the Creative Commons Attribution 4.0 License.
the Creative Commons Attribution 4.0 License.
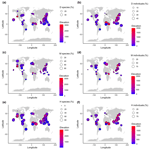
Regional effects and local climate jointly shape the global distribution of sexual systems in woody flowering plants
Minhua Zhang
Xiaoqing Hu
Fangliang He
Understanding the evolution and maintenance of plant sexual diversity needs to incorporate both regional processes and local climate factors across large geographic scales. Using data of woody flowering plants from a global set of large-scale forest plots and multinomial logistic regression, we quantified regional effects on the proportions of dioecious, monoecious, and hermaphroditic species and their abundance while incorporating evolutionary history and local climate factors. Our results showed that plants were more likely to be dioecious than hermaphroditic in Oceania and tropical Asia but were more likely to be monoecious than dioecious in Europe and North America compared with tropical Africa. We further found that plants were more likely to be monoecious than dioecious in island communities. Plants were more likely to be monoecious than dioecious in areas with high precipitation but were more likely to be dioecious than monoecious in areas with high precipitation of coldest quarter. Our results suggest that both regional processes and local climate factors play important roles in shaping the geographic distribution of plant sexual systems, providing a baseline for predicting future changes in forest communities in the context of global change.
- Article
(2679 KB) - Full-text XML
-
Supplement
(1064 KB) - BibTeX
- EndNote
Plants display a large variety of sexual systems that are known to play important roles in species evolution, colonization, and community assembly (Barrett, 2002; Vamosi, 2006; Pannell et al., 2015; Réjou-Méchain and Cheptou, 2015). Despite these advances in knowledge, the geographic patterns of plant sexual systems and their ecological drivers are not well understood (Vamosi and Queenborough, 2010; Wang et al., 2021). This lack of knowledge not only prevents us from understanding the selective pressures on plant sexual systems at the global scale but also hinders our ability to understand and predict their influence on biodiversity maintenance and community dynamics at the local scale (Charlesworth, 2006; Etterson and Mazer, 2016; Hultine et al., 2016; Tsuji and Fukami, 2020; Cronk, 2022).
Plant sexual systems can be mainly classified into three types (Barrett, 2002; Cardoso et al., 2018): dioecy (having male and female flowers in different individuals), monoecy (having male and female flowers in the same individuals), and hermaphroditism (having both male and female parts in the same flower). Although dioecy is generally rare, previous studies have shown a high incidence of dioecy in tropical forest and island communities, especially for woody species (Bawa and Opler, 1975; Baker and Cox, 1984; Renner and Ricklefs, 1995; Sakai and Weller, 1999; Chen and Li, 2008). In contrast, the incidence of monoecy is high in temperate forests because they are mostly wind pollinated (Regal, 1982; De Jong et al., 2008; Friedman and Barrett, 2009; Cronk, 2022). The proportion of hermaphroditism could be higher in tropical forests than in temperate forests as precipitation and the proportion of biotic pollination decrease with latitude. These former studies do not agree on the effects of elevations on the distribution of the three sexual systems. Dioecy and monoecy could both show high incidence in lowland forest communities, and thus hermaphroditism increases towards high elevation (Baker and Cox, 1984; Vamosi and Queenborough, 2010; but see Arroyo and Squeo, 1990). These geographic patterns are challenged by subsequent studies with more extended data and phylogenetic analyses (Vamosi et al., 2003; Wang et al., 2020, 2021). Thus, more inclusive studies are needed to explore the geographic patterns of plant sexual systems and to determine the ecological drivers underlying these patterns.
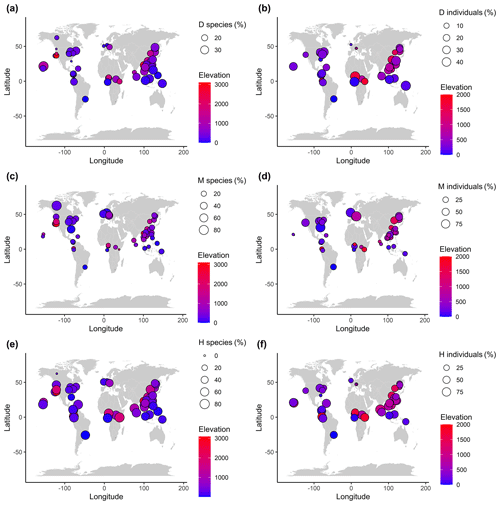
Figure 1Global distribution of the three sexual systems of woody flowering plants across the study plots. Panels (a), (c), and (e) are for the proportions of the sexual systems at the species level, and panels (b), (d), and (f) are for the proportion at the individual level. D, M, and H represent dioecy, monoecy, and hermaphroditism, respectively. Note data on the incidence of species sexual systems were available for all 55 plots (i.e., the species level), while only 45 of the plots had data on species abundance (i.e., the individual level).
