the Creative Commons Attribution 4.0 License.
the Creative Commons Attribution 4.0 License.
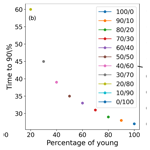
Modeling the growth and sporulation dynamics of the macroalga Ulva in mixed-age populations in cultivation and the formation of green tides
Uri Obolski
Thomas Wichard
Alvaro Israel
Alexander Golberg
Alexander Liberzon
Ulva is a widespread green algal genus with important ecological roles and promising potential as a seagriculture crop. One of the major challenges when cultivating Ulva is sudden biomass disappearance, likely caused by uncontrolled and unpredicted massive sporulation. However, the dynamics of this process are still poorly understood. In this study, we propose a mathematical model describing the biomass accumulation and degradation of Ulva, considering the potential impact of sporulation inhibitors. We developed a differential equation model describing the time evolution of Ulva biomass. Our model simulates biomass in compartments of different Ulva “age” classes, with varying growth and sporulation rates. Coupled with these classes is a differential equation describing the presence of a sporulation inhibitor, produced and secreted by the algae. Our model mimics observed Ulva dynamics. We present Ulva's biomass accumulation under different initial algae population, age distributions and sporulation rates. Furthermore, we simulate water replacement, effectively depleting the sporulation inhibitor, and examine its effects on Ulva's biomass accumulation. The model developed in this work is the first step towards understanding the dynamics of Ulva growth and degradation. Future work refining and expanding our results should prove beneficial to the ecological research and industrial growth of Ulva.
- Article
(2001 KB) - Full-text XML
-
Supplement
(231 KB) - BibTeX
- EndNote
The genus Ulva (Ulvales, Chlorophyta) comprises a group of green macroalgae, which are cosmopolitan species, both ecologically and economically. Its highly adaptive nature allows it to flourish in various environments, as can be seen from its widespread presence from the Arctic and Antarctic seas to the Equator. In natural populations, Ulva spp. are very common in littoral and sublittoral areas and also found at mesophotic depths (Spalding et al., 2016; Pyle et al., 2016). As Ulva in nature is a holobiome, its ecological role is vast and includes multiple interactions with other players of the marine ecosystems, such as protista, fungi, bacteria, viruses and various marine fauna. Ulva is highly relevant for aquaculture due to its fast growth rates and potential food, feed, materials, chemicals and energy applications. Hence, Ulva is considered a potential crop for controlled biomass production, onshore and offshore (Fernand et al., 2017). Multiple reports in the last decade addressed Ulva aquaculture alone or in multitrophic systems. In addition, Ulva biorefinery-enabling processes and technologies have made immense progress in the production of starch, protein, cellulose, ulvan, salts, methane, biocrude, biodiesel, bioethanol, and polyhydroxyalkanoates, just to mention a few (Bikker et al., 2016). Over the years, various systems including plastic sleeves, raceway ponds, tanks, dripping, ropes, nets, rafts and aerated cages have been proposed for Ulva biomass cultivation. The variation of cultivation systems ranges from closed, artificial and seawater, onshore systems with fresh seawater to near shore and far offshore production. Yet, one of the significant risks in Ulva cultivation is the sudden biomass loss when the algal tissue disintegrates and bleaches, most probably caused by uncontrolled and unpredicted massive sporulation (Gao et al., 2010; Bruhn et al., 2011).
Opposite to the controlled cultivation, Ulva green tides are massive, rapid natural accumulations of unattached green macroalgae biomass usually associated with eutrophicated marine environments.
While green tides of bladed, distromatic Ulva species are common (Fort et al., 2020; Zhao et al., 2019; Sfriso et al., 1992; Martins et al., 2001), mass occurrences of monostromatic tubular forms also occur (Blomster et al., 2002; Gao et al., 2010; Cai et al., 2021), which are the focus of this study. The impact of thalli morphology on the potential of Ulva species to generate green tides is yet to be determined.
Nevertheless, green tides seriously damage the coastal marine environment, on occasion modifying the shoreline structure, or affecting biodiversity and damaging ecosystem services such as navigation, fishery and recreation (Shan et al., 2019; Zhang et al., 2019). In addition, decaying seaweed biomass causes anoxia yielding hydrogen sulfide at toxic levels in coastal waters and on the shores (Nedergaard et al., 2002; Castel et al., 1996; Viaroli et al., 1995).
Various explanations have been proposed for the rapid accumulation and simultaneous collapse of Ulva-dominated green tides. Favorable environmental conditions for Ulva habitats, such as temperature, salinity, hydrodynamics and nutrient levels, affect the rapid biomass growth. In addition, recent studies showed that blooming leads to the selection of rapidly growing strains (Fort et al., 2020) with potentially differentially expressed genetic signatures (He et al., 2021). Furthermore, for Ulva prolifera, a strain dominating the Yellow Sea bloom, 91.6 %–96.4 % of the released spores developed into young seedlings, suggesting that 1 g fresh weight thallus was able to produce about 2.8 × 108–2.7 × 109 new younger seedlings, of free-floating biomass (Zhang et al., 2013).
Ulva sp. has a complex reproduction strategy with alternation of generations in which both isomorphic gametophytes and sporophytes coexist. The gametophytes produce biflagellated haploid gametes through mitosis while the sporophytes produce quadriflagellated haploid zoids through meiosis (Wichard, 2015).
