the Creative Commons Attribution 4.0 License.
the Creative Commons Attribution 4.0 License.
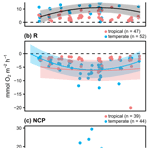
Reviews and syntheses: Spatial and temporal patterns in seagrass metabolic fluxes
Melissa Ward
Tye L. Kindinger
Heidi K. Hirsh
Tessa M. Hill
Brittany M. Jellison
Sarah Lummis
Emily B. Rivest
George G. Waldbusser
Brian Gaylord
Seagrass meadow metabolism has been measured for decades to gain insight into ecosystem energy, biomass production, food web dynamics, and, more recently, to inform its potential in ameliorating ocean acidification (OA). This extensive body of literature can be used to infer trends and drivers of seagrass meadow metabolism. Here, we synthesize the results from 56 studies reporting in situ rates of seagrass gross primary productivity, respiration, and/or net community productivity to highlight spatial and temporal variability in oxygen (O2) fluxes. We illustrate that daytime net community production (NCP) is positive overall and similar across seasons and geographies. Full-day NCP rates, which illustrate the potential cumulative effect of seagrass beds on seawater biogeochemistry integrated over day and night, were also positive overall but were higher in summer months in both tropical and temperate ecosystems. Although our analyses suggest seagrass meadows are generally autotrophic, the effects on seawater oxygen are relatively small in magnitude. We also find positive correlations between gross primary production and temperature, although this effect may vary between temperate and tropical geographies and may change under future climate scenarios if seagrasses approach thermal tolerance thresholds. In addition, we illustrate that periods when full-day NCP is highest could be associated with lower nighttime O2 and increased diurnal variability in seawater O2. These results can serve as first-order estimates of when and where OA amelioration by seagrasses may be likely. However, improved understanding of variations in ratios and increased work directly measuring metabolically driven alterations in seawater pH will further inform the potential for seagrass meadows to serve in this context.
- Article
(2368 KB) -
Supplement
(3011 KB) - BibTeX
- EndNote
- Included in Encyclopedia of Geosciences
Seagrasses are productive marine macrophytes, lauded for numerous ecosystem functions including habitat formation for diverse species assemblages, stabilization of marine sediments, storm surge buffering, and many others. Seagrass meadow productivity has been studied for decades, with community metabolism work published as early as 1956 (Odum, 1956). Foundational work assessed the role of seagrass community metabolism in local food web dynamics and ecosystem energy exchanges (e.g., Odum and Hoskin, 1958; Murray and Wetzel, 1987). In recent decades, seagrass metabolism has been highlighted for its potential role in reducing aqueous CO2 concentrations, thereby locally mitigating ocean acidification (OA) (e.g., Hendriks et al., 2014; Ricart et al., 2021) – an ecosystem function that has led to renewed interest in metabolism research.
At the global scale, seagrass meadows are considered net autotrophic (Duarte et al., 2010), suggesting that gross primary production (GPP, defined here as the rate of oxygen production) should exceed respiration (R, defined here as the rate of oxygen consumption, determined using incubations or measurements in the dark) on average. Past research has suggested that net autotrophic systems (or positive NCP, defined here as the GPP minus R, when both photosynthesis and respiration are accounted for) are likely to export biomass or energy (e.g., Duarte and Cebrián, 1996) or may serve to mitigate OA though the resulting water chemistry modifications (e.g., Unsworth et al., 2012; Ricart et al., 2021). However, periods of net autotrophy in seagrass meadows can be intermittent or transient in time. In particular, metabolic variability can be expected on several different temporal scales, resulting in subsequent variability in ambient water chemistry including dissolved oxygen and carbonate chemistry parameters. First, daytime and nighttime patterns in photosynthesis and respiration can cause substantial diurnal variability on ambient seawater chemistry. Moreover, tidal cycles and local hydrodynamics may alter the chemical impact of these processes on hourly timescales as well (Cyronak et al., 2018; Koweek et al., 2018). Local hydrodynamics significantly influence the time that a water mass experiences chemical alteration by a seagrass meadow, as well as the water depth though which light must penetrate to reach the seagrass canopy and the volume of water that must be modified, and therefore the magnitude of the change. On a longer timescale, seasonal patterns in temperature and light can also cause substantial seasonal variability in the biomass and productivity of submerged aquatic vegetation (Maher and Eyre, 2011; Clavier et al., 2014; Ricart et al., 2021). Spatial metabolic variation may also interact with temporal variation, further complicating resulting meadow metabolism. For example, diurnal variability in O2 fluxes and seawater chemistry could be more pronounced in geographies that support higher daytime GPP and nighttime R (Duarte et al., 2010). In addition, seasonal variability in NCP could be more pronounced in locations where there are larger fluctuations in light and other environmental drivers, such as high-latitude or temperate ecosystems.
