the Creative Commons Attribution 4.0 License.
the Creative Commons Attribution 4.0 License.
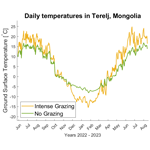
Impact of livestock activity on near-surface ground temperatures in central Mongolian grasslands
Robin Benjamin Zweigel
Avirmed Dashtseren
Khurelbaatar Temuujin
Anarmaa Sharkhuu
Clare Webster
Hanna Lee
Sebastian Westermann
Grazing by livestock can alter the surface conditions at grassland sites, impacting the transfer of energy between the atmosphere and ground and consequentially ground temperatures. In this study, we investigate surface cover in summer and winter and measure ground surface temperatures over 14 months at sites in central Mongolia that feature different grazing intensities (intensely and ungrazed) and topographic aspects (north- and south-facing). Overall, intense grazing leads to a substantially reduced vegetation cover, altered snow conditions, and lack of surface litter accumulation. Comparing intensely grazed and ungrazed plots shows large seasonal differences in ground surface temperatures, with grazed plots being up to +5.1 °C warmer in summer and −5.4 °C colder in winter at a south-facing site. We also find that the effect of grazing intensity depends on topographic aspect, with smaller seasonal differences of +1.4 °C and −2.5 °C found between grazed and ungrazed plots at a north-facing site. This relates to the lower available solar radiation at north-facing sites, which reduces the differences in vegetation cover between open and fenced plots. For both aspects, the seasonal differences largely offset each other, with both a small net cooling and warming depending on effects in spring and autumn. Our study suggests that livestock management could be used to modify the annual ground temperature dynamics, possibly even influencing local permafrost dynamics.
- Article
(4356 KB) - Full-text XML
- BibTeX
- EndNote
Livestock activity affects ground surface temperatures (GSTs) at grassland sites through grazing and trampling, which changes the surface cover and consequentially the exchange of energy between the atmosphere and ground surface (Shao et al., 2017). Intense grazing reduces vegetation height and density (Wang et al., 2022; Yan et al., 2018), inhibits formation of litter layers (Hou et al., 2020), and changes the accumulation of local snow cover (Yan et al., 2019). Trampling by livestock compacts snow and ground surface layers, which changes their thermal properties (e.g. Gan et al., 2012; Zimov et al., 2012). At grazed grassland sites, these changes in surface cover lead to an amplification of the seasonal cycle of GSTs with distinctly higher GSTs in summer and lower GSTs in winter (e.g. Hou et al., 2020; Odriozola et al., 2014; Yan et al., 2018; Zhao et al., 2011). Furthermore, soil moisture levels are altered by intense grazing, yielding generally drier soils (Yan et al., 2018; Zhao et al., 2011) and reduced freeze–thaw processes (Wang et al., 2023). However, most studies linking grazing and GSTs are limited to the growing season (e.g. Hou et al., 2020; Odriozola et al., 2014) or use controlled experiments where specified livestock loads are applied on fenced plots (Wang et al., 2023; Yan et al., 2018, 2019; Zhao et al., 2011). In reality, grazing intensities are not spatially and temporally homogeneous but determined by livestock and herders seeking optimal feeding conditions within their rangeland. The evaluation of the impacts of such gradients in grazing intensity on year-round GSTs has been limited.
In Mongolia, semi-nomadic pastoralism is widespread, and 70 %–80 % of the land area is used as rangeland for livestock (Angerer et al., 2008; Fernández-Giménez et al., 2018; Unified Land Territory Of Mongolia, 2024). Mongolia's shift to a market economy, a growing population, and high demand for cashmere wool have led to an increase and change in type of livestock over recent decades (Lkhagvadorj et al., 2013; Saizen et al., 2010). This intensified land use occurs together with intense climatic warming and has contributed to widespread land degradation in Mongolia (Wang et al., 2019, 2020). At the same time, Mongolian grasslands are often underlain by permafrost that is currently degrading (Ishikawa et al., 2018; Wang et al., 2022). Topography controls the local distribution of vegetation cover and permafrost, which are both strongly linked to incoming solar radiation (Klinge et al., 2021; Zweigel et al., 2024). So far, the impact of grazing and trampling on ground thermal dynamics in Mongolian grasslands and the relationship with topography has received little attention.
In this study, we investigate how differences in livestock activity due to local fencing and semi-nomadic pastoralism affect vegetation, snow cover, and associated GSTs and how these manifest at sites with opposing topographic aspect. To achieve this we measured GSTs at multiple plots at two sites with different exposure and surveyed snow and vegetation cover in winter and summer. Based on these field measurements, we investigate the following questions:
-
How do livestock grazing and topography affect vegetation and snow cover in these central Mongolian steppe environments?
-
How are seasonal and annual GSTs affected by differences in vegetation cover and litter amount induced by grazing and trampling?
We collected data over a period of 14 months at two field sites: Terelj and Udleg, located ca. 50 km apart in the Khentii Mountains in central Mongolia (ca. 48° N, 107° E). The climate of the region is characterized as continental and generally dry, with large annual variations in temperature. Both sites are located in grasslands within the forest–steppe ecotone, where topographic aspect and solar radiation produce distinct, local variations in vegetation cover (Klinge et al., 2021). In general, north-facing slopes feature boreal forest, while valley bottoms and south-facing slopes are dominated by grasslands (Ishikawa et al., 2005). The grasslands in this region experience extensive grazing from local livestock, which in both Terelj and Udleg consists predominantly of cattle, horses, goats, and sheep.
The Terelj study site is located at 1650 m a.s.l. on a south-facing slope, in proximity to an automatic weather station described in Dashtseren et al. (2014) (Fig. 1a). Dashtseren et al. (2014) report the local grassland on the south-facing slope to be permafrost-free with daily average air temperatures ranging from more than 20 °C in summer to less than −30 °C in winter, and the annual rainfall is less than 300 mm (2004–2007). At this intensely grazed site, sparse vegetation covered only about 60 % of the ground surface in 2004, consisting of Artemisia frigida, Potentilla acaulis, Agropyron cristatum, and Carex duriuscula (Dashtseren et al., 2014). In 2003, the area around the weather station was fenced, which created a sharp difference in livestock activity, as vegetation within the enclosure was fully protected from grazing and animal trampling (Fig. 2). This allowed grass vegetation to grow tall within the fenced enclosure each summer, while the growth of vegetation in the surrounding open grasslands was limited by intense grazing.
Our measurement efforts in Terelj target the strong contrast in vegetation and livestock activity related to the fenced weather station enclosure. We established two field plots in the immediate vicinity of the fence, referred to as the “open” and “fenced” plots (Fig. 1c), where GSTs were monitored from May 2022 to August 2023. At the fenced plot, we limit interference from other activity associated with the weather station by focusing our field observations to an area in the north-western part of the enclosure, away from the gate and instrumentation. The open plot borders the fenced plot and is located away from established animal and car tracks. We note that the main wind direction in Terelj is along of the main valley (east–west, Fig. 1c) and that plots are placed on either side of a fence that runs roughly parallel to the main valley (Fig. 1a), avoiding potential lee effects behind the fence.
In Udleg, the studied grassland site is located on a gentle northeast-facing slope, situated at the base of a steeper forested north-facing slope (Fig. 1b). The site is located at 1250–1290 m a.s.l., about 300 m lower in elevation than the Terelj site. From 2010–2012, meteorological and ground thermal data are available from a forest research tower in a boreal forest stand (Miyazaki et al., 2014) ca. 500 m away from our grassland sites (Fig. 1b). The meteorological conditions in Udleg are similar to those in Terelj, with monthly air temperatures ranging from −20 to −25 °C in winter to +15 to +20 °C in summer and annual rainfall between 230 and 317 mm. Miyazaki et al. (2014) found warm permafrost below the forest in Udleg, but the ground thermal state below the nearby grassland site is unknown. Vegetation surveys in Udleg in 2014 and 2015 show Asteraceae, Fabaceae and Caryophyllaceae to be the most abundant grassland vegetation (Lkhavgadorj et al., 2016). The research station and nearby grasslands are surrounded by a network of fences, which restrict livestock activity and thus create a gradient of grazing intensities at this site. For this reason, the upper parts of the slope are largely protected from livestock, while the lower part outside the fence system is intensely grazed. During the first part of our measurement period (Sect. 3.1), a breach in an outer fence allowed for low-intensity scattered grazing on the upper, southern part of the slope.
In Udleg, we establish three field plots aimed to capture the gradient in livestock activity (Fig. 1d). This includes an “open” plot on the intensely grazed lower part of the slope and two fenced plots ca. 400 m away in the upper part of the slope. These latter plots target the difference in vegetation on each side of the fence between the two enclosures, where scattered grazing occurred in the outer part (“fenced A”) due to a breach in the outer perimeter. This breach was repaired in spring 2023, and no livestock was allowed within fenced plot A during summer 2023. On the other side of the fence, there is a smaller enclosure that was protected from grazing in both summer 2022 and 2023 (“fenced B”). However, livestock was herded into fenced plot B for a period in winter 2023, leading to substantial trampling and grazing of standing litter. Similar to the Terelj site, the logger placement in Udleg avoids potential lee effects behind the fences, as the fences run roughly parallel to the main wind direction in Udleg, which is along the main valley (east-northeast–west-southwest; Fig. 1b, d).