The initiation of a green tide requires simultaneous sporulation and release of gametes of multiple thalli (Gao et al., 2010), but it can be also caused by inhibition of biomass allocation to sporulation (Hiraoka, 2021); the regulation of sporulation is involved in both cases. How this sporulation is achieved and controlled at the initial population is still a puzzling question. The simultaneous release of zoids and gametes in large numbers over a short period, combined with favorable environmental conditions, would provide the prerequisites for the formation of green tides. Indeed, mechanical or other factors, fragmentation of Ulva thalli would produce large amounts of spores giving rise to the rapid proliferation of the seaweed under field conditions. This idea was also considered because it likely supports the rapid accumulation of huge biomass of U. prolifera in the green tide observed along the Qingdao coasts in 2008 (Gao et al., 2010).
Ecological studies indicate that sporulation in Ulva is seasonal, and when it occurs a significant amount of parental biomass contributes to the massive production of swarmers (Amsler and Searles, 1980; Littler and Littler, 1980; Niesenbaum, 1988). The formation and release of swarmers is inhibited by “sporulation-inhibiting substances” excreted into the growth medium by the whole thalli, or their fragments (Nilsen and Nordby, 1975; Jónsson et al., 1985). Later studies identified sporulation inhibitors in Ulva mutabilis, Ulva linza and U. prolifera. The first sporulation inhibitor 1 (SI-1) is a glycoprotein isolated from the thalli media or the cell wall, and the second sporulation inhibitor 2 (SI-2) is a small molecular weight compound that was isolated from the inner space between the two blade cell layers (Stratmann et al., 1996; Jónsson et al., 1985; Kessler et al., 2018; Vesty et al., 2015). Importantly, removing both SIs induces the gametogenesis by washing (and mincing) the algae (Kessler et al., 2018) and activates specific transcription factors (Liu et al., 2022).
Furthermore, formed gametes were only released slowly and asynchronously in the presence of another substance known as a swarming inhibitor, the removal of which resulted in nearly immediate and complete swarming (Stratmann et al., 1996; Wichard and Oertel, 2010). This precise control of swarmer formation and release suggests that Ulva developed a tightly regulated mechanism to guarantee simultaneous release of swarmers to the environment, observed initially by Smith (1947) at the Pacific coast (Smith, 1947), probably to maximize the likelihood of sexual reproduction. Indeed, most recent studies on the floating U. prolifera showed that all tested thalli were sporophytes with sexual reproductive patterns (Zhao et al., 2019).
Notably, the decrease of SI-1 production coincides with the maturation of Ulva and finally causes sporulation (i.e., gametogenesis), first within the Ulva apical marginal zones of the thallus and subsequently within the whole alga. Unlike the SI-1, the concentration of SI-2 (per biomass) remains constant, but Ulva's sensitivity towards this inhibitor declines during aging (Stratmann et al., 1996). Unlike SI-2, the presence of SI-1 suppresses gametogenesis at all phases of the life cycle (Fig. 1). In other words, even if Ulva no longer produces enough SI-1 during aging, it is still sensitive to external SI-1 application by an aquaculture operator (Fig. 1).
Because only one of the two inhibitors is required to be present and active (Stratmann et al., 1996; Vesty et al., 2015; Kessler et al., 2018), our research concentrates on mathematical model experiments with SI-1, which is produced by the growing Ulva, released in excess in its environment and taken up by aging Ulva.
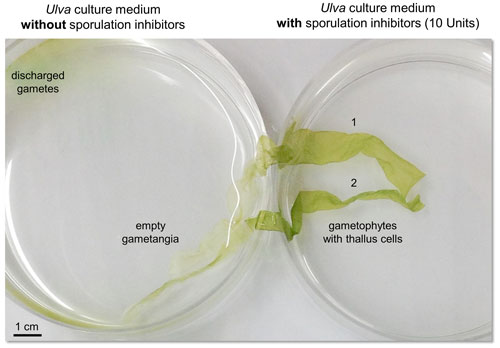
Figure 1Inhibition of gametogenesis by an externally supplied sporulation inhibitor (SI-1). Two thalli (1 and 2) were transferred into fresh UCM distributed over two Petri dishes. Mature gametophytes undergo gametogenesis upon removal of SIs by washing the thalli. Within three days, gametes were formed and released on the third day after induction (left). The addition of 10 units of SI-1 inhibited the differentiation of thallus cells into gametangia (right) (see Supplement for detailed method description according to Kessler et al., 2018).