Because local OA mitigation will depend on the community metabolism of the vegetation, accounting for spatial and temporal variability in metabolism is especially important for managers considering local OA mitigation strategies. Specifically, understanding whether GPP, R, and NCP vary predictably across time and in different geographies can provide important, first-order information about when and where local OA mitigation approaches might be effective. It can further inform whether potential OA mitigation aligns with windows of vulnerability for sensitive living resources or how variability is integrated though time by important species. Although potential local OA mitigation is caused by changes in the dissolved inorganic carbon (DIC) in seawater, which can be influenced by several other important biological and physical factors (Koweek et al., 2018; James et al., 2020), the relationship between O2 fluxes associated with seagrass metabolism and seawater DIC is roughly proportional (i.e., if O2 production goes up, DIC in seawater will go down). Variability in these proportions can be introduced by other environmental processes such as calcification and aerobic metabolism (Barrón et al., 2006; Van Dam et al., 2019). However, empirical studies of changes in seawater DIC are currently limited, and the comprehensive literature on seagrass community metabolism on O2 fluxes can provide important spatial and temporal context for managers interested in seagrass metabolism and its potential for local OA mitigation.
Here, we synthesize published studies of seagrass metabolism to characterize the variability in O2 fluxes associated with GPP, R, and NCP across seasons and geographies. In recognition of the substantial temporal diel variability in O2 fluxes associated with daytime NCP and nighttime respiration, as well as the uncertainty in our understanding of how this temporal variability translates to pH variability and is integrated by vulnerable marine organisms associated with seagrass beds, we focus on both hourly rates of NCP taken during peak daylight hours and full-day NCP. Hourly measurements of NCP collected during peak daylight hours can provide insight into the maximum elevation of seawater O2. Similarly, hourly measurements of respiration provide insight into the potential maximum depression of nighttime O2. In contrast, measurements of NCP taken over longer time periods or that incorporate the full 24 h cycle (full-day NCP) provide insight into the cumulative effect of seagrass on seawater chemistry. In particular, we tested (1) if seasonal variability is present in daytime and full-day O2 fluxes, (2) if the temporal variation in O2 fluxes varies among tropical and temperate geographies, and (3) how much of the residual variation in O2 fluxes not accounted for by seasons or geography can be attributed to variation in temperature and aboveground biomass of the seagrass assemblage.
We conducted a literature search for in situ measurements of seagrass community metabolism using the Web of Science. Search terms included [seagrass or eelgrass or submerged aquatic vegetation] and [metabol* or carbon/oxygen fluxes or community prod* community resp* or benthic incubation chambers or primary prod* or carbon* chemistry or pH]. For each paper, we then searched the literature cited for more applicable studies, as well as any papers listed in the Web of Science that cited the study in question. In addition, we searched the datasets used by Duarte et al. (2010) and Unsworth et al. (2012). Studies were included when O2 fluxes of a seagrass-associated community were measured in situ. While the study initially aimed to include both O2 and carbon fluxes, this analysis is limited to seagrass community metabolism studies directly measuring O2 due to the relative paucity of carbon flux studies and their methodological differences. This included studies using a variety of methods, including incubation chambers, eddy correlation techniques, mass balance estimates, and isotope enrichment, among others. Studies that were published prior to 1 January 2020 were included.
Within a single study, regardless of the methods used, each deployment/set of measurements was included as a data point in the synthesis when deployments/measurements were repeated across different locations, months, or species. We collected measurements of GPP, R, or NCP from each study using data reported in the text, tables, or graphs using software (GraphClick or Data Thief), or provided by the authors by request. In addition, we recorded information on other metadata associated with the study (i.e., species, location, temperature, month the study was conducted, etc.). We classified each study as either tropical or temperate based on the designation in the primary study and then classified the metabolic measurements as either (a) hourly rates or (b) daily rates. This classification was defined by the reporting within the studies (i.e., the primary authors either reported hourly or daily rates), but the difference in reporting was ostensibly due to differences in the length of the deployment used to measure metabolism (e.g., <4 h deployment is an hourly rate, ∼12–24 h deployment is a daily rate). The shorter hourly deployments were usually taken during peak daylight hours, which we used to infer the potential for any daytime local O2 elevation. In contrast, we use the daily rates to infer the cumulative, full-day local O2 fluxes – indicative of NCP. It is important to note that positive daily NCP can still encompass marked diel or diurnal variability in O2 fluxes and carbonate chemistry. This variability could still prove deleterious to seagrass-associated species during transient periods of low O2 or pH.
Positive NCP values represent net autotrophy and O2 fluxes to the water column from the seagrass tissue, and negative NCP values represent net heterotrophy and O2 fluxes from the water column to the seagrass tissue. For chamber-based studies, incubation chambers were all placed on the benthos (no mid-water column chambers) and had volumes ranging from 1.1 to 350 L. We interpret that these measurements captured seagrass community metabolism (benthos, seagrass, and water column) and could be compared with other community-based approaches. Nonetheless, we recognize variation could be introduced by methodological differences and differences in chamber volume. To assess differences in estimates based on the methods used to measure metabolism, we plotted the O2 fluxes as a function of study type. Based on these plots (Fig. S1 in the Supplement), we decided to perform separate analyses for studies that used the “mass balance” approach (Odum, 1956) versus other methods (e.g., incubations, eddy correlations). The studies using the mass balance approach may overrepresent water column productivity over benthic productivity compared to other methods and, as such, displayed a much higher range and magnitude of responses than those measured by other methods (see “Methodological analyses” in “Results” below).
