the Creative Commons Attribution 4.0 License.
the Creative Commons Attribution 4.0 License.
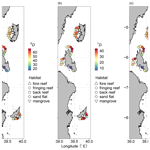
Reefal ostracod assemblages from the Zanzibar Archipelago (Tanzania)
Moriaki Yasuhara
Chih-Lin Wei
Tropical reefs encompass tremendous biodiversity yet are imperiled by increasing natural and anthropogenic disturbances worldwide. Meiobenthic biota on coral reefs, for example, ostracods, may experience substantial diversity loss and compositional changes even before being examined. In this study, we investigated the reefal ostracod assemblages from the highly diverse and productive ecosystem in the Zanzibar Archipelago (Pemba, Zanzibar, and Mafia islands), Tanzania, to understand how their diversity and faunal structure vary in response to water depth, benthic community type, and human impacts. We characterized four distinct ostracod faunas associated with different benthic habitats, which were deep fore reefs, shallow fringing reefs, degraded fringing reefs, and algae-covered intertidal flats. We identified typical ostracod associations, i.e., Bairdiidae versus Loxoconchidae–Xestoleberididae, that showed affinities towards hard corals or algae on the reef platforms, respectively. The highest diversity was found on shallow fringing reefs where coral-affined and algae-affined taxa exhibited maximum overlap of their distributional ranges, while the sand flats, mangrove, and marginal reefs within the intertidal zone had much lower diversity with a high dominance of euryhaline taxa. Along the western coast of Zanzibar Island, coastal development likely resulted in a unique faunal composition and comparatively low diversity of ostracod assemblages among those in reefal habitats, in conjunction with overall reef ecosystem degradation. This study represents the first large-scale assessment of shallow-marine ostracods in the Zanzibar Archipelago. It lays a solid foundation for future research into the ecological significance of ostracods on coral reefs.
- Article
(5300 KB) - Full-text XML
-
Supplement
(772 KB) - BibTeX
- EndNote
Coral reefs as the most diverse ecosystem in the marine realm hold great ecological and economic values, yet our knowledge of their enormous biodiversity is far from complete. Compared with well-studied, conspicuous macrofauna (Souza et al., 2023), meiofauna on coral reefs are highly under-represented in current research despite being ecologically essential components and contributing significantly to total biodiversity (Leray and Knowlton, 2015; Plaisance et al., 2011). Ostracoda (Crustacea) among all meiobenthos has a tight association with reef environments tracing back to the lower Paleozoic (Whatley and Watson, 1988). It is considered a useful model organism in modern- and paleo-biodiversity research because of its high fossilization potential, high abundance, and ubiquity in almost all marine ecosystems (Yasuhara et al., 2017). However, ostracods on coral reefs are poorly understood. Do ostracods exhibit higher diversity in reefal habitats compared with other soft-sediment environments? What are the characteristic ostracod taxa occupying different niches on coral reefs? Answers to these questions are important for a holistic understanding of the reef ecosystem and may hint at the underlying mechanisms that support such extraordinary reef diversity. With intensifying anthropogenic disturbances at local to global scales, the need to examine reefal ostracods before they perish is pressing.
Studies targeting tropical shallow-marine ostracods on coral reefs are surprisingly deficient. Across the circumtropical belt, the central Indo-Pacific receives the most attention for its diverse reefal ostracods, with pioneering studies identifying distinct faunas associated with depth habitats from the shallow intertidal to deep reefal zones (Whatley and Watson, 1988; Babinot and Degaugue-Michalski, 1996). Apart from bathymetry, the distribution of reefal ostracods seems also related to benthic community type (coral reefs versus seagrass/algal beds) and sediment type (i.e., sandy versus muddy deposits), in addition to local hydrology (i.e., exposure to wave energy) (Weissleader et al., 1989; Whatley and Watson, 1988; Babinot and Degaugue-Michalski, 1996; Tabuki, 1990, 1987). However, most of these works are confined to small geographic areas and based on limited (sub-)fossil materials. An extensive regional-scale survey of reefal ostracods has never been conducted. More importantly, the focus of previous studies mainly revolved around taxonomy, as well as biogeography to a lesser degree, while quantitative assessments of biodiversity are largely lacking (Tabuki, 1987, 1990; Mostafawi et al., 2005). The highest species richness (S=74) was reported for a reef slope environment in Pulau Seribu, Java (Whatley and Watson, 1988), in contrast to much lower values at lagoons (S=27–42) (Babinot and Degaugue-Michalski, 1996; Weissleader et al., 1989) and reef flats (S=34) (Mostafawi et al., 2005).
Reefal ostracods are even less known in other tropical regions outside of the central Indo-Pacific. Along the eastern coast of Africa, where the reef ecosystem is productive and biodiverse, the only studies on ostracod assemblages are perhaps Hartmann (1974) and Jellinek (1993) that document more than 200 species inhabiting the algae facies and reefal facies across the littoral zone in Kenya. Here we present the first large-scale study on reefal ostracods from the Zanzibar Archipelago, Tanzania, a biodiversity hotspot of great conservation interests and vulnerability to increasing anthropogenic impacts (Grimsditch et al., 2009). We investigated the geographical structure of ostracod diversity and composition in relation to environmental habitats among the three major islands of Pemba, Zanzibar, and Mafia. We compared the patterns with those of benthic foraminifera (Thissen and Langer, 2017) to explore complex environmental controls on the two groups of meiobenthos. This study is a major step towards better understanding tropical shallow-marine ostracods in eastern Africa and provides valuable insight into the ostracod–reef association in general.
The Zanzibar Archipelago is located along the eastern coast of Tanzania in the western Indian Ocean (Fig. 1) (Thissen and Langer, 2017). It belongs to the eastern African biogeographic province that stretches from Somalia to the northeastern coast of South Africa (Costello et al., 2017; Obura, 2012). The archipelago is strongly influenced by the warm, westward-flowing South Equatorial Current and the northward-flowing East African Coastal Current (Narayan et al., 2022). The western coastlines are more protected, with generally higher coral coverage, whereas the eastern coastlines are exposed to large physical disturbances and strong wave energy (Thissen and Langer, 2017). Tides there are semi-diurnal, with a maximum range of 4.5 m and a neap tidal range of 0.9 m (Thissen and Langer, 2017; Narayan et al., 2022). The islands possess a great variety of benthic habitats from the littoral to open-water zone, with mangroves, vegetated sand flats, and reef complexes. Reefs are mainly fringing reefs that are situated on the narrow continental shelf (Mafia, Zanzibar) or are separated from the African mainland by the deep Pemba Channel (Pemba) (Thissen and Langer, 2017). Noticeably, the major islands are subject to very different degrees of human exploration, as Zanzibar is densely populated and highly urbanized, while Mafia and Pemba are largely uninhabited (Narayan et al., 2022). Stone Town and Bawe, in particular, are faced with a direct discharge of untreated domestic sewage along the western coast of Zanzibar Island, where moderate levels of reef deterioration have been found with a decrease in diversity and coral cover loss (Bravo et al., 2021; Larsen et al., 2023). Although extensive long-term monitoring is still lacking, previous studies indicate that the Pemba reefs are likely in pristine condition with the highest coverage of live hard corals, while the Zanzibar reefs are often dominated by dead corals intermingled with algae and seagrass habitats (Ussi et al., 2019; Larsen et al., 2023; Grimsditch et al., 2009). No quantitative assessment of reef health has been conducted at Mafia Island, unfortunately, but our field observations suggested moderate to good conditions at our sampling sites.
