the Creative Commons Attribution 4.0 License.
the Creative Commons Attribution 4.0 License.
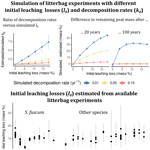
A synthesis of Sphagnum litterbag experiments: initial leaching losses bias decomposition rate estimates
Henning Teickner
Edzer Pebesma
Klaus-Holger Knorr
Our knowledge of the magnitude and controls of Sphagnum decomposition rates is derived to a large extent from litterbag experiments that do not explicitly consider initial leaching losses. Previous research on vascular plants suggests that decomposition rate (k0) estimates from litterbag experiments are biased when initial leaching losses (l0) are ignored. In contrast, the magnitude and variability of l0 for Sphagnum litterbag experiments are not well known, and it is therefore also not known how much Sphagnum k0 estimates are biased. As Sphagnum is the main peat-forming species in many northern peatlands, and biases in k0 estimates can propagate and amplify in long-term peatland models, minimizing such bias is necessary for accurate predictions of peat accumulation.
We present a meta-analysis of 15 Sphagnum litterbag studies to estimate initial leaching losses (l0), to analyze how much Sphagnum k0 estimates are biased when the decomposition model ignores initial leaching losses and to analyze how much the variance in k0 estimates increases due to initial leaching losses even when they are estimated by the decomposition model.
Average l0 estimates range between 3 mass-% to 18 mass-%, and average k0 estimates range between 0.01 to 1.16 yr−1. Simulations and models fitted to empirical data indicate that ignoring initial leaching losses leads to an overestimation of k0. An error analysis suggests that k0 and l0 can be estimated only with relatively large errors because of limitations in the design of most available litterbag experiments. Sampling the first litterbags shortly after the start of the experiments allows more accurate estimation of l0 and k0. We also estimated large l0 (>5 mass-%) for only air-dried samples, which could imply that Sphagnum litterbag experiments with dried litter are unrepresentative for natural decomposition processes in which l0 may be smaller according to leaching experiments with fresh litter.
We conclude that comparing results of litterbag experiments between experimental treatments and between studies and accurately estimating decomposition rates may only be possible if initial leaching losses are explicitly considered.
- Article
(2839 KB) - Full-text XML
-
Supplement
(10981 KB) - BibTeX
- EndNote
Decomposition is one of the major controls of long-term sequestration of carbon in northern peatlands, which are a large global store of carbon sequestered from the atmosphere (Yu, 2012). Our knowledge of the magnitude and controls of decomposition rates is derived to a large extent from litterbag experiments (Rydin et al., 2013), and these estimates are used as parameter values in long-term peatland models (e.g., Frolking et al., 2010). To make correct inferences about decomposition processes and past and future controls of peat accumulation, it therefore has to be validated that the decomposition rate estimates from litterbag experiments are unbiased.
In litterbag experiments, a defined mass of litter or peat is filled into bags which are buried in a peatland or laboratory container and after weeks to years of decomposition are excavated, dried, and reweighed. From the resulting mass trajectories over time, decomposition rates can be estimated with suitable decomposition models (e.g., Frolking et al., 2001; Rovira and Rovira, 2010) and how they depend on environmental conditions. These decomposition rate estimates are used as parameter values in long-term peatland models which allow us to analyze peat accumulation and interactions of decomposition with other processes controlling peat accumulation for time ranges exceeding the duration of litterbag experiments.
A potential problem with current estimates of Sphagnum decomposition rates is that many of them ignore initial leaching losses, which has the potential to bias decomposition rate estimates and therefore peat models. Initial leaching losses are defined here as the export and possible mineralization of water-extractable organic matter from litter within the first period of decomposition, typically observed within the first 2 d to 3 weeks for Sphagnum and peat (Coulson and Butterfield, 1978; Thormann et al., 2001; Moore and Dalva, 2001; Kim et al., 2014; Müller et al., 2023), after which mass loss rates decrease markedly. Ignoring initial leaching losses means estimating a one-pool decomposition rate from litterbag data and taking this to represent depolymerization. Depolymerization is typically slower than initial leaching, and ignoring initial leaching losses can therefore lead to larger decomposition rate estimates, which would overestimate depolymerization on longer timescales relevant to peatland models.
Yu et al. (2001) illustrated this by re-analyzing data from a Rubus chamaemorus litterbag experiment with a one-pool exponential model (ignoring initial leaching losses) and a two-pool exponential model, where the first pool represents initial leaching losses, and concluded that ignoring initial leaching losses causes a non-negligible overestimation of decomposition rates. Similar analyses with comparable outcomes have been performed for non-peatland vegetation (e.g., Bärlocher, 1997) and tea bags (Lind et al., 2022). A systematic analysis for Sphagnum litter, which often has smaller decomposition rates, may have smaller initial leaching losses, and often represents the bulk of peat material, has not been performed yet to our knowledge.
Available estimates from direct measurement and a few litterbag experiments suggest that initial leaching losses from Sphagnum range from <1 % to 18 % of the initial mass (mass-%) (Coulson and Butterfield, 1978; Scheffer et al., 2001; Moore and Dalva, 2001; Thormann et al., 2002; Limpens and Berendse, 2003; Castells et al., 2005; Moore et al., 2007; Del Giudice and Lindo, 2017; Mastný et al., 2018; Müller et al., 2023). Some studies argued that larger leaching losses of 8 % or more estimated by Scheffer et al. (2001) are artifacts from freeze-drying Sphagnum material, which disrupts cell walls (Limpens and Berendse, 2003), and that leaching from Sphagnum generally accounts for only few percent (Johnson and Damman, 1991). This is in line with small leaching losses reported in most of the studies that explicitly quantified initial leaching losses (Coulson and Butterfield, 1978; Moore and Dalva, 2001; Thormann et al., 2002; Limpens and Berendse, 2003; Castells et al., 2005; Mastný et al., 2018). However, larger potential leaching has also been reported or can be estimated for only air- or oven-dried samples, e.g., Moore et al. (2007) (Sect. S1 in the Supplement), Thormann et al. (2001), and Müller et al. (2023). In addition, experiments have shown that air-drying non-Sphagnum litter can increase initial leaching losses relative to undried litter (Gessner and Schwoerbel, 1989; Bärlocher, 1997) and that effects of drying are variable between species (Taylor and Bärlocher, 1996). This indicates that initial leaching losses from Sphagnum can be larger than the few percent assumed by some previous studies, even if the litter was only air-dried. With decomposition rates ranging from <0.01 to around 0.15 yr−1 (e.g., Moore et al., 2007; Turetsky et al., 2008), initial leaching losses in the range from <1 mass-% to 18 mass-% could bias decomposition rate estimates.
Our aims are to quantify the magnitude and variability of initial leaching losses for Sphagnum litterbag experiments, to analyze how much decomposition rate estimates are biased when initial leaching losses are ignored, and to analyze how one could improve the design of litterbag experiments to avoid such biases and more accurately estimate decomposition rates. Specifically, we address the following questions:
-
What is the magnitude of initial leaching losses in Sphagnum litterbag experiments and their variability between species and studies?
-
How does ignoring initial leaching losses bias decomposition rate estimates in Sphagnum litterbag experiments?
-
What conditions may cause small initial leaching losses from Sphagnum litter?
-
How can we design litterbag experiments to improve estimates of decomposition rates?
To address these questions, we first simulate Sphagnum litterbag experiments with initial leaching losses of different magnitude, fit a one-pool exponential decomposition model that ignores initial leaching losses, and analyze how much bias there is in the k0 estimates. Next, we re-analyze litterbag experiments collected from the literature with a one-pool decomposition model that ignores initial leaching losses and a two-pool model that estimates initial leaching losses from the data and compare their results. Finally, we use error and sensitivity analyses to test which litterbag experiment designs allow us to most accurately estimate l0 and k0.
Since our arguments about the importance of initial leaching losses are general and in line with findings for non-Sphagnum litter (e.g., Bärlocher, 1997; Lind et al., 2022), we expect that our study is also relevant for evaluating litterbag experiments of vascular plant and lichen litter in peatlands. Given that decomposition rates in long-term dynamic peatland models are mainly parameterized based on data from litterbag studies (e.g., Frolking et al., 2001; Bauer, 2004; Heijmans et al., 2008; Heinemeyer et al., 2010; Morris et al., 2012; Chaudhary et al., 2018; Bona et al., 2020), our analysis indicates that they should use decomposition rates obtained from litterbag experiments that consider initial leaching losses.
2.1 Modeling leaching losses in litterbag experiments
A general formula for the change in remaining mass with incubation duration t of a litterbag experiment is as follows (Frolking et al., 2001):
where m(t) is the remaining mass at time t after the start of the incubation, k0 is the decomposition rate constant, m0 is the initial mass (m(t=0)), and . α≥0 describes how the decomposition rate changes as mass is lost over time (if α<1, the decomposition rate increases as mass is lost; if α=1, the decomposition rate is constant; if α>1, the decomposition rate decreases as mass is lost).
