the Creative Commons Attribution 4.0 License.
the Creative Commons Attribution 4.0 License.
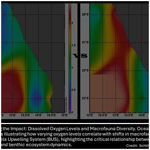
Influence of oxygen minimum zone on macrobenthic community structure in the northern Benguela Upwelling System: a macro-nematode perspective
Said Mohamed Hashim
Beth Wangui Waweru
Agnes Muthumbi
Understanding the dynamics of marine ecosystems, particularly the benthic communities, is crucial for predicting the impacts of climate change and human activities on oceanic biodiversity. In this context, we conducted a study on the macrobenthic communities in the Benguela Upwelling System (BUS). Macrobenthic samples were collected offshore of Namibia on board R/V Mirabilis during the 3rd Regional Research Graduate Network in Oceanography (RGNO) training and the National Marine Information and Research Centre's (NatMIRC's) plankton survey from 13 to 17 May 2016. Two transects, Terrace Bay (20° S) and Walvis Bay (23° S), hosted three stations each, while the third transect, Lüderitz (26° S), hosted only one station. From the results, three oxygen zones were identified, namely microxic (< 0.1 mL L−1), dysoxic (0.1–1.0 mL L−1), and oxic (> 1.0 mL L−1). A total of 20 macrobenthic taxa were identified; Nematoda, Polychaeta, Cumacea, and Oligochaeta were the most dominant taxa and were present in all oxygen zones. A total of 18 genera of macro-nematoda were identified; Desmolaimus and Paracomesoma dominated in all oxygen zones, Metoncholaimus recorded higher abundance in the dysoxic stations and were absent in the oxic stations, and the opposite was observed for Halanonchus and Dorylaimopsis. H′ diversity values for both the general macrofauna and macro-nematoda were higher in the oxic stations and lower in both the dysoxic and microxic stations, while an opposite trend was observed for dominance values. Density values were lower in microxic stations and higher in dysoxic stations, while at the oxic stations, the density values fell in between the two hypoxic zones. In conclusion, this study provides an overview of the distribution, diversity, and response to varying oxygen conditions of macrobenthic communities and their importance in marine ecosystems.
- Article
(1544 KB) - Full-text XML
-
Supplement
(448 KB) - BibTeX
- EndNote
In recent decades, the concentration of dissolved oxygen (DO) in the ocean, specifically in the tropics, has been decreasing. This not only increases the size of areas under hypoxia but also their prevalence (Breitburg et al., 2018). Hypoxia is ranked among the major threats to the actualization of the blue economy and the achievement of the 14th pillar of the Sustainable Development Goals (SDGs), specifically target 14.7, which aims at increasing the economic benefits of marine resource utilization through its sustainable use by developing countries (Rossi, 2023; Liu, 2023). Although hypoxia can result from natural phenomena like upwelling and thermal stratification, the current expansion of hypoxic areas is mainly a result of accelerated nutrient inputs in coastal areas; this increases algal production subsequently, resulting in higher organic matter production, which in turn results in increased aerobic microbial decomposition, lowering the levels of DO in the water (Gobler and Baumann, 2016). It has been projected that such changes may affect different organisms differently depending on their tolerance and reactions to lower dissolved oxygen in their habitats (Brodie Rudolph et al., 2020). Studies on ecosystems with hypoxia as a natural phenomenon can assist in predicting and understanding how human-induced hypoxia might affect and shape marine ecosystems in the face of the expansion of marine areas under hypoxia.
The Benguela Upwelling System (BUS) is located off the southwestern coast of Africa. It extends from Cape Frio in Angola to the southern tip of the continent in Cape Agulhas, South Africa, and is one of the most productive regions of the world's ocean (Magalhães, 2018). The high productivity provides a huge source of carbon resulting from photosynthesis, which gradually sinks through the water column, resulting in a rapid oxygen loss due to biochemical oxygen consumption. This consequently causes low dissolved oxygen concentrations and thus creates a permanent extensive shallow oxygen minimum zone (Bohata and Koppelmann, 2013; Emeis et al., 2018). When the oxygen minimum zone (OMZ) comes into contact with the seafloor, it creates a strong oxygen gradient at the benthic zone at depths ranging between 50 and 300 m, resulting in a hypoxic (< 0.5 mL L−1) inner shelf (Levin, 2003). The oxygen gradient created at the OMZ's benthic zone is believed to primarily regulate the benthic community distribution and diversity patterns (Zettler et al., 2013; Teuber et al., 2013). In these zones, microbial communities play a key role in the cycling of elements like carbon, nitrogen, and sulfur. The expansion of OMZs may increase nitrate use by nematode-associated microbes such as SAR11, a significant player in the marine nitrogen cycle. These nematodes and the communities they are part of influence biogeochemical processes and ecosystem dynamics within OMZs. For this reason, understanding these communities is crucial, especially with the ongoing expansion of OMZs due to climate change.
The general trend observed in most OMZs in global oceans, namely Walvis Bay (Namibia – the location of this study), California (USA), and the Oman margin (off the Arabian Peninsula), indicates that the densities of macrofauna (benthic organisms that are typically retained in a 0.5 mm sieve but pass through a 2.00 mm sieve; Bachelet, 1990) generally display a negative response to reducing oxygen levels within the OMZ, with a 30 % to 70 % reduction in densities in regions with less than 0.15 mL L−1 (Levin, 2003). Similarly, diversity is reduced as oxygen levels are reduced within the OMZ because of the loss of intolerant species and increased dominance of the tolerant species (Currie et al., 2018; Levin et al., 2009). Nematodes and some families from the annelid worms have been observed to be able to tolerate low oxygen, with Nematoda (in the meiofauna group) having 95 %–99 % abundance. Some polychaetes families like Spionidae, Dorvilleidae, and Lumbrineridae can also tolerate low oxygen in the OMZ by having a high gill surface area for increasing oxygen uptake. On the other hand, harpacticoid copepods are the most sensitive taxon to hypoxia (Levin et al., 2009; Zeppilli et al., 2015).
It has been observed that the macrofauna diversity is lowest off Walvis Bay, a city located on the western coast of Namibia, attributed to the perennial intense OMZ over the shelf (Currie et al., 2018). In contrast, the diversity increases significantly northwards off the Kunene River (Zettler et al., 2013), which flows from the highlands of Angola, along the border with Namibia, and into the Atlantic Ocean (see Fig. 1). This increase in diversity is proposed to be a result of moving away from the intense OMZ cells off Walvis Bay and also the reflection of the biogeography where diversity increases with lower latitude (Zettler et al., 2009). Outside the OMZ, bathymetry and latitude are said to be the factors affecting the infaunal communities at the Namibian shelf (Steffani et al., 2015).
