed strong vegetative stress.
The NDVI calculated with a threshold of 0.08, therefore quantifying all pixels having at least 8 % covered by photosynthetically active vegetation, quantified the area of the island covered by vegetation before the fire as 678.73 ha. Considering the described correlation between the dNBR and NDVI, and the area affected by the fire as computed by the dNBR, it can be concluded that roughly half (49.8 %) of the vegetated area of Stromboli was burned during the fire event.
Figure 5 shows the vegetation recovery in the area affected by the fire. According to the thresholds suggested by Key and Benson (2006) to categorize recovery levels from dNBR values, in the specific enhanced low and high regrowth for dNBR values ranging from −100 to −250 and smaller than −250, respectively, 1 year after the fire, 53.25 % of the burned area showed a high enhanced recovery, 30.84 % showed a low recovery, and 15.9 % showed no recovery. Among the three vegetation classes considered, 56.08 % of the pixels with high recovery levels were Saccharum, 38.2 % were garrigue, and 5.7 % were maquis. Conversely, 10.46 % of the areas with no recovery were maquis, 65.48 % were garrigue, and 23.86 % were Saccharum. Considering the distribution of recovery levels across the first growing season after the fire, Saccharum is clearly characterized by a faster recovery with respect to the maquis and the garrigue, particularly at the beginning of the first growing season after the fire (September–October 2022).
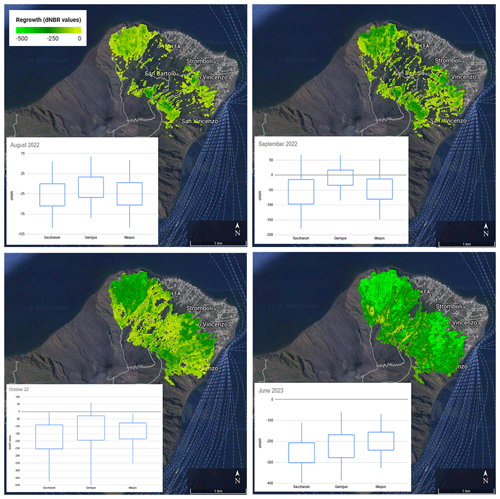
Figure 5Vegetation recovery in the area affected by the fire, estimated through dNBR values from different acquisitions of Sentinel-2 images, overlaid on a Google Earth base map (© Google Earth 2024, CNES/Airbus, European Space Imaging, Maxar Technologies). Box plots show the distribution of dNBR values associated with recovery in the areas occupied by Saccharum, garrigue, and maquis. Boxes and whiskers correspond to 1 and 2 standard deviations, accounting for 68 % and 95 % of the processed values, respectively. The following thresholds were suggested by Key and Benson (2006) to categorize levels of recovery from dNBR values rescaled by 1000: no change from 0 to −100, low enhanced recovery from −100 to −250, and high enhanced recovery (high) from −250. Saccharum is characterized by faster recovery than the maquis and the garrigue, particularly at the beginning of the first growing season after the fire (September–October 2022).
Referring to the vegetation recovery estimated in October 2022, the Kruskal–Wallis H test indicated that there is a significant difference among the vegetation classes (χ2(2)=8.41, p=0.015) with a mean rank score of 64.06 for Saccharum, 89 for garrigue, and 73.44 for maquis. The post hoc Dunn's test using a Bonferroni-corrected alpha of 0.017 indicated significant differences of Saccharum recovery towards both maquis and garrigue (Table 1).
Table 1Dunn's post hoc comparison for the dNBR-estimated recovery of the considered vegetation classes in the burned area on October 2022.

The results of the spectral evaluation of the vegetation recovery are confirmed by the on-site surveys. Table 2 shows the median values of percentage cover and height of resprouts and seedlings in the plots sampled in September 2022 and March and September 2023. The distribution of the plots across the vegetation classes was the following: 10 Saccharum, 16 garrigue, and 12 maquis. The Kruskal–Wallis H test indicated highly significant differences (p<0.001) between the cover values and height of resprouts and the cover of seedlings in the Saccharum plots compared to those ascribed to the other two vegetation classes. No significant difference was found in the height of the seedlings or even in the composition of the species across the vegetation classes (data not shown), which in all cases were largely dominated by annual plants such as Brassica fruticulosa, Ornithopus compressus, Lupinus angustifolius, Trifolium stellatum, and seedlings of Cistus sp. pl. (mainly Cistus creticus).
Table 2Median values of cover (%) and height (cm) of resprouts and seedlings in the validation plots. Values in parentheses indicate positive absolute deviations from the median values.
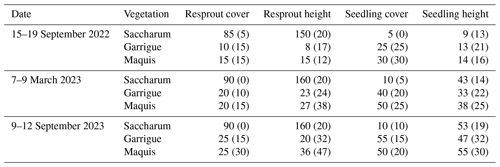
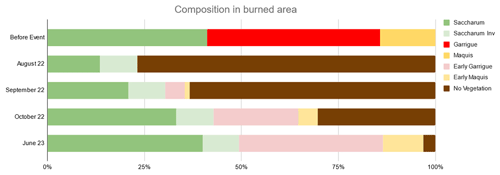
Figure 6Estimated vegetation composition in the study area (cover %). “Saccharum” vegetation patches occupied by Saccharum both before and after fire; “Saccharum Inv” sums the surface areas previously occupied by other vegetation units and invaded by Saccharum after fire. “Early garrigue” and “Early maquis” refer to early postfire successional stages of these two vegetation classes, dominated by annual plants and resprouted shrubs and seedlings of perennial seeders, chiefly Cistus sp. pl.
