the Creative Commons Attribution 4.0 License.
the Creative Commons Attribution 4.0 License.
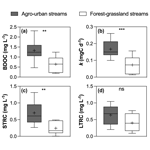
Enhanced bioavailability of dissolved organic matter (DOM) in human-disturbed streams in Alpine fluvial networks
Thibault Lambert
Pascal Perolo
Nicolas Escoffier
Marie-Elodie Perga
The influence of human activities on the role of inland waters in the global carbon (C) cycle is poorly constrained. In this study, we investigated the impact of human land use on the sources and biodegradation of dissolved organic matter (DOM) and its potential impact on bacterial respiration in 10 independent catchments of the Lake Geneva basin. Sites were selected along a gradient of human disturbance (agriculture and urbanization) and were visited twice during the winter high-flow period. Bacterial respiration and DOM bioavailability were measured in the laboratory through standardized dark bioassays, and the influence of human land uses on DOM sources, composition and reactivity was assessed from fluorescence spectroscopy. Bacterial respiration was higher in agro-urban streams but was related to a short-term bioreactive pool (0–6 d of incubation) of autochthonous origin, whose relative contribution to the total DOM pool increased with the degree of human disturbance. On the other hand, the degradation of a long-term (6–28 d) bioreactive pool related to terrestrial DOM was independent from the catchment land use and did not contribute substantially to aquatic bacterial respiration. From a greenhouse gas emission perspective, our results suggest that human activities may have a limited impact on the net C exchanges between inland waters and the atmosphere, as most CO2 fixed by aquatic producers in agro-urban streams is cycled back to the atmosphere after biomineralization. Although seasonal and longitudinal changes in DOM sources must be considered, the implications of our results likely apply more widely as a greater proportion of autochthonous-DOM signature is a common feature in human-impacted catchments. Yet, on a global scale, the influence of human activities remains to be determined given the large diversity of effects of agriculture and urbanization on freshwater DOM depending on the local environmental context.
- Article
(1418 KB) -
Supplement
(782 KB) - BibTeX
- EndNote
Continental surface waters receive more terrestrial carbon (C) than they export toward oceans, leading to the conceptualization of inland waters as active pipes that process, emit and store C during its transit from lands to oceans (Cole et al., 2007). Within this framework, the mineralization of terrestrial dissolved organic matter (DOM) by aquatic heterotrophic bacterial communities is a key process by which terrestrial C returns to the atmosphere through CO2 emissions (Fasching et al., 2014; Lapierre et al., 2013; Mayorga et al., 2005). The study of DOM transport and transformation along fluvial networks is therefore of primary importance, yet a major gap in knowledge revolves around the impact of human activities on the reactivity and bacterial use (i.e., respiration or allocation to biomass) of terrestrial DOM in inland waters (Creed et al., 2015; Xenopoulos et al., 2021).
Agricultural and urban land uses are major catchment features impacting DOM sources and composition in aquatic ecosystems from local (Wilson and Xenopoulos, 2009) to regional scales (Lambert et al., 2017; Williams et al., 2016). Streams draining agricultural landscapes and/or urban catchments are commonly enriched in DOM of low molecular weight compared to streams draining forested catchments whereby DOM is dominated by aromatic, high molecular weight compounds (Lambert et al., 2017; Williams et al., 2010). Greater proportions of lower molecular weight compounds in agro-urban streams can be the consequence of a greater autochthonous algal production and bacterial activity in nutrient-enriched waters (Fuß et al., 2017; Lu et al., 2014; Williams et al., 2010; Wu et al., 2019), reduced hydrological connection with terrestrial sources (Giling et al., 2014; Parr et al., 2015), or transfer of less humified soil organic matter due to agricultural practices (Humbert et al., 2020; Lambert et al., 2017; Landsman-Gerjoi et al., 2020). As lower molecular weight molecules are typically more labile and easily available for uptake to bacterial communities (Berggren et al., 2010; Catalán et al., 2017; Kaplan and Bott, 1989), greater DOM processing can be expected in agro-urban streams (Hosen et al., 2014; Parr et al., 2015). However, the destabilization of a stock of soil organic material built before the conversion of forests or wetlands for agriculture or urban development can lead to the mobilization of large amounts of humic and highly aromatic DOM into surface waters (Ekblad and Bastviken, 2019; Graeber et al., 2012; Hu et al., 2016; Petrone et al., 2011). As a consequence, the impact of human land uses on the dynamic of DOM in inland waters may be highly diverse depending on how agriculture and urbanization affect DOM sources, content, and composition as well as external drivers such as inorganic nutrients known to regulate bacterial DOM processing (Guillemette and del Giorgio, 2012; Reche et al., 1998).
Different scenarios about the consequences on net C exchanges for surface waters can be envisaged depending on the impact of human land uses on freshwater DOM. First, increasing delivery of colored and aromatic terrestrial DOM can lead to an increase in CO2 emissions supposing that this terrestrial material gets respired by bacterial communities (Fasching et al., 2014; Lapierre et al., 2013). Second, increase in the export of low molecular weight DOM, either derived from terrestrial sources or produced in-stream, can result in more DOM respired and emitted as CO2 into the atmosphere (Bodmer et al., 2016; Borges et al., 2018). However, if changes in DOM composition result from an enhancement in aquatic primary production, enhanced respiration of autochthonously produced DOM shall not lead to higher net CO2 emissions since the amount of C emitted into the atmosphere would be lower than or equivalent to the amount of CO2 previously fixed by primary aquatic producers. An alternative scenario would be that the release of simple and labile organic compounds derived from autochthonous sources enhances the degradation of terrestrial aromatic DOM by the so-called priming effect (Bianchi, 2011), inducing a final net increase of C emitted to the atmosphere. The priming effect is a process through which labile pool of DOM can enhance (“prime”) the degradation of a more recalcitrant DOM pool based on interactions between microbial communities and/or changes in their functions (Guenet et al., 2010; Kuzyakov et al., 2000), but its occurrence in aquatic ecosystems is highly debated (Attermeyer et al., 2014; Bengtsson et al., 2018; Lambert and Perga, 2019). To determine how human activities may impact the mineralization of terrestrial DOM and infer the consequence on the active role of inland waters into the global C cycle, it is therefore necessary to evaluate the consequences on DOM sources, composition, but also DOM bioavailability and to identify which fraction of the DOM pool fuels respiration. This information is critical to establish the role of human land uses on the linkage between terrestrial and aquatic ecosystems.
In this study, we aimed to investigate the impact of human land uses on the role of inland waters as bioreactors with regard to the processing of terrestrial DOM. Water samples were collected twice during the wet season in 10 independent catchments selected along a gradient of human pressure (agriculture and urbanization) in the Lake Geneva basin. Patterns in DOM degradation were investigated based on standardized dark degradation experiments and by the consumption of specific compounds of low molecular weights. Decreases in dissolved organic carbon (DOC) concentrations and changes in DOM composition (assessed by fluorescence spectroscopy) during incubations were used to unravel the contribution of different fractions to the bulk DOM kinetic degradation as well as to the bacterial respiration measured under field conditions. With this approach combining fieldwork and laboratory experiments, we specifically aimed to identify the origin of DOM contributing to bacterial respiration in human-impacted streams and to evaluate the impact of human activities on the biodegradation of terrestrial DOM in Alpine fluvial networks.