To assess the drivers of variability in O2 fluxes (hourly and daily rates of GPP, R, and NCP), we first assessed the collinearity in the primary drivers of interest: seawater temperature and aboveground biomass for those studies reporting both variables. Because temperature and aboveground biomass are correlated (temperature × geography: P=0.011, Fig. S2 in the Supplement), we decided to focus first on the effect of season, using month as a predictor variable, which ostensibly encompasses some of the variability in both temperature and aboveground biomass (Figs. S3 and S4 in the Supplement). Furthermore, the use of month as a predictor variable allowed us to include the maximum number of studies in the analysis, since not all studies reported temperature and aboveground biomass. To standardize months to seasons across the hemispheres, we used the numerical notation for months in the Northern Hemisphere (i.e., January is 1, etc.). For the Southern Hemisphere, we subtracted 6 from the numerical notation and used the absolute value. In addition, we tested for differences between seagrass communities in temperate and tropical geographies based on the hypothesis that seagrass meadows in temperate geographies have greater seasonality in light, temperature, and aboveground biomass and, thus, should have a more pronounced seasonal fluctuation (Figs. S3 and S4).
We then tested for effects of temperature and aboveground biomass on the residual variation of the monthly models. Specifically, we first fit mixed-effects models of both hourly and daily rates of GPP, R, and NCP using maximum likelihood with geography as a categorical factor (tropical vs. temperate) and a linear and quadratic term for month as fixed effects, as well as the two-way interactions between geography and each term for month. We also included replicate nested in study as a random effect to account for non-independence arising from the inclusion of repeated measures from the same sites over time and measurements from multiple sites within a single study:
We then used backward model selection to determine the significance of fixed effects based on likelihood ratio tests. Final models were fit using restricted maximum likelihood to calculate model estimates. We then performed two separate analyses using (1) environmental temperature and (2) aboveground biomass to assess any remaining variability in the residuals from the seasonal models. First, using just the subset of studies that either reported temperature or biomass, we fit the final seasonal models again using restricted maximum likelihood to obtain the conditioned residuals. Then, using these residuals, we fit linear models with geography as a categorical factor, plus a linear term for either temperature or aboveground biomass as well as the interaction between geography and temperature/biomass as fixed effects. We used backward model selection, comparing nested models with a series of ANOVAs. Finally, we tested for net autotrophy (NCP>0) using a one-tailed t test. All analyses were performed in R (version 3.6.2) (R Core Team, 2019) with the following packages as needed: nlme (version 3.1.145) (Pinheiro et al., 2020), broom (version 0.7.3) (Robinson et al., 2020), and broom.mixed (version 0.2.6) (Bolker and Robinson, 2020).
3.1 Description of the database
Using our search criteria, we identified 56 published papers (spanning 1956 to 2020) that reported in situ rates of seagrass community metabolism (Table S1 in the Supplement). The complete set of studies spanned temperate and tropical ecosystems. The inclusion of 22 temperate studies and an additional 7 tropical studies significantly expanded the scope of inference beyond previous reviews (Duarte et al., 2010; Unsworth et al., 2012). Many studies occurred in the western Atlantic and Mediterranean (Fig. 1), and there were no studies from the North Pacific. Most studies measured seagrass metabolism during the spring and summer months, while fewer studies measured the metabolism in fall and winter conditions (Fig. 1). Environmental temperature was highest during late summer/early fall months and was higher overall in tropical biomes (Fig. S3). Aboveground biomass was highest during summer months and higher in the temperate geographies (Fig. S4).
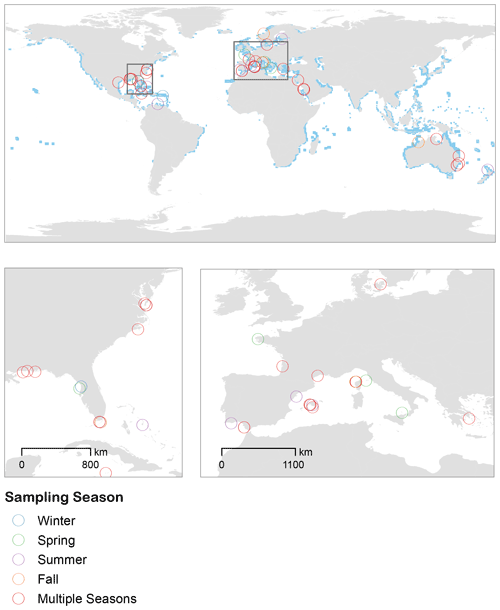
Figure 1Studies included in the analyses span temperate to tropical ecosystems, with many studies occurring in the western Atlantic and Mediterranean. Most studies measured seagrass metabolism during the spring and summer months, while fewer studies measured the metabolism in fall and winter conditions. Blue pixels represent the distribution of seagrasses from UNEP-WCMC and Short (2018).
