the Creative Commons Attribution 3.0 License.
the Creative Commons Attribution 3.0 License.
Preliminary evaluation of the potential of tree-ring cellulose content as a novel supplementary proxy in dendroclimatology
Malin M. Ziehmer
Kurt Nicolussi
Christian Schlüchter
Markus Leuenberger
Cellulose content (CC (%)) in tree rings is usually utilised as a tool to control the quality of the α-cellulose extraction from tree rings in the preparation of stable-isotope analysis in wooden tissues. Reported amounts of CC (%) are often limited to mean values per tree. For the first time, CC (%) series from two high-Alpine species, Larix decidua Mill. (European Larch, LADE) and Pinus cembra L. (Swiss stone pine, PICE) are investigated in modern wood samples and Holocene wood remains from the Early and mid-Holocene. Modern CC (%) series reveal a species-specific low-frequency trend independent of their sampling site over the past 150 years. Climate–cellulose relationships illustrate the ability of CC (%) to record temperature in both species but for slightly different periods within the growing season.
The Holocene CC (%) series illustrate diverging low-frequency trends in both species, independent of sampling site characteristics (latitude, longitude and elevation). Moreover, potential age trends are not apparent in the two coniferous species. The arithmetic mean of CC (%) series in the Early and mid-Holocene indicate low CC (%) succeeding cold events. In conclusion, CC (%) in tree rings show high potential to be established as novel supplementary proxy in dendroclimatology.
- Article
(3078 KB) - Full-text XML
-
Supplement
(1169 KB) - BibTeX
- EndNote
Highly resolved proxy records from the tree-ring archive contribute significantly to reconstructing and understanding past climate variability on versatile temporal and spatial scales (Büntgen et al., 2011; Esper et al., 2002; Mann et al., 1998). The annually resolved and calendar-dated tree-ring records are particularly useful in disentangling natural climate variability from the anthropogenic imprint on the global climate and its impact on the climate system, which enables the improvement of models in view of projecting future climate change (Keel et al., 2016; Keller et al., 2017).
Most dendroclimatological reconstructions are based on tree-ring width (TRW) and maximum latewood density (MXD) records (Büntgen et al., 2006; Ljungqvist et al., 2016; Trouet et al., 2009). However, the determination of stable-isotope ratios of carbon (δ13C), oxygen (δ18O) and hydrogen (δD) isotopes in tree components and their ability to reconstruct past climate has gradually opened up a new field of dendroclimatology over the past four decades (Frank et al., 2015; Leuenberger, 1998; McCarroll and Loader, 2004; Treydte et al., 2006). In addition, the potential of stable-isotope records in tree rings has been increased by improvements in both sample preparation and measurement techniques (Boettger et al., 2007; Filot et al., 2006; Laumer et al., 2009; Loader et al., 2015). Even though the isotopic composition has been determined in various components of a tree, e.g. bulk wood samples, leaves, methoxyl groups of lignin and leaf waxes (Anhäuser et al., 2017; Borella et al., 1999; Kahmen et al., 2011; Kimak et al., 2015), the most preferred component is α-cellulose extracted from tree rings (Borella et al., 1998, 1999; Loader et al., 2003, 2013; McCarroll and Loader, 2004; Treydte et al., 2007). A major advantage of using α-cellulose in isotope research is the extraction of one single chemical component of a tree ring. Thereby it allows researchers to circumvent the biases that potentially result from a changing composition (i.e. in the cellulose ∕ lignin ratio) when analysing bulk wood since different components exhibit significantly different isotope compositions (Borella et al., 1998, 1999).
The α-cellulose is the dominant component in tree rings and its extraction follows a standardised procedure (Boettger et al., 2007). The woody annual growth layers of trees, i.e. the tree rings, are composed of lignin (phenolic polymer), hemicellulose (heterogenous polysaccharides) and cellulose (β-1,4 glucan) in a ratio of (Freudenberg, 1965; Hu et al., 1999). Thereof, the part of cellulosic material which is insoluble in a 17 % sodium hydroxide (NaOH) solution is defined as α-cellulose (Burton and Rasch, 1931; Cross and Bevan, 1912). The various tree-ring components are known to differ in their isotopic composition, whereas α-cellulose is the most stable component, as the polymer is of long-term stability (Boettger et al., 2007; Leavitt and Danzer, 1993; McCarroll and Loader, 2004).
The quantity of the α-cellulose content (hereinafter referred to as CC (%)), generally calculated from the dry weight and the α-cellulose weight of a wood sample, is usually determined and used as a tool to test the quality of the cellulose extraction and the purity of the cellulose (Burton and Rasch, 1931).
However, variations in the amount of α-cellulose and the determined CC (%) (also cellulose yield; used rather simultaneously in the literature) are scarcely mentioned in dendroclimatological literature. Merely a few publications provide numbers on the mean extracted CC (%) (Cullen and Macfarlane, 2005; Gaudinski et al., 2005; Leavitt and Danzer, 1993; Loader et al., 1997). While reported results on the CC (%) are scarce in the literature, the importance of the CC (%) calculation as a tool for the determination of degradation state in subfossil wood is addressed within several studies (Brenninkmeijer, 1983; Loader et al., 2003 and references therein; Schleser et al., 1999).
Schleser et al. (1999) point out that the determination of isotopic ratios in cellulose from woody plant tissues often assumes constancy in the CC (%), which may hold true for modern samples from living trees. However, samples from subfossil or fossil wood have presumably experienced degradation processes with potential influence on the CC (%). The artificial degradation of samples revealed a discrimination of δ13C values up to 0.3 ‰ with decreasing CC (%) where the change in degradation behaviour over time splits into initially increased degradation of cellulose with a strong depletion in δ13C, followed by an inverse effect comprising an enrichment in δ13C with advancing degradation (Schleser et al., 1999).
Loader et al. (2003) discusses the use of whole wood versus α-cellulose in subfossil tree samples as the various wood components (lignin, hemicelluloses, cellulose) experience variable degrees of decomposition, changing the ratio of the individual components within a tree ring. The polysaccharide components, i.e. cellulose and hemicelluloses, decompose more rapidly than lignin, which leads to an altered ratio of cellulose and lignin in whole wood samples, which in turn modifies the isotopic composition of whole wood samples (Borella et al., 1998; Loader et al., 2003; Schleser et al., 1999). Therefore, cellulose is the preferred wood component in subfossil wood studies; however, the effect of partial decay of cellulose on the isotopic signature of tree rings and its implications for paleoclimate reconstructions is insufficiently investigated, although results from naturally degraded subfossil wood show no evidence for a change in the isotopic composition of cellulose due to degradation (Loader et al., 2003).
While studies on CC (%) and its implication for dendroclimatological studies are scarce, even less is known about the influence of environmental conditions on the annual variability of the CC (%) (Genet et al., 2011). For instance, Gindl et al. (2000) discussed lignin content as a temperature proxy for the late growing season (September–October). As lignin and cellulose are among the major components of a tree ring, and changes in one component affect the cellulose∕lignin ratio, a link between CC (%) and temperature can be expected as well. However, the study of Gindl et al. (2000) covers only a short time period of 10 years and the method used is rather time consuming, whereas the production of CC (%) is often a by-product during the extraction of α-cellulose for stable-isotope analysis in tree rings. A study on CC (%) in roots revealed influences of topography and soil moisture status on root CC (%) (Hales et al., 2009). However, variations in CC (%) over time may also be related to tree metabolism and the availability in non-structural carbon (NSC), which occurs in the form of starch and sugars. The sugars provide the nutrients for cell wall components such as cellulose (Haigler et al., 2001; Hoch et al., 2002). Studies on NSC concentrations at the Alpine treeline provide evidence that trees growing in the treeline ecotone are not depleted in carbon; on the contrary, NSC concentrations are found to increase with elevation, implying that the limitation of tree growth at the upper treeline does not result from insufficient nutrition but rather thermal conditions, i.e. temperature, which limits the sink activity and thereby growth (Hoch et al., 2002; Hoch and Körner, 2003, 2009, 2012; Körner, 2003). Applied to the CC (%) of a tree ring, it can be hypothesised that temperature acts as the main control, as it steers the sink activity, i.e. tissue formation.
This paper presents the results of a pilot study in which time series of modern and subfossil tree-ring cellulose are established, allowing a novel insight into a tree-ring component which is commonly used in dendroclimatology but hardly examined itself in its variability over time and in the factors driving its variability.