Mathematical models are essential tools to study and predict the behavior of complex biological systems. Several models have been developed to predict seaweed biomass growth and decomposition, the behavior of harmful green tides and the seaweed biomass production in seagriculture. Indeed, long-term ecological models that predict macroalgal productivity and seasonal blooms in prone ecosystems (Martins and Marques, 2002; Solidoro et al., 1997; Ren et al., 2014; Martins et al., 2007; Port et al., 2015; Brush and Nixon, 2010; Aldridge and Trimmer, 2009; Lavaud et al., 2020; Seip, 1980; Aveytua-Alcázar et al., 2008; Duarte and Ferreira, 1997) or culture models that focus mostly on onshore photobioreactors (Friedlander et al., 1990; Oca Baradad et al., 2019) and offshore cultivation (Broch and Slagstad, 2012; Petrell et al., 1993; Hadley et al., 2015) were developed. These models, which pursue a basic understanding of the thermodynamics of individual algae thalli and photobioreactors (Zollmann et al., 2018; Lehahn et al., 2016; Martins and Marques, 2002; Lee and Ang, 1991; Seip, 1980), provide important tools to predict the productivity and seasonal environmental effects on the seaweed population dynamics. However, such models treat the macroalgae population as a bulk and do not differentiate between ages of individual thalli within the population. As discussed above, thalli age is an important factor affecting the activity of sporulation inhibitors in the alga and the ultimate release of swarmers. Furthermore, these models do not consider the possible inter-thalli chemical interactions, some of which can be based on SI-1 secreted to the environment. The production and secretion to the environment of molecules such as SI-1 could provide insights into the molecular mechanisms behind the synchronization of massive spore release at the population level – a phenomenon crucial for both green tide formation and sudden biomass disappearance in Ulva seagriculture of species such as U. mutabilis (U. compressa), U. linza and U. prolifera.
This paper aims to introduce a novel framework for the description of population dynamics and collective thalli behavior of Ulva biomass, presumably controlled by shared sporulation inhibitors. We propose that various environmental and internal biological changes on the single thallus level predetermine the ability of the individual thallus to produce, and to donate to and receive from the population environment, factors that regulate the synchronized formation and release of swarmers. A natural tool to describe this process is offered by population dynamics models, often employed to describe bacterial and animal population dynamics (Succurro and Ebenhöh, 2018; Friedman and Gore, 2017).
In the following sections we develop and simulate such a mathematical model in an attempt to characterize the dynamics of Ulva biomass formation and degradation.
The model presented below consists of n+1 ordinary differential equations (ODEs), where n is set as the number of cultivation days (also equal to the number of age group equations). ODEs 0…n−1 describe the rate of change of biomass of n discrete age classes of Ulva thalli, denoted ai, and an additional n+1 equation for the rate of change of the inhibitor I. As described previously, there are at least three types of inhibitors involved in the process of Ulva swarmers release: SI-1, SI-2 and a swarming inhibitor (SWI). We aggregate these inhibitors into a single quantity that controls the simultaneous swarmers' release from thalli, followed by the biomass decrease.
The n ODEs follow a simple discretized version of a partial differential equation of m(a,t) where m is the biomass of Ulva in controlled volume (a bioreactor or a given sea volume, for example), a is the age of algae, and t is time. As previously shown, the growth rate for thalli decreases with age in U. mutabilis (Alsufyani et al., 2017).
The following equations specify the dynamics of the biomass of each age class ai, coupled with the dynamics of the inhibitor I, in the growth environment:
The model assumes a logistic growth of biomass with a growth rate parameter ri for each algae age class ai and a carrying capacity K, defined as the maximum Ulva biomass density in the growth environment. Biomass moves between compartment ai and ai+1 at rates λi, defining the “natural aging” of algae. At each age class ai, biomass is degraded at a rate σf(I) , where σ is the maximal destruction rate, and f(I) is a monotonically decreasing function of the inhibitor I (SI-1), scaled between 0 and 1. The degradation at a rate σ=0.3 is a conservative approximation estimated from a closed aquaculture system with U. mutabilis (Alsufyani et al., 2020).
Furthermore, we define μi as the SI-1 uptake rate of each age class ai; ξ the leakage or injection of the inhibitor I, which can be managed externally to the system (e.g., washing the algae, destroying the algae, injection nutrients); and γ is the nutrient supply flux in the units of inhibitor concentration. Because this is a novel theoretical model, there were no available empirical estimates of the specific functions underlying its dynamics. Hence, whenever a rate was modeled as some monotonic function which saturates, we used the standard in ecological modeling – the logistic equation and exponential decay (Jørgensen and Bendoricchio, 2001). The model functions and parameters are summarized in Table 1.
Finally, we note that our model does not take into account addition of new thalli due to sporulation events during the simulated timeframe. This may be construed as simulating an experiment where spores freely flow out of the container used for growing the algae (e.g., Prabhu et al., 2020). Or this may serve as an approximation if the number of new thalli produced by sporulation in the simulated experimental time frame can be neglected.
Here, we study the Ulva biomass population dynamics, controlled by sporulation inhibitor production and absorption, by simulating the biomass accumulation under various scenarios for mixed-age populations, for 120 d (Stratmann et al., 1996; Alsufyani et al., 2017). In all the following simulations, we set the initial density of seaweed in the cultivation media to ain=0.2 kg m−3, and at the maximum carrying capacity the biomass can reach a density of 10 kg m−3 (K). In addition, for the initial population conditions, we denoted young thalli as the population of a0 at t=0 and old thalli as the population of a120 at t=0. From a physiological point of view, young thalli are those thalli, whose cell differentiation is controlled by SI and old thalli are those thalli, which do not produce it.
In the following plots, we simulated the behavior of populations with a mixed-age composition. Each population was labeled by the percentage of young and old thalli at initial population. Thus, for example, labels an entirely young initial population (0.2 kg m−3 of thalli with ai=0 at t=0); labels a completely old initial population (0.2 kg m−3 of thalli with ai=120 d at t=0); and represents an initial population comprised of equal parts of old and young algae (0.1 kg m−3 of thalli with ai=0 at t=0 and 0.1 kg m−3 of thalli with ai=120 d at t=0).