Regional processes have been proposed to explain the distribution of plant sexual systems. As the Baker law suggests, colonization is less likely for plants that are unable to perform uniparental reproduction, especially for long-distance dispersal (Baker, 1955; Pannell et al., 2015); we would expect regions with different geographic and evolutionary histories to have different compositions of plant sexual systems (Ashton, 1967; Ricklefs and He, 2016; Zhang et al., 2016; Tsuji and Fukami, 2020). Previous studies have found a large clade of dioecious plants in tropical flora, which suggests dioecy might have originated in the tropical region (Bawa, 1980; Renner, 2014). Supposing abiotic and biotic conditions are equal, dioecious plant species would have a lower relative incidence in temperate regions, e.g., Europe and North America, than in tropical regions, e.g., tropical Asia and Oceania, compared with other sexual systems due to the low colonization rates of dioecious plants expected in temperate regions. In contrast, because dioecy could also be newly evolved from other sexual systems after colonization (Baker and Cox, 1984; Sakai et al., 1995), areas with younger species ages are expected to have a higher incidence of dioecy. However, some studies suggest a higher incidence of dioecy is associated with older flora (Renner and Ricklefs, 1995; Wang et al., 2020). There is a need to test the effects of region and evolutionary history on the incidences of plant sexual systems to resolve these contrasting results.
Local climate and habitat conditions have also been shown to affect the geographic patterns of plant sexual systems (Sakai and Weller, 1999; Vamosi and Queenborough, 2010; Wang et al., 2020, 2021). Studies have found dioecy to be more likely associated with dry or resource-poor habitats (Sakai and Weller, 1999; Ashman, 2006). As predicted by sex allocation theory, plants may not be able to maintain both sexes in a resource-poor environment (Ashman, 2006). This suggests the incidence of dioecy might be high while that of hermaphroditism and monoecy might be low in cold and dry areas. Recent studies find high temperatures are a disadvantage to dioecy and monoecy, while hermaphroditism favors warm and arid regions (Hultine et al., 2016; Wang et al., 2021). There is thus a lack of consensus on the climate factors that drive the patterns of different sexual systems. A possible source of the controversy could be that these studies do not simultaneously incorporate regional processes and local factors when studying biogeographic patterns of sexual systems (Sakai and Weller, 1999; Vamosi and Queenborough, 2010; Wang et al., 2021). In addition, most of these studies use occurrence data based on museum records that might have sampling bias. We intend to reveal regional effects on the incidence of plant sexual systems of woody flowering plants by incorporating both regional and local factors based on a global network of forest plots that accurately record not only the occurrence but also the abundance of each species.
In this study, we compiled a species list with information on sexual systems and abundance from 55 forest dynamics plots from the ForestGEO global plot network (http://www.forestgeo.si.edu/, last access: 16 March 2023) to investigate the ecological and evolutionary drivers of geographic patterns of sexual systems. We aimed to explore the geographic variation in plant sexual systems and test the effects of region, evolutionary history, and climate on shaping geographic patterns. We would expect that plants are more likely to be dioecious than hermaphroditic in tropical and adjacent regions, e.g., tropical Asia and Oceania, compared with other regions. We would also expect that plants are more likely to be monoecious than dioecious in temperate regions, e.g., Europe and North America, compared with other regions. We would also expect that plants in areas with younger species ages and lower precipitation are more likely to be dioecious than hermaphroditic and monoecious.
2.1 Data sources
Our study was based on 55 forest dynamic plots from the ForestGEO network (Fig. 1) with plot sizes varying from 4 to 60 ha. The 55 plots were established according to a standardized tree census protocol allowing comparison across sites, with each stem ≥ 1 cm diameter at breast height (DBH) being mapped and identified to species (Condit, 1998). We first checked the species name via the Taxonomic Name Resolution Service (TNRS) online tool to remove synonyms (https://tnrs.biendata.org/, last access: 2 August 2023).
Identification of species' sexual system was extracted from databases of eFloras (http://www.efloras.org/, last access: 28 July 2023), Plants of the World Online (http://powo.science.kew.org/, last access: 28 July 2023), World Flora Online (http://www.worldfloraonline.org/, last access: 23 March 2023), and the Global Plants database (https://plants.jstor.org/, last access: 20 March 2023). We also referred to literature that provided explicit information for the identification of species' or genus' sexual systems (e.g., Renner, 2014; Wang et al., 2021). In this study, we divided species into three categories based on their sexual systems – hermaphroditism, dioecy, and monoecy – according to Vamosi and Queenborough (2010), Cardoso et al. (2018), and Wang et al. (2021). We define hermaphroditism as a species having both male and female parts in the same flower, monoecy as a species that has separate male and female flowers on the same plant (thus including andromonoecy, gynomonoecy, and polygamous monoecy), and dioecy as a species with different sexual phenotypes (thus including androdioecy, gynodioecy, and polygamous dioecy). Species that were unidentified, species identified with a genus or family that has an uncertain sexual system, or species whose sexual system is unknown were excluded from our analysis. Those species account for a small proportion (< 1 %) of the species. In total, our data contain 8018 flowering plant species. We did not consider ferns, gymnosperms, and herbaceous plants because our interest was the sexual systems of woody flowering plants.
We collected plot information including latitude, longitude, region, isolation, area, the number of stems, elevation, mean annual temperature (MAT), and mean annual precipitation (MAP) of the 55 forest plots from recent studies (Ricklefs and He, 2016; Davies et al., 2021). We collected 17 other bioclimate factors (excluding BIO1 and BIO12) from WorldClim 2.0 (https://worldclim.org, last access: 11 July 2020). Annual potential evapotranspiration (PET) was collected from the Global Aridity and PET Database v2 (https://cgiarcsi.community/, last access: 15 September 2020).