This study aims to identify the relationship between the levels of dissolved oxygen and the macrobenthic community distribution across the northern Benguela Upwelling System as a guide on how the expansion of human-made hypoxia might influence the benthic fauna distribution on the seafloor.
2.1 Study area
The study area was located across the northern Benguela Upwelling System (between 26 and 20° S) along the Namibian continental shelf, which hosts a deep continental shelf (around 300 m) (Fig. 1). The intense upwelling in the study site has rendered the area highly productive, resulting in a characteristic shallow OMZ (50–200 m) with stronger productive cells around Lüderitz and Walvis Bay (Bohata and Koppelmann, 2013). The inner shelf is described to be extremely oxygen-depleted, caused by in situ organic matter decomposition and the warm poleward Angola current, which peaks in June–July while the continental slope below the OMZ is better-oxygenated (Levin et al., 2009; Emeis et al., 2004).
The benthic zone in the OMZ in northern BUS is characterized by extensive areas of diatomaceous mud, which are associated with high primary production at the ocean surface and low concentrations of dissolved oxygen (Levin et al., 2009; Steffani et al., 2015). These low oxygen levels, as per the findings of Levin et al. (2009), were found to be less than 0.5 mL L−1 in 55 % of the total shelf. Furthermore, extreme anoxia, defined as oxygen concentrations less than 0.02 mL L−1, was observed over an expansive area of almost 900 km2.
2.2 Sample collection
Samples were collected from three transects (off Lüderitz – OL at 26° S, off Walvis Bay – OWB at 23° S, and off Terrace Bay – OTB at 20° S) on board R/V Mirabilis during the 3rd Regional Research Graduate Network in Oceanography (RGNO) training and the National Marine Information and Research Centre's (NatMIRC's) plankton survey from 13 to 17 May 2016. The sampling stations were located at 2, 20, 40, or 70 nm from the shore at each transect, with the 26° S transect hosting only one station at 90 nm (Fig. 1). However, benthic samples from these stations were dependent on the prevailing weather conditions and the ability to get good core samples (Table 1).
Table 1Station information and abiotic factor information recorded from the stations in the northern Benguela Upwelling System. NR stands for no replicate.
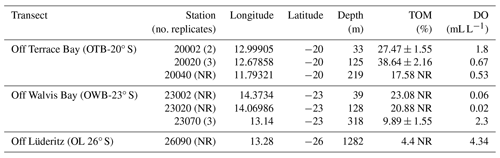
Sampling was done using a multi-corer from which individual cores were taken and subsampled for macrobenthos using a 6.4 cm diameter corer, and sediment samples for granulometry and organic matter analysis were taken simultaneously. Replicate samples were taken from a subsequent deployment of the multi-corer, where possible, to avoid pseudoreplication. The macrofauna cored samples were put in sampling bottles and immediately fixed with 5 % buffered formalin, while samples for organic matter analysis were frozen to arrest microbial decomposition.
Depth and temperature measurements were collected from a probe attached to the multi-corer, while dissolved oxygen concentrations in this study used the Winkler method from the overlying water (Montgomery et al., 1964).
2.3 Laboratory analysis
In the laboratory, macrobenthic samples were sieved between 2.00 and 0.45 mm sieves. Studies typically employ a sieve size of 0.5 mm; our study, however, utilized a 0.45 mm sieve due to its availability during the course of our research. This size, although slightly smaller, falls within the range of commonly used sieve sizes (0.3 to 0.5 mm) for macrofaunal research. The use of a 0.45 mm sieve is also not unprecedented in macrofaunal studies; studies by Li et al. (2018) and Zhang et al. (2022) successfully employed this sieve size. Therefore, while acknowledging the slight deviation, results remain valid and comparable to those obtained using the more common sieve sizes.
The sieve fraction retained in the 0.45 mm mesh sieve was then preserved in a 5 % buffered formalin solution, with three to five drops of rose bengal solution added to aid in sorting. After rinsing the samples with water, they were sorted under a dissecting microscope using the key provided by Thiel and Higgins (1988) to the class or order levels and then counted. Subsequently, the nematodes were pin-picked, fixed on permanent slides, and identified to the genus level using the key from Platt and Warwick (1988). Feeding types were determined for these genera following the methodology described by Wieser (1953), whereby nematodes are classified based on their buccal morphology, including shape, size, and presence or absence of buccal armature, resulting in four groups. Those lacking buccal armature are categorized as deposit feeders (group 1); they are further subdivided into selective deposit feeders (1A) and non-selective deposit feeders (1B) based on the size of their buccal cavity, with the former possessing a very small buccal cavity and the latter having a larger one (Moens and Vincx, 1997; Moens et al., 2013). Nematodes with buccal armature constitute group 2 and can be selective epigrowth feeders and herbivores (2A), using small teeth to graze diatoms, microalgae, and bacteria, or carnivores and omnivores (2B), characterized by the possession of teeth, mandibles, or onchia for piercing and consuming prey (Moens et al., 2013)
For sediment granulometry, samples were wet-sieved using 1.00, 0.5, 0.25, 0.105, and 0.063 mm sieves, and those below 0.063 mm (< 0.063 mm) were collected on a metallic pan. The sediments collected at the different sieves were then put on pre-weighed Petri dishes and dried in an oven at 60 °C until a constant weight was reached, and these dried weights were used for sediment granulometry (Wentworth, 1922).
The samples for total organic matter (TOM) were dried in an oven at 60 °C until a constant weight was achieved; a known weight of each sample was then placed in labeled aluminum foil, and the loss of weight on ignition (LOI) technique was used to determine TOM from the study site (Thiel and Higgins, 1988).
2.4 Data analysis
Out of the seven sampled stations, only three had replicates (Table 1), making direct statistical comparisons between stations impossible. To overcome this limitation, the sampling stations were grouped based on the measured environmental variable that provided the most significant relationship identified through stepwise regression analysis with the various biotic indices documented within the macrofauna community (see Supplement Tables S1–S4). The communities within these key “habitat types” were then compared using the indices and analyses outlined below.