The current study is embedded in the project Alpine Holocene Tree Ring Isotope Records (AHTRIR), which aims to reconstruct Holocene climate variability using a multi-proxy approach for the past 9000 years. Therefore, tree-ring material consists only partly of living wood material; by far the largest part is based on findings of Holocene wood remains from glacier forefields, peat bogs and small lakes in the central European Alps. Initially, the determination of CC (%) was used to determine the quality of the cellulose extraction before performing an analysis of triple isotopes (δ2H, δ18O, δ13C) on the tree-ring cellulose. However, it offers a unique opportunity to investigate the CC (%) and its variations in long-living trees from two high-Alpine coniferous tree species (Larix decidua Mill. and Pinus cembra L.) in Holocene wood remains found in glacier forefields and peat bogs in the European Alps as well as in modern trees sampled at the current Alpine tree line. The case study aims to (i) identify common trends in time series of CC (%) in tree rings from Early and mid-Holocene subfossil and modern wood material; (ii) investigate dependencies of CC (%) in relation to their sampling site (latitude, longitude, elevation), their age and the species; (iii) analyse climate–CC (%) relationships and finally (iv) determine the ability to utilise CC (%) as a supplementary proxy in dendroclimatological reconstructions.
2.1 Samples and sampling sites
Holocene wood remains and modern tree-ring material are sampled with the intent to reconstruct Holocene climate variability in a multi-proxy approach. Thereby, samples from two Alpine treeline species, namely the Swiss stone pine (Pinus cembra L., PICE) and the European larch (Larix decidua Mill., LADE) are collected as they represent the typical treeline species present at modern sampling sites in the form of living trees, and in glacier forefields and peat bogs as subfossil wood remains (Joerin et al., 2008; Nicolussi, et al., 2009; Nicolussi and Patzelt, 2000a). The sampling sites are located along a SW–NE transect in the central European Alps (46∘02–47∘03′ N, 7–12∘15′ E; Fig. 1, Tables 1 and 2). The modern tree-ring material was cored at three upper treeline sites close to the glacier forefield sites during fieldwork in 2015 (Fig. 2, Table 1). At each site, four trees per species were cored; however, at the sites UAZR and FPCR, only one of the two species is present. Cores are taken at breast height from three radial sections, two in parallel to the slope and one up-slope, thereby avoiding any kind of compression wood on the down-slope side of the tree.
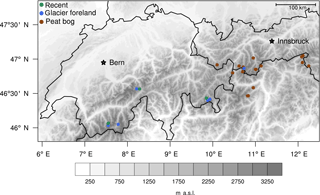
Figure 1Sampling sites of Holocene wood remains and living tree individuals. The sites are located along a SW–NE transect along the central European Alps and contain glacier forefields (blue), peat bogs and small lakes (maroon), where Holocene wood remains are collected, and modern sites, where living trees were cored (green). The modern sites are situated in the proximity of glacier forefield sites, thereby resembling similar growth conditions and serving as a connection between Holocene and present-day tree growth and environmental conditions (source: M. Messmer).
Table 1Site characteristics of modern sites. Coordinates, aspect and elevation describe their geographical distribution from west to east. Four trees are sampled per site and species, resulting in eight trees cored at VRR, where both species are present at the treeline. Site characteristics are complemented by the maximum growth period and the period covered by a sample replication of four trees in years AD as well as the mean segment length (MSL) in years.
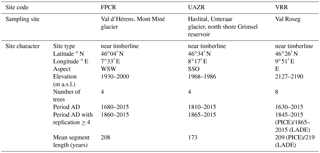
The Holocene wood remains stem from glacier forefields, peat bogs and small lakes and have been continuously collected over the last two decades (Joerin et al., 2006, 2008; Nicolussi, et al., 2009; Nicolussi et al., 2005; Nicolussi and Patzelt, 2000b). Wood material from the EACC (Eastern Alpine Conifer Chronology) has been merged and updated with subfossil samples of the same species and altitude collected by continued sampling of wood remains and stem discs in glacier forefields (Nicolussi and Schlüchter, 2012). Similarly to modern wood samples, the use of compression wood in these Holocene samples was avoided during further analysis.
2.2 Tree ring width and dating
Total tree-ring width (TRW) is measured for all samples. At least two radii are measured per tree with a precision of 0.001 mm by using a LINTAB device in connection to TSAPWin software (Rinn, 1996). For each sample, a mean TRW series is established by cross-dating and averaging the radii per tree. If the inner part of the stem is missing in a tree-ring series, the tree-ring number between the pith and the first tree ring measured is estimated (pith-offset, PO; Table 3). For the modern series, dating is simple as the exact sampling date is known. For the Holocene wood remains, the mean series are compared to local tree-ring series and reference chronologies (Nicolussi et al., 2009; Nicolussi and Schlüchter, 2012). Cross-dating is achieved by calculating statistical parameters and applying visual controls.
2.3 Sample preparation
Samples for CC (%) analysis are prepared as 5-year tree-ring blocks per tree, thus no pooling between trees is applied. For the Holocene samples, radial wedges are cut out of stem disks by using a bandsaw. Subsequently, blocks are prepared from the wedges and the cores under the microscope by using a commercial scalpel. The dry weight of each block is scaled and is defined to be between 20 and 50 mg, as a 60 % weight loss is expected during α-cellulose extraction. Further, the incipient degradation in the samples might have additionally reduced the CC (%). The blocks are further cut into small slices of wood material by first being sliced in parallel to the fibre direction and subsequently against the fibre direction. The resulting size of the cut sample is < 1 mm. Again, the dry weight of the cut sample is scaled to estimate the loss during the cutting procedure. For the following chemical treatment, the samples are packed into ANKOM filter bags (type F57), heat-sealed and labelled individually.
2.4 Cellulose extraction
The cellulose extraction is based on a modified Jayme–Wise procedure (Boettger et al., 2007; Borella et al., 1999; Leuenberger, 1998). The bleaching (delignification) of samples in a 1 % NaClO2+CH3COOH solution with a pH value of 4 is conducted at 70 ∘C for at least 30 h to remove lignin. In a second step, samples are treated in a 17 % NaOH solution at 25 ∘C for 45 min to extract the pure α-cellulose (Burton and Rasch, 1931; Cross and Bevan, 1912). Subsequently, samples are neutralised in a 1 % HCl solution using 25 % HCl and washed intensely with heated and cold distilled water until the pH of the washing water is ∼ 7. Samples are dried in an oven at 50 ∘C; the duration of the drying is thereby dependent on the sample size in the individual filter bags.
2.5 Calculation of CC (%)
The CC (%) is calculated from the dry weight of wood and the α-cellulose weight of a sample:
The wood dry weight is thereby defined as the sample weight of the individual wood sample after cutting, and the cellulose weight refers to the weight of the extracted α-cellulose after being removed from the ANKOM filter bag.
A remaining source of error is the collection of dry α-cellulose from the ANKOM filter bags. The described slicing of wood samples facilitates the removal of α-cellulose from filter bags compared to ground wood samples and thereby reduces the loss of α-cellulose material when it is collected from the filter bags. In this study, the resulting loss is examined for 42 samples, for which the filter bag including the α-cellulose and the emptied filter bag are scaled in addition to the α-cellulose weight to give an accurate estimate of the observed loss.
Table 3Individual tree characteristics per modern sampling site. Given are the tree species, start and end date of the α-cellulose content series in years b2k and years AD in brackets, respectively. The length of the individual series is enlisted in years and the estimation of the pith offset (PO) in annual resolution and calculated as the number of 5-year blocks. The number of 5-year cellulose blocks covering the length of the cellulose series is shown.
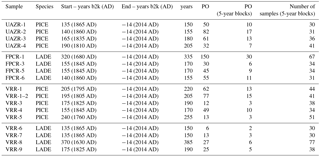
2.6 Outlier detection and correction
Outliers in CC (%) are observed for Holocene wood remains, for which the outermost rings have experienced a higher degree of degradation, e.g. due to abrasion at the glacier sites or the exposition of wood to environmental influences and associated weathering of the sample. The differential grades of degradation lead to pronounced decreases in CC (%) appearing within the outermost rings but also along cracks between individual rings and occasionally on the innermost parts of trees, which complicates the comparison of individual time series. Therefore, detection and elimination of outliers are performed by visual and statistical application of box plots (Tukey, 1977). Tukey (1977) defines outliers as samples, which lie outside the whiskers, whereby the extension of the whisker is limited by the 1.5 interquartile range (IQR). In addition to the box plots, visual comparisons of suspicious samples with other cellulose series are applied; thereby outliers close to the whiskers were revealed to be true values and the loss of samples by outlier detection and elimination could be minimised to the most extreme values.