2.1 Study sites and sampling
Stream and river waters were collected in 10 independent tributaries of Lake Geneva, the largest lake of western Europe located on the border between France and Switzerland in the Western Alps (Fig. 1). Lake Geneva lies in the Alpine Foreland between the Alps and the Jura Mountains and was carved during Quaternary glaciations mostly into the Tertiary Molasse. Drainage areas of streams and rivers ranged from 11 to 5240 km2, Strahler order from 2 to 7, and mean elevation from 614 to 2124 m, and land cover was dominated by forests (39 ± 19 %), croplands (30 ± 22 %), grasslands (13 ± 14 %), and urban areas (11 ± 14 %) according to the Swiss Federal Office for the Environment (FOEN). Agriculture is dominated by non-irrigated arable lands and vineyards and forests by coniferous and broad-leaved trees. Selected streams and rivers drained a mosaic of land cover categories and were classified as agro-urban or forest–grassland streams if the sum of croplands and urban areas extents was higher or lower than 50 %, respectively (Table 1).
Samples were collected on two occasions: at the end of autumn between 13 and 14 November 2018 and at the end of winter between 5 and 7 March 2019. Campaigns were thus carried out during the wet season, i.e., when high discharge conditions may favor greater export of terrestrial DOM (Lambert et al., 2013). The only exception was the Rhône River, which experiences higher water discharge in summer due to the glacio-nival regime of the river (Loizeau and Dominik, 2000). Water temperatures during field campaigns were 10.1 ± 1.8 and 6.6 ± 1.1 ∘C, respectively, but discharge and precipitation conditions at the time of collection were similar (FOEN data). Water was collected below surface in 2 L acid-washed high-density polyethylene (HDPE) bottles and filtered on site. A known volume of water (between 1000 and 1500 mL) was filtered on pre-combusted (450 ∘C for 4 h) Whatman glass fiber filters (GF/F grade, 0.7 µm nominal pore size, 47 mm diameter) for chlorophyll a (Chl a) measurements. This filtered water was either stored in 1 L acid-washed HDPE bottles for further use in the incubation experiments and the measurements of bacterial metabolism, as described below, or further filtered at 0.2 µm with polyethersulfone (PES) syringe encapsulated filters for dissolved organic carbon (DOC), colored and fluorescent DOM (CDOM and FDOM), soluble reactive phosphorus (SRP), and dissolved inorganic nitrogen (DIN) measurements. Syringe encapsulated filters were first rinsed with ultrapure water (60 mL) in the laboratory and then with 15–20 mL of water from the sampling site before collecting samples. Samples for DOC concentrations were stored in 40 mL acid-washed glass vials with polytetrafluoroethylene (PTFE)-coated septa, and samples for CDOM and FDOM were stored in 40 mL acid-washed amber glass vials with PTFE-coated septa. Samples for SRP and DIN were stored separately in 50 mL sterile centrifuge tubes. All samples and filters were brought back to the laboratory within 3 h in cool and dark conditions. Samples for SRP and Chl a measurements were frozen at −21 ∘C until analysis. Other samples were stored in a dark chamber at 4–5 ∘C until analysis typically done within the following 2 weeks.
2.2 Characterization of DOM degradation kinetics
Incubations were prepared once back to the laboratory. Water samples previously filtered on site at 0.7 µm were divided into 250 mL acid-washed glass flasks and incubated for 28 d in the dark at 20 ∘C. A fixed temperature was chosen in order to be able to compare DOM degradation dynamics across our sampling sites at different periods. Because biological activity is strongly impacted by temperature, using water temperature on the field for incubations may have masked patterns in degradation related to differences in the source and composition of DOM (del Giorgio and Davis, 2003). Using a fixed temperature allowed us to investigate how DOM bacterial degradation varied among streams, once the effect of temperature was removed. Biodegradable DOC (BDOC), bacterial respiration (BR), and the bacterial consumption of low molecular weight compounds were incubated in similar conditions (see below), ensuring comparability between water quality, bacterial metabolism, and DOM degradation dynamics. However, consumption and respiration rates should not be considered as representative of field conditions, as incubation temperature – and thus bacterial activity – was higher compared to field conditions.
Different subsets of flasks (in triplicates) were prepared and sacrificed for DOC measurements and DOM characterization every 3–5 d for the first 10 d and then every 5–8 d up to day 28. Samples were filtered at 0.2 µm with PES syringe encapsulated filters as described above and stored in a dark chamber at 4–5 ∘C. DOC measurements were done within 48 h after collection and CDOM and FDOM analyses over the following week. Dissolved oxygen depletion during the incubations was avoided by leaving a large headspace within the glass flasks and by a regular (every 3–4 d) renewal of the headspace.
Several models can be used to characterize DOM degradation kinetic. Here we applied a first-order exponential decay model in order to derive a decay constant describing the overall dynamic of DOM degradation (Lambert and Perga, 2019; Lu et al., 2013; Shang et al., 2018) as well as the size of the short- and a long-term reactive carbon pools (STRC and LTRC, respectively). Decreasing DOC concentrations during the incubations were modeled using GraphPad Prism 8 software according to the following equation:
where DOCt (in mg L−1) is the DOC concentration measured at the incubation time t (in days), DOCcons (in mg L−1) the amount of DOC consumed at the end of the incubation, k the decay constant (mg C d−1), and DOCresidual (in mg L−1) the concentration of the residual pool remaining in solution at the end of the incubation. Biodegradable DOC (BDOC) was calculated as the difference in DOC between the initial and final time. Furthermore, we used the k decay constant from the model to quantify the STRC and LTRC pools: STRC was defined as the amount of DOC consumed within the first six days of incubation and the LTRC as the amount of DOC degraded between days 6 and 28 (Fig. S1 in the Supplement). The separation between STRC and LTRC pools was based on a breakpoint in the degradation curves observed around the sixth day of incubation in almost all experiments. Finally, changes in DOM composition during the incubations were also monitored by fluorescence measurement coupled to parallel factor analysis (PARAFAC) as described below.
2.3 Degradation of low molecular weight compounds
We also determined the consumption of low molecular weight compounds including carbohydrates (CAR), carboxylic and acetic acids (C&AA), and amino acids (AA) using Biolog Ecoplates® (Garland and Mills, 1991; Weber and Legge, 2009, 2010). Ecoplates® are 96-well microplates containing 31 different carbon substrates (in triplicates) plus a tetrazolium dye. The bacterial respiration activity associated with a specific substrate reduces the tetrazolium dye and produces a color measurable at 590 nm in absorbance. The intensity of color development in color can be related to the amount of substrate consumed (e.g., Berggren and del Giorgio 2015). Water from each site (125 µL) filtered at 0.7 µm was added to each well of one Ecoplate® per site, which was then incubated in the dark at 20 ∘C for 3 to 9 d until the average well color development (AWCD) reached an asymptote. The absorbance at 590 nm was measured with a TECAN microplate reader one to two times per day. Color development of each carbon substrate was calculated as the blank-corrected absorbance at the time when the AWCD was closest to 0.5 (0.51 ± 0.11, n = 60) following recommendations of Weber and Legge (2010). Carbon substrates were then classified as CAR, C&AA, or AA according to Weber and Legge (2009). The color development for each of these categories was calculated as the mean of the color of the respective substrates normalized by the AWCD. While Ecoplates® also include other organic compounds such as polymers or amines/amides, we focused in this study on the consumption of low molecular weight compounds known to support bacterial respiration (Kaplan and Bott, 1989).
2.4 Bacterial respiration measurements
The 0.7 µm filtered waters used for incubation experiments were also used to measure BR in stream and river waters. BR was determined from the decrease in dissolved oxygen (DO) in 60 mL borosilicate serum bottles filled with water, sealed with a butyl stopper and crimped with an aluminum cap without headspace (three serum bottles per site). These vials were equipped with SP-PSt7 oxygen planar sensor spots (PreSens) in order to follow DO consumption after 24 h of incubation in the dark at 20 ∘C. Initial (1 h after the start of incubation) and final DO was recorded using a PreSens Fibox 4 equipped with a fiber-optic oxygen transmitter. Calibration of the PreSens Fibox 4 (two-point calibration at 0 and 100 % oxygen saturation) was performed and verified before measurements. BR data were converted into carbon units using a respiratory quotient of 1.