3.2 Methodological analyses
Our results illustrate greater variability in the ranges of response observed using the mass balance method, which extend in magnitude beyond those observed using other methods for measuring both GPP and R (Fig. S1a–f). This greater variability does not appear to be driven by timing of the measurements as the mass balance method produced metabolic measurements of higher variability or magnitude across seasons (Fig. S1g–l). Measurements taken using incubations, eddy correlation methods, and water column measurements using in situ sensors and an acoustic Doppler velocimeter (or other instrument capable of measuring flow) are generally of similar magnitude and variation.
3.3 Spatial and temporal patterns in oxygen fluxes
3.3.1 Daytime oxygen fluxes
Measurements of hourly O2 fluxes (N=83 for NCP), typically obtained from shorter-duration deployments conducted during peak sunlight hours, reveal differences in seasonal patterns of GPP and respiration. Both GPP and R peak during summer months across both ecosystems (Fig. 2a and b). Despite higher biomass in temperate systems during summer months (Fig. S4), we do not detect a statistical difference in the seasonal patterns among GPP in temperate and tropical ecosystems (Table 1). This result is highly influenced by two studies in tropical geographies (Morgan and Kitting, 1984; Herbert and Fourqurean, 2008); when these studies are not included, summertime GPP is higher in temperate geographies than in tropical geographies (Fig. S5 in the Supplement). Similarly, R peaks in summer months in both temperate and tropical ecosystems, and we detect a sharper increase and a higher seasonal peak in R in temperate ecosystems (Fig. 2b; Table 1). The seasonal peaks in GPP and R effectively cancel each other out, resulting in no statistically detectable difference in hourly NCP rates across seasons (Fig. 2c). Although the net hourly O2 flux associated with NCP does not vary seasonally, the mean net hourly O2 flux from the seagrass to the seawater is positive (mean hourly ), indicating a net production of O2 during peak daylight hours regardless of geography (one-tailed t test: t82=8.50, P<0.001). Ninety-two percent of the 83 measurements were net autotrophic.
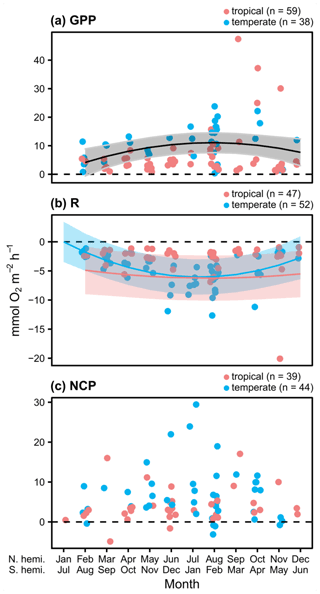
Figure 2Hourly rates for O2 fluxes () associated with seagrass communities as a function of time, with GPP measurements taken during peak sunlight hours (∼ 10:00 to 14:00 LT). Studies performed in temperate versus tropical ecosystems are illustrated by color (blue is temperate, red is tropical), and significance (P<0.05) is denoted by a fit line with a quadratic term and 95 % CI. The colors of the lines denote significant differences between tropical and temperate systems in GPP and R, while a black line denotes a significant relationship but no difference between temperate and tropical geographies.
3.3.2 Full-day oxygen fluxes
We found 164 measurements/deployments that reported full-day NCP using methods that span a wider range of photoperiods and environmental conditions, and thus provide insight into the potential for full-day net metabolic status and possible local OA mitigation potential. Based on the accompanying daily rates of GPP and R, there is evidence of a seasonal cycle in O2 fluxes to and from the water column associated with seagrass metabolism (Fig. 3a and b). The seasonal fluctuation differed statistically between temperate and tropical geographies, with a sharper slope in the seasonal fluctuation among the tropical studies (Fig. 2a). We did not detect a difference in R between geographies. In general, the seasonal fluctuation in GPP exceeds the seasonal fluctuation in respiration in both geographies, resulting in higher daily net O2 flux from the seawater to the seagrass associated with NCP in summer months (Fig. 3c). The seasonal fluctuation in NCP was greater among the tropical studies than the temperate studies (Table 2). The mean NCP for tropical geographies was 62.5 (±62.4 SD) , with 84 % of the 77 total reported measurements being autotrophic. The mean NCP for temperate geographies was 29.0 (±79.1) , with 68 % of the 187 total reported measurements being autotrophic. Overall, the seagrass meadows in both geographies were net autotrophic (one-tailed t test: tropical t76=8.78, P<0.001; temperate t186=5.02, P<0.001). Despite these overall trends, there are several individual studies that reported net heterotrophy and net O2 fluxes from the water column, even during summer months.