3.1 Samples
The 26 surface sediment samples were collected from 16 sites during two field campaigns in 2005 at the islands of Zanzibar and Pemba and in 2012 at Mafia Island (Table S1 in the Supplement). Depositional depths of all samples range from 0 to 42 m across the intertidal and subtidal zones. The selected sampling sites cover all major types of benthic habitats, including a nearshore mangrove habitat; coastal sand flats; and fringing, fore, and back reefs (Table 1). Note that the mangrove habitat may be underrepresented in the current study as we have only one such site, however. Samples were collected by scuba diving to scrape along the seabed and fill plastic containers with surface sediments from the top 2 cm in order to avoid the loss of finer particles due to suspension.
Table 1Ostracod assemblage information including raw species richness, number of counted individuals, and abundance per gram sediment in addition to a characterization of benthic habitat in terms of sediment type and algae coverage at each location.
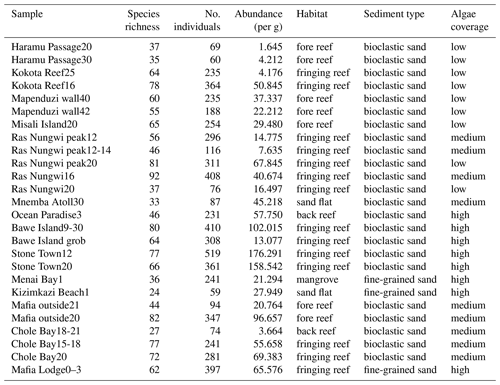
Most sampling sites were fine- to medium-grained carbonate-rich, bioclastic sands and deposits with some reef rubble. Sediments were washed through a 63 µm sieve and oven dried at 50 °C. The residue was dry sieved over a 150 µm mesh sieve, and ostracods were picked from the >150 µm size fraction because smaller individuals are usually early juveniles that are not preserved and/or are difficult to identify (Yasuhara et al., 2017). Large-volume samples were split into aliquot fractions using a microsplitter. The sample materials were primarily death assemblages, although a very small number of specimens were preserved with soft parts (less than 1 % among all observed individuals), indicating they were alive at the time of collection. Both live and dead specimens were included in the total count to represent time-averaged assemblages; this method effectively defines reef habitats and provides general environmental and diversity data useful in paleoecology (Glenn-Sullivan and Evans, 2001; Langer and Lipps, 2003). A single valve or a carapace was considered one individual, which is a standard counting method in ostracod research (Yasuhara et al., 2017). Selected specimens were imaged using a scanning electron microscope (SEM).
3.2 Quantitative analysis
We used Hill numbers (i.e., the effective number of equally abundant species) parameterized by a diversity order q to estimate ostracod diversity in each sample and island (Hill, 1973). Hill numbers have several major advantages over other diversity indices and have been increasingly adopted by ecologists (Chao et al., 2020). For example, the Hill numbers will double when combining two identically distributed but distinct communities, so they obey the “doubling property” and behave like species richness (Chao et al., 2014b). In other words, the unit of Hill numbers is also “species” and thus is more ecologically meaningful than other traditional diversity indices. Also, the order q of the Hill numbers controls the sensitivity of the diversity metric to species relative abundance. When the order q=0, the Hill number (0D) reduces to species richness; when the order q=1, the Hill number (1D) measures the diversity of the abundant species; and when the order q=2, the Hill number (2D) measures the diversity of dominant species (Chao et al., 2014b). Therefore, besides species richness, the Hill numbers also estimate the effective (or hypothetical) number of abundant and dominant species. Coincidentally, the Hill numbers 1D and 2D are equivalent to the exponential of Shannon entropy and Simpson index (hereafter referred to as Shannon and Simpson diversity), respectively (Chao et al., 2014b), making them conceptually easy to understand by ecologists. To make a fair comparison among multiple assemblages, we standardized the Hill numbers with rarefaction or extrapolation to the largest sample completeness possible across samples (82.5 %) and across islands (98.6 %) (Chao et al., 2020). The standard error and 95 % confidence intervals of the Hill numbers were estimated by bootstrap resampling, which was repeated 1000 times. Species evenness, , where qD denotes Hill numbers of order q and S denotes species richness, was quantified using the continuous profiles of Hill numbers as functions of order q (Chao and Ricotta, 2019). A gradual profile suggests a more even community in which the species richness and number of abundant and dominant species are similar. In contrast, a steep profile indicates an uneven community comprised of one or a few dominant species (Mamo et al., 2023).
To distinguish biofacies associated with different benthic habitats, we conducted hierarchical cluster analysis based on Ward's minimum variance and three Hill-number-based dissimilarity indices, including Sørensen (q=0), Horn (q=1), and Morisita–Horn (q=2), to estimate the effective proportion of unshared species in the ostracod assemblages (Chao et al., 2014a). Similarly, the order q controls the sensitivity of the Hill-number-based dissimilarities to species relative abundance. While the classic Sørensen dissimilarity is presence–absence based, the latter two indices are designed to quantify the compositional dissimilarities of abundant and dominant species, respectively. Ward's algorithm is preferred for delineating biofacies because it minimizes the error sum of squares within clusters and generates more balanced clusters. The number of clusters was determined by considering both the structure of the dendrograms and the average silhouette width, with a higher value indicating greater cohesion and separation of clusters. We also performed a non-metric multidimensional scaling (nMDS) to visualize and summarize faunal similarities among ostracod assemblages in two-dimensional space. Stress values were calculated to quantitatively weigh the “goodness of fit” between the original input data matrix and the ultrametric matrix of the resultant nMDS scatter plots (Hong et al., 2022; Kruskal, 1964). We used a compositional heat map to illustrate the relationships between samples by Horn dissimilarities and between species by Hellinger distances.
All analyses were implemented in RStudio. We used the package “iNEXT” to estimate diversity (Chao et al., 2014a; Hsieh et al., 2016) and “vegan” for our multivariate analyses (Oksanen et al., 2020). Figures and maps were constructed using “ggplot2” (Wickham, 2020).
4.1 Diversity
A total of 6262 ostracods were recovered from 26 samples at 16 locations around the Zanzibar Archipelago. They represent remarkably diverse ostracod assemblages comprised of 235 species under 77 genera. An exceedingly high abundance was found at Stone Town, while sites at Bawe Island and Mafia outside were also abundant in contrast to the lowest abundance at Haramu Passage and Chole Bay 1 (Table 1). Considering the alpha diversity of individual samples as measured by the Hill number of different orders q, the spatial diversity patterns were relatively consistent for rare (i.e., species richness, 0D) and abundant (1D) species. The highest values were recorded for fringing reefs at Chole Bay 2 and Ras Nungwi, followed by fringing reefs at Mafia outside and Ras Nungwi peak (Figs. 2a and 3a, b). Moderately high levels of diversity were observed at fore-reef sites at Pemba Island and fringing reefs at Bawe, Stone Town, and Mafia Lodge. In terms of the diversity of dominant (2D) species, there was a more homogenous distribution with similarly high values found at various fringing and fore reefs, including Chole Bay 2, Mafia outside, Haramu Passage, Bawe Island, Ras Nungwi, and Ras Nungwi peak (Figs. 2a and 3c). All remaining localities (Chole Bay 1, Mnemba Atoll, Ocean Paradise, and Kizimkazi Beach) characterized by sand flat and back reef habitats had consistently low diversity across all orders q, especially Menai Bay that was lined with mangrove stands (Figs. 2a and 3). Evenness was highest at Haramu Passage and lowest at Menai Bay for both orders q=1 and q=2 (Figs. 2b and S1 in the Supplement). With respect to the gamma diversity of each island, Mafia and Zanzibar were almost equally diverse across all orders q, while Pemba had significantly lower diversity for abundant and dominant species (Figs. 2c and S2 in the Supplement).