2.5 Analytical methods
DIN (defined as the sum of nitrate, ammonium, and nitrite) was measured by ion chromatography (Metrohm instrument). SRP was determined by spectrophotometry using the ammonium molybdate-potassium antimonyl tartrate method (AFNOR, 2005). DOC concentrations were measured with a total organic carbon analyzer (TOC-L Series, Shimadzu), with a detection limit of 0.01 mg L−1 and a precision better than ± 5 % based on duplicates and standards. Chl a concentrations were determined by spectrophotometry after ethanol extraction (90 %). Frozen filters were put in 15 mL sterile centrifuge tubes in which 10 mL of ethanol (90 %) was added. Tubes were vigorously shaken and then installed in an ultrasonic bath at 70 ∘C for 10 min. Tubes were then stored in a dark chamber for a night, centrifuged 5 min at 4000 rpm, and then 10 min at 4000 rpm. Chl a concentrations were determined from absorbance at 665 nm after correction of sample turbidity concomitantly measured at 750 nm. Absorbance for CDOM was measured with a Lambda 365 UV/vis spectrophotometer (Perkin Elmer) from 200 to 700 nm (1 nm increment) using a 10 cm quartz cuvette. Napierian absorption coefficients were calculated according to
where aλ is the absorption coefficient (m−1) at wavelength λ, Aλ the absorbance at wavelength λ, and L the path length of the optical cell in meters. Spectral slopes for the intervals 275–295 and 350–400 nm were determined from the linear regression of the log-transformed aλ spectra versus wavelength and used to determine the slope ratio (SR). The slope ratio SR, calculated as the ratio of S275–295 to S350–400, is inversely related to the molecular weight distribution of DOM (Helms et al., 2008).
FDOM was measured with a Fluorolog-3 spectrofluorometer (Horiba) using a 1 cm quartz cuvette across excitation wavelengths of 270–450 nm (5 nm increment) and emission wavelengths of 300–500 nm (2 nm increment) in order to build excitation-emission matrices (EEMs). Lamp intensity and instrument calibration were systematically verified before running samples. EEMs were acquired in sample emission to lamp reference mode, and a correction matrix provided by the manufacturer in both excitation and emission dimensions was automatically applied during acquisition. EEMs were then decomposed into individual components using PARAFAC algorithms (Stedmon et al., 2003). Additional samples collected in Lake Geneva and in the Rhône Basin upstream of Lake Geneva were included in the model (total numbers of EEMs > 800). EEMs preprocessing (Raman scattering removal and standardization to Raman units) was performed prior to the PARAFAC modeling. Normalization was done using a Milli-Q water sample run the same day as the sample. An eight-component PARAFAC model was obtained using the drEEM 0.3.0 Toolbox (Murphy et al., 2013) for MATLAB (MathWorks, Natick, MA, USA). Split-half analysis, random initialization, and visualization of residuals EEMs were used to test and validate the model. The positions of maximum peaks of the PARAFAC components were compared to previous studies with the open fluorescence database OpenFluor using the OpenFluor add-on for the open-source chromatography software OpenChrom (Murphy et al., 2014). The maximum fluorescence FMax values of each component for a particular sample provided by the model were summed to calculate the total fluorescence signal FTot of the sample in Raman units. The relative abundance of any particular PARAFAC component X was then calculated as % CX = . Precision of EEM-PARAFAC, based on replicate measurements (n = 5) of different samples, was ± 0.001 for FMax values of components C1 to C5 and ± 0.003 for FMax values of components C6 to C8, and ± 0.2 % for % CX for components C1 to C5 and ± 0.5 % for components C6 to C8. Finally, the variations of PARAFAC components during incubations were expressed as ( with t0 and tf the initial and final values of FMax, respectively. Based on the accuracy of EEM-PARAFAC measurements estimated by replicate measurements (see above), we considered variations to be significant if the median of response ratio was higher than ± 0.05 for components C1 to C5 and ± 0.1 for components C6 to C8.
2.6 Statistical analyses
Differences in water quality, DOM degradability, BR, and consumption of low molecular weight compounds between agro-urban and forest–grassland streams were tested with a Mann–Whitney unpaired t test at 0.05 confidence interval level. Differences between the sampling periods were investigated by paired t tests. The level of significance was set to 0.05. The ROUT method implemented in the GraphPad Prism 8 software was used to identify potential outliers. A principal component analysis (PCA) was performed to investigate the importance of human land uses on water quality relative to other geomorphological features (e.g., mean elevation) of the study sites. The data selected for the PCA were DOC, SRP, DIN, and the relative abundance of PARAFAC components collected during the two main campaigns. The PCA was done using the prcomp function in the R software, and the factoextra package was used to identify the variables that contribute the most to the first two dimensions of the PCA. The sum of cropland and urban area extents were used as descriptive variables in the PCA biplot.
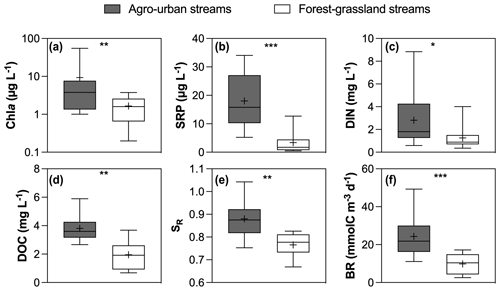
Figure 2Boxplots of (a) Chl a, (b) SRP, (c) DIN, (d) DOC concentrations and (e) SR values and (f) BR in agro-urban (grey) and forest–grassland (white) streams. The box represents the first and third quartile, the horizontal line corresponds to the median, the cross corresponds to the average, and the error bars correspond to the maximum and minimum. Mann–Whitney unpaired t tests were used to test for statistical differences: ns represents not significant, ∗ = p < 0.05, = p < 0.01, = p < 0.001, = p < 0.0001.
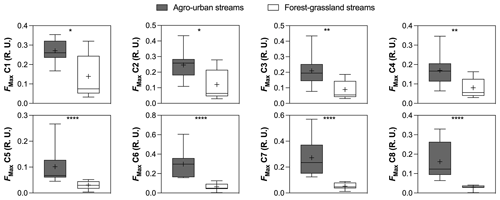
Figure 3Boxplots of FMax values of PARAFAC components in agro-urban (grey) and forest–grassland (white) streams. The box represents the first and third quartile, the horizontal line corresponds to the median, the cross corresponds to the average, and the error bars correspond to the maximum and minimum. Mann–Whitney unpaired t tests were used to test for statistical differences: ns represents not significant, ∗ = p < 0.05, = p < 0.01, = p < 0.001, = p < 0.0001.
3.1 Water quality and DOM composition across catchments
Hydro-climatic conditions were similar for the two sampling campaigns that occurred during high winter base flow level, and no significant difference was found in the overall water quality between the two campaigns (not shown). However, significant differences were observed between sampling sites depending on the dominant land cover (Figs. 2 and 3). Chl a (total range of variation between sites and periods from 0.2 to 54.9 µg L−1), SRP (from 0.5 to 34.5 µg L−1), DIN (from 0.3 to 8.8 mg L−1), and DOC (from 0.7 to 5.9 mg L−1) concentrations were higher in agro-urban streams that were also characterized by DOM of lower molecular weight (higher SR values) and higher BR values (Fig. 2).