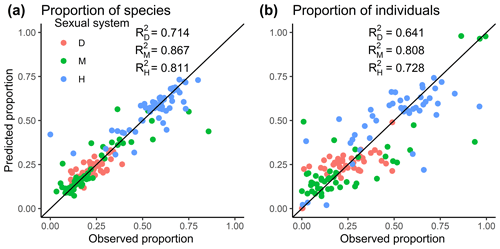
Figure 2The best selected multinomial logistic models showing the relationships between the observed proportion and the proportion predicted from the best multinomial logistic models for (a) species and (b) individuals. D, M, and H represent dioecy, monoecy, and hermaphroditism, respectively. R2 values for the models are given in each panel.
2.2 Data analysis
We modeled the proportions of the three sexual systems (π1, π2, π3) in relation to explanatory variables including 5 geographic factors (region, isolation, latitude, longitude, elevation), an evolutionary factor (mean species age), 3 plot characteristics (number of species, number of trees, area), and 22 climate factors (MAT, MAP, PET, and 19 bioclimate variables). We also consider the interactive term between mean species age and MAP in the models. In each plot, a species can only be either dioecious, monoecious, or hermaphroditic, leading to . This is a multinomial logistic regression model where the dependent variable is a nominal with three levels (dioecy, monoecy, and hermaphroditism). The multinomial logistic model has the following form:
where x is a set of explanatory variables.
Because the effect of region could be confounded with other variables, we developed a full model that included all geographic factors, plot characteristics, and climatic variables, together with the evolutionary factors. The term “region” was a character variable including seven regions (tropical Africa, Europe, the neotropics, North America, Oceania, temperate Asia, and tropical Asia). The term “island” was a binary variable with 12 plots being on island and 43 plots on mainland. Absolute latitude was used (four plots were from the Southern Hemisphere). To evaluate the effect of evolutionary history on the incidence of sexual systems across the study plots, we constructed a phylogenetic tree including all the species of each plot using R package V.PhyloMaker2 based on the updated big tree (Jin and Qian, 2019, 2022). We tested phylogenetic signals for plant sexual systems using lambda with the “fitDiscrete” function from R package geiger (Pagel, 1999; Pennell et al., 2014). We then compared it with a null model based on λ = 0 (for star tree) using the likelihood ratio test in R package picante (Kembel et al., 2010). We extracted species ages by taking the pendant branch length from the phylogeny and calculated the mean species age for each plot (Cadotte and Davies, 2016). The number of species, number of trees, and plot area were log-transformed, while other numeric variables were standardized to the 0–1 range by . The multinomial logistic model was estimated using the “multinom” function of R package nnet (Venables and Ripley, 2002).
Table 1The results of the multinomial logistic model for the proportion of dioecious, monoecious, and hermaphroditic species of woody flowering plants. Dioecy was set as the baseline system. M and H represent monoecy and hermaphroditism, respectively. The regional effects were coded in reference to tropical Africa. P values indicate the significance of the z test.
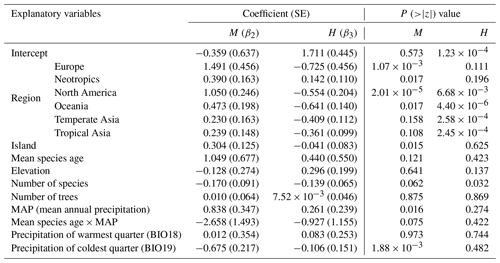
To reduce multicollinearity, we first excluded bioclimate variables with pairwise Pearson correlation coefficients > 0.95 and then excluded variables that had a variance inflation factor > 10 compared with other variables and kept variables with the clearest biological meaning (Tables S1, S2). Note the “multinom” function in R does not provide p values for the regression coefficients; p values were calculated using the Wald tests (here z tests). We performed the above analyses for the proportion of the three sexual systems at species and individual levels, respectively. The term “species level” means that the proportion of each sexual system (dioecy, monoecy, or hermaphroditism) was calculated from the incidence of species having a given sexual system over the total incidence of the three sexual systems, while “individual level” means the proportion was calculated from the number of trees of species (i.e., abundance) that have a given sexual system over the total number of trees in a plot. The latter requires species abundance data, and 45 of our 55 plots had such data.
All the analyses were conducted using R 4.2.2 (R Core Team, 2023) and related packages as mentioned above.
3.1 Geographic patterns of plant sexual systems
The spatial distribution of dioecy, monoecy, and hermaphroditism showed considerable variations on the global scale (Fig. 1). The proportions of dioecious species and individuals were negatively associated with latitude (Fig. S1a, b), while the incidence of monoecious species and individuals had a positive association with latitude (Fig. S1c, d). The negative association with latitude was also observed for the proportions of hermaphroditic species incidences and individuals (Fig. S1e, f). However, when the effects of region, plot characteristics, and climate factors were considered, the latitude variable was not retained in the final model, as it was closely correlated with the region and environmental factors (Tables 1, S2). The effects of elevation on the proportions of plant sexual systems were not significant at either the species level or the individual level when other variables were included (Tables 1, S2).