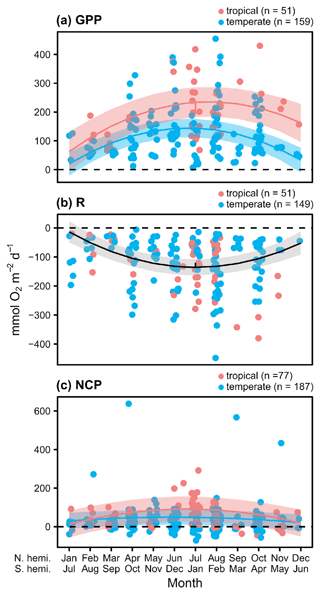
Figure 3Daily rates for O2 fluxes () associated with seagrass communities as a function of time. Studies performed in temperate versus tropical ecosystems are illustrated by color (blue is temperate, red is tropical), and significance (P<0.05) is denoted by a fit line with a quadratic term and 95 % CI. The colors of the lines denote significant differences between tropical and temperate systems.
3.4 Drivers of chemical variability
Within seasons, there is still marked variation in GPP and respiration (Figs. 2 and 3). Using the subset of studies that report environmental temperature (N=28), we found that temperature did not explain the residual variability in any metric besides hourly GPP (Fig. 4; Table 3), suggesting the seasonal models may generally account for hypothesized temperature effects. As noted, temperature explained some of the residual variability from the seasonal models of hourly GPP, with the effect differing among tropical and temperate geographies (Fig. S6 in the Supplement; hourly GPP geography × temperature: F42=8.67, P=0.005). Among studies reporting aboveground biomass (N=23), biomass explains some of the residual variability in daily NCP, although the effect depends on geography as well (Fig. 5; Table 3). Aboveground biomass also explains some of the residual variability in the seasonal models of hourly GPP, respiration, and NCP, and the effect of biomass on hourly GPP also depends on the geography (Fig. S7 in the Supplement; Table 3).
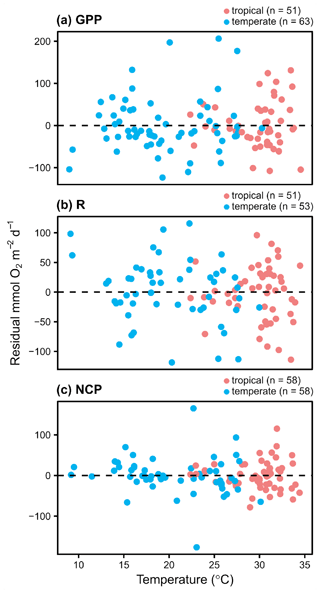
Figure 4Conditioned residuals of the daily rates of O2 fluxes () from a seasonal model as a function of temperature. Studies performed in temperate versus tropical ecosystems are illustrated by color (blue is temperate, red is tropical). None of the relationships are statistically significant.
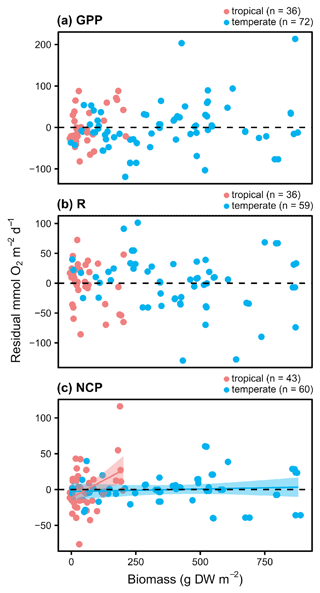
Figure 5Conditioned residuals of the daily rates for seagrass O2 fluxes () from a seasonal model as a function of aboveground biomass measured in the field during metabolism measurements. Studies performed in temperate versus tropical ecosystems are illustrated by color (blue is temperate, red is tropical). Significance is denoted by a fit line and 95 % CI.
4.1 Spatial and temporal variability in seagrass metabolism
Here, we report that the NCP of seagrass beds during daylight hours is positive and similar across seasons and geographies (Fig. 2c). This is ostensibly due to GPP generally exceeding R during daylight and the seasonal fluctuations in hourly rates of GPP and R balancing each other out. Our results suggest that metabolically driven alterations in seawater chemistry during daylight hours are similar across time and ecosystems but small in magnitude.
We also demonstrate that seagrass beds are generally net autotrophic over the length of the day (based on daily NCP), and the magnitude of this full-day NCP is more pronounced during summer months and in tropical geographies (Fig. 3c). However, underlying the summertime peak in full-day NCP is the potential for marked diurnal variability in O2. In particular, the demonstrated summertime peak in hourly respiration rates could drive more pronounced nighttime lows in O2 during the most pronounced windows for net autotrophy (i.e., summer months with the highest daily NCP). However, diurnal fluctuations in seawater chemistry associated with seagrass metabolism will also be influenced by hydrodynamics that are not captured in our synthesis (Koweek et al., 2018), and recent field studies of estuarine eelgrass by Ricart et al. (2021) demonstrated that sustained elevations in pH associated with seagrass meadows were not restricted to daylight hours.
