the Creative Commons Attribution 4.0 License.
the Creative Commons Attribution 4.0 License.
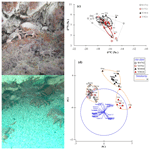
Differential feeding habits of the shallow-water hydrothermal vent crab Xenograpsus testudinatus correlate with their resident vent types at a scale of meters
Jing-Ying Wu
Siou-Yan Lin
Jung-Fu Huang
Chen-Tung Arthur Chen
Jia-Jang Hung
Shao-Hung Peng
The shallow-water hydrothermal vents (SVs) located off Kueishan (KS) Island, Taiwan, are one of the world's most intensively studied vent systems. It has long been known that white vents (WVs) and yellow vents (YVs) differ in the color and composition of the vent plumes. The endemic vent crabs (Xenograpsus testudinatus) are abundant in both vent types, and ovigerous females migrate to the vent periphery with a distance of 100–200 m to release their offspring. However, most research on the vent crabs was associated with WV or unspecified vent areas. To increase our knowledge of the crabs dwelling in other vent types, we compared the feeding habits of the vent crabs living in WV and YV with 2 sampling months. Specifically, we examined the benthic community of WV and YV, the isotopic niche width, and protein expression patterns of the crabs from the two vents at a distance of 100 m and sampled in July and August 2010. The coverage of sessile organisms and low-mobility fauna in WV was more abundant than in YV, based on the survey in August 2010. The δ13C and δ15N values of crabs differed spatially and temporally (multivariate analysis of variance test; p<0.05). The niche width of the vent crabs from YV-August (0.88 ‰2) narrowed substantially compared to the rest, i.e., YV-July (2.94 ‰2), WV-July (2.88 ‰2), and WV-August (3.62 ‰2; p<0.05), respectively. Based on the protein expression patterns, the vent crabs exhibited three groups, i.e., WV-July and YV-July, WV-August, and YV-August, respectively. Our results indicated that the dwelling crabs were associated with their living vent, and within-vent variability was more noticeable in YV compared to WV. We suggested that vent crabs inhabit their resident vent. Even at a scale of meters, trans-vent movement is probably rare as an adaptation to minimize predation risk.
- Article
(3006 KB) - Full-text XML
- BibTeX
- EndNote
Hydrothermal vents distribute in shallow water (SV <200 m) and the deep sea (DV >200 m), where they have unique physicochemical and geological characteristics and peculiar biological communities (Parson et al., 1995; Dando et al., 2000). The vents' plumes are rich in chemical compounds and gas, which are primarily reduced sulfur compounds, methane, and hydrogen gas. Based on hydrogen sulfide concentration, shallow-water hydrothermal vents (SVs) are divided into two groups, i.e. ≥ and <10 mmol mol−1 H2S (Tarasov, 2006). The low H2S concentration group was reported in SVs of Milos island (Greece; 4.9 mmol mol−1; Dando et al., 1995), Louise Harbor (Papua New Guinea; 8 mmol mol−1; Pichler et al., 1999a), Tutum Bay (Papua New Guinea; <0.3 mmol mol−1; Pichler et al., 1999b), Punta Mita (Mexico; <0.01 mmol mol−1; Prol-Ledesma et al., 2002), and Bahía Concepción (Mexico; undetected; Forrest et al., 2005). SVs off Kueishan (KS) Island in Taiwan belong to the group of high H2S concentrations (up to 172.4 mmol mol−1; Chen et al., 2016). These SVs are further distinguished according to the color of plumes, e.g., white vents (WVs) and yellow vents (YVs). The concentrations of H2S in WVs and YVs were 12.94±4.55 and 60.12±19.57 mmol mol−1, respectively (Chen et al., 2005b). WVs have a relatively low fluid temperature of 30–65 ∘C and a higher pH of 1.84–6.96; YVs have temperatures of 54–121 ∘C and a minimum pH of 1.52 (pH seawater scale; 25 ∘C; Table 1; Chen et al., 2005b; Lebrato et al., 2019; Mei et al., 2022). The fluid temperatures revealed diurnal and bimonthly cycles, which reach a maximum of 2 to 4 h after each high tide (Chen et al., 2005b).
Table 1Location and environmental measurements of the study sites. Mean ± SE (standard error)), n is the sample size, and the symbol “ means the same as above.
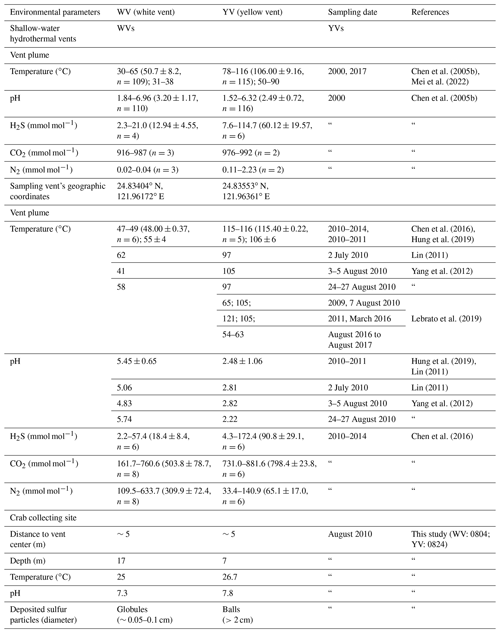
In WV, benthic organisms include bacteria, red algae, hexacoral (Tubastraea aurea), sea anemones (Anthopleura spp.), snails (Anachis misera and Nassarius sp.), chitons, serpulid polychaetes, and vent crabs (X. testudinatus; Jeng et al., 2004; Chang, 2006; Tang et al., 2013; Wang et al., 2014; Chen et al., 2015; Wang et al., 2015; Chan et al., 2016; Wu et al., 2021b). In YV, the only macrofauna is the endemic vent crabs. The species richness of macrofauna increases with horizontal distance from the mouth regions of WV and YV up to 2000 m away, indicating that the vent fluids may have a negative impact over several kilometers (Chan et al., 2016).
The vent crabs (X. testudinatus, Ng et al., 2000; family Xenograpsidae) are reddish- to greyish-brown in color, with quadrate carapace and sexual dimorphism (Fig. 1; Ng et al., 2000; Tseng et al., 2020). The wet weight and chela length of males (6.87±2.90 g and 1.37±0.40 cm; n=831) are significantly larger than females (4.17±1.25 g and 0.80±0.16 cm; n=274; Tseng et al., 2020). They inhabit the pits, fissures, and crevices of sulfur chimneys and forage in vent areas during slack water (Jeng et al., 2004; Chang et al., 2018; Allen et al., 2020). They were more abundant at 5 and 35 m from the vent mouth and less abundant at 20 m (Tseng et al., 2020). To avoid larval contact with toxic plumes, ovigerous females migrate to the vent periphery to release their offspring and return to the chimneys (Hung et al., 2019). The detection of high proteolytic enzyme activities in the midgut gland of the male crabs indicated that they are adaptive to irregular food availability (Hu et al., 2012). Based on the structure of the mouthparts and gastric mills, vent crabs are scavengers (Jeng et al., 2004). Through 16S ribosomal RNA gene amplicon pyrosequencing of crab's midgut, they found that its diet included eukaryotes (i.e., algae, fishes, bivalves, copepods, and anthozoans), prokaryotes (i.e., Rodobacteraceae, Oscillatoriphycidae, Mycoplasmataceae, and Helicobacteraceae) and symbiotic Epsilonproteobacteria and/or Gammaproteobacteria (Ho et al., 2015; Yang et al., 2016).