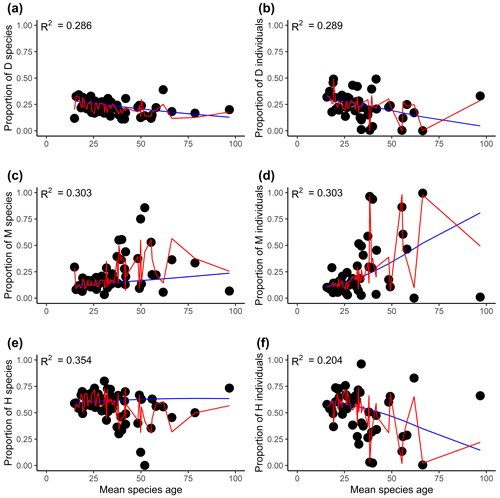
Figure 3The relationships between the proportions of flowering plants with different sexual systems and mean species ages (millions of years). Panels (a), (c), and (e) are for the proportion of species, and panels (b), (d), and (f) are for the proportion of the individual. D, M, and H represent dioecy, monoecy, and hermaphroditism, respectively. The blue line is the multinomial logistic model that includes only mean species age as the explanatory variable (no other variables are included). The red curves are the fitted proportions based on the best selected model, i.e., the model presented in Tables 1 and S3 for species and individuals, respectively. R2 values associated with models including only mean species age are given in each panel.
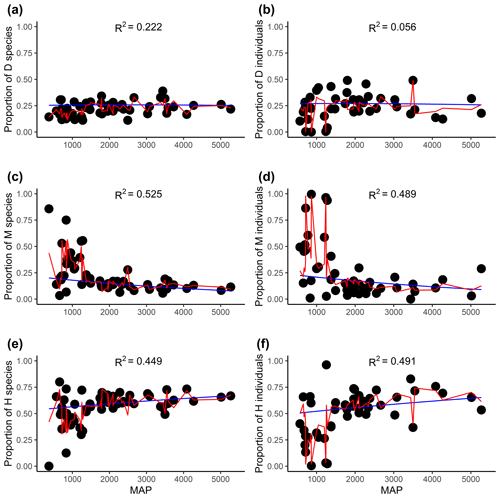
Figure 4The relationships between the proportion of flowering plants with different sexual systems and mean annual precipitation (MAP). Panels (a), (c), and (e) are for the proportion of species, and panels (b), (d), and (f) are for the proportion of the individual. D, M, and H represent dioecy, monoecy, and hermaphroditism, respectively. The blue line is the multinomial logistic model that includes only MAP as the explanatory variable (no other variables are included). The red curves are the fitted proportions based on the best selected model, i.e., the model presented in Tables 1 and S3 for species and individuals, respectively. R2 values associated with models including only MAP are given in each panel.
3.2 Regional effects on the incidence of plant sexual systems
The effects of region on the proportions of the three sexual systems were strong even when the effects of evolutionary history, plot characteristics, and local climate were considered (Tables 1, S3). Compared to tropical Africa, plants were more likely to be monoecious than dioecious, especially in Europe and North America, while in Oceania and tropical Asia, plants were less likely to be hermaphroditic than dioecious (Table 1). Plants were more likely to be monoecious than dioecious in island communities compared with mainland communities. We also found plants were more likely to be dioecious in areas of high species richness (Table 1). The model including regional and local factors explained 74.6 % of the variance in the incidence of dioecious species and 68.2 % of the variance in the incidence of dioecious individuals (Fig. 2; Tables 1, S3). For monoecy, the model explained 87.2 % of the variance in the incidence of species and 82.3 % of the variance in the incidence of individuals. The model explained 81.0 % of the variance in the incidence of hermaphroditic species and 69.6 % of the variance in the incidence of hermaphroditic individuals.
3.3 Effects of evolutionary history and climate on the incidence of plant sexual systems
Evolutionary history had significant effects on the proportions of the three sexual systems (Tables 1, S1). In most plots, plants showed significant phylogenetic signals (with λ significantly > 0) in sexual systems (https://github.com/Minhua322/plant-sex-system, last access: 31 July 2023; Fig. S2). Plants were more likely to be dioecious in areas with younger species ages, while they were more likely to be monoecious in areas with older species ages (Fig. 3). But the effect of mean species age was not significant when including other variables, and the interactive term between mean species age and mean annual precipitation showed negative effects on the proportion of monoecy (Table 1). Local climate also had significant effects on the proportions of the three sexual systems (Tables 1, S1). Plants were significantly more likely to be monoecious than dioecious in areas with high annual precipitation (Fig. 4). Plants were more likely to be dioecious than monoecious in areas with high precipitation of coldest quarter (Fig. S3).
Geographic patterns of sexual systems have attracted much interest from evolutionary ecologists because of their importance in driving patterns of biodiversity and phenotypic variations (Barrett, 2002; Tsuji and Fukami, 2020). Based on 55 forest dynamic plots, we documented the biogeographic patterns of sexual systems of woody flowering plants and tested region and climate effects on the proportions of three sexual systems on a global scale. We found a significant geographic pattern in the proportion of sexual systems with latitude and elevation and revealed the importance of region, evolutionary history, and climate effects in determining the global distribution of sexual systems in woody flowering plants (Bawa, 1980; Arroyo, 1990; Renner and Ricklefs, 1995; Sakai and Weller, 1999; Vamosi and Queenborough, 2010; Wang et al., 2021).