The biomass yield (density increase due to growth; i.e., initial density subtracted from final density) over time for various mixed-age populations is shown in Fig. 2a. The growth of mixed-aged populations with , and population mix of old and young thalli showed a typical sigmoidal growth, reaching 90 % of the maximum biomass density (9 kg m−3) at 27, 30 and 37 d, respectively. Populations with predominantly old biomass at the beginning () showed a long lag phase but exhibited positive growth, reaching 90 % of the maximum biomass density at day 87 (Fig. 2b). The population with only old algae () at the beginning of the cultivation showed degradation of the biomass from day 1 and never showed positive growth (Fig. 2b). The population with a mix of initial ages showed a small growth (positive yield) during the first 40 d but then showed biomass degradation and never reached 90 % of the maximum density (Fig. 2a, b).
As simulated in our model, such dynamics of the biomass growth could be explained by the dynamics of the production of the sporulation inhibitor (Fig. 2c). For populations , , and the rate of sporulation inhibitor generation is positive and increases over time, while for the and populations, although initial production of the sporulation inhibitor is observed, it is reduced over time (Fig. 2c). These findings show that sporulation inhibitor production by a small (20 % in our simulations) young population could potentially provide enough inhibitor to prevent the old algae population from biomass loss, thus leading to overall positive biomass production. Additionally, the rate of biomass accumulation increases with the increased fraction of the young thalli in the initial population. Nevertheless, the maximum inhibitor production decreases with time in all populations as all thalli age (Fig. 2c). To account for a less homogeneous initial population structure, the dynamics were also simulated with a different initial distributions of ages and produced qualitatively similar results (Supplement Fig. S1).
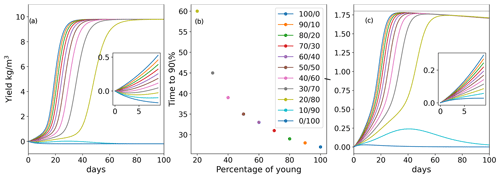
Figure 2(a) Yield for populations with various initial age mixes (biomass gain in kg m−3 up to the limit of 10 kg m−3 in a bioreactor with a starting density of 0.2 kg m−3 in total for each simulated age mix (young/old thalli in %)). (b) Ulva biomass growth kinetics as a function of the initial age distribution of thalli in the population. Values of the and populations are not presented as they did not reach 90 % of the maximal carrying capacity. In any other case, mixed populations achieved at least 90 % of the maximal biomass carrying capacity (c). Inhibitor (I) amount in the system over time. Insets in (a) and (c) present zoomed-in dynamics during the first 10 d.
As seaweeds rarely grow in closed bodies of water, where the inhibitor could accumulate continuously in the environment, we sought to stimulate the impact of inhibitor removal from the seaweed environment by water replacement. We simulate each event of water replacement as complete removal of the inhibitor produced by the seaweed during the time interval from the previous water replacement. Figure 3a shows the dynamics of various initial age-mixed populations yields when the inhibitor is removed by water replacement. In the scenario where water is replaced every 14 d, only few, relatively “young” populations (, , and ) achieved the 90 % of the maximum yield. Moreover, the time to reach this yield level increased from 27–30 d (without replacement for these four age groups) to 37–40 d, in the , , and populations, respectively (Fig. 3b). The growth yield shows fluctuating dynamics, showing in the initial overall growth for populations with predominantly young thalli during the first 60 cultivation days, followed by the overall reduction of the yield in the aging populations. Interestingly, these fluctuations yield dynamics that are similar to those previously reported by us during a 12-month offshore cultivation work with Ulva harvesting every week (Chemodanov et al., 2018). Although many other factors could be at play, it is possible that the weekly removal of the whole seaweed biomass from the sea and cages for weighting also removed the sporulation inhibitor accumulated in the boundary layer near the thalli. This suggestion, of course, requires further detailed experiments investigating the ability to monitor the dynamics of sporulation inhibitors production and accumulation/diffusion in the thalli environment. In our simulations, increasing the frequency of water exchange, and thus removal of SI-1 (Fig. 3c), reduced the ability of populations with a large portion of old algae to produce a positive yield during the whole cultivation period (Fig. 3a).
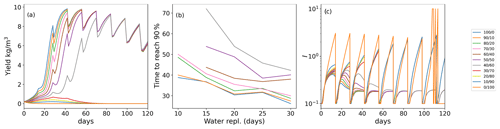
Figure 3(a) Yield (biomass gain in kg m−3 up to the limit of 10 kg m−3 in a bioreactor with a starting density of 0.2 g m−3) for populations with various initial age mixes (% of young/old thalli) with 14 d water replacement frequency. (b) Inhibitor (I) production in the population of the time with 14 d of water replacement frequency. (c) Ulva biomass growth kinetics as a function of the initial age distribution of thalli in the population with various frequencies of water replacement.