2.7 Meteorological data
CC (%) series of modern sampling sites are correlated with meteorological data from the HISTALP database to investigate the relationship between climate and CC (%) (Auer et al., 2007). Data are obtained in the form of coarse-resolution subregional means (CRSM), calculated as arithmetic means from homogenised individual station series for five defined subregions within the Greater Alpine Region (GAR), which have been identified by EOF-based regionalisation. Auer et al. (2007) especially recommend CRSM series for lower-frequency analysis for all climate parameters. Series from the sampling sites FPCR and UAZR are correlated to meteorological data from the NW subregion, and from VRR to data from the SW region, respectively, due to their geographical location.
For these subregions, monthly anomalies (calculated for the reference period 1961–1990) of temperature (T (∘C), 1760–2008), precipitation (PREC (mm), 1800–2008), cloud cover (CLOUD (%), 1840–2008) and sunshine (SUN (h), 1880–2008), including the arithmetic seasonal (MAM, JJA, SON, DJF), semi-annual (AMJJAS, ONDJFM) and annual means are extracted. For further analysis, 5-year mean values are calculated for the annually resolved climate data as CC (%) series were established in 5-year resolution and thereby integrate the mean climatic signal over five consecutive years.
Pearson's correlation coefficient is calculated between the mean CC (%) series per site and species, and the monthly, seasonal and annual values for the four climate variables for the time period 1865–2008 (1880–2008 in case of sunshine). We assume here that each data point (5-year blocked data) in the climate variable data sets and the CC (%) series is independent, since there is no data point overlap.
Table 4Calculated sample loss during unpacking of extracted α-cellulose from ANKOM filter bags (type F57) for 42 samples (sample tree MM-602, PICE). The expected sample weight is calculated by subtracting the scaled weight of the emptied filter bag from the weight of the filled bag. The difference between the expected weight of α-cellulose and the scaled weight of unpacked cellulose defines the loss, which is given here in (mg) and (%).
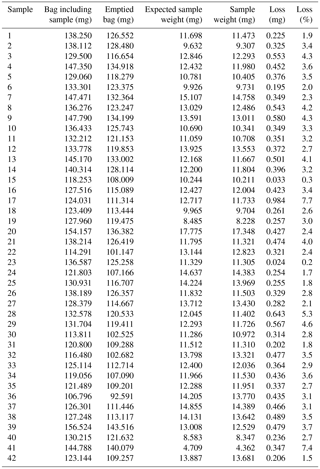
As the precise calculation of the CC (%) depends on error estimates for the dry weight of samples and the cellulose weight, two steps in the calculation of CC (%) are improved here: the precision of dry weight and the loss estimation in cellulose weight.
The mean sample loss during the cutting process amounts to 2.6 ± 1.7 % of the dry weight of an individual sample, for which mean loss values range from 0.3 up to 11.1 % per tree (data not shown here). Besides, very few individual samples experienced losses between 10 and 30 % caused by wood pieces bouncing off during cutting or loss of material due to powdery wood substance as a result of degradation. Hence, for the precise calculation of CC (%), a determination of the sample weight after cutting or milling is essential.
Secondly, when unpacking the cellulose material from the filter bags, there is always a risk that smallest fibres remain in the filter bag or fly away during the removal; therefore, we assume this error to be systematic. In this study, we estimated this error by analysing 42 filter bags, which revealed a mean loss of α-cellulose of 0.378 ± 0.163 mg (3.2 ± 1.4 %) (Table 4). Therefore, the calculation of the CC (%) results in the minimum CC (%) of the sample, as losses of cellulose of the order of 3.2 ± 1.4 % of the cellulose weight are to be expected, which would result in slightly increased CC (%) values. However, as the unpacking is accomplished equally for all samples, a systematic error is assumed which affects the CC (%) calculation in the same manner. It can be assumed that the systematic error does not influence the variability of the individual CC (%) series.
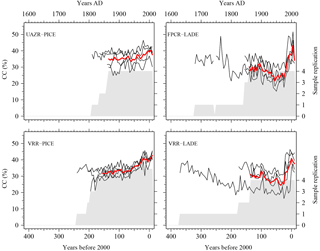
Figure 3Individual cellulose content series for two coniferous species (LADE, PICE) at the modern sites. The individual cellulose content time series (black lines) are supplemented by the mean cellulose content per species and site (red line), calculated as the arithmetic mean of the individual trees for the common growth period (sample replication ≥ 4). The sample replication (grey area) displays the temporal coverage per site.
Further, the relative uncertainty in cellulose weight transfers directly to the relative uncertainty in CC (%) determination as relative uncertainties are additive, with the uncertainty of the dry weight after cutting being negligible. Relevant for the CC (%) variation is the variation of the relative uncertainty (±1.4 %) and not the relative uncertainty itself (3.2 %), which only yields a mean offset of the whole curve. Although the relative uncertainty slightly limits the interpretation of minor differences between the individual 5-year CC (%) samples, it does not limit the investigation of trends in CC (%) series.
The improvement in the CC (%) calculation and the estimation of the relative error justify the use of the term “CC (%)” rather than “cellulose yield”, as sources of error are reduced and well estimated, and resulting calculations are close to the true values.
3.1 Modern CC (%) series
For a better understanding of CC (%) variations, modern CC (%) series are analysed for their temporal variability per species and site, for which the absolute values and variations in CC (%) are given in the unit CC %. Trees sampled at UAZR consist solely of PICE samples, as the treeline at that site is only composed of one tree species. Measured tree-ring width series vary in length between 150 and 205 years; the pith offset estimation varies between 32 and 82 for the individual trees (Table 3). The mean segment length (MSL) amounts to 173 years and the period covered by all four samples spans from 1860 to 2014 AD (Table 1). The mean cellulose content per tree varies between 31.6 and 39.7 CC %, where UAZR-1 (33.2 CC %) and UAZR-2 (33.2 CC %) show distinctly lower mean CC (%) values as UAZR-3 (38.6 CC %) and UAZR-4 (39.7 CC %). These differences are a result of low minimum values for UAZR-1 and UAZR-2, which are up to 10 CC % lower than for the other two trees (Table 5). Further, the time series of CC (%) for UAZR-1 and -2 show more variability in their series compared to UAZR-3 and -4, which show high common variability and very small fluctuations in their CC (%). This is further confirmed by the calculated Pearson's correlation coefficient (r), with the highest values for UAZR-3 and -4 (r=0.64) (Table 6). A significant correlation is also found between UAZR-2 and -3 (r=0.36), whereas the remaining correlation coefficients are non-significant (p>0.05). The arithmetic mean calculated from four samples in their common period (1860–2015 AD) indicates an increase in CC (%) over time by ∼ 5 CC % (red line, Fig. 3, top left).
Table 5General statistics of the individual α-cellulose content series per tree from the modern sites. Given are the arithmetic mean values, the standard deviation, maximum and minimum α-cellulose content values as well as the range per tree. To simplify the comparison between species, the species per site and tree individuum are enlisted once more.
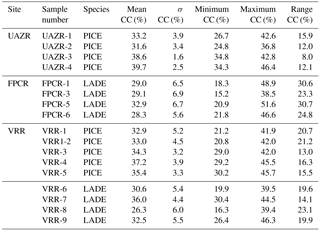
Table 6Pearson's correlation coefficient calculated for the individual trees per site and species calculated in their common growth period (sample replication ≥ 4 trees). Bold numbers indicate that correlations coefficients are significant (p<0.05).
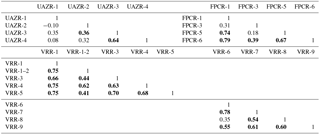
In contrast to UAZR, the sampling site FPCR consists solely of LADE trees, while tree-ring series contain between 155 and 335 years. The MSL amounts to 208 years, raised by the sample FPCR-1 (335 years), whereas the residual tree-ring series consist of 155 and 170 years (Table 1, Table 3). The four tree samples cover a common period from 1860 to 2015 AD (Table 1). The mean CC (%) per tree varies between 28.3 and 32.9 CC %, showing overall lower mean CC (%) values than observed for the PICE trees at UAZR (Table 5). Further, the range of CC (%) per individual tree is large, as LADE trees show low minimum CC (%) values between 15.2 and 21.8 CC % as well as high maximum values of up to 51.6 CC % (Table 5). In their common period, trees at FPCR show a decrease in CC (%) from 1860 to 1945 AD and a strong increase of nearly 20 CC % from 1980 to 2014 AD, which is common among the individual trees (Fig. 3, top right). Pearson's correlation coefficient illustrates the common variability among the samples FPCR-1, -5 and -6, showing high correlation coefficient between 0.67 and 0.79, whereas non-significant and lower correlation coefficients are found for FPCR-3 (Table 6).