4.1 Regional effects on the proportions of plant sexual systems
As stated by Baker's law, dioecy could hinder long-distance colonization because of such plants' disadvantage in reproduction with limited mate partners, while monoecy and hermaphroditism might facilitate long-distance colonization by self-pollination (Baker, 1955; Pannell et al., 2015). As temperate plant communities have been assembled from tropical flora (Qian and Ricklefs, 2016), tropical and the nearby regions where dioecy originated are expected to have higher incidences of dioecy than temperate regions (Bawa, 1980; Renner and Ricklefs, 1995; Sakai and Weller, 1999; Renner, 2014). Our study found a high proportion of dioecy in Oceania and tropical Asia (Tables 1, S3), which supports the deduction of Baker's law to explain the dispersal limitation effect on dioecy. We also found Europe and North America contain a high proportion of monoecious species (Table 1). Monoecious plants are mostly wind pollinated; they also show an increased proportion with latitude (Regal, 1982; Friedman and Barrett, 2009; Rech et al., 2016). Furthermore, we found an island effect on the incidence of plant sexual systems (Table 1), suggesting the disadvantage of dioecy might prevent their colonization on islands.
As the incidence of plant sexual systems changes with regions, the population density of each sexual system can also change and thus affect the assembly of forest plant communities (Vamosi, 2006; Vamosi and Queenborough, 2010; Réjou-Méchain and Cheptou, 2015). Our results also found significant regional effects on the proportions of individuals with different sexual systems (Table S3). For example, we found the proportion of dioecious individuals was lower in Europe, while the proportion of monoecious individuals was lower in Oceania. These observations may explain one of the oldest biogeographic patterns: species composition and the dominance of certain plant families vary markedly across different biogeographic regions (van Humboldt, 1816, cited in Barry Cox, 2001). The regions with higher dioecy contain a high proportion of species from Phyllanthaceae, Euphorbiaceae, and Ebenaceae, which are mostly animal pollinated (Ashton, 1969; Bawa, 1980; Chen and Li, 2008; Renner, 2014; Zhang and He, 2017). The regions with a high incidence of monoecy in Europe, temperate Asia, and North America contain a high proportion of species from Fagaceae, Betulaceae, and Sapindaceae. Our results suggest that the effect of sexual systems on colonization possibly drives forest community assembly.
4.2 Effects of evolutionary history on the proportions of plant sexual systems
Although documenting and understanding the effects of evolutionary history on geographic patterns of species diversity (e.g., latitudinal gradient of species diversity) comprise a major undertaking in ecology (Gaston, 2000; Willig et al., 2003; Schluter and Pennell, 2017), little is known about their role in the geographic distribution of plant sexual systems (but see Wang et al., 2020, 2021). Our study showed plants in regions of younger species ages are more likely to be dioecious than monoecious (Fig. 3). These results suggest evolutionary processes might diminish the effect of Baker's law (Baker, 1955; Sakai et al., 1995; Pannell et al., 2015). However, the effects of age were not significant after including other regional and local factors. The effect of species age on plant sexual systems was different at regional and global scales (Wang et al., 2020), which could be explained by the interactive effects between species age and precipitation across different regions.
4.3 Climate effects on the proportions of plant sexual systems
The effects of local climate on sexual systems have been found in several studies (Sakai and Weller, 1999; Ashman, 2006; Wang et al., 2021). We found that plants were more likely to be monoecious than dioecious in areas with high annual precipitation, while plants were more likely to be dioecious than monoecious in areas with high precipitation of coldest quarter (Table 1; Figs. 4, S3). This result does support that dioecy survives better in a drier environment as sexual function separates (Sakai and Weller, 1999; Ashman, 2006; Wang et al., 2021). As dioecious plants are disadvantageous in colonization, we thus expect that they could shift in the flowering phenology rather than habitat from hermaphroditism and monoecy. Previous studies have found that dioecious plants are more likely to flower in the dry season with low temperature than hermaphroditic and monoecious ones; thus precipitation of coldest quarter could be a limiting factor for dioecy but not for monoecy and hermaphroditism (Kang and Bawa, 2003; Zhang and He, 2021). For monoecious plants, they may favor low precipitation when they are flowering in the dry season because most of them are wind pollinated (Regal, 1982; De Jong et al., 2008; Rech et al., 2016). Our results highlight the role of precipitation in determining the distribution of plant sexual systems, through its effects on pollination and physiological processes, thus affecting the assembly of forest community (Regal, 1982; Friedman and Barrett, 2009; Zhang and He, 2021).
In summary, our study showed significant effects of region, evolutionary history, and climate on the geographic patterns of sexual systems in woody flowering plants on a global scale. Regional processes such as long-distance dispersal; the evolution of sexual systems after colonization; and local climate, e.g., precipitation, together could shape the global distribution of plant sexual systems. We see the need to collect more extensive data on plant sexual systems that include information on pollination modes and flowering phenology to further disentangle the mechanisms determining the geographic patterns of sexual systems and their influence on the assembly and dynamics of forest plant communities.
Data on the species information and sexual systems, the plot information, and the climate variables for the 55 ForestGEO plots that support the findings of this study are available on Zenodo (https://doi.org/10.5281/zenodo.11035112, Zhang, 2024).
The supplement related to this article is available online at: https://doi.org/10.5194/bg-21-2133-2024-supplement.
FH designed the study. MZ and XH collected and analyzed data. MZ and FH wrote the paper.
The contact author has declared that none of the authors has any competing interests.
Publisher’s note: Copernicus Publications remains neutral with regard to jurisdictional claims made in the text, published maps, institutional affiliations, or any other geographical representation in this paper. While Copernicus Publications makes every effort to include appropriate place names, the final responsibility lies with the authors.