Table 2Spectral properties (positions of maximum excitation (ex) and emission (em) peaks) of the eight PARARAC components identified in this study, general description and dominant sources based on previous studies.
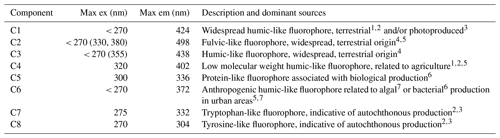
1 Stedmon and Markager (2005). 2 Yamashita et al. (2010). 3 Massicotte and Frenette (2011). 4 Graeber et al. (2012). 5 Lambert et al. (2017). 6 Stedmon et al. (2011). 7 Williams et al. (2016).
Eight PARAFAC components were identified in our study sites (Table 2, Fig. S2 in the Supplement), all of which having been already described in previous studies (Graeber et al., 2012; Lambert et al., 2017; Massicotte and Frenette, 2011; Stedmon et al., 2011; Stedmon and Markager, 2005; Williams et al., 2016; Yamashita et al., 2010). Components included three humic-like fluorophores (C1, C3, C4), one fulvic-like fluorophore (C2), two microbial protein-like fluorophores (C5 and C6), and the common tryptophane (C7) and tyrosine (C8) protein-like fluorophores. All components exhibited higher FMax values in agro-urban streams, although the most notable increases were observed for protein-like fluorophores (Fig. 3).
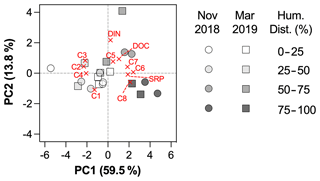
Figure 4PCA biplot, including loadings plot for the input variables and scores plot for stations. Markers are shaped according to the sampling period and colored according to a gradient of human disturbance (defined as the sum of % croplands and % urban areas, supplementary ordinal variable in the PCA).
The two first components of the PCA explained 72.4 % of the variance (Fig. 4). The first principal component (PC1) was related to protein-like components C6 to C8, DOC and SRP (positive scores), and to humic-like components C2 to C4 (negative scores), whereas the second principal component (PC2) was related to higher C5, DOC and DIN concentrations (positive scores), and lower C1 (negative score). The results of the PCA showed that the variability in DOM composition and nutrient loadings was largely driven by land uses (Fig. 4). Scores along PC1 were positively related to croplands (Pearson r = 0.49, p = 0.0265) and urban areas (Pearson r = 0.63, p = 0.0027) and negatively to forest (Pearson r = −0.72, p = 0.0003) and grasslands (Pearson r = −0.48, p = 0.0295), but not to catchment area (Pearson r = −0.04, p = 0.86), Strahler order (Pearson r = 0.13, p = 0.57) or mean elevation (Pearson r = −0.32, p = 0.18). No relationship was found between PC2 and geomorphological properties of sampling sites, suggesting an in-stream origin for the components C1 and C5. Based on the optical properties of PARAFAC components (Table 2), PC1 represented a shift in the dominant composition of DOM from terrestrial (components C2–C4) to autochthonous (C6–C8) signatures as human disturbance (croplands + urban areas) increases. PC2, however, reflected the in-stream generation of DOM through photodegradation (C1) and bacterial processing of DOM (C5).
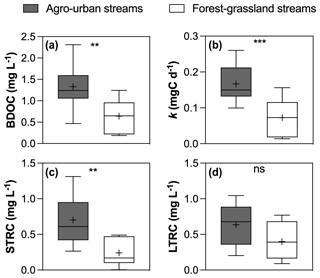
Figure 5Boxplots of (a) BDOC concentrations, (b) constant decay k, (c) STRC and (d) LTRC pools in agro-urban (grey) and forest–grassland (white) streams. The box represents the first and third quartile, the horizontal line corresponds to the median, the cross corresponds to the average, and the error bars correspond to the maximum and minimum. Mann–Whitney unpaired t tests were used to test for statistical differences: ns represents not significant, ∗ = p < 0.05, = p < 0.01, = p < 0.001, = p < 0.0001.
3.2 Kinetics of bacterial DOM degradation and consumption of low molecular weight compounds
All incubations were successfully modeled by a first-order exponential decay model (r2 = 0.98 ± 0.02), and we estimated the decay constants and the amounts of BDOC, STRC, and LTRC pools for all experiments (Fig. 5). BDOC ranged from 0.2 to 2.3 mg L−1 (mean = 1.0 ± 0.6 mg L−1), corresponding to 9.7 to 57.6 % of initial DOC (mean = 33.8 ± 11 %). A higher amount of BDOC in agro-urban streams was accompanied by higher decay constants (from 0.01 to 0.26 d−1, mean = 0.12 ± 0.07 d−1) and greater availability of STRC (from 0.01 to 1.3 mg L−1, mean = 0.5 ± 0.4 mg L−1), but no significant difference was observed regarding the amount of LTRC (from 0.1 to 1.0 mg L−1, mean = 0.5 ± 0.3 mg L−1) across stream categories. Both the STRC and LTRC pools were positively correlated with DOC concentrations (Pearson r = 0.79, p < 0.0001 and Pearson r = 0.68, p = 0.0013, respectively), leading to a positive but weak relationship between the STRC and LTRC pools (Person r = 0.49, p = 0.03). STRC was correlated to all components when expressed in FMax values, but only with protein-like components when expressed as a relative contribution to the total fluorescence signal, suggesting an autochthonous origin for this reactive C. On the contrary, the LTRC related to FMax values of C1–C4 components but not with the protein-like components, suggesting that this reactive C originated from terrestrial sources. The total amount of BDOC, decay constants, and the size of STRC were significantly related to the C6–C8 protein-like components (Fig. 6). There was however no relationship between the decay constant k and LTRC.
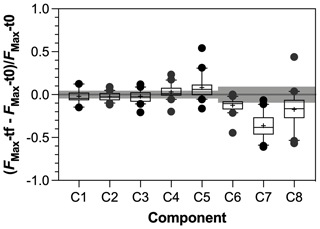
Figure 7Response ratio of PARAFAC components during incubation experiments with tf = day 28 and t0 = day 0. Grey bars represent threshold of significance above or below which significant production or consumption of component was identified. See text for details.
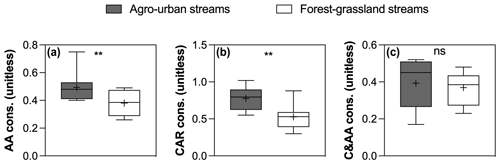
Figure 8Boxplots of (a) AA , (b) CAR, and (c) C&AA consumption in agro-urban (grey) and forest–grassland (white) streams. The box represents the first and third quartile, the horizontal line corresponds to the median, the cross corresponds to the average, and the error bars correspond to the maximum and minimum. Mann–Whitney unpaired t tests were used to test for statistical differences: ns represents not significant, ∗ = p < 0.05, = p < 0.01, = p < 0.001, = p < 0.0001.
The SR values decreased in all experiments (Fig. S3 in the Supplement), indicating an increase in the average molecular weight of DOM during incubations as low molecular weight compounds were preferentially degraded. Regarding the evolution of the different fractions of DOM during incubations, no significant changes in FMax values were observed for humic-like components during incubations (Fig. 7). Component C5, however, tended to be produced upon bacterial activity. On the contrary, the other protein-like components C6–C8 were consumed during incubations. Finally, the consumption of low molecular weights compounds was greater in agro-urban streams for AA and CAR molecules, but no difference was observed regarding the degradation of C&AA (Fig. 8).
