the Creative Commons Attribution 4.0 License.
the Creative Commons Attribution 4.0 License.
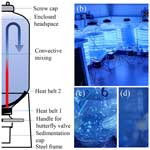
Technical note: Ocean Alkalinity Enhancement Pelagic Impact Intercomparison Project (OAEPIIP)
Lennart Thomas Bach
Aaron James Ferderer
Julie LaRoche
Kai Georg Schulz
Ocean alkalinity enhancement (OAE) aims to transfer carbon dioxide (CO2) from the atmosphere to the ocean by increasing the capacity of seawater to store CO2. The potential effects of OAE-induced changes in seawater chemistry on marine biology must be assessed to understand if OAE, operated at a climate-relevant scale, would be environmentally sustainable. Here, we describe the design of the Ocean Alkalinity Enhancement Pelagic Impact Intercomparison Project (OAEPIIP) – a standardised OAE microcosm experiment with plankton communities to be conducted worldwide. OAEPIIP provides funding for participating laboratories to conduct OAE experiments in their local environments. This paper constitutes a detailed manual on the standardised methodology that shall be adopted by all OAEPIIP participants. The individual studies will provide new insights into how plankton communities respond to OAE. The synthesis of these standardised studies, without publication bias, will reveal common OAE-responses that occur across geographic and environmental gradients and are therefore particularly important to determine. The funding available to OAEPIIP and the resulting data will be shared to maximise their value and accessibility. The globally coordinated effort has potential to promote scientific consensus about the potential effects of OAE on diverse plankton communities. Such consensus, through inclusion of the global community, will provide a sounder base to facilitate political decision making as to whether OAE should be scaled up or not.
- Article
(3430 KB) - Full-text XML
-
Supplement
(492 KB) - BibTeX
- EndNote
Ocean alkalinity enhancement (OAE) is an emerging carbon dioxide removal (CDR) approach (Oschlies et al., 2023; NASEM, 2022). OAE drives CDR through the introduction of alkaline substances into seawater, which shift the carbonate chemistry equilibrium,
from carbon dioxide (CO2) on the left to bicarbonate (HCO) and carbonate ions (CO) on the right. The decline in seawater CO2 concentration lowers the seawater CO2 partial pressure (pCO2), thereby enabling an influx of additional atmospheric CO2, or alternatively, reducing the efflux in cases where the surface ocean is a natural source of CO2 to the atmosphere. The OAE-induced shift in carbonate chemistry is measurable as an increase in seawater alkalinity – the name-giving feature of OAE. The viability of OAE to serve as a scalable CDR approach critically depends on whether it is environmentally safe. Surface ocean habitats are in the focus of the environmental OAE assessment because the surface ocean is where OAE would need to be implemented to enable CO2 exchange with the atmosphere (Bach et al., 2019).
The environmental OAE assessment is only just starting but seems to be evolving in a similar way to environmental assessments of other drivers of environmental change (e.g. ocean acidification) that have been set up in the past: research funding is provided to individual groups, who will perform individual studies in their local environments, allowing them to seek novelty. Each of these studies will be valuable, and exceeding previous research is central to scientific progress. However, previous research on environmental drivers has also shown that replication of experiments is perhaps equally important as seeking novelty, since replication allows us to reveal re-occurring response patterns across various scales and environments (Benton et al., 2007; Hamm et al., 2022; Stewart et al., 2013). In ocean acidification research, for example, an individual study found that the carbon to nitrogen () stoichiometry of plankton communities is increased under high-CO2 conditions due to CO2 fertilisation of the phytoplankton community (Riebesell et al., 2007). However, replication of the experiment at different locations found that zooplankton communities can strongly modify the response, to the point that the response can be significant in the opposite direction (lower under high CO2; Taucher et al., 2021). Arguably, the crucial progress in this example was understanding of the context dependency of the response to ocean acidification, which was made possible by replication of a sophisticated experiment across a wide geographical range (Riebesell et al., 2013). Likewise, the intercomparison of climate models via replicated numerical experiments (Dingley et al., 2023) has long been recognised as a cornerstone of the assessment of climate change (Masson-Delmotte et al., 2021), possibly more influential than the output of individual climate models.
The Ocean Alkalinity Enhancement Pelagic Impact Intercomparison Project (OAEPIIP) builds upon the insights from previous environmental assessments by establishing a platform that supports replication, while still enabling the pursuit of novelty. In essence, OAEPIIP provides funding for a cost-efficient and standardised OAE experiment, which can be conducted by scientists across the globe (Sect. 2). The experiments will use a microcosm setup to study the response of natural plankton communities to one widely considered OAE implementation strategy, and participants will determine the same set of response variables. Each experiment shall be published on an individual basis in a special issue of a peer-reviewed scientific journal under open access, with costs largely covered by OAEPIIP (Sect. 3). Individual publication of OAEPIIP experiments gives room to describe novel observations on how plankton communities respond to OAE. All datasets will be shared and synthesised in a meta-analysis. The standardised experimental design facilitates inclusion of individual datasets in the meta-analysis (Harrison, 2011). Likewise, the collection of all datasets, irrespective of their outcomes, avoids publication bias, which is a known problem of meta-analyses (Field and Gillett, 2010). We expect OAEPIIP to promote consensus among scientists concerning the potential environmental side effects of OAE on plankton communities, with significant potential for capacity building (Sect. 4). This paper provides a detailed manual for the OAEPIIP experimental setup and describes its benefits.
2.1 Microcosm setup
OAEPIIP utilises the microcosm setup developed by Ferderer et al. (2022), as it is cost-effective and relatively easy to set up and operate. The microcosms are 55 L polyethylene terephthalate (PET) tanks (FermZilla), which were originally designed for home brewing (Fig. 1) and are available worldwide (Table S1 in the Supplement). It is important that all OAEPIIP participants purchase the same type of microcosm incubators so that comparability can be established between individual studies (details on the availability of FermZilla tanks are provided in Table S1). The tanks are mounted on steel frames and have 120 and 70 mm openings at the top and bottom, respectively. The bottom opening is equipped with a butterfly valve and a sedimentation cup, used for the collection of settling material. The butterfly valve has a handle so that the sedimentation cup can be isolated from the water column. Butterfly valves should be closed during the experiment to keep the water column isolated from the sedimentation cup.