If α=1, the solution of Eq. (1) is the simple one-pool exponential decomposition model (Frolking et al., 2001):
In this study, we define initial leaching loss as the export of water-extractable organic matter from the litter due to diffusive or advective transport or respiration of soluble compounds within the first period (up to 3 weeks; Coulson and Butterfield, 1978; Thormann et al., 2001; Moore and Dalva, 2001; Kim et al., 2014; Müller et al., 2023) of a litterbag experiment to differentiate it from the subsequent decomposition of polymeric organic matter, which is the dominant process by which mass is lost in the long term. Initial leaching losses can be included in Eq. (2) as the constant parameter l0, which gets subtracted from m0 if t>0:
An alternative would be to define a two-pool exponential decomposition model where one of the pools represents initial leaching losses (e.g., Yu et al., 2001; Rovira and Rovira, 2010; Hagemann and Moroni, 2015). However if the data have no daily resolution, this is equivalent to the previous, simpler approach.
If α>1, the decomposition rate decreases as mass is lost, which is in line with the assumption that litter quality decreases during decomposition. With α>1, Eq. (1) has the following solution (Frolking et al., 2001):
Or, if initial leaching losses are considered as in Eq. (3):
Over longer time periods, α is an important control of remaining masses (Frolking et al., 2001) and is therefore included in the Holocene Peatland Model (Frolking et al., 2010), one of the most widely applied and tested peatland models.
Even though α has little influence on remaining masses during time ranges as covered by litterbag experiments (Frolking et al., 2001, 2010), it needs to be considered for an accurate estimate of l0. Figure 1 illustrates how different values for α, l0, and k0 can produce comparable fits to litterbag data while representing contrasting interpretations of the decomposition process. In the first case, α=2, and a larger l0 and smaller k0 fit the litterbag data; this corresponds to the decomposition process assumed in the Holocene Peatland Model (Frolking et al., 2010). In the second case, a comparable fit is achieved by setting l0=0 mass-% and instead increasing α and k0. In the latter case, the change in mass caused by initial leaching is captured by assuming a very large initial decomposition rate that decreases rapidly. This also implies strongly reduced decomposition rates when extrapolating to longer time ranges and therefore describes a completely different decomposition process than intended in the Holocene Peatland Model. Therefore α needs to be considered in order to obtain estimates for l0 which are consistent with a particular interpretation of the decomposition process.
In our simulation analysis, we assume α=1 in order to make the results comparable to previous evaluations of litterbag experiments. For the same reason, we also assume α=1 when analyzing how ignoring initial leaching losses biases k0 estimates for available litterbag data. To provide estimates for l0 and k0 in available litterbag experiments that consider some of the uncertainty about α, we estimate it from the litterbag data and constrain it to values near 2 to make sure that the model does not confound the slowdown of depolymerization as described by α in the Holocene Peatland Model with the slowdown of leaching losses after the initial period.
In Sect. S3 in the Supplement, we show that estimating α from the litterbag data while ignoring initial leaching losses causes an even larger bias of k0 estimates than when α is set to 1. In Sect. S8, we show that α cannot be accurately estimated even when combining data from available litterbag experiments and that uncertainty about α has little effect on the accuracy with which we could estimate k0 and l0, as long as α is forced to a value near 2.
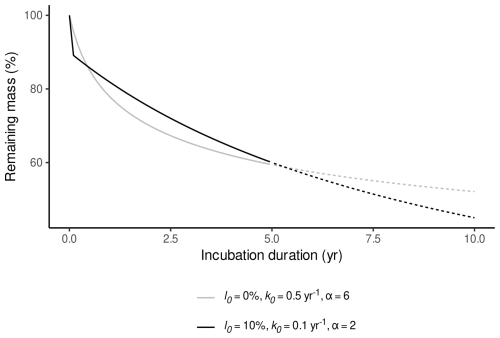
Figure 1Remaining masses during two hypothetical litterbag experiments, where decomposition is controlled by different sets of parameter values for l0, k0, and α. As can be seen, very similar remaining masses can be produced for a typical litterbag experiment (incubation duration ≤5 years) either with an initial leaching loss >0 mass-%, a small k0 and a small α or without initial leaching loss, a large k0, and a large α. Extrapolation to longer incubation durations shows that both models represent different interpretations of the decomposition process (dashed lines).
2.2 Database of Sphagnum litterbag decomposition data
Through a Scopus search with search string
( TITLE-ABS-KEY ( peat* AND ( "litter bag" OR "decomposition rate" OR "decay rate" OR "mass loss" ) ) AND NOT ( "tropic*" ) )
(17 December 2022), we identified studies that analyzed litterbag data in northern peatlands. These studies were further screened to exclude those which do not contain litterbag data or which recycle data from other studies which have already been obtained or which do not use Sphagnum litter (identified down to the species level). Authors of the selected studies not older than 10 years were contacted to obtain raw data. In cases where this was not successful or studies were older than 10 years, relevant data (remaining masses, species identified, mesh sizes, incubation durations, depths where litter was buried, senescence status of collected litter, water table depths) were extracted from the papers where possible. The data are accessible in the Peatland Decomposition Database (Teickner and Knorr, 2024).
In this study, we use data from 15 studies which sampled litterbags at least two time points after the start of the incubation because otherwise k0 and l0 become unidentifiable. The selected studies are Bartsch and Moore (1985), Vitt (1990), Johnson and Damman (1991), Szumigalski and Bayley (1996), Prevost et al. (1997), Scheffer et al. (2001), Thormann et al. (2001), Asada and Warner (2005), Trinder et al. (2008), Breeuwer et al. (2008), Straková et al. (2010), Hagemann and Moroni (2015), Bengtsson et al. (2017), Golovatskaya and Nikonova (2017), and Mäkilä et al. (2018). Samples originally classified as Sphagnum magellanicum are classified here as Sphagnum magellanicum aggr. (Hassel et al., 2018).
2.3 Simulation to check how initial leaching losses can potentially confound Sphagnum decomposition rate estimates
As a first step, we simulated Sphagnum litterbag data with initial leaching losses of different magnitude and then analyzed how k0 estimates are biased if the data are fitted with a one-pool exponential decomposition model that ignores initial leaching. We used Eq. (3) to simulate litterbag mass–time trajectories over 5 years, assuming m(t0)=1; l0 ranges between 0 mass-% and 18 mass-%; and k0 is either 0.01, 0.05, or 0.15 (the range roughly covered by Sphagnum in litterbag experiments; e.g., Moore et al., 2007; Turetsky et al., 2008). To avoid a perfect fit of the models, we added a small amount of noise to the trajectories. Figure 2 shows the result. We then simulated litterbag retrievals after 0.5 years, 1 year, 2 years, 3 years, and 5 years to simulate a litterbag study with relatively high temporal resolution and long duration. This results in a subset of the litterbag mass–time trajectory which mimics real litterbag data compatible with Eq. (3). This subset of the simulated masses is shown as points in Fig. 2.
These simulated masses were then used to fit the model ignoring initial leaching losses (Eq. 2) using non-linear least-squares regression to estimate the average and standard deviation for k0, as is often done in litterbag experiments. We compared these values to the decomposition rate values that were used to simulate the data. This allowed us to analyze how the bias in decomposition rate estimates differ when initial leaching losses differ and how their errors are influenced by initial leaching losses if they are ignored during data analysis. We also analyzed how the estimated models fit the remaining masses and how predicted remaining masses are biased when the decomposition rate estimates are used for extrapolations to 20 or 100 years, as would be the case if the estimates were directly used in a long-term peatland model and all conditions except the remaining mass were kept constant.
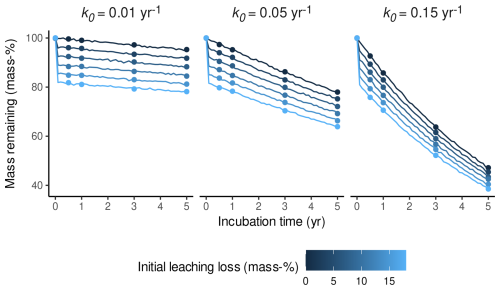
Figure 2Mass trajectories of simulated litterbag experiments over 5 years with three different decomposition rates (yr−1) indicated by panel titles and five initial leaching loss levels (indicated by the color gradient). Lines represent the remaining mass of a litter replicate over time, and points represent the simulated sampling dates for litterbag replicates after 0.5 years, 1 year, 2 years, 3 years, and 5 years.
2.4 Estimating the bias in k0 in available litterbag experiments when initial leaching is ignored
To analyze how k0 estimates for available litterbag data change when we consider or ignore initial leaching losses, we fitted a model that considers initial leaching losses (Eq. 3) and a model that ignores initial leaching losses (Eq. 2) to the synthesized litterbag data. As described in Sect. 2.1, we assume α=1 for this analysis. In Table S1 in the Supplement, we provide a list of all models computed in this study.
We excluded data from Bengtsson et al. (2017), a large laboratory study where litterbags were incubated in water-filled containers and for which the model estimated larger l0 than for any other study, to make sure that our estimates were representative for conditions similar to field conditions (160 out of 289 litterbag experiments were from Bengtsson et al., 2017). Results of the same models including data from Bengtsson et al. (2017) are shown in Sects. S4 and S10, and the average estimates for other studies were not much changed when data from Bengtsson et al. (2017) were excluded or included.
The models assumed a beta distribution for the fraction of initial mass remaining and a gamma distribution for the precision parameter (ϕ) of the beta distribution, which was computed from reported standard deviations (see Sect. S2). Where no standard deviation was reported, ϕ was estimated from the data. Remaining masses larger than 100 mass-% for some experiments were due to the net import of matter during the experiment and were corrected to 100 mass-% in order to make the data compatible with a beta distribution.