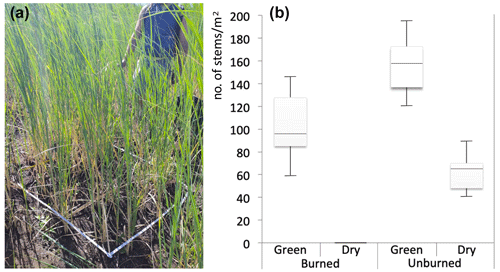
Figure 7(a) Measuring resprouted Saccharum biflorum stem density in one of the plots within the burned area (18 September 2022; photo by Riccardo Guarino). (b) Box plots of the stem density of Saccharum in burned and unburned patches.
The estimated vegetation composition in the study area shows that already in August, resprouting Saccharum had invaded approximately 13 % of areas previously occupied by other vegetation classes, especially along gullies. This latter percentage remained almost unchanged in the following months (Fig. 6). The fast recovery of the Saccharum patches, with their soft-green color standing out against the surrounding black, became evident as early as a few weeks after the fire (Sect. S1, Figs. S3–S5). Until the first rains, which occurred on the night of 12 August 2022, Saccharum was the only green spot in the fire-affected areas, and the high-resolution drone images captured on 17 August 2022 clearly show all Saccharum patches in their recovery phase (Fig. 4). In the Sentinel-2 images from September–October 2022, previous damage from the fire event appears to be mitigated. In more detail, a total of 110 ha of the previously burned area (roughly one-third) exhibit a dNBR value below −0.1, which represents a strong indicator of vegetation recovery. This was mostly due to Saccharum, demonstrating that this species can exert unchallenged dominance in the early stages of the postfire dynamics (succession), reaching vegetative stem densities only slightly lower than those of the unburned stands in a short time (Fig. 7).
Our study confirms that fire severity can be mapped with high accuracy using indices derived from Sentinel-2 imagery with supervised vegetation classifications based on spectral information (Gibson et al., 2020). Fire has been a major driving force for Mediterranean insular ecosystem dynamics since the emergence of the Mediterranean climate (Médail, 2021), particularly in volcanic island ecosystems (Irl et al., 2014). This paper provides the first report of how a single fire event significantly affected Stromboli island, burning 50 % of the vegetated island surface. This clearly influenced the island biota, particularly the native vegetation, which is rich in species of relevant biogeographic interest, such as Centaurea aeolica, Genista tyrrhena, Dianthus rupicola subsp. aeolicus, and Jacobaea maritima subsp. bicolor (Pasta et al., 2024). In addition, the highest and southernmost end of the study area included part of the Cytisus aeolicus population, one of the rarest and most emblematic endemic plant species of the Aeolian archipelago (Zaia et al., 2020).
Although we applied a permissive threshold (8 %) in the NDVI for our quantitative analysis, our conclusion that the fire that occurred on 25–26 May 2022 burned roughly half of Stromboli's vegetated area appears reasonably accurate when considering all the available data we used for validation. Our study confirms that burn severity levels, estimated by the dNBR, are higher in woody vegetation (Koutsias and Karteris, 2002), presumably due to the larger aboveground biomass and dead organic matter stock in the case of maquis (Rossetti et al., 2022) and to the high flammability of Mediterranean dwarf shrubs in the case of garrigue (Dimitrakopoulos, 2001). Despite the garrigue being mostly formed by pyrophytes and obligate seeders, and despite it being among the first shrubs to emerge after a fire (Palá-Paúl, 2005; Athanasiou et al., 2023), our study demonstrated that Saccharum exhibits even greater resilience compared to garrigue in the earliest stages after a fire, with a clear risk of altering the recovery patterns of native vegetation, which, especially on volcanic islands, is characterized by a high abundance of nitrogen fixers and annual species (Weiser et al., 2021).
The positive interaction between Saccharum and fire was already noticed in Stromboli by Richter (1984) and Richter and Lingenhöhl (2002). Fire spreads very easily across Saccharum vegetation due to the abundant presence of standing dry biomass (Sect. S1, Figs. S2, S4, S6). This result agrees with many recent studies focused on the role of fire as a promoter of C4 grasses (Scheiter et al., 2012; Hoetzel et al., 2013; Ripley et al., 2015). Although the native rockrose garrigue vegetation is also adapted to, and favored by, periodical fires (Pausas, 1999), its survival derives from the ability of Cistus to develop a long-lasting soil seed bank (Roy and Sonie, 1992; Scuderi et al., 2010). Too frequent fire events and runoff caused by heavy rainfall on sandy and incoherent soils may cause a critical depletion of the soil seed bank and favor sprouters against obligate seeders. For this purpose, we must point out that the autochthonous sprouters (such as Erica arborea, Pistacia lentiscus, and Olea europaea) have a slower growth rate than Saccharum and need a longer time to become established.