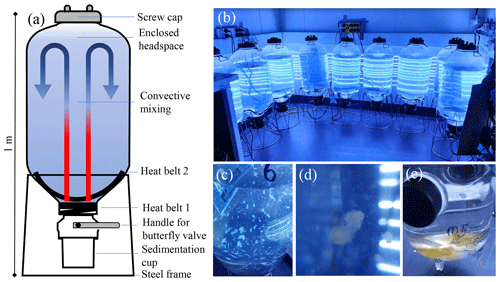
Figure 1Microcosm setup. (a) Schematic of the microcosm tanks. The two heat belts induce convective mixing within the tanks. (b) Arrangement of nine microcosms in a temperature-controlled room in front of a light source. Their position should be changed on a daily basis to minimise position-dependent differences in light and temperature over the course of the study. (c) A picture of a microcosm just after NaOH addition. The white flakes are brucite particles that need to be dissolved after NaOH addition by stirring the seawater within microcosms with a plastic spoon. (d) A close-up of a marine snow aggregate, which frequently forms after a phytoplankton bloom. (e) Marine snow aggregates collected in the sedimentation cup of the microcosm. Sampling these can be interesting, although this is not an essential parameter of OAEPIIP (Sect. 2.6). Please note that we highly recommend that the butterfly valves be kept closed during the experiment so that the sedimentation cup is isolated from the water column. Only in exceptional circumstances, when a participant decides to sample sediments, may they be opened in an OAEPIIP experiment.
The crucial steps for setting up the microcosms, their filling, and their operation are listed in Table S1, illustrated in Figs. 1 and 2 and Video supplements S1 (https://doi.org/10.5446/66751, Bach et al., 2024) and S2 (https://doi.org/10.5446/66753, Bach and Ferderer, 2024), and briefly described here. OAEPIIP experiments occupy approximately 9 m2 in a temperature-controlled room with a cooling capacity of roughly 6 °C below the temperature aimed for in the microcosm study (e.g. to 14 °C if the desired experimental water temperature is 20 °C). The microcosms need to be thoroughly cleaned in a two-step procedure before use, as detailed in Table S1.
Infrastructure needed for filling the microcosms with natural seawater (containing natural plankton communities) depends on the local environment at an OAEPIIP study site. At our site in Tasmania, we fill microcosms from a jetty using a small crane or davit (Fig. 2; Video supplement S1, https://doi.org/10.5446/66751, Bach et al., 2024). Natural seawater with plankton communities shall be collected by opening the top lid and butterfly valve at the bottom and lowering the microcosms slowly into seawater so that each microcosm is filled from bottom to top. Care must be taken to not enclose larger debris, nekton, or sediments. Once the microcosm is submerged and only the upper opening is above the sea surface, a rope attached to the handle of the butterfly valve is pulled so that the bottom opening is closed. The microcosm can now be lifted back onto shore and put back into its metal frame. Another possibility for filling microcosms is to slowly lower them from a low swimming pontoon or small boat and close the bottom manually. Filling microcosms by slowly lowering them into seawater is a very gentle way to collect plankton communities (Video supplement S1, https://doi.org/10.5446/66751, Bach et al., 2024), avoiding the physical disturbance to plankton imposed by pumping. Based on our experience, it takes roughly 45 min to fill nine microcosms. Longer timescales for the collection (i.e. ≫ 1 h) should be avoided to mitigate the risk of changes in seawater communities over the course of the filling procedure (e.g. through tidal water movement). This potential problem should also be minimised by filling the microcosms in random order. Furthermore, care should be taken to not expose the microcosms to excessive sunlight and/or heat after filling.
The weight of the enclosed seawater needs to be determined after the filling procedure, as this information is needed for establishing treatments (Sect. 2.5). This could be done using a balance or (if a balance is not available) volumetrically by determining weight with known volume, temperature, and salinity. Once the weight has been determined, microcosms need to be transported to the temperature-controlled room where the experiment takes place, and light and temperature control needs to be initiated immediately (see following section).
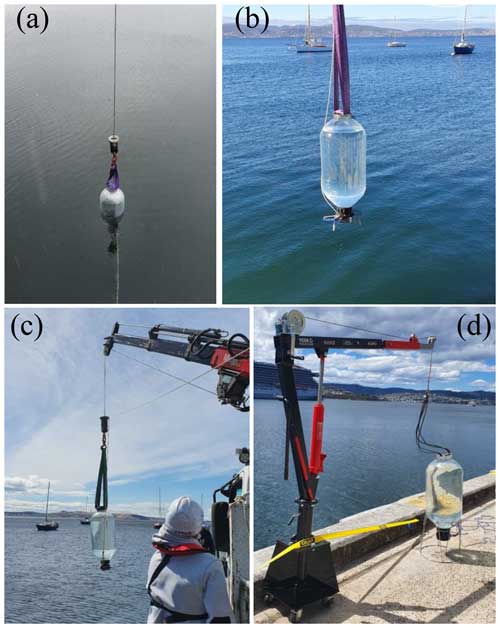
Figure 2Seawater collection for the microcosm experiments. (a) A microcosm is slowly lowered into seawater to gently collect a plankton community. (b, c) A filled microcosm being pulled back onto land. Please note that we mostly used a small crane mounted on a truck or a davit (as in d) for the seawater collection. However, microcosms filled with seawater only weigh about 60 kg, so lighter gear is probably sufficient for collection. A detailed description of seawater collection is provided in Table S1 and Video supplement S1 (https://doi.org/10.5446/66751, Bach et al., 2024).
2.2 Mixing, temperature, light, and nutrient conditions in OAEPIIP experiments
OAEPIIP utilises convection to mix the enclosed microcosm volume and keep plankton in suspension (Fig. 1). To establish convective mixing, two 30 W heat belts (see Table S1 for where these can be purchased) should be firmly attached to two distinct locations at the bottom of the microcosms (Fig. 1, Video supplement S2, https://doi.org/10.5446/66753, Bach and Ferderer, 2024). Based on our experience, these two 30 W heat belts increase the temperature of the enclosed seawater by ∼ 6 °C relative to the room temperature, so room temperature needs to be ∼ 6 °C lower than the target temperature in the experiments (please note that testing the temperature difference will be necessary prior to the experiment, as temperature offset may differ across temperature-controlled rooms). Once heat belts are attached, microcosms should be placed in front of the light source and heat belts should be plugged in to initiate the convective mixing.
While convection provides gentle and non-invasive mixing, there are several trade-offs regarding temperature control. Firstly, due to the removal of seawater during sampling, the total volume within the microcosms declines over the course of the experiment. Since the heat belts cannot be adjusted, there is an increase in heat energy input per litre of enclosed seawater and thus a gradual warming. To mitigate this issue, the external cooling may need to be increased over time by lowering the room temperature. In our experience, a reduction by 1 °C for every 5 L of seawater sampled from the microcosms is sufficient to keep the seawater temperature relatively constant over the course of the study. Secondly, small differences in ventilation at different locations in the temperature-controlled room can lead to seawater temperature differences of around 2 °C between microcosms (Ferderer et al., 2022; Guo et al., 2024). To mitigate this experimental constraint, the microcosm placement within the temperature-controlled room must be shuffled daily. Microcosms can easily be moved by pulling on the steel frames (Fig. 1), but care must be taken to briefly unplug the heat belts and plug them in again after shuffling their positions. Furthermore, fans can be utilised to remove heat pockets in the room, although care must be taken as the wind can have a strong cooling effect, resulting in a microcosm that was too warm quickly becoming too cold. Since temperature is a strong driver of physiological processes, it is highly advisable to thoroughly test the setup with all microcosms prior to the experiment (the careful addition of food dye can be used to test advection, as explained by Ferderer et al., 2022). The goal should be to have as little variation in temperatures between microcosms as possible, and the seawater temperature should be as the plankton community would have experienced it at the location and the season it was collected.