We used mixed-effects models (Bayesian hierarchical models) to pool information across relevant groups. Group-level intercepts for k0, l0, and ϕ were estimated for species, study–species combinations, and individual experiments within studies but not for different experimental conditions. For example, l0 for sample (litterbag experiment) i is computed as follows:
where l_2_p1 is the global intercept and l_2_p2[species[i]], l_2_p3[species×study[i]], and l_2_p4[i] are the group-level intercepts for the species, species × study combination, and litterbag experiment (one value per group), respectively. Each of these is assumed to follow a normal distribution, with standard deviation following a half-normal distribution:
where the unknowns are parameters for the prior distributions (see Sect. S2). There are reasonable objections against this choice of hierarchical levels, most importantly because different experimental designs clearly cause systematic differences in decomposition rates, and that these differences should be explicitly considered, and because there is consensus that k0 is smaller for some species (e.g. S. fuscum) than others (S. fallax), and one may wish to incorporate this prior information into the analysis instead of assuming all Sphagnum species to be exchangeable (Gelman et al., 2014).
However, the litterbag experiments are heterogeneous and report heterogeneous information on experimental conditions. Explicitly considering all relevant additional information would therefore require a much more complex model. In addition, where sufficient data are available for individual species, species-specific differences in parameters could be estimated, and, where this is not the case, it seems a reasonable choice to assume exchangeability. Future models may consider additional factors. For example, in a future study, we plan to add to the model another model that describes how decomposition rates change along the gradient from oxic to anoxic conditions.
For k0 and ϕ, we assume the same model structure with appropriate link functions. All intercepts are assumed to have a normal distribution. Further details are described in Sect. S2.
2.5 Estimating l0 and k0 from available litterbag experiments
To estimate k0 and l0 while considering some of the uncertainty about α, we additionally fitted the data with a model that also estimates α from the data (Eq. 3), where we assume the same hierarchical structure as for l0, k0, and ϕ in the previous model. Here, we did not estimate group-level standard deviations for α because it is known that litterbag experiments provide little information about α, as mentioned in Sect. 2.1, and fixing group-level standard deviations avoided potential computational problems.
In Sect. S8, we analyzed how sensitive parameter estimates are to our prior choices. The sensitivity analysis allowed us to explore what biases can be expected for specific true values of k0, l0, and α, and this is a rough estimate of the accuracy and errors of the parameters estimated from available litterbag data under different experimental designs. The results indicate that parameter values (except α) can be estimated accurately with our method when the models are a good approximation of the data-generating process. In particular, our estimates for l0 are conservative.
2.6 Error analysis
Error analysis allows us to estimate the influence that the error of one parameter has on the error of another parameter. Here, we analyze how estimation errors in k0 are related to errors in l0 and how this relation depends on aspects of the litterbag experiment. If k0 estimates have larger errors due to errors in l0, this indicates that we can reduce errors in k0 estimates by measuring l0 more accurately.
We computed the error analysis as suggested in Eriksson et al. (2019). Briefly, this method computes a sensitivity index for some model parameter α in dependency of another model parameter Θi (Si(α)) using the Markov Chain Monte Carlo (MCMC) draws representing the posterior distribution of a model as
where Θ−i is all model parameters except Θi and α, is the expected value of α over all parameters except Θi when Θi is fixed to a specific value, is the variance over the expected values for different values of Θi, and V(α) is the unconditional variance of α. Thus, each sensitivity index Si(α) is the variance of expectations of α if Θi is fixed to different specific values (while other parameters Θ−i are allowed to vary conditional on the fixed value of Θi) divided by the variance of α. Larger values of Si(α) indicate that α is more sensitive to Θi.
We are interested in the sensitivity of the decomposition rates for each replicate litterbag (k0) conditional on initial leaching losses for each replicate (l0), . If differences in between litterbag experiments are related to an aspect of the experimental design, this may provide information on how to design litterbag experiments to get more accurate estimates for both l0 and k0. We computed for each litterbag experiment with MCMC draws from our model.
Intuitively, it would make sense that initial leaching losses can be estimated more accurately if the first litterbag retrieval in a litterbag experiment occurs shortly after the start of the incubation, and, in these cases, we would also expect a small because the model already has enough information to separate initial leaching losses and decomposition rates. To test this hypothesis, we computed linear regression models between and the duration until the first time a litterbag was retrieved in a litterbag experiment conditional on l0 and k0.
2.7 Bayesian data analysis
Bayesian data analysis was used to compute all models to account for relevant error sources and include relevant prior knowledge (for example, that Sphagnum decomposition rates are unlikely to be larger than 0.5 yr−1). Bayesian computations were performed using Markov Chain Monte Carlo (MCMC) sampling with Stan (2.32.2) (Stan Development Team, 2021a) and rstan (2.32.5) (Stan Development Team, 2021b) using the NUTS sampler (Hoffman and Gelman, 2014), with four chains, 4000 total iterations per chain, and 2000 warmup iterations per chain. All models used the same priors for the same parameters and prior choices are listed and justified in Table S2. Further information on the Bayesian data analysis is described in Sect. S9.
3.1 Fit of the models to available litterbag data and errors in parameter estimates
Average predicted remaining masses of all models, considering or ignoring initial leaching losses, fitted the data well, but errors were often large, and the models ignoring initial leaching losses did not fit the data as well, unless α was also estimated from the data (Figs. S21 and S7 in the Supplement). Some litterbag experiments fitted badly under either model. These experiments had average reported remaining masses which increased over time, sampling dates with much larger mass losses compared to previous dates than explainable by the models, or the incubation began in autumn and the replicates experienced cold winters that probably delayed mass losses from both leaching and depolymerization (data from Golovatskaya and Nikonova, 2017) (Fig. S22).
Estimated errors for all parameters were comparatively large for initial leaching losses, decomposition rates, and α, with median coefficients of variation of 28 %, 44 %, and 38 %, respectively, indicating that none of the parameters can be estimated very accurately from available litterbag data.
3.2 Magnitude and variation in initial leaching losses and decomposition rates estimated from available litterbag data
Estimates for l0 ranged between 3 mass-% to 18 mass-% (3 mass-% and 33 mass-% when data from Bengtsson et al. (2017) are also included). There was a large posterior probability (>99 %) that l0>5 mass-% for 42 out of 289 litterbag experiments, that l0>10 mass-% for 6 experiments, that l0<5 mass-% for none of the experiments, and that l0<10 mass-% for 16 experiments. The posterior probability was larger than 70 % that l0<5 mass-% for 13 experiments from Bartsch and Moore (1985), Prevost et al. (1997), and Golovatskaya and Nikonova (2017). Overall, the estimates agree well with the range given in the Introduction when data from Bengtsson et al. (2017) are excluded.
The average l0 varied between species and studies (Fig. 3, Table 1). The median within-species variance was 0.3 times as large as the between-species variance (logit scale). Replicates from Bengtsson et al. (2017) had the largest leaching losses across species, which appears to be a result of the laboratory setup (Fig. 3). For species where data from several studies were available, the variation in l0 was relatively large. For example, for Sphagnum fuscum, average l0 estimates ranged from 3 mass-% to 18 mass-% (3 mass-% to 19 mass-% with data from Bengtsson et al. (2017)), with the largest data values from Thormann et al. (2001), the study already mentioned in the Introduction as support for the existence of large initial leaching losses, and from Asada and Warner (2005). This is similar to the range of initial leaching losses estimated across all species. Small average initial leaching losses (<5 %) were estimated for Sphagnum spec., either peat from 10 to 30 cm depth (Prevost et al., 1997) or hollow and hummock Sphagna from the surface (Bartsch and Moore, 1985); for S. lindbergii (also from Bartsch and Moore, 1985); for S. fuscum replicates (incubated in central Sweden (Breeuwer et al., 2008) or in Western Siberia (Golovatskaya and Nikonova, 2017)); and for S. auriculatum (Trinder et al., 2008). A large average l0 (often > 10 mass-%) was estimated for S. angustifolium, S. balticum, S. fallax, and S. russowii.
Average decomposition rates are in the range of 0.01 to 2.09 yr−1 (0.01 to 1.16 yr−1 without data from Bengtsson et al., 2017). As for initial leaching losses, Fig. 3b indicates some differences between species and studies, and the median within-species variance was 0.9 times as large as the between-species variance (log scale). Decomposition rates were particularly small and consistent for Sphagnum fuscum (range: 0.01 to 0.06 yr−1) and were also small for peat samples from 10 or 20 cm depth (Prevost et al., 1997) and unidentified lawn and hummock mosses (Bartsch and Moore, 1985) (Fig. 3b). Replicates for which the model estimated larger initial leaching losses also had on average larger estimated decomposition rates (Sect. S10).
Estimates for α, the parameter controlling how fast the decomposition rate decreases over time, were variable, but average values did not differ much between species or studies and were similar to the prior average of ∼ 2 (the posterior average α is 2.56 (2.04, 3.1), 95 % confidence interval), indicating that available litterbag data do not provide much information against or in favor of a decrease in decomposition rate with progressing decomposition if initial leaching losses are considered. An exception is the S. russowii and S. capillifolium litters from Hagemann and Moroni (2015) for which we estimated a larger α, though with large errors (9.34 (5.03, 12.15)).