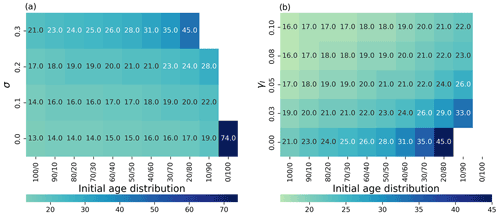
Figure 4(a) Time to achieve 90 % of the maximum carrying capacity as a heatmap (color represents the time in days) depending on the initial age distribution (x axis; (% of young/old thalli)) and the degradation parameter σi (y axis). (b) Time to 90 % as a heatmap (color represents the time in days; note the different scales in a and b) depending on the initial age distribution (x axis) and the addition of the external inhibitor γ (y axis). White color means that the population never achieves 90 % of the maximum carrying capacity.
As seaweed in the natural environment usually lives in high-energy conditions, we also studied the coupled effects of degradation (σ) and population age distribution on the ability of the population biomass yield (Fig. 4a). Higher rates of degradation prevent positive yields in all mixed-age populations. Lower degradation affects a smaller portion of the population with higher initial portions of young thalli (Fig. 4a) showing again the regenerative ability of the populations with high growth (lower sporulation) capabilities.
We investigated the impact of direct addition of the sporulation inhibitor to the seaweed growth media computationally (Fig. 4b). Adding an external sporulation inhibitor (up to 0.1) reduced the time to achieve 90 % of the maximum yield from 21 d (without inhibitor addition) to 16 d for group, 24 d (without inhibitor addition) to 17 d for group, from 28 d (without inhibitor addition) to 18 d for the group and from 45 d (without inhibitor addition) to 21 d for the group. No effects at this maximum concentration have been observed for the group.
In this study, we aimed to further understand the growth and sporulation dynamics of Ulva using a mathematical model. We found that successful accumulation of Ulva biomass depends on the age distribution of the Ulva thalli of a given seed stock, where older starter populations produce lower yields at a given time. However, this age-dependent effect can be mitigated, leading to prolonged maintenance of Ulva's aquacultures, by external addition of sporulation inhibitors. We note that our modeling study is a first attempt to uncover the mechanism underlying the heterogeneity of vegetative growth stability in different Ulva populations. At this point, we developed a general model, which is not species specific. Indeed, several studies have revealed that the SIs of U. linza, U. compressa (U. mutabilis) and U. intestinalis are exchangeable (Vesty et al., 2015; Steinhagen et al., 2019; Stratmann et al., 1996). We assume the more closely related the Ulva species are, the more likely the SIs will be interchangeable between the species. The SI-1, in fact, cannot be swapped between U. compressa and U. rigida (Stratmann et al., 1996).
Bioassays with SI-1 have supported the finding of the model that age-mixed populations are more stable than uniform ones. When purified SI-1 is added to mature and induced Ulva thalli, it can be still perceived by Ulva and used to regulate gametogenesis such in the case of aging cultures. We thus conclude that the sporulation event is delayed or even inhibited in age-mixed cultures composed of young, smaller thalli and old, larger thalli.
Overall the sporulation phenomenon creates unique constraints on the age structure of Ulva populations. In higher plants, sexual reproduction and vegetative propagation compete for nutrients, but the competition may be mitigated by separating these processes through time (Evans and Black, 1993). However, in Ulva, the whole thallus can be transformed into gametangia and sporangia while flowering plants assign only a specific portion of biomass to reproductive structures. Ulva thus requires a strict regulation of sporulation, e.g., through the age-dependent production of SI. Only if the SI-1 synthesis ceases during the Ulva's development cycle and its concentration falls below a critical threshold concentration, gametogenesis is induced at positions of the blade where the SI-2 concentration between the cell layers is also sufficiently low or not perceived anymore (Stratmann et al., 1996). Our findings thus imply that the more SI provided by young algae in mixed cultures, the higher the growth rate and biomass yields. As purified SIs are not yet widely available in large quantities, the use of mixed-aged cultures can be an important tool to maintain them at adequate nutrient levels, e.g., in integrated multi trophic aquaculture. The modeling of Ulva's growth indicates the importance of SI-producing algae for sustainable and successful seagriculture and paves the way for a better understanding of the green tide formation in coastal areas.
The model creation process is explained in detail in the Methods. Code for creating the figures is available upon request. All the analyses in the paper can be recreated through the code deposited here: https://github.com/alexliberzonlab/mixed_age_algae_population_modeling/tree/1.0 (Obolski and Liberzon, 2022).
The supplement related to this article is available online at: https://doi.org/10.5194/bg-19-2263-2022-supplement.
AG and AL conceived the initial idea for the study; UO, AG and AL designed the study and the mathematical model; AL produced the results; TW produced the experimental results; all authors interpreted the results and wrote the manuscript.
The contact author has declared that neither they nor their co-authors have any competing interests.
Publisher’s note: Copernicus Publications remains neutral with regard to jurisdictional claims in published maps and institutional affiliations.
Ralf Kessler (Friedrich Schiller University Jena, Germany) is acknowledged for carrying out the shown biotest with Ulva.
The authors thank the Israel Ministry of Health (grant no. 3-16052) for the support. This article is based upon work from COST Action CA20106, supported by COST (European Cooperation in Science and Technology, https://www.cost.eu, last access: 31 March 2022). This research has been supported by the Deutsche Forschungsgemeinschaft through grant no. SFB 1127/2 ChemBioSys–239748522 (Thomas Wichard).
This paper was edited by Andrew Thurber and reviewed by Erik-jan Malta and one anonymous referee.