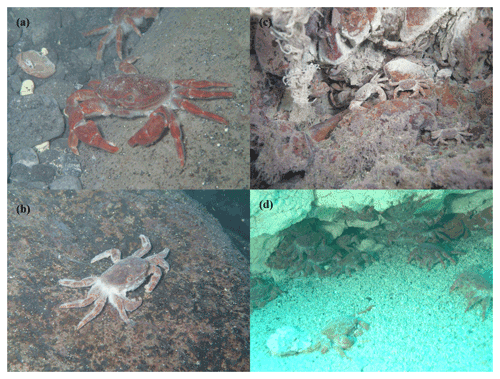
Figure 1Photos of the vent crabs (Xenograpsus testudinatus) inhabiting SVs. (a) Male crab. (b) Female crab. (c) Vent crabs in a white vent. (d) Vent crabs in a yellow vent.
Stable isotope analysis is commonly applied in the study of animal feeding ecology. Through the processes of assimilation, consumers increase with stable isotope values of 0.0 ‰–1.3 ‰ for δ13C and 1.4 ‰–5 ‰ for δ15N in each trophic transfer (DeNiro and Epstein, 1978, 1981; Post, 2002; McCutchan et al., 2003). With the measured isotopic data, the consumers' trophic position and niche width can be calculated (Layman et al., 2012). Trophic studies in SVs in KS Island revealed that dead zooplankton killed by sulfur plumes (as in plankton-derived production) is essential to scavengers and carnivores, based on the δ13C and δ15N data (Wang et al., 2014; Chang et al., 2018; Wu et al., 2021b). The importance of dead zooplankton to vent crabs decreases from the vent center to the periphery (Wang et al., 2022). Furthermore, vent crabs collected from YV had significantly lower δ13C and δ15N values than those in the WV (Wu et al., 2021b). However, it is unclear whether the heterogeneous isotopic results are associated with vent environments or vent types.
Under changing environments, proteome analysis is also a helpful approach to better understand the physiological states of organisms (López-Pedrouso et al., 2020). For example, the variation in the protein patterns of the dove snail (A. misera) was consistent with the diffusion of local vent fluids in KS Island (Chen et al., 2015). Proteomic studies exhibited differential expression signatures in the Chinese mitten crab (Eriocheir sinensis), when treated with different feeds (Wei et al., 2018), or hyper-osmotic stress (Yang et al., 2022) in the mud crab (Scylla olivacea), when exposed to heavy metals (Razali et al., 2019), and in the Mediterranean crab (Carcinus maenas) from different harbors (Jebali et al., 2014). Similarly, we can extend our knowledge of the within- and between-vent variations in the physiological states of crabs living in SVs by applying proteomic tools.
Although the vent crab (X. testudinatus) is one of the most intensively studied species in SV systems, most research was associated with WV or unspecified vent areas. Comparative studies on the feeding ecology of the vent crabs over time or for different vent types are rare. Therefore, we investigated the benthic community of a WV and a YV at a distance of 100 m and the feeding habits of the vent crabs from both sites by analyzing isotopic niche width and protein expression patterns collected in July and August 2010.
2.1 Sampling sites
KS Island is located northeast of Taiwan (Fig. 2). Eastward there is a cluster of over 30 vents within an area of 0.5 km2 at depths of 5 to 30 m (Chen et al., 2005a). The most common vent types are WV and YV. A summary of their environmental characteristics is displayed in Table 1.
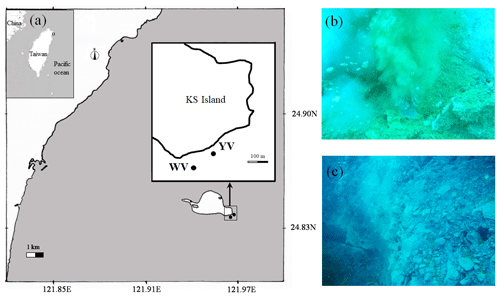
Figure 2Map of the Kueishan Island that shows the sampling sites. (a) The study sites. (b) Photograph of the yellow vent. (c) Photograph of the white vent. KS Island is Kueishan Island, WV is the white vent, and YV is the yellow vent.
In this study, a WV (24.83404∘ N, 121.96172∘ E) and a YV (24.83553∘ N, 121.96361∘ E) were sampled in July and August 2010, and the distance between the two vents was about 100 m. The vent plume temperature varied between 41–62 ∘C in the WV and 54–121 ∘C in the YV (Yang et al., 2012; Chen et al., 2016; Hung et al., 2019; Lebrato et al., 2019). The pH in the WV was 4.83–5.74, while the YV had a range from 2.22 to 2.82 (Lin, 2011; Yang et al., 2012; Hung et al., 2019). The H2S concentration of the plumes was 18.4±8.4 and 90.8±29.1 mmol mol−1, respectively (Chen et al., 2016). The gas compositions of the two vents were dominated by CO2 (503.8±78.7 and 798.4±23.8 mmol mol−1, respectively) and N2 (309.9±72.4 and 65.1±17.0 mmol mol−1, respectively; Chen et al., 2016). The recorded environmental parameters of our sampling locations on 4 and 24 August 2010 in the WV and YV included temperature, pH, and depth (i.e., 25.0 and 26.7 ∘C, 7.3 and 7.8, and 17 and 7 m, respectively; Table 1).
2.2 The survey of benthic communities in the WV and YV
The WV and YV benthic communities were investigated by SCUBA diving on 4 and 24 August 2010, respectively. Three quadrates (15×15 cm2) were set at distances of 3, 8, and 13 m from the vent mouth in the north, east, south, and west directions. A total of 12 quadrats in WV were surveyed. In contrast, it was 10 in YV because the distance to shore was only 7 m in the northerly direction. We photographed the quadrats, and the number of low-mobility fauna was counted (i.e., amphipod, columbellid snail, sea anemone, calyptraeid snail, chiton, vermetid snail, polychaete, sipunculid, and mytilid mussel). Vent crabs were not quantified due to the difficulty of counting fast-moving objects. The coverage of the attached organisms was scored by ImageJ (i.e., macroalgae, periphyton, and sponge). Low-mobility fauna or attached organisms were collected by hand with hammers or shovels for identification purposes. The collected samples were brought to the laboratory and preserved in 75 % ethanol. Identifications of small crustaceans, mollusks, annelids, and other invertebrates were based on the references of Carlgren (1949), Fauchald (1977), Miyake (1982), Cutler (1994), Okutani (2000), and Hooper et al. (2002).
2.3 Sampling of the vent crabs from the WV and YV
Vent crabs gathered 5 m away from the mouths of the WV and YV on the sampling dates of 2 July (both vents), 4 August (WV), and 24 August (YV) 2010, respectively. Each collected crab was covered with aluminum foil and kept in liquid nitrogen and then frozen at −80 ∘C for later use. Crab samples were examined for cleaning debris and epibionts; then their carapace width and wet weight were measured before dissection (Fan et al., 2016). The specimens used in the isotope niche width and proteomic studies differed with samples taken in July but were the same as those in August.
2.4 Determination of isotope niche width of the vent crabs from the WV and YV
About 0.3 g of leg muscle from one crab was taken, freeze-dried, and homogenized to powder. We weighed and encapsulated approximately 1 mg of the powder in a tin capsule for carbon and nitrogen isotope analyses. A PDZ Europa ANCA-GSL elemental analyzer interfaced with a PDZ Europa 20-20 isotope ratio mass spectrometer (Sercon Limited, Cheshire, UK) was used at the stable isotope laboratory at the University of California at Davis, CA, USA. Stable isotope abundances were performed in conventional delta (δ) notation and units in per thousand (‰) relative to the Pee Dee Belemnite for carbon and atmospheric N2 standards for nitrogen, respectively (Sharp, 2005). During analysis, several standard samples from at least two different laboratories were interspersed, e.g., nylon and USGS40 (L-glutamic acid) with certified δ13C of −27.8 ‰ and −28.9 ‰ and δ15N of −9.8 ‰ and −4.3 ‰, respectively. Analytical accuracy was obtained by comparing the measured values for the known values of the reference materials (e.g., acetanilide; i.e., 0.2 ‰ for δ13C and 0.3 ‰ for δ15N). Analytical precision for both δ13C and δ15N was <0.2 ‰, based on the standard deviation of the internal standards. Those data were reported in the studies by Wu et al. (2021a, b). Here, we used SPSS Statistics to analyze the published data by Pearson correlation tests between carapace width, wet weight, and the δ13C and δ15N values, and we used a two-way multivariate analysis of variance (MANOVA) to test the effects of the vent type and sampling month on the δ13C and δ15N values of crabs.