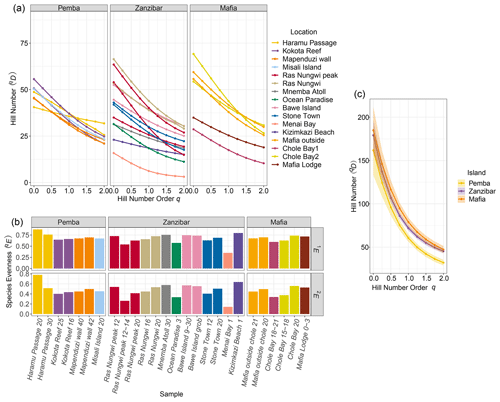
Figure 2Diversity results of the Zanzibar Archipelago ostracods. (a) Alpha diversity of each sample shown by Hill number profile based on 82.5 % sample coverage. The overall elevation of the profile indicates the diversity based on hill number across different orders q. The levelness of the line indicates species evenness of the assemblage because a completely leveled diversity profile would suggest that the numbers of total, common, and dominant species are all the same. (b) Evenness of each sample as the normalized slope of Hill number profile for orders q=1 and q=2 based on 82.5 % sample coverage. (c) Gamma diversity of each island shown by Hill number profile based on 98.6 % sample coverage. The shaded area shows the 95 % confidence interval of the profile.
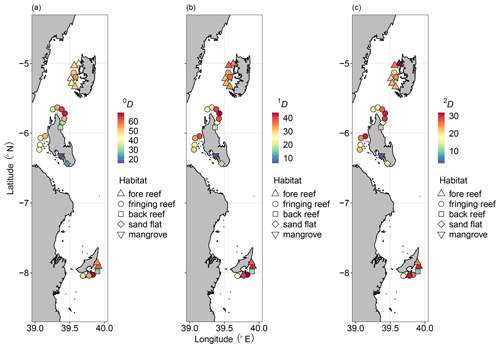
Figure 3Diversity maps of the Zanzibar Archipelago ostracods. Distributions of Hill numbers 0D (a q=0, species richness), 1D (b q=1, exponential Shannon), and 2D (c q=2, inversed Simpson). We used 82.5 % sample coverage to standardize the Hill number estimates. Diversity and habitat are represented by color and shape as shown in the legends, respectively.
4.2 Multivariate analysis
First, cluster analyses based on Sørensen, Horn, and Morisita–Horn dissimilarities delineated biofacies considering faunal composition in terms of species occurrence, relative abundance of abundant species, and relative abundance of dominant species, respectively. The greatest average silhouette width suggested the division of samples into 10 clusters for all three dissimilarity measures; however, it is beyond being interpretable having too many clusters given the size of our dataset. We therefore referred to the structure of the dendrograms based on three dissimilarity measures, determining the optimum number of clusters to be four (Fig. S3 in the Supplement). The nMDS results showed a clear separation of four biofacies based on Horn and Morisita–Horn dissimilarities but not Sørensen dissimilarity, which was calculated with a relatively high stress value (0.26) (Fig. S4 in the Supplement). Ostracod faunas at Pemba Island constituted a distinct group across all levels of faunal composition from presence/absence to relative abundance (Biofacies 1; Fig. 4). Ras Nungwi, Ras Nungwi peak, and nearby Mnemba Atoll were congregated with different sites around Zanzibar and Mafia in Biofacies 2, including Mafia outside and Chole Bay 2 in Sørensen; Mafia outside, Chole Bay 1, and Chole Bay 2 in Horn; and Ocean Paradise, Kizimkazi Beach, and Mafia Lodge in Morisita–Horn analyses (Fig. 4). Samples assigned to Biofacies 3 and 4 strongly varied depending on the dissimilarity matrix used, indicating these biofacies have different ecological meaning among the three cluster analyses (Fig. 4). Specifically, they scattered around the entire Zanzibar Island based on Sørensen dissimilarity. Biofacies 4 was distributed along the western coast of Zanzibar, including Stone Town and Bawe, and Biofacies 3 covered the remaining Zanzibar locations (Menai Bay, Ocean Paradise, and Kizimkazi Beach) in addition to Mafia Lodge based on Horn dissimilarity. On the other hand, when Morisita–Horn dissimilarity was applied, Menai Bay was different from all other sites as a distinctive Biofacies 3, while most Mafia sites (Mafia outside, Chole Bay 1, and Chole Bay 2) aggregated in Biofacies 4. Considering the performance of multivariate analyses to reflect and interpret biological patterns, we think that cluster and nMDS results based on Horn dissimilarity most reasonably captured the underlying ecological significance of reefal versus non-reefal facies as determined by benthic community, depth, and possible anthropogenic disturbances (see the Discussion section). We therefore focus on the four biofacies as divided by Horn-based analysis to scrutinize their diversity and compositional structure in relation to a set of environmental variables.
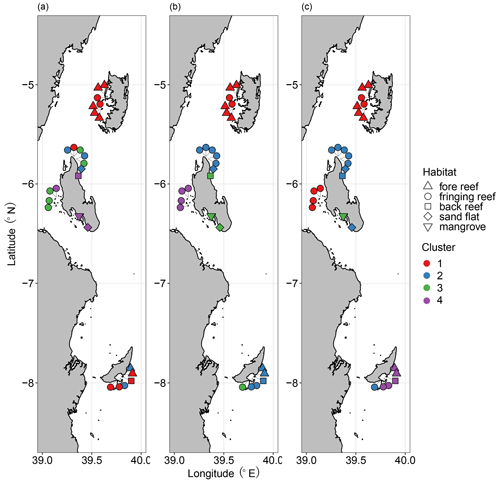
Figure 4Distribution of ostracod Biofacies 1–4 based on (a) Sørensen, (b) Horn, and (c) Morisita–Horn dissimilarities and Ward's minimum variance cluster analysis. Note that the color schemes are independent among panels; thus, the biofacies based on different dissimilarities are unrelated. Cluster and habitat are represented by color and shape as shown in the legends, respectively.
Each biofacies based on Horn dissimilarity index was demonstrated with the top 10 species of highest mean relative abundance as shown in Table 2 and Fig. 5. Noticeably, the Pemba fauna in Biofacies 1 was dominated by genera Neonesidea (N. cf. crepidula and N. schulzi) and Bosasella (B. profunda and B. elongate), together with Paracytheridea tschoppi (Fig. 6; Table 2). Biofacies 2 included the most diverse sites in Zanzibar and Mafia, which all shared similar faunal structures with a high abundance of Loxocorniculum sp. 2, Xestoleberis rotunda, Paracytheridea albatros, and Loxoconcha sp. 3. Biofacies 3 composed of low-diversity sites in Zanzibar and Mafia was distinguished by highly abundant Perissocytheridea estuaria, Xestoleberis hanaii, and three Loxoconcha species (L. sp. 3, L. ghardaqensis, and L. lilljeborgii). Finally, the faunal structure of Biofacies 4 in western Zanzibar showed some similarities to that of Biofacies 1 in Pemba with many common species; however, they clearly differed by the dominance of Xestoleberis hanaii and Patrizia nucleuspersici in Biofacies 4.
Table 2List of the top 10 species of the highest mean relative abundance for Biofacies 1–4 based on Horn dissimilarity.