After the fire, our study area was exposed to full solar radiation; dark sandy surfaces were subject to extreme microclimatic (surface temperatures up to 80 °C; see Richter, 1984) and dry conditions. These were not favorable for the germination of the soil seed bank since sprouters faced almost no competition until the first rains, which occurred on 12 August 2022. The first and most important beneficiary of these contrasting conditions was Saccharum, which, over time, was able to colonize large surfaces of tephra in the northern and eastern parts of the island, likely due to a positive interaction between land abandonment, repeated fires, and volcanic ash deposition. Saccharum is extremely competitive thanks to a variety of functional strategies (e.g., C4 photosynthetic pathway, large resource allocation into clonal and bud-bearing rhizomes which ensures a quick resprouting, space occupancy, and resource uptake) under current and probably also under predicted conditions (likely more disturbed), which could affect and define different ecosystems on Stromboli.
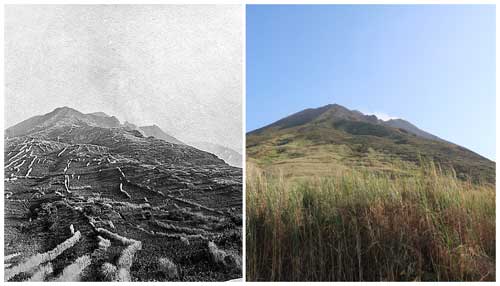
Figure 8The left panel shows a historical photo of terraced vineyards on Stromboli (1891; anonymous photographer) with rows of Saccharum biflorum used as windbreaks. The right panel shows the same view 130 years later (16 July 2021; photo by Pietro Lo Cascio).
According to Lojacono (1878), Saccharum was planted along the vineyards to shelter them from the northerly winds (Fig. 8). This condition lasted until the eruption on 11 September 1930, which is considered, so far, to be the most violent and destructive event in the historical records of Stromboli's activity (Rittmann, 1931). Facilitated by the winter rains and by a rapid expansion via rhizomes, Saccharum first benefited from the emigration of most inhabitants and subsequent abandonment of terraced fields, which, in a very short time lapse, were almost completely sealed off by a dense monospecific bed; this made it difficult for other species to establish themselves (Ferro and Furnari, 1968; Richter, 1984). Since then, competition for space between local native vegetation and Saccharum beds has been regulated mainly by the periodical occurrence of fires. Further studies are needed to understand the duration of the Saccharum expansion phases. Our preliminary results suggest that the expansion of Saccharum is surprisingly fast, but the decline may also be relatively rapid. There are no data on the longevity of Saccharum rhizomes and related senescence processes or on the effects of volcanic ash deposition on rhizome burial. However, there are reasonable indications that, if the vegetation is not too frequently affected by fire, Saccharum could be gradually replaced by native vegetation within a few decades, as captured in the maps published as “Fig. 4” by Richter and Lingenhöhl (2002).
On 12 August 2022, a severe thunderstorm triggered disastrous erosion processes over the entire area affected by the fire on 25–26 May. Large quantities of mud, stones, and volcanic ash flooded the streets in the villages of San Bartolo and San Vincenzo (Sect. S1, Fig. S7). In the burned area, the traces of runoff and surface rill erosion were still very evident during our inspections on 18–19 September 2022. However, just as evident was the ambivalent role of Saccharum, which, on the one hand, clearly prevails over native species and, on the other hand, thanks to its dense mat of rhizomes, proves to be much more efficient than the burned native vegetation in counteracting hydrogeological instability. The latter is a very relevant aspect on a volcanic island where the soils are largely made up of loose tephra ashes.
Over time, Saccharum beds have become an important secondary habitat for many animal species. In fact, they represent the main breeding site for at least 70 % of breeding bird species on Stromboli (Massa et al., 2015) and host conspicuous populations of almost all terrestrial vertebrates occurring on the island (especially Tarentola mauritanica, Podarcis siculus, and Hierophis viridiflavus). Some of the invertebrates that are found in the Saccharum beds are of considerable biogeographic interest, such as Caulostrophus zancleanus, a regional endemic (Lo Cascio et al., 2022), and the recently described Catomus aeolicus, an endemic of the northeastern sector of the Aeolian archipelago (Ponel et al., 2020). Although not specialized on Saccharum, the rhizophagous larvae of the melolonthid Anoxia orientalis, a species considered rare at the national scale in Italy, feed on its rhizomes. Surprisingly enough, S. biflorum does not seem to be an attractive fodder for the mammals introduced in historical (Oryctolagus cuniculus) or more recent (Capra hircus) times nor have significant infestations of phytophagous insects ever been observed. Thus, herbivory does not seem to be a limiting factor to the expansion of Saccharum on Stromboli.
Remotely sensed data provide fast, accurate, and reliable information for postfire damage analysis, being spectrally sensitive to vegetation features and structure. Multitemporal data acquisition allows observations on early-stage vegetation dynamics which, in our case, point out the outstanding pioneer role played by Saccharum biflorum, showcasing its ability to colonize and dominate large areas, potentially altering the recovery patterns of native vegetation. On the other hand, Saccharum proves to be efficient in stabilizing the soil, especially on a volcanic island with loose tephra ashes, thus mitigating the erosion processes. Our findings underscore the complex interplay between fire, vegetation dynamics, and ecosystem recovery on Stromboli, emphasizing the need for further research to better understand the long-term dynamics of Saccharum expansion, its interactions with native biota, and its potential use in environmental restoration. While considering the fragility of the context, considering that Saccharum is already widespread on the island, its rhizomes could be used to contain the disastrous effects of erosion caused by rainfall, later supporting the biological succession through the manual thinning of Saccharum culms and the sowing of the native woody species typical of local garrigue.