Aldridge, J. and Trimmer, M.: Modelling the distribution and growth of “problem” green seaweed in the Medway estuary, UK, in: Eutrophication in Coastal Ecosystems, Springer, 107–122, 2009.
Alsufyani, T., Weiss, A., and Wichard, T.: Time course exo-metabolomic profiling in the green marine macroalga Ulva (Chlorophyta) for identification of growth phase-dependent biomarkers, Mar. Drugs, 15, 14, https://doi.org/10.3390/md15010014, 2017.
Alsufyani, T., Califano, G., Deicke, M., Grueneberg, J., Weiss, A., Engelen, A. H., Kwantes, M., Mohr, J. F., Ulrich, J. F., and Wichard, T.: Macroalgal–bacterial interactions: identification and role of thallusin in morphogenesis of the seaweed Ulva (Chlorophyta), J. Exp. Bot., 71, 3340–3349, 2020.
Amsler, C. D. and Searles, R. B.: Vertical distribution of seaweed spores in a water column offshore of North Carolina, J. Phycol., 16, 617–619, 1980.
Aveytua-Alcázar, L., Camacho-Ibar, V. F., Souza, A. J., Allen, J., and Torres, R.: Modelling Zostera marina and Ulva spp. in a coastal lagoon, Ecol. Model., 218, 354–366, 2008.
Bikker, P., van Krimpen, M. M., van Wikselaar, P., Houweling-Tan, B., Scaccia, N., van Hal, J. W., Huijgen, W. J., Cone, J. W., and López-Contreras, A. M.: Biorefinery of the green seaweed Ulva lactuca to produce animal feed, chemicals and biofuels, J. Appl. Phycol., 28, 3511–3525, 2016.
Blomster, J., Bäck, S., Fewer, D. P., Kiirikki, M., Lehvo, A., Maggs, C. A., and Stanhope, M. J.: Novel morphology in Enteromorpha (Ulvophyceae) forming green tides, Am. J. Bot., 89, 1756–1763, 2002.
Broch, O. J. and Slagstad, D.: Modelling seasonal growth and composition of the kelp Saccharina latissima, J. Appl. Phycol., 24, 759–776, 2012.
Bruhn, A., Dahl, J., Nielsen, H. B., Nikolaisen, L., Rasmussen, M. B., Markager, S., Olesen, B., Arias, C., and Jensen, P. D.: Bioenergy potential of Ulva lactuca: biomass yield, methane production and combustion, Bioresour. Technol., 102, 2595–2604, 2011.
Brush, M. J. and Nixon, S. W.: Modeling the role of macroalgae in a shallow sub-estuary of Narragansett Bay, RI (USA), Ecol. Model., 221, 1065–1079, 2010.
Cai, C., Gu, K., Zhao, H., Steinhagen, S., He, P., and Wichard, T.: Screening and verification of extranuclear genetic markers in green tide algae from the Yellow Sea, PloS One, 16, e0250968, https://doi.org/10.1371/journal.pone.0250968, 2021.
Castel, J., Caumette, P., and Herbert, R.: Eutrophication gradients in coastal lagoons as exemplified by the Bassin d'Arcachon and the Etang du Prévost, Hydrobiologia, 329, ix–xxviii, 1996.
Chemodanov, A., Jinjikhashvily, G., Habiby, O., Liberzon, A., Israel, A., Yakhini, Z., and Golberg, A.: Net primary productivity, biofuel production and CO2 emissions reduction potential of Ulva sp.(Chlorophyta) biomass in a coastal area of the Eastern Mediterranean, Energy Convers. Manag., 166, 772–772, 2018.
Duarte, P. and Ferreira, J.: A model for the simulation of macroalgal population dynamics and productivity, Ecol. Model., 98, 199–214, 1997.
Evans, R. and Black, R. A.: Growth, photosynthesis, and resource investment for vegetative and reproductive modules of Artemisia tridentata, Ecology, 74, 1516–1528, 1993.
Fernand, F., Israel, A., Skjermo, J., Wichard, T., Timmermans, K. R., and Golberg, A.: Offshore macroalgae biomass for bioenergy production: Environmental aspects, technological achievements and challenges, Renew. Sustain. Energy Rev., 75, 35–45, 2017.
Fort, A., Mannion, C., Fariñas-Franco, J. M., and Sulpice, R.: Green tides select for fast expanding Ulva strains, Sci. Total Environ., 698, 134337, https://doi.org/10.1016/j.scitotenv.2019.134337, 2020.
Friedlander, M., Galai, N., and Farbstein, H.: A model of seaweed growth in an outdoor culture in Israel, Hydrobiologia, 204, 367–373, 1990.
Friedman, J. and Gore, J.: Ecological systems biology: The dynamics of interacting populations, Curr. Opin. Syst. Biol., 1, 114–121, 2017.
Gao, S., Chen, X., Yi, Q., Wang, G., Pan, G., Lin, A., and Peng, G.: A strategy for the proliferation of Ulva prolifera, main causative species of green tides, with formation of sporangia by fragmentation, PLoS One, 5, e8571, https://doi.org/10.1371/journal.pone.0008571, 2010.
Hadley, S., Wild-Allen, K., Johnson, C., and Macleod, C.: Modeling macroalgae growth and nutrient dynamics for integrated multi-trophic aquaculture, J. Appl. Phycol., 27, 901–916, 2015.