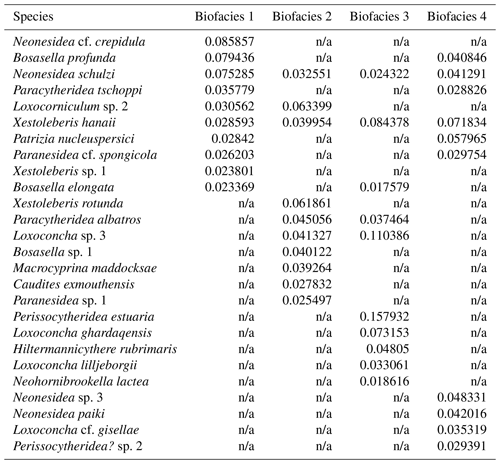
n/a: not applicable.
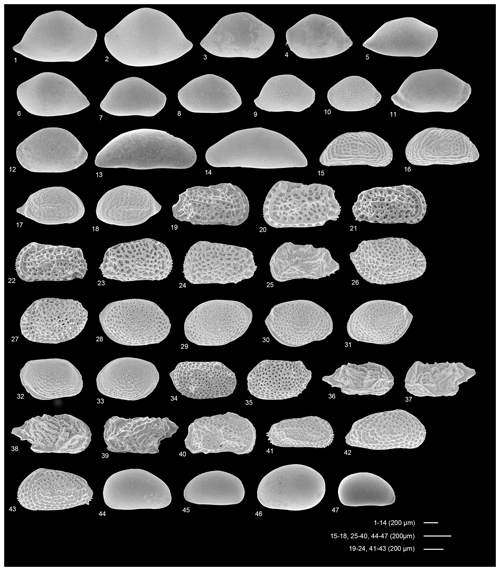
Figure 5Scanning electron microscopy images of the top 10 ostracod species of the highest mean relative abundance for Biofacies 1–4 based on Horn dissimilarity. 1, Neonesidea cf. crepidula, RV (right valve), Kokota Reef25; 2, Neonesidea cf. crepidula, LV (left valve), Haramu Passage30; 3, Neonesidea paiki, RV, Bawe Island9-30; 4, Neonesidea paiki, LV, Kokota Reef25; 5, Neonesidea schulzi, RV, Mapenduzi wall42; 6, Neonesidea schulzi, LV, Kokota Reef25; 7, Neonesidea sp. 3, RV, Bawe Island9-30; 8, Neonesidea sp. 3, LV, Bawe Island9-30; 9, Paranesidea cf. spongicola, RV, Bawe Island9-30; 10, Paranesidea cf. spongicola, LV, Kokota Reef25; 11, Paranesidea sp. 1, RV, Chole Bay18-21; 12, Paranesidea sp. 1, LV, Chole Bay18-21; 13, Macrocyprina maddocksae, RV, Haramu Passage20; 14, Macrocyprina maddocksae, LV, Kokota Reef16; 15, Perissocytheridea estuaria, RV, Menai Bay1; 16, Perissocytheridea estuaria, LV, Menai Bay1; 17, Perissocytheridea? sp. 2, RV, Bawe Island grob; 18, Perissocytheridea? sp. 2, LV, Bawe Island9-30; 19, Bosasella elongate, RV, Haramu Passage30; 20, Bosasella elongate, LV, Mapenduzi wall42; 21, Bosasella profunda, RV, Haramu Passage20; 22, Bosasella profunda, LV, Mapenduzi wall42; 23, Bosasella sp. 1, RV, Kokota Reef25; 24, Bosasella sp. 1, LV, Kokota Reef16; 25, Caudites exmouthensis, LV, Ras Nungwi16; 26, Loxoconcha ghardaqensis, RV, Mnemba Atoll30; 27, Loxoconcha ghardaqensis, LV, Ras Nungwi peak12; 28, Loxoconcha cf. gisellae, RV, Bawe Island9-30; 29, Loxoconcha cf. gisellae, LV, Bawe Island9-30; 30, Loxoconcha lilljeborgii, RV, Bawe Island9-30; 31, Loxoconcha lilljeborgii, LV, Bawe Island grob; 32, Loxoconcha sp. 3, RV, Stone Town20; 33, Loxoconcha sp. 3, LV, Stone Town20; 34, Loxocorniculum sp. 2, RV, Haramu Passage30; 35, Loxocorniculum sp. 2, LV, Kokota Reef16; 36, Paracytheridea albatross, RV, Kokota Reef25; 37, Paracytheridea albatross, LV, Kokota Reef16; 38, Paracytheridea tschoppi, RV, Kokota Reef25; 39, Paracytheridea tschoppi, LV, Mapenduzi wall42; 40, Neohornibrookella lactea, RV, Misali Island20; 41, Hiltermannicythere rubrimaris, RV, Stone Town20; 42, Patrizia nucleuspersici, RV, Stone Town20; 43, Patrizia nucleuspersici, LV, Stone Town12; 44, Xestoleberis hanaii, RV, Bawe Island9-30; 45, Xestoleberis hanaii, LV, Kokota Reef25; 46, Xestoleberis rotunda, LV, Ras Nungwi peak12; 47, Xestoleberis sp. 1, RV, Mapenduzi wall42. All adults and lateral views.
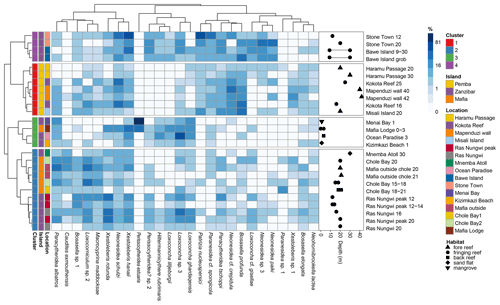
Figure 6Dendrograms based on Horn dissimilarity between samples and Hellinger distances between the top 10 species of highest mean relative abundance in each cluster. The blue heatmap indicates the relative (%) abundance of each species in each sample. The side panel shows water depth and habitat type of each sample (note that several samples are shown by their corresponding depth ranges).
Through Hill number profile and multivariate analyses, we quantified a highly diverse ostracod fauna in the Zanzibar Archipelago composed of four distinct biofacies. The delineation of biofacies varied considerably depending on the dissimilarity matrix used, indicating inconsistent faunal structures across different levels of species information from occurrence to relative abundance (Fig. 4). In terms of the presence/absence of species (Sørensen dissimilarity), all Pemba sites united in Biofacies 1, but the assignment of Zanzibar and Mafia sites into Biofacies 1–4 seemingly conformed to a noisy pattern (Fig. 4a). Accordingly, four biofacies intersected with each other in nMDS space with a relatively high stress value (Fig. S4a). A possible explanation is that the occurrence of individual species may be homogenous among sites in similar environmental conditions within a finite geographic region. Many species are likely to be ubiquitous across the entire neritic zone despite showing certain ecological preferences, and the redeposition processes may further facilitate the mixing of death assemblages to blur the spatial signal at a local scale (Frenzel and Boomer, 2005). Consequently, species presence in all available habitats may translate to considerable faunal similarities among biofacies as measured by the Sørensen index. When considering the composition of abundant species (Horn dissimilarity) (Fig. 4b), the identification of four biofacies instead reflected significant changes in ostracod assemblages along two important environmental gradients, which are benthic community type and water depth. Specifically, Biofacies 1 and 2 characterize typical fore reefs in the deep subtidal zone (sampling depth 16–42 m) and fringing reefs in the shallow subtidal zone (12–30 m), respectively (Fig. 6). Biofacies 3 indicates intertidal habitats with plant cover (0–3 m), and finally Biofacies 4 features degraded fringing reefs in the shallow subtidal zone (9–30 m) (see discussion below).
