the Creative Commons Attribution 4.0 License.
the Creative Commons Attribution 4.0 License.
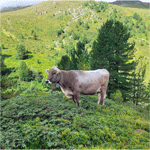
Human and livestock faecal biomarkers at the prehistorical encampment site of Ullafelsen in the Fotsch Valley, Stubai Alps, Austria – potential and limitations
Marcel Lerch
Tobias Bromm
Clemens Geitner
Jean Nicolas Haas
Dieter Schäfer
Bruno Glaser
Michael Zech
The Ullafelsen at 1869 m above sea level (a.s.l.) in the Tyrolean Stubai Alps next to Innsbruck is an important (geo)archeological reference site for the Mesolithic period. Buried fireplaces on the Ullafelsen plateau were dated at 10.9 to 9.5 ka cal BP and demonstrate together with thousands of flint stone artifacts the presence of hunter-gatherers during the Early Holocene. Grazing livestock has been a predominant anthropozoological impact in the Fotsch Valley presumably since the Bronze Age (4.2–2.8 ka). In order to study the human and/or livestock faeces input on the Ullafelsen, we carried out steroid analyses on 2 modern ruminant faeces samples from cattle and sheep, 37 soil samples from seven archeological soil profiles, and 9 soil samples from five non-archeological soil profiles from the Fotsch Valley used as reference sites. The dominance of 5β-stigmastanol and deoxycholic acid in modern cattle and sheep faeces can be used as markers for the input of ruminant faeces in soils. The OAh horizons, which have accumulated and developed since the Mesolithic, revealed high contents of steroids (sterols, stanols, stanones and bile acids); the eluvial light layer (E (LL)) horizon coinciding with the Mesolithic living floor is characterized by medium contents of steroids. By contrast, the subsoil horizons Bh, Bs and BvCv contain low contents of faecal biomarkers, indicating that leaching of steroids into the podsolic subsoils is not an important factor. High content of 5β-stigmastanol and deoxycholic acid in all soil samples gives evidence for faeces input of ruminants. The steroid patterns and ratios indicate a negligible input of human faeces on the Ullafelsen. In conclusion, our results reflect a strong faeces input by livestock, rather than by humans as found for other Anthrosols such as Amazonian dark earths. Further studies need to focus on the question of the exact timing of faeces deposition.
- Article
(7921 KB) - Full-text XML
-
Supplement
(90 KB) - BibTeX
- EndNote
Archeological research in high mountain regions has received increasing attention during the last few decades. Based on the finding of the Copper Age mummy called Ötzi at the Tisenjoch in the Ötztal Alps in 1991, archeological research projects were also launched in the Central Alps (Schäfer, 2011b). Mesolithic hunter-gatherers lived in the Alpine regions from the beginning of the Holocene (Fontana et al., 2016). For instance, Schäfer et al. (2016) and Cornelissen and Reitmaier (2016) provided evidence for the presence of Mesolithic people at the upper subalpine or alpine zones in the central and southeastern Swiss Alps.
Concerning the Tyrolean Alps, the Mesolithic site of Ullafelsen (1869 m above sea level (a.s.l.)) in the Fotsch Valley was discovered by the archeologist Dieter Schäfer in 1994 and became an important archeological reference site (Schäfer, 2011a, b) (Figs. 1 and 2). At this site, thousands of archeological artifacts and many buried fireplaces were found. This provides clear evidence for the presence and the human–environment interaction of our ancestors (Schäfer, 2011a, b). Previous archeological research demonstrates that the Ullafelsen and its surroundings were used as a summer camp for hunting by Mesolithic hunter-gatherers during the Preboreal and Boreal from around 10.9 to 9.5 ka (Schäfer, 2011a). The soils on the Ullafelsen are strongly influenced by this Mesolithic impact, and additionally since the Bronze Age alpine pastoralism has changed the vegetation of the Fotsch Valley dramatically (Schäfer, 2011a; Zech et al., 2021).
From a pedological point of view, a striking and frequently occurring light layer (LL) below the topsoils was described for the Ullafelsen and was a focus of previous investigations (Geitner and Schäfer, 2010; Geitner et al., 2011, 2014). Similar light horizons are typical of soils developed in the subalpine zone of the Central Alps and are usually interpreted as eluvial (E) horizons of Podzols (Zech and Wilke, 1977; Egli et al., 2008) or as eventual loess deposit (Geitner et al., 2011; Schäfer, 2011a). At the Ullafelsen, most artifacts and Mesolithic fireplaces were found within and directly on the top of the E (LL) horizon (Schäfer, 2011a). Therefore, the E (LL) horizon is regarded as the Mesolithic living floor and the humic-rich subsoil below the E (LL) horizon was considered late glacial buried former topsoil (2Ahb horizon) (Geitner et al., 2011; Schäfer, 2011a).
Most recently, Zech et al. (2021) demonstrated the great potential of n-alkane and black carbon biomarkers for contributing to a better understanding of pedogenesis and landscape evolution. Black carbon results based on benzene polycarboxylic acid (BPCA) analyses corroborated fire-induced human impact on the E (LL) horizon. The absence of leaf-wax-derived n-alkane biomarkers in the subsoils together with the absence of late glacial radiocarbon ages challenges the existence of a late glacial buried topsoil (2Ahb horizon) and rather points to a humus-enriched podzolic Bh horizon (Zech et al., 2021).
In the context of alpine pastoralism (grazing, dairying) from the Neolithic period in different parts of the Alps, (cattle) husbandry and agriculture became increasingly important for human society (Reitmaier et al., 2018; Gilck and Poschlod, 2019). Grazing livestock such as cattle and sheep have been a predominant anthropozoological impact for the Ullafelsen and its surroundings presumably since the Bronze Age (4.2–2.8 ka) as suggested by previous analyses (Zech et al., 2021).
Faecal biomarker analyses have become an attractive tool in paleoenvironmental and archeological research during the last few decades (Baeten et al., 2012; Glaser and Birk, 2012; Prost et al., 2017). The respective molecules are considered diagnostic markers for detecting ancient faecal inputs in soils (Bull et al., 1999b), whereby steroids (Δ5-sterols, 5α-stanols, 5β-stanols, epi-5β-stanols, stanones and bile acids) are the relevant compound classes (Bull et al., 2005). These provide insights into ancient agricultural practices and the former presence of animals or humans (Prost et al., 2017).
Previous studies prove the specific steroid signal for various animals (Bull et al., 2005; Birk et al., 2011; Prost et al., 2017; Haurrault et al., 2019). Accordingly, faecal biomarker analyses allow us to distinguish between faeces of herbivores and/or ruminants, pigs, and humans. Based on their specific steroid signal, the origin of faeces input can be detected in soils and sediments (von der Lühe et al., 2013; Haurrault et al., 2019). However, plants also show a specific steroid signal, which has to be considered during interpretations (Evershed et al., 1997; Hartmann, 1998). A finer differentiation between faeces of different livestock can be achieved by the combination of several steroids (Δ5-sterols, 5α-stanols, 5β-stanols, epi-5β-stanols, stanones and bile acids) (Prost et al., 2017).
Faecal steroids such as organic compounds/lipid molecules can accumulate and persist in sediments and soils for more than thousands of years (Bull et al., 2001; Schroeter et al., 2020). These have a low water solubility and are thus usually neither leached into deeper soil horizons (Bull et al., 2002) nor detectable in soil leachates (Lloyd et al., 2012). It remains to be investigated whether this also holds true for very low soil pH values < 4 like in our study area, where organic substances can become mobile via complexation with metal ions.
The 5β-stanols coprostanol and 5β-stigmastanol are products of anaerobic microbial reduction of Δ5-sterols. In mammals, this reduction is performed by gut bacteria and results in different ratios of 5β-stanols depending on food intake (Bull et al., 1999a). The Δ5-sterol cholesterol is the precursor molecule of coprostanol, while the Δ5-sterols β-sitosterol and stigmasterol are the precursor molecules of 5β-stigmastanol and epi-5β-stigmastanol (Schroeter et al., 2020). Stanones are intermediate products of the transformation of Δ5-sterols to 5β-stanols, epi-5β-stanols and 5α-stanols, occurring in the guts of animals and also in the environment (Prost et al., 2017). Epimerization of 5β-stanols, which occurs in soils due to microbial and diagenetic transformation, has to be considered when applying steroid ratios (Bull et al., 1999a; von der Lühe et al., 2018).
Up to now, analyses of livestock faeces show 5β-stigmastanol and deoxycholic acid (DCA) as the dominant steroid compounds for ruminants (cattle and sheep), whereas coprostanol is a faecal marker for omnivores such as humans and pigs (Glaser and Birk, 2012; Prost et al., 2017; Haurrault et al., 2019). In contrast, plants contain high amounts of β-sitosterol and stigmasterol (Δ5-sterols) in roots and litter (Piironen et al., 2000; Verma and Gupta, 2013).
Bile acids are formed from cholesterol in the liver and transformed via the bile into the gut of mammals as primary bile acids (e.g., cholic acid (CA) or chenodeoxycholic acid (CDCA)). These primary bile acids are further mediated to form secondary bile acids (e.g., lithocholic acid (LCA) or deoxycholic acid (DCA)) in the intestine by microorganisms (Bull et al., 2002; Kuhajda et al., 2006; Prost et al., 2017). Human faeces show high abundance of LCA, whereas ruminant faeces have a dominance of DCA (Prost et al., 2017; Shillito et al., 2020). Hence, steroid compounds can be useful markers for reconstructing the settlement history of a site based on past faecal inputs.