Following the approach of Levin (2003), stations with DO levels less than 0.1 mL L−1 were grouped as “microxic”, those with DO levels between 0.1 and 1.0 mL L−1 were grouped as “dysoxic”, and those with DO levels of 1.0 mL L−1 and above were grouped as “oxic”. This approach was taken to investigate the role of DO levels in these communities. It was further justified by the application of stepwise regression using SPSS, which indicated that DO was a key determinant in macrobenthic community structures (R2=0.521, P=0.012) (Table S2a, b).
The data recorded were analyzed for abundance (density and relative abundance) in Excel. Additionally, the Paleontological Statistics Software package (PAST v2.17c) (Hammer et al., 2001) was used to calculate the diversity and the diversity t test between the stations. For this study, H′ diversity is calculated using the natural log (loge), while dominance is the complement of the Simpson index (1-Simpson index).
Bray–Curtis similarity, analysis of similarity (ANOSIM), and similarity percentages (SIMPER) were conducted using Plymouth Routines in Multivariate Ecological Research (PRIMER v5.2.9) (Clarke and Gorley, 2005).
3.1 Abiotic variables
Total organic matter (% TOM) demonstrated an inverse relationship with depth, with higher organic matter values recorded in the shallower stations. For example, the shallowest stations 20020, 20002, and 23002 had the highest TOM of 38.6 % ± 2.16 %, 27.5 % ± 1.55 %, and 23.1 %, respectively, while the lowest TOM values were found in most offshore stations, stations 23070 and 26090, with 9.89 ± 1.55 and 4.4, respectively (Table 1). The lowest oxygen values were recorded on the OWB 23° S transect, with the two most onshore stations (23020 and 23002) recording the lowest levels of oxygen (0.02 and 0.06 mL L-1,, respectively). The inshore station from transect OTB 20° S (20002) had a higher DO (1.8 mL L−1) than stations 20020 and 20040 from the same transect, where the DO levels recorded were 0.67 and 0.53 mL L−1, respectively, while the offshore stations 23070 in transect OWB 23° S and 26090 in transect OL 26° S recorded the highest dissolved oxygen (2.30 and 4.34 mL L−1, respectively) (Table 1).
Fine sand was the most common sediment size, ranging from 30 %–38.8 % in all stations. Most stations from the sites also recorded higher proportions of medium sand and silt, except for station 23020 in transect OWB 23° S, which recorded a lower proportion of silt and an increased abundance of coarse sand compared to the other stations (Table 2).
3.2 Macrobenthic assemblages
Macrofaunal densities differed significantly across the various oxygen zones (ANOVA; P<0.05). In the microxic stations, very low densities were observed (4661 ± 4834 ind. m−2) (Fig. 2), and the lowest number of taxa was recorded, with only six taxa present. These included Nematoda, Polychaeta, Oligochaeta, and Cumacea, the only peracarid crustacean found across all oxygen zones (Fig. 3). Despite the low number of taxa (S=6), these stations recorded slightly higher H′ diversity than the dysoxic stations, while the dominance index was intermediate (0.5 ± 0.18) (Fig. 5).
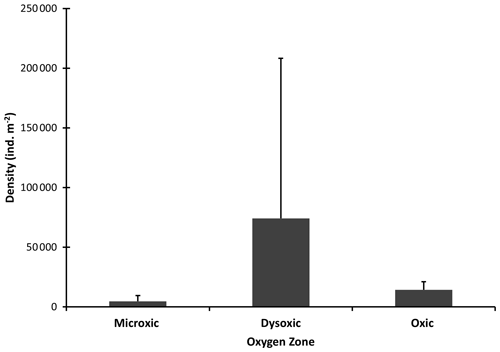
Figure 2Macrobenthic densities in the different oxygen zones in the northern Benguela Upwelling System. Error bars represent the standard deviation (+1 SD).
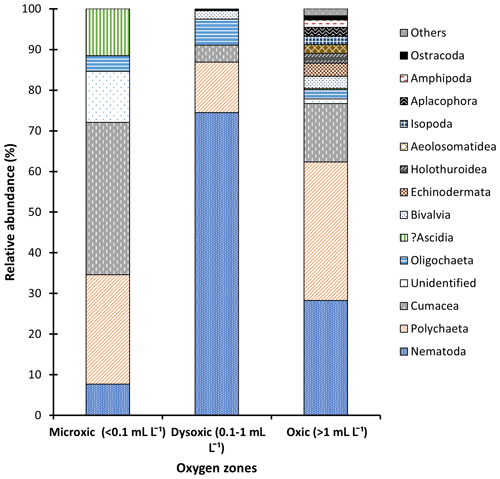
Figure 3Macrofauna relative abundance in the northern Benguela Upwelling System based on the oxygen zones identified.
Dysoxic stations in contrast recorded the highest densities (74 108 ± 134 126 ind. m−2) (Fig. 2). One station within this oxygen range, station (20040), recorded an extremely high density of 274 991 ind. m−2, primarily due to a high abundance of Nematoda and Oligochaeta (Fig. 3). Although the taxa in the dysoxic stations were similar to those of the microxic stations, the composition and dominance differed significantly. The macro-nematoda was the most dominant taxon in this zone, with numbers reaching as high as 233 354 ind. m−2 in one of the stations. Interestingly, Bivalvia and Ostracoda, absent in the microxic stations, were present here, albeit in low abundance (Fig. 3). Dominance was highest in these stations (0.6 ± 0.28) (Fig. 5).
Oxic stations demonstrated the highest number of taxa (18) and the second-highest average density (14 345 ± 6726 ind. m−2) (Fig. 2). These stations recorded taxa like Echinodermata (Ophiuroidea), Holothuroidea, Aeolosomatidea, Isopoda, Aplacophora, and Amphipoda, which all had average abundances of more than 1 %. Nemertina, Turbellaria, and Cnidaria were also recorded in these stations; however, their abundances were below 1 %, and they were grouped as “others” (Fig. 3). These stations recorded the highest H′ diversity (1.46 ± 0.4) and the lowest dominance (0.31 ± 0.18) (Fig. 5).
All the oxygen zones were dissimilar to one another based on multivariate community analysis using Bray–Curtis analysis of dissimilarity. The highest dissimilarity was observed between the dysoxic and microxic zones, which were 77.99 % dissimilar despite both stations being characterized by low dissolved oxygen levels. The oxic stations were also highly dissimilar to the microxic and dysoxic zones, with values of 68.58 % and 65.91 %, respectively (Fig. 4).
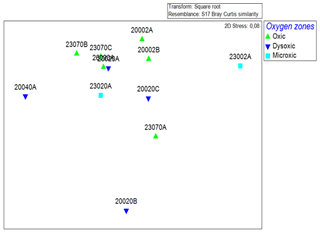
Figure 4Non-metric multidimensional scaling (nMDS) plot based on the Bray–Curtis similarity index of macrobenthic fauna communities recorded in the northern Benguela Upwelling System.