Like temperature, light conditions set up for the experiment should reproduce the natural site-specific conditions as much as possible. This applies to the light : dark cycle, the light intensity, and the light spectrum (the light spectrum should be between 400 and 750 nm, i.e. cool white light). Since many OAEPIIP participants may not have access to sophisticated computer-controlled light sources, we recommend the application of constant light over a fixed light : dark cycle. In an OAE study in Tasmania, for example, we provided constant light at 200 µmol photons m−2 s−1 with a 12 : 12 h light : dark cycle. These conditions were considered the representative average level for the surface mixed layer at the location where and season when the natural plankton community was sourced in Tasmania. The light : dark cycle can be achieved by plugging the light source into a timer socket. The microcosms need to be positioned in such a way that light is very similar inside each microcosm. A light meter shall be used to determine light intensity inside the microcosms prior to the experiments and at the end (Table 1). Positioning can be critical since movement by a few centimetres can often lead to noticeable changes in measured light intensity that are undetectable by the human eye. It is therefore important to adjust light conditions before starting the experiment and to mark the spot on the floor where individual microcosms must be placed to ensure replicable light levels. It is also important to have all the other microcosms at their respective locations while doing the adjustments, as they might shade each other. The daily shuffling of microcosm positions inside the room, which is essential for the temperature control (see above), will also help to mitigate systematic bias in the light regime between microcosms.
OAEPIIP experiments shall not add organisms, nutrients, or any substances other than alkalinity and/or DIC (Sect. 2.4) to the microcosms during or before the experiments.
2.3 OAE method in the focus of OAEPIIP
OAE can be implemented using different approaches and by applying different alkaline feedstocks, such as solid materials like olivine, calcium/magnesium hydroxides, carbonates, and steel slag, or liquid materials like sodium hydroxide (NaOH) dissolved in seawater (Eisaman et al., 2023; NASEM, 2022). Each alkalinity source has different environmental implications, as it is associated with different environmental perturbations (Bach et al., 2019). A widely considered approach is electrochemical OAE, where liquid NaOH is the alkalinity source (de Lannoy et al., 2018). NaOH-based OAE is in the focus of OAEPIIP due to the following reasons. First, electrochemical OAE was recently evaluated as ranking among the highest OAE approaches with regards to its “technological readiness level” (Eisaman et al., 2023), with field trials already underway. Second, liquid NaOH is suitable as an alkalinity source for applications in pelagic environments, as it delivers quasi-instantaneous OAE in seawater. Other methods that involve more slowly dissolving minerals (e.g. olivine) are considered less suitable for pelagic applications as they would partially sink into the deep ocean before dissolving (Köhler et al., 2013; Fakhraee et al., 2023). Third, electrochemical OAE is chemically relatively similar to other OAE methods such as OAE with magnesium hydroxides or ocean liming based on calcium hydroxides. Like NaOH, magnesium and calcium hydroxides dissolve relatively quickly and are comparatively clean sources of alkalinity due to a generally smaller amount of bioactive elements like iron or nickel than, for example, olivine when derived from carbonates (Bach et al., 2019; Renforth et al., 2022) or magnesium hydroxides when produced chemically (Eisaman et al., 2023). As such, the primary potential of these hydroxides to affect pelagic communities is by changing seawater carbonate chemistry. Thus, results from NaOH-based OAE experiments also have potential to inform these other approaches. Fourth, NaOH is readily available worldwide, which is logistically beneficial for OAEPIIP.
2.4 Experimental design
NaOH-based OAE reduces seawater pCO2 within seconds (Zeebe and Wolf-Gladrow, 2001), whereas the subsequent equilibration with atmospheric CO2 takes months to years (Jones et al., 2014; Wang et al., 2023; Mu et al., 2023) or potentially even centuries (He and Tyka, 2023). The carbonate chemistry perturbation is much greater before the equilibration has happened, so more pronounced effects on communities would be expected shortly after alkalinity addition (Bach et al., 2019). As such, an argument can be made to study OAE at two different time points when using rapidly dissolving alkalinity sources like NaOH or other hydroxides. These are the “unequilibrated” time point, simulating the fact that CO2 influx has not yet happened right after alkalinity addition, and the “equilibrated” time point, assuming the alkalinity enhanced seawater has already CO2 equilibrated with the atmosphere. Equilibration could either happen naturally through air–sea CO2 influx (Ho et al., 2023) or even be enforced within a facility so that alkalinity-enhanced seawater equilibrated with the atmosphere is discharged into the ocean.
The nine microcosms available for OAEPIIP experiments will provide triplicate incubations for controls, unequilibrated, and equilibrated treatments. An important aspect for OAEPIIP experiments is that the amount of alkalinity added to the treatments is consistent among all studies. Modelling studies suggest that gigatonne-scale OAE sustained for 80 years would increase surface ocean alkalinity by about 100–200 µmol kg−1 (Burt et al., 2021; Lenton et al., 2018). This seemingly modest perturbation is due to dilution by the huge volume of the ocean (i.e. 9.44×1017 m3; Sarmiento and Gruber, 2006). However, the perturbation can be more pronounced at sites where alkalinity is added, before being diluted by unperturbed seawater at a rate that depends on the location (He and Tyka, 2023; Wang et al., 2023). In fast dilution regimes, for example, 1 molar NaOH added at 5 m3 s−1 from a large ship would initially raise pH to 11 but dilute to a pH of 8.5 within minutes to hours (He and Tyka, 2023). As such, there is a trade-off for OAE experimentation between the realism and thus the relevance of a simulated OAE perturbation at the timescales (weeks) proposed here (requiring rather low alkalinity perturbation) and the detectability of biological effects (facilitated when simulated perturbations are more extreme). For OAEPIIP, we determined an alkalinity enhancement of 500 µmol kg−1 to both the unequilibrated and equilibrated treatments. Our rationale for the rather high perturbation is that OAEPIIP has a strong focus on capacity building in OAE research. Setting up clearly distinguishable treatments facilitates data analysis and interpretation, particularly for those entering the field. We emphasise, however, that a 500 µmol kg−1 perturbation over the duration of OAEPIIP studies (i.e. weeks; Sect. 2.7) is on the higher end of what is plausible for OAE (Wang et al., 2023; He and Tyka, 2023), except for perhaps in proximity to a continuous NaOH release site. Thus, the relatively extreme perturbation needs to be taken into account for the eventual interpretation and communication of OAEPIIP studies – since it is likely that a less extreme perturbation would also cause a smaller environmental effect.