Measurements of isotopic niche width, proposed by Layman et al. (2007), were calculated for vent crabs (i.e., the corrected standard ellipse area or SEAc), which was a measure of the mean score of the isotopic niche occupied by all crab individuals in each group and their potential primary food sources in the δ13C and δ15N space (Jackson et al., 2011). This metric corrects the bias generated when sample sizes are small, represents the total amount of niche occupied in the isotopic space, and allows for robust statistical comparisons between datasets with different sample sizes (Jackson et al., 2011, 2012). Moreover, this metric also calculated the overlap area of the standard ellipses (and respective percentages) between sites and months, for which a diet similarity was observed. The metric was run with the SIBER v2.1.6 (Stable Isotope Bayesian Ellipses in R) package in R 4.2.2 software (R Development Core Team, 2013) and RStudio 2022.12.0-353.
2.5 Determination of protein expression patterns of the vent crabs from the WV and YV
The protein expression profiles of the vent crabs were determined by one-dimensional sodium dodecyl sulfate polyacrylamide gel electrophoresis (1D SDS-PAGE), following Chen et al. (2015) and Lin (2011). The sample sizes of the vent crabs from WV-July, YV-July, WV-August, and YV-August were 11, 6, 9, and 7, respectively. About 0.1 g leg muscle from one crab was taken and homogenized with 1 mL lysis buffer (7 M urea, 2 M thiourea, 4 % CHAPS detergent, and a protease inhibitor cocktail of two tablets per 100 mL) for the proteomic sample preparation. We centrifuged the homogenates at 12 000 g for 30 min at 4 ∘C and collected the supernatant. The protein concentration was determined by Bradford assay, with bovine serum albumin as the standard. We prepared the stacking and resolving gels with percentages of 5 % and 12 % (Hoefer SEM 260 system; Amersham Biosciences). In each sample lane, electrophoresis was run for 30 min at 120 V after loading 25 µg protein and then for 4 h at 180 V. The gels were stained by Coomassie Brilliant blue G-250 (Candiano et al., 2004). Stained gels were scanned and transformed into digitalized images using an Image Scanner (Amersham Biosciences). We utilized the Multi Gauge software V2.2 (FUJIFILM) for protein quantification. The protein bands were assigned band numbers, and their intensity levels were calculated as their relative area to the total protein area on the gel.
The Bray–Curtis similarity (BCS) measure is frequently used by ecologists to quantify the differences between samples based on abundance or count data. A cluster analysis of the BCS indices was employed to quantify the differences in the expressed protein bands of each vent crab from different vents and sampling months. We applied a square root transformation on the protein bands and then ran a cluster analysis of the BCS indices in the Primer 6.0 software (Clarke and Warwick, 2001). In addition, the contribution of each protein band was further determined by principal component analysis (PCA) in the Primer 6.0 software. The purpose of this analysis was to obtain the contribution of each protein band to the quantified differences among vent crabs by BCS (Paukert and Witting, 2002).
3.1 Composition of the benthic community in the WV and YV
The coverage of the attached organisms and the abundance of low-mobility benthos in the two vents differed. In the WV, the coverage of macroalgae, periphyton, and sponge was 39.2±6.6 %, 29.0±6.4 %, and 3.6±2.4 % (n=12), respectively. YV only had periphyton of 77.0±16.2 % (n=10; Fig. 3). Dominant low-mobility fauna in the WV were sea anemones (17.7±4.5 individuals per 100 cm2), calyptraeid snails (2.7±1.1 individuals per 100 cm2), and columbellid snails (1.0±0.3 individuals per 100 cm2), respectively. The remaining species were quite a few, including polychaete, chiton, sipunculid, and mytilid mussel, while, within the surveyed area of YV, no low-mobility fauna were observed. In addition, fast-moving vent crabs were always present at both sites.
3.2 Isotopic niche width of the vent crabs from the WV and YV
Table 2 and Fig. 4 show the size ranges of the vent crabs and their δ13C and δ15N values from the two vents in July and August 2010. There was no correlation between the carapace width or wet weight and δ13C or δ15N values (Pearson correlation; p>0.05). For WV crabs, the mean values were ‰ and ‰ for δ13C and 7.8±0.2 ‰ and 7.7±0.4 ‰ for δ15N, respectively. For YV crabs, the data were ‰ and ‰ for δ13C and 6.4±0.8 ‰ and 7.0±0.3 ‰ for δ15N, respectively. The analysis of the two-way MANOVA on δ13C and δ15N isotopes revealed significant effects of the vent type and sampling month (Pillai's trace; p<0.05), and there was no interaction between the two factors.
Table 2The isotopic data and statistical results of the vent crabs (Xenograpsus testudinatus) from the white and yellow vents in July and August 2010. (a) The δ13C and δ15N values of the vent crabs. (b) Results of the two-way multivariate analysis of variance (MANOVA; Pillai's trace). W is the white vent, Y is the yellow vent, bold text is p<0.05, the sampling dates are 2 July (0702), 4 August (0804), and 24 August (0824), n is the sample size, df is the degree of freedom, F is the F-statistic value, Num df is the numerator degree of freedom, and Sig. is the statistical significance.
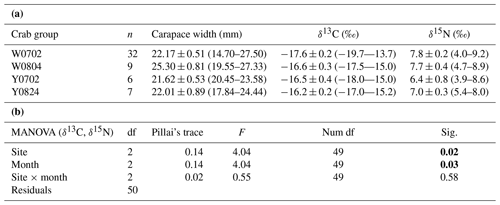
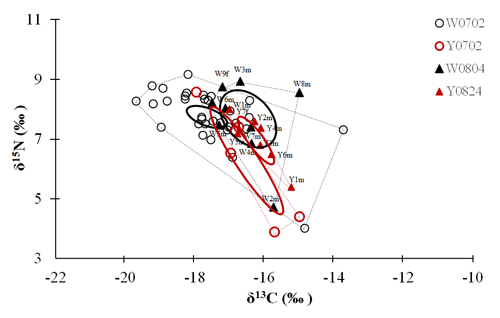
Figure 4Plot of the convex hull and standard ellipse areas based on the δ13C and δ15N values of the vent crabs (Xenograpsus testudinatus) from the white and yellow vents. Dotted lines show the convex hull areas, solid lines show the standard ellipse areas (SEAc), W is the white vent, Y is the yellow vent, the sampling dates are 2 July (0702), 4 August (0804), and 24 August (0824), m is for male, and f is for female. The crabs with labels are same individuals for the stable isotope and proteomic experiments.
The isotopic niche width of crabs from YV-August was significantly narrower than those of YV-July, WV-July, and WV-August, respectively. Their SEAc areas were 0.88 ‰2 vs. 2.94 ‰2, 2.88 ‰2, and 3.62 ‰2 (p<0.05), respectively (Table 3 and Fig. 4). The overlapping SEAc area between the two vents was 1.47 ‰2 in July, while it was 0.86 ‰2 in August (Table 3). In July, the overlap percentage was similar in both WV and YV (51.02 % vs. 50.03 %). In contrast, the overlap percentage in WV was low (23.68 %) compared to YV (97.87 %) in August. These results indicate that the temporal variations in the food sources in YV were more significant than in WV.