The bacterial degradation of DOM along fluvial networks contributes to CO2 emissions toward the atmosphere (Lapierre et al., 2013). Human activities are expected to alter the role of inland waters in the global carbon cycle by disturbing DOM sources and composition (Xenopoulos et al., 2021). Keeping in mind that our study focused mainly on small size catchments during the wet period, our results highlighted that the enhanced production and accumulation of autochthonous DOM in human-disturbed streams was quickly cycled back to the atmosphere by heterotrophic bacteria. From a greenhouse gas emission perspective, the respiration of this highly reactive DOM pool may have a limited impact on C budgets in human-disturbed catchments.
4.1 Origin and biodegradability of DOM in agro-urban streams
DOM in human-disturbed streams was characterized by a lower average molecular weight compared to forest–grassland streams reflecting the influence of human activities on DOM sources and composition in the Lake Geneva basin. The increase in protein-like DOM due to a greater autochthonous productivity is a recurrent observation across aquatic ecosystems draining agricultural and urban landscapes (Stanley et al., 2012; Xenopoulos et al., 2021). Enrichment in nutrients and increased light exposure in agriculture and urban streams can promote primary production (Catford et al., 2007; Taylor et al., 2004), and greater algal biomass in our study sites was evidenced by higher Chl a concentrations (Fig. 2) and the subsequent release of protein-like components (C6–C8) related to algal DOM (Fig. 3, Table 2). Although C5 also relates to autochthonous biological production (Stedmon et al., 2011), its accumulation during incubation experiments implies that this component reflected DOM recently produced by bacterial activity (Fig. 7). Higher FMax values of C5 in human-impacted catchments thus represented a positive feedback loop where greater primary production enhanced bacterial activity that shaped DOM composition toward a more bacterial signature (Harfmann et al., 2019; Williams et al., 2010). Although of lower amplitude, higher FMax values of components associated with terrestrial inputs (C2–C4, Table 2) and/or photobleaching (C1) indicated a more efficient export of terrestrial material in agro-urban streams. As urbanization tends to limit the hydrological connection between terrestrial and aquatic ecosystems (e.g., Hosen et al. 2014), it is likely that this pattern reflected greater erosion of agricultural soils (Celik, 2005; Graeber et al., 2012).
Along with changes in DOM sources and composition, the bioavailability of DOM was strongly impacted by croplands and urbanization (Fig. 5). The positive effect of human land use on the total amount and decay constants of bioavailable DOM agrees with previous studies (Hosen et al., 2014; Parr et al., 2015), but our results further link this effect to the generation of a highly reactive pool of organic molecules derived from in stream primary production. Algae are known to be a major source of low molecular weight compounds in aquatic ecosystems through exudation and cell lysis (Kaplan and Bott, 1989) which are rapidly taken up by heterotrophic bacteria (Descy, 2002). A higher consumption of amino acids and carbohydrates concomitant with higher Chl a concentrations in agro-urban streams agrees with the generation of labile molecules derived from primary production. The loss of protein-like components paralleled by an increase in the average molecular weight during incubations also evidences the efficient degradation of this DOM from algal origin. Moreover, the strong relationships between the amount of BDOC, the decay constants k, and the size of the STRC pool with the initial contribution of protein-like components C6–C8 (Fig. 6) provide more evidence that greater DOM bioavailability in agro-urban streams resulted from greater in-stream production.
Contrary to STRC, the LTRC pools were similar across agro-urban and forest–grassland streams. In line with a recent study carried out in Swedish inland waters (Soares et al., 2019), the STRC and LTRC pools were comparable in size but no evidence of interaction was observed between the bioavailability of DOM on short and long timescales. The positive but weak relationship between STRC and LTRC likely reflected a greater amount of bioavailable DOM as human disturbance increased, as the latter enhanced both primary production and terrestrial export. Moreover, each pool related to specific DOM fractions. Similar observations were reported in Swedish rivers (Soares et al., 2019), in southern Québec (Guillemette and del Giorgio, 2011), or also in the Hudson River (del Giorgio and Pace, 2008). Overall, our findings are in good agreement with the idea that STRC is sustained by algal growth, whereas the consumption of DOC at longer timescales is rather related to terrestrial inputs of DOM (e.g., Guillemette and del Giorgio, 2011).
The humic-like components showed no significant variations during incubations (Fig. 7) despite the ability of bacterial communities to degrade complex aromatic molecules (Catalán et al., 2017; Fasching et al., 2014; Logue et al., 2016). While the stability of the C1 component during bioassays is consistent with the fact that photoproduced molecules may be resistant to further bacterial degradation (Tranvik et al., 2001), the lack of variation of C2–C4 components may reflect an equilibrium between the bacterial consumption and production of molecules contributing to the humic-like signatures. Experimental and field studies have shown that heterotrophic bacterial communities are able to produce molecules fluorescing in the region of EEMs commonly attributed to humic-like material from terrestrial origin (Amaral et al., 2016; Fox et al., 2017; Guillemette and del Giorgio, 2012). It is therefore possible that the alteration of terrestrial DOM upon bacterial activity may not have been captured by optical measurements. Addressing this point would require the characterization of DOM at the molecular level (e.g., Kim et al., 2006).
4.2 Linking bacterial respiration to DOM origin and implication of human activities on the role of inland water in the C cycle
The positive influence of enhanced primary production on the absolute amount of biodegradable DOM in human-disturbed streams agrees with previous studies (Hosen et al., 2014; Parr et al., 2015), but our results suggest that the impact regarding the role of inland waters in the context of the C cycle may be limited. Higher BR in agro-urban streams was indeed mostly related to the accumulation and mineralization of molecules generated by aquatic primary producers (Fig. 9a), although the photodegradation of terrestrial DOM could also fuel BR through the transformation of complex and aromatic molecules into compounds of lower molecular weight (Bertilsson and Tranvik, 1998) as suggested by the positive relationship between BR and C1 (Fig. 9b). Therefore, our results point to a limited effect of STRC on greenhouse gas emission as most of the C released toward the atmosphere upon bacterial respiration corresponded to atmospheric CO2 previously fixed by aquatic producers and converted into biomass.
We observed, however, an influence of human activities on the transformation of DOM along fluvial networks. Despite the large range of size of our sampling sites (Table 1), we found no relationship between Strahler order (ranging from 2 to 7) and DOM composition and reactivity. This observation contrasts with a recent study where stream order (ranging from 1 to 4) correlated negatively with humic-like DOM but positively with protein-like DOM (Shang et al., 2018), a pattern consistent with a general conceptual trend describing DOM transformations along the fluvial continuum. Indeed, the control of DOM dynamic along the river continuum is expected to shift from a dominant influence of terrestrial inputs in the headwaters to a dominant influence of in-stream removal and autochthonous production as stream order increases (Creed et al. 2015). In our study, however, human land uses had a major role in controlling DOM sources and reactivity at the basin scale (Fig. 4). The only exception was a positive correlation between Strahler order and the relative proportion of C5 (Fig. S4 in the Supplement), indicating that the degradation of autochthonous DOM in agro-urban streams led to a bacterial imprint on the DOM pool that persists along the aquatic continuum (Harfmann et al., 2019; Williams et al., 2010).
In this study, human land uses were found to alter the terrestrial and aquatic sources of freshwater DOM in an Alpine fluvial network. Enhanced primary production in human-disturbed catchments led to the accumulation of highly reactive molecules of low molecular weight which in turn stimulated bacterial respiration. Despite a dominant influence on DOM composition and reactivity at the basin scale, our study suggests that human land uses may have a limited effect in terms of net C flux exchanges between inland waters and atmosphere related to DOM mineralization by heterotrophic bacterial communities. However, further studies should perform incubation and respiration measurements at in situ conditions to improve our understanding of different bioreactive DOC pools to better constrain C budgets.