We summarized the ecological preferences of dominant genera in each biofacies based on Horn dissimilarity (Table 3 and Fig. 6) and investigated how key environmental factors (benthic community type, water depth, and anthropogenic disturbance) may control the distribution and diversity of reefal ostracod assemblages. First of all, Neonesidea and Paranesidea (family Bairdiidae) are typical reefal genera that reach their maximum diversity and incidence on reefs and reef-associated habitats in tropical shallow-marine environments (Whatley and Watson, 1988; Maddocks, 2013; Titterton and Whatley, 1988). Their dominance in Biofacies 1 is consistent with our background understanding that the Pemba reefs were pristine and healthy (Ussi et al., 2019; Grimsditch et al., 2009). However, it should be noted that individual species of these genera likely have different environmental tolerance. For example, N. cf. crepidula were restricted to Biofacies 1, while N. schulzi were widespread among four biofacies inhabiting both reef and algae habitats (Fig. 6) (Mostafawi et al., 2005). Bosasella as another prominent component of Biofacies 1 is also known to occur on coral reefs in the western Indian Ocean (Munef et al., 2012; Jellinek, 1993). Paracytheridea and Caudites on the other hand are loosely categorized as reefal genera, as their dominance on coral reefs was reported but not studied in detail (Whatley and Watson, 1988; Keyser and Mohammed, 2021). In this study, they were common on fore and fringing reefs in Biofacies 1 and 2 (Fig. 6). Loxoconcha and Loxocorniculum (family Loxoconchidae) as two phylogenetically related and ecologically similar genera exhibited ubiquitous distribution around the Zanzibar Archipelago with the highest relative abundance in Biofacies 3 followed by Biofacies 2. As generalists, they thrive on a wide variety of benthic habitats across the neritic zone and show affinities towards plant substrates (algae and seagrass beds) in particular (Munef et al., 2012; Keyser and Mohammed, 2021; Kamiya, 1988). The ecology of Xestoleberis is very similar to that of Loxoconchidae, living both on coral reefs and algal flats (Keyser and Mohammed, 2021; Munef et al., 2012; Whatley and Watson, 1988; Kamiya, 1988). This genus was almost equally weighted in all biofacies, although individual species clearly preferred different environments, as X. hanaii prevailed in Biofacies 3 and 4, whereas X. rotunda only did in Biofacies 2 (Fig. 6). Patrizia is documented as a reefal genus in the lower littoral zone along the eastern coast of tropical Africa (Jellinek, 1993). It dominated the relatively deep fringing-reef faunas of Biofacies 4, which were subject to sewage-derived nutrient and trace metal pollution from Zanzibar Town (Narayan et al., 2022; Bravo et al., 2021). Different from all the above-discussed genera, Hiltermannicythere and Perissocytheridea are restricted to shallow intertidal environments as phytal and sediment-dwelling taxa, respectively (Jellinek, 1993), which explains their abundance in our Biofacies 3. Perissocytheridea is especially considered a bioindicator of brackish water facies, adapting to euryhaline conditions (Nogueira and Ramos, 2016; Keyser, 1977). Furthermore, we revealed a more generalized pattern of the compositional differences among biofacies with the top five families of highest mean relative abundance in each biofacies (Fig. 7).
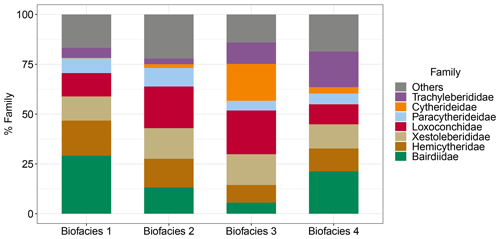
Figure 7Family composition of Biofacies 1–4 based on Horn dissimilarity. The top five families of the highest percentage of relative abundance in each biofacies are shown.
Thus, our study indicates that the distribution of shallow-marine ostracods in the Zanzibar Archipelago is characterized by three reefal facies and one intertidal facies. Yet slight differences in bathymetry, benthic community type, and anthropogenic impacts likely contributed to subtle faunal changes among reefal Biofacies 1, 2, and 4. The fore reefs in Pemba (Biofacies 1) were deepest with a high incidence and diversity of live hard corals (Gavrilets and Losos, 2009; Ussi et al., 2019), which accounted for the definite dominance of ostracod reefal taxa (Bairdiidae and Bosasella) over algal taxa (Loxoconchidae and Xestoleberididae) (Figs. 6 and 7). Moderately high levels of diversity in terms of rare, abundant, and dominant species were observed for these ostracod assemblages (Fig. 3). The Pemba reefs are thereby considered the most mature and authentic reef ecosystem, serving as a natural reference for comparing with other sites. The fringing-reef fauna of western Zanzibar (Stone Town and Bawe, Biofacies 4) exhibited certain similarities to the Pemba fauna as indicated by the prevalence of Bosasella profunda, Paracytheridea tschoppi, and Paranesidea cf. spongicola in both facies (Fig. 6). Indeed, they were grouped together based on the composition of dominant species (Morisita–Horn analysis) (Fig. 4c). Faunal similarities between Pemba and Stone Town make sense as they are in comparable baseline conditions of water depths and hydrology along the protected western coast of the Zanzibar Archipelago in contrast to Ras Nungwi and Chole Bay that are exposed to oceanic disturbances from the east (Fig. 1). However, Biofacies 4 was differentiated from Biofacies 1 by the dominance of Patrizia in conjunction with the absence of Neonesidea cf. crepidula. It also had the highest relative abundance of Trachyleberididae genera among all facies (Fig. 7), for example, Adencythere, Strobilocythere, Bradyon, and Actinocythereis, but their ecologies are not well understood. Stressful environmental conditions in terms of overexploitation, tourism, and coastal pollution offer the most possible explanation for such a unique faunal composition and the comparatively low diversity of Biofacies 4 (Fig. 3) (Bravo et al., 2021; Larsen et al., 2023). Consistently, foraminifera and coral surveys indicated early stages of reef degradation there (Narayan et al., 2022; Bravo et al., 2021; Thissen and Langer, 2017). It is possible that ongoing anthropogenic disturbances near Stone Town will eventually exceed the critical threshold levels and cause more pronounced changes in ostracod faunal structures in terms of dominant species through a shift in benthic habitat (Narayan et al., 2022; Hong et al., 2022). Other than Biofacies 1 and 4, Biofacies 2 mostly represented the different type of relatively shallow (12–20 m) fringing-back reef habitats of Ras Nungwi, Chole Bay, and Mafia outside, in addition to the deeper (30 m) sand flat of Mnemba Atoll (Fig. 6). Algal taxa (Loxoconchidae and Xestoleberididae) and reefal taxa (Bairdiidae, Bosasella, Paracytheridea, and Caudites) reached equally high levels of relative abundance there (Figs. 6 and 7). Microhabitats on the reef platforms of Biofacies 2 are believed to be diverse and heterogenous with interlaced live and dead corals, algae and seagrass, calcareous sands, and bare substrate rock (Ussi et al., 2019; Larsen et al., 2023), which facilitated the coexistence of reefal and algal ostracods and consequently the highest diversity of local assemblages (Fig. 3). The remainder of Biofacies 3 corresponded to the shallowest intertidal habitats with various benthic communities, including a marginal back reef, a marginal fringing reef, a sand flat, and a mangrove habitat (Fig. 6). Typical reefal taxa (Bairdiidae and Bosasella) dropped to their lowest relative abundance in this facies, replaced by large numbers of Loxoconchidae, Perissocytheridea, and Hiltermannicythere that are well-adapted to shallow euryhaline conditions (Figs. 6 and 7). Not surprisingly, the diversity of Biofacies 3 was much lower than that of open-ocean reefal facies, as drastic changes in temperature, salinity, dissolved oxygen, and wave energy in the intertidal zone may be too challenging for many marine taxa (Fig. 3) (Morley and Hayward, 2007; Frenzel and Boomer, 2005). The mangrove habitat at Menai Bay was unique concerning the absolute dominance of Perissocytheridea in line with its lowest diversity and evenness (Figs. 2b and 3). It indeed constituted an independent biofacies based on Morisita–Horn analysis (Fig. 4c).