Given the positive correlations between GPP and temperature, we assume that most of these measurements were taken when the environmental temperatures were not physiologically stressful to the seagrass community. Thus, the positive relationship between environmental temperature and GPP should not necessarily be viewed through the lens of temperature exposures associated with future warming driven by climate change. Continued warming associated with climate change could cause photosynthesis and respiration to decline at stressful temperatures. The probability that the relationship between temperature and metabolic responses will change with future warming is likely to differ geographically based on how close a community is to its thermal limit and the scope for acclimation or adaptation. This may partially explain the differences in the relationships between temperature and the residuals of the seasonal model between geographies, with increasing temperature negatively related to the residuals from tropical geographies and positively related to the residuals from temperate geographies. Many tropical seagrass species are growing close to their photosynthetic and physiological optima (Lee et al., 2007; Koch et al., 2012), and elevated temperatures in these geographies may be detrimental, causing metabolism (in this case hourly GPP) to be less than expected based on seasonal patterns. In contrast, the positive relationship between the residuals from the seasonal model and temperature in temperate geographies indicates that there may be a stimulatory effect before a thermal tolerance threshold is crossed and metabolism decreases.
The significant relationships between aboveground biomass and the residual variation in hourly GPP and NCP indicate that aboveground biomass also plays an important role in O2 fluxes beyond that which is already captured by any seasonal fluctuations in biomass. Although the relationships between aboveground biomass and the residual variation in hourly GPP are generally what would be expected (higher biomass means higher metabolic rates than expected based on the seasonal model), the negative relationship between aboveground biomass and the residual variation in hourly NCP is somewhat surprising. This relationship suggests that deployments/measurements in seagrass beds with higher aboveground biomass generally had lower hourly NCP than what is predicted by the seasonal model. This negative relationship may be explained by self-shading in dense meadows, or it could be due to other organisms that contribute to daytime respiration (e.g., heterotrophs) that are associated with the higher biomass meadows due to its structural complexity or other habitat features but are not accounted for in the aboveground biomass measurements. Dedicated experiments may be able to determine the mechanism for these findings; however, the positive relationships between aboveground biomass and the residual variation in daily NCP suggests that, overall, higher aboveground biomass generally increases production relative to respiration.
4.2 Implications for local OA mitigation and management
If O2 fluxes translate proportionally to community drawdown of CO2, our results can yield insights regarding local OA mitigation potential. Specifically, as with oxygen production, maximum pH elevations likely also occur during daylight hours and are similar across time and ecosystems but small in magnitude. OA mitigation potential may be similarly influenced by the factors identified by model outputs, including biomass, geography, and temperature. However, we recognize that additional sources of variability may alter the ratio of NCP based on carbon fixed and oxygen evolved, making conclusions regarding OA mitigation potential from oxygen metabolism difficult. Although the ratio between O2 produced and carbon fixed by an individual seagrass is generally assumed to be balanced (i.e., 1:1), the other processes that occur in a seagrass meadow, including respiration from organisms living within the seagrass and carbonate production and dissolution, also influence the dissolved inorganic carbon (DIC) concentration in the seawater. Current empirical measurements of in seagrass meadows range from 0.3 to 6.8 (Ziegler and Benner, 1998; Barrón et al., 2006), suggesting the effect of seagrass NCP on seawater pH could be substantially more or less pronounced than illustrated here. Because of this variability in the relationship between O2 and DIC, care must be taken when drawing OA mitigation conclusions from these data. A better understanding of the in particular meadows will better inform how the demonstrated seasonal variability and the potential diurnal variability in oxygen translates to carbonate chemistry variability and potential for OA mitigation. It will be additionally important to understand how this variability is integrated over time by organisms, especially under conditions projected for coastal habitats in the future. Finally, the utility of seagrass as a climate mitigation tool will depend on the goal of the management and, in most cases, will require more research. For example, if the goal of management is to prevent negative effects of ocean acidification on oyster growth, then studies that quantify the sensitivity of oyster growth to the variability in pH observed here are still required.
Few conservation or restoration efforts currently take into account the potential chemical ecosystem services of seagrasses and other submerged aquatic vegetation. Here, we demonstrate that daytime O2 fluxes associated with seagrass metabolism are likely to be similar across seasons and geography, while the full-day O2 fluxes peak during summer months in both tropical and temperate geographies. Integrating across seasons, seagrass meadows are net autotrophic. Integrating across seasons, seagrass meadows are net autotrophic, which could potentially translate to small changes in surrounding seawater chemistry. These seasonal patterns largely capture the present-day effects of variability in temperature and aboveground biomass on seagrass metabolism but likely do not adequately model the effects of future warming as it becomes physiologically stressful.
These results highlight challenges, as well as gaps in our understanding, that may be relevant to the use of seagrasses for sustained local OA mitigation and need to be addressed by the scientific community. In particular, we demonstrate that while peak daytime O2 fluxes are similar across seasons and geographies, nighttime respiration is highest during summer months. Thus, although seagrass beds are generally net autotrophic, nighttime respiration could reduce seawater O2 during periods of greatest autotrophy. This work also elucidated that certain geographies, such as the North Pacific, are currently underrepresented in our dataset. Thus, continued studies of seagrass metabolism and its effects on seawater carbonate chemistry are needed to expand our area of inference. In addition, studies are needed to constrain the relationship between dissolved oxygen fluxes and DIC, and this relationship may be important to elucidate at local scales to truly understand the potential for OA mitigation at a given location. Finally, more information is needed to understand how vulnerable organisms respond to the chemical variability highlighted in our study (Gimenez et al., 2018; Lowe et al., 2018) and, in particular, how this variability is integrated though time. Despite the considerations of geographic and temporal variability in O2 fluxes illustrated here, we recognize that seagrass conservation and restoration may be important strategies for climate adaptation for numerous other reasons, including carbon sequestration and habitat provisioning.
