the Creative Commons Attribution 4.0 License.
the Creative Commons Attribution 4.0 License.
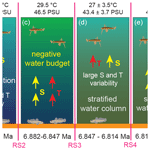
Coupled otolith and foraminifera oxygen and carbon stable isotopes evidence paleoceanographic changes and fish metabolic responses
Konstantina Agiadi
Iuliana Vasiliev
Geanina Butiseacă
George Kontakiotis
Danae Thivaiou
Evangelia Besiou
Stergios Zarkogiannis
Efterpi Koskeridou
Assimina Antonarakou
Andreas Mulch
Capturing the mechanisms leading to the local extirpation of a species in deep time is a challenge. Here, by combining stable oxygen and carbon isotopic analyses on benthic and planktonic foraminifera and the otoliths of pelagic and benthic fish species, we reveal the paleoceanographic regime shifts that took place in the eastern Mediterranean from 7.2 to 6.5 Ma, in the precursor phase to the Messinian salinity crisis, and discuss the fish response to these events. The stepwise restriction of the Mediterranean–Atlantic gateway impacted the metabolism of fishes in the Mediterranean, particularly those dwelling in the lower, deeper part of the water column. An important shift in the Mediterranean paleoceanographic conditions took place between 6.951 and 6.882 Ma, from predominantly temperature to salinity control, which was probably related to stratification of the water column. A regime shift at 6.814 Ma, due to changes in the influx, source and/or preservation of organic matter, led to pelagic–benthic decoupling of the fish fauna. At that time, the oxygen isotopic composition of benthic fish otoliths reflects higher salinity in the lower part of the water column that is accompanied by a rapid fluctuation in the carbon isotopic composition (a proxy for the metabolic rate), ultimately leading to the local extirpation of the benthic species. Overall, our results confirm that otolith stable oxygen and carbon isotope ratios are reliable proxies for paleoceanographic studies and, when combined with those of foraminifera, can reveal life history changes and migration patterns of teleost fishes in deep time.
- Article
(3832 KB) - Full-text XML
- BibTeX
- EndNote
The modern trajectory of climate change makes the need for an improved understanding of the long-term resilience of marine fishes and their populations urgent. Over time, in the face of environmental change, the response of organisms shifts from phenotypic plasticity to physiological changes, evolutionary adaptation or extinction (Donelson et al., 2019). To this end, deep-time studies can contribute greatly, because they provide the necessary baselines, potential past analogues, and long time series that cover the onset and recovery from such events (Dietl et al., 2015; Leonhard and Agiadi, 2023). The paleoceanographic changes in the Mediterranean during the Messinian (7.25–5.33 Ma) and their impact on higher organisms can offer unique insights into the resilience of marine ecosystems under extreme environmental conditions. Late Miocene cooling (Herbert et al., 2016) superimposed on the gradual restriction of the marine gateway with the Atlantic starting at the Tortonian–Messinian boundary (Krijgsman et al., 1999; Flecker et al., 2015) led to profound environmental changes in the Mediterranean, culminating in the Messinian salinity crisis (MSC; 5.97–5.33 Ma; Hsü et al., 1973). Basin's restriction resulted in high-amplitude shifts in sea surface temperature (SST) and sea surface salinity (SSS) as well as episodic water-column stratification and dysoxia on the sea bottom, even before the MSC (e.g., Moissette et al., 2018; Vasiliev et al., 2019; Sabino et al., 2020; Bulian et al., 2021; Zachariasse et al., 2021; Kontakiotis et al., 2022; Mancini et al., 2024). However, the impact of these events on higher organisms, such as fishes, remains unclear. In the early Messinian, the presence of new endemics (Girone et al., 2010) and Paratethyan-affinity fish species (Schwarzhans et al., 2020) in the Mediterranean has been attributed to the changing paleoceanographic conditions. This study presents a new approach on how climate affected marine life in the geological past, based on oxygen (δ18O) and carbon (δ13C) isotopic analyses of otoliths, aiming to (1) test the hypothesis that the pre-MSC amplitude of changes in important paleoceanographic parameters (SST, SSS, organic matter source and primary productivity) had a negative impact on the physiology of marine fishes and (2) identify the principal parameter controlling fish populations.