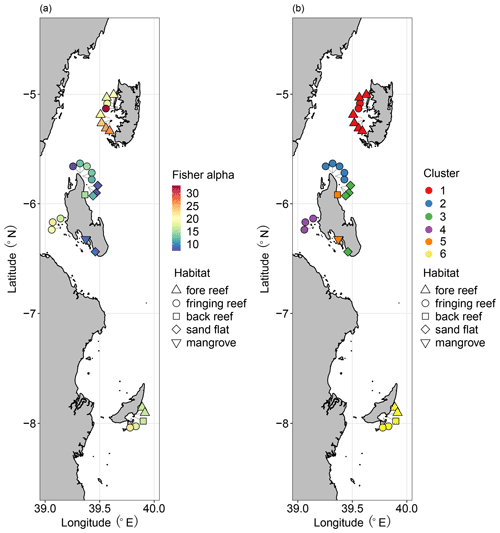
Figure 8Distributions of benthic foraminifera: (a) diversity measured as Fisher alpha index; (b) cluster groups based on Q-mode cluster analysis. Modified from Thissen and Langer (2017). Diversity/cluster and habitat are represented by color and shape as shown in the legends, respectively.
The division scheme of four biofacies based on Horn dissimilarity explicitly revealed spatial patterns of ostracod distribution in the aspects of diversity and composition, as discussed above. Our results are generally concordant with a previous study on benthic foraminifera, which separated six clusters of Pemba, Stone Town, Mafia Bay, Ras Nungwi, Mnemba Atoll, and Menai Bay (Fig. 8b) (Thissen and Langer, 2017). Each of these foraminifera clusters corresponded to major habitat types, as argued by the authors (Thissen and Langer, 2017), and we accordingly point out the consistent role of habitat factors in shaping the biogeography of both ostracod and foraminifera biota. However, the diversity patterns of these two groups were apparently different among reefal habitats (Figs. 3 and 8a). High, moderate, and low levels of diversity were recorded on fore reefs (Pemba), fringing reefs (Mafia and Zanzibar), and intertidal zones (Zanzibar) for foraminifera in contrast to fringing reefs (Mafia and Zanzibar), fore reefs (Pemba), and intertidal zones (Zanzibar) for ostracods, respectively. Such discrepancies may imply a tight association of foraminifera with the reef ecosystem and their ultra-sensitivity to reef health, since their diversity generally decreased from pristine, mature reefs to degraded, marginal reefs. Ostracods on the other hand may be less confined or specific to reef habitats. The occupation of coral and algae substrate by distinct faunal groups allows them to thrive in the transitional zone between marginal and true reefs. Another important factor accounting for the different distributional patterns between ostracods and foraminifera is likely their tolerance to eutrophication and pollution. Previous studies indicate that an intermediate level of eutrophication is beneficial to ostracods and many other soft-sediment benthos, which are also resistant to heavy metal pollution in highly urbanized areas (Hong et al., 2022). Consistently, our sampling sites at Stone Town reported the highest abundance of ostracods (Table 1). Foraminifera on the other hand are susceptible to environmental stressors, as shown by low taxonomic richness and high dominance of the faunas in eutrophic conditions (Mamo et al., 2023). In our case, it makes sense that the highest diversity of foraminifera was found in pristine and oligotrophic Pemba waters.
Most importantly, this study established a clear benthic community axis along which the composition and diversity of ostracod assemblages vary, i.e., from coral reefs to algae turfs. We identified typical reefal association (Bairdiidae–Bosasella) versus algal association (Loxoconchidae–Xestoleberididae) (Fig. 7), and their relative dominance may be used as a direct indication of benthic community type. As there is a growing interest to monitor the degradation of reef ecosystems from the coral-dominated phase to the algae-dominated phase (Roth et al., 2018; Knowlton and Jackson, 2008; Knowlton, 2012), our finding is of potential conservation value. Ostracod species diversity was higher on shallow fringing reefs than on deep fore reefs, as the former ecosystem harbored evenly weighted reefal and algal taxa within a dynamic mosaic of microhabitats. Our results thus strongly indicate the importance of coral reefs in harboring conspicuously high levels of meiobenthic biodiversity, likely through finer niche partitioning (Kohn et al., 1997; Fox and Bellwood, 2013). Along with the benthic community factor, we quantified prominent changes in faunal structure and diversity along a depth gradient, as the intertidal euryhaline assemblages transited to subtidal fully marine assemblages. It is widely recognized that shallow-marine biota are especially susceptible to depth-associated changes, such as temperature, salinity, wave action, and light penetration (Carvalho et al., 2012; Tian et al., 2022). This study showed that a narrow depth zone across the intertidal and subtidal zones (∼40 m) was further divided and occupied by distinct biofacies. Such a finely tuned vertical gradient of diversity and faunal composition added to an exceedingly large regional species pool (235 species) in this tropical shallow-marine setting. Last but not least, it should be noted that the effects of depth and benthic community type are often intertwined with each other in determining ostracod assemblages, as the habitat-building corals and algae essentially exhibit depth distributions. At a regional scale like the Zanzibar Archipelago, the combined effects of water depth and benthic community characteristics should be considered in studying the spatial patterns of benthic organisms.
In conclusion, this study showed that the diversity and faunal composition of reefal ostracod assemblages vary along benthic community and bathymetric gradients, which may also be altered by local anthropogenic disturbances. Ostracod faunas on shallow fringing reefs were especially diverse, which may be explained by high levels of habitat complexity and heterogeneity. The relative dominance of reefal taxa (Bairdiidae) versus algal taxa (Loxoconchidae–Xestoleberididae) is likely determined by the proportion of coral versus algae cover on the reef platforms, though more extensive studies beyond this region are needed to confirm the universality of this pattern. Coral reefs worldwide are vulnerable to ongoing climate changes and other human impacts at local to global scales, and many reefal species are at risk of extinction. It is of great importance that we inspect and understand the immense biodiversity of meiobenthos on coral reefs as an indispensable part of the ecosystem.
Ostracod census data are available in the Supplement.
The supplement related to this article is available online at: https://doi.org/10.5194/bg-21-3523-2024-supplement.
Each named author has participated sufficiently in the work to take public responsibility for the content. SYT and ML developed the concept. ML collected the samples. SYT performed the research and collected the data. SYT and CLW analyzed the data. SYT drafted the manuscript. ML, MY, and CLW reviewed and edited the manuscript.
The contact author has declared that none of the authors has any competing interests.
Publisher's note: Copernicus Publications remains neutral with regard to jurisdictional claims made in the text, published maps, institutional affiliations, or any other geographical representation in this paper. While Copernicus Publications makes every effort to include appropriate place names, the final responsibility lies with the authors.
We thank Stephanie Pietsch, Jens Thissen, Anna Weinmann, and Michael Kunert for their help with fieldwork and Jingfang He for her help in the lab.