In fact, although the expansion of Saccharum proves to be surprisingly fast, its decline may also be relatively rapid as well if local vegetation is no longer affected by fire. After the abandonment of the agricultural practices in the highest portion of the island, the rewilding process could lead to the replacement of the large beds dominated by this invasive grass by native woody vegetation within a few decades.
The code is available as a Google Earth Engine application (in the cloud) on demand by contacting the authors.
The optical satellite data used in this study are open and available as Copernicus Sentinel-2 (ESA, 2021; https://doi.org/10.5270/S2_-znk9xsj). Furthermore, the data are accessible on the cloud through the Google Earth Engine environment (https://earthengine.google.com, Google Earth Enginge, 2024).
The supplement related to this article is available online at: https://doi.org/10.5194/bg-21-2717-2024-supplement.
RG and DC developed the research idea, DC processed the satellite and drone imagery, RG and RZ conducted the fieldwork, RG led the writing process, and all authors discussed the results and contributed to the manuscript.
The contact author has declared that none of the authors has any competing interests.
Publisher's note: Copernicus Publications remains neutral with regard to jurisdictional claims made in the text, published maps, institutional affiliations, or any other geographical representation in this paper. While Copernicus Publications makes every effort to include appropriate place names, the final responsibility lies with the authors.
This article is part of the special issue “The role of fire in the Earth system: understanding interactions with the land, atmosphere, and society (ESD/ACP/BG/GMD/NHESS inter-journal SI)”. It is a result of the EGU General Assembly 2020, 4–8 May 2020.
We would like to thank Giuseppe De Rosa, who brought Daniele Cerra and Riccardo Guarino together, and Antonio Zimbone for driving the drone flight and taking the pictures used to check the quality of the information derived from the dNBR analysis. Gianluigi Ottaviani and three anonymous reviewers are gratefully acknowledged for their suggestions on an earlier version of the manuscript.
This research has been supported by the Ministero dell'Istruzione e del Merito (grant no. PRIN B53D23032530001).
This paper was edited by Kirsten Thonicke and reviewed by three anonymous referees.
Amalraj, V. A., and Balasundaram, N.: On the taxonomy of the members of “Saccharum complex”, Genet. Resour. Crop Ev., 53, 35–41, https://doi.org/10.1007/s10722-004-0581-1, 2006.
Amani, M., Ghorbanian, A., Ahmadi, S. A., Kakooei, M., Moghimi, A., Mirmazloumi, S. M., Moghaddam, S. A. H., Mahdavi, S., Ghahremanloo, M., Parsian, S., Wu, Q., and Brisco, B.: Google earth engine cloud computing platform for remote sensing big data applications: A comprehensive review, IEEE J. Sel. Top. Appl., 13, 5326–5350, https://doi.org/10.1109/JSTARS.2020.3021052, 2020.
Athanasiou, M., Martinis, A., Korakaki, E., and Avramidou, E. V.: Development of a Fuel Model for Cistus spp. and Testing Its Fire Behavior Prediction Performance, Fire, 6, 247, https://doi.org/10.3390/fire6070247, 2023.
Baudena, M., Santana, V. M., Baeza, M. J., Bautista, S., Eppinga, M. B., Hemerik, L., Garcia Mayor, A., Rodriguez, F., Valdecantos, A., Vallejo, V. R., Vasques, A., and Rietkerk, M.: Increased aridity drives post-fire recovery of Mediterranean forests towards open shrublands, New Phytol., 225, 1500–1515, https://doi.org/10.1111/nph.16252, 2020.
Bazan, G., Marino, P., Guarino, R., Domina, G., and Schicchi, R.: Bioclimatology and vegetation series in Sicily: A geostatistical approach, Ann. Bot. Fenn., 52, 1–18, https://doi.org/10.5735/085.052.0202, 2015.
Bergmeier, E., Capelo, J., Di Pietro, R., Guarino, R., Kavgacı, A., Loidi, J., Tsiripidis, J., and Xystrakis, F.: “Back to the Future” – Oak wood-pasture for wildfire prevention in the Mediterranean, Plant Sociology, 58, 41–48, https://doi.org/10.3897/pls2021582/04, 2021.
Bellard, C., Cassey, P., and Blackburn, T. M.: Alien species as a driver of recent extinctions, Biol. Lett., 12, 20150623, https://doi.org/10.1098/rsbl.2015.0623, 2016.
Bond, W. J. and Wilgen, B. W.: Why and how do ecosystems burn?, Fire and plants: Population and Community Biology Series 14, 16–33, Springer, Dordrecht, https://doi.org/10.1007/978-94-009-1499-5, 1996.
Braun-Blanquet, J.: Pflanzensoziologie, Grundzüge der Vegetationskunde, 3rd Edn., Springer-Verlag, Berlin, Germany, 631 pp., https://doi.org/10.1007/978-3-7091-8110-2, 1964.