Table 3The ellipse analyses of the vent crabs (Xenograpsus testudinatus) from the white and yellow vents in July and August 2010. (a) Comparisons of the SEAc areas among crab groups, using Layman metrics, based on the posterior distribution (95 % credited intervals) of the modes (p<0.05; A > B). (b) The overlapping percentage of the ellipse area among groups. W is the white vent, Y is the yellow vent, SEAc is the standard ellipse area corrected, and the sampling dates are 2 July (0702), 4 August (0804), and 24 August (0824).
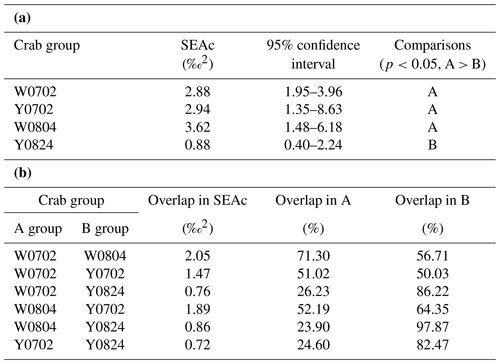
3.3 Protein expression patterns of the vent crabs from the WV and YV
A total of 27 protein bands were selected for BCS analysis (Fig. 5). Vent crabs were clustered into three groups, i.e., WV-July and YV-July, WV-August, and YV-August, respectively (Fig. 6). The first to the fifth principal components accounted for 42.9 %, 22.4 %, 9.9 %, 7.4 %, and 5.4 % of the total variance, respectively. The first principal component (PC1) mainly contributed to the separation, i.e., bands 5, 7, 23, 26, and 27. August samples with the lowest and highest PC1 values were crabs W8m and Y5m, which corresponded to their δ13C and δ15N values of −15.0 ‰ and 8.6 ‰ vs. −16.8 ‰ and 7.2 ‰, respectively (Fig. 3). Further identification of specific protein bands can characterize their structures and functions. In brief, as the isotopic results, the vent crabs exhibited temporal and spatial variations in protein expression patterns.
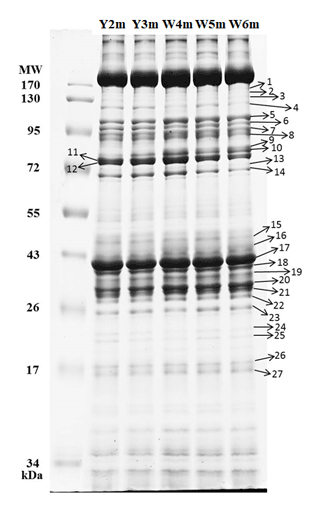
Figure 5Gel electropherogram with the molecular markers of the vent crabs (Xenograpsus testudinatus) from the white and yellow vents. Y2–3m show the male individuals from the yellow vent, W4–6m show the male individuals from the white vent, MW is the molecular weight, the unit is in kilodaltons (kDa), and the number is the protein band serial number.
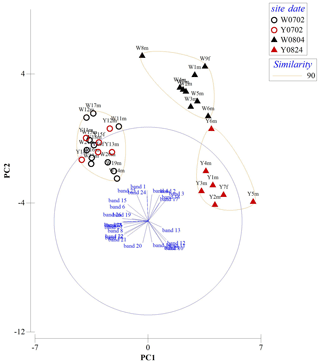
Figure 6Results from the combined principal component analysis (PCA) and cluster analysis of Bray–Curtis similarity (BCS) indices using standardized overall protein expressions. W is the white vent, Y is the yellow vent, m is for male, f is for female, the bands 1–27 show the variable of the protein bands, and the sampling dates are 2 July (0702), 4 August (0804), and 24 August (0824).
This study compared the feeding habits of the vent crabs (X. testudinatus) from a WV and a YV within 100 m. The benthic community of the two vents, stable isotope values, and protein expression patterns of the dwelling crabs were associated with their habitats and showed temporal variation. The δ13C and δ15N values significantly differed for the vent crabs between sites and sampling month. The niche width of the crabs from YV-August narrowed considerably than those of YV-July, WV-July, and WV-August, respectively. The protein profiles revealed three groups, i.e., WV-July and YV-July, WV-August, and YV-August, respectively. Based on the results, we suggested that the endemic vent crabs inhabit their vent types, and within-vent variability is more dramatic in YV compared to WV.
4.1 The benthic community in the WV and YV
In this study, within the area of 15 m from the center of WV, the coverage of the attached benthos was 72 %, including macroalgae, periphyton, and sponge. In YV, it only had periphyton for 77 %. Our previous study found three types of periphyton in YV, while WV had two sponge species, two brown algae, two red algae, five green algae, and one kind of periphyton (Wu et al., 2021b). Along a 50 m transect line near the vent mouths of WV and YV, Chan et al. (2016) and Wang et al. (2022) reported that encrusting red algae Hildenbrandia spp. and three red turf algae occupied 5 % of the substrate.
Low-mobility fauna were absent in the 15 m area from the center of YV. In contrast, there were nine species in WV, including sea anemones (17.7±4.5 individuals per 100 cm2), calyptraeid snails (2.7±1.1 individuals per 100 cm2), and columbellid snails (1.0±0.3 individuals per 100 cm2). Polychaete, chiton, sipunculid, and mytilid mussels were all with the density <1 individual per 100 cm2. By comparison, Wu et al. (2021b) did not record any low-mobility benthic fauna in YV in 2009 and 2010, while in WV there were 14 species, including shrimp (Alpheus lobidens), four snail species, chiton (Ischnochiton comptus), sea anemones (Anthopleura sp.), coral (T. aurea), and four polychaete species. In 2014, Chan et al. (2016) reported that, along a 50 m transect line from the center of YV, sea anemones (Anthopleura sp.) occupied 5±4 % of the substrate. The fauna in WV were more abundant, which included sea anemones (17.5±28.9 %), immobile snails (Bostrycapulus aculeatus and Dendropoma dragonella; 7 %–30 %), and other four snail species (1–3 individuals per 25 × 25 cm2). Overall, the investigated results across studies were consistent with higher species diversity and greater abundance of benthic communities in WV than in YV.
4.2 The isotopic niche width of the vent crabs from the WV and YV
Wu et al. (2021b) and Hung et al. (2019) reported that the δ13C and δ15N values of the vent crabs significantly differed between WV and YV. However, both studies combined specimens from 2 sampling months. Wu et al. (2021b) conducted their experiments in July and August 2010, with the values of ‰ (WV; n=44) and ‰ (YV; n=17) for δ13C and 7.8±0.14 ‰ (WV) vs. 6.7±0.3 ‰ (YV) for δ15N, respectively. Hung et al. (2019) gathered their samples in April and July 2010. They found that the male crabs from YV differed from all other groups, i.e., YV-female, WV-male, and WV-female, respectively (sample size and data not shown).