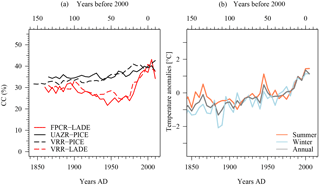
Figure 4Modern cellulose content chronologies per site and species (a) and temperature anomalies shown for the NW and SW subregions within the Greater Alpine Region from HISTALP for summer (April–September) and winter (October–March) half-years and per year (b). The CC (%) chronologies are calculated as arithmetic means from individual series for their common period (sample replication ≥ 4). HISTALP data are given for the summer season (April–September), winter season (October–March) and annual anomalies calculated as 5-year averages with respect to the sample resolution of CC (%) series. Temperature anomalies for SW and NW subregions in the displayed season means are equal.
The sampling site VRR located in the Val Roseg in eastern Switzerland, and in the proximity of the Tschierva glacier, is the only sampling site where both tree species, LADE and PICE are found, thereby allowing a direct comparison of species under the same climatic growing conditions.
PICE trees at VRR exhibit tree-ring series of 170 to 255 years, with a common period between 1845 and 2015 AD and a MSL of 209 years (Table 1, Table 3). Mean CC (%) varies between 32.9 and 37.2 CC %, at which the samples VRR-1 and VRR-1–2 (same tree) reveal a range of > 20 CC %, whereas the other PICE trees at VRR exhibit sizes between 13 and 16.3 CC % (Table 5). Minimum values are around 20 CC % for VRR-1 (and 1–2) and around 30 CC % for all the other trees, and maximum values exceed 40 CC % with maximum values > 45 CC % (Table 5). Calculated correlation coefficients are almost all significant (p<0.05) and are between 0.41 and 0.75 (Table 6). The arithmetic mean series for VRR-PICE displays an increase in mean CC (%) of > 10 CC % in the common period (Fig. 3, bottom left).
The LADE tree-ring series at the same location, with a common growth period between 1865 and 2015 AD and MSL of 219 years, consist of 2 times 150 years, 190 and 385 years (Table 1, Table 3). Mean CC (%) is between 26.3 and 36.0 CC % with minimum values ranging from 16.3 to 30.4 CC %, whereas the maximum values per tree show a lower spread between 39.4 and 46.3 CC % (Table 5). As for PICE, LADE CC (%) series reveal significant correlation coefficients among the individual trees (Table 6). The arithmetic mean series shows a decrease in CC (%) of approximately 5 CC % in the period 1865–1980 AD, followed by a strong increase of more than 10 CC % during the last three decades (Fig. 3, bottom right).
The investigation of individual tree-ring CC (%) series from three sampling sites (UAZR, FPCR, VRR) and two coniferous tree species (PICE, LADE) displays common variability of trees from the same species, independent of the sampling site. Individual CC (%) per site and species show a general good agreement, represented in their correlation coefficients, which allows the establishment of arithmetic mean chronologies for their common growth periods (comparable time periods for all sites).
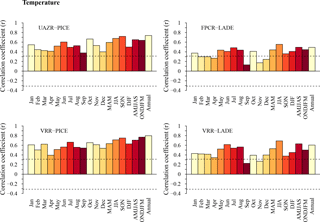
Figure 5Pearson's correlation coefficient for mean CC (%) and temperature anomalies (with reference to 1961–1990) for the period 1865–2005 AD. UAZR and FPCR are correlated with HISTALP NW region data, whereas VRR species are correlated with the SW region data set. The dashed horizontal lines indicate the level of significance (p<0.05).
Common trends in the tree species, independent of the sampling site, indicate a common influence from environmental factors on CC (%). All PICE series reveal a continuous increase of CC (%) over time, whereas the LADE samples show a decrease over the common period that is replaced by a rapid increase over the past three decades (Fig. 4, left). The close relationship between the site series of the same tree species is also revealed by the calculated Pearson's correlation coefficient, where r=0.86 for LADE and r=0.76 for PICE mean series.
3.2 Climate–cellulose relationships
To test the influence of environmental conditions on CC (%), Pearson's correlation coefficient is calculated between the mean CC (%) series per site and species and the climate variables of temperature (cf. Fig. 4, right), precipitation, sunshine as well as cloud cover (Figs. 5–6, Figs. S1–S2 in the Supplement).
A temperature signal is clearly recorded in both tree species, independent of the sampling site (Fig. 5). Both PICE sites show significant correlations with temperature throughout the year, with high correlation during the growing season and, interestingly, in October (r=0.67 for UAZR-PICE, r=0.65 for VRR-PICE). Seasonal temperature averages result in the highest correlations for PICE; correlations for the summer season (JJA) result in r=0.68–0.71 and for autumn (SON) in r=0.72–0.75 for UAZR-PICE and VRR-PICE, respectively. In LADE, correlations with temperature are lower than for PICE and significant correlations are found mostly during the growing season (May–August). Seasonal averages in temperature anomalies result in the highest correlations for the summer season (JJA) for both FPCR-LADE (r=0.55) and VRR-LADE (r=0.69).
Precipitation is best recorded in PICE at UAZR during the early growing season, revealing the highest correlation coefficients in May (r=0.68). In contrast, LADE mean series at both sites and PICE at VRR mostly show non-significant correlations with precipitation records (Fig. 6).
The influence of cloud cover is the highest in PICE trees during the early growing season as well as towards the end (Fig. S1). Significant positive correlations are found in MAM for UAZR-PICE (r=0.49) and for JJA in both UAZR-PICE (r=0.41) and VRR-PICE (r=0.42). Interestingly, the correlation coefficient for cloud cover and LADE mean series reveals significant negative correlations during the winter season (DJF; for FPCR-LADE and for VRR-LADE), especially in January for FPCR-LADE and in February for VRR-LADE .
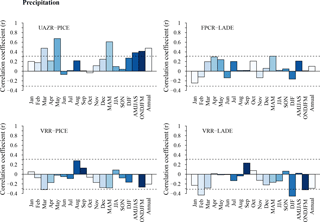
Figure 6Pearson's correlation coefficient for mean CC (%) and temperature anomalies (with reference to 1961–1990) for the period 1865–2005 AD. UAZR and FPCR are correlated with HISTALP NW region data, whereas VRR species are correlated with the SW region data set. The dashed horizontal lines indicate the level of significance (p<0.05).
High, significant correlations with sunshine are observed for the late autumn and winter seasons, whereas negative correlations are found during summer season, being significant at both PICE sites in August, when VRR-PICE reveals significant negative correlations with sunshine from May to August (Fig. S2). For UAZR-PICE, the highest correlations with sunshine are found in January (r=0.86) as well as in February (r=0.76) and for the entire winter season (DJF, r=0.87), whereas for VRR-PICE, the highest correlations are found for November and December (r=0.84 and r=0.82, respectively) as well as for the winter season (r=0.79). The pattern is similar for FPCR: significant positive correlations occur in late autumn and winter (N, D, J, F) and are highest in January (r=0.62). In contrast, VRR-LADE shows the highest correlations in late autumn (November) and in February (r=0.66 and r=0.55, respectively).
In summary, the results of the Pearson's correlation coefficient analysis between HISTALP data and mean CC (%) per species and site partly indicate a significant influence of environmental factors (temperature, precipitation, cloud cover, sunshine duration) on the CC (%) in tree rings and the potential to reconstruct past climate from this novel supplementary proxy. However, the comparison of the two coniferous species further indicates a species-specific response from CC (%) to the environmental conditions.
3.3 Holocene CC (%) series
In a further step, CC (%) series are established for LADE and PICE tree-ring samples from glacier forefields, peat bogs and small lakes for the period from 8550 to 3500 years b2k (Fig. 7). To investigate trends in the CC (%), the individual series are averaged per species (PICE in blue, LADE in green), and an arithmetic mean is also calculated over all series (black), which is smoothed by a spline (orange) to illustrate long-term changes (Fig. 7). The arithmetic mean series shows the high variability in the CC (%) over time, fluctuating between 22 and 40 CC %. Further, the series exhibits interesting low-frequency trends with rapidly decreasing CC (%) in the periods 8250–7950 years b2k (Δ CC (%) ∼ 5 CC %), 6250–5950 years b2k (Δ CC (%) ∼ 2 CC %), 5650–5450 years b2k (Δ CC (%) ∼ 4 CC %), 5300–5000 years b2k (Δ CC (%) ∼ 5.5 CC %) and 4500–4000 years b2k (Δ CC (%) ∼ 6 CC %). Besides these phases of rapid decreases, CC (%) is increasing on a multi-centennial scale between 7350 and 6250 years b2k (Δ CC (%) ∼ 4.5 CC %) and more rapidly after low CC (%) phases, e.g. in the periods 7950–7750 years b2k (Δ CC (%) ∼ 3.5 CC %), 5450–5300 years b2k (Δ CC (%) ∼ 7 CC %) and 4000–3650 years b2k (Δ CC (%) ∼ 8.5 CC %). The calculated arithmetic mean series per species exhibit mostly lower mean values in the LADE series (green) and more positive values for PICE (blue). LADE shows extremely low CC (%) between 4700 and 4200 years b2k, and extremely high values between 4000 and 3900 years b2k. The PICE average series shows extremely low values around 8000 years b2k as well as around 4000 years b2k; otherwise both the LADE and PICE series mostly fluctuate between 30 and 40 CC %.