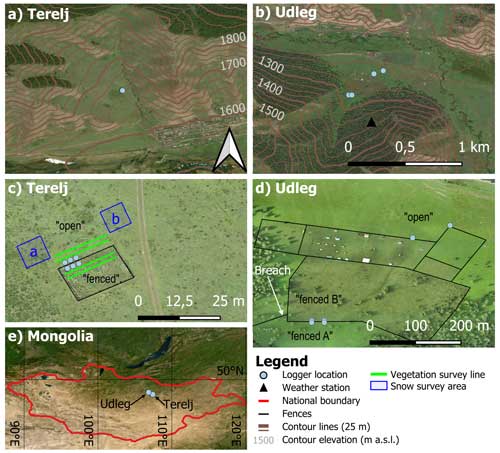
Figure 1Overview showing the location of the temperature loggers in Terelj (a, c) and Udleg (b, d) and the location of our study sites within Mongolia (e). The location of our plots within each site is indicated by black text in (c) and (d), while the white arrow shows the location of the fence breach in 2022. The boxes a and b in (c) indicate the snow surveys of areas with and without recent signs of trampling, respectively. All figures are oriented with north facing up, and (a) and (b) have the same scale. Background: (a), (b), and (e) are satellite imagery (ESRI, 2023), and (c) and (d) are drone-based orthomosaics acquired on 17 and 15 August 2023, respectively, and processed in Agisoft Metashape (Agisoft LLC, 2023).
3.1 Ground surface temperature monitoring
In this study, we used iButton (© Maxim) temperature loggers that were installed at the different plots in summer 2022 (Table 1). These loggers are small (ca. 1.5×0.3 cm) and can be inserted at 2–3 cm below in the ground surface with minimal disturbance to vegetation and soil matrix. We use iButton loggers (DS1925 and DS1922L) that feature an accuracy of 0.5 °C, a numerical resolution of 0.0625 °C, and an operation range from −40 to + 85 °C (Maxim Integrated, 2015, 2020). For this study, we compute daily average GSTs from measurements at 4 h intervals.
The logger placement aims to capture the variation in livestock activity and terrain among the plots. In Terelj, we deploy three logger pairs (numbered 1–3 from west to east; Fig. 1c), each consisting of two loggers that are placed 2 m away from the fence on each site. This setup allows for redundancy in case of logger failure while also capturing some of the spatial variability within the open-fenced and fenced plots. In Udleg, we first placed two logger pairs along the fence between fenced plots A and B, with each logger 3 m from the fence. Later in summer 2022, we supplemented loggers at two more locations in the open plot ca. 400 m away. The individual logger locations in Udleg are named “west” and “east”, based on their relative location within each plot (Fig. 1d). From the individual logger measurements, we calculate daily average GSTs within each plot, which we use throughout our analysis (Sect. 4.3). For all logger locations we derived local terrain parameters from the GLO-30 digital elevation model (DEM) by ESA and Sinergise (2021). Furthermore, we quantify the differences in solar radiation among our plots by calculating the mean annual incoming solar radiation as in Zweigel et al. (2024), which considers the effects of both local slope and blocking by surrounding terrain (Table 2).
We calculated seasonal and annual average GSTs for all loggers with sufficient data. To ensure direct comparability among mean annual GSTs (MAGSTs), we calculated these over the 365 d period ending on 13 August 2023, which is contained in all logger measurements. For comparison of seasonal temperature signals, we averaged daily GSTs for 3-month periods from June 2022 to August 2023. As loggers were placed and read out during summer, we did not obtain a complete record for the summer season (June, July, and August) for all loggers. For the analysis, we still provide an estimate of the mean GST for summer for plots where daily GSTs are available for at least two-thirds of the days.
Table 1Overview of ground surface temperature measurements used in this study. Coordinates were obtained from phone GPS. An asterisk indicates loggers that are placed substantially closer than the resolution of the DEM (30 m) and are thus considered to have the same position and terrain parameters as in the further analysis.
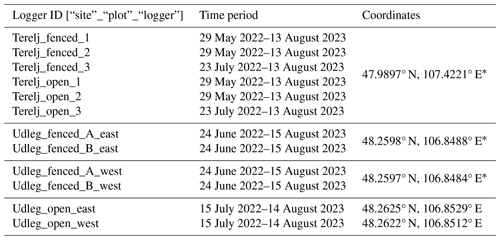
Table 2Terrain parameters for the GST measurement locations as derived from the GLO-30 DEM. Aspect is given in degrees anticlockwise from south following the right-hand notation by Dozier and Frew (1990), with compass directions indicated in brackets. SVF: sky view fraction. Sin: mean annual incoming solar radiation, calculated over the same period as MAGSTs (Sect. 4.3) using ERA5 reanalysis data (Hersbach et al., 2020) and the terrain adjustment methodology in Zweigel et al. (2024).
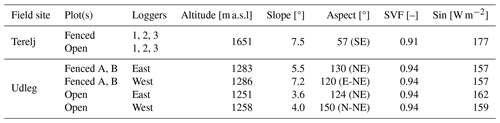
3.2 Additional field observations
We assessed the surface cover at the fenced and open plots in Terelj and Udleg in summer 2022 and 2023, as well as winter 2023. During the growing season, we measured the typical vegetation height and took hemispherical photographs at the ground surface from which vegetation density was estimated (see below). We also noted the occurrence and thickness of intact litter layers at the ground surface and the bare soil fraction, i.e. the surface fraction not covered by vegetation. For consistency, we use the term “vegetation” for live plants, whereas “litter layers” or “standing litter” is used respectively for intact, dead plants that stand upright or form a largely horizontal layer near the ground surface. During the winter field campaign, we surveyed snow conditions with a focus on spatial variability due to livestock activity. These measurements of vegetation and snow properties were done in the immediate vicinity of the GST logger locations (Table 1, Fig. 1), so as to avoid disturbance of the ongoing measurements. In Terelj, we also conducted a more comprehensive survey of vegetation and snow cover in winter and summer 2023 to capture the small-scale spatial variability in the near surroundings of the logger locations. For these surveys, vegetation was surveyed at 30 points along lines in the open and fenced plots, while snow cover was surveyed at 100 points within two areas in the open plot (Fig. 1c).
We used the hemispherical photographs to estimate leaf area index (LAI) of the grassland vegetation. The concept is to distinguish between pixels containing vegetation and sky across the 180° hemisphere up-looking perspective and calculate the leaf area per unit ground area that would produce such a fractional distribution of vegetation gaps. While such LAI calculations are subject to assumed vegetation properties and are sensitive to lighting conditions and exposure (Thimonier et al., 2010; Zhang et al., 2005), they provide a quantitative measure of vegetation density that can be compared among plots. In this study we estimated LAI with the software Hemisfer (Schleppi et al., 2007) using the methodology by Lang (1987). An overview of the processing of hemispherical photographs is provided in Appendix A.
At each plot, we measured snow depths and densities in winter 2023. The number of measurements per plot depended on the apparent variability of the snow cover at the time of visit. We determined the column-average density of snow from the mass of snow sampled by a cylinder of known dimensions that was vertically inserted into the snowpack. For sites featuring a clear layering, we also determined the density for the dominant layers. Furthermore, at each plot we observed the dominant snow grain sizes and litter content within the snow layers.
4.1 Summer – vegetation
4.1.1 Terelj
At the Terelj site we find strong contrasts in vegetation height and density between the open and fenced plots (Fig. 2). In late May 2022, the open plot featured only low grass vegetation, with a bare soil fraction around 50 %. At the same time, the ground at the fenced plot was completely covered by a thick litter layer, with no live vegetation visible (Fig. 2a). By July 2022, a thick vegetation cover had established at the fenced plot, while the ground below was still covered by a litter layer. At this time the grass at the open plot was still low with a high bare soil fraction (Table 3), except for scattered patches of Artemisia frigida (Fig. 2c). A similar vegetation cover was found in August 2023 (Figs. 2b, d, 4a), where a survey of 30 points at the open plot showed a median vegetation height of 3 cm and 10 % of the area covered by ca. 20 cm tall Artemisia frigida (Fig. 3a). This survey also showed that large parts of the ground surface at the open plot were still exposed to the atmosphere (Fig. 3b). A similar survey of 30 points at the fenced plot showed that while standing vegetation was ca. 40–45 cm tall, the median height was 20 cm due to large parts of the grassland vegetation being blown over at this time (Figs. 2d, 3a, 4b–c). The survey at the fenced plot also showed that the ground was covered by a thick litter layer with a median thickness of 6 cm (Figs. 2b and 3c).