Brooks, M. L., D'Antonio, C. M., Richardson, D. M., Di Tomaso, J. M., Grace, J. B., Hobbs, R. J., Keeley, J. E., Pellant, M., and Pyke, D.: Effects of invasive alien plants on fire regimes, Bioscience, 54, 677–688, https://doi.org/10.1641/0006-3568(2004)054[0677:EOIAPO]2.0.CO;2, 2004.
Canelles, Q., Aquilué, N., Duane, A., and Brotons, L.: From stand to landscape: modelling post-fire regeneration and species growth, Ecol. Model., 404, 103–111, https://doi.org/10.1016/j.ecolmodel.2019.05.001, 2019.
Cavallaro, F., Morabito, M., Navarra, E., Pasta, S., Lo Cascio, P., Campanella, P., Cavallaro, M., Cavallaro, A., Merenda, A., Di Procolo, G., Rühl, J., and Ioppolo, G.: Piano di Gestione dei Siti Natura 2000 delle Isole Eolie, Regione Siciliana, Assessorato Territorio e Ambiente, 2009.
Celesti-Grapow, L., Bassi, L., Brundu, G., Camarda, I., Carli, E., D'Auria, G., Del Guacchio, E., Domina, G. Ferretti, G., Foggi, B., Lazzaro, L., Mazzola, P., Peccenini, S., Pretto, F., Stinca, A., and Blasi, C.: Plant invasions on small Mediterranean islands: An overview, Plant Biosyst., 150, 1119–1133, https://doi.org/10.1080/11263504.2016.1218974, 2016.
Cervellini, M., Zannini, P., Di Musciano, M., Fattorini, S., Jiménez-Alfaro, B., Rocchini, D., Field, R., Vetaas O. R., Irl, S. D. H., Beierkuhnlein, C., Hoffmann, S., Fischer, J.-C., Casella, L., Angelini, P., Genovesi, P., Nascimbene, J., and Chiarucci, A.: A grid-based map for the Biogeographical Regions of Europe, Biodiversity Data Journal, 8, e53720, https://doi.org/10.3897/BDJ.8.e53720, 2020.
Chiarucci, A., Guarino, R., Pasta, S., La Rosa, A., Lo Cascio, P., Médail, F., Pavon, D., Fernández-Palacios, J. M., and Zannini, P.: Species-area relationship and small-island effect of vascular plant diversity in a young volcanic archipelago, J. Biogeogr., 48, 2919–2931, https://doi.org/10.1111/jbi.14253, 2021.
Dimitrakopoulos, A. P.: A statistical classification of Mediterranean species based on their flammability components, Int. J. Wildland Fire, 10, 113–118, https://doi.org/10.1071/WF01004, 2001.
Driscoll, D. A., Armenteras, D., Bennett, A. F., Brotons, L., Clarke, M. F., Doherty, T. S., Haslem, A., Kelly, L. T., Sato, C. F., Sitters, H., Aquilué, N., Bell, K., Chadid, M., Duane, A., Meza-Elizalde, M. C., Giljohann, K. M., González, T. M., Jambhekar, R., Lazzari, J., Morán-Ordóñez, A., and Wevill, T.: How fire interacts with habitat loss and fragmentation, Biol. Rev., 96, 976–998, https://doi.org/10.1111/brv.12687, 2021.
Drusch, M., Del Bello, U., Carlier, S., Colin, O., Fernandez, V., Gascon, F., Hoersch, B., Isola, C., Laberinti, P., Martimort, P., Meygret, A., Spoto, F., Sy, O., Marchese, F., and Bargellini, P.: Sentinel-2: ESA's optical high-resolution mission for GMES operational services, Remote Sens. Environ., 120, 25–36, https://doi.org/10.1016/j.rse.2011.11.026, 2012.
ESA: Copernicus Sentinel-2 Collection 1 MSI Level-2A (L2A), https://doi.org/10.5270/S2_-znk9xsj, ESA [data set], 2021.
Ferro, G. and Furnari, F.: Flora e vegetazione di Stromboli (Isole Eolie), Archivio Botanico e Biografico Italiano, 44, 21–45, 1968.
Fois, M., Podda, L., Médail, F., and Bacchetta, G.: Endemic and alien vascular plant diversity in the small Mediterranean islands of Sardinia: Drivers and implications for their conservation, Biol. Conserv., 244, 108519, https://doi.org/10.1016/j.biocon.2020.108519, 2020.
Fornaciai, A., Bisson, M., Landi, P., Mazzarini, F., and Pareschi, M. T.: A LiDAR survey of Stromboli volcano (Italy): Digital elevation model-based geomorphology and intensity analysis, Int. J. Remote Sens., 31, 3177–3194, https://doi.org/10.1080/01431160903154416, 2010.
Francalanci, L., Lucchi, F., Keller, J., De Astis, G., and Tranne, C. A.: Eruptive, volcanotectonic and magmatic history of the Stromboli volcano (northeastern Aeolian archipelago), in: The Aeolian Islands Volcanoes, edited by: Lucchi, F., Peccerillo, A., Keller, J., Tranne, C. A., and Rossi, P. L., Geological Society of London, Memoirs, 37, 397–471, 2013.
Gibson, R., Danaher, T., Hehir, W., and Collins, L.: A remote sensing approach to mapping fire severity in south-eastern Australia using sentinel 2 and random forest, Remote Sens. Environ., 240, 111702, https://doi.org/10.1016/j.rse.2020.111702, 2020.