This research has been supported by the Alexander von Humboldt-Stiftung (Humboldt Research Fellowship); the Deutsche Forschungsgemeinschaft (grant no. LA 884/10-1); the Research Grants Council, University Grants Committee (grant nos. HKU 17306023 and G-HKU709/21); and the National Science and Technology Council (grant no. NSTC 112-2611-M-002-011).
This open-access publication was funded by the University of Bonn.
This paper was edited by Helge Niemann and reviewed by Peter Frenzel, Ilaria Mazzini, and Andreas Haas.
Babinot, J.-F. and Degaugue-Michalski, F.: Lagoonal to reefal ostracod assemblages from Holocene and Recent deposits, Chesterfield Islands and northern New Caledonia (southwestern Pacific), Micropaleontology, 42, 351–362, https://doi.org/10.2307/1485957, 1996.
Bravo, H., Cannicci, S., Huyghe, F., Leermakers, M., Sheikh, M. A., and Kochzius, M.: Ecological health of coral reefs in Zanzibar, Reg. Stud. Mar. Sci., 48, 102014, https://doi.org/10.1016/j.rsma.2021.102014, 2021.
Carvalho, S., Cunha, M. R., Pereira, F., Pousão-Ferreira, P., Santos, M., and Gaspar, M.: The effect of depth and sediment type on the spatial distribution of shallow soft-bottom amphipods along the southern Portuguese coast, Helgoland Mar. Res., 66, 489–501, https://doi.org/10.1007/s10152-011-0285-9, 2012.
Chao, A. and Ricotta, C.: Quantifying evenness and linking it to diversity, beta diversity, and similarity, Ecology, 100, e02852, https://doi.org/10.1002/ecy.2852, 2019.
Chao, A., Chiu, C.-H., and Jost, L.: Unifying species diversity, phylogenetic diversity, functional diversity, and related similarity and differentiation measures through Hill numbers, Annu. Rev. Ecol. Evol. S., 45, 297–324, https://doi.org/10.1146/annurev-ecolsys-120213-091540, 2014a.
Chao, A., Gotelli, N. J., Hsieh, T., Sander, E. L., Ma, K., Colwell, R. K., and Ellison, A. M.: Rarefaction and extrapolation with Hill numbers: a framework for sampling and estimation in species diversity studies, Ecol. Monogr., 84, 45–67, https://doi.org/10.1890/13-0133.1, 2014b.
Chao, A., Kubota, Y., Zelený, D., Chiu, C. H., Li, C. F., Kusumoto, B., Yasuhara, M., Thorn, S., Wei, C. L., and Costello, M. J.: Quantifying sample completeness and comparing diversities among assemblages, Ecol. Res., 35, 292–314, https://doi.org/10.1111/1440-1703.12102, 2020.
Costello, M. J., Tsai, P., Wong, P. S., Cheung, A. K. L., Basher, Z., and Chaudhary, C.: Marine biogeographic realms and species endemicity, Nat. Commun., 8, 1–10, https://doi.org/10.1038/s41467-017-01121-2, 2017.
Fox, R. and Bellwood, D.: Niche partitioning of feeding microhabitats produces a unique function for herbivorous rabbitfishes (Perciformes, Siganidae) on coral reefs, Coral Reefs, 32, 13–23, https://doi.org/10.1007/s00338-012-0945-5, 2013.
Frenzel, P. and Boomer, I.: The use of ostracods from marginal marine, brackish waters as bioindicators of modern and Quaternary environmental change, Palaeogeogr. Palaeocl., 225, 68–92, https://doi.org/10.1016/j.palaeo.2004.02.051, 2005.
Gavrilets, S. and Losos, J. B.: Adaptive radiation: contrasting theory with data, Science, 323, 732–737, https://doi.org/10.1126/science.1157966, 2009.
Glenn-Sullivan, E. C. and Evans, I.: The effects of time-averaging and taphonomy on the identification of reefal sub-environments using larger foraminifera: Apo Reef, Mindoro, Philippines, Palaios, 16, 399–408, https://doi.org/10.1669/0883-1351(2001)016%3C0399:TEOTAA%3E2.0.CO;2, 2001.
Grimsditch, G. D., Tamelander, J., Mwaura, J., Zavagli, M., Takata, Y., and Gomez, T.: Coral reef resilience assessment of the Pemba channel conservation area, Tanzania, IUCN, ISBN: 978-2-8317-1181-2, 2009.
Hartmann, G.: Zur Kenntnis des Eulitorals der afrikanischen Westküste zwischen Angola und Kap der Guten Hoffnung und der afrikanischen Ostküste von Südafrika und Mocambique unter besonderer Berücksichtigung der Polychaeten und Ostracoden, Mitteil. Hamburg. Zoolog. Mus. Inst., 69, 229–521, 1974.
Hill, M. O.: Diversity and evenness: a unifying notation and its consequences, Ecology, 54, 427–432, https://doi.org/10.2307/1934352, 1973.
Hong, Y., Yasuhara, M., Iwatani, H., Harnik, P. G., Chao, A., Cybulski, J. D., Liu, Y., Ruan, Y., Li, X., and Wei, C.-L.: Benthic ostracod diversity and biogeography in an urbanized seascape, Mar. Micropaleontol., 174, 102067, https://doi.org/10.1016/j.marmicro.2021.102067, 2022.
Hsieh, T., Ma, K., and Chao, A.: iNEXT: an R package for rarefaction and extrapolation of species diversity (Hill numbers), Methods Ecol. Evol., 7, 1451–1456, https://doi.org/10.1111/2041-210X.12613, 2016.
Jellinek, T.: Zur Ökologie und Systematik rezenter Ostracoden aus dem Bereich des kenianischen Barriere-riffs, Senckenb. Lethaea, 73, 83–335, 1993.
Kamiya, T.: Morphological and ethological adaptations of Ostracoda to microhabitats in Zostera beds, Dev. Palaeontol. Stratigr., 11, 303–318, https://doi.org/10.1016/S0920-5446(08)70191-2, 1988.
Keyser, D.: Ecology and zoogeography of recent brackish-water ostracoda (Crustacea) from south-west Florida, in: Aspects of ecology and zoogeography of recent and fossil Ostracoda, W. Junk, The Hague, 207–222, ISBN: 9061935814, 1977.
Keyser, D. and Mohammed, M.: Taxonomy of recent shallow marine Ostracods from Al-Hudeida City-Yemen, Mar. Micropaleontol., 164, 101974, https://doi.org/10.1016/j.marmicro.2021.101974, 2021.
Knowlton, N.: Iconic coral reef degraded despite substantial protection, P. Natl. Acad. Sci. USA, 109, 17734–17735, https://doi.org/10.1073/pnas.1215836109, 2012.
Knowlton, N. and Jackson, J. B. C.: Shifting baselines, local impacts, and global change on coral reefs, PLoS Biol., 6, e54, https://doi.org/10.1371/journal.pbio.0060054, 2008.
Kohn, A. J., Ormond, R., Gage, J., and Angel, M.: Why are coral reef communities so diverse, in: Marine biodiversity: Patterns and processes, Cambridge University Press, 201–215, ISBN: 0521552222, 1997.
Kruskal, J. B.: Multidimensional scaling by optimizing goodness of fit to a nonmetric hypothesis, Psychometrika, 29, 1–27, https://doi.org/10.1007/BF02289565, 1964.
Langer, M. and Lipps, J.: Foraminiferal distribution and diversity, Madang reef and lagoon, Papua New Guinea, Coral Reefs, 22, 143–154, https://doi.org/10.1007/s00338-003-0298-1, 2003.