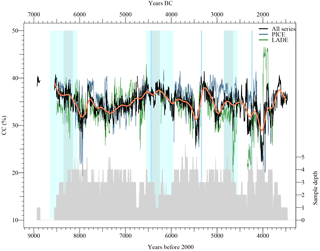
Figure 7Progression of mean cellulose content during the time period 9000 to 3500 years b2k. Mean cellulose content is calculated for the two species Larix decidua Mill. (LADE, green line) and Pinus cembra L. (PICE, grey line); the overall mean series is shown in black and its smoothed values in orange. The sample depth is on the right side (grey polygon). Major cold phases are indicated as cyan rectangles, where darker colours implicit the cold events and the lighter colours their entire duration (Wanner et al., 2011). Additional cold events are marked as blue vertical lines (Wanner et al., 2015).
For most of the investigated time period, the sample replication amounts to three trees, two gaps at 7300 and 6300 years b2k are solely covered by a single tree and a replication of ≥ 4 trees is partly achieved (Fig. 7, bottom).
Figure 7 further displays identified cold phases (light-blue bars) analysed by Wanner et al. (2011), where the darker bars illustrate the cold phases which were identified by three different phenomena (cold phases, glacier advances, Bond cycles) and the lighter areas mark the entire length of the cold phases; additional cold periods are marked as blue vertical lines (Wanner et al., 2011, 2015). Interestingly, the strong decreases in cellulose content emerge mostly after the indicated cold phases.
As modern wood samples already displayed differential trends in the CC (%), mean series of LADE and PICE and their low-frequency trends are investigated more closely in Fig. S3, where the contribution of individual trees per species is revealed in the sample replication (Fig. S3d). The mean series reveal distinct offsets over time (Fig. S3a). In the periods 8100–7800 years b2k and 5600–4600 years b2k, the smoothed values exhibit a common long-term trend, whereas in the remnant phases, the series even show opposed trends. The difference between the two arithmetic mean series emphasises that the differences between the species are not constant over time (Fig. S3b).
Although the Holocene samples stem from various sites, the phases 8900–8200 years b2k and 7500–6700 years b2k are dominated by glacier forefield sites at Mont Miné and Tschierva glacier, respectively (Fig. S3c). For both intervals, the two coniferous species are present, and as the sampling site is equal, similar as well as divergent trends in the species result, presumably from the impact of environmental factors on CC (%).
In order to also confirm the non-existing influence of the sampling site location on CC (%) series in Holocene samples, the arithmetic mean of CC (%) per site and species is calculated and compared to latitude, longitude and elevation of the individual sites (Fig. S4). Calculated linear regressions (dotted lines) per species do not indicate a dependence on CC (%) and sampling site locations, as linear regressions are non-significant (p>0.05).
To further exclude non-climatic influences on CC (%), individual CC (%) series per tree are aligned according to their biological age by taking the estimated pith-offset into account (Fig. S5). Both PICE (upper panel) and LADE (lower panel) do not reveal any distinct low-frequency trends in the age-aligned series.
The determination of CC (%) is a common procedure in tree-ring laboratories that measure stable-isotope ratios in tree-ring cellulose. However, to our current knowledge, existing records of CC (%) were simply used as a tool to ascertain the quality of the extracted cellulose; the CC (%) is, if mentioned in the literature, given as a mean value and its temporal variability is not regarded any further.
The investigation of CC (%) in modern tree samples of two high-Alpine coniferous species (LADE, PICE) at three sites in the Swiss Alps reveals common variations within the tree species, independent of the sampling site, and points out a common environmental driver. While PICE trees show a continuous increase over the investigated common period, LADE trees are characterised by a decrease throughout the 20th century followed by a strong increase in CC (%) from the 1980s to today.
In order to exclude any age-related biases, possible age trends in CC (%) series have been investigated, even though they were not expected as the trends in modern CC (%) series of the two tree species diverge and do not reveal a common increase or decrease over time. The age alignment of both modern and Holocene CC (%) series by their biological age, taking their estimated pith-offset (PO) into account, illustrates that CC (%) series are not biased by age trends, which leads us to the conclusion that the trends which we find in CC (%) series are most probably driven by climatic variables (Fig. S5).
Further, the two different species Larix decidua Mill. and Pinus cembra L. at the sampling site Val Roseg (VRR) exhibit different trends in their mean chronologies. As they experience the same climate, the role of the biological factors here is undoubted. This is rather obvious as the two coniferous species are compared: Larix decidua Mill. is a deciduous species, whereas Pinus cembra L. is an evergreen species. Therefore, differences in their metabolism are to be expected. Although the two species are found at the upper treeline and known to be adapted to the harsh environmental conditions, LADE is characterised as a light-demanding pioneer species which is often found in open settings, e.g. on glacier forefields, whereas PICE is, under undisturbed conditions, the highest rising species in the inner sections of the Alps and therefore adapted to short vegetation periods (Ellenberg, 1996). The fact that individual CC (%) series from the same species at different sites are similar calls for a common driving factor of regional extent, such as temperature.
The investigation of the climate–cellulose relationships by correlating mean CC (%) series with climate variables (temperature, precipitation, cloud cover, sunshine duration) extracted from the HISTALP database (Auer et al., 2007) over a common period of 140 years (1865–2008 AD; 1880–2008 for sunshine) reveals interesting correlation coefficients between CC (%) and climate. Temperature is recorded in both species, revealing the highest correlations during the summer season, and additionally for the autumn season in PICE trees. An increase in CC (%) in trees with increasing temperature may be explained by the enhanced sink activity in trees at the upper treeline. In the attempt to establish a functional explanation of the Alpine treeline, Körner (1998) compiled five hypotheses in which the climate-driven treeline is either driven by stress, disturbance, reproduction, carbon balance or growth limitation (Körner, 1998). Since then, several publications have shown that concentrations of non-structural carbon (NSC) in trees at the upper treeline increase with elevation, thereby indicating no evidence that trees at high elevations experience a carbon shortage but rather implying a lowered sink activity at the upper treeline resulting in a growth-limitation (Hoch et al., 2002; Hoch and Körner, 2009, 2012; Körner, 2003). As photosynthetic activity is less sensitive to low temperatures than growth, it may still be active at 0 ∘C, at which temperatures are too low for tree growth (minimum temperatures for tree growth are ∼ 5–7 ∘C) (Hoch and Körner, 2012; Körner, 1998; Tranquilini, 1979). Therefore, the carbon acquisition in a tree can still be active, although low temperatures prevent tissue formation. As a consequence, the concentration of NSC in trees at the upper treeline is increasing, as the source is still active, but the sink is not. In conclusion, lower temperatures during the growing season reduce the tissue formation in tree rings, resulting in less CC (%) in the tree ring as the source activity is low. Regarding the investigated species in this study, the LADE as a pioneer species that explores the upper treeline greatly benefits from the increase in temperature associated with global warming and which has been especially pronounced since the 1980s (Rebetez and Reinhard, 2008). As temperatures during the growing season increase and the growing season is potentially prolonged, the sink activity and the CC (%) in tree rings increase. This might explain the correlations found with winter temperatures, especially for PICE trees, i.e. potential photosynthetic activity at low temperatures in winter when tissue formation is no longer possible (Hoch et al., 2002 and references therein). Thereby, the concentration of NSC is increasing and is already available for tissue formation as soon as temperatures allow for it. Future studies on CC (%) on an annual and even intra-annual resolution could help to improve our understanding of the influence of winter temperatures on the CC (%) in tree rings.
In PICE trees, the CC (%) slowly and steadily increases over time, potentially reacting more slowly to increased temperatures, prolonged growing seasons and rising mean annual temperatures. In contrast, LADE series from modern trees exhibit a rapid increase over the past 30 years, obviously benefitting from increased temperatures during the growing season. The CC (%) in Holocene wood remains and modern wood samples could therefore serve as a novel additional proxy in multi-proxy approaches, offering the opportunity to test the temperature sensitivity of diverse tree species, before combining them into chronologies. A combination of differently reacting tree species allows a complementation of temperature–CC (%) relationships, which may result in more robust climate reconstructions.