Faecal biomarkers are currently used in various scientific disciplines all over the world. Glaser et al. (2001), Glaser and Birk (2012), and Wiedner et al. (2015) investigated anthropogenic dark earths, also known as terra preta de índio, in central Amazonia. By applying steroid markers, they provided evidence for settlement activities in this part of the tropical rainforest. High nutrient contents induced by the deposition of human and livestock faeces clearly demonstrated the anthropogenic origin of terra preta de índio (Birk et al., 2011, 2012; Glaser and Birk, 2012). Another study used steroids for identification of temporary mass graves of concentration camp prisoners at the end of World War II (von der Lühe et al., 2020). Findings revealed elevated faecal steroid contents and thus corroborate the former input of human decomposition products as well as faecal and tissue constituents of buried bodies (von der Lühe et al., 2020).
The aim of our geoarcheological study presented here was to contribute to a better understanding of human and livestock impact at the prehistorical encampment site of Ullafelsen with the use of faecal biomarkers. More specifically, the following questions are addressed: (i) do faecal biomarker patterns of modern ruminant faeces around the Ullafelsen reflect the steroid patterns reported in the literature and is a clear distinction from human faecal biomarker patterns possible? (ii) Do the steroid contents and patterns of the soil profiles at the Ullafelsen allow discrimination between human and livestock faeces input? (iii) Do the faecal steroid ratios of the soil profiles on the Ullafelsen allow the reconstruction of the faecal input history during the Holocene? We hypothesize that human faeces input is detectable in the E horizon representing the Mesolithic living floor (LL), whereas livestock faeces input dominates in the overlaying OAh horizon. (iv) Is there any evidence for leaching of steroid biomarkers in soils with very low pH values such as in our study area?
2.1 Study area – the Ullafelsen as a prehistorical encampment site in the Fotsch Valley, Stubai Alps, Austria
The prehistorical encampment site of Ullafelsen, also called Riegelschrofen, is located in the Fotsch Valley at an altitude of 1869 m a.s.l. The 13 km long Fotsch Valley belongs to the Stubai Alps southwest of Innsbruck, the capital of the Austrian state Tyrol. The Ullafelsen is a round hump at the eastern site of the Fotsch Valley and is located in the subalpine vegetation zone (Fig. 1). This rock ledge lies 40 m above the level of the adjacent creek, called Fotscherbach (Schäfer, 2011a). The geographic coordinates of the archeological excavation area at the Ullafelsen are 47.14702∘ N, 11.21475∘ E (WGS84).
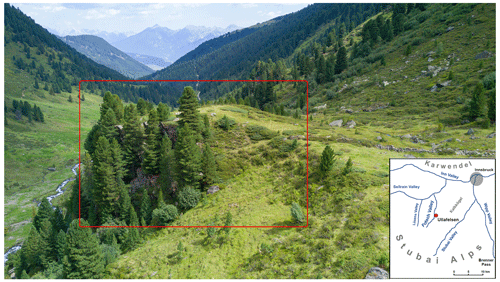
Figure 1The prehistorical encampment site of Ullafelsen in the Fotsch Valley, Stubai Alps, southwest of Innsbruck, Tyrol, Austria. Northward view from the inner Fotsch Valley over the Ullafelsen (1869 m a.s.l.) to the Karwendel mountain range in the Northern Limestone Alps (photo: Eike Hüsing, 2018).
As a part of the transition zone between the wetter Northern Alps and the drier Central Alps, our study area is characterized by a temperate climate with a mean annual temperature of 10 ∘C in the summertime (July) and −3 ∘C in the wintertime (January). The mean annual precipitation is approx. 1500 mm (Schäfer, 2011a; Schlosser, 2011).
The vegetation is predominated by Swiss stone pine (Pinus cembra) and Juniper (Juniperus communis ssp. alpina). Furthermore, there are scattered European larch (Larix decidua), Norway spruce (Picea abies), green alder (Alnus viridis) and birch (Betula pendula). Alpine rose (Rhododendron ferrugineum), lingonberry (Vaccinium vitis-idaea), European blueberry (Vaccinium myrtillus) and ling heather (Calluna vulgaris) occur as alpine dwarf shrubs. The vegetation cover also consists of several herbs and grasses (Kemmer, 2011; Zech et al., 2021).
From a geological point of view, the Fotsch Valley represents a part of the “Öztal–Stubai–crystalline complex”. Typical rocks for this study area are the metamorphic rocks mica slate and paragneiss. In addition, there also exist a variety of unconsolidated quaternary sediments (Nittel, 2011). The basic material under the anthropogenically influenced soils at the Ullafelsen consists, among others, of weathered till (Nittel, 2011). Despite human influence, these soils were mainly formed by podsolization during the Holocene (Zech et al., 2021). Typical soils in the alpine and subalpine zone of the Fotsch Valley are Cambisols and Leptosols. Under alpine dwarf shrub vegetation, Podzols have frequently developed, whereas in flatter valley floors and on some slope positions Histosols can also be found (Geitner et al., 2014).
2.2 Sampling of modern faeces and archeological and reference soil profiles
As part of fieldwork in July and August 2017 and 2018, we collected 37 soil samples from seven profile walls of archeological soil profiles on the Ullafelsen, 9 soil samples from five non-archeological soil profiles from the Fotsch Valley as reference sites, and 2 faeces samples from cattle and sheep (Table 1). Four profile walls are directly from the archeological excavation area (1.1 C4w, 1.1 B5s, 1.1 B5w, 1.1 G5n). Three profile walls are from a nearby trench (1.9 NW, 1.9 NE, 1.9 SW) (Fig. 2). The latter is located 2 m below the archeological excavation area at an altitude of approximately 1867 m a.s.l. Samples of reference sites were collected from the soil profiles 4.4, 4.11, 4.12, 5.5 and 5.6w, which are primarily located along pathways and river sections on hillsides above 2000 m a.s.l. in the Fotsch Valley (Table 1). Sampling was conducted by soil horizons, which were classified according to the World Reference Base for Soil Resources (IUSS Working Group WRB, 2015).
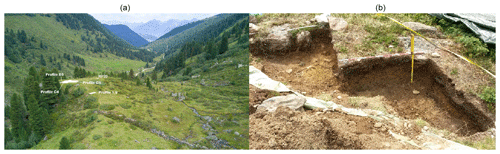
Figure 2(a) NNW view over the Ullafelsen in the Fotsch Valley, Stubai Alps, Austria, at the upper timberline. The geoarcheological excavation area on the Ullafelsen plateau with the reopened and sampled soil profiles 1.1 B5, 1.1 C4 and 1.1 G5 and the newly opened and sampled soil profile 1.9 several meters towards the southeast are shown. (b) Reopened archeological excavation area. View to the southwestern part of the sampled soil profiles (Zech et al., 2021).
Samples of cattle faeces were collected on the Ullafelsen, while samples of sheep faeces were collected from the Fotsch Valley above 2000 m a.s.l. We chose these sampling sites for collecting faeces samples because of a high density of grazing livestock mainly consisting of sheep. The food of grazing livestock from the Ullafelsen and its surroundings consists of local vegetation, mostly grasses and alpine dwarf shrubs. After drying and grinding our faeces samples, we stored these separately in snap cap vials.
Table 1Overview of all soil profiles, soil and modern faeces samples from the prehistorical encampment site of Ullafelsen and reference sites in the Fotsch Valley, Stubai Alps, Austria. Analytical results of TOC, TN and TOC N are presented.
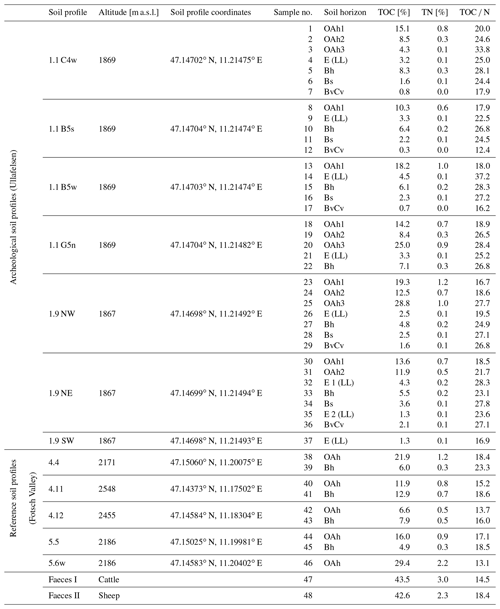
For data evaluation, soil samples from soil profiles on the Ullafelsen were sorted by horizons (n=37): OAh1 (n=6), OAh2 (n=4), OAh3 (n=3), E (LL) (n=8), Bh (n=6), Bs (n=5) and BvCv (n=5). The samples investigated here from reference soil profiles were from the OAh (n=5) and Bh (n=4) horizons (Table 1). Zech et al. (2021) carried out total organic carbon (TOC) and TOC N analyses for all these 46 soil samples. Figure 3 illustrates the soil profile 1.9 NW with the horizons OAh1–OAh2–OAh3–E (LL)–Bh–Bs–BvCv. All other investigated soil profiles have a similar sequence of horizons. Results of grain size analyses for the soil profiles at the Ullafelsen show a dominance of sand (Geitner et al., 2011). In comparison to the other soil horizons, the E (LL) horizon is characterized by remarkably higher amounts of silt (Geitner et al., 2011).