We thank colleagues of the ForestGEO network for kindly providing us with their plot data. We thank ForestGEO for organizing the 2018 Analytical Workshop in the Czech Republic, from which we received valuable feedback on the present study.
This study was funded by the National Natural Science Foundation of China (grant no. 31901105), the Fundamental Research Funds for the Central Universities, the ECNU-Albert Joint Lab for Biodiversity Study, and the NSERC (Canada).
This paper was edited by Anja Rammig and reviewed by two anonymous referees.
Ashton, P. S.: Speciation among tropical forest trees: some deductions in the light of recent evidence, Biol. J. Linn. Soc., 1, 155–196, https://doi.org/10.1111/j.1095-8312.1969.tb01818.x, 1969.
Arroyo, M. T. K. and Squeo, F. A.: Relationship between plant breeding systems and pollination, in: Biological approaches and evolutionary trends in plants, edited by: Kawano, S., Academic Press, London, 205–227, https://doi.org/10.1016/B978-0-12-402960-6.50004-4, 1990.
Ashman, T.: The evolution of separate sexes: a focus on the ecological context, in: Ecology and Evolution of Flowers, edited by: Harder, L. D. and Barrett, S. C. H., Oxford University Press, Oxford, 204–222, ISBN 97801985708519780198570868, 2006.
Baker, H. G.: Self-Compatibility and Establishment After “Long-Distance” Dispersal, Evolution, 9, 347–349, https://doi.org/10.2307/2405656, 1955.
Baker, H. G. and Cox, P. A.: Further Thoughts on Dioecism and Islands, Ann. Missouri Bot. Gard., 71, 244–253, https://doi.org/10.2307/2399068, 1984.
Barrett, S. C. H.: The evolution of plant sexual diversity, Nat. Rev. Genet., 3, 274–284, https://doi.org/10.1038/nrg776, 2002.
Barry Cox, C.: The biogeographic regions reconsidered, J. Biogeogr., 28, 511–523, https://doi.org/10.1046/j.1365-2699.2001.00566.x, 2001.
Bawa, K. S.: Evolution of Dioecy in Flowering Plants, Annu. Rev. Ecol. Syst., 11, 15–39, https://doi.org/10.1146/annurev.es.11.110180.000311, 1980.
Bawa, K. S. and Opler, P. A.: Dioecism in Tropical Forest Trees, Evolution, 29, 167–179, https://doi.org/10.2307/2407150, 1975.
Cadotte, M. W. and Davies, T. J. (Eds.): Phylogenies in ecology: a guide to concepts and methods, Princeton University Press, Princeton, NJ, ISBN 9780691157689, 2016.
Cardoso, J. C. F., Viana, M. L., Matias, R., Furtado, M. T., Caetano, A. P. de S., Consolaro, H., and de Brito, V. L. G.: Towards a unified terminology for angiosperm reproductive system, Acta Bot. Brasilica, 32, 329–348, https://doi.org/10.1590/0102-33062018abb0124, 2018.
Charlesworth, D.: Evolution of Plant Breeding Systems, Curr. Biol., 16, PR726–R735, https://doi.org/10.1016/j.cub.2006.07.068, 2006.
Chen, X.-S. and Li, Q.-J.: Patterns of plant sexual systems in subtropical evergreen broad-leaved forests in Ailao Mountains, SW China, J. Plant Ecol., 1, 179–185, https://doi.org/10.1093/jpe/rtn019, 2008.
Condit, R. S.: Tropical Forest Census Plots-Methods and Results from Barro Colorado Island, Panama and a Comparison with Other Plots, Springer Verlag and R G. Landes Company, Berlin and Georgetown, TX, ISBN 3540641440, 1998.
Cronk, Q.: The distribution of sexual function in the flowering plant: from monoecy to dioecy, Philos. T. R. Soc. B, 377, 20210486, https://doi.org/10.1098/rstb.2021.0486, 2022.