Within-vent variability in the δ13C and δ15N values of the vent crabs was also documented in several studies. Hung et al. (2019) collected their samples in April and July 2010, and the δ13C and δ15N values of both male and female crabs exhibited no difference between the center and edge of a WV (sample size and data not shown). In Wang et al. (2022), crabs from one site influenced by both WV and YV and three peripheral groups (150–300 m) presented a wide range of δ13C (−20.5 ‰ to −14.3 ‰) and δ15N (3.2 to 9.8 ‰) values sampled in June and July 2014. There was no significant difference in the isotopic data among the four groups (p>0.05); i.e., ‰ and 8.1±0.94 ‰ (n=6), ‰ and 7.5±1.01 ‰ (n=40), ‰ and 7.2±1.43 ‰ (n=156), and ‰ and 8.3±1.17 ‰ (n=10), respectively. The food of the vent crabs included dead zooplankton ( ‰ and 7.2±1.0 ‰; n=13), bacteria ( ‰ and 5.8±2.5 ‰; n=12), green algae and benthic deposited particulate organic matters ( ‰ and 5.2±1.6 ‰; n=84), and algae film (−10.2 ‰ and −0.5 ‰; n=1), respectively. The contribution of the above food items varied from the vent center to the periphery (i.e., 34 %, 13 %, 18 %, and 39 %, 14 %, 8 %, 23 %, and 14 %, 26 %, 58 %, 25 %, and 31 %, and 26 %, 21 %, 34 %, and 26 %, respectively). Dead zooplankton was more critical to crabs from the vent center than for those on the periphery. We also analyzed the isotopic data published by Chang et al. (2018) for comparison. They gathered vent crabs from a WV along the southwestern transect in August and September 2015. The δ13C and δ15N values were significantly different between the center and periphery (70–100 m; MANOVA; p=0.01), with ‰ and 5.33±4.06 ‰ (n=4), together with ‰ and 8.85±0.79 ‰ (n=10), respectively. The food of the vent crabs in this study included dead zooplankton ( ‰ and 6.1±1.0 ‰; n=20), vent particulate organic matter ( ‰ and ‰; n=2), and epibenthic crustaceans (including Amphidpoda, Mysida, and Euphausiacea; ‰ and 6.0±0.6 ‰; n=2), respectively. The contribution of the above food items differed between the center and periphery (i.e., 6 %–38 % vs. 16 %–42 %, 11 %–87 % vs. 6 %–31 %, and 7 %–53 % vs. 46 %–61 %, respectively). In this case, the importance of the dead zooplankton was similar in the two sites.
In this study, the δ13C and δ15N values of the vent crabs differed significantly between vent types and sampling months (MANOVA test; Table 2). Our results showed that the crabs' isotopic niche width (shown as the SEAc area) was considerably narrower for those in YV-August (0.88 ‰2) than for those in YV-July, WV-July, and WV-August (2.94 ‰2, 2.88 ‰2, and 3.62 ‰2; p<0.05), respectively (Table 3). In the southwestern Mediterranean, seasonal variations in the δ13C and δ15N values of the Sally Lightfoot crab Percnon gibbesi ranged from −18.33 ‰ to −13.08 ‰ and from 3.71 ‰ to 8.2 ‰ in 2016 (Bada et al., 2022). The isotopic niche width of P. gibbesi varied from 1.4 ‰2 in winter to 4.5 ‰2 in autumn, while the data were 1.5 ‰2 and 2 ‰2 in spring and summer, respectively. It showed that the diets of P. gibbesi in autumn had the widest niche (food variability) linked to the local variability in algal resources. In the Pechora Sea, the isotopic niche width in scavenger hermit crab Pagurus pubescens varied between sites of 4N and 9N with a distance of 13 km because of a significant difference in their macrobenthic abundance (Gebruk et al., 2021). The isotopic niche width for the hermit crab was 0.15 ‰2 at site 4N and 0.27 ‰2 at site 9N, with 0.05 ‰2 overlapped. Differences in diet sources were correlated with local macrobenthic clams, as shown at site 4N, characterized by low Astarte montagui (32 g m−2), which is in contrast to the high biomass of A. borealis and Macoma calcarea (500 g m−2) at site 9N. The niche width of this hermit crab had an even smaller overlapping SEAc area than our between-vent comparisons (i.e., 1.47 ‰2 in July and 0.86 ‰2 in August 2010). In brief, our study clearly shows that the isotopic signatures of the resident vent crabs reflected temporal and spatial heterogeneities. The different results among studies indicate explicit state sampling information, including size, date, and location, is essential.
4.3 Protein expression patterns of the vent crabs from the WV and YV
Our proteomic results indicated that vent crabs were distinguishable as groups of WV-July and YV-July, WV-August, and YV-August, respectively. In the case of dove snails, A. misera inhabiting in WVs of KS Island, their protein expression patterns were related to the diffusion of locally emitted vent fluids (Chen et al., 2015). The naturally acidified seawater in the southward sampling site had pH ranges from 7.78 to 7.82, while it was 7.31–7.83 in the eastern, southwestern, and northwestern locations. Based on the expressed protein profiles, the Anachis snails were classified into the southern location and another group. In a CO2 SV off Vulcano island in Sicily, sea anemones Anemonia viridis were collected at a distance of 350–800 m from a vent, where the pH values were 7.6, 7.9, and 8.2, respectively (Urbarova et al., 2019). Gene expression patterns of A. viridis revealed two clades, i.e., a low pH group (pH 7.6) vs. high pH ones (pH 7.9 and 8.2). Overall, mobile vent crabs, slow-moving dove snails, and sessile sea anemones all demonstrated adaptive abilities associated with their environments.
Organisms respond to environmental changes in a time-dependent manner. When the Chinese mitten crabs E. sinensis were transferred to high salinity (25 psu) for 6 d, then the protein profiles of the posterior gills were different from the control group (0 psu; Yang et al., 2022). The nutritional value of linoleic acid (18:2n-6; LA) and alpha-linolenic acid (18:3n-3; LNA) in the Chinese mitten crabs E. sinensis was evaluated in the laboratory for 107 d (Wei et al., 2018). A total of 186 proteins were expressed differentially in the hepatopancreas between the groups of LA and LNA. In the Teboulba fishing harbor in Tunisia, the sediments had high levels of aliphatic and aromatic hydrocarbon pollutants (Jebali et al., 2014). The Mediterranean crabs C. maenas showed differential protein expression patterns in hepatopancreas between the control (day 0) and exposed groups over 15, 30, and 60 d. These proteomic-based studies exhibited the earliest responses of the tested crabs to environmental changes detected at least on day 6. In this study, the protein expression patterns of the vent crabs changed in 1 month (Fig. 5), indicating that the vent environments probably fluctuated often.
4.4 Association of the crabs' feeding habits with vent types
It has long been known that WVs and YVs in KS Island differ in the color and composition of the vent plumes (Chen et al., 2005b; Lebrato et al., 2019; Mei et al., 2022). There is a relatively low fluid temperature and high pH in WVs compared to YVs (30–65 vs. 54–121 ∘C and 1.84–6.96 vs. 1.52–6.32; pH seawater scale at 25 ∘C; Table 1). The diffusion of the vent fluid relates to local circulation. Therefore, fluctuations in the fluid temperature and pH reveal diurnal and bimonthly cycles (Chen et al., 2005b). Recently, Lebrato et al. (2019) studied the temporal biogeochemical changes in this SV system during 2009–2018. Their principal findings are that the catastrophic earthquake and typhoon (Nepartak) in 2016 shaped the seabed morphology, seawater chemistry, vent fluid composition and flow rate, and benthic ecology, which then gradually recovered in 2018. In addition, the reduction in venting activity and fluid flow in YV was more severe than in WV. Moreover, the feeding habits of the vent crabs presented by isotopic and proteomic results did reflect the geochemical characteristics of the vent types.
Previous studies reported that the movement of the vent crabs reveals different spatial scales. The daily foraging takes place in the vent area (Jeng et al., 2004; Chang et al., 2018; Allen et al., 2020). During the reproductive season, ovigerous females move to the vent periphery, release their larvae, and then return to the chimneys (Hung et al., 2019). The migratory distance was about 100–200 m horizontally from the vent mouth. Besides, vent crabs were absent in the by-catch of nearby non-vent fisheries (Wang et al., 2013). But the holotype of this species was collected from a 15 m deep rocky reef in the Gengxin Fish Port, Yilan county, Taiwan (Ng et al., 2000). These investigations indicate that vent crabs can actively move and survive in vent and non-vent environments. However, how far and how often the crabs move around is unknown. Here, we demonstrated that the vent crabs exhibited temporal and spatial variations in isotopic niche width and protein expression patterns (Table 3 and Fig. 6). Even with a distance of 100 m, the endemic vent crabs are strongly associated with their vent types. In addition, within-vent variability in food sources is more dramatic in the YV compared to the WV.