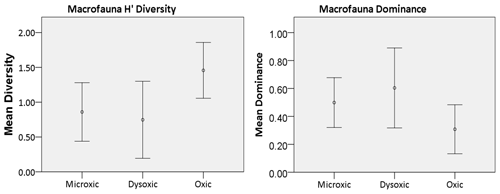
Figure 5Macrofauna diversity indices recorded in the various oxygen zones in the northern Benguela Upwelling System. H′ is diversity, calculated using the natural logarithm (loge), while dominance represents the complement of the Simpson index (1-Simpson index). Error bars represent the standard deviation (± 1 SD).
3.3 Macro-nematode density and diversity
On average, macro-nematodes were the most dominant taxon in this study as a result of their dominance in the dysoxic station. Nematodes were abundant in all oxygen zones, recording relative abundances of 8 %, 74 %, and 24 % in microxic, dysoxic, and oxic zones, respectively (see Fig. 3).
In this study, 18 different genera of macro-nematodes were identified. Notably, Desmolaimus and Paracomesoma were detected across all stations and were the only genera present in microxic stations. These two, along with Metoncholaimus, exhibited their highest abundance in dysoxic stations. Additionally, in these dysoxic environments, Paralongicyatholaimus and Neochromadora recorded high abundances, each constituting > 4 % of the total population (Fig. 6). Thalassolaimus, Paramesacanthion, Enoploides, Halanonchus, Rhabdodemania, and Dorylaimopsis recorded high abundances in oxic stations but were absent in dysoxic stations except for Thalassolaimus. Metoncholaimus and Paralongicyatholaimus were present in dysoxic stations but absent in oxic stations, while Paramesacanthion, Enoploides, and Rhabdodemania were present in the oxic station and absent in the dysoxic stations. For the purposes of graphing the relative abundance, Thoracostomopsis, Anticoma, Cephalanticoma, Trileptium, Mesacanthoides, Terschellingia, and Marylinnia were grouped as “others” as they recorded low abundances (< 4 %) and were absent in dysoxic stations except for Marylinnia and Terschellingia; the former was absent in the oxic station, while the latter was present in both oxygen zones (see Fig. 6).
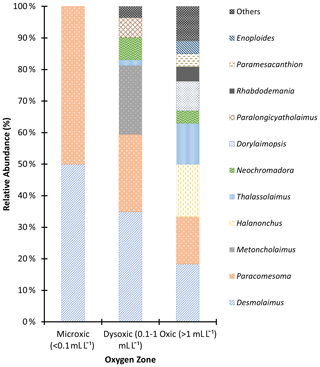
Figure 6Macronematoda relative abundance in the northern Benguela Upwelling System based on the oxygen zones identified.
The feeding guild composition exhibited variation across distinct oxygen zones within the study site. Selective epigrowth feeders and herbivores, categorized as Type 2A, emerged as the predominant nematodes within dysoxic zones, comprising 40 % of the population. This was closely followed by non-selective deposit feeders (Type 1B), contributing to 35 % of nematodes within the same zone. Carnivores and omnivores, classified as Type 2B, constituted 28 % of the nematode population, while selective deposit feeders and bacteriovores, categorized as Type 1A, accounted for 10 %. Conversely, in the oxic zone, non-selective deposit feeders (Type 1B) and selective epigrowth feeders and herbivores (Type 2A) predominated, representing 35 % and 32 % of the nematodes, respectively. Carnivores and omnivores (Type 2B) as well as selective deposit feeders (Type 1A) accounted for 18 % and 15 %, respectively (Fig. 7). Within the feeding modes, selective deposit feeders had the largest differences in composition between the two oxygen zones, with the highest concentration observed in the oxic zones, while 2A and 2B had slightly higher values in the dysoxic zone compared to the oxic zones.
The nematode diversity exhibited a pattern akin to the overall macrofaunal diversity, wherein one of the microxic stations (23002) was devoid of nematodes, while its counterpart station (23020) harbored only two nematodes. Consequently, microxic stations were excluded from the diversity analysis. Across the remaining oxygen ranges (dysoxic and oxic), a parallel trend was observed as in the broader macrofaunal analysis within the study area. Specifically, oxic stations displayed higher H′ diversity (1.38 ± 0.5) compared to dysoxic stations (0.81 ± 0.84). Conversely, dysoxic stations exhibited greater dominance (0.59 ± 0.39) relative to oxic stations (0.32 ± 0.18) (Fig. 8).
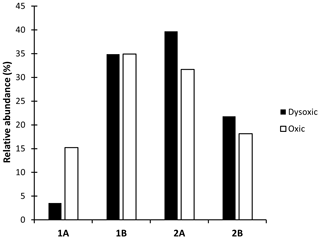
Figure 7Feeding guilds based on Wieser (1953) of macro-nematoda from the northern Benguela Upwelling System (1A: selective deposit feeder, 1B: non-selective deposit feeder, 2A: epigrowth feeders, 2B: predators and omnivores).
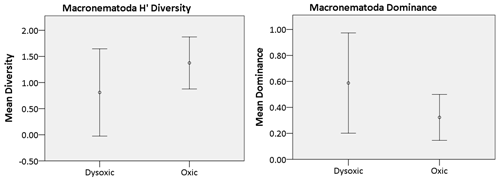
Figure 8Macro-nematoda diversity indices recorded in the various oxygen zones in the northern Benguela Upwelling System. H′ is diversity, calculated using the natural logarithm (loge), while dominance represents the complement of the Simpson index (1-Simpson index). Error bars represent the standard deviation (± 1 SD).
Upwelling systems are known for their high surface productivity and oxygen minimum zones (OMZs), which impinge on the benthic zone, creating strong oxygen gradients on the seafloor and acting as the dominant driver for benthos diversity in these zones (Zettler et al., 2013). Despite the Benguela Upwelling System (BUS) being recognized as one of the major eastern boundary upwelling systems, there is limited information on the structure and composition of the benthic communities. Information on macrofauna communities in the BUS will not only improve the existing database on benthic fauna but also provide insight into how increasing hypoxic areas in the ocean might structure benthic communities.