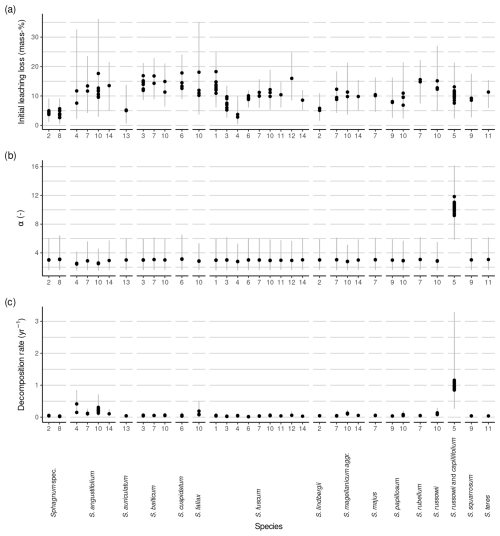
Figure 3Estimated initial leaching losses (a), the parameter controlling a decrease in decomposition rates over time (α) (b), and decomposition rates (c) grouped by species and study. Points represent averages, and error bars represent 95 % confidence intervals. The study is indicated by numbers on the x axis: (1) Asada and Warner (2005), (2) Bartsch and Moore (1985), (3) Breeuwer et al. (2008), (4) Golovatskaya and Nikonova (2017), (5) Hagemann and Moroni (2015), (6) Johnson and Damman (1991), (7) Mäkilä et al. (2018), (8) Prevost et al. (1997), (9) Scheffer et al. (2001), (10) Straková et al. (2010), (11) Szumigalski and Bayley (1996), (12) Thormann et al. (2001), (13) Trinder et al. (2008), and (14) Vitt (1990). Sphagnum spec. are samples that have been identified only to the genus level.
Table 1Averages and 95 % confidence intervals for initial leaching losses (l0), decomposition rates (k0), and rates at which decomposition rates decrease with increasing mass loss (α) of Sphagnum species for available litterbag studies (without data from Bengtsson et al., 2017).
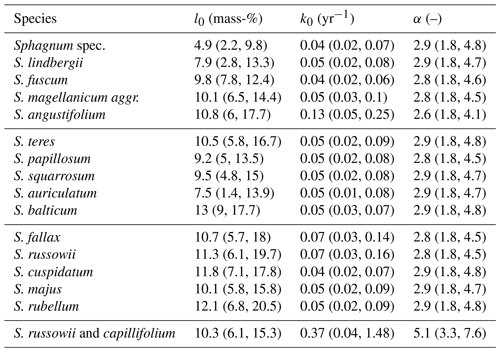
3.3 Ignoring initial leaching losses results in larger estimated decomposition rates
Decomposition rates were overestimated in our simulation when initial leaching losses were ignored. The larger the simulated initial leaching losses were, the larger the bias became (Fig. 4a). This indicates that, if there actually are initial leaching losses as described by Eq. (3) but these are not considered, Sphagnum k0 will be overestimated in proportion to the actual initial leaching losses.
This overestimation did result in misfits to the data within the 5-year period which are similar to misfits of models fitted to real data (Fig. 4c). The minimum difference between the simulated and estimated remaining mass is −15 %, and the maximum difference is 9 %, which is compatible with the median error in remaining masses of replicates in our synthesized litterbag data, 3.2 mass-%. However, the overestimation of k0 when ignoring initial leaching losses becomes important when extrapolating from the typical duration of litterbag studies to longer time ranges. For example, extrapolating the models to 20 or 100 years generally increases the difference between simulated and estimated remaining masses, as shown in Fig. 4b. After 100 years with k0=0.01 yr−1, the models that account for initial leaching losses will yield about 30 % more peat stock than those that do not consider initial leaching losses because of the overestimated decomposition rate. For litter with k0=0.15 yr−1, even large l0 values cause only a small bias because overall mass loss is dominated by decomposition. However, for example, for litter with k0<0.05 yr−1, predicted average masses would be 5 % to 30 % smaller than if initial leaching losses had been considered. The differences are therefore not any more negligible for predictions of peatland models, particularly for peat decomposing at smaller rates and – if k0<0.05 yr−1 – even if l0<5 %.
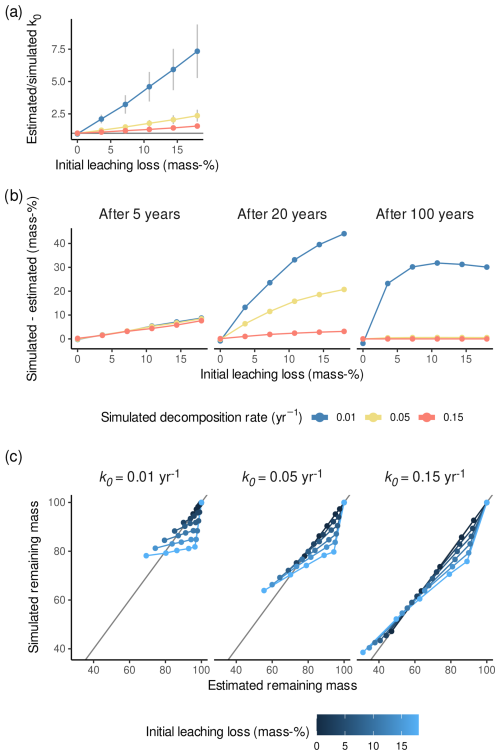
Figure 4Results of the simulation experiment. (a) Estimated divided by simulated decomposition rates (k0) versus simulated initial leaching losses (l0) for the three simulated decomposition rates. Error bars are standard errors. The horizontal gray line represents a ratio of estimated to simulated decomposition rates of 1. (b) Simulated remaining masses (considering different amounts of initial leaching losses) minus remaining masses predicted by the model ignoring initial leaching losses, either after 5, 20, or 100 years of decomposition. Positive values mean that remaining masses are underestimated with k0 estimated while ignoring initial leaching losses. (c) Simulated remaining masses versus remaining masses predicted by the model ignoring initial leaching losses for the three simulated decomposition rates and the simulated litterbag retrieval times.
The analysis of the synthesized litterbag data reproduces both patterns we have observed in the simulation. Firstly, the average k0 as estimated by the model ignoring initial leaching losses increased with increasing l0 (as estimated by the model considering initial leaching losses) (Fig. 5a). On average, k0 estimates were 1.4- to 9.5-fold larger when initial leaching losses are ignored compared to when initial leaching losses are considered (1.2- to 9.5-fold larger with data from Bengtsson et al., 2017). Secondly, the standard deviation of k0 increased with increasing l0, even though this is also the case for some species for the model that considered initial leaching (Fig. 5b).
Overall, both our simulation and our analysis of available litterbag data suggest that k0 will be overestimated and have larger errors when initial leaching losses are ignored.
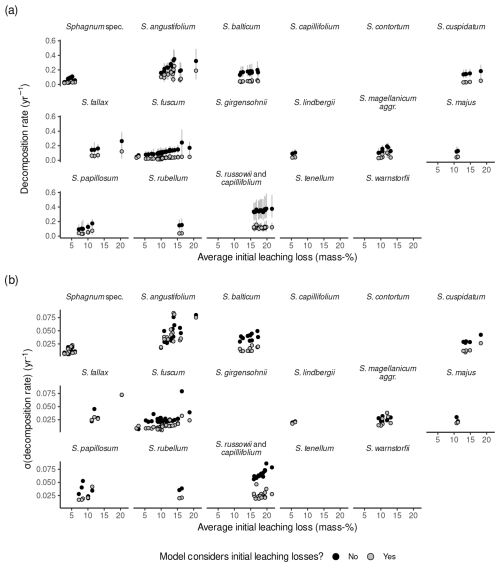
Figure 5(a) Decomposition rate estimates, either considering leaching (gray) or ignoring leaching (black) versus average initial leaching losses estimated by the model considering initial leaching losses. Points are average estimates, and error bars are 95 % prediction intervals. (b) Standard deviation of decomposition rate estimates, either considering leaching (gray) or ignoring leaching (black) versus average initial leaching losses estimated by the model considering initial leaching losses. Both plots show values for species with at least five estimates and exclude data from Bengtsson et al. (2017).
3.4 Sensitivity of k0 and l0 to the design of litterbag experiments
For litterbag experiments with small estimated l0, k0 was less sensitive to l0 if the first litterbags were collected shortly after the start of the litterbag experiment, as expected. In contrast, for litterbag experiments with larger estimated l0, this relation was less pronounced or apparently absent (Fig. 6b). Because average initial leaching losses and decomposition rates are positively related (Pearson correlation coefficients and 95 % confidence interval: 0.16 (0.06, 0.26)), a similar relation can be observed if the data are grouped by the estimated k0 (Fig. 6c); i.e. for litterbag experiments with small estimated decomposition rates, the sensitivity indices were smaller if the first litterbags were collected shortly after the start of the litterbag experiment, and the pattern is less pronounced for larger decomposition rates.
A rough approximation based on Fig. 6b indicates that the average sensitivity of decomposition rates to initial leaching losses can be halved if the first litterbags are collected 20 d after the start of the incubation instead of after 1 year, if initial leaching losses are smaller than approximately 9 % and decomposition rates are smaller than approximately 0.07 yr−1.