2.5 Establishing treatments
Alkalinity enhancement shall be performed on day 0 of the experiment, shortly after microcosms have been positioned in the temperature-controlled room. Before adding alkalinity, carbonate chemistry samples (i.e. alkalinity and one other carbonate chemistry parameter; Sect. 2.6) should be collected to constrain carbonate chemistry conditions in all microcosms before OAE.
The three control microcosms will not receive any alkalinity addition and will remain untreated. The three microcosms of the unequilibrated treatment will receive 500 µmol kg−1 of NaOH. The simplest way to achieve this is by purchasing and using a 1 molar NaOH solution (ideally in “analytical quality”) and adding 500 µL kg−1 of enclosed seawater. For example, if 54.5 kg of seawater have been enclosed then 54.5 ⋅ 500 = 27 250 µL of 1 molar NaOH solution needs to be added to the respective microcosm.
The equilibrated treatment is slightly more complicated to establish. Here, most of the alkalinity needs to be added as sodium bicarbonate (NaHCO3) solution and a smaller amount as NaOH solution. We provide an R script based on Seacarb (Gattuso et al., 2021) that can be used to calculate additions of NaHCO3 and NaOH (OAEPIIP, 2024). Furthermore, video tutorials provide detailed instructions on how to use the R script or how to do these calculations with CO2SYS for MS Excel (Pierrot et al., 2021; Video supplements S3 and S4; https://doi.org/10.5446/66754, https://doi.org/10.5446/66752, Bach, 2024a, b). Briefly, in a first step, initial carbonate chemistry conditions need to be calculated for the unperturbed seawater enclosed in the microcosms. For this calculation, one needs to assume a current CO2 partial pressure (e.g. 420 µatm), the target temperature for the experiment, and a salinity and alkalinity estimate based on what the experimentalist expects for their region (or ideally has measured just before collecting the seawater for the microcosm experiment). Next, the calculation is repeated for the same conditions except for alkalinity where 500 µmol kg−1 is added to the assumed value (e.g. 2850 µmol kg−1 when the assumed value of the unperturbed water was 2350 µmol kg−1). The second calculation represents the desired conditions in the equilibrated treatment after the alkalinity enhancement. The calculated dissolved inorganic carbon (DIC) concentrations of the initial carbonate system (DICinitial) need to be subtracted from the calculated DIC of the calculated treatment (DICequilibrated),
where NaHCO3 addition is the amount of NaHCO3 that needs to be added per kilogram of enclosed seawater (in µmol kg−1). The addition of NaHCO3 provides equal amounts of DIC and alkalinity. However, OAE can only absorb ∼ 0.85 mol of DIC per mole of alkalinity added (He and Tyka, 2023; Schulz et al., 2023), so reaching +500 µmol kg−1 requires the addition of slightly more alkalinity without DIC. NaOH is used for this purpose, and the exact amount that needs to be added is calculated as
where 500 is the targeted alkalinity enhancement in µmol kg−1. NaHCO3 and NaOH additions need to be multiplied by the weight of the enclosed microcosm seawater to calculate how much NaHCO3 and NaOH need to be added per individual microcosm.
It is recommended to use 1 molar stock solutions for both NaHCO3 and NaOH for treatment manipulations because in that case, required additions in µmol per microcosm are equivalent to µL per microcosm. For example, in the equilibrated treatment, a typical addition would be 420 µL kg−1 of NaHCO3 and 80 µL kg−1 of NaOH (i.e. 22.89 mL per microcosm NaHCO3 and 4.36 mL per microcosm NaOH when 54.5 kg of seawater were enclosed) in case both solutions are 1 molar. One molar NaHCO3 stock solutions can be prepared by dissolving 8.4 g NaHCO3 powder (dried at 60 °C overnight; note that NaHCO3 decomposes at higher temperatures) in 100 mL deionised water. One molar NaOH (ideally in “analytical quality”) should be purchased as such.
The addition of NaOH to seawater causes precipitation of brucite (Mg(OH)2), which appears as white flakes (Fig. 1c). The brucite flakes bind the alkalinity added via NaOH in particulate form and need to be re-dissolved so that dissolved alkalinity is increased by the intended 500 µmol kg−1. Furthermore, brucite formation can precipitate phosphates (Karl and Tien, 1992). This must be avoided, as the loss of phosphate from the dissolved phase in the treatments would be a problematic confounding factor. The formation of brucite will be particularly pronounced in the unequilibrated treatment where all alkalinity is added as NaOH. To dissolve all brucite, microcosms should be gently stirred with a clean plastic paddle during and after NaOH additions until all white flakes disappear. Our previous experiments resembling the OAEPIIP approach (Ferderer et al., 2022) revealed that dissolution of all brucite by gentle stirring leads to the desired outcome; i.e. alkalinity was increased by 500 µmol kg−1 and no phosphate was lost. For consistency, control and equilibrated microcosms should be stirred as much as the unequilibrated microcosms. If OAEPIIP participants do not have prior practical experience with seawater carbonate chemistry manipulation, it is advised to test the abovementioned procedures (including the measurement of resulting carbonate chemistry parameter changes, such as in TA and DIC) before commencing the main OAEPIIP experiment.
2.6 Essential parameters to be measured in OAEPIIP experiments
Next to an identical experimental design and setup, the same parameters need to be measured in individual OAEPIIP experiments to make them comparable (Iglesias-Rodríguez et al., 2023). A list of “core” parameters with justifications for their choice is provided in Table 1, and additional recommendations on how to sample and process these are provided in Table S2. The core parameters (Table 1) should provide a relatively comprehensive yet cost-efficient insight into processes within the plankton community. Although all core parameters need to be measured in all participating OAEPIIP studies, there may be unsurmountable logistical constraints that keep a participant from determining a core parameter. Such cases should be mentioned upon application for OAEPIIP participation so that mitigation pathways can be explored and potential participants with less infrastructure capacity still have the opportunity to participate if possible (see also Sect. 4).
If they wish to do so, OAEPIIP participants can also measure additional parameters to maximise their individual experimental outcomes. However, the following issues should be considered.
-
Not more than approximately one-third of the microcosm volume should be sampled over the course of the study (a) to limit the build-up of a headspace and (b) to avoid too much heat input per litre of enclosed volume via the heat belts (the room temperature might need lowering to compensate for reducing volume throughout the experiment; Sect. 2.2).
-
Any type of contamination (particulate, dissolved organic, or inorganic) must be kept at a minimum.
-
It is possible to sample mesozooplankton with a customised net (Guo et al., 2023), but sampling should be restricted to three occasions during the experiment (e.g. beginning, middle, and end) to avoid overfishing.
-
Aggregation and sedimentation are often observed in these microcosm studies, and sampling settling materials from the sediment trap is encouraged (Ferderer et al., 2022). However, care must be taken to not remove significant volumes of seawater.