He, Y., Shen, S., Yu, D., Wang, Y., Yin, J., Wang, Z., and Ye, Y.: The Ulva prolifera genome reveals the mechanism of green tides, J. Oceanol. Limnol., 39, 1458–1470, 2021.
Hiraoka, M.: Massive Ulva green tides caused by inhibition of biomass allocation to sporulation, Plants, 10, 2482, https://doi.org/10.3390/plants10112482, 2021.
Jónsson, S., Laur, M.-H., and Pham-Quang, L.: Mise en évidence de différents types de glycoprotéines dans un extrait inhibiteur de la gamétogenèse chez Enteromorpha prolifera, Chlorophycée marine, Cryptogam. Algol., 6, 253–264, 1985.
Jørgensen, S. E. and Bendoricchio, G.: Fundamentals of ecological modelling, Elsevier, Book ISBN: 9780080532103, 2001.
Kessler, R. W., Alsufyani, T., and Wichard, T.: Purification of sporulation and swarming inhibitors from Ulva: application in algal life cycle controlling, in: Protocols for macroalgae research, edited by: Charrier, B., Wichard, T., and Reddy, C. R. K., CRC Press, Francis & Taylor Group, Boca Raton, eBook ISBN9781351132954, 139–157, 2018.
Lavaud, R., Filgueira, R., Nadeau, A., Steeves, L., and Guyondet, T.: A Dynamic Energy Budget model for the macroalga Ulva lactuca, Ecol. Model., 418, 108922, https://doi.org/10.1016/j.ecolmodel.2019.108922, 2020.
Lee, C. and Ang Jr., P.: A simple model for seaweed growth and optimal harvesting strategy, Ecol. Model., 55, 67–74, 1991.
Lehahn, Y., Ingle, K. N., and Golberg, A.: Global potential of offshore and shallow waters macroalgal biorefineries to provide for food, chemicals and energy: feasibility and sustainability, Algal Res., 17, 150–160, 2016.
Littler, M. M. and Littler, D. S.: The evolution of thallus form and survival strategies in benthic marine macroalgae: field and laboratory tests of a functional form model, Am. Nat., 116, 25–44, 1980.
Liu, X., Blomme, J., Bogaert, K., D'hondt, S., Wichard, T., Thomas, Deforce, D., Van Nieuwerburgh, F., and De Clerck, O.: Transcriptional profiling of gametogenesis in the green seaweed Ulva mutabilis identifies an RWP-RK transcription factors linked to reproduction, BMC Plant Biol., 22, 19, https://doi.org/10.1186/s12870-021-03361-3, 2022.
Martins, I. and Marques, J.: A model for the growth of opportunistic macroalgae (Enteromorpha sp.) in tidal estuaries, Estuar. Coast. Shelf Sci., 55, 247–257, 2002.
Martins, I., Pardal, M., Lillebø, A., Flindt, M., and Marques, J.: Hydrodynamics as a major factor controlling the occurrence of green macroalgal blooms in a eutrophic estuary: a case study on the influence of precipitation and river management, Estuar. Coast. Shelf Sci., 52, 165–177, 2001.
Martins, I., Lopes, R., Lillebø, A., Neto, J., Pardal, M., Ferreira, J., and Marques, J.: Significant variations in the productivity of green macroalgae in a mesotidal estuary: implications to the nutrient loading of the system and the adjacent coastal area, Mar. Pollut. Bull., 54, 678–690, 2007.
Nedergaard, R. I., Risgaard-Petersen, N., and Finster, K.: The importance of sulfate reduction associated with Ulva lactuca thalli during decomposition: a mesocosm experiment, J. Exp. Mar. Biol. Ecol., 275, 15–29, 2002.
Niesenbaum, R. A.: The ecology of sporulation by the macroalga Ulva lactuca L.(Chlorophyceae), Aquat. Bot., 32, 155–166, 1988.
Nilsen, G. and Nordby, Ø.: A sporulation-inhibiting substance from vegetative thalli of the green alga Ulva mutabilis, Føyn, Planta, 125, 127–139, 1975.
Obolski, U. and Liberzon, A.: Modeling the growth and sporulation dynamics of the macroalga Ulva in mixed-age populations in cultivation and the formation of green tides, GitHub [code], https://github.com/alexliberzonlab/mixed_age_algae_population_modeling/tree/1.0, last access: 6 April 2022.
Oca Baradad, J., Cremades Ugarte, J., Jiménez de Ridder, P., Pintado, J., and Masaló Llorà, I.: Culture of the seaweed Ulva ohnoi integrated in a Solea senegalensis recirculating system, J. Appl. Phycol., 31, 1–7, 2019.
Petrell, R., Tabrizi, K. M., Harrison, P., and Druehl, L.: Mathematical model of Laminaria production near a British Columbian salmon sea cage farm, J. Appl. Phycol., 5, 1–14, 1993.
Port, A., Bryan, K. R., Pilditch, C. A., Hamilton, D. P., and Bischof, K.: Algebraic equilibrium solution of tissue nitrogen quota in algae and the discrepancy between calibrated parameters and physiological properties, Ecol. Model., 312, 281–291, 2015.