Goberna, M., García, C., Insam, H., Hernández, M. T., and Verdú, M.: Burning fire-prone Mediterranean shrublands: immediate changes in soil microbial community structure and ecosystem functions, Microbial Ecol., 64, 242–255, https://doi.org/10.1007/s00248-011-9995-4, 2012.
Google Earth Enginge: A planetary-scale platform for Earth science data & analysis, Google Earth Enginge [data set], https://earthengine.google.com, last access: 4 June 2024.
Gussone, G.: Supplementum ad Florae Siculae Prodromum, Edit. Tramater, Neapoli, 314 pp., 1832.
Gandhi, M., Parthiban, S., Thummalu, N., and Christy, A.: NDVI: Vegetation change detection using remote sensing and GIS – A case study of Vellore District, Procedia Computing Science, 57, 1199–1210, https://doi.org/10.1016/j.procs.2015.07.415, 2015.
Guyette, R. P., Muzika, R. M., and Dey, D. C.: Dynamics of an anthropogenic fire regime, Ecosystems, 5, 472–486, https://doi.org/10.1007/s10021-002-0115-7, 2002.
Harris, R. M., Remenyi, T. A., Williamson, G. J., Bindoff, N. L., and Bowman, D. M.: Climate–vegetation–fire interactions and feedbacks: trivial detail or major barrier to projecting the future of the Earth system?, WIRes Clim. Change, 7, 910–931, https://doi.org/10.1002/wcc.428, 2016.
Hearst, M. A., Dumais, S. T., Osman, E., Platt, J., and Schölkopf, B.: Support vector machines, IEEE Intelligent Systems and their applications, 13, 18–28, 1998.
Hijmans, R. J., Cameron, S. E., Parra, J. L., Jone, P. G., and Jarvis, A.: Very high resolution interpolated climate surfaces for global land areas, Int. J. Climatol., 25, 1965–1978, https://doi.org/10.1002/joc.1276, 2005.
Hoetzel, S., Dupont, L., Schefuß, E., Rommerskirchen, F., and Wefer, S.: The role of fire in Miocene to Pliocene C4 grassland and ecosystem evolution, Nat. Geosci., 6, 1027–1030, https://doi.org/10.1038/ngeo1984, 2013.
IPCC: Climate Change 2021: The Physical Science Basis. Contribution of Working Group I to the Sixth Assessment Report of the Intergovernmental Panel on Climate Change, edited by: Masson-Delmotte, V., Zhai, P., Pirani, A., Connors, S. L., Péan, C., Berger, S., Caud, N., Chen, Y., Goldfarb, L., Gomis, M. I., Huang, M., Leitzell, K., Lonnoy, E., Matthews, J. B. R., Maycock, T. K., Waterfield, T., Yelekçi, O., Yu, R., and Zhou, B., Cambridge University Press, Cambridge, United Kingdom and New York, NY, USA, 2391 pp., https://doi.org/10.1017/9781009157896, 2021.
Irl, S. D. H., Steinbauer, M. J., Messinger, J., Blume-Werry, G., Palomares-Martínez, Á., Beierkuhnlein, C., and Jentsch, A.: Burned and devoured – Introduced herbivores, fire, and the endemic flora of the high-elevation ecosystem on La Palma, Canary Islands, Arct. Antarct. Alp. Res., 46, 859–869, https://doi.org/10.1657/1938-4246-46.4.859, 2014.
Jouffroy-Bapicot, I., Pedrotta, T., Debret, M., Field, S., Sulpizio, R., Zanchetta, G., Sabatier, P., Roberts, N., Tinner, W., Walsh, K., and Vannière, B.: Olive groves around the lake. A ten-thousand-year history of a Cretan landscape (Greece) reveals the dominant role of humans in making this Mediterranean ecosystem, Quaternary Sci. Rev., 267, 107072, https://doi.org/10.1016/j.quascirev.2021.107072, 2021.
Karadimou, E., Tsiripidis, I., Kallimanis, A. S., Raus, T., and Dimopoulos, P.: Functional diversity reveals complex assembly processes on sea-born volcanic islands, J. Veg. Sci., 26, 501–512, https://doi.org/10.1111/jvs.12255, 2015.
Keeley, J. E.: Fire intensity, fire severity and burn severity: a brief review and suggested usage, Int. J. Wildland Fire, 18, 116–126, https://doi.org/10.1071/WF07049, 2009.
Keeley, J. E. and Brennan, T. J.: Fire-driven alien invasion in a fire-adapted ecosystem, Oecologia, 169, 1043–1052, https://doi.org/10.1007/s00442-012-2253-8, 2012.
Key, C. H. and Benson N. C.: Landscape assessment (LA) sampling and analysis methods, USDA Forest Service, Rocky Mountain Research Station, General Technical Report RMRS-GTR-164-CD, 2006.
Koutsias, N. and Karteris, M.: Classification analyses of vegetation for delineating forest fire fuel complexes in a Mediterranean test site using satellite remote sensing and GIS, Int. J. Remote Sens., 24, 3093–3104, https://doi.org/10.1080/0143116021000021152, 2002.
Le Houérou, H. N.: Land degradation in Mediterranean Europe: can agroforestry be a part of the solution? A prospective review, Agroforest. Syst., 21, 43–61, https://doi.org/10.1007/BF00704925, 1993.