Otoliths are accretionary, mostly aragonitic structures in the inner ear of teleost fishes, and they are metabolically inert, making them excellent paleoceanographic archives (Campana, 1999). The oxygen isotope ratio in otoliths (δ18Ooto) is a function of temperature and the δ18O of ambient water, the latter of which depends on salinity: δ18Ooto is not affected by somatic growth or the otolith precipitation rate, but it varies with species-specific lifestyle patterns (Kalish, 1991). The carbon isotopic composition of fish otoliths (δ13Coto) reflects the δ13C values of the fish diet and the dissolved inorganic carbon (DIC) in ambient water (Kalish, 1991; Solomon et al., 2006; Trueman et al., 2016; Chung et al., 2019a). The δ13Coto is a proxy for the fish metabolic rate (Wurster and Patterson, 2003; Solomon et al., 2006; Trueman et al., 2016; Chung et al., 2019a, b; Martino et al., 2020; Smoliński et al., 2021; Trueman et al., 2023; Jones et al., 2023), reflecting the amount of energy that the fish uses to live and grow, which impacts its behavior and resilience in the face of environmental change (Gauldie, 1996; Chung et al., 2019a). At the evolutionary level, higher metabolic rates in a population are generally expected to lead to higher genetic mutation rates (Trueman et al., 2016). In addition, δ13Coto is linked to oxygen consumption through the metabolic oxidation of dietary carbon (Chung et al., 2019b), while a high metabolic rate results in higher oxygen consumption, higher activity, higher respiration and greater carbon export (Chung et al., 2019a). Respiratory (or metabolic) carbon has a 15 ‰ lower δ13C than DIC because of the preferential incorporation of 12C during photosynthesis (Kroopnick, 1985).
Here, we investigate the link between fish physiology and paleoceanographic change due to the restriction of the marine gateways connecting the Mediterranean Sea with the Atlantic Ocean in the Late Miocene. We first detect regime shifts by analyzing time series of independently obtained proxies. Then, we examine the fish response by comparing δ18Ooto and δ13Coto time series obtained for two very common zooplanktivorous species, the pelagic Bregmaceros albyi and the benthic Lesueurigobius friesii, from the eastern Mediterranean for the interval 7.2–6.5 Ma.
2.1 Material and sampling
A total of 47 sediment samples were collected from the ∼ 25 m thick pre-MSC Messinian laminated (sapropelic) and homogeneous marl succession of Agios Myron (35°23′35.90′′ N, 25°12′67.91′′ E; Crete, eastern Mediterranean; Fig. 1). The age model for the Agios Myron section was published by Zachariasse et al. (2021). The Agios Myron section presents lithological precessional cycles (confirmed by the vanadium concentrations in the bulk sediment and spectral analysis) that have been orbitally tuned through correlation with the Metochia section (Hilgen et al., 1995, 1997; Krijgsman et al., 1995, 1999) and anchoring in planktonic foraminifera bioevents and ash layers (Zachariasse et al., 2021). The horizon from which each sample was taken and their lithology, position along the section, age and sample number are provided in the Supplement. We sampled approximately 1 kg of sediment from each analyzed horizon, including both sapropels and calcareous marls, and used plain water to break down the sediment.
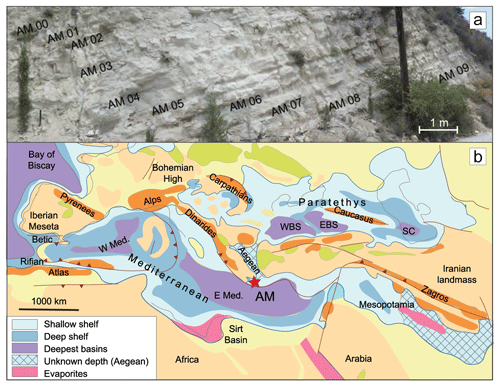
Figure 1Study section: (a) photograph of the section showing the lithological alternations and the sampling approach; (b) paleogeographic map of the Mediterranean–Paratethys domain during Late Miocene (Ilyina et al., 2004). Note the paleolatitudinal locations and their approximate position within the Late Miocene Mediterranean Basin for Agios Myron (AM). “W Med” denotes the western Mediterranean, whereas “E Med” refers to the eastern Mediterranean.
The samples were wet-sieved using various mesh sizes (minimum 63 µm). Up to five individuals of the shallow-dwelling planktonic foraminifer species Globigerinoides obliquus and the benthic foraminifer Cibicides (pseudo)ungerianus were picked from the 250–300 µm sieve fraction of the same samples, according to standard practices (Seidenkrantz et al., 2000; Elderfield et al., 2002). The otoliths were handpicked from the sieved sediment residues and identified to the species level. From the picked specimens, the two target species, Bregmaceros albyi and Lesueurigobius friesii, were identified in 20 and 14 samples, respectively. Single otoliths from each level (and duplicates from samples AM05 and AM20C) were measured (L: length; W: width), cleaned and analyzed. We selected well-preserved otoliths, making sure that all of their morphological characteristics were still present and that there was no particular coloration and/or signs of bioerosion or encrustation that may point toward extensive diagenetic alteration (Agiadi et al., 2022). Only otoliths of adult individuals were analyzed to avoid differences in species-specific vital effects that affect δ18Ooto values (Darnaude et al., 2014).