Davies, S. J., Abiem, I., Abu Salim, K., Aguilar, S., Allen, D., Alonso, A., Anderson-Teixeira, K., Andrade, A., Arellano, G., Ashton, P. S., Baker, P. J., Baker, M. E., Baltzer, J. L., Basset, Y., Bissiengou, P., Bohlman, S., Bourg, N. A., Brockelman, W. Y., Bunyavejchewin, S., Burslem, D. F. R. P., Cao, M., Cárdenas, D., Chang, L. W., Chang-Yang, C. H., Chao, K. J., Chao, W. C., Chapman, H., Chen, Y. Y., Chisholm, R. A., Chu, C., Chuyong, G., Clay, K., Comita, L. S., Condit, R., Cordell, S., Dattaraja, H. S., de Oliveira, A. A., den Ouden, J., Detto, M., Dick, C., Du, X., Duque, Á., Ediriweera, S., Ellis, E. C., Obiang, N. L. E., Esufali, S., Ewango, C. E. N., Fernando, E. S., Filip, J., Fischer, G. A., Foster, R., Giambelluca, T., Giardina, C., Gilbert, G. S., Gonzalez-Akre, E., Gunatilleke, I. A. U. N., Gunatilleke, C. V. S., Hao, Z., Hau, B. C. H., He, F., Ni, H., Howe, R. W., Hubbell, S. P., Huth, A., Inman-Narahari, F., Itoh, A., Janík, D., Jansen, P. A., Jiang, M., Johnson, D. J., Jones, F. A., Kanzaki, M., Kenfack, D., Kiratiprayoon, S., Král, K., Krizel, L., Lao, S., Larson, A. J., Li, Y., Li, X., Litton, C. M., Liu, Y., Liu, S., Lum, S. K. Y., Luskin, M. S., Lutz, J. A., Luu, H. T., Ma, K., Makana, J. R., Malhi, Y., Martin, A., McCarthy, C., McMahon, S. M., McShea, W. J., Memiaghe, H., Mi, X., Mitre, D., Mohamad, M., Monks, L., Muller-Landau, H. C., Musili, P. M., Myers, J. A., Nathalang, A., Ngo, K. M., Norden, N., Novotny, V., O’Brien, M. J., Orwig, D., Ostertag, R., Papathanassiou, K., Parker, G. G., Pérez, R., Perfecto, I., Phillips, R. P., Pongpattananurak, N., Pretzsch, H., Ren, H., Reynolds, G., Rodriguez, L. J., Russo, S. E., Sack, L., Sang, W., Shue, J., Singh, A., Song, G. Z. M., Sukumar, R., Sun, I. F., Suresh, H. S., Swenson, N.G., Tan, S., Thomas, S. C., Thomas, D., Thompson, J., Turner, B. L., Uowolo, A., Uriarte, M., Valencia, R., Vandermeer, J., Vicentini, A., Visser, M., Vrska, T., Wang, X., Wang, X., Weiblen, G. D., Whitfeld, T. J. S., Wolf, A., Wright, S. J., Xu, H., Yao, T. L., Yap, S. L., Ye, W., Yu, M., Zhang, M., Zhu, D., Zhu, L., Zimmerman, J. K., and Zuleta, D.: ForestGEO: Understanding forest diversity and dynamics through a global observatory network, Biol. Conserv., 253, 108907, https://doi.org/10.1016/j.biocon.2020.108907, 2021.
De Jong, T. J., Shmida, A., and Thuijsman, F.: Sex allocation in plants and the evolution of monoecy, Evol. Ecol. Res., 10, 1087–1109, 2008.
Etterson, J. R. and Mazer, S. J.: How climate change affects plants' sex lives, Science, 353, 32–33, https://doi.org/10.1126/science.aag1624, 2016.
Friedman, J. and Barrett, S. C. H.: Wind of change: New insights on the ecology and evolution of pollination and mating in wind-pollinated plants, Ann. Bot., 103, 1515–1527, https://doi.org/10.1093/aob/mcp035, 2009.
Gaston, K. J.: Global patterns in biodiversity, Nature, 405, 220–227, 2000.
Hultine, K. R., Grady, K. C., Wood, T. E., Shuster, S. M., Stella, J. C., and Whitham, T. G.: Climate change perils for dioecious plant species, Nat. Plants, 2, 16109, https://doi.org/10.1038/nplants.2016.109, 2016.
Jin, Y. and Qian, H.: V.PhyloMaker: an R package that can generate very large phylogenies for vascular plants, Ecography, 42, 1353–1359, https://doi.org/10.1111/ecog.04434, 2019.
Jin, Y. and Qian, H.: V.PhyloMaker2: An updated and enlarged R package that can generate very large phylogenies for vascular plants, Plant Divers., 44, 335–339, https://doi.org/10.1016/j.pld.2022.05.005, 2022.
Kang, H. and Bawa, K. S.: Effects of successional status, habit, sexual systems, and pollinators on flowering patterns in tropical rain forest trees, Am. J. Bot., 90, 865–876, https://doi.org/10.3732/ajb.90.6.865, 2003.
Kembel, S. W., Cowan, P. D., Helmus, M. R., Cornwell, W. K., Morlon, H., Ackerly, D. D., Blomberg, S. P., and Webb, C. O.: Picante: R tools for integrating phylogenies and ecology, Bioinformatics, 26, 1463–1464, https://doi.org/10.1093/bioinformatics/btq166, 2010.
Pagel, M.: Inferring the historical patterns of biological evolution, Nature, 401, 877–884, 1999.
Pannell, J. R., Auld, J. R., Brandvain, Y., Burd, M., Busch, J. W., Cheptou, P. O., Conner, J. K., Goldberg, E. E., Grant, A. G., Grossenbacher, D. L., Hovick, S. M., Igic, B., Kalisz, S., Petanidou, T., Randle, A. M., de Casas, R. R., Pauw, A., Vamosi, J. C., and Winn, A. A.: The scope of Baker's law, New Phytol., 208, 656–667, https://doi.org/10.1111/nph.13539, 2015.
Pennell, M. W., Eastman, J. M., Slater, G. J., Brown, J. W., Uyeda, J. C., Fitzjohn, R. G., Alfaro, M. E., and Harmon, L. J.: Geiger v2.0: An expanded suite of methods for fitting macroevolutionary models to phylogenetic trees, Bioinformatics, 30, 2216–2218, https://doi.org/10.1093/bioinformatics/btu181, 2014.
Qian, H. and Ricklefs, R. E.: Out of the tropical lowlands: latitude versus elevation, Trends Ecol. Evol., 31, 738–741, https://doi.org/10.1016/j.tree.2016.07.012, 2016.
R Core Team: R: A language and environment for statistical computing. R Foundation for Statistical Computing, Vienna, Austria, https://www.R-project.org/ (last access: 17 August 2023), 2023.