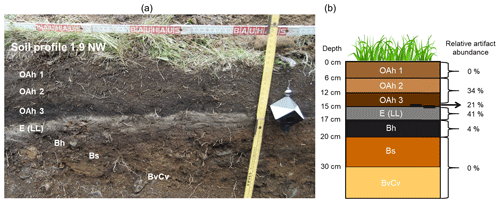
Figure 3(a) Soil profile 1.9 NW, which represents a typical soil profile of the Ullafelsen in the Fotsch Valley, Stubai Alps, Austria. (b) Schematic horizons of the soil profiles on the Ullafelsen. Note that the soil profiles reveal a high heterogeneity. Nevertheless, the soil horizons OAh3 and Bh are characterized by a humus enrichment. The E (LL) horizon (light layer) reveals the highest relative artifact abundance (∼ 41 %) and is overlain by several fireplaces on the Ullafelsen. This horizon is considered the living floor of the Mesolithic hunter-gatherers (Geitner et al., 2011, 2014). Due to TOC content partly ≥ 15 %, we adopted the soil horizon classification by Zech et al. (2021).
TOC values of soil samples from archeological soil profiles on the Ullafelsen ranged from 0.3 % to 28.8 % (Table 1). The maximum TOC content was observed in the OAh3 horizon, being in accordance with a darker color and higher density of charcoal particles. Total nitrogen contents of the investigated soil profiles ranged from 0.0 % to 1.2 %. The TOC N ratios ranged from 12.4 to 37.2 with the highest ratios in the OAh3 horizon coinciding with charcoal particles in this soil horizon, related to former fireplaces, and high amounts of other organic material (Zech et al., 2021). High TOC N ratios in the Bh horizons reflect podsolization processes (Glaser and Birk, 2012; Zech et al., 2014). TOC and TN values of soil samples from reference soil profiles from the Fotsch Valley ranged from 4.9 % to 29.4 % and 0.3 % to 2.2 %, respectively. The highest TOC and TN values were measured in the OAh horizons (Table 1).
Radiocarbon-dated Mesolithic charcoal yielded 14C ages ranging from 10.9 to 9.5 kyr cal BP (Schäfer, 2011a). More recently, Zech et al. (2021) yielded 14C ages for bulk n-alkanes ranging from 8.2 to 4.9 kyr cal BP. This discrepancy suggests that an n-alkane producing vegetation cover, consisting of herbs, grasses and alpine dwarf shrubs, did not predominate immediately after the Mesolithic abandonment. Rather, it must be assumed that non-n-alkane-producing conifers, such as Pinus cembra or Picea abies, predominated the vegetation cover after the Mesolithic life on the Ullafelsen (Zech et al., 2021).
In addition to the 37 soil samples at the archeological site on the Ullafelsen and the 9 soil samples from reference soil profiles from the Fotsch Valley, we analyzed 2 mixed faeces samples from cattle and sheep, which belong to the typical livestock at the Ullafelsen and its surroundings. TOC contents of cattle and sheep ranged from 42.6 % to 43.5 % (Table 1).
2.3 Faecal biomarker analyses
Firstly, all 46 soil and both faeces samples were air-dried, sieved (≤ 2 mm) and finely ground. Using an elemental analyzer coupled to an isotope ratio mass spectrometry (EA-IRMS) instrument, the total carbon (TC) and total nitrogen (TN) of soil samples were determined by Zech et al. (2021). Due to the non-carbonate parent rock material as well as the low pH values (< 4 in CaCl2) of the soils and sediments on the Ullafelsen (Geitner et al., 2011), the measured TC values can be considered TOC values. From these data, the TOC N ratio was calculated.
Steroid (sterol, stanol, stanone and bile acid) analyses were performed according to Birk et al. (2012), Wiedner et al. (2015) and von der Lühe et al. (2020). The weight of sample depended on the TOC content of the sample and ranged for the 46 analyzed soil samples from 1.0 to 5.0 g of finely ground material. For faeces, it is necessary to use a much smaller weight due to the higher TOC contents. In the case of our two faeces samples (cattle and sheep), the weight of sample taken was ∼ 60 mg. Prior to extraction, 100 µg of α-preganol and 100 µg of iso-deoxycholic acid were added to each sample as the recovery standard (internal standard 1, IS1) for sterol stanol and bile acid fractions, respectively. We used no internal standard for the stanone fractions. Regarding data analysis and interpretation, we consider no stanones in our calculated ratios. The total lipid extract (TLE) was obtained by soxhlet extraction for 36 h using a solvent mixture of dichloromethane methanol (2:1, , 180 mL).
After extraction, the solvent was removed using a rotary evaporator, and the TLEs were saponified using 3.5 mL of 0.7 M KOH in methanol at room temperature overnight for approx. 12 h. Neutral lipids (sterols, stanols, stanones) as well as acidic lipids (bile acids, fatty acids) were separated by sequential liquid–liquid extraction. To obtain the neutral lipid fraction, the extracts were spiked with 10 mL of H2O and afterwards separated from the aqueous phase with 3 × 15 mL of chloroform. For gaining the acidic lipid fraction, the aqueous solution was acidified with 6 M HCl (pH ≤ 3–4) and the bile acids were extracted with 3 × 15 mL chloroform. Both fractions were separately collected in flasks and evaporated under nitrogen.
Before purification by solid-phase extraction (SPE), acidic fractions were methylated by adding 1 mL of 1.25 M HCl in methanol and heating at 80 ∘C for 2 h. Bile acids and fatty acid methyl esters were extracted with 1 mL of H2O and 3 × 1 mL of n-hexane. SPE was performed using glass columns (∅ 11 mm) containing 1.5 cm activated silica gel (mesh 70–230, pore size 100 Å, type Merck) in n-hexane. After preconditioning with 5 mL of dichloromethane n-hexane (2:1, ), the extracts were transferred with dichloromethane n-hexane (2:1, ) on the silica column. After elution of the fatty acid methyl esters to waste with 5 mL of dichloromethane n-hexane (2:1, ), bile acid methyl esters were eluted with 5 mL of dichloromethane methanol (2:1, ) and collected in reactivials. The dried bile acid methyl esters were redissolved in 50 µL of toluene and silylated with 98 µL of BSTFA (N,O-bis-(trimethylsilyl)-trifluoracetamid; puriss; Sigma-Aldrich) and 2 µL of TSIM (1-(trimethylsilyl)imidazol; puriss; Sigma-Aldrich) at 80 ∘C for 1 h. After cooling, 50 µL of 5α-cholestane (10 ng µL−1 in toluene) was added as internal standard 2 (IS2). All substances were transferred into GC vials afterwards.
For the SPE of the neutral lipid fractions, activated silica gel (mesh 70–230, pore size 100 Å, type Merck) was deactivated with 5 % H2O. The neutral lipid extract was transferred with n-hexane to the SPE glass columns (∅ 11 mm) containing 1.5 cm of deactivated silica gel. Preconditioning was carried out with 5 mL of n-hexane. By adding 5 mL of n-hexane, aromatic and aliphatic fractions were eluted but not used for further analyses. The sterol-, stanol- and stanone-containing fraction was eluted with 3 mL of dichloromethane and 2 mL of dichloromethane acetone (2:1, ). The eluates were collected in reactivials before drying under nitrogen (N2). Subsequently, the fractions of sterols, stanols and stanones were silylated by adding 100 µL of Sylon HTP (a mixture of HMDS + TMCS + pyridine (, ); puriss; Supelco) and derivatized at 70 ∘C for 1 h. The eluates were dried with N2 after cooling. A total of 50 µL of 5α-cholestane (10 ng µL−1 in toluene) as internal standard 2 (IS2) and 100 µL of toluene were added to the dried eluates and transferred into GC vials.
For external calibration, six concentrations from a stock solution (10 ng µL−1 in hexane) containing all target sterols, stanols and stanones as well as the recovery standard (IS1) were prepared ranging from 50 to 2500 ng per vial. After derivatization 500 ng of 5α-cholestane (dissolved in dry toluene, 50 µL, 10 ng µL−1) was added as internal standard 2 (IS2). Peak areas of the analytes were divided by the peak area of IS2, and calibration curves for each substance were calculated using these ratios. Calibration curves for the bile acids were prepared accordingly. All calibration curves had a coefficient of determination (R2) > 0.98. The limit of detection was defined as the signal-to-noise ratio of 3:1 and varied for the analytes between 0.6 ng per gram of soil for all Δ5-sterols, stanols and stanones and 4 ng per gram of soil for all bile acids. Finally, recoveries of the first internal standard (IS1) were calculated for all samples. Recovery of IS1 of all soil samples ranged between 51 %–116 % for sterols, stanols and stanones and 50 %–122% for bile acids. All results for the samples were corrected for the losses during extraction and purification with those individually calculated recoveries.
Quantification of all steroid substances took place using gas chromatography mass spectrometry (GC-MS) with a 5971A quadrupole mass spectrometer connected to a HP 5090 gas chromatograph (both made by Hewlett-Packard) with a DB-5 MS 30 m fused silica column (25 mm i.d. and 0.25 µm film thickness, Agilent Technologies).