Code is available at https://doi.org/10.5281/zenodo.5834528 (Ward et al., 2022).
All data used in this analysis are publicly available via the published studies. The compiled database used herein can be viewed at https://doi.org/10.5281/zenodo.5834528 (Ward et al., 2022).
The supplement related to this article is available online at: https://doi.org/10.5194/bg-19-689-2022-supplement.
MW contributed to the investigation, writing, review and editing. KJK contributed to the conceptualization, funding acquisition, investigation, methodology, and the writing of the original draft. TLK contributed to the formal analysis, visualization, writing, review, and editing. HKH contributed to the investigation, visualization, writing, review, and editing; TMH contributed to the conceptualization, funding acquisition, writing, review, and editing. BMJ, SL, EBR, and GGW contributed to the conceptualization, writing, review, and editing. BG contributed to the conceptualization, funding acquisition, methodology, writing, review, and editing.
The contact author has declared that neither they nor their co-authors have any competing interests.
Publisher's note: Copernicus Publications remains neutral with regard to jurisdictional claims in published maps and institutional affiliations.
We would like to thank David A. Koweek, Kerry J. Nickols, and Yui Takeshita for their helpful comments and suggestions, which greatly improved the manuscript. This work was initiated by a working group of seagrass and biogeochemistry experts, convened at Bodega Marine Laboratory with support from California Sea Grant. The publication was prepared by Kristy J. Kroeker under NOAA grant no. NA14OAR4170075, California Sea Grant College Program project no. R/HCME-03, though NOAA'S National Sea Grant College Program, U.S. Department of Commerce. The statements, findings, conclusions and recommendations are those of the author(s) and do not necessarily reflect the views of California Sea Grant, NOAA or the U.S. Dept. of Commerce. In addition, Kristy J. Kroeker and Tye L. Kindinger received support from the David and Lucile Packard Foundation, and Kristy J. Kroeker received funding from the Alfred P. Sloan Foundation.
This research has been supported by NOAA (grant no. NA14OAR4170075) and California Sea Grant (grant no. R/HCME-03).
This paper was edited by Perran Cook and reviewed by two anonymous referees.
Barrón, C., Duarte, C. M., Frankignoulle, M., and Borges, A. V.: Organic carbon metabolism and carbonate dynamics in a Mediterranean seagrass (Posidonia oceanica) meadow, Estuar. Coast., 29, 417–426, 2006.
Bolker, B. and Robinson, D.: broom.mixed: Tidying Methods for Mixed Models. R package version 0.2.6, https://CRAN.R-project.org/package=broom.mixed (last access: 10 October 2021), 2020.
Clavier, J., Chauvaud, L., Amice, E., Lazure, P., van der Geest, M., Labrosse, P., Diagne, A., Carlier, A., and Chauvaud, S.: Benthic metabolism in shallow coastal ecosystems of the Banc d'Arguin, Mauritania, Mar. Ecol. Prog. Ser., 501, 11–23, 2014.
Cyronak, T., Andersson, A. J., D'Angelo, S., Bresnahan, P., Davidson, C., Griffin, A., Kindeberg, T., Pennise, J., Takeshita, Y., and White, M.: Short-term spatial and temporal carbonate chemistry variability in two contrasting seagrass meadows: implications for pH buffering capacities, Estuar. Coast., 41, 1282–1296, 2018.
Duarte, C. M. and Cebrián, J.: The fate of marine autotrophic production, Limnol. Oceanogr., 41, 1758–1766, 1996.
Duarte, C. M., Marbà, N., Gacia, E., Fourqurean, J. W., Beggins, J., Barrón, C., and Apostolaki, E. T.: Seagrass community metabolism: Assessing the carbon sink capacity of seagrass meadows, Global Biogeochem. Cy., 24, GB4032, 2010.
Gimenez, I., Waldbusser, G. G., and Hales, B.: Ocean acidification stress index for shellfish (OASIS): Linking Pacific oyster larval survival and exposure to variable carbonate chemistry regimes, Elementa Sci. Anth., 6, 15, 2018.
Hendriks, I. E., Olsen, Y. S., Ramajo, L., Basso, L., Steckbauer, A., Moore, T. S., Howard, J., and Duarte, C. M.: Photosynthetic activity buffers ocean acidification in seagrass meadows, Biogeosciences, 11, 333–346, https://doi.org/10.5194/bg-11-333-2014, 2014.
Herbert, D. A. and Fourqurean, J. W.: Ecosystem structure and function still altered two decades after short-term fertilization of a seagrass meadow, Ecosystems, 11, 688–700, 2008.