Moreover, the PICE trees at two modern sampling sites showed highly significant correlation coefficients with sunshine duration especially during the winter season. As evergreen conifers are known to be photosynthetically active throughout the year as long as temperatures permit photosynthetic activity (Hoch et al., 2002 and references therein), PICE trees may potentially benefit from increased sunshine duration and be able to increase the carbon acquisition during the winter season. Therefore, the CC (%) in PICE trees may even represent an annual signal. On the other hand, significant correlations found for sunshine duration and cloud cover with LADE CC (%) series may represent artefacts, as LADE trees lose their needles in autumn and carbon acquisition is only possible during the growing season.
As the framework of the project AHTRIR included analyses of both living and subfossil wood, Holocene wood remains were also investigated for signs of degradation. Most samples were well-preserved, and for the period from 9000 to 3500 years b2k, the CC (%) also varied between 30 and 40 CC %. Therefore, the CC (%) in living and subfossil wood samples is comparable. Only a small number of outliers were found (see also Sect. 2.6), where CC (%) values showed pronounced decreases, mostly appearing in the outermost rings as well as along cracks in the wooden material. We assume that these tree-ring sections have been affected by weathering and therefore reveal a high degree of degradation, whereas the other rings have been well preserved (Fig. S6). Although the potential degradation of subfossil wood might have an impact, CC (%) of modern and subfossil wood is comparable despite a few outliers, which leads to the conclusion that long-term variations in Holocene CC (%) could serve as an indicator of climate variations. Moreover, there is no trend detected in CC (%) over time (i.e. towards the past) which would be expected if degradation was a major driver of CC (%) variations.
The low-frequency trends exhibited in the mean series of Holocene CC (%) in the period from 9000 to 3500 years b2k illustrate the potential of CC (%) as an additional proxy. The arithmetic mean CC (%) series shows pronounced decreases after known cold events in the Early and mid-Holocene, whereas a continuous increase is observed between 7350 and 6250 years b2k, which could be the result of increased temperatures and more favourable growing conditions for trees at the upper treeline. However, the investigations of individual species also illustrate differences in variation between LADE and PICE, approving the observed differences in species within modern samples. A complete understanding of CC (%) variations in different tree species and the influence of environmental conditions on CC (%) will help to further improve the robustness of this novel proxy.
The presented study could benefit from the framework of the project in which it was performed, but was at the same time limited by it: the vast advantage of the presented study is thousands of individual cellulose samples from both living and subfossil wood material distributed over large parts of the Holocene, which allowed the investigation of their CC (%) and served as a testbed for the temporal study of CC (%) in tree rings. However, we were at the same time limited by the high number of samples, which so far has not allowed for an analysis of replicates within this project. Further, the high-Alpine tree species used in this project often reveal very narrow rings and the amount of extracted α-cellulose was just sufficient for further analysis. As the initial aims of the project did not include the closer analysis of CC (%) and its variation but was rather a concept that developed during the progress of the project, the sampling and analysis of replicates has not been conducted so far. Yet, in a study performed earlier from the Lötschental in Switzerland, we evaluated the natural variability of CC (%) on different LADE tree-ring cores over time (Figs. S7, S8). It documents a mean standard deviation of 3.7 % in CC (%) for five individual cores from different trees of the same location. This standard deviation would even be significantly smaller if the values of the different cores were adjusted according to their mean values. Therefore, we are confident that replicates of LADE samples of the present study would be the same within a few couple of percent (approx. 3 to 4 %). In the current study, first measures to minimise and quantify the error of CC (%) have been presented; however, in future studies, it will be essential to accomplish a robust error estimation by a replicate sampling of the same tree.
For the first time, CC (%) and its temporal variability of the two coniferous Alpine treeline species Larix decidua Mill. (European larch, deciduous) and Pinus cembra L. (Swiss stone pine, evergreen) have been investigated on a centennial scale for modern samples from living trees and on a multi-millennial scale for the Early and mid-Holocene including the transition to the Late Holocene. The established CC (%) series revealed species-specific long-term trends, independent of the location of the sampling site along the Swiss Alps in both past and present tree samples. First investigations of climate–cellulose relationships display the ability of CC (%) to record temperature at the Alpine treeline.
As higher CC (%) values have been observed for low-elevation tree-ring material (unpublished data), the influence of elevation ought to be examined along an altitudinal gradient to quantify the decrease in α-cellulose and thereby verify the hypothesis that not carbon, but temperature is the limiting factor, resulting in limited sink activity which results in turn in lower CC (%) values. This goes along the line of non-structural carbon investigations.
In a succeeding study, the site and species sensitivity of multiple proxies (TRW, CC (%), δD, δ13C, δ18O) and the correlations between the individual proxies will be examined in relation to the environmental conditions per site to quantify their ability for multi-millennial reconstructions based on a multi-proxy approach from tree rings (Ziehmer et al., 2018). Thereby, the effects of variations in CC (%) on triple isotope records will be tested.
Moreover, CC (%) series ought to be established in annual resolution to establish a robust calibration and verification of the CC (%)–climate relationship as the reduced resolution of our samples (5-year tree-ring blocks) prohibits the proper analysis of the climatic response of cellulose and a robust calibration against instrumental data. The existence of numberless CC (%) series in dendro-laboratories all over the world will allow a broad investigation of CC (%) in various species from widespread sampling sites in temporal resolution, reaching from intra-annual to decadal tree-ring samples.
A future interlaboratory comparison comparable to the study by Boettger et al. (2007) could confirm the comparability of α-cellulose series between individual laboratories. Such a comparison should also include investigations on the influence of preparation methods (milling vs. cutting wood), the individual steps and duration of the extraction, the role of the sample size, as well as the influence of tree species, juvenile vs. mature tree rings, and heartwood vs. sapwood. Further, purity of the extracted α-cellulose can be checked by FTIR spectra (Galia, 2015). Such an interlaboratory comparison is essential and a prerequisite for the assessment of the accuracy of CC (%) and comparison of CC (%) series among different tree-ring laboratories.
Further, the analysis of a relationship between CC (%) and wood density would be highly interesting. These two proxies are usually not investigated within the same research project. However, establishing a link between these two variables might allow conclusions to be drawn on wood density by determining the CC (%) and vice versa. Hence, further research on the relationship between CC (%) and other tree-ring proxies (tree-ring width, maximum latewood density, stable isotopes) is essential. Although evaluating CC (%) is obviously not easier than measuring tree-ring width, there is the significant potential to use it as an additional supplementary proxy, especially in those cases in which CC (%) series are already existent in tree-ring laboratories and climate is to be reconstructed in a multi-proxy approach.
A complete comprehension of environmental factors controlling the CC (%) in diverse tree species will result in an improved understanding of physiological and biochemical processes in forming annual growth layers, and thus serve as an additional proxy in dendroclimatology. This study represents a first step in this direction and intends to motivate other tree-ring researchers in the field of dendroclimatology and stable-isotope analysis to investigate their existing CC (%) series and to carry out further research on these data.
At present, data can be obtained upon request. As agreed among the project participants, data sets will be made available to the public after the official completion of the Alpine Holocene Tree Ring Isotope Records (AHTRIR) project.
The supplement related to this article is available online at: https://doi.org/10.5194/bg-15-1047-2018-supplement.
MMZ and ML designed the study. The subfossil wood sampling was mainly done by KN and CS, whereas the living wood sampling was performed by all authors. The preparation of the experiments as well as the statistical analysis and interpretation were carried out by MMZ. The drafting of the manuscript was accomplished by MMZ with contributions from all co-authors.
The authors declare that they have no conflict of interest.
We thank Andrea Thurner and Andreas Oesterreicher from the Alpine Tree-Ring Group at the University of Innsbruck for the sample preparation. Further thanks go to Jonathan Lamprecht, Yannick Rohrer, Igor Zelenovic, Regula Mülchi and Martina Messmer for assistance during sample preparation. Many thanks go to Peter Nyfeler for technical support and experience.
The project is funded by the Swiss National Science Foundation (SNSF,
2000212_144255) and the Austrian Science Fund (FWF, grant
I-1183-N19) and is supported by the Oeschger Center for Climate Change
Research, University of Bern, Bern, Switzerland (OCCR).
Edited by: David Gillikin
Reviewed by: two anonymous referees
Anhäuser, T., Greule, M., Polag, D., Bowen, G. J., and Keppler, F.: Mean annual temperatures of mid-latitude regions derived from δ2H values of wood lignin methoxyl groups and its implications for paleoclimate studies, Sci. Total Environ., 574, 1276–1282, https://doi.org/10.1016/j.scitotenv.2016.07.189, 2017.