Larsen, J., Maar, M., Rasmussen, M. L., Hansen, L. B., Hamad, I. Y., and Stæhr, P. A. U.: High-resolution hydrodynamics of coral reefs and tracing of pollutants from hotel areas along the west coast of Unguja Island, Zanzibar, Mar. Pollut. Bull., 191, 114968, https://doi.org/10.1016/j.marpolbul.2023.114968, 2023.
Leray, M. and Knowlton, N.: DNA barcoding and metabarcoding of standardized samples reveal patterns of marine benthic diversity, P. Natl. Acad. Sci. USA, 112, 2076–2081, https://doi.org/10.1073/pnas.1424997112, 2015.
Maddocks, R. F.: Revision of Recent Bairdiidae (Ostracoda), in: Bulletin of the United States National Museum, United States National Museum, https://doi.org/10.5479/si.03629236.295.1, 1969.
Maddocks, R. F.: New and poorly known species of Neonesidea (Bairdiidae, Ostracoda, Crustacea) from French Frigate Shoals, the Hawaiian Islands, Zootaxa, 3608, 457–510, https://doi.org/10.11646/zootaxa.3608.6.3, 2013.
Mamo, B. L., Cybulski, J. D., Hong, Y., Harnik, P. G., Chao, A., Tsujimoto, A., Wei, C.-L., Baker, D. M., and Yasuhara, M.: Modern biogeography of benthic foraminifera in an urbanized tropical marine ecosystem, Geological Society, London, Special Publications, 529, SP529-2022-2175, https://doi.org/10.1144/SP529-2022-175, 2023.
Morley, M. S. and Hayward, B. W.: Intertidal and shallow-water ostracoda of the Waitemata Harbour, New Zealand, Records of the Auckland Museum, 17–32, https://www.jstor.org/stable/42905892 (last access: 31 July 2024), 2007.
Mostafawi, N., Colin, J.-P., and Babinot, J.-F.: An account on the taxonomy of ostracodes from recent reefal flat deposits in Bali, Indonesia, Revue de Micropaleontologie, 48, 123–140, https://doi.org/10.1016/j.revmic.2004.12.001, 2005.
Munef, M. A., Al-Wosabi, M. A., Keyser, D., and Al-Kadasi, W. M.: Distribution and taxonomy of shallow marine Ostracods from northern Socotra Island (Indian Ocean)-Yemen, Revue de Micropaléontologie, 55, 149–170, https://doi.org/10.1016/j.revmic.2012.06.004, 2012.
Narayan, G. R., Herrán, N., Reymond, C. E., Shaghude, Y. W., and Westphal, H.: Local Persistence of Large Benthic Foraminifera (LBF) under Increasing Urban Development: A Case Study from Zanzibar (Unguja), East Africa, J. Earth Sci., 33, 1434–1450, https://doi.org/10.1007/s12583-022-1702-5, 2022.
Nogueira, A. A. E. and Ramos, M. I. F.: The genus Perissocytheridea Stephenson, 1938 (Crustacea: Ostracoda) and evidence of brackish water facies along the Oligo-Miocene, Pirabas Formation, eastern Amazonia, Brazil, J. S. Am. Earth Sci., 65, 101–121, https://doi.org/10.1016/j.jsames.2015.11.007, 2016.
Obura, D.: The diversity and biogeography of Western Indian Ocean reef-building corals, PLoS one, 7, e45013, https://doi.org/10.1371/journal.pone.0045013, 2012.
Oksanen, J., Blanchet, F., Friendly, M., Kindt, R., Legendre, P., McGlinn, D., Minchin, P., O'Hara, R., Simpson, G., and Solymos, P.: Vegan community ecology package version 2.5 – 7 November 2020, R Project for Statistical Computing [code], Vienna, Austria, https://doi.org/10.32614/CRAN.package.vegan, 2020.
Plaisance, L., Caley, M. J., Brainard, R. E., and Knowlton, N.: The diversity of coral reefs: what are we missing?, PloS one, 6, e25026, https://doi.org/10.1371/journal.pone.0025026, 2011.
Roth, F., Saalmann, F., Thomson, T., Coker, D. J., Villalobos, R., Jones, B., Wild, C., and Carvalho, S.: Coral reef degradation affects the potential for reef recovery after disturbance, Mar. Environ. Res., 142, 48–58, https://doi.org/10.1016/j.marenvres.2018.09.022, 2018.
Souza, M. C. S., Massei, K., Vianna, P. C. G., Santos, C. A. G., Mishra, M., and da Silva, R. M.: Assessment of macrobenthos diversity and a zoning proposal for Seixas coral reefs (northeastern Brazil), Mar. Pollut. Bull., 195, 115443, https://doi.org/10.1016/j.marpolbul.2023.115443, 2023.
Tabuki, R.: Preliminary report on ostracode fauna from Sekisei-sho area, Yaeyama Islands, Bulletin of College of Education, 31, 323–335, https://cir.nii.ac.jp/crid/1572824499572084608, 1987.
Tabuki, R.: The Ostracoda of the Sekisei-sho area, Ryukyu Islands, Japan: a preliminary report on the ostracods from coral reefs in the Ryukyu Islands, Ostracoda and Global Events, 365, 365–373, https://cir.nii.ac.jp/crid/1573668924502213632, 1990.
Thissen, J. and Langer, M.: Spatial Patterns and Structural Composition of Foraminiferal Assemblages from the Zanzibar Archipelago (Tanzania), Palaeontogr. Abt. A, 308, 1–67, https://doi.org/10.1127/pala/308/2017/1, 2017.
Tian, S. Y., Yasuhara, M., Robinson, M. M., and Huang, H.-H. M.: Ostracod eye size: A taxonomy-free indicator of the Paleocene-Eocene Thermal Maximum sea level, Mar. Micropaleontol., 174, 101994, https://doi.org/10.1016/j.marmicro.2021.101994, 2022.
Titterton, R. and Whatley, R.: Recent Bairdiinae (Crustacea, Ostracoda) from the Solomon Islands, J. Micropalaeontol., 7, 111–142, https://doi.org/10.1144/jm.7.2.111, 1988.
Ussi, A., Mohammed, M., Muhando, C., and Yahya, S.: Ecological impact of thermal stress in reefs of Zanzibar following the 2016 elevated higher sea surface temperatures, in: Climate Change and Coastal Resources in Tanzania, Springer Climate, Springer Cham, 93–115, https://doi.org/10.1007/978-3-030-04897-6, 2019.
Weissleader, L. S., Gilinsky, N. L., Ross, R. M., and Cronin, T. M.: Biogeography of marine podocopid ostracodes in Micronesia, J. Biogeogr., 16, 103–114, https://doi.org/10.2307/2845084, 1989.
Whatley, R. C. and Watson, K.: A preliminary account of the distribution of Ostracoda in recent reef and reef associated environments in the Pulau Seribu or Thousand Island Group, Java Sea, Dev. Palaeontol. Stratigr., 11, 399–411, https://doi.org/10.1016/S0920-5446(08)70197-3, 1988.
Wickham, H.: reshape2: flexibly reshape data: a reboot of the reshape package, R package version [code], 1, https://doi.org/10.32614/CRAN.package.reshape2, 2020.
Yasuhara, M., Tittensor, D. P., Hillebrand, H., and Worm, B.: Combining marine macroecology and palaeoecology in understanding biodiversity: microfossils as a model, Biol. Rev., 92, 199–215, https://doi.org/10.1111/brv.12223, 2017.