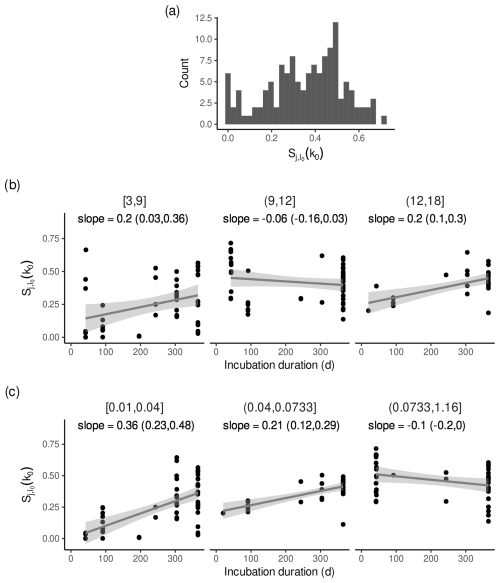
Figure 6Sensitivity indices for decomposition rates conditional on initial leaching losses with all data except from Bengtsson et al. (2017). (a) Histogram of the sensitivity indices. (b) Sensitivity indices versus the duration after the start of the litterbag experiment after which the first litterbags were retrieved for three groups of initial leaching losses. Panel titles are initial leaching losses in mass-%. (c) Same as panel (b) but for three groups of decomposition rates. Panel titles are decomposition rates in yr−1. In panels (b) and (c), the line is a regression line fitted to the data and the shaded area is the 95 % confidence interval. “slope” is the slope of the regression line given as the average with the lower and upper limit of the 95 % confidence interval (yr−1).
We have estimated initial leaching losses and decomposition rates of Sphagnum from available litterbag data, and results indicate that initial leaching losses are not small in general and are large enough to bias predictions of peat accumulation rates over longer time periods. Our sensitivity analysis indicates that our estimates for l0 are conservative for available litterbag data, and the risk that we have overestimated them is low (Sect. S8). We can thus build on our estimates to discuss the following three points.
Firstly, we discuss which factors may have caused small initial leaching losses in previous studies and in litterbag experiments where we estimated small initial leaching losses. If we can identify factors that cause small initial leaching losses, we may in turn explain under what conditions there are larger initial leaching losses. It would also allow us to assess whether initial leaching losses estimated from litterbag experiments are representative for those under natural conditions. Next, we discuss the consequences of ignoring initial leaching losses for decomposition rate estimates but also for studies that do not estimate decomposition rates and instead simply interpret mass loss differences between experimental groups as decomposition. Finally, we make suggestions on how to design litterbag experiments to improve estimates of k0 and l0.
4.1 Possible causes of variations in initial leaching losses between studies
We suggest that small initial leaching losses (<5 mass-%) in many of the studies that found small initial leaching losses can be explained by four factors, of which the first three (litter has already been pre-leached, with mild drying and little water volume or water movement) indeed cause small initial leaching losses and the fourth (underestimated mass losses due to influx of external matter during the incubation) is a measurement artifact.
The following studies quantified or reported small initial leaching losses (< 5 mass-%): Coulson and Butterfield (1978), Thormann et al. (2002), and Castells et al. (2005) directly quantified or reported small initial leaching losses without litterbag experiments. In Moore et al. (2007), some litterbag samples, but not all, have small estimated l0 (Sect. S1). Our synthesis adds to these small l0 estimates for replicates from Prevost et al. (1997) (peat), from Bartsch and Moore (1985) (hollow and hummock mosses and S. lindbergii), from Breeuwer et al. (2008) (S. fuscum from northern Sweden incubated in central Sweden), from Golovatskaya and Nikonova (2017) (S. fuscum incubated in Western Siberia), and from Trinder et al. (2008) (S. auriculatum) (Fig. 3). In the following paragraphs, we suggest causes for small initial leaching losses in these studies.
Litter was pre-leached or already decomposed
Prevost et al. (1997) used peat samples from depths of 10 to 30 cm as litterbag material. This material had probably already experienced decomposition and lost the cytoplasm contents; therefore no large initial leaching losses were observed. Moore and Dalva (2001) quantified larger net DOC losses from fresh oven-dried Sphagnum litter and Sphagnum peat than from more decomposed peat.
Litter was dried only mildly so that Sphagnum plants did not die (completely)
Castells et al. (2005) used fresh Sphagnum plants in their study, where they quantified only small initial leaching losses. Bartsch and Moore (1985) air-dried their samples for only 24 to 48 h, and Schipperges and Rydin (1998) (their Figs. 2 and 3) showed that Sphagna can survive drying for several hours if the water content does not decrease too much. Therefore, the Sphagnum plants may not have been completely dead, which would have reduced initial leaching losses.
The incubation environment was closed, with small volume and little water movement
Thormann et al. (2002) incubated S. fuscum in Petri dishes in the laboratory. We suggest that initial leaching losses were small because leachates could not be exported, there was little water movement, and the volume of the Petri dishes was small. Similarly, Golovatskaya and Nikonova (2017) started their experiment in September, and we assume that the peat was either already partly frozen at this time or that cold temperatures limited leaching (Lind et al., 2022). This is supported by small initial leaching losses and by large and rapid mass losses during spring from S. angustifolium samples incubated in the same study during the same period (see Fig. 2 in Golovatskaya and Nikonova, 2017).
Measurement artifact: not properly subtracting mass influx from remaining masses
Trinder et al. (2008) used oven-dried Sphagnum samples, where we would expect larger initial leaching losses than indicated by our model. However, Trinder et al. (2008) report that there was mass influx from the peat matrix (as supported by recorded remaining masses larger than 100 mass-%) and that they tried to correct this by estimating the amount of peat matrix influx from replicates at the end of the decomposition experiment and assuming a linear influx over time. This procedure does not seem to be robust because many of the corrected remaining masses still are larger than 100 mass-%. Consequently, mass influxes that are not properly subtracted are a plausible explanation for apparently small initial leaching losses (and probably also decomposition rates) in this case.
Possible counterexamples
The four factors can explain small initial leaching losses in many litterbag experiments and studies directly measuring leaching we are aware of, except for one Sphagnum replicate from Breeuwer et al. (2008), some replicates in Moore et al. (2007), and direct leaching loss measurements in Coulson and Butterfield (1978). Both a lack of knowledge about the controls of the initial leaching and a lack of information in the studies make it difficult to explain small initial leaching losses in these studies.
Samples from Breeuwer et al. (2008) were S. fuscum stems that were not yet decomposed and that were oven-dried at 30 °C for 48 h and incubated in Sweden in the field starting in spring, making it unlikely that one of the first three factors is responsible for the small initial leaching losses. Breeuwer et al. (2008) mention no external mass influx into litterbags (except ingrown roots, which were removed), but, for some replicates, the remaining masses increased over time (Fig. 3 in Breeuwer et al., 2008), indicating that measurement artifacts may have played a role here, too.
In Moore et al. (2007), senesced Sphagnum samples were air-dried, but it is not described what properties of the samples indicated senescence or how long they were dried for. Estimated initial leaching losses were larger than 5 mass-% for some samples but particularly small if incubated in a pond, suggesting that the incubation environment may have caused small initial leaching losses in some cases if there were no measurement artifacts.
Coulson and Butterfield (1978) used air- or oven-dried complete shoots of S. recurvum and measured initial leaching losses in the laboratory by placing litter in water-filled containers over 7 d. This study reported leaching losses of 0.0 mass-%, which deviates extremely compared to other studies where initial leaching losses were directly measured, even over much shorter durations (Moore and Dalva, 2001; Castells et al., 2005; Mastný et al., 2018). The samples were collected in spring, and, if contents of water-extractable compounds are smaller in spring (Sytiuk et al., 2023), this may explain small leaching losses but still not zero leaching.
Our suggestions here are incomplete, and there are many potential confounding factors which appear to have received little attention in litterbag experiments. Available litterbag data do not allow us to analyze whether there is a seasonal pattern of initial leaching losses, as can be expected based on studies analyzing contents of water-extractable organic matter (Edwards et al., 2018; Sytiuk et al., 2023), or whether initial leaching losses differ between studies that discard capitula, use whole plants, or use stem parts of different length, as can be expected from previous studies and from the observation that Sphagnum litter that is already senesced or decomposed has smaller initial leaching losses (Moore and Dalva, 2001). Systematic experiments are necessary to test the suggested causes for small initial leaching losses and potential confounding factors.
To summarize, small initial leaching losses estimated in many existing studies appear to be linked to at least four factors (pre-leaching, only mild drying such that the Sphagnum plants do not die, closed incubation environments with small volume and little water movement, and measurement artifacts). Conversely, even only air-drying can cause large (>5 mass-%) initial leaching losses, as has been observed for non-Sphagnum litter (e.g., Bärlocher, 1997). Since many Sphagnum litterbag studies oven-dry or air-dry their samples and such procedures are poorly standardized, this could explain some part of the large inter-study variation in initial leaching losses we observed in available litterbag data.
4.2 Relevance of considering initial leaching losses in litterbag experiments
If initial leaching losses are small only under very specific conditions, as suggested in the previous section, but not in general, our results suggest that ignoring initial leaching losses can bias decomposition rate estimates. We discuss four reasons why Sphagnum litterbag studies should consider initial leaching losses.