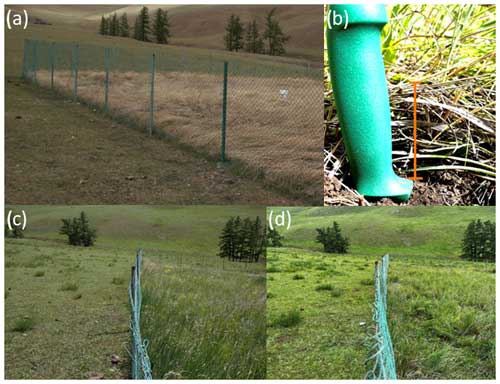
Figure 2The evolution of vegetation and ground cover at the Terelj site throughout the summer season. (a) For 29 May 2022, the open plot featured low grass vegetation, while the fenced plot was covered by a thick litter layer. (b) For 17 August 2023, there was an example of the litter layer which at all times covered the ground surface in the fenced plot. (c) For 16 July 2022, continuous vegetation cover of 30–50 cm had formed at the fenced plot, while the open plot was covered mostly by low vegetation. (d) For 17 August 2023, the open plot still featured sparse vegetation, while large fractions of the vegetation at the fenced plot had fallen/blown over.
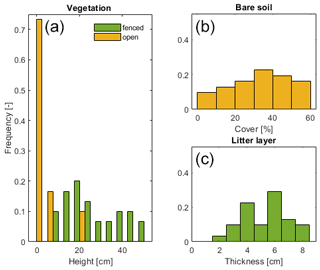
Figure 3Observations from the vegetation survey in the Terelj site on 17 August 2023 (n=30 in each plot). (a) Distribution of vegetation height in the open (median = 3cm) and fenced plot (median = 20 cm). (b) Distribution of bare soil fraction at the open plot (median = 30 %). (c) The thickness of intact litter layers covering the ground surface at the fenced plot (median = 6 cm). For readability, the bars in (a) are plotted next to each other within each 5 cm interval.
The LAI estimates differ considerably between the open and fenced plots in Terelj, showing a similar pattern to vegetation height (Fig. 4a–c and Table 3). We found that throughout the summer, LAI at the open plot is at least 1 order of magnitude smaller than at the fenced plot.
Table 3Estimated LAI and vegetation height for the plots in Terelj from field visits in summer 2022 and 2023. LAIs are the mean of observations listed in Table A1. An asterisk denotes vegetation heights are the median of the survey presented in Fig. 3a. n.m.: not measured.
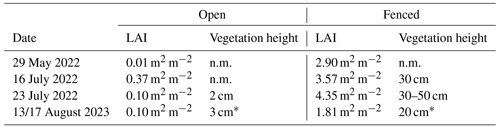
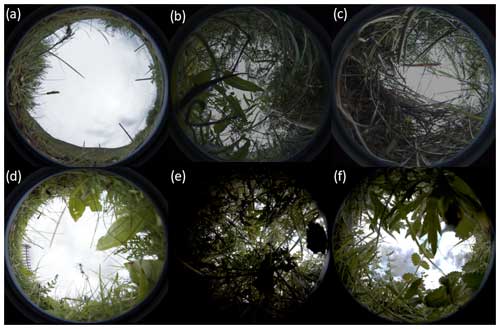
Figure 4Example of hemispheric photographs used to derive LAI. Terelj: (a) 13 August 2023 for open plot (LAI = 0.19), (b) 13 August 2023 for fenced plot (LAI = 2.10), (c) 13 August 2023 for fenced plot with wind-blown vegetation (LAI = 1.77). Udleg: (d) 16 August 2023 for open plot (LAI = 0.77), (e) 18 July 2022 for fenced plot B (LAI = 4.65), (f) 18 July 2022 for fenced plot A (LAI = 2.71).
4.1.2 Udleg
The three plots in Udleg feature a gradient in vegetation cover (Fig. 5). Vegetation heights were lower in fenced plot A compared to fenced plot B in June and July 2022, which is likely due to a fence breach that allowed for scattered grazing at fenced plot A. This difference in vegetation height was maintained even after the fence breach was repaired in spring 2023 (Table 4 and Fig. 5c). Contrary to the fenced plot in Terelj, we only observed shallow litter layers in Udleg, measuring 0.5 and 1 cm at fenced plots A and B, respectively, in August 2023. The vegetation height at the open plot in Udleg was 5 cm in both July 2022 and August 2023 (Table 4) but was denser than at the open plot in Terelj and completely covered the ground surface (Figs. 5b and 4d).
The LAI-based estimates of vegetation density at Udleg provide additional insight into the vegetation height measurements (Table 4). When fenced plot A was subject to scattered grazing in 2022, it had a considerably lower LAI than the ungrazed fenced plot B. However, in 2023, when both fenced sites were protected from grazing, fenced plot A reached a somewhat higher LAI than fenced plot B. We note that this was contrary to the observed vegetation heights, which still were markedly higher at fenced site B.
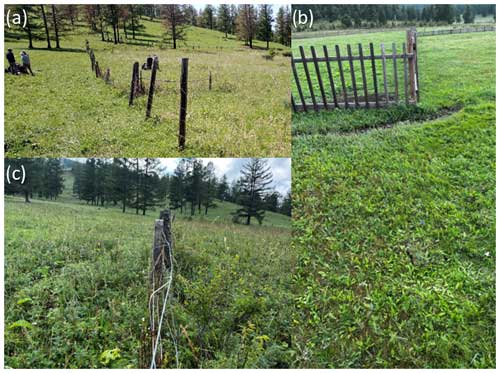
Figure 5Vegetation cover at the plots in the Udleg study area. (a) On 24 June 2022, a fence breach led to sparse grazing and lower vegetation heights left of the depicted fence (fenced plot A). There is higher vegetation to the right of the fence where no grazing animals have access (fenced plot B). (b) On 18 July 2022, the open plot is characterized by intense grazing and low vegetation. (c) On 14 August 2023, after repairing the fence in spring 2023, the vegetation density is more similar in fenced plots A and B.
4.2 Winter
4.2.1 Terelj
The snow cover in the fenced plot in Terelj was deeper than in the open plot, and it was substantially affected by the presence of litter in late winter 2023 (Fig. 6). Data from two snow pits at the fenced plot showed the snowpack to be 21–32 cm deep, with a clear layering due to a basal litter layer. The top layer of snow was 7–14 cm thick with a density of 215 kg m−3, which was located on top of a 14–18 cm basal layer consisting of a mix of intact litter and snow. In this basal layer, the litter component provided structural support for the top snow layer, and the snow matrix was discontinuous and dominated by chains and pockets of depth hoar, with a snow density of 135 kg m−3. In many places, standing litter also protruded through the snow surface (Fig. 6).
In the open plot, the snow cover was rather shallow and did not show any clear horizontal layering. In the immediate vicinity of the GST logger locations, we measured 10–15 cm deep snow that did not show signs of disturbance due to trampling since the last snowfall. However, trampling due to livestock grazing was evident in the wider, open area, leading to the compaction or locally even the complete removal of snow cover. We investigated the effect of trampling on the variability of the snow depths by measuring 100 sites in two areas, one of which had experienced substantial trampling, while the second showed no signs of trampling since the last snowfall (Fig. 1c). Figure 7 shows that while the average snow depth in these areas was comparable, there is a stronger variability in snow depths in the trampled area. We also sampled column-average snow density at 25 locations in the visually undisturbed area, yielding an average snow density of 166 kg m−3.
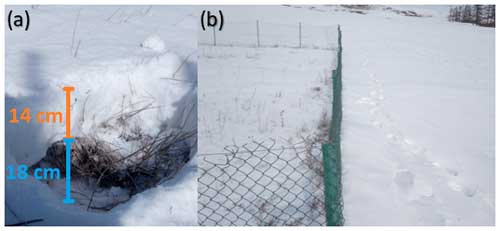
Figure 6Snow cover at the open and fenced plot in Terelj (27 February 2023). (a) At the fenced plot, a denser layer of snow sits on top of a thick litter layer and depth hoar. (b) Standing litter still protrudes through the snowpack at the fenced plot. Snow cover in the open plot is more continuous and characterized by local trampling.
4.2.2 Udleg
At Udleg, the snow cover is characterized by high spatial variability due to livestock trampling. Livestock had access to all plots in the period prior to our field visit in late February 2023 (Fig. 8), with the most intense trampling visible at fenced plot B. Fenced plot B featured a large variability in both snow depth and density, but undisturbed locations in both fenced plot A and B showed snow depths of 20 cm or more (Fig. 9). However, locations visually undisturbed at our time of visit could have experienced previous disturbances that since have been infilled with snow, which could lead to the large variability of snow densities in fenced plot B (Fig. 9). While there was also scattered trampling at the open plot, no disturbances were observed in the vicinity of the logger locations at the time of our field visit. At the open plot, snow densities were consistently around 180 kg m−3, while snow depths were consistently lower than undisturbed locations in the fenced plots (Fig. 9). The variability in snow depths in the open plot is likely linked to local wind redistribution of snow, consistent with observed wind erosion features at the snow surface (Fig. 8b). We also observe a basal layer with high content of standing litter in the snowpack at both fenced plots (Fig. 10).