Lentile, L. B., Holden, Z. A., Smith, A. M. S., Falkowski, M. J., Hudak, A. T., Morgan, P., Lewis, S. A., Gessler, P. E., and Benson, N. C.: Remote sensing techniques to assess active fire characteristics and post-fire effects, Int. J. Wildland Fire, 15, 319–345, https://doi.org/10.1071/WF05097, 2006.
Lo Cascio, P., Ponel, P., and Altadonna, G.: Diversity and distribution of beetles in a Mediterranean volcanic archipelago: an updated checklist of the Coleoptera on the Aeolian Islands (Sicily, Italy), Biodivers. J., 13, 531–585, https://doi.org/10.31396/Biodiv.Jour.2022.13.3.531.585, 2022.
Lojacono, M.: Le Isole Eolie e la loro vegetazione, con enumerazione delle piante spontanee vascolari, Palermo, Lorsnaider, 147 pp., 1878.
Lozano, O. M., Salis, M., Ager, A. A., Arca, B., Alcasena, F. J., Monteiro, A. T., Finney, M. A., Del Giudice, L., Scoccimarro, E., and Spano, D.: Assessing climate change impacts on wild fire exposure in Mediterranean areas, Risk Anal., 37, 1898–1916, https://doi.org/10.1111/risa.12739, 2017.
Mantero, G., Morresi, D., Marzano, R., Motta, R., Mladenoff, D. J., and Garbarino, M.: The influence of land abandonment on forest disturbance regimes: a global review, Landscape Ecol., 35, 2723–2744, https://doi.org/10.1007/s10980-020-01147-w, 2020.
Massa, B., Lo Cascio, P., Ientile, R., Canale, E., and La Mantia, T.: Gli uccelli delle isole circumsiciliane, Naturalista siciliano, 39, 105–373, 2015.
Médail, F.: Plant Biogeography and Vegetation Patterns of the Mediterranean Islands, Bot. Rev., 88, 63–129, https://doi.org/10.1007/s12229-021-09245-3, 2021.
Moriondo, M., Good, P., Durao, R., Bindi, M., Giannokopoulos, C., and Core-Real, J.: Potential impact of climate change on fire risk in the Mediterranean area, Clim. Res., 31, 85–95, https://doi.org/10.3354/cr031085, 2006.
Novara, A., Gristina, L., Rühl, J., Pasta, S., D'Angelo, G., La Mantia, T., and Pereira, P.: Grassland fire effect on soil organic carbon reservoirs in a semiarid environment, Solid Earth, 4, 381–385, https://doi.org/10.5194/se-4-381-2013, 2013.
Palá-Paúl, J., Velasco-Negueruela, A., Pérez-Alonso, M. J., and Sanz, J.: Seasonal variation in chemical composition of Cistus albidus L. from Spain, J. Essent. Oil Res., 17, 19–22, https://doi.org/10.1080/10412905.2005.9698818, 2005.
Pasta, S., Ardenghi, N. M. G., Badalamenti, E., La Mantia, T., Livreri Console, S., and Parolo, G.: The alien vascular flora of Linosa (Pelagie Islands, Strait of Sicily): update and management proposals, Willdenowia, 47, 135–144, https://doi.org/10.3372/wi.47.47205, 2017.
Pasta, S., Guarino, R., La Rosa, A., Lo Cascio, P., Chiarucci, A., Médail, F., Pavon, D., Zannini, P., and Fernández-Palacios, J. M., Tentamen Florae Aeolicae: comprehensive checklist and biogeographic analysis of the vascular flora of the Aeolian Archipelago (Sicily, Italy), Mediterranean Botany, submitted, 2024.
Pauchard, A., García, R. A., Pena, E., González, C., Cavieres, L. A., and Bustamante, R. O.: Positive feedbacks between plant invasions and fire regimes: Teline monspessulana (L.) K. Koch (Fabaceae) in central Chile, Biol. Invasions, 10, 547–553, https://doi.org/10.1007/s10530-007-9151-8, 2008.
Pausas, J. G.: Response of plant functional types to changes in the fire regime in Mediterranean ecosystems: a simulation approach, J. Veg. Sci., 10, 717–722, https://doi.org/10.2307/3237086, 1999.
Pausas, J. G.: Simulating Mediterranean landscape pattern and vegetation dynamics under different fire regimes, Plant Ecol., 187, 249–259, https://doi.org/10.1007/s11258-006-9138-z, 2006.
Pausas, J. G. and Keeley, J. E.: A burning story: the role of fire in the history of life, BioScience, 59, 593–601, https://doi.org/10.1525/bio.2009.59.7.10, 2009.
Pausas, J. G. and Millán, M. M.: Greening and browning in a climate change hotspot: the Mediterranean Basin, BioScience, 69, 143–151, https://doi.org/10.1093/biosci/biy157, 2019.
Picotte, J. J. and Robertson, K. M.: Accuracy of remote sensing wildland fire-burned area in southeastern US Coastal plain habitats, Tall Timb. Fire Ecol., 24, 86–93, 2010.
Pignatti, S., Guarino, R., and La Rosa, M.: Flora d'Italia, 4 vols. Edagricole, Edizioni Agricole di New Business Media, Bologna, ISBN 978-88-506-5242-6 (Vol. 1), 978-88-506-5243-3 (Vol. 2), 978-88-506-5244-0 (Vol. 3), 978-88-506-5245-7 (Vol. 4), 2017–2019.