Considering that an enrichment in protein-like DOM due to greater autochthonous production is a recurrent observation in agricultural and urban catchments (Stanley et al., 2012; Xenopoulos et al., 2021), our results are likely not limited to the Lake Geneva basin. However, seasonal and longitudinal variations in DOM sources and composition should be considered along with the fact that the net effects of agriculture and urbanization on freshwater DOM vary widely depending on the environmental context (Stanley et al., 2012). While our results are in line with previous works (Hosen et al., 2014; Parr et al., 2015), they contrast with studies reporting no influence of human land uses on the bacterial consumption of DOM (Kadjeski et al., 2020; Lu et al., 2013) or higher DOM degradability in agricultural streams (Shang et al., 2018). Therefore, additional works on the links between human activities and DOM reactivity and fate are needed in order to fully assess the future of inland waters in the context of the global C cycle.
Data used in the manuscript are available in the Supplement (Tables S1 and S2).
The supplement related to this article is available online at: https://doi.org/10.5194/bg-19-187-2022-supplement.
TL conceived the study with contribution from MEP. TL, PP, and NE collected field samples. TL made the laboratory analysis. TL drafted the manuscript, which was substantially commented upon and amended by MEP, PP, and NE. All co-authors approved the manuscript.
The contact author has declared that neither they nor their co-authors have any competing interests.
Publisher's note: Copernicus Publications remains neutral with regard to jurisdictional claims in published maps and institutional affiliations.
We thank Laetitia Monbaron and Micaela Faria for assistance in the laboratory and Janine Rüegg for her comments on an initial version of the manuscript. We gratefully acknowledge the anonymous reviewers, who provided very constructive and insightful comments on the earlier versions of the manuscript.
The research was funded by the Swiss National Science Foundation grant number 200021_175530 (project CARBOGEN).
This paper was edited by Yuan Shen and reviewed by two anonymous referees.
AFNOR: NF EN ISO 6878: Qualité de l'eau Dosage du phosphore – Méthode spectrométrique au molybdate d'ammonium, AFNOR, 2005.
Amaral, V., Graeber, D., Calliari, D., and Alonso, C.: Strong linkages between DOM optical properties and main clades of aquatic bacteria, Limnol. Oceanogr., 61, 906–918, https://doi.org/10.1002/lno.10258, 2016.
Attermeyer, K., Hornick, T., Kayler, Z. E., Bahr, A., Zwirnmann, E., Grossart, H.-P., and Premke, K.: Enhanced bacterial decomposition with increasing addition of autochthonous to allochthonous carbon without any effect on bacterial community composition, Biogeosciences, 11, 1479–1489, https://doi.org/10.5194/bg-11-1479-2014, 2014.
Bengtsson, M. M., Attermeyer, K., and Catalán, N.: Interactive effects on organic matter processing from soils to the ocean: are priming effects relevant in aquatic ecosystems?, Hydrobiologia, 822, 1–17, https://doi.org/10.1007/s10750-018-3672-2, 2018.
Berggren, M. and del Giorgio, P. A.: Distinct patterns of microbial metabolism associated to riverine dissolved organic carbon of different source and quality, J. Geophys. Res.-Biogeo., 120, 989–999, https://doi.org/10.1002/2015JG002963, 2015.
Berggren, M., Laudon, H., Haei, M., Ström, L., and Jansson, M.: Efficient aquatic bacterial metabolism of dissolved low-molecular-weight compounds from terrestrial sources, ISME J., 4, 408–416, https://doi.org/10.1038/ismej.2009.120, 2010.
Bertilsson, S. and Tranvik, L. J.: Photochemically produced carboxylic acids as substrates for freshwater bacterioplankton, Limnol. Oceanogr., 43, 885–895, https://doi.org/10.4319/lo.1998.43.5.0885, 1998.
Bianchi, T. S.: The role of terrestrially derived organic carbon in the coastal ocean: A changing paradigm and the priming effect, P. Natl. Acad. Sci. USA, 108, 19473–19481, https://doi.org/10.1073/pnas.1017982108, 2011.
Bodmer, P., Heinz, M., Pusch, M., Singer, G., and Premke, K.: Carbon dynamics and their link to dissolved organic matter quality across contrasting stream ecosystems, Sci. Total Environ., 553, 574–586, https://doi.org/10.1016/j.scitotenv.2016.02.095, 2016.
Borges, A. V., Darchambeau, F., Lambert, T., Bouillon, S., Morana, C., Brouyère, S., Hakoun, V., Jurado, A., Tseng, H. C., Descy, J. P., and Roland, F. A. E.: Effects of agricultural land use on fluvial carbon dioxide, methane and nitrous oxide concentrations in a large European river, the Meuse (Belgium), Sci. Total Environ., 610–611, 342–355, https://doi.org/10.1016/j.scitotenv.2017.08.047, 2018.
Catalán, N., Casas-Ruiz, J. P., von Schiller, D., Proia, L., Obrador, B., Zwirnmann, E., and Marcé, R.: Biodegradation kinetics of dissolved organic matter chromatographic fractions, a case study in an intermittent river, J. Geophys. Res.-Biogeo., 122, 131–144, https://doi.org/10.1002/2016JG003512, 2017.
Catford, J. A., Walsh, C. J., and Beardall, J.: Catchment urbanization increases benthic microalgal biomass in streams under controlled light conditions, Aquat. Sci., 69, 511–522, https://doi.org/10.1007/s00027-007-0907-0, 2007.
Celik, I.: Land-use effects on organic matter and physical properties of soil in a southern Mediterranean highland of Turkey, Soil Till. Res., 83, 270–277, https://doi.org/10.1016/j.still.2004.08.001, 2005.
Cole, J. J., Prairie, Y. T., Caraco, N. F., McDowell, W. H., Tranvik, L. J., Striegl, R. G., Duarte, C. M., Kortelainen, P., Downing, J. A., Middelburg, J. J., and Melack, J.: Plumbing the global carbon cycle: Integrating inland waters into the terrestrial carbon budget, Ecosystems, 10, 171–184, https://doi.org/10.1007/s10021-006-9013-8, 2007.
Creed, I. F., McKnight, D. M., Pellerin, B. A., Green, M. B., Bergamaschi, B. A., Aiken, G. R., Burns, D. A., Findlay, S. E. G., Shanley, J. B., Striegl, R. G., Aulenbach, B. T., Clow, D. W., Laudon, H., McGlynn, B. L., McGuire, K. J., Smith, R. A., and Stackpoole, S. M.: The river as a chemostat: fresh perspectives on dissolved organic matter flowing down the river continuum, Can. J. Fish. Aquat. Sci., 72, 1272–1285, https://doi.org/10.1139/cjfas-2014-0400, 2015.
del Giorgio, P. A. and Davis, J.: Pattenrs in DOM lability and consumption across aquatic ecosystems, in: Aquatic ecosystems: interactivity of dissolved organic matter, edited by: Findlay, S. and Sinsabaugh, R. L., Academic Press, 399–424, 2003.
Descy, J.-P.: Phytoplankton production, exudation and bacterial reassimilation in the River Meuse (Belgium), J. Plankton Res., 24, 161–166, https://doi.org/10.1093/plankt/24.3.161, 2002.
Ekblad, A. and Bastviken, D.: Deforestation releases old carbon, Nat. Geosci., 12, 499–500, https://doi.org/10.1038/s41561-019-0394-7, 2019.
Fasching, C., Behounek, B., Singer, G. A., and Battin, T. J.: Microbial degradation of terrigenous dissolved organic matter and potential consequences for carbon cycling in brown-water streams, Sci. Rep.-UK, 4, 1–7, https://doi.org/10.1038/srep04981, 2014.