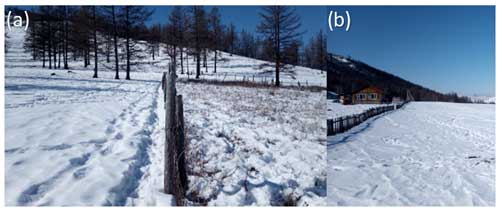
Figure 8Snow conditions in the Udleg study area on 25 February 2023. (a) Fenced plot A (left of fence) received less trampling than fenced plot B (right of the fence). (b) Open site with scattered livestock tracks and wind erosion features at the snow surface.
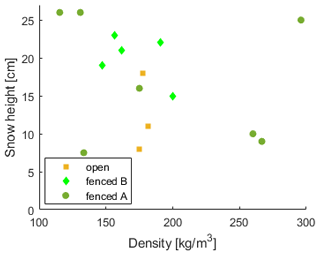
Figure 9Snow measurements from Udleg on 25 February 2023. Observations were made in the immediate vicinity of the GST logger locations, showing the spatial variability of snow depth and densities in each plot.
4.3 Ground surface temperatures
4.3.1 Terelj
The open and fenced plots in Terelj display different seasonal patterns in GSTs. Differences are largest between April and September when GSTs in the intensely grazed open are substantially warmer than at the ungrazed fenced plot and from December to February when GSTs in the open are colder (Figs. 11, 12). Notably, the open and fenced plots feature rather similar GSTs for 2 months from the onset of freezing in September until the middle of November, after which GSTs are strongly different between the plots for the remainder of winter. This sudden deviation of GSTs could be related to the start of significant livestock activity at the logger locations in the open plot, which disturbed the snow cover. While the absolute differences in summer and winter GSTs are of a similar magnitude, the open plot features warmer GSTs also in spring and autumn (Table 5). Over the entire year, the open plot features a 0.7 °C warmer MAGST than the fenced plot.
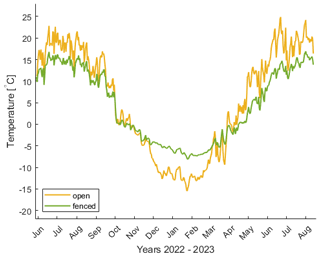
Figure 11Average daily GSTs for the plots in Terelj. The GST evolution of the individual loggers is presented in Fig. B1.
Table 5Annual and seasonal 3-month average GSTs for the plots in Terelj. JJA: June, July, and August. SON: September, October, and November, DJF: December, January, and February, MAM: March, April, and May. MAGST is calculated over 365 days, ending on 13 August 2023. Corresponding annual and seasonal GSTs of the individual loggers are presented in Table B1.

4.3.2 Udleg
At Udleg, the differences in ground temperatures between open and fenced plots are less pronounced compared to Terelj (Fig. 12). However, GSTs are still significantly warmer in the open plot in summer, but they are colder than in the fenced plots not only in winter but also in autumn and spring (Fig. 13 and Table 6). The largest difference in GSTs between the open and fenced plots is observed in winter, while the differences in the transitional periods in spring and autumn are small. Overall, MAGSTs are lower in the open plot compared to the fenced plots, but this difference is smaller than the spatial variation within each plot (Table B2). Similar to Terelj, we note a strongly deviating GST evolution for one logger in the open plot starting in December, which potentially is linked to the onset of snow disturbance by livestock (Fig. B2). Furthermore, we note that the annual and most seasonal GSTs are colder in Udleg compared to the corresponding plots in Terelj, despite Udleg being located at ca. 300 m lower elevation, which is likely caused by the difference in aspect.
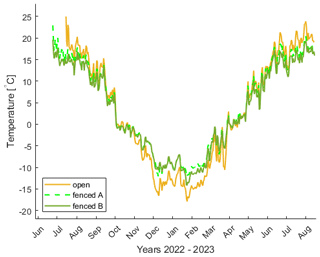
Figure 13Daily GSTs from the loggers placed in Udleg. Note that GSTs are measured at fenced plot A and B from 24 June 2022, while the open plot was added on the 15 July 2022. The GST evolution of the individual loggers is presented in Fig. B2.
Table 6As Table 5 but for the plots in Udleg. Note that the loggers in the open have insufficient measurements for JJA in 2022. Corresponding annual and seasonal GSTs of the individual loggers are presented in Table B2.
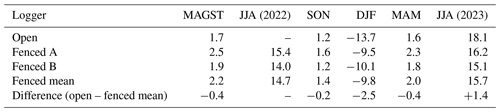
We also observe differences in annual and seasonal GSTs between the two fenced plots in Udleg (Table 6). Fenced plot A, which has lower vegetation height and density (Table 4), has higher GSTs than fenced plot B (Fig. 13). This difference is consistent throughout the year, and plot A has average MAGSTs 0.6 °C warmer than plot B (Table 6). The largest temperature difference between the two fenced plots is observed in the summer seasons, also in 2023 when both fenced plots were protected from grazing (Table 6 and Fig. 13). However, fenced plots A and B feature a similar seasonal evolution of GSTs, distinctly different from the open plot.
In this study, we use measured GSTs and observations of grassland vegetation and snow cover from the Khentii Mountains in central Mongolia to quantify and compare the different temperature regimes across contrasts of grazing intensity and topography. Overall, we find that in this landscape dominated by traditional pastoralism, the exclusion of grazing livestock allows for higher and denser vegetation cover (Tables 3 and 4) and a dampened seasonal cycle in GSTs (Figs. 11, 13). Furthermore, we find that topographic exposure shapes the local GST regime with the largest differences between intensely grazed and ungrazed plots observed at the south-facing Terelj site. Here, monthly GST differences range from −5.8 °C colder to 6.8 °C, whereas at the north-facing Udleg site, monthly GST differences range from −4.1 to 3.6 °C (Fig. 12). The effect of intense grazing on MAGSTs is however small, with the open plot being 0.7 °C warmer than the fenced plot in Terelj, while it is 0.4 °C colder in Udleg (Tables 5 and 6). The difference in MAGSTs is small compared to differences in monthly GSTs, which range from −5.8 °C colder to 6.8 °C warmer at the south-facing Terelj site and from −4.1 °C colder to 3.6 °C warmer at the north-facing Udleg site (Fig. 12).
Our study complements previous research on the relationship between grazing intensity, vegetation cover, and ground temperatures. For example, a recent study found that removal of vegetation in cold climates can affect summertime GSTs through reduced shading (Jaroszynska et al., 2023). Vegetation removal is a main grazing effect, which we find to cause higher summertime GSTs at grassland sites in semi-arid central Mongolia. Furthermore, several studies have shown an intensification of the annual GST cycle with grazing intensity leading to several degrees higher GSTs in summer that are partly offset by colder wintertime GSTs (Yan et al., 2018; Zhao et al., 2011). However, most previous research linking livestock activity and GST dynamics have used controlled experiments, and the effect of grazing gradients within a landscape dominated by pastoralism has received little attention. Our observations of GSTs and surface conditions under direct grazing effects thus represent a valuable dataset on how these effects manifest at sites with traditional livestock management.
5.1 Grazing impact on ground surface temperatures
Livestock grazing and trampling changes the surface properties at grassland sites, including reduced vegetation cover and compaction of ground, snow, and litter (e.g. Gan et al., 2012; Yan et al., 2018; Zimov et al., 2012). These changes modulate the exchange of energy between the atmosphere and ground, which drive the observed differences in GSTs between open and fences areas. The altered surfaces interact with other factors and processes, such as wind redistribution of snow and terrain-driven differences in radiation. Here, we provide an overview of the processes relevant at our sites and relate them to observations of surface properties and GSTs.
Vegetation cover intercepts solar radiation, which leads to a lower net shortwave radiation at the ground surface. Such shading of the ground below lowers GSTs during the growing season (e.g. Yan et al., 2018). Zhang et al. (2022) showed that this shading effect increases with vegetation density and is greater for live than dead vegetation. Furthermore, Shao et al. (2017) found that although grazing reduced the net radiation above grassland sites due to a higher albedo, it also led to a higher ground heat flux. These findings agree well with the dampened seasonal cycle and lower summertime GSTs observed at the fenced plots at the sites in Terelj and Udleg (Figs. 11, 13).
We also find differences in GSTs between open and fenced plots outside the growing season, especially during late spring in Terelj (Fig. 12). Here, the fenced plot warms considerably more slowly than the open plot, which could be linked to the insulation of the litter layers that were observed in summer. Such litter layers affect the ground surface thermal regime by intercepting radiation, limiting turbulent exchange, and lowering the ground heat flux (Yan et al., 2018). This reduced energy transfer can for example slow ground warming in spring, leading to a delayed plant phenology, increased soil moisture, and lower GSTs (Hou et al., 2020). While the exact role is unclear, litter layers could thus be an important mediator of GSTs at the Terelj site, especially in spring when all plots are snow-free, and grazing-induced differences in vegetation have not yet become manifest.
Livestock activity affects the snow cover directly through trampling (see below) as well as indirectly by inducing local variations in snow ablation. Sublimation of snow can remove a substantial part of the available snow cover at continental sites (Zhang et al., 2004, 2008), and the rate of sublimation depends heavily on the snow surface temperature and consequently the surface energy balance. During our field visit in winter we observe snow-free conditions on steeper south-facing slopes that receive high insolation in winter and early spring, and Sentinel-2 satellite imagery confirms that snow cover on these slopes is of intermittent nature (Copernicus Browser: Sentinel-2 L2A True colour imagery). Similarly, areas with trampling by livestock will feature a snow microtopography where small south-facing surfaces are likely subject to increased sublimation, thus potentially reducing the snow cover compared to undisturbed areas.