All measurements of steroids were conducted with the following settings for GC-MS: injection volume, 1 µL; carrier gas, helium (purity of 5); injector temperature, 290 ∘C, injection in splitless mode; interface temperature, isotherm at 280 ∘C. The column temperature program of the gas chromatography was held at 80 ∘C for 1.5 min and then raised at 12 ∘C min−1 to 265 ∘C. Further steps are increases in the temperature at 0.8 ∘C min−1 to 288 ∘C and at 10 ∘C min−1 to 300 ∘C afterwards, whereas it was kept for 12 min.
2.4 Data analysis
For a detailed interpretation of the measured results in terms of degradation effects and distinguishing between omnivore-, herbivore- or plant-derived faecal biomarkers, the following ratios were calculated and plotted as boxplot diagrams:
According to Bull et al. (1999a) and Schroeter et al. (2020), we used ratio 1 to estimate microbial degradation of steroids (5β-stanols, epi-5β-stanols and 5α-stanols) and to identify human faeces input (Wiedner et al., 2015). It is known that soil microorganisms contribute to degradation of steroids in soils (Bull et al., 2001); 5α-cholestanol is a degradation product of the Δ5-sterol precursor cholesterol, transformed by soil microorganisms, and occurs naturally in the environment (Prost et al., 2017). This ratio considers the input and preservation of stanols. High values for ratio 1 (0.7–1) indicate increased faeces deposition, while values < 0.7 indicate low faeces input in soils (Bull et al., 1999a; Schroeter et al., 2020).
For distinguishing between human and herbivore faeces, ratio 2 can be applied. A dominant 5β-stanol marker in omnivore faeces such as humans or pigs is coprostanol, whereas 5β-stigmastanol is a typical 5β-stanol marker for herbivore faeces. To account for ongoing epimerization of 5β-stanols in soils, both epimers are included in this ratio. Major input of human faeces results in values > 1, whereas values < 1 indicate an input of herbivore faeces (Ossendorf et al., 2019).
Ratio 3 was calculated for differentiation between plant-derived steroids and livestock-derived steroids. According to Prost et al. (2017), β-sitosterol belongs to the typical Δ5-sterols, which are characteristic of plant biomass and thus normally occur at high abundance in soils. Values between 0 and 0.5 suggest low input of β-sitosterol, and values between 0.5 and 1 point at a high occurrence of this plant Δ5-sterol. It has to be considered that faeces of ruminants can also contain high amounts of β-sitosterol due to the ruminants' plant-dominated diet (Haurrault et al., 2019; Schroeter et al., 2020).
Ratio 4 was calculated for all soil samples and can be used as proxy for degradation of 5β-stigmastanol. Epi-5β-stigmastanol is a transformation product (epimer) of 5β-stigmastanol and is often found in anaerobic environments and soils (von der Lühe et al., 2018). With increasing degradation, ratio 4 becomes lower due to the higher proportion of epi-5β-stigmastanol.
The following equation was applied for calculating a bile acid ratio:
Based on the bile acids DCA and LCA, ratio 5 can be used for distinguishing ruminant from human faeces. Prost et al. (2017) published reference values, which are characteristic of ruminant species and humans, respectively. Human faeces contain high amounts not only of coprostanol but also of LCA. In contrast, ruminant faeces show a dominance of DCA (Shillito et al., 2020). A small ratio 5 (3–5) indicates a dominance of human faeces, whereas high values (5–21) show a dominance of ruminant faeces such as cattle or sheep (Prost et al., 2017).
3.1 Biomarker patterns of modern livestock faeces
The total sterol, stanol and stanone contents of modern cattle and sheep faeces ranged from 2401.6 to 2671.6 µg g−1 and 2109.8 to 2421.9 µg g−1, respectively (Table S3). The total bile acid contents of modern cattle and sheep faeces ranged from 55.9 to 86.8 µg g−1 and 78.6 to 216.9 µg g−1, respectively (Table S4).
For checking the reproducibility of our results, we repeated the analyses of our faeces samples twice (Tables S3, S4). Laboratory replicate analyses show that the method of faecal biomarker analyses works correctly and our results are reproducible. Figure 4 illustrates the steroid (sterols, stanols, stanones and bile acids) contents and their patterns in modern cattle and sheep faeces. The following results refer to measurement 1 (Tables S3, S4).
The predominant steroid in cattle faeces is 5β-stigmastanol (930.9 µg g−1), whereas epi-5β-stigmastanol (666.5 µg g−1) shows a predominance in sheep faeces (Fig. 4). For comparison, Prost et al. (2017) did not find epi-5β-stigmastanol to predominate in sheep faeces. It cannot be excluded that the predominance of epi-5β-stigmastanol in our sample is induced by strong epimerization, which is possibly influenced by long dry storage of the sample.
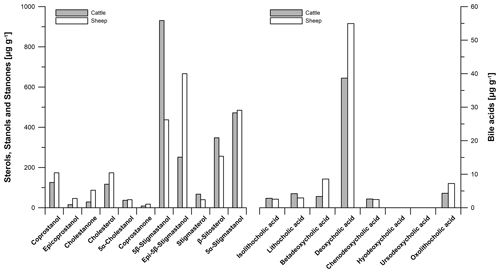
Figure 4Biomarker patterns of modern faeces from predominant livestock at the prehistorical encampment site of Ullafelsen in the Fotsch Valley, Stubai Alps, Austria, and it surroundings. Steroid (sterols, stanols, stanones and bile acids) contents of cattle and sheep faeces are given in micrograms per gram of dry matter.
The coprostanol content in modern cattle faeces is 125.7 µg g−1, whereas the coprostanol content of 173.4 µg g−1 was detected in modern sheep faeces. These low contents of human-related faecal biomarkers in our modern ruminant faeces samples have to be considered for the identification of faeces origin in our soil samples. The plant-derived steroid β-sitosterol shows 347.9 µg g−1 in modern cattle faeces and 255.9 µg g−1 in modern sheep faeces (Fig. 4).
We calculated ratios 1, 2, 3 and 5 for our modern cattle and sheep faeces regarding their steroid patterns to obtain reference ratios for comparing our analyzed soil samples. Bull et al. (1999a) and Prost et al. (2017) introduced ratio 1 for the identification of faeces input. A typical ratio 1 is ∼ 0.8 for cattle and sheep, whereas ∼ 1 is typical of human faeces. Our results revealed a ratio 1 of 0.8 for both modern faeces samples. These results are in agreement with Prost et al. (2017). Ratio 2 can be used as proxy for the identification of faeces origin. Ratios > 1 are characteristic of human or other omnivore faeces, whereas ratios < 1 represent herbivore and/or ruminant faeces. Our modern cattle and sheep faeces yielded ratio 2 values of 0.1 and 0.2, respectively. These results are also in agreement with data of Prost et al. (2017). Ratio 3 reflects the predominance of plant-derived steroids (β-sitosterol) over livestock-derived steroids (5β-stigmastanol and epi-5β-stigmastanol). Ratios ≤ 0.5 indicate dominance of β-sitosterol, whereas ratios ≥ 0.5 show dominance of 5β-stigmastanol and epi-5β-stigmastanol. Our modern faeces samples yielded a ratio 3 of ∼ 0.2 for cattle and sheep, which demonstrates a dominant content of livestock-derived steroids over plant-derived steroids in our ruminant faeces.
The most dominant bile acid in our modern ruminant faeces is DCA (38.7 and 55.0 µg g−1 in cattle and sheep faeces, respectively), in agreement with literature data of Kuhajda et al. (2006) and Prost et al. (2017). LCA as a marker for the input of human faeces was found in modern ruminant faeces only at low amounts (4.2 and 2.9 µg g−1 in cattle and sheep faeces, respectively (Fig. 4)). Unexpectedly, our results of modern cattle and sheep faeces show low contents of CDCA (2.6 and 2.4 µg g−1 in cattle and sheep faeces, respectively). Prost et al. (2017) did not detect CDCA in cattle and sheep faeces but in goat, horse, goose and human faeces instead.
According to Prost et al. (2017), ratio 5 ranged from 5–21 and 8–12 for cattle and sheep faeces, respectively. Based on our results, ratio 5 showed a ratio of 9.2 for cattle faeces and 18.8 for sheep faeces. For comparison, ratio 5 is typical of human faeces when in a range from 3 to 4.5. Accordingly, ratio 5 is highly promising for distinguishing between ruminant and human faeces in soils. Unfortunately, steroid patterns of our analyzed ruminant faeces allow no differentiation between cattle and sheep.
All three measurements yielded similar content for sterols, stanols and stanones. By contrast, measurement 1 showed higher contents of the bile acid DCA but lower contents of oxolithocholic acid (OLCA) for both modern faeces samples (Table S4). The latter acid can be synthesized by oxidation of the hydroxyl groups of DCA (Sakai et al., 1980; Kuhajda et al., 2006; Marion et al., 2019).
Based on the steroid patterns of our modern cattle and sheep faeces from the Ullafelsen and its surroundings, it is possible to evaluate the faecal biomarker results of our soil samples. Calculated steroid ratios (ratios 1, 2, 3 and 5) help us to identify the sources of dominant faeces input in soils.