The isotopic niche and proteomic studies linked the physiological states of the vent crabs to SV environments. Suggestions for further studies include more replicates of different vent types, collecting crab samples simultaneously, increasing sample size, and considering genders. More stable isotopes from other tissues will also help us to better understand the nutrition sources and tissue-specific isotopic incorporation rates of the vent crabs. For example, the study of snails (Alviniconcha sp. and Ifremeria nautilei) from deep-sea vents in Vienna Woods, Manus Basin, Papua New Guinea, with isotopes of δ13C, δ15N, and δ34S on the feet and chitin shells, allows us to determine the isotopic discrimination between inorganic water compounds used by organisms as nutritional sources and the symbiont or host itself (Bojar et al., 2023). In a laboratory study on a freshwater shrimp (Macrobrachium borellii), the time period for incorporating the isotopic signatures (δ13C and δ15N) of muscle and hepatopancreas is evaluated to understand how an animal uses resources over time (Viozzi et al., 2021). If this is also combined with proteomic analysis, then we can elucidate more thoroughly how the physiological states of the vent crabs cope with different vent types.
This study compared the benthic community, isotopic niche width, and protein expression patterns of the endemic vent crabs (Xenograpsus testudinatus) from different types of SVs at 100 m. The δ13C and δ15N values significantly differed in vent crabs between sites (WV and YV) and sampling months (July and August). The dwelling crabs were associated with their resident vent, and within-vent variability is larger in the YV compared to the WV. It is concluded that vent crabs are faithful to their inhabiting vent. Trans-vent movement, even at a scale of meters, is probably sporadic and as an adaptation for minimizing predation risk.
The R code used for the data processing is available at https://CRAN.R-project.org/package=SIBER (Jackson and Parnell, 2023).
The isotopic data presented in this article are available in Wu et al. (2021a).
SYL and SHP helped collect samples and conducted the study. JFH, CTAC, and JJH were involved in the study design. JYW and LLL designed the study, analyzed the data, and wrote the paper. All authors discussed the results and commented on the paper.
The contact author has declared that none of the authors has any competing interests.
Publisher’s note: Copernicus Publications remains neutral with regard to jurisdictional claims in published maps and institutional affiliations.
We thank the Stable Isotope Facility at the University of California (Davis) for analyzing our samples. Additionally, we thank Ya-Jou Chen for her assistance in conducting the experiment. We also thank Jhih-hui Hung, Jeng-Ren Lia, Chih-Hsien Chang, Yalan Chou, and the SeaWatch Company (Taiwan) for help with sample collection.
This research has been supported by the Asia-Pacific Ocean Research Center, National Sun Yat-sen University, funded by the Ministry of Education, Taiwan.
This paper was edited by Kenneth Rose and reviewed by Yiming Wang and two anonymous referees.
Allen, G. J. P., Kuan, P. L., Tseng, Y. C., Hwang, P. P., Quijada-Rodriguez, A. R., and Weihrauch, D.: Specialized adaptations allow vent-endemic crabs (Xenograpsus testudinatus) to thrive under extreme environmental hypercapnia, Sci. Rep.-UK, 10, 11720, https://doi.org/10.1038/s41598-020-68656-1, 2020.
Bada, N., Da Ros, Z., Rindi, F., Busi, S., Azzurro, E., Derbal, F., and Fanelli, E.: Seasonal trophic ecology of the invasive crab Percnon gibbesi (Brachyura, Plagusiidae) in the southwestern Mediterranean: Insights from stomach contents and stable isotope analyses, Mar. Environ. Res., 173, 105513, https://doi.org/10.1016/j.marenvres.2021.105513, 2022.
Bojar, A. V., Lecuyer, C., Maher, W., Bojar, H. P., Fourel, F., and Vasile, Ş.: Multi-element stable isotope geochemistry and arsenic speciation of hydrothermal vent fauna (Alviniconcha sp., Ifremeria nautilei and Eochionelasmus ohtai manusensis), Manus Basin, Papua New Guinea, Chemosphere, 324, 138258, https://doi.org/10.1016/j.chemosphere.2023.138258, 2023.
Candiano, G., Bruschi, M., Musante, L., Santucci, L., Ghiggeri, G. M., Carnemolla, B., Orecchia, P., Zardi, L., and Righetti, P. G.: A very sensitive colloidal Coomassie G-250 staining for proteome analysis, Electrophoresis, 25, 1327–1333, 2004.
Carlgren, O. H.: A Survey of the Ptychodactiaria, Corallimorpharia and Actiniaria, Kungliga, Svenska Vetenskaps, 4, 1–121, 1949.
Chan, B. K., Wang, T. W., Chen, P. C., Lin, C. W., Chan, T. Y., and Tsang, L. M.: Community structure of macrobiota and environmental parameters in shallow water hydrothermal vents off Kueishan Island, Taiwan, PLoS ONE, 11, e0148675, https://doi.org/10.1371/journal.pone.0148675, 2016.
Chang, C. M.: Sulfide Tolerance and Detoxification of the Vent Crab, Xenograpsus testudinatus, Master thesis, National Taiwan University, Taipei, Taiwan, 49 pp., 2006.
Chang, N. N., Lin, L. H., Tu, T. H., Jeng, M. S., Chikaraishi, Y., and Wang, P. L.: Trophic structure and energy flow in a shallow-water hydrothermal vent: Insights from a stable isotope approach, PLoS ONE, 13, e0204753, https://doi.org/10.1371/journal.pone.0204753, 2018.
Chen, C. T. A., Wang, B. J., Huang, J., Lou, J., Kuo, F. W., and Tu, Y. Y.: Investigation into extremely acidic hydrothermal fluids off Kueishan Tao, Taiwan, Acta Oceanol. Sin., 24, 125–133, 2005a.
Chen, C. T. A., Zeng, Z., Kuo, F. W., Yang, T. F., Wang, B. J., and Tu, Y. Y.: Tide-influenced acidic hydrothermal system offshore NE Taiwan, Chem. Geol., 224, 69–81, https://doi.org/10.1016/j.chemgeo.2005.07.022, 2005b.
Chen, X. G., Zhang, H. Y., Li, X. H., and Chen, C. T. A.: The chemical and isotopic compositions of gas discharge from shallow-water hydrothermal vents at Kueishantao, offshore northeast Taiwan, Geochem. J., 50, 341–355, https://doi.org/10.2343/geochemj.2.0425, 2016.
Chen, Y. J., Wu, J. Y., Chen, C. T. A., and Liu, L. L.: Effects of low-pH stress on shell traits of the dove snail, Anachis misera, inhabiting shallow-vent environments off Kueishan Islet, Taiwan, Biogeosciences, 12, 2631–2639, https://doi.org/10.5194/bg-12-2631-2015, 2015.
Clarke, K. R. and Warwick, R. M.: Change in Marine Communities: An Approach to Statistical Analysis and Interpretation, 2nc edn., Primer-E Ltd, Plymouth Marine Laboratory, United Kingdom, https://www.primer-e.com/ (last access: 2017), 2001.
Cutler, E. B.: The Sipuncula: Their Systematics, Biology and Evolution, Cornell University, Ithaca, New York, U.S., 453 pp., ISBN 0801428432, 1994.
Dando, P. R., Hughes, J. A., Leahy, Y., Niven, S. J., Taylor, L. J., and Smith, C.: Gas venting rates from submarine hydrothermal areas around the island of Milos, Hellenic Volcanic Arc, Cont. Shelf Res., 15, 913–929, https://doi.org/10.1016/0278-4343(95)80002-U, 1995.
Dando, P. R., Aliani, S., Arab, H., Bianchi, C. N., Brehmer, M., Cocito, S., Fowler, S. W., Gundersen, J., Hooper, L. E., Kölbl, R., Kuever, J., Linke, P., Makropoulos, K. C., Meloni, R., Miquel, J. C., Morri, C., Müller, S., Robinson, C., Schlesner, H., Sievert, S., Stöhr, R., Stüben, D., Thomm, M., Varnavas, S. P., and Ziebis, W.: Hydrothermal studies in the Aegean Sea, Phys. Chem. Earth Pt. B, 25, 1–8, https://doi.org/10.1016/S1464-1909(99)00112-4, 2000.