In this study, we adapted Levin's grouping system (Levin, 2003), classifying the different stations into three zones based on the oxygen levels recorded (microxic zone, < 0.1 mL L−1; dysoxic zone, 0.1–1.0 mL L−1; oxic zone, > 1.0 mL L−1), which was supported by stepwise regression. The structure and composition of the macrofauna communities varied among the various oxygen zones. Most macrofaunal studies identify Polychaeta as the most abundant macrofauna taxon in both oxic and hypoxic areas (Eisenbarth and Zettler, 2016; Soto et al., 2017). However, in this study, Polychaeta only had the highest relative abundance in the oxic zones. In the microxic zone, the abundant taxon was Cumacea, followed by Polychaeta. It is essential to note that numerically Polychaeta was the most abundant taxon in this oxygen zone, but the presence of many individuals of other taxa in one of the microxic stations reduced their proportional representation. The presence of cumaceans in high abundance in the core OMZ, comparable to the microxic zone in this study, has been reported by Zettler et al. (2013) and Eisenbarth and Zettler (2016), who described them as possible opportunistic species colonizing permanent hypoxic areas from adjacent areas, and thus their abundance may be season-specific. Currie et al. (2018) attributed the presence of Cumacea and other macrofauna taxa in the BUS to the sulfur-oxidizing bacteria, possibly providing a detoxified condition in this area. In this case, the mobility of the cumaceans gives them an advantage over other tolerant taxa like polychaetes and nematodes at the core and hence their high relative abundances at the OMZs core.
Some polychaete families have physiological adaptations to tolerate the low oxygen concentrations found in OMZs (Hanz et al., 2019; Joydas and Damodaran, 2014; Levin et al., 2009). At the microxic zone, polychaetes had the numerical abundance in the microxic stations despite cumaceans leading in relative abundance. In station 23002, where Cumacea was located, only two taxa were present. As a result, cumaceans accounted for 75 % of the population despite having a density of only 932 ind. m−2. In contrast, Polychaeta in station 23020 had a numerical high of 4350 ind. m−2, which is relatively higher than the number recorded by cumaceans in station 23002. However, the diversity of other taxa in this station was much higher, and as a result, despite the high density, Polychaeta's proportion of the whole population was reduced to 53 %, hence the lower relative abundance.
In the dysoxic zone, the macro-nematoda was the most dominant taxon, outcompeting the polychaetes in all the dysoxic stations, recording more than 70 % relative abundance. Oxygen can cause shifts in community structure and trophic transfer (Neira et al., 2018), as evidenced in this study. Nematoda as a taxon has not received significant attention in the macrofaunal size range in most studies despite evidence of its presence therein (Joydas and Damodaran, 2014; Sharma et al., 2011). An increase in the size of nematodes to macrobenthic class sizes has been reported in chemosynthetic environments that experience similar characteristics as OMZs, i.e., low oxygen and high sulfidic contents (Vanreusel et al., 2010). Apart from the increase in size, OMZs also tend to enhance the regional dominance of tolerant organisms such as nematodes with high biomass recorded in response to organic matter inputs. The high abundances are thought to reflect the availability of organic matter, a significant nutrient source for macrofauna, coupled with a reduction in predation by larger fauna that are adversely affected by the reduced oxygen concentrations (Moens et al., 2013).
High nematode abundance in partnership with Oligochaeta occurred in the dysoxic zone; oxygen conditions were low enough to exclude some taxa but sufficient for tolerant species to survive and reproduce. Such conditions are referred to as the “edge effect”, and such high densities are characteristics of the edge of the OMZs, where various species have been observed to have abnormally high densities. The reasons for these high abundances are not well understood, but Gutiérrez et al. (2008) suggested that the nematode population can multiply in low oxygen conditions, which experience high loads of organic matter input. Despite their tolerance to anoxia, nematodes cannot survive long-term exposure, as observed in the microxic zone (Moens et al., 2013). It is not clearly understood then whether the high abundance of nematodes in one of the stations is characteristic of the study site or just congregation to a food source. Thus, the patchiness and high variability in the dysoxic zone call for more studies (Buhl-Mortensen et al., 2010).
At dysoxic sites (DO 0.1–1.0 mL L−1), other taxa like Ostracoda and Bivalvia were observed, albeit in low numbers. Despite ostracods flourishing better in well-oxygenated marine areas, various families (Platycopina) have been observed to tolerate and thrive in oxygen minimum zones. At oxic sites, where DO levels were above 1.0 mL L−1, more taxa were recorded, and these numbers increased even more when DO levels surpassed 2.0 mL L−1.
Our findings were in line with the various studies in OMZs whereby a decrease in richness and diversity of macrofaunal species is observed towards the core and vice versa (McClain and Schlacher, 2015). In the core (microxic) area, the macrofauna taxa had the lowest density and diversity. Each square meter of core area contained 1243 individuals, indicating low species density. A similar number was recorded by Zettler et al. (2009). In this study, an increase in the number of taxa recorded in sites with DO levels above 1 mL L−1 was also observed. The high dominance of the tolerant taxa, as evidenced in the microxic and dysoxic areas, was reduced. Taxa such as Amphipoda, Isopoda, Echinodermata, Nemertina, Aeolosomatidae, Aplacophora, Holothuroidea, and Cnidaria were only recorded in the oxic zones, indicating low tolerance to low oxygen levels. Of this fauna, crustaceans were the most abundant. This conforms to the observations of Soto et al. (2017) at oxic sites in an upwelling system in Chile. Conversely, Zettler et al. (2009) recorded amphipod species in low-oxygen areas. These contradictory results indicate that, at least amongst the Amphipoda, tolerance and intolerance to hypoxia are species-specific.
Macro-nematoda abundance varied across the OMZ, with very low abundance in the microxic zones, extremely high numbers at the dysoxic zones, and a substantial amount at the oxic stations, accounting for only 28 % of the total abundance. Nematodes are considered one of the most tolerant taxa in the marine environment, with the ability to tolerate low-oxygen and highly sulfidic environments characteristic of OMZs, and may reach very high abundances in these environments (Neira et al., 2018; Gutierrez et al., 2008). This was the case at the dysoxic zone, where nematodes recorded high abnormal densities in one station, indicating the high tolerance of these taxa and their ability to dominate the macrofaunal component. Even with such high tolerance levels, nematode abundance can be impacted by microxic conditions, as observed in the microxic areas with a recording of only 621 nematodes per square meter in this oxygen zone. This low value, however, may be at the macrofauna level, and the case may be different at the meiofauna level, where nematodes have recorded substantial densities in microxic environments (Neira et al., 2018; Steyaert et al., 2007).