Wind redistribution of snow can be the main driver of snow depth variability within an area, which, especially at cold sites, can drive large differences in wintertime GSTs (Zweigel et al., 2021). In the open plot at Udleg, wind is channelled through the main valley, and we observe snow structures associated with wind erosion the measurement sites (Fig. 8b), as well as deeper snow drifts in the surrounding area. We also observe a snow cover with relatively homogeneous density but variable snow depths (Fig. 9), which could be explained by the effects of wind redistribution. Wind-induced differences in snow depth could thus be an additional factor causing the difference in wintertime GSTs, possibly in interplay with vegetation removal by grazing. Yan et al. (2019) found that intense grazing can substantially reduce the snow accumulation at grassland sites, which they link to the lowering of snow holding capacity due to lower vegetation. Such differences in wind redistribution might also explain some of the differences in snow depth between the open and fenced plots at both sites, where we observed the snowpack in the open plots to be thinner than in the fenced plots, which also feature substantial standing litter within the snowpack (Figs. 6, 10). Wind redistribution of snow further interacts with snow trampling by grazing livestock by infilling depressions caused by grazing animals, thus again smoothing the surface. While both these changes to the snow cover are event-based, trampling is a highly local and random effect, while wind distribution is spatially more consistent. Spatial differences in snow depth induced by livestock activity can be smoothed out by subsequent wind drift events, which affects snow cover and densities. Overall, the effect of wind on snow cover and its interaction with direct and indirect grazing effects must be considered at grassland sites when studying local variations in wintertime GSTs.
Vegetation densities at grazed plots vary between our sites, which can be related to differences between south- and north-facing slopes with respect to grazing intensity and soil moisture availability. Throughout our observations, the open plot in Udleg features a continuous grass cover and higher LAI than the open plot in Terelj, which also has a high fraction of bare soil (Figs. 3b, 4a, and d and Tables 3 and 4). These variations in vegetation density could be linked to differences in livestock activity, which can be induced by seasonal grazing patterns where, for example, south-facing slopes are preferentially grazed in summer (see below). Alternatively, terrain-induced differences in solar radiation (Table 2) can drive stronger evaporation on south-facing slopes, which can limit the soil moisture available for plant growth. For example, previous studies have shown that evapotranspiration consumes all available precipitation and soil moisture on south-facing slopes in the Terelj area, while a surplus of soil water is available below forested north-facing slopes nearby (Iijima et al., 2012). Soil moisture levels are also affected by recharge from snowmelt, which in this region is affected by sublimation that consumes 20 %–29 % of the annual snowfall at flat sites (Zhang et al., 2008). Zweigel et al. (2024) showed that gentle south-facing slopes in Terelj receive more solar radiation than north-facing slopes especially during winter, autumn, and spring, which can drive a faster ablation of snow cover in spring. With less snow water equivalent available for infiltration and an earlier snow melt-out, a soil moisture deficit on gentle south-facing slopes could thus build up already prior to the main growing season. Additionally, plant growth in north-facing slopes, such as Udleg, can be supported by meltwater inflow from local permafrost-underlain forest (Klinge et al., 2021). As gentle north- and south-facing slopes receive rather similar solar radiation in summer (Zweigel et al., 2024), differences in summertime GSTs among the grazed plots in Terelj and Udleg are mostly related indirectly to terrain exposure through its impact on local vegetation density.
Whether grazing leads to higher or lower MAGSTs depends on the relative magnitude and timing of summer and winter effects. The Terelj and Udleg sites mainly differ in the incoming solar radiation (10 %–13 % higher in Terelj; Table 2) and the quantity of litter layers in the fenced plots. The higher MAGSTs in Terelj for both open and fenced plots, despite this site being located more than 300 m higher than Udleg, suggest that the solar radiation is a key driver of ground thermal regime at this south-facing site. As solar radiation is most intense in summer, the effects of shading vegetation during the growing season dominate the annual GST signal at this site. The difference in GSTs in Terelj is further increased by the slower warming of the fenced site due to thick litter layers, with the largest effect in late spring (Fig. 12). Overall, the Terelj data indicate warmer MAGSTs in response to intense grazing. In contrast, the Udleg site displays more similar GSTs across all plots in the warm season, and the net effect of grazing on MAGSTs depends largely on wintertime effects (Table 6). Here, local disturbances of the snow cover due to wind drift and livestock grazing seem to determine whether MAGSTs in the open are higher or lower than those at fenced plots (Table B2).
Previous studies have suggested that snow trampling by livestock has a strong and decisive impact on the ground thermal regime, leading to generally colder ground temperatures (e.g. Zimov et al., 2012). For Siberia, Zimov et al. (2012) report that herbivores are limited by the available feed during long winters, while grasslands can establish a substantial vegetation cover during moderate grazing in summer. In such a system, vegetation can shade the ground from solar radiation during the growing season, while the snow cover in winter is subject to extensive trampling, producing overall cooling of ground temperatures. Our study region is located about 20° further south compared to the site studied by Zimov et al. (2012) and thus experiences higher amounts of solar radiation, as well as a shorter winter and longer and warmer growing seasons. Here, we observe intense grazing to limit the establishment of substantial vegetation cover in grazed areas in summer (Tables 3 and 4), leaving the ground directly exposed to the atmosphere especially on south-facing slopes (Sect. 4.1.1 Terelj). While we did not observe direct trampling at our logger sites during the visit in winter, we observed that trampling leads to increased variability of snow depths and densities (Figs. 7, 9), and we note sudden shifts in GSTs during winter for loggers in the open plots (Terelj: mid-November (Fig. 11); Udleg: start of December (Fig. B2)), which are highly likely caused by livestock disturbance of the local snow cover. These grazed and trampled loggers experience the coldest wintertime GSTs in Terelj and Udleg, respectively, but we do not observe a general cooling on an annual basis (Tables 5 and B2). Notably, the disturbed loggers in Terelj feature higher MAGSTs than the undisturbed loggers in the fenced plot, indicating that wintertime disturbances do not dominate the annual GST cycle at this site. Overall, the effects grazing has on GSTs during the growing season seem to dominate the livestock impact on MAGSTs in our study region.
5.2 Limitations
Our study presents 14 months of GST measurements, which is a limiting factor for the analysis of the interplay of grazing, vegetation, and GSTs. However, multi-year studies in grassland ecosystems show similar seasonal differences in GSTs to those found in this study (Yan et al., 2018; Zhao et al., 2011). For example, Yan et al. (2018) observed summertime soil temperatures in heavily grazed plots on average 2.6 °C warmer than in ungrazed plots across years with highly variable precipitation conditions. Furthermore, Zhang et al. (2011) analysed 5 years of data from Inner Mongolia and found that denser vegetation cover at ungrazed sites provides insulation, resulting in colder summertime and warmer wintertime soil temperatures. Thus, our observations likely capture the key properties and dynamics of grazed and ungrazed grasslands for these latitudes.
The complementary field observations of surface conditions are limited to summer and late winter, which limits our investigation of the causal relationships between livestock activity, ground surface conditions, and GSTs at other times. The presence of reflecting and insulating snow cover or litter layers will significantly impact the rates of ground warming and cooling. For example, we note strong deviations in wintertime GSTs in some plots that are likely linked to disturbance of surface cover (Figs. 11, B2) but lack observations for the direct attribution of these events to livestock trampling. However, Sentinel-2 satellite imagery shows well-established snow cover at both field sites at these times (since 31 October at both sites; ESA, 2024), and the deviating GSTs are thus likely linked to local disturbances of snow cover. Similarly, our interpretation of litter layers as the main cause of delayed ground warming in spring in Terelj remains uncertain without information on surface conditions at that time. To investigate the role of grazing outside the growing season, future studies should include observations of surface conditions in spring, autumn, and early winter.
Our study sites represent differences in livestock activity where the grazing intensity is not controlled (but rather a product of local livestock) and herders search for optimal feeding conditions. For example, the fenced plot B in Udleg was subject to substantial trampling and winter grazing, and we measured a large variability of snow depth and densities in this plot in late winter (Figs. 8, 9). However, we do not observe different GSTs between fenced plot B and the visually undisturbed fenced plot A, neither prior nor after our field visit (Fig. 13). It is unclear whether this is because GSTs are rather insensitive to variations in snow cover towards the end of the cold season or whether the GST logger locations were largely unaffected by the disturbances. Conversely, a fence breach that was repaired in spring 2023 allowed scattered grazing in fenced plot A during the first part of our measurement period. While we do observe differences in vegetation cover between fenced plots A and B in both summer 2022 and 2023 (Fig. 5a, c), these plots feature highly similar GSTs throughout the measurement period (Figs. 13 and Table 6). Overall, the variability in GSTs among the fenced plots in Udleg is small compared to the difference between the fenced plots and the intensely grazed open plot.