3.2 Ancient faecal marker – contents and patterns in soils
The total sterol, stanol and stanone contents of the 37 soil samples from the Ullafelsen ranged from 1.2 to 198.1 µg g−1 (Fig. 5). The OAh1 and OAh3 horizons yielded maxima (12.2 to 198.1 µg g−1) coinciding with TOC maxima. The lowest content was measured in the Bh, Bs and BvCv horizons (1.2 to 22.3 µg g−1), whereas the content of the E (LL) horizon was intermediate (2.3 to 58.6 µg g−1; Fig. 5). In contrast, the sterol, stanol and stanone contents of the 9 soil samples from reference soil profiles from the Fotsch Valley ranged from 3.3 to 106.3 µg g−1. Maximum contents were measured in the OAh horizons (24.3 to 106.3 µg g−1), whereas Bh horizons yielded minimum contents (3.3 to 6.8 µg g−1) of sterols, stanols and stanones (Fig. 5).
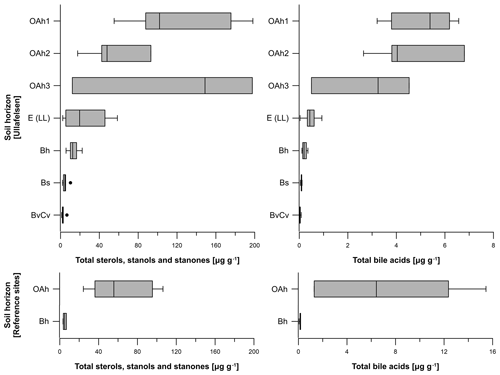
Figure 5Boxplots illustrating the total steroid (sterols, stanols, stanones and bile acids) contents (in micrograms per gram of dry matter) of the investigated soil profiles from the prehistorical encampment site of Ullafelsen and reference sites in the Fotsch Valley, Stubai Alps, Austria, categorized by the soil horizons OAh1 (n=6), OAh2 (n=4), OAh3 (n=3), E (LL) (n=8), Bh (n=6), Bs (n=5) and BvCv (n=5).
The total bile acid content of the 37 soil samples from the Ullafelsen ranged from 0 to 6.8 µg g−1 and shows its maximum in the topsoil horizons OAh1 and OAh2 (Fig. 5). In comparison to the maximum sterol, stanol and stanone contents in the OAh1 horizon, the maximum bile acid content of 6.8 µg g−1 was detected in the OAh2 horizon. Similarly to the sterol, stanol and stanone contents, the bile acid contents are much higher in the topsoil horizons OAh1, OAh2 and OAh3 (0.5 to 6.8 µg g−1) and lower in the subsoil horizons E (LL), Bh, Bs and BvCv (0 to 0.9 µg g−1) (Fig. 5). The total bile acid content of the 9 soil samples from reference soil profiles from the Fotsch Valley ranged from 0.1 to 15.5 µg g−1. The OAh horizons yielded maximum contents (1.3 to 15.5 µg g−1), whereas minimum contents of bile acids were measured in the Bh horizons (0.1 to 0.2 µg g−1; Fig. 5).
Steroids in the subsoils (Bh, Bs and BvCv horizon) can be induced by bioturbation and/or roots of plants (Piironen et al., 2000). The most detected steroid in the subsoils is β-sitosterol, which is a plant-derived Δ5-sterol. Thomas and Hale (1983) as well as Verma and Gupta (2013) found that roots or root exudates also contain a significant content of β-sitosterol. We assume that the small contents of steroids in the subsoil horizons of the archeological soils on the Ullafelsen and the reference soils in the Fotsch Valley are mainly caused by the influence of β-sitosterol due to the strong rooting of grasses and alpine dwarf shrubs in the soil matrix. There is no deformation of soil horizons; thus we exclude the influence of bioturbation.
The most abundant steroid in all soil samples from the Ullafelsen is β-sitosterol, with a maximum content of 150.1 µg g−1 measured in an OAh3 horizon (Fig. 6; Table S1). β-Sitosterol is a typical plant-derived Δ5-sterol compound in plant biomass and reflects the predominant vegetation signal (Holtvoeth et al., 2010; Prost et al., 2017; von der Lühe et al., 2018). Due to the plant diet, β-sitosterol is eaten by ruminants and can be detected in their faeces (Haurrault et al., 2019). Cholesterol as the dominating Δ5-sterol in animal tissues has a maximum content of 6.2 µg g−1, detected in an OAh1 horizon (Fig. 6; Table S1).
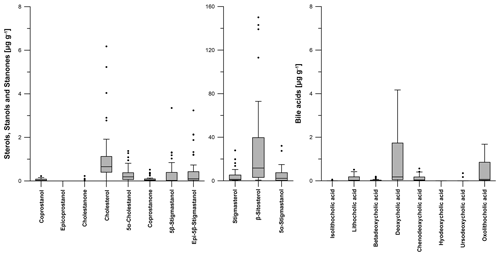
Figure 6Boxplots visualizing the content (in micrograms per gram of dry matter) of steroid (sterols, stanols, stanones and bile acids) patterns for all soil samples from the prehistorical encampment site of Ullafelsen in the Fotsch Valley, Stubai Alps, Austria. For a better overview, the steroids stigmastanol, β-sitosterol and 5α-stigmastanol were plotted on a separate y axis because of their high contents.
Due to microbial degradation in soils, 5α-stigmastanol and 5α-cholestanol (both are 5α-stanols) are partly produced from their Δ5-sterol precursors β-sitosterol and cholesterol, respectively (Björkhem and Gustafsson, 1971). These 5α-stanols also occur in small amounts in fresh plant material and animal tissue (Noda et al., 1988; Bull et al., 2002; Prost et al., 2017). The maximum content of 5α-stigmastanol is 32.1 µg g−1 in an OAh3 horizon, whereas 1.4 µg g−1 is the maximum content of 5α-cholestanol detected in an OAh1 horizon (Fig. 6; Table S1).
The 5β-stanol compound coprostanol as a marker for human faeces was detected at its highest content of 0.2 µg g−1 in an OAh1–OAh2 horizon. Epicoprostanol as a transformation product of coprostanol due to microbial degradation (Bull et al., 1999a; Lauer et al., 2014) was not detectable in our soils (Fig. 6; Table S1). As markers for ruminant faeces, 5β-stigmastanol and epi-5β-stigmastanol (epimerization product of 5β-stigmastanol) have their maximum contents of 3.3 and 3.2 µg g−1 in an OAh1 horizon, respectively (Fig. 6; Table S1).
The most abundant bile acid in the analyzed soil samples from the Ullafelsen was DCA with a maximum content of 4.2 µg g−1 in an OAh1–OAh2 horizon (Fig. 6; Table S1). It predominates in ruminants such as cattle and sheep (Kuhajda et al., 2006; Prost et al., 2017). LCA as the dominating bile acid in human faeces (Shillito et al., 2020) showed a maximum content of 0.5 µg g−1 in an OAh2 horizon (Fig. 6; Table S1). Wiedner et al. (2015) reported similar DCA and LCA contents for anthropogenic dark earths in northern Germany.
Furthermore, we found CDCA in the topsoil horizons OAh1, OAh2 and OAh3 as well as in the E (LL) horizon, with a maximum content of 0.6 µg g−1 detected in an OAh2 horizon. Results of our modern cattle and sheep faeces also showed low contents of CDCA. Therefore, we explain the detected CDCA in our soils by the input of ruminant faeces.
OLCA was also detected in our soil samples from the Ullafelsen and has a maximum content of 1.7 µg g−1 in an OAh1 horizon (Fig. 6; Table S1). As discussed in Sect. 3.1, ruminant faeces also containing OLCA at low abundance. However, according to Marion et al. (2019), we cannot exclude the possibility that OLCA is formed by microorganisms in soils, such as Clostridium scindens, due to transformation of DCA to OLCA.
The most abundant sterol in the reference soils was β-sitosterol, with a maximum content of 56.0 µg g−1 (Table S1). Deoxycholic acid is the dominant bile acid in the reference soils, with a maximum content of 10.0 µg g−1 (Table S2). Both steroids were detected in the OAh horizon of reference soil profile 5.6w. Maximum contents of 5β-stigmastanol and deoxycholic acid as livestock-derived steroids were also found in this reference soil profile.
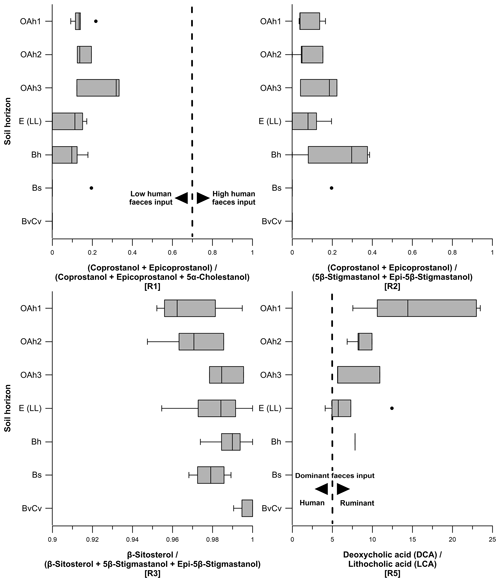
Figure 7Boxplots indicating steroid ratios for estimating the origin of faecal matter. Ratio 1 describes the input of human faeces in soils, whereas ratio 2 and ratio 5 determine the input of human vs. ruminant faeces. Thresholds for ratio 1: ratios < 0.7 assume low human faeces input, and ratios > 0.7 show high human faeces input. Thresholds for ratio 5: ratios < 5 point to a dominant human faeces input, and ratios > 5 represent a dominant ruminant faeces input. Ratio 3 considers the input of β-sitosterol to be a plant-derived steroid.