Auer, I., Reinhard, B., Jurkovic, A., Lipa, W., Orlik, A., Potzmann, R., Sch, W., Ungersb, M., Matulla, C., Briffa, K., Jones, P., Efthymiadis, D., Brunetti, M., Nanni, T., Maugeri, M., Mercalli, L., Mestre, O., Moisselin, J., Begert, M., and Gerhard, M.: HISTALP – historical instrumental climatological surface time series of the Greater Alpine Region, 46, 17–46, https://doi.org/10.1002/joc.1377, 2007.
Boettger, T., Haupt, M., Knller, K., Weise, S. M., Waterhouse, J. S., Rinne, T., Loader, N. J., Sonninen, E., Jungner, H., Masson-delmotte, V., Guillemin, M., Pierre, M., Pazdur, A., Leuenberger, M., Filot, M., Saurer, M., Reynolds, C. E., Helle, G., and Schleser, G. H.: Wood Cellulose Preparation Methods and Mass Spectrometric Analyses of δ13C, δ18O, and Nonexchangeable δ2H Values in Cellulose, Sugar, and Starch: An Interlaboratory Comparison, Sug, Anal. Chem., 79, 4603–4612, https://doi.org/10.1021/ac0700023, 2007.
Borella, S., Leuenberger, M., Saurer, M., and Siegwolf, R.: C analysis of tree rings: Pooling, milling, and cellulose extraction, J. Geophys. Res., 103, 19519, https://doi.org/10.1029/98JD01169, 1998.
Borella, S., Leuenberger, M., and Saurer, M.: O in tree rings: Wood-cellulose comparison and method dependent sensitivity, J. Geophys. Res., 104, 19267, https://doi.org/10.1029/1999JD900298, 1999.
Brenninkmeijer, C. A. M.: Deuterium, oxygen-18 and carbon-13 in tree rings and peat deposits in relation to climate, PhD thesis, University of Groningen, the Netherlands, 1983.
Büntgen, U., Frank, D. C., Nievergelt, D., and Esper, J.: Summer temperature variations in the European Alps, AD 755-2004, J. Clim., 19, 5606–5623, https://doi.org/10.1175/JCLI3917.1, 2006.
Büntgen, U., Tegel, W., Nicolussi, K., McCormick, M., Frank, D., Trouet, V., Kaplan, J. O., Herzig, F., Heussner, K.-U., Wanner, H., Luterbacher, J., and Esper, J.: 2500 years of European climate variability and human susceptibility, Science, 331, 578–582, https://doi.org/10.1126/science.1197175, 2011.
Burton, J. O. and Rasch, R. H.: The determination of the alpha-cellulose content and copper number of paper, Bur. Stand. J. Res., 6, 603, https://doi.org/10.6028/jres.006.037, 1931.
Cross, C. F. and Bevan, E. J.: Researches on cellulose III (190–1910), Longmans, Green, and Company, London, 1912.
Cullen, L. E. and Macfarlane, C.: Comparison of cellulose extraction methods for analysis of stable-isotope ratios of carbon and oxygen in plant material, Tree Physiol., 25, 563–569, https://doi.org/10.1093/treephys/25.5.563, 2005.
Ellenberg, H.: Vegetation Mitteleuropas mit den Alpen in ökologischer, dynamischer und historischer Sicht, Ulmer, Stuttgart, 1996.
Esper, J., Cook, E. R., and Schweingruber, F. H.: Low-frequency signals in long tree-ring chronologies for reconstructing past temperature variability, Science, 295, 2250–2253, https://doi.org/10.1126/science.1066208, 2002.
Filot, M. S., Leuenberger, M., Pazdur, A., and Boettger, T.: Rapid online equilibration method to determine the D/H ratios of non-exchangeable hydrogen in cellulose, Rapid Commun. Mass Spectrom., 20, 3337–3344, https://doi.org/10.1002/rcm.2743, 2006.
Frank, D. C., Poulter, B., Saurer, M., Esper, J., Huntingford, C., Helle, G., Treydte, K., Zimmermann, N. E., Schleser, G. H., and Ahlström, A.: Water-use efficiency and transpiration across European forests during the Anthropocene, Nat. Clim. Chang., 5, 579–583, 2015.
Freudenberg, K.: Lignin: Its Constitution and Formation from p-Hydroxycinnamyl Alcohols: Lignin is duplicated by dehydrogenation of these alcohols; intermediates explain formation and structure, Science, 148, 595–600, https://doi.org/10.1126/science.148.3670.595, 1965.
Galia, A., Schiavo, B., Antonetti, C., Galletti, A. M. R., Interrante, L., Lessi, M., Scialdone, O., and Valenti, M. G.: Autohydrolysis pretreatment of Arundo donax: a comparison between microwave-assisted batch and fast heating rate flow-through reaction systems, Biotechnology for Biofuels, 8, 1–18 https://doi.org/10.1186/s13068-015-0398-5, 2015.
Gaudinski, J. B., Dawson, T. E., Quideau, S., Schuur, E., Roden, J. S., Trumbore, S. E., Sandquist, D. R., Oh, S.-W., and Wasylishen, R. E.: Comparative Analysis of Cellulose Preparation Techniques for Use with 13C, 14C, and 18O Isotopic Measurements, Anal. Chem., 77, 7212–7224, https://doi.org/10.1021/ac050548u, 2005.
Genet, M., Li, M., Luo, T., Fourcaud, T., Clément-Vidal, A., and Stokes, A.: Linking carbon supply to root cell-wall chemistry and mechanics at high altitudes in Abies georgei, Ann. Bot., 107, 311–320, https://doi.org/10.1093/aob/mcq237, 2011.
Gindl, W., Grabner, M., and Wimmer, R.: The influence of temperature on latewood lignin content in treeline Norway spruce compared with maximum density and ring width, Trees – Struct. Funct., 14, 409–414, https://doi.org/10.1007/s004680000057, 2000.
Haigler, C. H., Ivanova-Datcheva, M., Hogan, P. S., Salnikov, V. V, Hwang, S., Martin, K., and Delmer, D. P.: Carbon partitioning to cellulose synthesis, Plant Mol. Biol., 47, 29–51, https://doi.org/10.1023/A:1010615027986, 2001.
Hales, T. C., Ford, C. R., Hwang, T., Vose, J. M., and Band, L. E.: Topographic and ecologic controls on root reinforcement, J. Geophys. Res.-Solid Earth, 114, 1–17, https://doi.org/10.1029/2008JF001168, 2009.
Hoch, G. and Körner, C.: The carbon charging of pines at the climatic treeline: a global comparison, Oecologia, 135, 10–21, https://doi.org/10.1007/s00442-002-1154-7, 2003.
Hoch, G. and Körner, C.: Growth and carbon relations of tree line forming conifers at constant vs. variable low temperatures, J. Ecol., 97, 57–66, https://doi.org/10.1111/j.1365-2745.2008.01447.x, 2009.
Hoch, G. and Körner, C.: Global patterns of mobile carbon stores in trees at the high-elevation tree line, Glob. Ecol. Biogeogr., 21, 861–871, https://doi.org/10.1111/j.1466-8238.2011.00731.x, 2012.
Hoch, G., Popp, M., and Körner, C.: Altitudinal increase of mobile carbon pools in Pinus cembra suggests sink limitation of growth at the Swiss treeline, Oikos, 98, 361–374, https://doi.org/10.1034/j.1600-0706.2002.980301.x, 2002.
Hu, W. J., Harding, S. A, Lung, J., Popko, J. L., Ralph, J., Stokke, D. D., Tsai, C. J., and Chiang, V. L.: Repression of lignin biosynthesis promotes cellulose accumulation and growth in transgenic trees, Nat. Biotechnol., 17, 808–812, https://doi.org/10.1038/11758, 1999.
Joerin, U. E., Stocker, T. F., and Schlüchter, C.: Multicentury glacier fluctuations in the Swiss Alps during the Holocene, The Holocene, 16, 697–704, https://doi.org/10.1191/0959683606hl964rp, 2006.
Joerin, U. E., Nicolussi, K., Fischer, A., Stocker, T. F., and Schlüchter, C.: Holocene optimum events inferred from subglacial sediments at Tschierva Glacier, Eastern Swiss Alps, Quaternary Sci. Rev., 27, 337–350, https://doi.org/10.1016/j.quascirev.2007.10.016, 2008.
Kahmen, A., Dawson, T. E., Vieth, A., and Sachse, D.: Leaf wax n-alkane δD values are determined early in the ontogeny of Populus trichocarpa leaves when grown under controlled environmental conditions, Plant, Cell Environ., 34, 1639–1651, https://doi.org/10.1111/j.1365-3040.2011.02360.x, 2011.