James, R. K., van Katwijk, M. M., van Tussenbroek, B. I., van der Heide, T., Dijkstra, H. A., van Westen, R. M., Pietrzak, J. D., Candy, A. S., Klees, R., Riva, R. E. M., Slobbe, C. D., Katsman, C. A., Herman, P. M. J., and Bouma, T. J.: Water motion and vegetation control the pH dynamics in seagrass-dominated bays, Limnol. Oceanogr., 65, 349–362, 2020.
Koch, M., Bowes, G., Ross, C., and Zhang, X.-H.: Marine macro-autotrophs and climate change, Global Change Biol., 19, 103–132, 2012.
Koweek, D. A., Zimmerman, R. C., Hewett, K. M., Gaylord, B., Giddings, S. N., Nickols, K. J., Ruesink, J. L., Stachowicz, J. J., Takeshita, Y., and Caldeira, K.: Expected limits on the ocean acidification buffering potential of a temperate seagrass meadow, Ecol. Appl., 28, 1694–1714, 2018.
Lee, K. S., Park, S. R., and Kim, Y. K.: Effects of irradiance, temperature, and nutrients on growth dynamics of seagrasses: a review, J. Exp. Mar. Biol. Ecol., 350, 144–175, 2007.
Lowe, A. T., Kobelt, J., Horwith, M., and Ruesink, J.: Ability of eelgrass to alter oyster growth and physiology is spatially limited and offset by increasing predation risk, Estuar. Coast., 42, 743–754, 2018.
Maher, D. and Eyre, B. D.: Benthic carbon metabolism in southeast Australian estuaries: habitat importance, driving forces, and application of artificial neural network models, Mar. Ecol. Prog. Ser., 439, 97–115, 2011.
Morgan, C. M. and Kitting, C.: Productivity and utilization of the seagrass Halodule wrightii and its attached epiphytes, Limnol. Oceanogr., 29, 1066–1076, 1984.
Murray, L. and Wetzel, R. L.: Oxygen production and consumption associated with the major autotrophic components in two temperate seagrass communities, Mar. Ecol. Prog. Ser., 38, 231–239, 1987.
Odum, H. T.: Primary production in flowing waters, Limnol. Oceanogr., 1, 102–117, 1956.
Odum H. T. and Hoskin, C. M.: Comparative studies on the metabolism of marine waters, Publ. Inst. Mar. Sci. Univ. Texas, 5, 16–46, 1958.
Pinheiro, J., Bates, D., DebRoy, S., Sarkar, D., and R Core Team: nlme: Linear and Nonlinear Mixed Effects Models. R package version 3.1-145, https://CRAN.R-project.org/package=nlme (last access: 10 October 2021), 2020.
R Core Team: R: A language and environment for statistical computing, R Foundation for Statistical Computing, Vienna, Austria, https://www.R-project.org/ (last access: 10 October 2021), 2019.
Ricart, A. M., Ward, M., Hill, T. M., Sanford, E., Kroeker, K. J., Takeshita, Y., Merolla, S., Shukla, P., Ninokawa, A., Elsmore, K., and Gaylord, B. P.: Coast-wide evidence of low pH amelioration by seagrass ecosystems, Global Change Biol., 27, 2580–2591, 2021.
Robinson, D., Hayes, A., and Couch, S.: broom: Convert Statistical Objects into Tidy Tibbles. R package version 0.7.3, https://CRAN.R-project.org/package=broom (last access: 10 October 2021), 2020.
UNEP-WCMC and Short, F. T.: Global distribution of seagrasses (version 6.0). Sixth update to the data layer used in Green and Short (2003), UN Environment World Conservation Monitoring Centre, Cambridge, UK, http://data.unep-wcmc.org/datasets/7 (last access: 1 November 2021), 2018.
Unsworth, R. K. F., Collier, C. J., Henderson, G. M., and McKenzie, L. J.: Tropical seagrass meadows modify seawater carbon chemistry: implications for coral reefs impacted by ocean acidification, Environ. Res. Lett., 7, 024026, 2012.
Van Dam, B. R., Lopes, C., Osburn, C. L., and Fourqurean, J. W.: Net heterotrophy and carbonate dissolution in two subtropical seagrass meadows, Biogeosciences, 16, 4411–4428, https://doi.org/10.5194/bg-16-4411-2019, 2019.
Ward, M., Kindinger, T., Hirsh, H., Hill, M., Jellison, B., Lummis, S., Rivest, E., Waldbusser, G., Gaylord, B., and Kroeker, K.: Reviews and syntheses: Spatial and temporal patterns in seagrass metabolic fluxes. Seagrass metabolic fluxes, Zenodo [data set and code], https://doi.org/10.5281/zenodo.5834528, 2022.
Ziegler, S. and Benner, R.: Ecosystem metabolism in a subtropical, seagrass-dominated meadow, Mar. Ecol. Prog. Ser., 173, 1–12, 1998.
- Article
(2368 KB) - Full-text XML