Overall, our results indicate that a strong input of faeces from cattle and sheep into the soils on the Ullafelsen occurred. By contrast, low LCA and coprostanol contribution point to a minor influence of human faeces. We suggest that the faeces input by wild animals such as chamois, marmot or fox can be neglected due to the high density of ruminant species at the Ullafelsen and its surroundings.
According to Lloyd et al. (2012), our steroid results of the archeological soils on the Ullafelsen and also of the reference soils from the Fotsch Valley show no leaching or transport in deeper soil horizons due to the significantly lower steroid contents.
In comparison to the reference soils, the higher contents of total sterols, stanols and stanones in the OAh horizons of the archeological soils on the Ullafelsen confirm an enrichment at this site. Unexpectedly, the total bile acid contents in the OAh horizons of the reference soils indicate a large range with partly higher contents compared to the archeological soils and thus show no increased bile acid contents at the Ullafelsen. The highest contents of livestock-derived steroids (5β-stigmastanol, epi-5β-stigmastanol, deoxycholic acid) of all reference soil profiles from the Fotsch Valley were detected in soil profile 5.6w. We explain these high contents by a strong input of sheep faeces into the soil of soil profile 5.6w.
3.3 Identification of faeces origin on the Ullafelsen based on specific steroid ratios
Ratio 1 ((coprostanol + epicoprostanol) (coprostanol + epicoprostanol + 5α-cholestanol)) ranged from 0 to 0.3 (Fig. 7). Human faeces normally exhibit a ratio 1>0.7 (Bull et al., 1999a; Prost et al., 2017). Therefore, our results corroborate a very low input of human faecal markers such as coprostanol and suggest a negligible input of human faeces into soils on the Ullafelsen. Furthermore, we exclude a strong degradation of coprostanol in the topsoil horizons due to the non-detected epicoprostanol as a transformation product of coprostanol in terms of epimerization over time. Contents of 5α-cholestanol (≤ 1.4 µg g−1) as a transformation product of cholesterol (plant-derived) also indicate low degradation in the topsoil horizons.
Ratio 2 ((coprostanol + epicoprostanol) (5β-stigmastanol + epi-5β-stigmastanol)) ranged from 0 to 0.4 (Fig. 7) and showed a maximum in the Bh horizon due to the higher content of epi-5β-stigmastanol compared with the content of 5β-stigmastanol (Table S1). Our results confirm a predominance of 5β-stigmastanol and epi-5β-stigmastanol, which indicates a strong input of ruminant faeces into soils at the Ullafelsen.
Ratio 3 (β-sitosterol (β-sitosterol + 5β-stigmastanol + epi-5β-stigmastanol)) ranged from 0.95 to 1 (Fig. 7), demonstrating a strong influence of plant-derived steroids (high β-sitosterol contents) in soils at the Ullafelsen caused by the high contribution of β-sitosterol (Fig. 6). A high ratio 3 in the Bh, Bs and BvCv horizons can be explained by decreasing 5β-stigmastanol and epi-5β-stigmastanol contents. Roots of plants contribute to an input of β-sitosterol into subsoils (Piironen et al., 2000).
Ratio 4 (5β-stigmastanol epi-5β-stigmastanol) ranged from 0 to 1.0 (Fig. 8), decreasing from the topsoils to the subsoils. As discussed in Sect. 3.1, we observed a degradation effect of 5β-stigmastanol in our soils. Our results corroborate a higher degradation in the E (LL), Bh, Bs and BvCv horizons. We explain this with a slightly higher epi-5β-stigmastanol contribution in these subsoil horizons (Fig. 8; Table S1) due to epimerization (Bull et al., 1999a, b, 2003; Prost et al., 2017). For a reliable identification of faeces origin, we recommend considering the degradation effect and including epi-5β-stanols (e.g., epi-5β-stigmastanol and/or epicoprostanol) in steroid ratios (Bull et al., 2001).
Ratio 5 (deoxycholic acid lithocholic acid) ranged from 4.1 to 23.5 (Fig. 7), decreasing from top to bottom. A ratio < 5 indicates a dominance of human faeces, whereas a ratio > 5 corroborates a dominance of ruminant faeces (Prost et al., 2017). No LCA was detected in the Bs and BvCv horizons. Apart from the outlier of 4.1, our results showed a clear dominance of ruminant faeces input into soils at the Ullafelsen due to the high content of DCA. The outlier of 4.1, detected in an E (LL) horizon, can be allocated to a nearby trench soil profile (1.9 NE E 1 (LL)), which is beyond the archeological excavation area on the Ullafelsen. For this E (LL) horizon, we cannot exclude the input of human faeces regarding ratio 5, being based on the content of DCA (0.3 µg g−1) and LCA (0.1 µg g−1) (Table S2).
This study presents the first results of faecal biomarker analyses carried out on the prehistorical encampment site of Ullafelsen, Fotsch Valley, Austria. Steroid patterns of contemporary ruminants showed a dominance of 5β-stigmastanol, whereas epi-5β-stigmastanol as a degradation product of 5β-stigmastanol has to be considered for sheep. DCA was detected as the dominant bile acid for cattle and sheep. These data together with data of Prost et al. (2017) were used for the interpretation of the faecal biomarker results from the geoarcheological soil samples.
The highest sterol, stanol, stanone and bile acid contents in archeological soils on the Ullafelsen were found in the OAh3 and OAh2 horizons. The dominant steroid in our soils is β-sitosterol as plant-derived Δ5-sterol compound. The faecal markers for ruminants 5β-stigmastanol and DCA occurring in high contents in all topsoils corroborate current grazing by cattle and sheep, which can be associated with the alpine pastoralism in the Fotsch Valley. Human faeces could be detected only to a minor extent. Calculated ratios (ratios 1–5) confirmed the negligible input of human faeces and the dominant input of ruminant faeces (cattle and sheep) at the Ullafelsen. Modern vegetation and ruminant faeces, associated with the plant-based diet of cattle and sheep, could have induced the high input of plant-derived steroids in our soils. Reference soils from the Fotsch Valley show lower contents of steroids except for bile acids and confirm the enrichment of sterols, stanols and stanones in the soils on the Ullafelsen. We observed no leaching of steroids into subsoil horizons.
Soil profiles on the Ullafelsen represent the modern signal of ruminant faeces and of plant-derived steroids. Given the low contents of human-derived faecal biomarkers combined with our evaluation of faecal biomarker ratios (Fig. 7), we see no evidence for a prehistoric input of human faeces at the Ullafelsen and its surroundings. The archeological site of Ullafelsen was used for fireplaces and social living in the Mesolithic period. However, it cannot be excluded that human faeces markers will be detected in higher amounts close to the archeological excavation area. Future faecal biomarker analyses on further soil profiles at the Ullafelsen will aim at more specific insight into this hypothesis.
A robust age control for faecal biomarkers on the Ullafelsen is challenging because of a lack of age chronology. This study thus allows no reconstruction for the onset of alpine pastoralism. We assume the input of faecal steroids in the Holocene has taken place since the beginning of the alpine pastoralism in the Neolithic and Bronze Age.
In order to chronologically identify the history of land use in the Fotsch Valley, we suggest investigating faecal biomarkers on mire archives in the Fotsch Valley. Previous studies of two subalpine mire archives in the near surroundings of the prehistorical encampment site of Ullafelsen demonstrate the high potential for paleoenvironmental reconstructions.
TOC, TN and TOC N data are available from Zech et al. (2021). Faecal biomarker data of the archeological soil profiles on the Ullafelsen and reference soil profiles from the Fotsch Valley are available in the Supplement.
Table S1 gives an overview of all faecal biomarker soil samples from the prehistorical encampment site of Ullafelsen and reference sites in the Fotsch Valley, Stubai Alps, Austria. Sterol, stanol and stanone content (in micrograms per gram of dry matter) as well as ratios are presented. Table S2 gives an overview of all faecal biomarker soil samples from the prehistorical encampment site of Ullafelsen and reference sites in the Fotsch Valley, Stubai Alps, Austria. Bile acid content (in micrograms per gram of dry matter) as well as ratios are presented. Table S3 gives sterol, stanol and stanone content (in micrograms per gram of dry matter) for three replication measurements of modern cattle and sheep faeces from the prehistorical encampment site of Ullafelsen and reference sites in the Fotsch Valley, Stubai Alps, Austria. Table S4 gives bile acid content (in micrograms per gram of dry matter) for three replication measurements of modern cattle and sheep faeces from the prehistorical encampment site of Ullafelsen and reference sites in the Fotsch Valley, Stubai Alps, Austria. The supplement related to this article is available online at: https://doi.org/10.5194/bg-19-1135-2022-supplement.
The project idea was developed by MZ in cooperation with BG, DS and CG. Fieldwork was carried out by ML, MZ, JNH, DS and CG. ML performed most of the laboratory work with contributions made by TB, BG and MZ. The manuscript was prepared by ML. All co-authors contributed to the discussion of the results and read and approved the manuscript.