DeNiro, M. J. and Epstein, S.: Influence of diet on the distribution of carbon isotopes in animals, Geochim. Cosmochim. Ac., 42, 495–506, https://doi.org/10.1016/0016-7037(78)90199-0, 1978.
DeNiro, M. J. and Epstein, S.: Influence of diet on the distribution of nitrogen isotopes in animals, Geochim. Cosmochim. Ac., 45, 341–351, https://doi.org/10.1016/0016-7037(81)90244-1, 1981.
Fan, T. W., Lane, A. N., and Higashi, R. M.: Stable isotope resolved metabolomics studies in ex vivo tissue slices, Bio-protocol, 6, e1730, https://doi.org/10.21769/BioProtoc.1730, 2016.
Fauchald, K.: The polychaete worms. Definitions and keys to the orders, families and genera, Natural History Museum of Los Angeles County, Science Series, 28, 1–188, 1977.
Forrest, M. J., Ledesma-Vázquez, J., Ussler III, W., Kulongoski, J. T., Hilton, D. R., and Greene, H. G.: Gas geochemistry of a shallow submarine hydrothermal vent associated with the El Requesón fault zone, Bahía Concepción, Baja California Sur, México, Chem. Geol., 224, 82–95, https://doi.org/10.1016/j.chemgeo.2005.07.015, 2005.
Gebruk, A., Zalota, A. K., Dgebuadze, P., Ermilova, Y., Spiridonov, V. A., Shabalin, N., Henry, L. A., Henley, S. F., and Mokievsky, V. O.: Trophic niches of benthic crustaceans in the Pechora Sea suggest that the invasive snow crab Chionoecetes opilio could be an important competitor, Polar Biol., 44, 57–71, https://doi.org/10.1007/s00300-020-02775-3, 2021.
Ho, T. W., Hwang, J. S., Cheung, M. K., and Kwan, H. S.: Dietary analysis on the shallow-water hydrothermal vent crab Xenograpsus testudinatus using Illumina sequencing, Mar. Biol., 162, 1787–1798, https://doi.org/10.1007/s00227-015-2711-z, 2015.
Hooper, J. N. A., Van Soest, R. W. M., and Willenz, P. (Eds.): Systema Porifera: A Guide to the Classification of Sponges, Springer, Boston, Massachusetts, U.S., 1707 pp., ISBN 1461507475, https://doi.org/10.1007/978-1-4615-0747-5_1, 2002.
Hu, M. Y. A., Hagen, W., Jeng, M. S., and Saborowski, R.: Metabolic energy demand and food utilization of the hydrothermal vent crab Xenograpsus testudinatus (Crustacea: Brachyura), Aquat. Biol., 15, 11–25, 2012.
Hung, J. J., Peng, S. H., Chen, C. A., Wei, T. P., and Hwang, J. S.: Reproductive adaptations of the hydrothermal vent crab Xenograpus testudinatus: An isotopic approach, PLoS ONE, 14, e0211516, https://doi.org/10.1371/journal.pone.0211516, 2019.
Jackson, A. L. and Parnell, A. C.: SIBER: Stable Isotope Bayesian Ellipses in R, CRAN, the R Foundation [code], https://CRAN.R-project.org/package=SIBER (last access: 15 February 2023), 2023.
Jackson, A. L., Parnell, A. C., Inger, R., and Bearhop, S.: Comparing isotopic niche widths among and within communities: SIBER – Stable Isotope Bayesian Ellipses in R, J. Anim. Ecol., 80, 595–602, https://doi.org/10.1111/j.1365-2656.2011.01806.x, 2011.
Jackson, M. C., Donohue, I., Jackson, A. L., Britton, J., Harper, D. M., and Grey, J.: Population-level metrics of trophic structure based on stable isotopes and their application to invasion ecology, PLoS ONE, 7, e31757, https://doi.org/10.1371/journal.pone.0031757, 2012.
Jebali, J., Chicano-Galvez, E., Fernandez-Cisnal, R., Banni, M., Chouba, L., Boussetta, H., Lopez-Barea, J., and Alhama, J.: Proteomic analysis in caged Mediterranean crab (Carcinus maenas) and chemical contaminant exposure in Teboulba Harbour, Tunisia, Ecotox. Environ. Safe., 100, 15–26, https://doi.org/10.1016/j.ecoenv.2013.11.025, 2014.
Jeng, M. S., Ng, N. K., and Ng, P. K.: Feeding behaviour: hydrothermal vent crabs feast on sea 'snow', Nature, 432, 969, https://doi.org/10.1038/432969a, 2004.
Layman, C. A., Arrington, D. A., Montaña, C. G., and Post, D. M.: Can stable isotope ratios provide for community-wide measures of trophic structure?, Ecology, 88, 42–48, https://doi.org/10.1890/0012-9658(2007)88[42:csirpf]2.0.co;2, 2007.
Layman, C. A., Araujo, M. S., Boucek, R., Hammerschlag-Peyer, C. M., Harrison, E., Jud, Z. R., Matich, P., Rosenblatt, A. E., Vaudo, J. J., Yeager, L. A., Post, D. M., and Bearhop, S.: Applying stable isotopes to examine food-web structure: an overview of analytical tools, Biol. Rev., 87, 545–562, https://doi.org/10.1111/j.1469-185X.2011.00208.x, 2012.
Lebrato, M., Wang, Y. V., Tseng, L. C., Achterberg, E. P., Chen, X. G., Molinero, J. C., Bremer, K., Westernstroer, U., Soding, E., Dahms, H. U., Kuter, M., Heinath, V., Johnck, J., Konstantinou, K. I., Yang, Y. J., Hwang, J. S., and Garbe-Schonberg, D.: Earthquake and typhoon trigger unprecedented transient shifts in shallow hydrothermal vents biogeochemistry, Sci. Rep.-UK, 9, 16926, https://doi.org/10.1038/s41598-019-53314-y, 2019.
Lin, S. Y.: The Study of Feeding Habits and Protein Expression Pattern of Xenograpsus testudinatus in Shallow-Water Hydrothermal Vents of Kueishan Island, Master thesis, Institute of Marine Biology, National Sun Yat-sen University, Kaohsiung, Taiwan, 53 pp., 2011 (in Chinese).
López-Pedrouso, M., Varela, Z., Franco, D., Fernández, J. A., and Aboal, J. R.: Can proteomics contribute to biomonitoring of aquatic pollution? A critical review, Environ. Pollut., 267, 115473, https://doi.org/10.1016/j.envpol.2020.115473, 2020.
McCutchan, J. H., Lewis, W. M., Kendall, C., and McGrath, C. C.: Variation in trophic shift for stable isotope ratios of carbon, nitrogen, and sulphur, OIKOS, 102, 378–390, https://doi.org/10.1034/j.1600-0706.2003.12098.x, 2003.
Mei, K., Wang, D., Jiang, Y., Shi, M., Chen, C. T. A., Zhang, Y., and Tang, K.: Transformation, fluxes and impacts of dissolved metals from shallow water hydrothermal vents on nearby ecosystem offshore of Kueishantao (NE Taiwan), Sustainability, 14, 1754, https://doi.org/10.3390/su14031754, 2022.
Miyake, S.: Japanese Crustacean Decapods and Stomatopods in Color, Vol. 1: Macruca, Anomura and Stomatopoda, Hoikusha publishing, Osaka, Japan, 261 pp., ISBN 4586300624, 1982 (in Japanese).
Ng, N. K., Huang, J. F., and Ho, P. H.: Description of a new species of hydrothermal crab, Xenograpsus testudinatus (Crustacea: Decapoda: Brachyura: Grapsidae) from Taiwan, National Taiwan Museum Special Publication Series, 10, 191–199, 2000.