Despite their high abundance in OMZs, not all nematodes are tolerant to low oxygen levels (Moens et al., 2013), as observed in this study. Metoncholaimus, Paracomesoma, and Desmolaimus dominated the dysoxic zone; these three genera are members of Oncholaimidae, Comesomatidae, and Linhomoeidae, respectively. Members of the family Oncholaimidae have large bodies that can disperse rapidly and colonize carcasses of macrofauna and even fish that may have succumbed to the low levels of oxygen found in the dysoxic zone (Moens et al., 2013). Nevertheless, their bodies are large enough to fit within the macrofauna size range, while their ability to swim ensures they actively locate their food source (Moens et al., 2013). Their high abundance in this study might reflect a congregation upon a food source that had attracted nearby members in large numbers. Their ability to colonize the “food source” in such numbers in a dysoxic environment indicates their tolerance to low oxygen levels.
On the other hand, Comesomatidae and Linhomoeidae members have been noted to have high abundances in enriched sediments with low oxygen, indicating tolerance to anoxic conditions (Steyaert et al., 2007). Their long and slender bodies might be the reason for their records at the macrofauna level. Despite this generalization at the family level and the assumptions that members of the same family may portray similar life strategies (Bongers et al., 1991), tolerance of nematodes to hypoxia is species-specific (Moens et al., 2013) as Steyaert et al. (2007) observed members of the same genera (Sabatiera) reacting differently to hypoxic and anoxic conditions. Thus, further analysis should be done to identify the species that are tolerant to hypoxia at these OMZs at macrofauna levels. Tolerance to hypoxia is indicated by both the presence and absence of taxa; most genera present in the oxic zone were absent in the dysoxic area and may be seen as genera intolerant to low oxygen levels.
Wieser's feeding types, as outlined in his study (Wieser, 1953), have long been used to assess the trophic structure of nematode communities. This study had a high abundance of non-selective feeders (1B) and selective epigrowth feeders and herbivores (2A), with the latter dominating the dysoxic zone, while the former dominated the oxic zones. Non-selective deposit feeders are commonly observed to dominate substrates characterized by high levels of organic matter and smaller grain size, indicating their adaptation to environments with elevated organic matter input, as observed in this study (Singh and Ingole, 2016; Heip et al., 1985). In contrast to previous findings on meiofaunal nematodes from oxygen minimum zones (OMZs) (Singh and Ingole, 2016; Neira et al., 2013) and macro-nematodes in deep-sea habitats (Sharma and Bluhm, 2011), which reported lower abundances of selective epigrowth feeders, our study revealed a higher abundance of selective epigrowth feeders and herbivores (2A). Within OMZs, such as our study site, the presence of giant bacteria such as Thioploca, Beggiatoa, and Thiomargarita forming extensive mats (Brüchert et al., 2009) may provide a substantial food source for nematodes (Giere, 2008).
Adjacent to OMZs, the prevalence of diatomaceous mud and the settling of fresh phytodetritus contribute significantly to the diet of epistratum feeders, leading to their high abundance in dysoxic areas (Singh and Ingole, 2016; Sharma and Bluhm, 2011; Moens and Vincx, 1987; Heip et al., 1985). However, in regions offshore from the OMZ, where the OMZ no longer interacts with the benthic zone, reduced diatom production diminishes the abundance of epistratum feeders, resulting in the dominance of non-selective deposit feeders.
While non-selective deposit feeders typically dominate muddy and silty environments, selective deposit feeders and carnivores have been observed to exhibit lower abundances in these habitats (Heip et al., 1985). Our study aligns with this trend; however, other investigations have reported contradictory findings, with selective deposit feeders being identified among the most dominant nematodes in similar environments (Neira et al., 2013; Singh and Ingole, 2016). Additionally, carnivores and omnivores (2B) exhibited higher abundances in the dysoxic zone compared to the oxic zone, with their overall abundance surpassing that of selective deposit feeders. These observations suggest potential differences in nematode composition between the meiofaunal and macrofaunal levels. Sharma and Bluhm (2011) observed that category 2B feeders were the second most abundant feeding type in numerous stations within the Arctic deep-sea Canada Basin. They attributed these findings to the omission of larger nematodes in previous studies, as carnivores and omnivores are often large-bodied species.
In conclusion, this study provides insights into the intricate dynamics of macrofaunal communities in oxygen minimum zones (OMZs). By classifying stations into microxic, dysoxic, and oxic zones based on oxygen levels, we observed variations in macrofaunal composition and abundance across these zones. Contrary to typical findings, Polychaeta dominated only in oxic zones, while Cumacea and Nematoda were prevalent in microxic and dysoxic zones, respectively. The presence of tolerant taxa like nematodes and Oligochaeta in dysoxic zones suggests their ability to thrive under low oxygen conditions. Conversely, taxa intolerant to low oxygen were absent in dysoxic zones, highlighting the importance of oxygen levels in shaping macrofaunal communities.
Macro-nematodes in general were the most abundant taxon. The high nematode densities suggest the ability of macro-nematodes to tolerate and thrive in low-oxygen environments. Our findings highlight the importance of considering macro-nematodes in studies of OMZ ecosystems and underscore the need for further research to elucidate their ecological roles and responses to environmental changes. Understanding the dynamics of macro-nematode populations coupled with their meiofaunal counterparts in OMZs is crucial for comprehensively assessing the impacts of oxygen depletion on benthic communities and ecosystem functioning.
Currently, the data are not available but can be released upon request to the authors.
The supplement related to this article is available online at: https://doi.org/10.5194/bg-21-2995-2024-supplement.
SMH was responsible for the conceptualization of the study. SMH and BWW conducted the investigation, formal analysis, and drafting of the original paper. AM was responsible for supervision, review, and editing of the final draft.
The contact author has declared that none of the authors has any competing interests.
Publisher's note: Copernicus Publications remains neutral with regard to jurisdictional claims made in the text, published maps, institutional affiliations, or any other geographical representation in this paper. While Copernicus Publications makes every effort to include appropriate place names, the final responsibility lies with the authors.
This article is part of the special issue “Low-oxygen environments and deoxygenation in open and coastal marine waters”. It is a result of the 53rd International Colloquium on Ocean Dynamics (3rd GO2NE Oxygen Conference), Liège, Belgium, 16–20 May 2022.