Ponel, P., Lo Cascio, P., and Soldati, F.: A new species of Catomus Allard, 1876 (Coleoptera: Tenebrionidae: Helopini) from the Aeolian Archipelago (Sicily, Italy), Zootaxa, 4743, 295–300, https://doi.org/10.11646/zootaxa.4743.2.14, 2020.
Richter, M.: Vegetationsdynamik auf Stromboli, Aachener Geographische Arbeiten, 16, 41–110, 1984.
Richter, M. and Lingenhöhl, D.: Landschaftsentwicklung auf den Äolischen Inseln. Betrachtung in verschiedenen Zeitskalen, Geographische Rundschau, 54, 20–26, 2002.
Ripley, B. S., Visser, V., Christin, P.-A., Archibald, S., Martin, T., and Osborne, C.: Fire ecology of C3 and C4 grasses depends on evolutionary history and frequency of burning but not photosynthetic type, Ecology, 96, 2679–2691, https://doi.org/10.1890/14-1495.1, 2015.
Rittmann, M.: Der Ausbruch des Stromboli am 11. September 1930, Zeitschrift für Vulkanologie, 14, 47–77, 1931.
Rivas-Martínez, S.: Clasificación Bioclimática de la Tierra, https://webs.ucm.es/info/cif/book/bioc/global_bioclimatics_2.htm (last access: 28 November 2022), 2004.
Rossetti, I., Cogoni, D., Calderisi, G., and Fenu, G.: Short-Term Effects and Vegetation Response after a Megafire in a Mediterranean Area, Land, 11, 2328, https://doi.org/10.3390/land11122328, 2022.
Roy, J. and Sonie, L.: Germination and population dynamics of Cistus species in relation to fire, J. Appl. Ecol., 29, 647–655, https://doi.org/10.2307/2404472, 1992.
Salis, M., Del Giudice, L., Jahdi, R., Alcasena-Urdiroz, F., Scarpa, C., Pellizzaro, G., Bacciu, V., Schirru, M., Ventura, A., Casula, M., Pedes, F., Canu, A., Duce, P., and Arca, B.: Spatial patterns and intensity of land abandonment drive wildfire hazard and likelihood in Mediterranean agropastoral areas, Land, 11, 1942, https://doi.org/10.3390/land11111942, 2022.
Scheiter, S., Higgins, S. I., Osborne, C. P., Bradshaw, C., Lunt, D., Ripley, B. S., Taylor, L. L., and Beerling, D. J.: Fire and fire-adapted vegetation promoted C4 expansion in the late Miocene, New Phytol., 195, 653–666, https://doi.org/10.1111/j.1469-8137.2012.04202.x, 2012.
Scuderi, D., Di Gregorio, R., Toscano, S., Cassaniti, C., and Romano, D.: Germination behaviour of four mediterranean Cistus L. species in relation to high temperature, Ecological Questions, 12, 171–180, https://doi.org/10.12775/v10090-010-0011-2, 2010.
Soverel, N. O., Perrakis, D. D. B., and Coops, N. C.: Estimating burn severity from Landsat d NBR and Rd NBR indices across western Canada, Remote Sens. Environ., 114, 1896–1909, https://doi.org/10.1016/j.rse.2010.03.013, 2010.
Trabaud, L.: Postfire plant community dynamics in the Mediterranean Basin, in: The Role of Fire in Mediterranean-Type Ecosystems, edited by: Moreno, J. M. and Oechel, W. C., Springer, New York, NY, 1–15, https://doi.org/10.1007/978-1-4613-8395-6_1, 1994.
Velasco, A. G. V., Probanza, A., Mañero, F. G., Treviño, A. C., Moreno, J. M., and Garcia, J. L.: Effect of fire and retardant on soil microbial activity and functional diversity in a Mediterranean pasture, Geoderma, 153, 186–193, https://doi.org/10.1016/j.geoderma.2009.08.005, 2009.
Vallejo, V. R., Allen, E. B., Aronson, J., Pausas, J. G., Cortina, J., and Gutiérrez, J. R.: Restoration of Mediterranean-type woodlands and shrublands, in: Restoration Ecology: The New Frontier, edited by: Andel, J. and Aronson, J., Blackwell Publishing Ltd., Oxford, UK, 130–144, 2012.
Weiser, F., Sauer, A., Gettueva, D., Field R., Irl, S. D. H., Vetaas, O., Chiarucci, A., Hoffmann, S., Fernández-Palacios, J. M., Otto, R., Jentsch, A., Provenzale, A., and Beierkuhnlein, C.: Impacts of forest fire on understory species diversity in Canary pine ecosystems on the island of La Palma, Forests, 12, 1638, https://doi.org/10.3390/f12121638, 2021.
Zaia, R., Pasta, S., Di Rita, F., Laudicina, V. A., Lo Cascio, P., Magri, D., Troia, A., and Guarino, R.: Staying alive on an active volcano: 80 years population dynamics of Cytisus aeolicus (Fabaceae) from Stromboli (Aeolian Islands, Italy), Ecol. Process., 9, 1–15, https://doi.org/10.1186/s13717-020-00262-5, 2020.