Table 1List of core parameters that need to be measured in all individual OAEPIIP studies. The “samplings” column indicates how often all nine microcosms need to be sampled for a specific parameter during the study. “Daily” means that this parameter needs to be measured every day, irrespective of the temperature-dependent duration of the study. “B/e” means that samples need to be taken at the beginning and the end of the experiment.
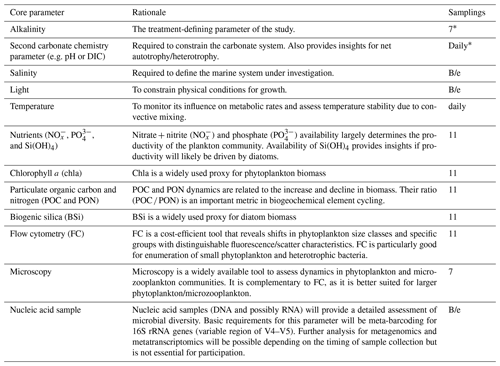
* These parameters must be sampled directly before and after establishment of the OAE treatments in all nine microcosms. All other parameters must be sampled for the first time after establishment of the treatments.
2.7 Duration of experiment
To the best of our knowledge, there is no general rule for the ideal duration of microcosm experiments. Experiments that are too short may miss important responses of plankton communities, while long experiments may exacerbate so-called “bottle effects”, non-specific effects from confinement rather than the experimental perturbation itself (Pernthaler and Amann, 2005). For example, 3 d of experiment may be too short to observe a differentiation of plankton species composition between treatments, while a community contained for 2 months could be dominated by those that best survive in a laboratory environment. Based on experiments with the OAEPIIP setup in Tasmania, we consider 20 d as a good compromise for an experiment at 15 °C. However, metabolic rates increase with temperature, so the experimental duration needs to be adjusted based on respective locations. Informed by Q10 temperature dependencies (Sherman et al., 2016), we recommend the following framework: 20 d is the reference duration at 15 °C. The duration (in days) increases/decreases from this reference point using Q10 kinetics,
where 0.5611 is the reference growth rate at 15 °C, Texp is the anticipated temperature in the OAEPIIP experiment, and 1.47 is the Q10 factor derived by Sherman et al. (2016). For example, an experiment at 25 °C should last for 14 d and an experiment at 5 °C for 29 d.
2.8 Sampling operations and logistics
All microcosm incubators shall be closed with the black screw caps after the filling procedure (Fig. 1) and kept closed over the course of the experiment except during the establishment of treatments (Sect. 2.5) and sampling. The enclosed headspace (Fig. 1) may vary slightly between microcosms after the filling procedure (Sect. 2.1) and will increase over the course of the experiment due to the withdrawal of samples. While an increasing headspace will lead to some CO2 exchange between the atmosphere and the enclosed volume, previous studies with the same setting found that this has no effect on the OAE treatments established in the experiments (Guo et al., 2024; Ferderer et al., 2022). The convective system mixes the water column, so no manual mixing is needed prior to sampling. A peristaltic pump is recommended to withdraw the seawater samples from the microcosms.
The total number of samplings for specific parameters is listed in Table 1 (for example, POC and PON need to be sampled 11 times in total). The frequency of sampling needs to be adjusted based on the temperature-dependent duration of the experiment (Sect. 2.7). OAEPIIP experiments at higher temperatures require higher sampling frequency because metabolic processes are faster. Table 1 lists the minimum number of days a parameter should be sampled. This number is to guarantee that there will be enough comparable data points across OAEPIIP experiments. For example, nutrient samples should be taken from each microcosm at least 11 times during the experiment. For an experiment at 15 °C (20 d), this could mean sampling on day 0 (directly after establishing treatments) and then days 2, 4, 6, …, 20. However it may also be reasonable to increase frequency during periods of phytoplankton blooms (e.g. daily) and then reduce the frequency (e.g. every 4 d) when nutrients are depleted. In general, OAEPIIP experimentalists can best decide on an individual basis what sampling schedule is most appropriate for their experiment, but the total number of samplings must be at least as defined in Table 1 for each of the listed parameters.
Sampling for all OAEPIIP experiments should begin 2 h after the onset of the light period on a sampling day. This coordination of initial sampling ensures that the plankton community is in a similar diurnal growth state. Hence, sampling of all nine microcosms should ideally not last longer than 3 h.
2.9 Statistical analyses
Microcosm data contain complex ecological data that require specific (often complicated) statistical tools for their analysis. A common issue is the presence of nonlinear relationships, which prevent the fitting of data to linear models without gross transformation of the variables . Furthermore, OAEPIIP microcosms will be sampled several times over an extended period. This sampling strategy results in temporal-pseudoreplication, where observations are not independent of each other and therefore violate the assumption of independence required for simple linear models and generalised additive models (GAMs) (Zuur et al., 2009; Wood, 2017). The expansion of GAMs to generalised additive mixed models (GAMMs) allows for correlations between observations and for the modelling of data structures that are nested, as well as for nonlinear relationships between the response and explanatory variables.
To facilitate and standardise statistical analyses of individual datasets, we provide an R-based pipeline (OAEPIIP, 2024). This pipeline is tailored towards the evaluation of individual OAEPIIP datasets using GAMMs. The files contain a workflow that demonstrates the use of GAMMs and facilitates the seamless integration of individual datasets gathered during OAEPIIP experiments into the workflow. Theoretical background, knowledge, and details on how to fit such models can be found in the textbooks by Zuur et al. (2009) and Wood (2017).
Basic instructions and updates on OAEPIIP will be provided on the OAEPIIP website (https://appliedbgc.imas.utas.edu.au/ocean-alkalinity-enhancement-pelagic-impact-intercomparison-project/, last access: 2 August 2024).
3.1 Eligibility and funding
To join OAEPIIP, participants need to be capable of performing an OAEPIIP study and providing all data by December 2025. This capacity shall be confirmed on a simple one-page form (available on the OAEPIIP website) that potential participants need to fill in and send to the email provided on the form. Career stage, publication record, or other parameters of a scientist's curriculum vitae have no relevance for OAEPIIP. As such, application success is determined by logistical and infrastructure-related aspects, for example whether a participant has access to a temperature-controlled room and can provide the data in the given time frame (but see also Sect. 4 for suggestions on how to mitigate individual infrastructure limitations). Ultimately, participation is restricted by the total funding available to OAEPIIP. Should there be more applications than there is funding, participants will be selected based on two criteria: first, we will consider the locations of the experiments to obtain the best possible geographic spread. Second, participants that reached out first will be selected, should there be clusters of applications in close geographic proximity.
OAEPIIP provides a maximum of around USD 12 000 per study in materials and funding for analytical costs and publication fees (the exact amount is slightly variable due to exchange rates). All materials and standardised components like the microcosms shall be purchased by the individual participants, with ∼ USD 10 400 made available to them for the experiments. The OAEPIIP administration will provide all necessary information for the purchase of standardised components so that all experiments are conducted in the same type of incubators. The remaining ∼ USD 1600 will be retained by the OAEPIIP administration and made available to support the publication of individual OAEPIIP studies (see Sect. 3.2).