5.3 Future research
The timing and intensity of grazing and trampling depends on the type and number of livestock, which is controlled by herding practices, such as seasonal movement of pastures. The seasonally contrasting effects of grazing on GSTs indicate that the seasonality of pasture use can be crucial for the net impact on GSTs. Only few studies have investigated how active herding and seasonal migrations localize grazing in time and space, but Lkhagvadorj et al. (2013) provide an overview from the Khangai Mountains (central Mongolia): flat grasslands in the mountain outskirts are the main grazing areas, while forest edges are grazed mostly at the end of the growing season. During autumn, winter, and spring, herder families relocate livestock to drier areas where they have designated winter camps (Lkhagvadorj et al., 2013). Such preferential grazing of forest edges in autumn could allow grass to grow tall in summer while inhibiting buildup of litter layers prior to winter, leading to overall colder GSTs in these areas. Klinge et al. (2021) report permafrost extending several tens of metres into the steppe downslope of a permafrost-underlain forest in the Khangai Mountains, which could be partially supported by such seasonal grazing patterns. However, it is unclear whether this movement of pastures is also prevalent in our study region in the Khentii Mountains, as we observe livestock at our field sites also in winter. Furthermore, the composition of livestock in Mongolia has shifted towards more goats, which have the strongest impact on grassland ecosystems (Saizen et al., 2010). Such changes in livestock composition can possibly influence local grazing patterns, especially in winter when snow depth might limit smaller animals to areas that are more exposed to wind erosion or ablation such as ridges and south-facing slopes. Local herders in Udleg also report that smaller livestock such as sheep and goat are herded into enclosures overnight during winter, while horses, yak, and cattle largely roam freely. If widespread, such active herding in winter will localize the impact this livestock has on both vegetation and snow cover. Understanding and quantifying the impact of spatiotemporal patterns of livestock activity on GSTs are beyond the scope of our study at this time and should be a topic for future research.
The observed differences in GST seasonality and dynamics have the potential to affect the subsurface thermal regime, including the presence of permafrost. In general, the thermal offset, i.e. the difference between MAGSTs and annual average temperature at the base of the seasonally frozen ground or top of permafrost, increases with increasing annual GST range (Smith and Riseborough, 1996). As we observe an amplified seasonal GST cycle in the intensely grazed open plots (Fig. 12), deeper ground temperatures could be lowered below these plots compared to the adjacent fenced sites. However, livestock activity also limits the buildup of insulating soil organics (e.g. Gan et al., 2012; Odriozola et al., 2014) and leads to a drying of ground surface layers (e.g. Yan et al., 2018; Zhao et al., 2011), which is usually associated with a lower thermal offset (Romanovsky and Osterkamp, 1995; Smith and Riseborough, 1996). Due to these opposing effects, the net impact of grazing on the thermal offset is not clear.
Further studies could explore the potential of livestock management to lower ground temperatures at grassland sites, which could be a relevant strategy to protect marginal permafrost. Ishikawa et al. (2018) report degradation of permafrost across Mongolia in response to current warming, especially in low elevations and in grassland ecosystems, which coincides with the main rangelands. This study shows that excluding livestock leads to the formation of dense vegetation in grasslands, which lowers summertime GSTs by several degrees. This insight could be used to inform land use strategies, where, for example, animals are excluded from grasslands with marginal permafrost during the growing season, while intense grazing or hay harvesting in autumn is used to limit the buildup of insulating litter layers and vegetation snow capture during the cold season. We find that combining GSTs from the fenced plot (April–October) with the GSTs from the open plot (November–March) would lower MAGSTs by 0.8–1.3 °C in Udleg compared to the open and fenced plots, respectively. Such land-use-induced cooling of local GSTs could partially offset the 2.2 °C increase of air temperatures observed across Mongolia (1940–2015; Zamba, 2018), potentially extending the climatic window for permafrost occurrence that is vital for downstream water supply and ecosystem function (Dashtseren et al., 2021; Ishikawa et al., 2018; Klinge et al., 2021). While the GST cooling effect of such grazing management or hay production would be greater on south-facing slopes (1.5–2.5 °C in Terelj), these slopes are generally permafrost-free (e.g. Dashtseren et al., 2014; Ishikawa et al., 2005).
Finally, the rate of carbon cycling in grasslands is greatly affected by grazing, which removes above-ground carbon and can drive long-term shifts in plant composition (Liang et al., 2021). The net effect of grazing on soil carbon stocks depends on factors such as precipitation, soil texture, and dominant grass species; both an increase and a decrease in soil organic carbon with increasing grazing intensity have been reported (McSherry and Ritchie, 2013). In this study, we have studied GSTs on grazed and ungrazed plots, which can further affect the rates of soil carbon cycling. Higher ground temperatures generally stimulate soil microbial activity and associated soil respiration, increasing the release of carbon to the atmosphere (e.g. Schindlbacher et al., 2009). However, the effect of ground temperatures on soil carbon cycling can be limited by available soil moisture (e.g. Poll et al., 2013). We thus suggest future studies to investigate the rates of carbon uptake and release across the grazing gradients detailed in this study. Such investigations can reveal the potential impact of livestock management on the carbon balance of Mongolian grassland ecosystems.
In this study we investigate the variations of ground surface temperatures (GSTs) across local gradients of grazing intensity in the mountains of central Mongolia. We present 14 months of measured daily GSTs from fenced and open plots at two field sites with different topographic aspects and grazing history. These measurements are accompanied by surveys of snow and vegetation cover.
Grazed plots feature a substantially altered surface cover compared to ungrazed plots, with grazing leading to lower vegetation height and density, lower snow depths, and no accumulation of surface litter layers. This leads to an intensified transfer of energy between the ground and atmosphere by reducing mechanisms such as canopy shading and insulation by snow and litter layers. Overall, we find a larger seasonal amplitude in GSTs at intensely grazed plots compared to ungrazed plots, with grazed plots being 1.4 to 5.1 °C warmer in summer and −2.5 to −5.4 °C colder in winter. The difference in GSTs between grazed and ungrazed plots is strongly affected by topographic aspect, with the greatest difference found at the south-facing site. We link this directly, as well as indirectly through vegetation cover, to overall higher solar radiation at this site compared to the north-facing site. However, the observed warming of grazed plots in summer is largely offset by colder wintertime GSTs, and the net effect of grazing on annual GSTs remains site-specific.
Our findings suggest that local ground temperatures can be influenced by livestock management, which can be favourable, for example, for the protection of marginal permafrost. Colder GSTs could be achieved through shielding select areas from grazing in the growing season while allowing or even promoting trampling and grazing in the snow season.
We derive the leaf area index (LAI) from hemispherical photographs taken at the logger locations. The photographs are taken using an Insta360 ×2 (© Arashi Vision Inc.) camera that is placed horizontally below the vegetation at each location. The images thus cover the upward-looking 180° perspective, including all protruding vegetation elements. The processing of the images in order to derive the LAI is done with the software Hemisfer (Schleppi et al., 2007; Thimonier et al., 2010). To distinguish between pixels that are showing the sky or canopy, we apply a threshold to the blue band of each image, which can most easily separate bright and blue sky from dark and green vegetation. Due to differences in lighting conditions between images, the optimal threshold value had to be determined for each image individually. The LAI is subsequently calculated using the methodology by Lang (1987). As our sites are on gentle slopes (Table 2) and the hemispherical photographs typically feature large gaps in the canopy (Fig. 4), we also apply the available corrections for slope (Schleppi et al., 2007) and canopy clumping (Chen and Cihlar, 1995). The resulting LAIs (and threshold values) for all images are presented in Zweigel (2024b).
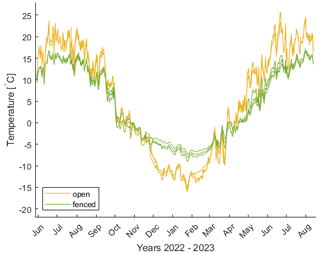
Figure B1As Fig. 11 but showing the GST evolution of the individual loggers in Terelj. Note that two measurements per plot are available from 29 May 2022, while a third measurement was added on 23 July 2022.
Table B1As Table 5 but showing the GSTs of the individual loggers in Terelj. Loggers added on 23 July have insufficient data for summer (JJA) in 2022.
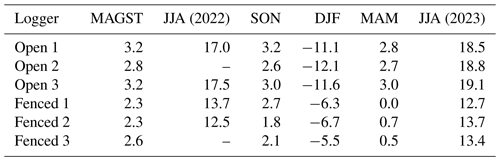
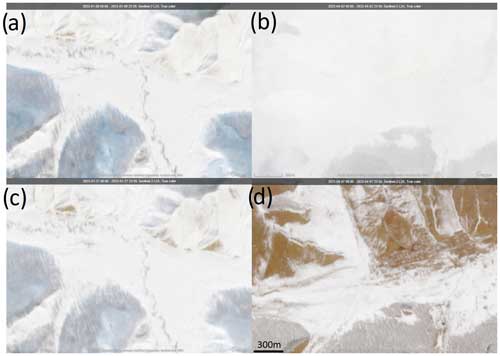
Figure C1Sentinel-2 L2A True colour composite imagery of snow conditions in Udleg on (a) 9 January 2023 and (c) 27 January 2023 and in Terelj on (b) 2 April 2023 and (d) 7 April 2023. The imagery from Udleg shows how snow preferentially ablates from steep south-facing slopes even during the low solar radiation available in mid-winter. The Terelj imagery shows widespread ablation of snow on south-facing slopes in late winter. All imagery has the same scale and was downloaded through the Copernicus Browser (Copernicus Browser: Sentinel-2 L2A True colour imagery).