Rech, A. R., Dalsgaard, B., Sandel, B., Sonne, J., Svenning, J. C., Holmes, N., and Ollerton, J.: The macroecology of animal versus wind pollination: ecological factors are more important than historical climate stability, Plant Ecol. Divers., 9, 253–262, https://doi.org/10.1080/17550874.2016.1207722, 2016.
Regal, P. J.: Pollination by wind and animals: ecology of geographic patterns, Annu. Rev. Ecol. Syst., 13, 497–524, https://doi.org/10.1146/annurev.es.13.110182.002433, 1982.
Réjou-Méchain, M. and Cheptou, P. O.: High incidence of dioecy in young successional tropical forests, J. Ecol., 103, 725–732, https://doi.org/10.1111/1365-2745.12393, 2015.
Renner, S. and Ricklefs, R.: Dioecy and its correlates in the flowering plants, Am. J. Bot., 82, 596–606, 1995.
Renner, S. S.: The relative and absolute frequencies of angiosperm sexual systems: Dioecy, monoecy, gynodioecy, and an updated online database, Am. J. Bot., 101, 1588–1596, https://doi.org/10.3732/ajb.1400196, 2014.
Ricklefs, R. E. and He, F.: Region effects influence local tree species diversity, P. Natl. Acad. Sci. USA, 113, 674–679, https://doi.org/10.1073/pnas.1523683113, 2016.
Sakai, A. K. and Weller, S. G.: Gender and Sexual Dimorphism in Flowering Plants: A review of Terminology, Biogeographic Patterns, Ecological Correlates, and Phylogenetic Approaches, in: Gender and Sexual Dimorphism in Flowering Plants, edited by: Geber, M. A., Dawson, T. E., and Delph, L. F., Springer-Verlag, Berlin Heidelberg, 1–31, https://link.springer.com/chapter/10.1007/978-3-662-03908-3_1 (last access: 26 July 2023), 1999.
Sakai, A. K., Wagner, W. L., Ferguson, D. M., and Herbst, D. R.: Biogeographical and ecological correlates of dioecy in the Hawaiian flora, Ecology, 76, 2530–2543, https://doi.org/10.2307/2265826, 1995.
Schluter, D. and Pennell, M. W.: Speciation gradients and the distribution of biodiversity, Nature, 546, 48–55, https://doi.org/10.1038/nature22897, 2017.
Tsuji, K. and Fukami, T.: Sexual Dimorphism and Species Diversity: from Clades to Sites, Trends Ecol. Evol., 35, 105–114, https://doi.org/10.1016/j.tree.2019.09.001, 2020.
Vamosi, J. C., Otto, S. P., and Barrett, S. C. H.: Phylogenetic analysis of the ecological correlates of dioecy in angiosperms, J. Evol. Biol., 16, 1006–18, 2003.
Vamosi, S. M.: A Reconsideration of the Reproductive Biology of the Atlantic Forest in the Volta Velha Reserve, Biodivers. Conserv., 15, 1417–1424, https://doi.org/10.1007/s10531-005-0308-4, 2006.
Vamosi, S. M. and Queenborough, S. A.: Breeding systems and phylogenetic diversity of seed plants along a large-scale elevational gradient, J. Biogeogr., 37, 465–476, https://doi.org/10.1111/j.1365-2699.2009.02214.x, 2010.
Venables, W. N. and Ripley, B. D.: Modern Applied Statistics with S, 4th Edn., Springer, New York, NY, ISBN 978-1-4899-2821-4, 2002.
Wang, Y., Luo, A., Lyu, T., Dimitrov, D., Xu, X., Freckleton, R. P., Li, Y., Su, X., Li, Y., Liu, Y., Sandanov, D., Li, Q., Hao, Z., Liu, S., and Wang, Z.: Global distribution and evolutionary transitions of angiosperm sexual systems, Ecol. Lett., 24, 1835–1847, https://doi.org/10.1111/ele.13815, 2021.
Wang, Y., Lyu, T., Shrestha, N., Lyu, L., Li, Y., Schmid, B., Freckleton, R. P., Dimitrov, D., Liu, S., Hao, Z., and Wang, Z.: Drivers of large-scale geographical variation in sexual systems of woody plants, Glob. Ecol. Biogeogr., 29, 546–557, https://doi.org/10.1111/geb.13052, 2020.
Willig, M. R., Kaufman, D. M., and Stevens, R. D.: Latitudinal Gradients of Biodiversity: Pattern, Process, Scale, and Synthesis, Annu. Rev. Ecol. Evol. Syst., 34, 273–309, https://doi.org/10.1146/annurev.ecolsys.34.012103.144032, 2003.
Zhang, J., Nielsen, S. E., Mao, L., Chen, S., and Svenning, J. C.: Regional and historical factors supplement current climate in shaping global forest canopy height, J. Ecol., 104, 469–478, https://doi.org/10.1111/1365-2745.12510, 2016.
Zhang, M.: Minhua322/plant-sex-system: v1.0.0 (v1.0.0), Zenodo [data set], https://doi.org/10.5281/zenodo.11035112, 2024.
Zhang, M. and He, F.: Plant sex affects the structure of plant–pollinator networks in a subtropical forest, Oecologia, 185, 269–279, https://doi.org/10.1007/s00442-017-3942-0, 2017.
Zhang, M. and He, F.: Plant breeding systems influence the seasonal dynamics of plant-pollinator networks in a subtropical forest, Oecologia, 195, 751–758, https://doi.org/10.1007/s00442-021-04863-5, 2021.