The funds for materials and standardised components will be transferred via invoicing. Thus, participants must have a bank account associated with their affiliation to which funding can be transferred from Australia. This criterion therefore excludes laboratories in countries under relevant sanctions from Australia from receiving funding, although they are still welcome to be part of the OAEPIIP community. In practice, participants will send two invoices to the University of Tasmania: one at the beginning of the experiment to support purchasing of materials and standardised components and the second one towards the end when the data are available and have been submitted. OAEPIIP cannot provide funding for salaries. Therefore, the experiment was designed to be suitable for a Master's thesis or a chapter of a PhD thesis.
3.2 Data management and publication
Datasets of individual OAEPIIP studies should be formatted using a standardised template available on the OAEPIIP home page (Sect. 3) and submitted to OAEPIIP as soon as they are available. All data must be uploaded and made available under open access. Participants will be listed on the OAEPIIP home page and their individual datasets will be linked to their names and affiliations as soon as they are made available. OAEPIIP experiments shall be published on an individual basis in an OAEPIIP special issue (publication fees of up to USD 1600 are provided by OAEPIIP). Individual publication will enable identification of novel observations regarding how plankton communities respond to OAE. If participants prefer not to publish their data, they still need to submit their data to OAEPIIP so that they can be included in the OAEPIIP synthesis. This is critically important because the synthesis must avoid publication bias.
The OAEPIIP synthesis will be prepared once all datasets have been delivered. First and last authors of individual studies will automatically be co-authors on the synthesis publication(s) at the end of the project, unless they prefer not to be.
OAEPIIP has potential benefits that go beyond scientific knowledge gain. The community effort helps to build a network of OAE scientists and provides an incentive for and access to those who have not yet engaged with OAE research. Indeed, growing the OAE research community is essential to accelerate the OAE assessment and make it more comprehensive. Providing the same amount of funding, regardless of the location, may increase the attractiveness of OAEPIIP studies to those that currently have less funding. We aim for the participation of scientists worldwide since the OAE assessment requires the inclusion of the global community. Indeed, participation in the process of assessing marine CDR methods (such as OAE), rather than being on the receiving end of information only, has been expressed as an important aspect by stakeholders from developing countries.
We are aware that the infrastructure demands for OAEPIIP (Sect. 2) still put up barriers to participation. To mitigate those barriers, potential participants from more experienced laboratories can offer to serve as a partner for a less experienced laboratory. Likewise, potential participants from less experienced laboratories can indicate if they need support from an experienced laboratory. This information shall be disclosed on the application form (available on the OAEPIIP website) so that OAEPIIP can establish partnerships between participants. Partners can support each other through knowledge exchange but also more practically by analysing samples for each other. For example, if an interested participant has no capacity to measure alkalinity or flow cytometry samples, they may partner with another participant to share analytical duties. The distribution of funding for analytical costs via invoicing allows for such flexibility, as it provides an opportunity to easily redistribute funding between project participants when this is communicated with the OAEPIIP administration. For example, when two laboratories partner, they together have access to twice the funding (∼ USD 24 000), which they share between themselves for the two experiments that they would have to do (the two experiments must be at different locations to guarantee geographical diversity).
Furthermore, potential participants that simply have no chance to measure one (or more) core parameters due to insurmountable logistical constraints can still hand in an application if they indicate the parameters that they are unable to deliver on their application form (available on the OAEPIIP website). The OAEPIIP administration will then evaluate such applications on a case-by-case basis and explore if there is a way to participate despite this limitation. This pathway is set in place specifically for potential participants with less developed infrastructure.
Altogether, we hope that the cost-efficient design of OAEPIIP, its eligibility criteria that refrain from classic measures of scientific success, and potential support via an evolving OAEPIIP community could promote an inclusive assessment of OAE. One primary goal of OAEPIIP is capacity building to provide more informed decisions concerning OAE that encompass data from a geographically diverse range of plankton ecosystems.
All code provided for experimental design and statistical analysis can be found at the following address: https://doi.org/10.5281/zenodo.10783751 (OAEPIIP, 2024).
The Video supplements are available at TIB (https://doi.org/10.5446/66751, https://doi.org/10.5446/66753, https://doi.org/10.5446/66754, https://doi.org/10.5446/66752, Bach et al., 2024; Bach and Ferderer, 2024; Bach, 2024a, b).
The supplement related to this article is available online at: https://doi.org/10.5194/bg-21-3665-2024-supplement.
LTB designed the study with comments from all authors. AJF composed the statistical procedures. JL drafted the metagenomic methods. LTB drafted the manuscript, with contributions from all authors.
The contact author has declared that none of the authors has any competing interests.
Publisher’s note: Copernicus Publications remains neutral with regard to jurisdictional claims made in the text, published maps, institutional affiliations, or any other geographical representation in this paper. While Copernicus Publications makes every effort to include appropriate place names, the final responsibility lies with the authors.
This article is part of the special issue “Environmental impacts of ocean alkalinity enhancement”. It is not associated with a conference.
We thank 3 anonymous reviewers for insightful comments and Axel Durand for supporting OAEPIIP infrastructure at the University of Tasmania.
OAEPIIP has been funded by the Carbon-to-Sea Initiative. This research has been supported by the Australian Research Council through Future Fellowship granted to LTB (FT200100846).
This paper was edited by Patricia Grasse and reviewed by two anonymous referees.
Bach, L.: How to calculate the “equilibrated treatment” for OAEPIIP experiments with R, TIB [video], https://doi.org/10.5446/66754, 2024a.
Bach, L.: How to calculate the “equilibrated treatment” for OAEPIIP experiments with MS Excel, TIB [video], https://doi.org/10.5446/66752, 2024b.
Bach, L. and Ferderer, A.: Attach heat belts to microcosms for OAEPIIP experiments, TIB [video], https://doi.org/10.5446/66753, 2024.
Bach, L., Ferderer, A., and Durand, A.: Filling microcosms for OAEPIIP experiments, TIB [video], https://doi.org/10.5446/66751, 2024.
Bach, L. T., Gill, S. J., Rickaby, R. E. M., Gore, S., and Renforth, P.: CO2 Removal With Enhanced Weathering and Ocean Alkalinity Enhancement: Potential Risks and Co-benefits for Marine Pelagic Ecosystems, Front. Clim., 1, 7, https://doi.org/10.3389/fclim.2019.00007, 2019.
Benton, T. G., Solan, M., Travis, J. M. J., and Sait, S. M.: Microcosm experiments can inform global ecological problems, Trend. Ecol. Evol., 22, 516–521, https://doi.org/10.1016/j.tree.2007.08.003, 2007.