The ground surface temperature data are available from Zweigel (2024a). The snow and vegetation surveys are available from Zweigel (2024c). The hemispherical photographs and calculated LAIs are available from Zweigel (2024b). The ERA5 reanalysis data used to compute solar radiation are available from Hersbach et al. (2020).
RBZ and SW conceptualized the study. All authors contributed to data curation. RBZ did the formal analysis, investigation, and visualization. AD and SW administrated the project, and HL and SW acquired funding and contributed to supervision. All authors contributed to the writing of the original manuscript.
The contact author has declared that none of the authors has any competing interests.
Publisher’s note: Copernicus Publications remains neutral with regard to jurisdictional claims made in the text, published maps, institutional affiliations, or any other geographical representation in this paper. While Copernicus Publications makes every effort to include appropriate place names, the final responsibility lies with the authors.
We thank Luc Girod for processing drone imagery from the Terelj site.
This research has been supported by Norges Forskningsråd (grant nos. 301639 and 309625) and the European Space Agency (grant no. 4000123681/18/I-NB).
This paper was edited by Paul Stoy and reviewed by two anonymous referees.
Agisoft LLC: Agisoft Metashape Professional (version 2.1.0) [software], retrieved from http://www.agisoft.com/downloads/installer/ (last access: 12 July 2024), 2023.
Angerer, J., Han, G., Fujisaki, I., and Havstad, K.: Climate Change and Ecosystems of Asia With Emphasis on Inner Mongolia and Mongolia, Rangelands, 30, 46–51, https://doi.org/10.2111/1551-501X(2008)30[46:CCAEOA]2.0.CO;2, 2008.
Chen, J. M. and Cihlar, J.: Quantifying the effect of canopy architecture on optical measurements of leaf area index using two gap size analysis methods, IEEE Trans. Geosci. Remote Sens., 33, 777–787, https://doi.org/10.1109/36.387593, 1995.
Dashtseren, A., Ishikawa, M., Iijima, Y., and Jambaljav, Y.: Temperature regimes of the active layer and seasonally frozen ground under a forest-steppe mosaic, Mongolia, Permafr. Periglac. Process., 25, 295–306, https://doi.org/10.1002/ppp.1824, 2014.
Dashtseren, A., Temuujin, K., Westermann, S., Batbold, A., Amarbayasgalan, Y., and Battogtokh, D.: Spatial and Temporal Variations of Freezing and Thawing Indices From 1960 to 2020 in Mongolia, Front. Earth Sci., 9, 1059–1059, 2021.
Dozier, J. and Frew, J.: Rapid calculation of terrain parameters for radiation modeling from digital elevation data, IEEE Trans. Geosci. Remote Sens., 28, 963–969, https://doi.org/10.1109/36.58986, 1990.
ESA and Sinergise: Copernicus Global Digital Elevation Model, https://doi.org/10.5270/ESA-c5d3d65, 2021.
Fernández-Giménez, M. E., Allington, G. R. H., Angerer, J., Reid, R. S., Jamsranjav, C., Ulambayar, T., Hondula, K., Baival, B., Batjav, B., Altanzul, T., and Baasandorj, Y.: Using an integrated social-ecological analysis to detect effects of household herding practices on indicators of rangeland resilience in Mongolia, Environ. Res. Lett., 13, 075010, https://doi.org/10.1088/1748-9326/aacf6f, 2018.
Gan, L., Peng, X., Peth, S., and Horn, R.: Effects of grazing intensity on soil thermal properties and heat flux under Leymus chinensis and Stipa grandis vegetation in Inner Mongolia, China, Soil Till. Res., 118, 147–158, https://doi.org/10.1016/j.still.2011.11.005, 2012.
Hersbach, H., Bell, B., Berrisford, P., Hirahara, S., Horányi, A., Muñoz-Sabater, J., Nicolas, J., Peubey, C., Radu, R., Schepers, D., Simmons, A., Soci, C., Abdalla, S., Abellan, X., Balsamo, G., Bechtold, P., Biavati, G., Bidlot, J., Bonavita, M., Chiara, G., Dahlgren, P., Dee, D., Diamantakis, M., Dragani, R., Flemming, J., Forbes, R., Fuentes, M., Geer, A., Haimberger, L., Healy, S., Hogan, R. J., Hólm, E., Janisková, M., Keeley, S., Laloyaux, P., Lopez, P., Lupu, C., Radnoti, G., Rosnay, P., Rozum, I., Vamborg, F., Villaume, S., and Thépaut, J.-N.: The ERA5 global reanalysis, Q. J. R. Meteorol. Soc., 146, 1999–2049, https://doi.org/10.1002/qj.3803, 2020.
Hou, D., Liu, C., Qiao, X., and Guo, K.: Asymmetric effects of litter accumulation on soil temperature and dominant plant species in fenced grasslands, Ecosphere, 11, e03289, https://doi.org/10.1002/ecs2.3289, 2020.
Iijima, Y., Ishikawa, M., and Jambaljav, Y.: Hydrological cycle in relation to permafrost environment in forest-grassland ecotone in Mongolia, J. Jpn. Assoc. Hydrol. Sci., 42, 119–130, https://doi.org/10.4145/jahs.42.119, 2012.
Ishikawa, M., Sharkhuu, N., Zhang, Y., Kadota, T., and Ohata, T.: Ground thermal and moisture conditions at the southern boundary of discontinuous permafrost, Mongolia, Permafrost Periglac., 16, 209–216, https://doi.org/10.1002/PPP.483, 2005.
Ishikawa, M., Jamvaljav, Y., Dashtseren, A., Sharkhuu, N., Davaa, G., Iijima, Y., Baatarbileg, N., and Yoshikawa, K.: Thermal states, responsiveness and degradation of marginal permafrost in Mongolia, Permafrost Periglac., 29, 271–282, https://doi.org/10.1002/PPP.1990, 2018.
Jaroszynska, F., Althuizen, I., Halbritter, A. H., Klanderud, K., Lee, H., Telford, R. J., and Vandvik, V.: Bryophytes dominate plant regulation of soil microclimate in alpine grasslands, Oikos, 2023, e10091, https://doi.org/10.1111/oik.10091, 2023.
Klinge, M., Schneider, F., Dulamsuren, C., Arndt, K., Bayarsaikhan, U., and Sauer, D.: Interrelations between relief, vegetation, disturbances, and permafrost in the forest-steppe of central Mongolia, Earth Surf. Process. Landf., https://doi.org/10.1002/esp.5116, 2021.
Lang, A. R. G.: Simplified estimate of leaf area index from transmittance of the sun's beam, Agric. Forest Meteorol., 41, 179–186, https://doi.org/10.1016/0168-1923(87)90078-5, 1987.
Liang, M., Smith, N. G., Chen, J., Wu, Y., Guo, Z., Gornish, E. S., and Liang, C.: Shifts in plant composition mediate grazing effects on carbon cycling in grasslands, J. Appl. Ecol., 58, 518–527, https://doi.org/10.1111/1365-2664.13824, 2021.
Lkhagvadorj, D., Hauck, M., Dulamsuren, C., and Tsogtbaatar, J.: Twenty Years After Decollectivization: Mobile Livestock Husbandry and Its Ecological Impact in the Mongolian Forest-Steppe, Hum. Ecol., 41, 725–735, https://doi.org/10.1007/s10745-013-9599-3, 2013.
Lkhavgadorj, H., Iderzorig, B., and Kwon, O.: Changes in plant community structure in relation to climate change and restoration plot areas in Mongolia, J. Ecol. Environ., 39, 119–125, https://doi.org/10.5141/ecoenv.2016.013, 2016.
Maxim Integrated: DS1922L/DS1922T data sheet, https://www.analog.com/media/en/technical-documentation/data-sheets/ds1922l-ds1922t.pdf, (last access: 12 November 2024), 2015.
Maxim Integrated: DS1925 data sheet, https://cdn.shopify.com/s/files/1/0164/3524/files/DS1925-F5_Thermochron_Datasheet_PDF.pdf?v=1724040602, (last access: 12 November 2024), 2020.
McSherry, M. E. and Ritchie, M. E.: Effects of grazing on grassland soil carbon: a global review, Glob. Change Biol., 19, 1347–1357, https://doi.org/10.1111/gcb.12144, 2013.
Miyazaki, S., Ishikawa, M., Baatarbileg, N., Damdinsuren, S., Ariuntuya, N., and Jambaljav, Y.: Interannual and seasonal variations in energy and carbon exchanges over the larch forests on the permafrost in northeastern Mongolia, Spec. Issue Third Int. Symp. Arct. Res. ISAR-3, 8, 166–182, https://doi.org/10.1016/j.polar.2013.12.004, 2014.