Ignoring initial leaching losses leads to overestimated decomposition rates
Firstly, our simulation suggests that ignoring initial leaching losses leads to overestimation of decomposition rates and that this is not negligible even for leaching losses <5 mass-% if the decomposition rates are small and if decomposition is extrapolated to longer durations (e.g. 20 years), as is the case in peatland models. That this risk is real can be inferred from the overview of published leaching losses given in the Introduction and from our analysis of available litterbag data, which indicates that average initial leaching losses range from 3 mass-% to 18 mass-% in past litterbag studies under natural conditions and that leaching losses >5 mass-% may not be uncommon (in laboratory studies (Bengtsson et al., 2017), initial leaching losses can be larger, up to 33 mass-%). Thus, ignoring initial leaching losses can bias decomposition rate estimates.
Ignoring initial leaching losses can bias differences between experimental groups
Secondly, available litterbag data indicate that initial leaching losses differ between studies (Fig. 3a). Some of the differences between different studies can be explained by differences in litter pre-treatment or experimental setup, as described in the previous section. This indicates that results from different litterbag studies cannot be compared directly if the aim is to understand decomposition of the polymer fractions of litter. Moreover, if initial leaching losses differ between two experimental groups within the same experiment – for example, because decomposition of samples under different moisture conditions is compared (Lind et al., 2022) – but all mass loss is interpreted as decomposition, this can bias results within the same study.
Thus, in general, relative differences in decomposition rates or mass losses due to decomposition may not be preserved between different experimental groups in the same study if initial leaching losses are not the same, and this can lead to erroneous conclusions on decomposition in peatlands, even if only the remaining masses are compared.
Better knowledge of initial leaching losses allows us to more accurately estimate decomposition rates
Thirdly, our analysis suggests that errors in all model parameters (l0, k0, and α) are large and that the errors in k0 are sensitive to initial leaching losses (and vice versa). This indicates that a more accurate estimation of initial leaching losses allows us to more accurately estimate decomposition rates. Since small differences in decomposition rates can cause larger differences in accumulated carbon over time (Fig. 4), this increased accuracy is necessary for more accurate long-term predictions of peatland models.
Does Sphagnum litter pre-treatment change decomposition qualitatively and alter microbial colonization patterns?
If Sphagnum mosses leach much less of their initial mass over a longer time range under natural conditions, or if they leach compounds inhibiting or facilitating decomposition at different proportions (e.g. phenolics, sphagnan (Fenner and Freeman, 2011; Hájek et al., 2011; Hájek and Urbanová, 2024), or nutrients), this may change how microbials colonize and decompose litter, possibly making Sphagnum litterbag experiments unrepresentative for decomposition under natural conditions; this has already been discussed for non-Sphagnum litter (Bärlocher, 1997). Future studies should test whether not drying Sphagnum litter decreases initial leaching losses and what consequences this may have on microbial colonization patterns and decomposition rates.
4.3 How can we improve litterbag experiments?
The design of litterbag experiments, and specifically when the first litterbags after the start of the experiment are sampled, is an important contributor to the relatively large errors in k0 and l0 estimated from available litterbag experiments. The error analysis indicates that, when the first litterbag is collected 1 year after the start of the experiment, errors and biases in average k0 estimates are larger if the decomposition rate is larger than approximately 0.05 yr−1 and if there are large initial leaching losses. Similarly, our sensitivity analysis suggests that it is difficult to accurately estimate both l0 and k0 if the first litterbag was collected a longer time after the start of the experiment (Sect. S8). In these cases, the mass loss until collection of the first litterbags may be explained either by a large initial leaching loss or by a larger initial decomposition rate which slows down over time, as mentioned in Sect. 2.1 (Fig. 1).
For available experiments, the first litterbag was collected 1 year after the start of the incubation points in only 52 out of 129 cases. In 22 litterbag experiments, the first litterbags were collected within 60 d after the start of the experiment, and only 1 experiment collected the first litterbags within 20 d after the start of the experiment. This indicates that the design of available litterbag experiments is an important contributor to the errors in k0 and l0 estimates. Experiments where the first litterbags were collected within approximately 20 d after the start of the experiment, or where the true decomposition rate is small, can be expected to provide the most accurate estimates for l0 and k0.
Based on this, we make the following suggestions for the design of litterbag experiments:
-
One batch of litterbags should be collected shortly after the beginning of the experiment (for example, after 2 d or 1 week). The mass loss measured for this batch should be a good estimate of initial leaching losses, whereas subsequent mass losses are mass losses attributable to decomposition (including all subsequent leaching losses). Decomposition rates can be estimated by subtracting out initial leaching losses either statistically (i.e., using a model similar to that used here) or experimentally (by using only remaining mass values recorded after initial leaching has occurred).
-
Environmental conditions which are expected to postpone initial leaching losses (e.g. due to freezing) should be avoided when possible. If this is not possible, extra batches should ideally be sampled directly before and after the initial leaching process took place.
-
Even though we have not explicitly tested the effect of increasing the temporal resolution by more frequently retrieving litterbags during an experiment, we expect that this is another step to estimate k0 and l0 more accurately and which does not require the development of novel methods. In addition, more than two litterbag collection time points are necessary to experimentally subtract out initial leaching losses and correctly estimate decomposition rates as described in point 1. Most of the available Sphagnum litterbag experiments have only two sampling time points at most after the start of the experiment.
Additional information that should be provided to correctly interpret litterbag experiments is the date when litter to use in an experiment was collected in the field (to allow future studies to evaluate possible influences of seasonal variations in concentrations of soluble compounds), whether the litter collected in the field was already dry (e.g. as water content measurements), and how intensely the litter was dried (e.g. drying temperature and residual water content).
Our results indicate that, to develop more specific recommendations and standards for reporting Sphagnum litterbag experiments, further conceptual research with the aim to address the knowledge gaps outlined in the previous two sections is necessary. Specifically, in our opinion, the next important experimental steps are (1) to define sample preprocessing conditions that are considered natural such that the decomposition process measured in litterbag experiments represents the process intended to be measured, (2) to analyze whether and how commonly applied sampling protocols (e.g. due to seasonal variations in water-extractable compounds) and preprocessing steps (in particular, different drying methods) cause different initial leaching losses and potentially different decomposition pathways, and (3) to develop litter preprocessing methods that are similar to natural conditions and at the same time allow the accurate measurement of initial dry masses. Methods that may be helpful here are experiments similar to those conducted by Lind et al. (2022) or described in Bärlocher (1997) and a combination (or replacement) of litterbag experiments with stable isotope labeling and direct measurement of different mass fluxes (e.g., Kammer and Hagedorn, 2011; Cotrufo et al., 2015) to improve measurement accuracy and exclude additional potential confounding factors such as the long-debated influence of meshes on initial leaching losses and litter fragmentation (e.g., Bokhorst and Wardle, 2013).
Also, with regard to refining decomposition rate parameter values in long-term peatland models, more research is necessary, particularly to understand the slowdown of decomposition rates when litter chemistry changes during decomposition. As discussed in previous studies (Frolking et al., 2001; Clymo et al., 1998) and shown here, current litterbag experiments do not allow the estimation of such a slowdown. Therefore, more precise decomposition rate estimates are a necessary but not a sufficient condition for addressing this problem.
Simulations, estimated initial leaching losses from 15 litterbag studies, and an error analysis suggest that decomposition rates are overestimated if initial leaching losses are ignored. With average initial leaching loss magnitudes as reported in previous studies and as estimated here (3 mass-% to 18 mass-%), this implies an underestimation of remaining masses up to several tens of percent during decades of decomposition. Our estimates indicate that initial leaching losses > 5 mass-% are not uncommon and vary as much within species as overall, somewhat contradictory to the results of many previous studies measuring small initial leaching losses from Sphagnum. This may be explained by the pre-treatment of litter (even only air-drying), which may increase initial leaching losses compared to fresh Sphagnum and may cause large intra- and inter-study variation in initial leaching losses for the same species, similar to what has been observed for leaves from trees. We therefore suggest that a correct estimation of mass losses due to decomposition and of decomposition rates in Sphagnum litterbag experiments requires us to explicitly estimate initial leaching losses.
Our analyses also suggest that future Sphagnum litterbag experiments should sample a batch of litterbags a few days to weeks after the start of the experiment because this allows a more accurate estimation of both initial leaching losses and decomposition rates than is possible with currently available data, especially in experiments with small decomposition rates.
Finally, if differences in sampling protocols (e.g. seasonal variations in contents of water-extractable compounds) and drying procedures (even only air-drying) cause different amounts of initial leaching and change relative amounts of leaching of compounds inhibiting or facilitating decomposition, this may make litterbag experiments with large initial leaching losses caused by pre-treatment unrepresentative for decomposition under natural conditions, where our results suggest less initial leaching losses.
The data and code used in this article are available in Teickner et al. (2024; https://doi.org/10.5281/zenodo.14643240). The data used in this study are derived from Teickner and Knorr (2024; https://doi.org/10.5281/zenodo.11276065).
The supplement related to this article is available online at: https://doi.org/10.5194/bg-22-417-2025-supplement.
HT: conceptualization, methodology, software, validation, formal analysis, investigation, data curation, writing (original draft), visualization, and project administration. EP: supervision, funding acquisition, and writing (review and editing). KHK: supervision, funding acquisition, and writing (review and editing).