Prabhu, M., Levkov, K., Levin, O., Vitkin, E., Israel, A., Chemodanov, A., and Golberg, A.: Energy efficient dewatering of far offshore grown green macroalgae Ulva sp. biomass with pulsed electric fields and mechanical press, Bioresour. Technol., 295, 122229, https://doi.org/10.1016/j.biortech.2019.122229, 2020.
Pyle, R. L., Boland, R., Bolick, H., Bowen, B. W., Bradley, C. J., Kane, C., Kosaki, R. K., Langston, R., Longenecker, K., Montgomery, A., and others: A comprehensive investigation of mesophotic coral ecosystems in the Hawaiian Archipelago, PeerJ, 4, e2475, https://doi.org/10.7717/peerj.2475, 2016.
Ren, J. S., Barr, N. G., Scheuer, K., Schiel, D. R., and Zeldis, J.: A dynamic growth model of macroalgae: application in an estuary recovering from treated wastewater and earthquake-driven eutrophication, Estuar. Coast. Shelf Sci., 148, 59–69, 2014.
Seip, K. L.: A computational model for growth and harvesting of the marine alga Ascophyllum nodosum, Ecol. Model., 8, 189–199, 1980.
Sfriso, A., Pavoni, B., Marcomini, A., and Orio, A.: Macroalgae, nutrient cycles, and pollutants in the Lagoon of Venice, Estuaries, 15, 517–528, 1992.
Shan, J., Li, J., and Xu, Z.: Estimating ecological damage caused by green tides in the Yellow Sea: A choice experiment approach incorporating extended theory of planned behavior, Ocean Coast. Manag., 181, 104901, https://doi.org/10.1016/j.ocecoaman.2019.104901, 2019.
Smith, G. M.: On the reproduction of some Pacific coast species of Ulva, Am. J. Bot., 1947, 34, 80–87, 1947.
Solidoro, C., Pecenik, G., Pastres, R., Franco, D., and Dejak, C.: Modelling macroalgae (Ulva rigida) in the Venice lagoon: Model structure identification and first parameters estimation, Ecol. Model., 94, 191–206, 1997.
Spalding, H. L., Conklin, K. Y., Smith, C. M., O'Kelly, C. J., and Sherwood, A. R.: New Ulvaceae (Ulvophyceae, Chlorophyta) from mesophotic ecosystems across the Hawaiian archipelago, J. Phycol., 52, 40–53, 2016.
Steinhagen, S., Barco, A., Wichard, T., and Weinberger, F.: Conspecificity of the model organism Ulva mutabilis and Ulva compressa (Ulvophyceae, Chlorophyta), J. Phycol., 55, 25–36, 2019.
Stratmann, J., Paputsoglu, G., and Oertel, W.: Differentiation of Ulva mutabilis (Chlorophyta) gametangia and gamete release are controlled by extracellular inhibitors, J. Phycol., 32, 1009–1021, 1996.
Succurro, A. and Ebenhöh, O.: Review and perspective on mathematical modeling of microbial ecosystems, Biochem. Soc. Trans., 46, 403–412, 2018.
Vesty, E. F., Kessler, R. W., Wichard, T., and Coates, J. C.: Regulation of gametogenesis and zoosporogenesis in Ulva linza (Chlorophyta): comparison with Ulva mutabilis and potential for laboratory culture, Front. Plant Sci., 6, 15, https://doi.org/10.3389/fpls.2015.00015, 2015.
Viaroli, P., Bartoli, M., Bondavalli, C., and Naldi, M.: Oxygen fluxes and dystrophy in a coastal lagoon colonized by Ulva rigida (Sacca di Goro, Po River Delta, northern Italy), Fresenius Environ. Bull., 4, 381–386, 1995.
Wichard, T.: Exploring bacteria-induced growth and morphogenesis in the green macroalga order Ulvales (Chlorophyta), Front. Plant Sci., 6, 86, https://doi.org/10.3389/fpls.2015.00086, 2015.
Wichard, T. and Oertel, W.: Gametogenesis and gamete release of Ulva mutabilis and Ulva lactuca (Chlorophyta): regulatory effects and chemical characterization of the “swarming inhibitor”, J. Phycol., 46, 248–259, 2010.
Zhang, J., Huo, Y., Yu, K., Chen, Q., He, Q., Han, W., Chen, L., Cao, J., Shi, D., and He, P.: Growth characteristics and reproductive capability of green tide algae in Rudong coast, China, J. Appl. Phycol., 25, 795–803, 2013.
Zhang, Y., He, P., Li, H., Li, G., Liu, J., Jiao, F., Zhang, J., Huo, Y., Shi, X., Su, R., et al.: Ulva prolifera green-tide outbreaks and their environmental impact in the Yellow Sea, China, Natl. Sci. Rev., https://doi.org/10.1093/nsr/nwz026, 2019.
Zhao, X., Cui, J., Zhang, J., Shi, J., Kang, X., Liu, J., Wen, Q., and He, P.: Reproductive strategy of the floating alga Ulva prolifera in blooms in the Yellow Sea based on a combination of zoid and chromosome analysis, Mar. Pollut. Bull., 146, 584–590, 2019.
Zollmann, M., Traugott, H., Chemodanov, A., Liberzon, A., and Golberg, A.: Exergy efficiency of solar energy conversion to biomass of green macroalgae Ulva (Chlorophyta) in the photobioreactor, Energy Convers. Manag., 167, 125–133, 2018.