Odriozola, I., García-Baquero, G., Laskurain, N. A., and Aldezabal, A.: Livestock grazing modifies the effect of environmental factors on soil temperature and water content in a temperate grassland, Geoderma, 235/236, 347–354, https://doi.org/10.1016/j.geoderma.2014.08.002, 2014.
Poll, C., Marhan, S., Back, F., Niklaus, P. A., and Kandeler, E.: Field-scale manipulation of soil temperature and precipitation change soil CO2 flux in a temperate agricultural ecosystem, Agr. Ecosyst. Environ., 165, 88–97, https://doi.org/10.1016/j.agee.2012.12.012, 2013.
Romanovsky, V. E. and Osterkamp, T. E.: Interannual variations of the thermal regime of the active layer and near-surface permafrost in northern Alaska, Permafrost Periglac., 6, 313–335, https://doi.org/10.1002/ppp.3430060404, 1995.
Saizen, I., Maekawa, A., and Yamamura, N.: Spatial analysis of time-series changes in livestock distribution by detection of local spatial associations in Mongolia, Clim. Change Appl. Geogr.–Place Policy Pract., 30, 639–649, https://doi.org/10.1016/j.apgeog.2010.01.002, 2010.
Schindlbacher, A., Zechmeister-Boltenstern, S., and JANDL, R.: Carbon losses due to soil warming: Do autotrophic and heterotrophic soil respiration respond equally?, Glob. Change Biol., 15, 901–913, https://doi.org/10.1111/j.1365-2486.2008.01757.x, 2009.
Schleppi, P., Conedera, M., Sedivy, I., and Thimonier, A.: Correcting non-linearity and slope effects in the estimation of the leaf area index of forests from hemispherical photographs, Agr. Forest Meteorol., 144, 236–242, https://doi.org/10.1016/j.agrformet.2007.02.004, 2007.
Shao, C., Chen, J., Li, L., Dong, G., Han, J., Abraha, M., and John, R.: Grazing effects on surface energy fluxes in a desert steppe on the Mongolian Plateau, Ecol. Appl., 27, 485–502, https://doi.org/10.1002/eap.1459, 2017.
Smith, M. W. and Riseborough, D. W.: Permafrost monitoring and detection of climate change, Permafrost Periglac., 7, 301–309, https://doi.org/10.1002/(SICI)1099-1530(199610)7:4<301::AID-PPP231>3.0.CO;2-R, 1996.
Thimonier, A., Sedivy, I., and Schleppi, P.: Estimating leaf area index in different types of mature forest stands in Switzerland: a comparison of methods, Eur. J. Forest Res., 129, 543–562, https://doi.org/10.1007/s10342-009-0353-8, 2010.
Unified Land Territory Of Mongolia: https://1212.mn/en/statistic/statcate/573072/table-view/DT_NSO_2400_001V1, last access: 29 September 2024.
Wang, J., Cheng, K., Liu, Q., Zhu, J., Ochir, A., Davaasuren, D., Li, G., Wei, H., Chonokhuu, S., Namsrai, O., and Bat-Erdene, A.: Land cover patterns in Mongolia and their spatiotemporal changes from 1990 to 2010, Arab. J. Geosci., 12, 778, https://doi.org/10.1007/s12517-019-4893-z, 2019.
Wang, J., Wei, H., Cheng, K., Ochir, A., Davaasuren, D., Li, P., Shun Chan, F. K., and Nasanbat, E.: Spatio-Temporal Pattern of Land Degradation from 1990 to 2015 in Mongolia, Resour. Use Ecosyst. Restor. Green Dev., 34, 100497, https://doi.org/10.1016/j.envdev.2020.100497, 2020.
Wang, Q., Okadera, T., Watanabe, M., Wu, T., and Ochirbat, B.: Ground warming and permafrost degradation in various terrestrial ecosystems in northcentral Mongolia, Permafrost Periglac., 33, 406–424, https://doi.org/10.1002/ppp.2161, 2022.
Wang, X., Zi, H., Wang, J., Guo, X., Zhang, Z., Yan, T., Wang, Q., and He, J.-S.: Grazing-induced changes in soil microclimate and aboveground biomass modulate freeze–thaw processes in a Tibetan alpine meadow, Agr. Ecosyst. Environ., 357, 108659, https://doi.org/10.1016/j.agee.2023.108659, 2023.
Yan, Y., Yan, R., Chen, J., Xin, X., Eldridge, D. J., Shao, C., Wang, X., Lv, S., Jin, D., Chen, J., Guo, Z., Chen, B., and Xu, L.: Grazing modulates soil temperature and moisture in a Eurasian steppe, Agr. Forest Meteorol., 262, 157–165, https://doi.org/10.1016/j.agrformet.2018.07.011, 2018.
Yan, Y., Yan, R., Wang, X., Xu, X., Xu, D., Jin, D., Chen, J., and Xin, X.: Grazing affects snow accumulation and subsequent spring soil water by removing vegetation in a temperate grassland, Sci. Total Environ., 697, 134189, https://doi.org/10.1016/j.scitotenv.2019.134189, 2019.
Zamba, B.: Third National Communication Of Mongolia, Ulaanbaatar, Mongolia, https://unfccc.int/documents/66255 (last access: 12 November 2024), 2018.
Zhang, H., Fan, J., Gao, D., Liu, Y., and Du, H.: Effect of Decreasing the Interception of Solar Illuminance by Vegetation on Ground Temperature in Degraded Grasslands, Sustainability, 14, 4488, https://doi.org/10.3390/su14084488, 2022.
Zhang, Y., Suzuki, K., Kadota, T., and Ohata, T.: Sublimation from snow surface in southern mountain taiga of eastern Siberia, J. Geophys. Res.-Atmos., 109, D21103, https://doi.org/10.1029/2003JD003779, 2004.
Zhang, Y., Chen, J. M., and Miller, J. R.: Determining digital hemispherical photograph exposure for leaf area index estimation, Agr. Forest Meteorol., 133, 166–181, https://doi.org/10.1016/j.agrformet.2005.09.009, 2005.
Zhang, Y., Ishikawa, M., Ohata, T., and Oyunbaatar, D.: Sublimation from thin snow cover at the edge of the Eurasian cryosphere in Mongolia, Hydrol. Process., 22, 3564–3575, https://doi.org/10.1002/hyp.6960, 2008.
Zhao, Y., Peth, S., Reszkowska, A., Gan, L., Krümmelbein, J., Peng, X., and Horn, R.: Response of soil moisture and temperature to grazing intensity in a Leymus chinensis steppe, Inner Mongolia, Plant Soil, 340, 89–102, https://doi.org/10.1007/s11104-010-0460-9, 2011.
Zimov, S. A., Zimov, N. S., Tikhonov, A. N., and Chapin, F. S.: Mammoth steppe: a high-productivity phenomenon, Quaternary Sci. Rev., 57, 26–45, https://doi.org/10.1016/j.quascirev.2012.10.005, 2012.
Zweigel, R. B.: Ground surface temperature measurements at grazed and ungrazed plots in Central Mongolia, Zenodo [data set], https://doi.org/10.5281/zenodo.11562067, 2024a.
Zweigel, R. B.: Hemispherical photographs and calculated Leaf Area Index for study sites in Central Mongolia, Zenodo [data set], https://doi.org/10.5281/zenodo.11562206, 2024b.
Zweigel, R. B.: Survey of surface cover at two study sites in Central Mongolia, Zenodo [data set], https://doi.org/10.5281/zenodo.11562218, 2024c.
Zweigel, R. B., Westermann, S., Nitzbon, J., Langer, M., Boike, J., Etzelmüller, B., and Vikhamar Schuler, T.: Simulating Snow Redistribution and its Effect on Ground Surface Temperature at a High-Arctic Site on Svalbard, J. Geophys. Res.-Earth, 126, e2020JF005673-e2020JF005673, https://doi.org/10.1029/2020JF005673, 2021.
Zweigel, R. B., Dashtseren, A., Temuujin, K., Aalstad, K., Webster, C., Stuenzi, S. M., Aas, K. S., Lee, H., and Westermann, S.: Simulating the Thermal Regime and Surface Energy Balance of a Permafrost-Underlain Forest in Mongolia, J. Geophys. Res.-Earth, 129, e2023JF007609, https://doi.org/10.1029/2023JF007609, 2024.
- Abstract
- Introduction
- Study area
- Methods
- Results
- Discussion
- Conclusions
- Appendix A: Calculation of leaf area index
- Appendix B: GST data for individual loggers
- Appendix C: Satellite imagery of snow conditions
- Data availability
- Author contributions
- Competing interests
- Disclaimer
- Acknowledgements
- Financial support
- Review statement
- References
- Abstract
- Introduction
- Study area
- Methods
- Results
- Discussion
- Conclusions
- Appendix A: Calculation of leaf area index
- Appendix B: GST data for individual loggers
- Appendix C: Satellite imagery of snow conditions
- Data availability
- Author contributions
- Competing interests
- Disclaimer
- Acknowledgements
- Financial support
- Review statement
- References