The contact author has declared that none of the authors has any competing interests.
Publisher's note: Copernicus Publications remains neutral with regard to jurisdictional claims made in the text, published maps, institutional affiliations, or any other geographical representation in this paper. While Copernicus Publications makes every effort to include appropriate place names, the final responsibility lies with the authors.
We thank Cristian Estop-Aragonés for helpful comments that improved an earlier version of this article.
This study was funded by the Deutsche Forschungsgemeinschaft (DFG, German Research Foundation) grant no. KN 929/23-1 to Klaus-Holger Knorr and grant no. PE 1632/18-1 to Edzer Pebesma.
This open-access publication was funded by the University of Münster.
This paper was edited by Frank Hagedorn and reviewed by Lukas Kohl and one anonymous referee.
Asada, T. and Warner, B. G.: Surface Peat Mass and Carbon Balance in a Hypermaritime Peatland, Soil Sci. Soc. Am. J., 69, 549–562, https://doi.org/10.2136/sssaj2005.0549, 2005. a, b, c
Bärlocher, F.: Pitfalls of Traditional Techniques When Studying Decomposition of Vascular Plant Remains in Aquatic Habitats, Limnetica, 13, 1–11, 1997. a, b, c, d, e, f
Bartsch, I. and Moore, T. R.: A Preliminary Investigation of Primary Production and Decomposition in Four Peatlands near Schefferville, Québec, Can. J. Bot., 63, 1241–1248, https://doi.org/10.1139/b85-171, 1985. a, b, c, d, e, f, g, h
Bauer, I. E.: Modelling Effects of Litter Quality and Environment on Peat Accumulation over Different Time-Scales: Peat Accumulation over Different Time-Scales, J. Ecol., 92, 661–674, https://doi.org/10.1111/j.0022-0477.2004.00905.x, 2004. a
Bengtsson, F., Granath, G., and Rydin, H.: Data from: Photosynthesis, Growth, and Decay Traits in Sphagnum – a Multispecies Comparison, Dryad [data set], https://doi.org/10.5061/DRYAD.62054, 2017. a, b, c, d, e, f, g, h, i, j, k, l, m, n, o
Bokhorst, S. and Wardle, D. A.: Microclimate within Litter Bags of Different Mesh Size: Implications for the “Arthropod Effect” on Litter Decomposition, Soil Biol. Biochem., 58, 147–152, https://doi.org/10.1016/j.soilbio.2012.12.001, 2013. a
Bona, K. A., Shaw, C., Thompson, D. K., Hararuk, O., Webster, K., Zhang, G., Voicu, M., and Kurz, W. A.: The Canadian Model for Peatlands (CaMP): A Peatland Carbon Model for National Greenhouse Gas Reporting, Ecol. Model., 431, 109164, https://doi.org/10.1016/j.ecolmodel.2020.109164, 2020. a
Breeuwer, A., Heijmans, M., Robroek, B. J. M., Limpens, J., and Berendse, F.: The Effect of Increased Temperature and Nitrogen Deposition on Decomposition in Bogs, Oikos, 117, 1258–1268, https://doi.org/10.1111/j.0030-1299.2008.16518.x, 2008. a, b, c, d, e, f, g, h
Castells, E., Peñuelas, J., and Valentine, D. W.: Effects of Plant Leachates from Four Boreal Understorey Species on Soil N Mineralization, and White Spruce (Picea Glauca) Germination and Seedling Growth, Ann. Bot., 95, 1247–1252, https://doi.org/10.1093/aob/mci139, 2005. a, b, c, d, e
Chaudhary, N., Miller, P. A., and Smith, B.: Biotic and Abiotic Drivers of Peatland Growth and Microtopography: A Model Demonstration, Ecosystems, 21, 1196–1214, https://doi.org/10.1007/s10021-017-0213-1, 2018. a
Clymo, R. S., Turunen, J., and Tolonen, K.: Carbon Accumulation in Peatland, Oikos, 81, 368–388, https://doi.org/10.2307/3547057, 1998. a
Cotrufo, M. F., Soong, J. L., Horton, A. J., Campbell, E. E., Haddix, M. L., Wall, D. H., and Parton, W. J.: Formation of Soil Organic Matter via Biochemical and Physical Pathways of Litter Mass Loss, Nat. Geosci., 8, 776–779, https://doi.org/10.1038/ngeo2520, 2015. a
Coulson, J. C. and Butterfield, J.: An Investigation of the Biotic Factors Determining the Rates of Plant Decomposition on Blanket Bog, J. Ecol., 66, 631–650, https://doi.org/10.2307/2259155, 1978. a, b, c, d, e, f, g
Del Giudice, R. and Lindo, Z.: Short-Term Leaching Dynamics of Three Peatland Plant Species Reveals How Shifts in Plant Communities May Affect Decomposition Processes, Geoderma, 285, 110–116, https://doi.org/10.1016/j.geoderma.2016.09.028, 2017. a
Edwards, K. R., Kaštovská, E., Borovec, J., Šantrůčková, H., and Picek, T.: Species Effects and Seasonal Trends on Plant Efflux Quantity and Quality in a Spruce Swamp Forest, Plant Soil, 426, 179–196, https://doi.org/10.1007/s11104-018-3610-0, 2018. a
Eriksson, O., Jauhiainen, A., Maad Sasane, S., Kramer, A., Nair, A. G., Sartorius, C., and Hellgren Kotaleski, J.: Uncertainty Quantification, Propagation and Characterization by Bayesian Analysis Combined with Global Sensitivity Analysis Applied to Dynamical Intracellular Pathway Models, Bioinformatics, 35, 284–292, https://doi.org/10.1093/bioinformatics/bty607, 2019. a
Fenner, N. and Freeman, C.: Drought-Induced Carbon Loss in Peatlands, Nat. Geosci., 4, 895–900, https://doi.org/10.1038/ngeo1323, 2011. a
Frolking, S., Roulet, N. T., Moore, T. R., Richard, P. J. H., Lavoie, M., and Muller, S. D.: Modeling Northern Peatland Decomposition and Peat Accumulation, Ecosystems, 4, 479–498, https://doi.org/10.1007/s10021-001-0105-1, 2001. a, b, c, d, e, f, g, h
Frolking, S., Roulet, N. T., Tuittila, E., Bubier, J. L., Quillet, A., Talbot, J., and Richard, P. J. H.: A new model of Holocene peatland net primary production, decomposition, water balance, and peat accumulation, Earth Syst. Dynam., 1, 1–21, https://doi.org/10.5194/esd-1-1-2010, 2010. a, b, c, d
Gelman, A., Carlin, J., Stern, H., Dunson, D., Vehtari, A., and Rubin, D. B.: Bayesian Data Analysis, Chapman & Hall/CRC Texts in Statistical Science, CRC Press, Boca Raton, 3rd edn., ISBN 978-1-4398-4095-5, 2014. a
Gessner, M. O. and Schwoerbel, J.: Leaching Kinetics of Fresh Leaf-Litter with Implications for the Current Concept of Leaf-Processing in Streams, Arch. Hydrobiol., 115, 81–90, 1989. a
Golovatskaya, E. A. and Nikonova, L. G.: The Influence of the Bog Water Level on the Transformation of Sphagnum Mosses in Peat Soils of Oligotrophic Bogs, Eurasian Soil Sci., 50, 580–588, https://doi.org/10.1134/S1064229317030036, 2017. a, b, c, d, e, f, g, h
Hagemann, U. and Moroni, M. T.: Moss and Lichen Decomposition in Old-Growth and Harvested High-Boreal Forests Estimated Using the Litterbag and Minicontainer Methods, Soil Biol. Biochem., 87, 10–24, https://doi.org/10.1016/j.soilbio.2015.04.002, 2015. a, b, c, d
Hájek, T. and Urbanová, Z.: Enzyme Adaptation in Sphagnum Peatlands Questions the Significance of Dissolved Organic Matter in Enzyme Inhibition, Sci. Total Environ., 911, 168685, https://doi.org/10.1016/j.scitotenv.2023.168685, 2024. a
Hájek, T., Ballance, S., Limpens, J., Zijlstra, M., and Verhoeven, J. T. A.: Cell-Wall Polysaccharides Play an Important Role in Decay Resistance of Sphagnum and Actively Depressed Decomposition in Vitro, Biogeochemistry, 103, 45–57, https://doi.org/10.1007/s10533-010-9444-3, 2011. a
Hassel, K., Kyrkjeeide, M. O., Yousefi, N., Prestø, T., Stenøien, H. K., Shaw, J. A., and Flatberg, K. I.: Sphagnum Divinum (Sp. Nov.) and S. Medium Limpr. and Their Relationship to S. Magellanicum Brid., J. Bryol., 40, 197–222, https://doi.org/10.1080/03736687.2018.1474424, 2018. a
Heijmans, M. M., Mauquoy, D., Van Geel, B., and Berendse, F.: Long-term Effects of Climate Change on Vegetation and Carbon Dynamics in Peat Bogs, J. Veg. Sci., 19, 307–320, https://doi.org/10.3170/2008-8-18368, 2008. a