The contact author has declared that neither they nor their co-authors have any competing interests.
Publisher’s note: Copernicus Publications remains neutral with regard to jurisdictional claims in published maps and institutional affiliations.
We greatly acknowledge Sixten Bussemer for support of fieldwork and helpful discussions on pedogenesis of the archeological soil profiles from the Ullafelsen. Special thanks go to Oliver Kiewert and his team from the Bergheim hostel for excellent food and accommodation during the period of fieldwork. We also thank Corinna Heinrich, Heike Maennicke and Marianne Zech (formerly Benesch) for supporting laboratory work and for their pleasant collaboration throughout the research as well as Eike Hüsing for making a drone video of the Ullafelsen and its surroundings. Last but not least, we thank Eva Lehndorff and the anonymous reviewer for their constructive reviews that greatly helped to improve our manuscript.
This research has been supported by the Deutsche Forschungsgemeinschaft (grant no. GL 327/23-1 and ZE 844/12-1).
This open-access publication was funded by the Technische Universität Dresden (TUD).
This paper was edited by Petr Kuneš and reviewed by Eva Lehndorff and one anonymous referee.
Baeten, J., Marinova, E., Laet, V. de, Degryse, P., de Vos, D., and Waelkens, M.: Faecal biomarker and archaeobotanical analyses of sediments from a public latrine shed new light on ruralisation in Sagalassos, Turkey, J. Archaeol. Sci., 39, 1143–1159, https://doi.org/10.1016/j.jas.2011.12.019, 2012.
Birk, J. J., Teixeira, W. G., Neves, E. G., and Glaser, B.: Faeces deposition on Amazonian Anthrosols as assessed from 5β-stanols, J. Archaeol. Sci., 38, 1209–1220, https://doi.org/10.1016/j.jas.2010.12.015, 2011.
Birk, J. J., Dippold, M., Wiesenberg, G. L. B., and Glaser, B.: Combined quantification of faecal sterols, stanols, stanones and bile acids in soils and terrestrial sediments by gas chromatography–mass spectrometry, J. Chromatogr. A, 1242, 1–10, https://doi.org/10.1016/j.chroma.2012.04.027, 2012.
Björkhem, I. and Gustafsson, J.: Microbial transformation of cholesterol into coprostanol, Eur. J. Biochem., 21, 428–432, https://doi.org/10.1111/j.1432-1033.1973.tb02968.x, 1971.
Bull, I. D., Simpson, I. A., Bergen van, P. F., and Evershed, R. P.: Muck “n” molecules: organic geochemical methods for detecting ancient manuring, Antiquity, 73, 86–96, https://doi.org/10.1017/S0003598X0008786X, 1999a.
Bull, I. D., Simpson, I. A., Dockrill, S. J., and Evershed, R. P.: Organic geochemical evidence for the origin of ancient anthropogenic soil deposits at Tofts Ness, Sanday, Orkney, Org. Geochem., 30, 535–556, https://doi.org/10.1016/S0146-6380(99)00020-0, 1999b.
Bull, I. D., Betancourt, P. P., and Evershed, R. P.: An Organic Geochemical Investigation of the Practice of Manuring at a Minoan site on Pseira Island, Crete, Geoarchaeology, 16, 223–242, https://doi.org/10.1002/1520-6548(200102)16:2<223::AID-GEA1002>3.0.CO;2-7, 2001.
Bull, I. D., Lockheart, M. J., Elhmmali, M. M., Roberts, D. J., and Evershed, R. P.: The origin of faeces by means of biomarker detection, Environ. Int., 27, 647–654, https://doi.org/10.1016/S0160-4120(01)00124-6, 2002.
Bull, I. D., Elhmmali, M. M., Roberts, D. J., and Evershed, R. P.: The application of steroidal biomarkers to track the abandonment of a Roman wastewater course at the Agora (Athens, Greece), Archaeometry, 45, 149–161, https://doi.org/10.1111/1475-4754.00101, 2003.
Bull, I. D., Elhmmali, M. M., Perret, V., Matthews, W., Roberts, D. J., and Evershed, R. P.: Biomarker evidence of faecal deposition in archaeological sediments at Çatalhöyük, in: Changing materialities at Çatalhöyük: reports from the 1995–1999 seasons, edited by: Hodder, I., British Institute at Ankara, McDonald Institute for Archaeological Research, Cambridge, Great Britain, 415–420, https://doi.org/10.18866/j.ctt1pk874x, 2005.
Cornelissen, M. and Reitmaier, T.: Filling the gap: Recent Mesolithic discoveries in the central and south-eastern Swiss Alps, Quaternary Int., 423, 9–22, https://doi.org/10.1016/j.quaint.2015.10.121, 2016.
Egli, M., Mirabella, A., and Sartori, G.: The role of climate and vegetation in weathering and clay mineral formation in late Quaternary soils of the Swiss and Italian Alps, Geomorphology, 102, 307–324, https://doi.org/10.1016/j.geomorph.2008.04.001, 2008.
Evershed, R. P., Bethell, P. H., Reynolds, P. J., and Walsh, N. J.: 5β-Stigmastanol and Related 5β-Stanols as Biomarkers of Manuring: Analysis of Modern Experimental Material and Assessment of the Archaeological Potential, J. Archaeol. Sci., 24, 485–495, https://doi.org/10.1006/jasc.1996.0132, 1997.
Fontana, F., Visentin, D., and Wierer, U.: MesoLife. A Mesolithic perspective on Alpine and neighbouring territories, Quaternary Int., 423, 1–4, https://doi.org/10.1016/j.quaint.2016.06.016, 2016.
Geitner, C. and Schäfer, D.: Interdisziplinäre Zusammenarbeit an der Schnittstelle von Archäologie und Bodenkunde im Gebirge – Grundsätzliche Überlegungen und Beispiele des Mesolithfundplatzes Ullafelsen (Tirol), in: Archäologie in den Alpen: Alltag und Kult, edited by: Mandl, F. and Stadler, H., ANISA 3, Nearchos 19, Verein für alpine Forschung, Haus im Ennstal, 25–42, ISBN 978-3-901-07121-8, 2010.
Geitner, C., Bussemer, S., Ehrmann, O., Ikinger, A., Schäfer, D., Traidl, R., and Tscherko, D.: Bodenkundlich-stratigraphische Befunde am Ullafelsen im hinteren Fotschertal sowie ihre landschaftsgeschichtliche Interpretation, in: Das Mesolithikum-Projekt Ullafelsen (Teil 1), Mensch und Umwelt im Holozän Tirols 1, edited by: Schäfer, D., Philipp von Zabern GmbH, Darmstadt, Germany, 109–151, ISBN 978-3-8053-4375-6, 2011.
Geitner, C., Schäfer, D., Bertola, S., Bussemer, S., Heinrich, K., and Waroszewski, J.: Landscape archaeological results and discussion of Mesolithic research in the Fotsch valley (Tyrol), in: From the foreland to the Central Alps – Field trips to selected sites of Quaternary research in the Tyrolean and Bavarian Alps (DEUQUA EXCURSIONS), E&G Quaternary Science Journal, edited by: Kerschner, H., Krainer, K., and Spötl, C., Geozon Science Media, Berlin, Germany, 106–115, https://doi.org/10.3285/g.00011, 2014.
Gilck, F. and Poschlod, P.: The origin of alpine farming: A review of archaeological, linguistic and archaeobotanical studies in the Alps, Holocene, 29, 1503–1511, https://doi.org/10.1177/0959683619854511, 2019.
Glaser, B. and Birk, J. J.: State of the scientific knowledge on properties and genesis of Anthropogenic Dark Earths in Central Amazonia (terra preta de Indio), Geochim. Cosmochim. Ac., 82, 39–51, https://doi.org/10.1016/j.gca.2010.11.029, 2012.
Glaser, B., Haumaier, L., Guggenberger, G., and Zech, W.: The “terra preta” phenomenon: a model for sustainable agriculture in the humid tropics, Naturwissenschaften, 88, 37–41, https://doi.org/10.1007/s001140000193, 2001.
Hartmann, M.-A.: Plant sterols and the membrane environment, Trend. Plant Sci., 3, 170–175, https://doi.org/10.1016/S1360-1385(98)01233-3, 1998.
Haurrault, L., Milek, K., Jarde, E., Jeanneau, L., Derrien, M., and Anderson, D. G.: Faecal biomarkers can distinguish specific mammalian species in modern and past environments, PLoS ONE, 14, 1–26, https://doi.org/10.1371/journal.pone.0211119, 2019.
Holtvoeth, J., Vogel, H., Wagner, B., and Wolff, G. A.: Lipid biomarkers in Holocene and glacial sediments from ancient Lake Ohrid (Macedonia, Albania), Biogeosciences, 7, 3473–3489, https://doi.org/10.5194/bg-7-3473-2010, 2010.
IUSS Working Group WRB: World Reference Base for Soil Resources 2014, update 2015. International soil classification system for naming soils and creating legends for soil maps, 106, World Soil Resources Reports FAO, FAO publications, Rome, E-ISBN 978-92-5-108370-3, 2015.