Burt, D. J., Fröb, F., and Ilyina, T.: The Sensitivity of the Marine Carbonate System to Regional Ocean Alkalinity Enhancement, Front. Clim., 3, 624075, https://doi.org/10.3389/fclim.2021.624075, 2021.
de Lannoy, C.-F., Eisaman, M. D., Jose, A., Karnitz, S. D., DeVaul, R. W., Hannun, K., and Rivest, J. L. B.: Indirect ocean capture of atmospheric CO2: Part I. Prototype of a negative emissions technology, Int. J. Greenh. Gas Con., 70, 243–253, https://doi.org/10.1016/j.ijggc.2017.10.007, 2018.
Dingley, B., O'Rourke, E., Turner, B., CMIP Panel Members, WGCM Infrastructure Panel Members, and CMIP7 Task Team Members: CMIP Annual Report 2022–2023, Zenodo, https://doi.org/10.5281/zenodo.8101810, 2023.
Eisaman, M. D., Geilert, S., Renforth, P., Bastianini, L., Campbell, J., Dale, A. W., Foteinis, S., Grasse, P., Hawrot, O., Löscher, C. R., Rau, G. H., and Rønning, J.: Assessing the technical aspects of ocean-alkalinity-enhancement approaches, in: Guide to Best Practices in Ocean Alkalinity Enhancement Research, edited by: Oschlies, A., Stevenson, A., Bach, L. T., Fennel, K., Rickaby, R. E. M., Satterfield, T., Webb, R., and Gattuso, J.-P., Copernicus Publications, State Planet, 2-oae2023, 3, https://doi.org/10.5194/sp-2-oae2023-3-2023, 2023.
Fakhraee, M., Li, Z., Planavsky, N. J., and Reinhard, C. T.: A biogeochemical model of mineral-based ocean alkalinity enhancement: impacts on the biological pump and ocean carbon uptake, Environ. Res. Lett., 18, 044047, https://doi.org/10.1088/1748-9326/acc9d4, 2023.
Ferderer, A., Chase, Z., Kennedy, F., Schulz, K. G., and Bach, L. T.: Assessing the influence of ocean alkalinity enhancement on a coastal phytoplankton community, Biogeosciences, 19, 5375–5399, https://doi.org/10.5194/bg-19-5375-2022, 2022.
Field, A. P. and Gillett, R.: How to do a meta-analysis, Brit. J. Math. Stat. Psy., 63, 665–694, https://doi.org/10.1348/000711010X502733, 2010.
Gattuso, J.-P., Epitalon, J.-M., Lavigne, H., and Orr, J. C.: Seacarb: seawater carbonate chemistry, R package version 3.3.0., CRAN, https://CRAN.R-project.org/package=seacarb (last access: 2 August 2024), 2021.
Guo, J. A., Strzepek, R. F., Swadling, K. M., Townsend, A. T., and Bach, L. T.: Influence of ocean alkalinity enhancement with olivine or steel slag on a coastal plankton community in Tasmania, Biogeosciences, 21, 2335–2354, https://doi.org/10.5194/bg-21-2335-2024, 2024.
Hamm, T., Barkhau, J., Gabriel, A.-L., Gottschalck, L. L., Greulich, M., Houiller, D., Kawata, U., Tump, L. N., Leon, A. S., Vasconcelos, P., Yap, V., Almeida, C., Chase, Z., Hurd, C. L., Lavers, J. L., Nakaoka, M., Rilov, G., Thiel, M., Wright, J. T., and Lenz, M.: Plastic and natural inorganic microparticles do not differ in their effects on adult mussels (Mytilidae) from different geographic regions, Sci. Total Environ., 811, 151740, https://doi.org/10.1016/j.scitotenv.2021.151740, 2022.
Harrison, F.: Getting started with meta-analysis, Meth. Ecol. Evol., 2, 1–10, https://doi.org/10.1111/j.2041-210X.2010.00056.x, 2011.
He, J. and Tyka, M. D.: Limits and CO2 equilibration of near-coast alkalinity enhancement, Biogeosciences, 20, 27–43, https://doi.org/10.5194/bg-20-27-2023, 2023.
Ho, D. T., Bopp, L., Palter, J. B., Long, M. C., Boyd, P. W., Neukermans, G., and Bach, L. T.: Monitoring, reporting, and verification for ocean alkalinity enhancement, in: Guide to Best Practices in Ocean Alkalinity Enhancement Research, edited by: Oschlies, A., Stevenson, A., Bach, L. T., Fennel, K., Rickaby, R. E. M., Satterfield, T., Webb, R., and Gattuso, J.-P., Copernicus Publications, State Planet, 2-oae2023, 12, https://doi.org/10.5194/sp-2-oae2023-12-2023, 2023.
Iglesias-Rodríguez, M. D., Rickaby, R. E. M., Singh, A., and Gately, J. A.: Laboratory experiments in ocean alkalinity enhancement research, in: Guide to Best Practices in Ocean Alkalinity Enhancement Research, edited by: Oschlies, A., Stevenson, A., Bach, L. T., Fennel, K., Rickaby, R. E. M., Satterfield, T., Webb, R., and Gattuso, J.-P., Copernicus Publications, State Planet, 2-oae2023, 5, https://doi.org/10.5194/sp-2-oae2023-5-2023, 2023.
Jones, D. C., Ito, T., Takano, Y., and Hsu, W.-C.: Spatial and seasonal variability of the air-sea equilibration timescale of carbon dioxide, Global Biogeochem. Cy., 28, 1163–1178, https://doi.org/10.1002/2014GB004813, 2014.
Karl, D. M. and Tien, G.: MAGIC: A sensitive and precise method for measuring dissolved phosphorus in aquatic environments, Limnol. Oceanogr., 37, 105–116, https://doi.org/10.4319/lo.1992.37.1.0105, 1992.
Köhler, P., Abrams, J. F., Völker, C., Hauck, J., and Wolf-Gladrow, D. A.: Geoengineering impact of open ocean dissolution of olivine on atmospheric CO2, surface ocean pH and marine biology, Environ. Res. Lett., 8, 014009, https://doi.org/10.1088/1748-9326/8/1/014009, 2013.
Lenton, A., Matear, R. J., Keller, D. P., Scott, V., and Vaughan, N. E.: Assessing carbon dioxide removal through global and regional ocean alkalinization under high and low emission pathways, Earth Syst. Dynam., 9, 339–357, https://doi.org/10.5194/esd-9-339-2018, 2018.
Masson-Delmotte, V., Zhai, P., Pirani, A., Connors, S. L., Péan, C., Berger, S., Caud, N., Chen, Y., Goldfarb, L., Gomis, M. I., Huang, M., Leitzell, K., Lonnoy, E., Matthews, J. B. R., Maycock, T. K., Waterfield, T., Yelekçi, Ö., Yu, R., and Zhou, B. (Eds.): Climate Change 2021: The Physical Science Basis. Contribution of Working Group I to the Sixth Assessment Report of the Intergovernmental Panel on Climate Change, Cambridge University Press, Cambridge, United Kingdom and New York, NY, USA, https://doi.org/10.1017/9781009157896, 2021.