Fox, B. G., Thorn, R. M. S., Anesio, A. M., and Reynolds, D. M.: The in situ bacterial production of fluorescent organic matter; an investigation at a species level, Water Res., 125, 350–359, https://doi.org/10.1016/j.watres.2017.08.040, 2017.
Fuß, T., Behounek, B., Ulseth, A. J., and Singer, G. A.: Land use controls stream ecosystem metabolism by shifting dissolved organic matter and nutrient regimes, Freshwater Biol., 62, 582–599, https://doi.org/10.1111/fwb.12887, 2017.
Garland, J. L. and Mills, A. L.: Classification and characterization of heterotrophic microbial communities on the basis of patterns of community-level sole-carbon-source utilization, Appl. Environ. Microb., 57, 2351–2359, https://doi.org/10.1128/aem.57.8.2351-2359.1991, 1991.
Giling, D. P., Grace, M. R., Thomson, J. R., Mac Nally, R., and Thompson, R. M.: Effect of Native Vegetation Loss on Stream Ecosystem Processes: Dissolved Organic Matter Composition and Export in Agricultural Landscapes, Ecosystems, 17, 82–95, https://doi.org/10.1007/s10021-013-9708-6, 2014.
Graeber, D., Gelbrecht, J., Pusch, M. T., Anlanger, C., and von Schiller, D.: Agriculture has changed the amount and composition of dissolved organic matter in Central European headwater streams, Sci. Total Environ., 438, 435–446, https://doi.org/10.1016/j.scitotenv.2012.08.087, 2012.
Guenet, B., Danger, M., Abbadie, L., and Lacroix, G.: Priming effect: bridging the gap between terrestrial and aquatic ecology, Ecology, 91, 2850–2861, https://doi.org/10.1890/09-1968.1, 2010.
Guillemette, F. and del Giorgio, P. A.: Reconstructing the various facets of dissolved organic carbon bioavailability in freshwater ecosystems, Limnol. Oceanogr., 56, 734–748, https://doi.org/10.4319/lo.2011.56.2.0734, 2011.
Guillemette, F. and del Giorgio, P. A.: Simultaneous consumption and production of fluorescent dissolved organic matter by lake bacterioplankton, Environ. Microbiol., 14, 1432–1443, https://doi.org/10.1111/j.1462-2920.2012.02728.x, 2012.
Harfmann, J. L., Guillemette, F., Kaiser, K., Spencer, R. G. M., Chuang, C. Y., and Hernes, P. J.: Convergence of Terrestrial Dissolved Organic Matter Composition and the Role of Microbial Buffering in Aquatic Ecosystems, J. Geophys. Res.-Biogeo., 124, 3125–3142, https://doi.org/10.1029/2018JG004997, 2019.
Helms, J. R., Stubbins, A., Ritchie, J. D., Minor, E. C., Kieber, D. J., and Mopper, K.: Absorption Spectral Slopes and Slope Rations As Indicators of Molecular Weight, Source, and Photoleaching of Chromophoric Dissolved Organic Matter, Limnol. Oceanogr., 53, 955–969, https://doi.org/10.4319/lo.2008.53.3.0955, 2008.
Hosen, J. D., McDonough, O. T., Febria, C. M., and Palmer, M. A.: Dissolved organic matter quality and bioavailability changes across an urbanization gradient in headwater streams, Environ. Sci. Technol., 48, 7817–7824, https://doi.org/10.1021/es501422z, 2014.
Hu, Y., Lu, Y. H., Edmonds, J. W., Liu, C., Wang, S., Das, O., Liu, J., and Zheng, C.: Hydrological and land use control of watershed exports of dissolved organic matter in a large arid river basin in northwestern China, J. Geophys. Res.-Biogeo., 121, 466–478, https://doi.org/10.1002/2015JG003082, 2016.
Humbert, G., Parr, T. B., Jeanneau, L., Dupas, R., Petitjean, P., Akkal-Corfini, N., Viaud, V., Pierson-Wickmann, A. C., Denis, M., Inamdar, S., Gruau, G., Durand, P., and Jaffrézic, A.: Agricultural Practices and Hydrologic Conditions Shape the Temporal Pattern of Soil and Stream Water Dissolved Organic Matter, Ecosystems, 23, 1325–1343, https://doi.org/10.1007/s10021-019-00471-w, 2020.
Kadjeski, M., Fasching, C., and Xenopoulos, M. A.: Synchronous Biodegradability and Production of Dissolved Organic Matter in Two Streams of Varying Land Use, Front. Microbiol., 11, 1–14, https://doi.org/10.3389/fmicb.2020.568629, 2020.
Kaplan, L. A. and Bott, T. L.: Diel fluctuations in bacterial activity on streambed substrata during vernal algal blooms: Effects of temperature, water chemistry, and habitat, Limnol. Oceanogr., 34, 718–733, https://doi.org/10.4319/lo.1989.34.4.0718, 1989.
Kim, S., Kaplan, L. A., and Hatcher, P. G.: Biodegradable dissolved organic matter in a temperate and a tropical stream determined from ultra – high resolution mass spectrometry, Limnol. Oceanogr., 51, 1054–1063, 2006.
Kuzyakov, Y., Friedel, J. K., and Stahr, K.: Review of mechanisms and quantification of priming effects, Soil Biol. Biochem., 32, 1485–1498, https://doi.org/10.1016/S0038-0717(00)00084-5, 2000.
Lambert, T. and Perga, M.-E.: Non-conservative patterns of dissolved organic matter degradation when and where lake water mixes, Aquat. Sci., 81, 64, https://doi.org/10.1007/s00027-019-0662-z, 2019.
Lambert, T., Pierson-Wickmann, A. C., Gruau, G., Jaffrezic, A., Petitjean, P., Thibault, J. N., and Jeanneau, L.: Hydrologically driven seasonal changes in the sources and production mechanisms of dissolved organic carbon in a small lowland catchment, Water Resour. Res., 49, 5792–5803, https://doi.org/10.1002/wrcr.20466, 2013.
Lambert, T., Bouillon, S., Darchambeau, F., Morana, C., Roland, F. A. E., Descy, J. P., and Borges, A. V.: Effects of human land use on the terrestrial and aquatic sources of fluvial organic matter in a temperate river basin (The Meuse River, Belgium), Biogeochemistry, 136, 191–211, https://doi.org/10.1007/s10533-017-0387-9, 2017.
Landsman-Gerjoi, M., Perdrial, J. N., Lancellotti, B., Seybold, E., Schroth, A. W., Adair, C., and Wymore, A.: Measuring the influence of environmental conditions on dissolved organic matter biodegradability and optical properties: a combined field and laboratory study, Biogeochemistry, 149, 37–52, https://doi.org/10.1007/s10533-020-00664-9, 2020.
Lapierre, J. F., Guillemette, F., Berggren, M., and Del Giorgio, P. A.: Increases in terrestrially derived carbon stimulate organic carbon processing and CO2 emissions in boreal aquatic ecosystems, Nat. Commun., 4, 1–7, https://doi.org/10.1038/ncomms3972, 2013.
Logue, J. B., Stedmon, C. A., Kellerman, A. M., Nielsen, N. J., Andersson, A. F., Laudon, H., Lindström, E. S., and Kritzberg, E. S.: Experimental insights into the importance of aquatic bacterial community composition to the degradation of dissolved organic matter, ISME J., 10, 533–545, https://doi.org/10.1038/ismej.2015.131, 2016.
Loizeau, J. L. and Dominik, J.: Evolution of the upper Rhone river discharge and suspended sediment load during the last 80 years, Aquat. Sci., 62, 54–67, https://doi.org/10.1007/s000270050075, 2000.