The authors would like to thank the 3rd Regional Research Graduate Network in Oceanography (RGNO) organizers, all the sponsors, and participants for technically and financially supporting the authors in the participation and subsequent sampling that resulted in the successful completion of this study. The assistance from the Namibian National Marine Information and Research Centre (NatMIRC) was instrumental in successfully sampling, processing, and transporting samples. The crew of the sampling vessel R/V Mirabilis ensured a smooth sampling experience, and hence we take this opportunity to appreciate their services.
We also extend our gratitude to the reviewers for their invaluable feedback and constructive criticism, which significantly enhanced the quality and clarity of this paper. We deeply appreciate their time, expertise, insights, suggestions, and dedication to improving the paper. Their contributions have undoubtedly strengthened the final version of this paper, and we are sincerely grateful for their inputs.
This paper was edited by Lisa Levin and reviewed by Simon Forster and one anonymous referee.
Bachelet, G.: The choice of a sieving mesh size in the quantitative assessment of marine macrobenthos: a necessary compromise between aims and constraints, Mar. Environ. Res., 30, 21–35, 1990.
Bohata, K. and Koppelmann, R.: Chaetognatha of the Namibian upwelling region: taxonomy, distribution and trophic position, PloS One, 8, e53839, https://doi.org/10.1371/journal.pone.0053839, 2013.
Bongers, T., Alkemade, R., and Yeates, G. W.: Interpretation of disturbance-induced maturity decrease in marine nematode assemblages by means of the Maturity Index, Mar. Ecol.-Prog. Ser., 76, 135–142, https://doi.org/10.3354/meps076135, 1991.
Breitburg, D., Levin, L. A., Oschlies, A., Grégoire, M., Chavez, F. P., Conley, D. J., Garçon, V., Gilbert, D., Gutiérrez, D., and Isensee, K.: Declining oxygen in the global ocean and coastal waters, Science, 359, 6371, https://doi.org/10.1126/science.aam7240, 2018.
Brodie Rudolph, T., Ruckelshaus, M., Swilling, M., Allison, E. H., Österblom, H., Gelcich, S., and Mbatha, P.: A transition to sustainable ocean governance, Nat. Commun., 11, 3600, https://doi.org/10.1038/s41467-020-17410-2, 2020.
Brüchert, V., Currie, B., and Peard, K. R.: Hydrogen sulphide and methane emissions on the central Namibian shelf, Prog. Oceanogr., 83, 169–179, 2009.
Buhl-Mortensen, L., Vanreusel, A., Gooday, A. J., Levin, L. A., Priede, I. G., Buhl-Mortensen, P., Gheerardyn, H., King, N. J., and Raes, M.: Biological structures as a source of habitat heterogeneity and biodiversity on the deep ocean margins, Mar. Ecol., 31, 21–50, 2010.
Clarke, K. R. and Gorley, R. N.: PRIMER: Plymouth Routines In Multivariate Ecological Research, PRIMER-E Ltd., Plymouth, 2005.
Currie, B., Utne-Palm, A. C., and Salvanes, A. G. V.: Winning ways with hydrogen sulphide on the Namibian shelf, Front. Mar. Sci., 5, 341, https://doi.org/10.3389/fmars.2018.00341, 2018.
Eisenbarth, S. and Zettler, M. L.: Diversity of the benthic macrofauna off northern Namibia from the shelf to the deep sea, J. Mar. Syst., 155, 1–10, 2016.
Emeis, K., Brüchert, V., Currie, B., Endler, R., Ferdelman, T., Kiessling, A., Leipe, T., Noli-Peard, K., Struck, U., and Vogt, T.: Shallow gas in shelf sediments of the Namibian coastal upwelling ecosystem, Cont. Shelf. Res., 24, 627–642, https://doi.org/10.1016/j.csr.2004.01.007, 2004.
Emeis, K., Eggert, A., Flohr, A., Lahajnar, N., Nausch, G., Neumann, A., Rixen, T., Schmidt, M., Van der Plas, A., and Wasmund, N.: Biogeochemical processes and turnover rates in the Northern Benguela Upwelling System, J. Mar. Syst., 188, 63–80, 2018.
Giere, O.: Meiobenthology: the microscopic motile fauna of aquatic sediments, Springer Science and Business Media, Springer-Verlag, Berlin, Heidelberg, Germany, e-ISBN 978-3-540-68661-3, 2008.
Gobler, C. J. and Baumann, H.: Hypoxia and acidification in ocean ecosystems: Coupled dynamics and effects on marine life, Biol. Lett., 12, 27146441, https://doi.org/10.1098/rsbl.2015.0976, 2016.
Gutiérrez, D., Enríquez, E., Purca, S., Quipúzcoa, L., Marquina, R., Flores, G., and Graco, M.: Oxygenation episodes on the continental shelf of central Peru: Remote forcing and benthic ecosystem response, Prog. Oceanogr., 79, 177–189, 2008.
Hammer, Ø., Harper, D. A. T., and Ryan, P. D.: PAST: paleontological statistics software package for education and data analysis, Palaeontol. Electron., 4, 9 pp., 2001.
Hanz, U., Wienberg, C., Hebbeln, D., Duineveld, G., Lavaleye, M., Juva, K., Dullo, W.-C., Freiwald, A., Tamborrino, L., Reichart, G.-J., Flögel, S., and Mienis, F.: Environmental factors influencing benthic communities in the oxygen minimum zones on the Angolan and Namibian margins, Biogeosciences, 16, 4337–4356, https://doi.org/10.5194/bg-16-4337-2019, 2019.
Heip, C., Vincx, M., and Vranken, G.: The ecology of marine nematodes, Oceanogr. Mar. Biol., 23, 399–489, 1985.
Joydas, T. V. and Damodaran, R.: Infaunal macrobenthos of the oxygen minimum zone on the Indian western continental shelf, Mar. Ecol., 35, 22–35, 2014.
Levin, L. A.: Oxygen minimum zone benthos: adaptation and community response to hypoxia, Oceanogr. Mar. Biol. Annu. Rev, 41, 1–45, 2003.
Levin, L. A., Ekau, W., Gooday, A. J., Jorissen, F., Middelburg, J. J., Naqvi, S. W. A., Neira, C., Rabalais, N. N., and Zhang, J.: Effects of natural and human-induced hypoxia on coastal benthos, Biogeosciences, 6, 2063–2098, https://doi.org/10.5194/bg-6-2063-2009, 2009.
Li, S., Cui, B., Xie, T., Bai, J., Wang, Q., and Shi, W.: What drives the distribution of crab burrows in different habitats of intertidal salt marshes, Yellow River Delta, China. Ecol. Indic., 92, 99–106, 2018.