Heinemeyer, A., Croft, S., Garnett, M. H., Gloor, E., Holden, J., Lomas, M. R., and Ineson, P.: The MILLENNIA Peat Cohort Model: Predicting Past, Present and Future Soil Carbon Budgets and Fluxes under Changing Climates in Peatlands, Clim. Res., 45, 207–226, https://doi.org/10.3354/cr00928, 2010. a
Hoffman, M. D. and Gelman, A.: The No-U-turn Sampler: Adaptively Setting Path Lengths in Hamiltonian Monte Carlo, J. Mach. Learn. Res., 15, 1593–1623, 2014. a
Johnson, L. C. and Damman, A. W. H.: Species-Controlled Sphagnum Decay on a South Swedish Raised Bog, Oikos, 61, 234, https://doi.org/10.2307/3545341, 1991. a, b, c
Kammer, A. and Hagedorn, F.: Mineralisation, leaching and stabilisation of δ13C-labelled leaf and twig litter in a beech forest soil, Biogeosciences, 8, 2195–2208, https://doi.org/10.5194/bg-8-2195-2011, 2011. a
Kim, Y., Ullah, S., Moore, T. R., and Roulet, N. T.: Dissolved Organic Carbon and Total Dissolved Nitrogen Production by Boreal Soils and Litter: The Role of Flooding, Oxygen Concentration, and Temperature, Biogeochemistry, 118, 35–48, https://doi.org/10.1007/s10533-013-9903-8, 2014. a, b
Limpens, J. and Berendse, F.: How Litter Quality Affects Mass Loss and N Loss from Decomposing Sphagnum, Oikos, 103, 537–547, https://doi.org/10.1034/j.1600-0706.2003.12707.x, 2003. a, b, c
Lind, L., Harbicht, A., Bergman, E., Edwartz, J., and Eckstein, R. L.: Effects of Initial Leaching for Estimates of Mass Loss and Microbial Decomposition – Call for an Increased Nuance, Ecol. Evol., 12, https://doi.org/10.1002/ece3.9118, 2022. a, b, c, d, e
Mäkilä, M., Säävuori, H., Grundström, A., and Suomi, T.: Sphagnum Decay Patterns and Bog Microtopography in South-Eastern Finland, Mires Peat, 1–12, https://doi.org/10.19189/MaP.2017.OMB.283, 2018. a, b
Mastný, J., Kaštovská, E., Bárta, J., Chroňáková, A., Borovec, J., Šantrůčková, H., Urbanová, Z., Edwards, K., and Picek, T.: Quality of DOC Produced during Litter Decomposition of Peatland Plant Dominants, Soil Biol. Biochem., 121, 221–230, https://doi.org/10.1016/j.soilbio.2018.03.018, 2018. a, b, c
Moore, T. R. and Dalva, M.: Some Controls on the Release of Dissolved Organic Carbon by Plant Rissues and Soils, Soil Sci., 166, 38–47, https://doi.org/10.1097/00010694-200101000-00007, 2001. a, b, c, d, e, f, g
Moore, T. R., Bubier, J. L., and Bledzki, L.: Litter Decomposition in Temperate Peatland Ecosystems: The Effect of Substrate and Site, Ecosystems, 10, 949–963, https://doi.org/10.1007/s10021-007-9064-5, 2007. a, b, c, d, e, f, g
Morris, P. J., Baird, A. J., and Belyea, L. R.: The DigiBog Peatland Development Model 2: Ecohydrological Simulations in 2D, Ecohydrology, 5, 256–268, https://doi.org/10.1002/eco.229, 2012. a
Müller, R., Zahorka, A., Holawe, F., Inselsbacher, E., and Glatzel, S.: Incubation of Ombrotrophic Bog Litter and Mixtures of Sphagnum, Betula and Calluna Results in the Formation of Single Litter-Specific Decomposition Patterns, Geoderma, 440, 116702, https://doi.org/10.1016/j.geoderma.2023.116702, 2023. a, b, c, d
Prevost, M., Belleau, P., and Plamondon, A. P.: Substrate Conditions in a Treed Peatland: Responses to Drainage, Écoscience, 4, 543–554, https://doi.org/10.1080/11956860.1997.11682434, 1997. a, b, c, d, e, f, g
Rovira, P. and Rovira, R.: Fitting Litter Decomposition Datasets to Mathematical Curves: Towards a Generalised Exponential Approach, Geoderma, 155, 329–343, https://doi.org/10.1016/j.geoderma.2009.11.033, 2010. a, b
Rydin, H., Jeglum, J. K., and Bennett, K. D.: The Biology of Peatlands, Biology of Habitats, Oxford University Press, Oxford, 2nd edn., ISBN 978-0-19-960299-5, 2013. a
Scheffer, R. A., Van Logtestijn, R. S. P., and Verhoeven, J. T. A.: Decomposition of Carex and Sphagnum Litter in Two Mesotrophic Fens Differing in Dominant Plant Species, Oikos, 92, 44–54, https://doi.org/10.1034/j.1600-0706.2001.920106.x, 2001. a, b, c, d
Schipperges, B. and Rydin, H.: Response of Photosynthesis of Sphagnum Species from Contrasting Microhabitats to Tissue Water Content and Repeated Desiccation, New Phytol., 140, 677–684, https://doi.org/10.1046/j.1469-8137.1998.00311.x, 1998. a
Stan Development Team: Stan Modeling Language Users Guide and Reference Manual, version 2.32.2, 2021a. a
Stan Development Team: RStan: The R Interface to Stan, version 2.32.5, 2021b. a
Straková, P., Anttila, J., Spetz, P., Kitunen, V., Tapanila, T., and Laiho, R.: Litter Quality and Its Response to Water Level Drawdown in Boreal Peatlands at Plant Species and Community Level, Plant Soil, 335, 501–520, https://doi.org/10.1007/s11104-010-0447-6, 2010. a, b
Sytiuk, A., Hamard, S., Céréghino, R., Dorrepaal, E., Geissel, H., Küttim, M., Lamentowicz, M., Tuittila, E. S., and Jassey, V. E. J.: Linkages between Sphagnum Metabolites and Peatland CO2 Uptake Are Sensitive to Seasonality in Warming Trends, New Phytol., 237, 1164–1178, https://doi.org/10.1111/nph.18601, 2023. a, b
Szumigalski, A. R. and Bayley, S. E.: Decomposition along a Bog to Rich Fen Gradient in Central Alberta, Canada, Can. J. Bot., 74, 573–581, https://doi.org/10.1139/b96-073, 1996. a, b
Taylor, B. R. and Bärlocher, F.: Variable Effects of Air-Drying on Leaching Losses from Tree Leaf Litter, Hydrobiologia, 325, 173–182, https://doi.org/10.1007/BF00014982, 1996. a
Teickner, H. and Knorr, K.-H.: Peatland Decomposition Database (1.0.0), Zenodo [data set], https://doi.org/10.5281/ZENODO.11276065, 2024. a, b, c
Teickner, H., Pebesma, E., and Knorr, K.-H.: Compendium of R Code and Data for “A Synthesis of Sphagnum Litterbag Experiments: Initial Leaching Losses Bias Decomposition Rate Estimates” and “Underestimation of Anaerobic Decomposition Rates in Sphagnum Litterbag Experiments by the Holocene Peatland Model Depends on Initial Leaching Losses”, Zenodo [code, data set], https://doi.org/10.5281/zenodo.14643240, 2024. a, b
Thormann, M. N., Bayley, S. E., and Currah, R. S.: Comparison of Decomposition of Belowground and Aboveground Plant Litters in Peatlands of Boreal Alberta, Canada, Can. J. Bot., 79, 9–22, https://doi.org/10.1139/b00-138, 2001. a, b, c, d, e, f
Thormann, M. N., Currah, R. S., and Bayley, S. E.: The Relative Ability of Fungi from Sphagnum Fuscum to Decompose Selected Carbon Substrates, Can. J. Microbiol., 48, 204–211, https://doi.org/10.1139/w02-010, 2002. a, b, c, d
Trinder, C. J., Johnson, D., and Artz, R. R.: Interactions among Fungal Community Structure, Litter Decomposition and Depth of Water Table in a Cutover Peatland, FEMS Microbiol. Ecol., 64, 433–448, https://doi.org/10.1111/j.1574-6941.2008.00487.x, 2008. a, b, c, d, e, f
Turetsky, M. R., Crow, S. E., Evans, R. J., Vitt, D. H., and Wieder, R. K.: Trade-Offs in Resource Allocation among Moss Species Control Decomposition in Boreal Peatlands, J. Ecol., 96, 1297–1305, https://doi.org/10.1111/j.1365-2745.2008.01438.x, 2008. a, b
Vitt, D. H.: Growth and Production Dynamics of Boreal Mosses over Climatic, Chemical and Topographic Gradients, Bot. J. Linn. Soc., 104, 35–59, https://doi.org/10.1111/j.1095-8339.1990.tb02210.x, 1990. a, b
Yu, Z., Turetsky, M., Campbell, I., and Vitt, D.: Modelling Long-Term Peatland Dynamics. II. Processes and Rates as Inferred from Litter and Peat-Core Data, Ecol. Model., 145, 159–173, https://doi.org/10.1016/S0304-3800(01)00387-8, 2001. a, b
Yu, Z. C.: Northern peatland carbon stocks and dynamics: a review, Biogeosciences, 9, 4071–4085, https://doi.org/10.5194/bg-9-4071-2012, 2012. a