Lu, Y., Bauer, J. E., Canuel, E. A., Yamashita, Y., Chambers, R. M., and Jaffé, R.: Photochemical and microbial alteration of dissolved organic matter in temperate headwater streams associated with different land use, J. Geophys. Res.-Biogeo., 118, 566–580, https://doi.org/10.1002/jgrg.20048, 2013.
Lu, Y. H., Bauer, J. E., Canuel, E. A., Chambers, R. M., Yamashita, Y., Jaffé, R., and Barrett, A.: Effects of land use on sources and ages of inorganic and organic carbon in temperate headwater streams, Biogeochemistry, 119, 275–292, https://doi.org/10.1007/s10533-014-9965-2, 2014.
Massicotte, P. and Frenette, J. J.: Spatial connectivity in a large river system: Resolving the sources and fate of dissolved organic matter, Ecol. Appl., 21, 2600–2617, https://doi.org/10.1890/10-1475.1, 2011.
Mayorga, E., Aufdenkampe, A. K., Masiello, C. A., Krusche, A. V., Hedges, J. I., Quay, P. D., Richey, J. E., and Brown, T. A.: Young organic matter as a source of carbon dioxide outgassing from Amazonian rivers, Nature, 436, 538–541, https://doi.org/10.1038/nature03880, 2005.
Murphy, K. R., Stedmon, C. A., Graeber, D., and Bro, R.: Fluorescence spectroscopy and multi-way techniques. PARAFAC, Anal. Methods-UK, 5, 6557, https://doi.org/10.1039/c3ay41160e, 2013.
Murphy, K. R., Stedmon, C. A., Wenig, P., and Bro, R.: OpenFluor– an online spectral library of auto-fluorescence by organic compounds in the environment, Anal. Methods-UK, 6, 658–661, https://doi.org/10.1039/C3AY41935E, 2014.
Parr, T. B., Cronan, C. S., Ohno, T., Findlay, S. E. G., Smith, S. M. C., and Simon, K. S.: Urbanization changes the composition and bioavailability of dissolved organic matter in headwater streams, Limnol. Oceanogr., 60, 885–900, https://doi.org/10.1002/lno.10060, 2015.
Petrone, K. C., Fellman, J. B., Hood, E., Donn, M. J., and Grierson, P. F.: The origin and function of dissolved organic matter in agro-urban coastal streams, J. Geophys. Res.-Biogeo., 116, G01028, https://doi.org/10.1029/2010JG001537, 2011.
Reche, I., Pace, M. L., and Cole, J. J.: Interactions of photobleaching and inorganic nutrients in determining bacterial growth on colored dissolved organic carbon, Microb. Ecol., 36, 270–280, https://doi.org/10.1007/s002489900114, 1998.
Shang, P., Lu, Y. H., Du, Y. X., Jaffé, R., Findlay, R. H., and Wynn, A.: Climatic and watershed controls of dissolved organic matter variation in streams across a gradient of agricultural land use, Sci. Total Environ., 612, 1442–1453, https://doi.org/10.1016/j.scitotenv.2017.08.322, 2018.
Soares, A. R. A., Lapierre, J., Selvam, B. P., Lindström, G., and Berggren, M.: Controls on dissolved organic carbon bioreactivity in river systems, Sci. Rep., 9, 14897, https://doi.org/10.1038/s41598-019-50552-y, 2019.
Stanley, E. H., Powers, S. M., Lottig, N. R., Buffam, I., and Crawford, J. T.: Contemporary changes in dissolved organic carbon (DOC) in human-dominated rivers: Is there a role for DOC management?, Freshwater Biol., 57, 26–42, https://doi.org/10.1111/j.1365-2427.2011.02613.x, 2012.
Stedmon, C. A. and Markager, S.: Resolving the variability in dissolved organic matter florescence in a temperate estuary and its catchment using PARAFAC analysis., Limnol. Oceanogr., 50, 686–697, https://doi.org/10.4319/lo.2005.50.2.0686, 2005.
Stedmon, C. A., Markager, S., and Bro, R.: Tracing dissolved organic matter in aquatic environments using a new approach to fluorescence spectroscopy, Mar. Chem., 82, 239–254, https://doi.org/10.1016/S0304-4203(03)00072-0, 2003.
Stedmon, C. A., Thomas, D. N., Papadimitriou, S., Granskog, M. A., and Dieckmann, G. S.: Using fluorescence to characterize dissolved organic matter in Antarctic sea ice brines, J. Geophys. Res.-Biogeo., 116, 1–9, https://doi.org/10.1029/2011JG001716, 2011.
Taylor, S. L., Roberts, S. C., Walsh, C. J., and Hatt, B. E.: Catchment urbanisation and increased benthic algal biomass in streams: Linking mechanisms to management, Freshwater Biol., 49, 835–851, https://doi.org/10.1111/j.1365-2427.2004.01225.x, 2004.
Tranvik, L., Bertilsson, S., and Letters, E.: Contrasting effects of solar UV radiation on dissolved organic sources for bacterial growth, Ecol. Lett., 4, 458–463, https://doi.org/10.1046/j.1461-0248.2001.00245.x, 2001.
Weber, K. P. and Legge, R. L.: One-dimensional metric for tracking bacterial community divergence using sole carbon source utilization patterns, J. Microbiol. Meth., 79, 55–61, https://doi.org/10.1016/j.mimet.2009.07.020, 2009.
Weber, K. P. and Legge, R. L.: Community-Level Physiological Profiling, in: Bioremediation, edited by: Stephen P. Cummings, Humana Press, 263–281, 2010.
Williams, C. J., Yamashita, Y., Wilson, H. F., Jaffe, R., and Xenopoulos, M. A.: Unraveling the role of land use and microbial activity in shaping dissolved organic matter characteristics in stream ecosystems, Limnol. Oceanogr., 55, 1159–1171, https://doi.org/10.4319/lo.2010.55.3.1159, 2010.
Williams, C. J., Frost, P. C., Morales-Williams, A. M., Larson, J. H., Richardson, W. B., Chiandet, A. S., and Xenopoulos, M. A.: Human activities cause distinct dissolved organic matter composition across freshwater ecosystems, Glob. Change Biol., 22, 613–626, https://doi.org/10.1111/gcb.13094, 2016.
Wilson, H. F. and Xenopoulos, M. A.: Effects of agricultural land use on the composition of fluvial dissolved organic matter, Nat. Geosci., 2, 37–41, https://doi.org/10.1038/ngeo391, 2009.
Wu, Z., Wu, W., Lin, C., Zhou, S., and Xiong, J.: Deciphering the origins, composition and microbial fate of dissolved organic matter in agro-urban headwater streams, Sci. Total Environ., 659, 1484–1495, https://doi.org/10.1016/j.scitotenv.2018.12.237, 2019.
Xenopoulos, M. A., Barnes, R. T., Boodoo, K. S., Christina, C. D. A., Nu, D. B., Kothawala, D. N., Pisani, O., Solomon, C. T., Spencer, R. G. M., Williams, C. J., and Wilson, H. F.: How humans alter dissolved organic matter composition in freshwater: relevance for the Earth's biogeochemistry, Biogeochemistry, 1–26, https://doi.org/10.1007/s10533-021-00753-3, 2021.
Yamashita, Y., Scinto, L. J., Maie, N., and Jaffé, R.: Dissolved Organic Matter Characteristics Across a Subtropical Wetland's Landscape: Application of Optical Properties in the Assessment of Environmental Dynamics, Ecosystems, 13, 1006–1019, https://doi.org/10.1007/s10021-010-9370-1, 2010.