Keel, S. G., Joos, F., Spahni, R., Saurer, M., Weigt, R. B., and Klesse, S.: Simulating oxygen isotope ratios in tree ring cellulose using a dynamic global vegetation model, Biogeosciences, 13, 3869–3886, https://doi.org/10.5194/bg-13-3869-2016, 2016.
Keller, K. M., Lienert, S., Bozbiyik, A., Stocker, T. F., Churakova (Sidorova), O. V., Frank, D. C., Klesse, S., Koven, C. D., Leuenberger, M., Riley, W. J., Saurer, M., Siegwolf, R., Weigt, R. B., and Joos, F.: 20th century changes in carbon isotopes and water-use efficiency: tree-ring-based evaluation of the CLM4.5 and LPX-Bern models, Biogeosciences, 14, 2641–2673, https://doi.org/10.5194/bg-14-2641-2017, 2017.
Kimak, A., Kern, Z., and Leuenberger, M.: Qualitative Distinction of Autotrophic and Heterotrophic Processes at the Leaf Level by Means of Triple Stable Isotope (C-O-H) Patterns, Front. Plant Sci., 6, 1008, https://doi.org/10.3389/fpls.2015.01008, 2015.
Körner, C.: A re-assessment of high elevation treeline psoitions and their explanation, Oecologia, 115, 445–459, 1998.
Körner, C.: Carbon limitation in trees, J. Ecol., 91, 4–17, https://doi.org/10.1046/j.1365-2745.2003.00742.x, 2003.
Laumer, W., Andreu, L., Helle, G., Schleser, G. H., Wieloch, T., and Wissel, H.: A novel approach for the homogenization of cellulose to use micro-amounts for stable isotope analysis, Rapid Commun. Mass Spectrom., 23, 1934–1940, 2009.
Leavitt, S. W. and Danzer, S. R.: Method for batch processing small wood samples to holocellulose for stable-carbon isotope analysis, Anal. Chem., 65, 87–89, https://doi.org/10.1021/ac00049a017, 1993.
Leuenberger, M.: Stable isotopes in tree rings as climate and stress indicators, vdf Hochschulverlag AG, 1998.
Ljungqvist, F. C., Krusic, P. J., Sundqvist, H. S., Zorita, E., Brattström, G., and Frank, D.: Northern Hemisphere hydroclimate variability over the past twelve centuries, Nature, 532, 94–98, https://doi.org/10.1038/nature17418, 2016.
Loader, N. J., Robertson, I., Barker, A. C., Switsur, V. R., and Waterhouse, J. S.: An improved technique for the batch processing of small wholewood samples to α-cellulose, Chem. Geol., 136, 313–317, https://doi.org/10.1016/S0009-2541(96)00133-7, 1997.
Loader, N. J., Robertson, I., and McCarroll, D.: Comparison of stable carbon isotope ratios in the whole wood, cellulose and lignin of oak tree-rings, Palaeogeogr. Palaeoclimatol. Palaeoecol., 196, 395–407, https://doi.org/10.1016/S0031-0182(03)00466-8, 2003.
Loader, N. J., Young, G. H., McCarroll, D., and Wilson, R. J.: Quantifying uncertainty in isotope dendroclimatology, The Holocene, 23, 1221–1226, https://doi.org/10.1177/0959683613486945, 2013.
Loader, N. J., Street-Perrott, F. A., Daley, T. J., Hughes, P. D. M., Kimak, A., Levanič, T., Mallon, G., Mauquoy, D., Robertson, I., Roland, T. P., van Bellen, S., Ziehmer, M. M., and Leuenberger, M.: Simultaneous Determination of Stable Carbon, Oxygen, and Hydrogen Isotopes in Cellulose, Anal. Chem., 87, 376–380, https://doi.org/10.1021/ac502557x, 2015.
Mann, M. E., Bradley, R. S., and Hughes, M. K.: Global-scale temperature patterns and climate forcing over the past six centuries, Nature, 392, 779–787, https://doi.org/10.1038/nature02478, 1998.
McCarroll, D. and Loader, N. J.: Stable isotopes in tree rings, in Quaternary Sci. Rev., 23, 771–801, Elsevier, 2004.
Nicolussi, K. and Patzelt, G.: Discovery of early-Holocene wood and peat on the forefield of the Pasterze Glacier, Eastern Alps, Austria, The Holocene, 10, 191–199, https://doi.org/10.1191/095968300666855842, 2000a.
Nicolussi, K. and Patzelt, G.: Untersuchungen zur holozänen Gletscherentwicklung von Pasterze und Gepatschferner (Ostalpen), Zeitschrift für Gletscherkd. und Glazialgeol., 36, 1–87, 2000b.
Nicolussi, K. and Schlüchter, C.: The 8.2 ka event-Calendar-dated glacier response in the Alps, Geology, 40, 819–822, https://doi.org/10.1130/G32406.1, 2012.
Nicolussi, K., Kaufmann, M., Patzelt, G., Plicht Van Der, J., and Thurner, A.: Holocene tree-line variability in the Kauner Valley, Central Eastern Alps, indicated by dendrochronological analysis of living trees and subfossil logs, Veg. Hist. Archaeobot., 14, 221–234, https://doi.org/10.1007/s00334-005-0013-y, 2005.
Nicolussi, K., Kaufmann, M., Melvin, T. M., van der Plicht, J., Schiessling, P., and Thurner, A.: A 9,111 year long conifer tree-ring chronology for the European Alps: a base for environmental and climatic investigations, The Holocene, 19, 909–920, https://doi.org/10.1177/0959683609336565, 2009.
Rebetez, M. and Reinhard, M.: Monthly air temperature trends in Switzerland 1901–2000 and 1975–2004, Theor. Appl. Climatol., 91, 27–34, https://doi.org/10.1007/s00704-007-0296-2, 2008.
Rinn, F.: Tsap V 3.6 Reference manual: computer program for tree-ring analysis and presentation, Rinntech, Heidelb., 1996.
Schleser, G. H., Frielingsdorf, J., and Blair, A.: Carbon isotope behaviour in wood and cellulose during artificial aging, Chem. Geol., 158, 121–130, https://doi.org/10.1016/S0009-2541(99)00024-8, 1999.
Tranquilini, W.: Physiological Ecology of the Alpine Timberline, Ecol. Stu., Vol. 31, Springer-Verlag, Berlin, 1979.
Treydte, K., Frank, D., Esper, J., Andreu, L., Bednarz, Z., Berninger, F., Boettger, T., D'Alessandro, C. M., Etien, N., Filot, M., Grabner, M., Guillemin, M. T., Gutierrez, E., Haupt, M., Helle, G., Hilasvuori, E., Jungner, H., Kalela-Brundin, M., Krapiec, M., Leuenberger, M., Loader, N. J., Masson-Delmotte, V., Pazdur, A., Pawelczyk, S., Pierre, M., Planells, O., Pukiene, R., Reynolds-Henne, C. E., Rinne, K. T., Saracino, A., Saurer, M., Sonninen, E., Stievenard, M., Switsur, V. R., Szczepanek, M., Szychowska-Krapiec, E., Todaro, L., Waterhouse, J. S., Weigl, M., and Schleser, G. H.: Signal strength and climate calibration of a European tree-ring isotope network, Geophys. Res. Lett., 34, L24302, https://doi.org/10.1029/2007GL031106, 2007.
Treydte, K. S., Schleser, G. H., Helle, G., Frank, D. C., Winiger, M., Haug, G. H., and Esper, J.: The twentieth century was the wettest period in northern Pakistan over the past millennium, Nature, 440, 1179–1182, https://doi.org/10.1038/nature04743, 2006.
Trouet, V., Esper, J., Graham, N. E., Baker, A., Scourse, J. D., and Frank, D. C.: Persistent positive North Atlantic oscillation mode dominated the Medieval Climate Anomaly, Science, 324, 78–80, https://doi.org/10.1126/science.1166349, 2009.
Tukey, J. W.: Exploratory Data Analysis, Addison-Wesley Publishing Company, 1977.
Wanner, H., Solomina, O., Grosjean, M., Ritz, S. P., and Jetel, M.: Structure and origin of Holocene cold events, Quaternary Sci. Rev., 30, 3109–3123, https://doi.org/10.1016/j.quascirev.2011.07.010, 2011.
Wanner, H., Mercolli, L., Grosjean, M., and Ritz, S. P.: Holocene climate variability and change; a data-based review, J. Geol. Soc. London., 172, 254–263, https://doi.org/10.1144/jgs2013-101, 2015.
Ziehmer, M. M., Nicolussi, K., Schlüchter, C., and Leuenberger, M.: Tree-species and site sensitivity of two high-Alpine tree species in the Swiss Alps, in preparation, 2018.