Mu, L., Palter, J. B., and Wang, H.: Considerations for hypothetical carbon dioxide removal via alkalinity addition in the Amazon River watershed, Biogeosciences, 20, 1963–1977, https://doi.org/10.5194/bg-20-1963-2023, 2023.
NASEM (Natl. Acad. Sci. Eng. Med.): A research strategy for ocean-based carbon dioxide removal and sequestration, Rep., NASEM, Washington, DC, https://doi.org/10.17226/26278, 2022.
OAEPIIP: Additional resources on carbonate chemistry calculation and statistical evaluations of OAEPIIP, Zenodo [data set], https://doi.org/10.5281/zenodo.10783751, 2024.
Oschlies, A., Bach, L. T., Rickaby, R. E. M., Satterfield, T., Webb, R., and Gattuso, J.-P.: Climate targets, carbon dioxide removal, and the potential role of ocean alkalinity enhancement, in: Guide to Best Practices in Ocean Alkalinity Enhancement Research, edited by: Oschlies, A., Stevenson, A., Bach, L. T., Fennel, K., Rickaby, R. E. M., Satterfield, T., Webb, R., and Gattuso, J.-P., Copernicus Publications, State Planet, 2-oae2023, 1, https://doi.org/10.5194/sp-2-oae2023-1-2023, 2023.
Pernthaler, J. and Amann, R.: Fate of Heterotrophic Microbes in Pelagic Habitats: Focus on Populations, Microbiol. Mol. Biol. R., 69, 440–461, https://doi.org/10.1128/mmbr.69.3.440-461.2005, 2005.
Pierrot, D., Epitalon, J.-M., Orr, J. C., Lewis, E., and Wallace, D. W. R.: MS Excel program developed for CO2 system calculations – version 3.0, GitHub repository, https://github.com/dpierrot/co2sys_xl (last access: 2 August 2024), 2021.
Renforth, P., Baltruschat, S., Peterson, K., Mihailova, B. D., and Hartmann, J.: Using ikaite and other hydrated carbonate minerals to increase ocean alkalinity for carbon dioxide removal and environmental remediation, Joule, 6, 2674–2679, https://doi.org/10.1016/j.joule.2022.11.001, 2022.
Riebesell, U., Schulz, K. G., Bellerby, R. G. J., Botros, M., Fritsche, P., Meyerhöfer, M., Neill, C., Nondal, G., Oschlies, A., Wohlers, J., and Zöllner, E.: Enhanced biological carbon consumption in a high CO2 ocean, Nature, 450, 545–548, https://doi.org/10.1038/nature06267, 2007.
Riebesell, U., Czerny, J., von Bröckel, K., Boxhammer, T., Büdenbender, J., Deckelnick, M., Fischer, M., Hoffmann, D., Krug, S. A., Lentz, U., Ludwig, A., Muche, R., and Schulz, K. G.: Technical Note: A mobile sea-going mesocosm system – new opportunities for ocean change research, Biogeosciences, 10, 1835–1847, https://doi.org/10.5194/bg-10-1835-2013, 2013.
Sarmiento, J. L. and Gruber, N.: Ocean biogeochemical dynamics, Princeton University Press, 2006.
Schulz, K. G., Bach, L. T., and Dickson, A. G.: Seawater carbonate chemistry considerations for ocean alkalinity enhancement research: theory, measurements, and calculations, in: Guide to Best Practices in Ocean Alkalinity Enhancement Research, edited by: Oschlies, A., Stevenson, A., Bach, L. T., Fennel, K., Rickaby, R. E. M., Satterfield, T., Webb, R., and Gattuso, J.-P., Copernicus Publications, State Planet, 2-oae2023, 2, https://doi.org/10.5194/sp-2-oae2023-2-2023, 2023.
Sherman, E., Moore, J. K., Primeau, F., and Tanouye, D.: Temperature influence on phytoplankton community growth rates, Global Biogeochem. Cy., 30, 550–559, https://doi.org/10.1002/2015GB005272, 2016.
Stewart, R. I. A., Dossena, M., Bohan, D. A., Jeppesen, E., Kordas, R. L., Ledger, M. E., Meerhoff, M., Moss, B., Mulder, C., Shurin, J. B., Suttle, B., Thompson, R., Trimmer, M., and Woodward, G.: Chapter Two – Mesocosm Experiments as a Tool for Ecological Climate-Change Research, in: Advances in Ecological Research, Vol. 48, edited by: Woodward, G. and O'Gorman, E. J., Academic Press, 71–181, https://doi.org/10.1016/B978-0-12-417199-2.00002-1, 2013.
Taucher, J., Boxhammer, T., Bach, L. T., Paul, A. J., Schartau, M., Stange, P., and Riebesell, U.: Changing carbon-to-nitrogen ratios of organic-matter export under ocean acidification, Nat. Clim. Change, 11, 52–57, https://doi.org/10.1038/s41558-020-00915-5, 2021.
Wang, H., Pilcher, D. J., Kearney, K. A., Cross, J. N., Shugart, O. M., Eisaman, M. D., and Carter, B. R.: Simulated Impact of Ocean Alkalinity Enhancement on Atmospheric CO2 Removal in the Bering Sea, Earth's Future, 11, e2022EF002816, https://doi.org/10.1029/2022EF002816, 2023.
Wood, S. N.: Generalized Additive Models: An Introduction with R, 2nd Edn., Chapman and Hall/CRC, Boca Raton, 496 pp., https://doi.org/10.1201/9781315370279, 2017.
Zeebe, R. E. and Wolf-Gladrow, D. (Eds.): CO2 in Seawater: Equilibrium, Kinetics, Isotopes, Elsevier Oceanography Series, Amsterdam, 1–346, ISBN 9780444509468, 2001.
Zuur, A. F., Ieno, E. N., Walker, N., Saveliev, A. A., and Smith, G. M. (Eds.): Mixed effects models and extensions in ecology with R, Springer, New York, NY, https://doi.org/10.1007/978-0-387-87458-6, 2009.
- Abstract
- Rationale for the Ocean Alkalinity Enhancement Pelagic Impact Intercomparison Project (OAEPIIP)
- Experimental infrastructure, operation, and design
- Logistics and administration
- Capacity building and inclusivity
- Data availability
- Video supplement
- Author contributions
- Competing interests
- Disclaimer
- Special issue statement
- Acknowledgements
- Financial support
- Review statement
- References
- Supplement
- Abstract
- Rationale for the Ocean Alkalinity Enhancement Pelagic Impact Intercomparison Project (OAEPIIP)
- Experimental infrastructure, operation, and design
- Logistics and administration
- Capacity building and inclusivity
- Data availability
- Video supplement
- Author contributions
- Competing interests
- Disclaimer
- Special issue statement
- Acknowledgements
- Financial support
- Review statement
- References
- Supplement