Okutani, T.: Marine Mollusks in Japan, Tokai University Press, Japan, 1224 pp., ISBN 4486014065, 2000 (in English and Japanese).
Parson, L. M., Walker, C. L., and Dixon, D. R.: Hydrothermal vents and processes, Geol. Soc. Spec. Publ., 87, 1–2, https://doi.org/10.1144/gsl.Sp.1995.087.01.01, 1995.
Paukert, C. P. and Witting, T. A.: Applications of multivariate statistical methods in fisheries, Fish. Res., 27, 16–22, 2002.
Pichler, T., Giggenbach, W. F., McInnes, B. I. A., Buhl, D., and Duck, B.: Fe sulfide formation due to seawater-gas-sediment interaction in a shallow-water hydrothermal system at Lihir Island, Papua New Guinea, Econ. Geol., 94, 281–288, https://doi.org/10.2113/gsecongeo.94.2.281, 1999a.
Pichler, T., Veizer, J., and Hall, G. E. M.: The chemical composition of shallow-water hydrothermal fluids in Tutum Bay, Ambitle Island, Papua New Guinea and their effect on ambient seawater, Mar. Chem., 64, 229–252, https://doi.org/10.1016/S0304-4203(98)00076-0, 1999b.
Post, D. M.; Using stable isotopes to estimate trophic position: models, methods, and assumptions, Ecology, 83, 703–718, https://doi.org/10.1890/0012-9658(2002)083[0703:USITET]2.0.CO;2, 2002.
Prol-Ledesma, R. M., Canet, C., Melgarejo, J. C., Tolson, G., Rubio-Ramos, M. A., Cruz-Ocampo, J. C., Ortega-Osorio, A., Torres-Vera, M. A., and Reyes, A.: Cinnabar deposition in submarine coastal hydrothermal vents, Pacific Margin of central Mexico, Econ. Geol., 97, 1331–1340, https://doi.org/10.2113/97.6.1331, 2002.
Razali, N. S. M., Amin, N. M., Omar, W. B. W., Ikhwanuddin, M., and Kadir, N. H. A.: Proteomic analysis and assessment of heavy metals in hepatopancreas of mud crabs from Setiu and Kuala Sepetang, Asian J. Agric. and Biol., Special Issue, 17–24, 2019.
R Development Core Team: R: A Language and Environment for Statistical Computing, R Foundation for Statistical Computing, Vienna, Austria, ISBN 3-900051-07-0, 2013.
Sharp, Z.: Principles of Stable Isotope Geochemistry, Pearson Prentice Hall, Hoboken, New Jersey, U.S., 344 pp., ISBN 0130091391, 2005.
Tang, K., Liu, K., Jiao, N., Zhang, Y., and Chen, C. T.: Functional metagenomic investigations of microbial communities in a shallow-sea hydrothermal system, PLoS ONE, 8, e72958, https://doi.org/10.1371/journal.pone.0072958, 2013.
Tarasov, V. G.: Effects of shallow-water hydrothermal venting on biological communities of coastal marine ecosystems of the western Pacific, Adv. Mar. Biol., 50, 267–421, https://doi.org/10.1016/S0065-2881(05)50004-X, 2006.
Tseng, L. C., Yu, P. Y., and Hwang, J. S.: Distribution and sexual dimorphism of the crab Xenograpsus testudinatus from the hydrothermal vent field of Kueishan Island, northeastern Taiwan, PLoS ONE, 15, e0230742, https://doi.org/10.1371/journal.pone.0230742, 2020.
Urbarova, I., Foret, S., Dahl, M., Emblem, A., Milazzo, M., Hall-Spencer, J. M., and Johansen, S. D.: Ocean acidification at a coastal CO2 vent induces expression of stress-related transcripts and transposable elements in the sea anemone Anemonia viridis, PLoS ONE, 14, e0210358, https://doi.org/10.1371/journal.pone.0210358, 2019.
Viozzi, M. F., Martínez del Rio, C., and Williner, V.: Tissue-specific isotopic incorporation turnover rates and trophic discrimination factors in the freshwater shrimp Macrobrachium borellii (Crustacea: Decapoda: Palaemonidae), Zool. Stud., 60, e32, https://doi.org/10.6620/ZS.2021.60-32, 2021.
Wang, L., Cheung, M. K., Kwan, H. S., Hwang, J. S., and Wong, C. K.: Microbial diversity in shallow-water hydrothermal sediments of Kueishan Island, Taiwan as revealed by pyrosequencing, J. Basic Microb., 55, 1308–1318, https://doi.org/10.1002/jobm.201400811, 2015.
Wang, T. W., Chan, T. Y., and Chan, B. K. K.: Diversity and community structure of decapod crustaceans at hydrothermal vents and nearby deep-water fishing grounds off Kueishan Island, Taiwan: a high biodiversity deep-sea area in the NW Pacific, B. Mar. Sci., 89, 505–528, https://doi.org/10.5343/bms.2012.1036, 2013.
Wang, T. W., Chan, T. Y., and Chan, B. K. K.: Trophic relationships of hydrothermal vent and non-vent communities in the upper sublittoral and upper bathyal zones off Kueishan Island, Taiwan: a combined morphological, gut content analysis and stable isotope approach, Mar. Biol., 161, 2447–2463, https://doi.org/10.1007/s00227-014-2479-6, 2014.
Wang, T. W., Lau, D. C. P., Chan, T. Y., and Chan, B. K. K.: Autochthony and isotopic niches of benthic fauna at shallow-water hydrothermal vents, Sci. Rep.-UK, 12, 6248, https://doi.org/10.1038/s41598-022-09839-w, 2022.
Wei, B., Yang, Z., Cheng, Y., Zhou, J., Yang, H., Zhang, L., and Yang, X.: Proteomic analysis of the hepatopancreas of Chinese mitten crabs (Eriocheir sinensis) fed with a linoleic acid or alpha-linolenic acid diet, Front. Physiol., 9, 1430, https://doi.org/10.3389/fphys.2018.01430, 2018.
Wu, J. Y., Lin, S. Y., Peng, S. H., Hung, J. J., Chen, C. T. A., and Liu, L. L.: Data on isotopic niche differentiation in benthic consumers from shallow-water hydrothermal vents and nearby non-vent rocky reefs in northeastern Taiwan, Data in Brief, 37, 107216, https://doi.org/10.1016/j.dib.2021.107216, 2021a.
Wu, J. Y., Lin, S. Y., Peng, S. H., Hung, J. J., Chen, C. T. A., and Liu, L. L.: Isotopic niche differentiation in benthic consumers from shallow-water hydrothermal vents and nearby non-vent rocky reefs in northeastern Taiwan, Prog. Oceanogr., 195, 102596, https://doi.org/10.1016/j.pocean.2021.102596, 2021b.
Yang, L., Hong, H., Guo, W., Chen, C. T. A., Pan, P. I., and Feng, C. C.: Absorption and fluorescence of dissolved organic matter in submarine hydrothermal vents off NE Taiwan, Mar. Chem., 128–129, 64–71, https://doi.org/10.1016/j.marchem.2011.10.003, 2012.
Yang, S. H., Chiang, P. W., Hsu, T. C., Kao, S. J., and Tang, S. L.: Bacterial community associated with organs of shallow hydrothermal vent crab Xenograpsus testudinatus near Kuishan Island, Taiwan, PLoS ONE, 11, e0150597, https://doi.org/10.1371/journal.pone.0150597, 2016.
Yang, Z., Zhou, J., Zhu, L., Chen, A., and Cheng, Y.: Label-free quantification proteomics analysis reveals acute hyper-osmotic responsive proteins in the gills of Chinese mitten crab (Eriocheir sinensis), Comp. Biochem. Phys. D, 43, 101009, https://doi.org/10.1016/j.cbd.2022.101009, 2022.