Kemmer, I.: Die rezente Vegetation im inneren Fotschertal/Nördliche Stubaier Alpen, in: Das Mesolithikum-Projekt Ullafelsen (Teil 1), Mensch und Umwelt im Holozän Tirols 1, edited by: Schäfer, D., Philipp von Zabern GmbH, Darmstadt, Germany, 155–193, ISBN 978-3-8053-4375-6, 2011.
Kuhajda, K., Kandrac, J., Kevresan, S., Mikov, M., and Fawcett, J. P.: Structure and origin of bile acids: An overview, Eur. J. Drug Metab. Ph., 31, 135–143, https://doi.org/10.1007/BF03190710, 2006.
Lauer, F., Prost, K., Gerlach, R., Pätzold, S., Wolf, M., Urmersbach, S., Lehndorff, E., Eckmeier, E., and Amelung, W.: Organic Fertilization and Sufficient Nutrient Status in Prehistoric Agriculture? – Indications from Multi-Proxy Analyses of Archaeological Topsoil Relicts, PLoS ONE, 9, e106244, https://doi.org/10.1371/journal.pone.0106244, 2014.
Lloyd, C. E. M., Michaelides, K., Chadwick, D. R., Dungait, J. A. J., and Evershed, R. P.: Tracing the flow-driven vertical transport of livestock-derived organic matter through soil using biomarkers, Org. Geochem., 43, 56–66, https://doi.org/10.1016/j.orggeochem.2011.11.001, 2012.
Marion, S., Studer, N., Desharnais, L., Menin, L., Escrip, S., Meibom, A., Hapfelmeier, S., and Bernier-Latmani, R.: In vitro and in vivo characterization of Clostridium scindens bile acid transformations, Gut Microbes, 10, 481–503, https://doi.org/10.1080/19490976.2018.1549420, 2019.
Nittel, P.: Geologie, Hydrogeologie und Geomorphologie des Fotschertals – Kartierungsergebnisse Projekt “Sellrain”, in: Das Mesolithikum-Projekt Ullafelsen (Teil 1), Mensch und Umwelt im Holozän Tirols 1, edited by: Schäfer, D., Philipp von Zabern GmbH, Darmstadt, Germany, 61–92, ISBN 978-3-8053-4375-6, 2011.
Noda, M., Tanaka, M., Seto, Y., Aiba, T., and Oku, C.: Occurrence of cholesterol as a major sterol component in leaf surface lipids, Lipids, 23, 439–444, https://doi.org/10.1007/BF02535517, 1988.
Ossendorf, G., Groos, A. R., Bromm, T., Tekelemariam, M. G., Glaser, B., Lesur, J., Schmidt, J., Akcar, N., Bekele, T., Beldados, A., Demissew, S., Kahsay, T. H., Nash, B. P., Nauss, T., Negash, A., Nemomissa, S., Veit, H., Vogelsang, R., Woldu, Z., Zech, W., Opgenoorth, L., and Miehe, G.: Middle Stone Age foragers resided in high elevations of the glaciated Bale Mountains, Ethiopia, Science, 365, 583–587, https://doi.org/10.1126/science.aaw8942, 2019.
Piironen, V., Lindsay, D. G., Miettinen, T. A., Toiva, J., and Lampi, A.: Plant sterols: biosynthesis, biological function and their importance to human nutrition, J. Sci. Food Agr., 80, 939–966, https://doi.org/10.1002/(SICI)1097-0010(20000515)80:7<939::AID-JSFA644>3.0.CO;2-C, 2000.
Prost, K., Birk, J. J., Lehndorff, E., Gerlach, R., and Amelung, W.: Steroid Biomarkers Revisited – Improved Source Identification of Faecal Remains in Archaeological Soil Material, PLoS ONE, 12, 1–30, https://doi.org/10.1371/journal.pone.0164882, 2017.
Reitmaier, T., Doppler, T., Pike, A. W. G., Deschler-Erb, S., Hajdas, I., Walser, C., and Gerling, C.: Alpine cattle management during the Bronze Age at Ramosch-Mottata, Switzerland, Quaternary Int., 484, 19–31, https://doi.org/10.1016/j.quaint.2017.02.007, 2018.
Sakai, K., Makino, T., Kawai, Y., and Mutai, M.: Intestinal Microflora and Bile Acids – Effect of Bile Acids on the Distribution of Microflora and Bile Acid in the Digestive Tract of the Rat, Microbiol. Immun., 24, 187–196, https://doi.org/10.1111/j.1348-0421.1980.tb00578.x, 1980.
Schäfer, D.: Das Mesolithikum-Projekt Ullafelsen – Landschaftlicher Rahmen und archäologische Befunde, Arbeitsstand 2009/2010, in: Das Mesolithikum-Projekt Ullafelsen (Teil 1), Mensch und Umwelt im Holozän Tirols 1, edited by: Schäfer, D., Philipp von Zabern GmbH, Darmstadt, Germany, 245–351, ISBN 978-3-8053-4375-6, 2011a.
Schäfer, D. (Ed.): Das Mesolithikum-Projekt Ullafelsen (Teil 1), Mensch und Umwelt im Holozän Tirols 1, Philipp von Zabern GmbH, Darmstadt, Germany, 560 pp., ISBN 978-3-8053-4375-6, 2011b.
Schäfer, D., Bertola, S., Pawlik, A., Geitner, C., Waroszewski, J., and Bussemer, S.: The landscape-archaeological Ullafelsen Project (Tyrol, Austria), Preistoria Alpina, 48, 29–38, 2016.
Schlosser, E.: Das Fotschertal – regionale Klimatologie und gebirgsmeteorologische Aspekte, in: Das Mesolithikum-Projekt Ullafelsen (Teil 1), Mensch und Umwelt im Holozän Tirols 1, edited by: Schäfer, D., Philipp von Zabern GmbH, Darmstadt, Germany, 11–16, ISBN 978-3-8053-4375-6, 2011.
Schroeter, N., Lauterbach, S., Stebich, M., Kalanke, J., Mingram, J., Yildiz, C., Schouten, S., and Gleixner, G.: Biomolecular Evidence of Early Human Occupation of a High-Altitude Site in Western Central Asia During the Holocene, Front. Earth Sci., 8, 1–20, https://doi.org/10.3389/feart.2020.00020, 2020.
Shillito, L.-M., Whelton, H. L., Blong, J. C., Jenkins, D. L., Connolly, T. J., and Bull, I. D.: Pre-Clovis occupation of the Americas identified by human fecal biomarkers in coprolites from Paisley Caves, Oregon, Sci. Adv., 6, 1–8, https://doi.org/10.1126/sciadv.aba6404, 2020.
Thomas, L. K. and Hale, M. G.: Effects of kinetin in the rooting medium on root exudation of free fatty acids and sterols from roots of Arachis hypogaea L. “argentine” under axenic conditions, Soil Biol. Biochem., 15, 125–126, https://doi.org/10.1016/0038-0717(83)90131-1, 1983.
Verma, S. and Gupta, R.: Comparative estimation of β-sitosterol in roots, leaves and flowers of Clerodendrum infortunatum L., Int. J. Green Ph., 7, 131–135, https://doi.org/10.4103/0973-8258.116394, 2013.
von der Lühe, B., Dawson, L., Mayes, R. W., Forbes, S. L., and Fiedler, S.: Investigation of sterols as potential biomarkers for the detection of pig (S. s. domesticus) decomposition fluid in soils, Forensic Sci. Int., 230, 68–73, https://doi.org/10.1016/j.forsciint.2013.03.030, 2013.
von der Lühe, B., Birk, J. J., Dawson, L., Mayes, R. W., and Fiedler, S.: Steroid fingerprints: efficient biomarkers of human decomposition fluids in soil, Org. Geochem., 124, 228–237, https://doi.org/10.1016/j.orggeochem.2018.07.016, 2018.
von der Lühe, B., Prost, K., Birk, J. J., and Fiedler, S.: Steroids aid in human decomposition fluid identification in soils of temporary mass graves from World War II, J. Archaeol. Sci.-Rep., 32, 102431, https://doi.org/10.1016/j.jasrep.2020.102431, 2020.
Wiedner, K., Schneeweiß, J., Dippold, M., and Glaser, B.: Anthropogenic Dark Earth in Northern Germany – The Nordic Analogue to terra preta de Índio in Amazonia, Catena, 132, 114–125, https://doi.org/10.1016/j.catena.2014.10.024, 2015.
Zech, M., Lerch, M., Bliedtner, M., Bromm, T., Seemann, F., Szidat, S., Salazar, G., Zech, R., Glaser, B., Haas, J. N., Schäfer, D., and Geitner, C.: Revisiting the subalpine Mesolithic site Ullafelsen in the Fotsch Valley, Stubai Alps, Austria – new insights into pedogenesis and landscape evolution from leaf-wax-derived n-alkanes, black carbon and radiocarbon dating, E&G Quaternary Sci. J., 70, 171–186, https://doi.org/10.5194/egqsj-70-171-2021, 2021.
Zech, W. and Wilke, B.: Vorläufige Ergebnisse einer Bodenchronosequenzstudie im Zillertal, Mitt. Deutsch. Bodenkundl. Gesell., 25, 571–586, 1977.
Zech, W., Schad, P., and Hintermaier-Erhard, G.: Böden der Welt, 2, Springer Spektrum, Berlin Heidelberg, Germany, E-ISBN 978-3-642-36575-1, 164 pp., 2014.