Liu, S.: Interactions within Sustainable Development Goals (SDGs): the economic and environmental dimensions of SDG Indicator 14.7, in: Interlinkages Between the Sustainable Development Goals, edited by: Swain, R. B. and Min, Y., Edward Elgar Publishing, https://doi.org/10.4337/9781803924946.00008, 37-51, 2023.
Magalhães, A. V.: Comparison of zooplankton communities between cold and hot seasons in Lobito-Angola, Doctoral thesis, KOICA-PKNU International Graduate Program of Fisheries Science, Graduate School of Global Fisheries, Pukyong National University, 2018.
McClain, C. R. and Schlacher, T. A.: On some hypotheses of diversity of animal life at great depths on the sea floor, Mar. Ecol., 36, 849–872, 2015.
Moens, T. and Vincx, M.: Observations on the feeding ecology of estuarine nematodes, J. Mar. Biol. Assoc. UK, 77, 211–227, 1997.
Moens, T., Braeckman, U., Derycke, S., Fonseca, G., Gallucci, F., Gingold, R., Guilini, K., Ingels, J., Leduc, D., and Vanaverbeke, J.: Ecology of free-living marine nematodes, in: Handbook of Zoology, Nematoda, edited by: Schmidt-Rhaesa, A., De Gruyter, Vol. 2, 109–152, https://doi.org/10.1515/9783110274257.109, 2013.
Montgomery, H., Thom, N. S., and Cockburn, A.: Determination of dissolved oxygen by the Winkler method and the solubility of oxygen in pure water and sea water, J. Appl. Chem., 14, 280–296, 1964.
Neira, C., Ingels, J., Mendoza, G., Hernandez-Lopez, E., and Levin, L. A.: Distribution of meiofauna in bathyal sediments influenced by the oxygen minimum zone off Costa Rica, Front. Mar. Sci., 5, 448, https://doi.org/10.3389/fmars.2018.00448, 2018.
Neira, C., King, I., Mendoza, G., Sellanes, J., De Ley, P., and Levin, L. A.: Nematode community structure along a central Chile margin transect influenced by the oxygen minimum zone, Deep-Sea Res. Pt. I, 78, 1–15, 2013.
Platt, H. M. and Warwick, R. M.: Freeliving marine nematodes: Part II. British Chromadorida, Synopses of the British Fauna No. 38, edited by: Brill, E. J., Dr. W. Backhuys for the Linnean Society of London and the Estuarine and Brackish-water Sciences Association, 1988.
Rossi, S.: A Comprehensive Overview of SDG 14: Life Below Water_Final, in: SDG 14: Life Below Water, Springer, Cham, 1–62, https://doi.org/10.1007/978-3-031-19467-2_1, 2023.
Sharma, J. and Bluhm, B. A.: Diversity of larger free-living nematodes from macrobenthos (> 250 µm) in the Arctic deep-sea Canada Basin, Mar. Biodivers., 41, 455–465, https://doi.org/10.1007/s12526-010-0060-1, 2011.
Sharma, J., Baguley, J., Bluhm, B. A., and Rowe, G.: Do meio-and macrobenthic nematodes differ in community composition and body weight trends with depth?, PLoS One, 6, e14491, https://doi.org/10.1371/journal.pone.0014491, 2011.
Singh, R. and Ingole, B. S.: Structure and function of nematode communities across the Indian western continental margin and its oxygen minimum zone, Biogeosciences, 13, 191–209, https://doi.org/10.5194/bg-13-191-2016, 2016.
Soto, E., Quiroga, E., Ganga, B., and Alarcón, G.: Influence of organic matter inputs and grain size on soft-bottom macrobenthic biodiversity in the upwelling ecosystem of central Chile, Mar. Biodivers., 47, 433–450, 2017.
Steffani, N., Sedick, S., Rogers, J., and Gibbons, M. J.: Infaunal benthic communities from the inner shelf off Southwestern Africa are characterised by generalist species, Plos One, 10, e0143637, https://doi.org/10.1371/journal.pone.0143637, 2015.
Steyaert, M., Moodley, L., Nadong, T., Moens, T., Soetaert, K., and Vincx, M.: Responses of intertidal nematodes to short-term anoxic events, J. Exp. Mar. Biol. Ecol., 345, 175–184, https://doi.org/10.1016/j.jembe.2007.03.001, 2007.
Teuber, L., Schukat, A., Hagen, W., and Auel, H.: Distribution and ecophysiology of calanoid copepods in relation to the oxygen minimum zone in the eastern tropical Atlantic, PloS One, 8, e77590, https://doi.org/10.1371/journal.pone.0077590, 2013.
Thiel, H. and Higgins, R. P.: Introduction to the study of meiofauna, Smithsonian Institution Press, Washington D.C., 488 pp., ISBN 0-87474-488-1, 1988.
Vanreusel, A., De Groote, A., Gollner, S., and Bright, M.: Ecology and biogeography of free-living nematodes associated with chemosynthetic environments in the deep sea: a review, PLoS One, 5, e12449, https://doi.org/10.1371/journal.pone.0012449, 2010.
Wieser, W.: Die Beziehung zwischen Mundhohlengestalt, Emahrungsweise und Vorkommen bei freilebenden marinen Nematoden, Eine skologisen-morphologische studie, Ark. Zool., 4, 439–484, 1953.
Wentworth, C. K.: A scale of grade and class terms for clastic sediments, J. Geol., 30, 377–392, 1922.
Zeppilli, D., Sarrazin, J., Leduc, D., Arbizu, P. M., Fontaneto, D., Fontanier, C., Gooday, A. J., Kristensen, R. M., Ivanenko, V. N., and Sørensen, M. V.: Is the meiofauna a good indicator for climate change and anthropogenic impacts?, Mar. Biodivers., 45, 505–535, 2015.
Zettler, M. L., Bochert, R., and Pollehne, F.: Macrozoobenthos diversity in an oxygen minimum zone off northern Namibia, Mar. Biol., 156, 1949–1961, 2009.
Zettler, M. L., Bochert, R., and Pollehne, F.: Macrozoobenthic biodiversity patterns in the northern province of the Benguela upwelling system, Afr. J. Mar. Sci., 35, 283–290, 2013.
Zhang, Z., Xia, S., Sun, X., and Zhou L.: Effects of Macrobenthos Relative to Floating-Leaved Plants on the Wintering Shorebird Assemblages at Shengjin Lake, China, Diversity, 14, 1072, https://doi.org/10.3390/d14121072, 2022.