2.2 Ecological information about the selected fish species
The two fish species, whose otoliths were analyzed, were selected because of (a) their great abundance and frequency in Neogene and Quaternary Mediterranean marine sediments, which would render their isotopic compositions useful as paleoceanographic proxies, and (b) their well-established ecology, allowing more accurate interpretation of isotopic analyses results. Bregmaceros albyi is one of the most common extinct fish species in the Neogene and Quaternary Mediterranean, found in great abundance as both articulated skeletons and otoliths (Landini and Sorbini, 2005; Agiadi and Karakitsios, 2012; Agiadi et al., 2013). Bregmaceros spp. are small pelagic fishes with 14 extant species distributed in the Atlantic, Indian and Pacific oceans and mostly occupying the euphotic zone (FishBase, 2024). Even though Bregmaceros sp. was still present in the eastern Mediterranean until the Late Pleistocene (Cornée et al., 2019), B. nectabanus Whitley, 1941, is the only representative currently inhabiting the basin, and it is considered a non-indigenous species (Agiadi and Albano, 2020). Bregmaceros nectabanus has been reported up to 350 m depth, but it is also able to migrate temporarily into dysoxic waters (FishBase, 2024). Bregmaceros spp. feed on zooplanktonic invertebrates, particularly copepods, and some species also on phytoplankton (FishBase, 2024).
Lesueurigobius friesii is a benthic, subtropical marine fish living at depths of between 10 and 130 m in the eastern Atlantic and the Mediterranean Sea and feeding mainly on polychaetes, small crustaceans and mollusks (FishBase, 2024). Today, it has a preferred temperature of between 7.2 and 18.4 °C, with a mean of 10.4 °C (AquaMaps: predicted range maps for aquatic species). Its presence in the Mediterranean fossil record goes back to the late Tortonian (Agiadi et al., 2017), and it is common in shallow waters until the present (Agiadi et al., 2019; Agiadi and Albano, 2020). As a benthic species, L. friesii is site-attached; therefore, its isotopic composition is expected to closely reflect the local conditions on the seafloor (Mirasole et al., 2017).
2.3 Oxygen and carbon isotopic analyses on otoliths and planktonic foraminifera
The otoliths and the foraminifera specimens were sonicated in methanol for about 10 s to remove clay particles adhering to the tests and rinsed at least five times in ultraclean water. Randomly selected specimens of foraminifera and otoliths were observed using a JEOL JSM-6360 scanning electron microscope or a stereoscope, respectively, to confirm their good preservation state, following the established practices in the field (Antonarakou et al., 2019; Agiadi et al., 2022; Lopes et al., 2022). Complete and intact otoliths were crushed and homogenized, and the results have been interpreted to reflect whole-life conditions. Generally, otoliths seem to suffer little from diagenetic alteration, mostly maintaining their microstructure, chemistry and mineralogy. In fact, in their study of Pliocene otoliths from the Mediterranean, Dufour et al. (2000) discovered that most specimens remained aragonitic, while pyrite appeared in only a few otoliths. Moreover, the isotopic composition of otoliths depends on the taxon, rather than the geologic age (Dufour et al., 2000). Indeed, any diagenetic alteration is focused mostly on the outer part of the otolith (Cook et al., 2015).
Stable carbon and oxygen isotopic analyses were performed using a Thermo Scientific MAT 253 Plus mass spectrometer coupled to a GasBench II, in continuous-flow mode, using a thermostated sample tray and a gas chromatography PAL autosampler at the Goethe Universität–Senckenberg BiK-F Joint Stable Isotope Facility in Frankfurt am Main, Germany. Each sample (between ∼ 80 and ∼ 110 µg) was weighed and put in a 12 mL glass vial. The airtight glass vials were placed into a JUMO iTRON 16 temperature controller of the A200S autosampler of the Finnigan™ GasBench II from Thermo Scientific, which is attached to the mass spectrometer. The autosampler allows automated isotope ratio determination of small CO2 gas samples. To displace the atmospheric gas in the glass vials, each sample was flushed with 1.2 bar He (purity > 99.999 %) for 300 s. After this flush fill, viscous water-free 98 g mol−1 orthophosphoric acid (H3PO4) was injected with a syringe through the septum into each vial. CO2 gas, phosphates of calcium and water will be formed by carbonate reacting with the acid. The vials were heated in the temperature controller to a constant temperature of 72 °C to speed up the reaction between the carbonates and H3PO4 and to prevent the crystallization of the acid at room temperature. Analytical precision was 0.08 ‰ for δ18O and 0.06 ‰ for δ13C. In both cases, we analyzed replicates of 10 % of the data to confirm the reproducibility of the results; natural sample variability was better than 0.1 ‰. Results are reported against the Vienna Pee Dee Belemnite (VPDB) standard using the δ notation and are expressed in per mill (‰).
2.4 Additional biogeochemical data used for interpretation
The results of the otolith isotopic analyses were additionally interpreted considering the following: (a) the δ18O and δ13C values of C. (pseudo)ungerianus (Zachariasse et al., 2021); (b) paleodepth estimates based on foraminifera (Zachariasse et al., 2021); (c) tetraether index (TEX)-derived SST and TEX-δ18OG. obliquus-based SSS estimates (Kontakiotis et al., 2022); (d) the ratio of isoprenoidal glycerol dialkyl glycerol tetraethers 2 and 3 (isoGDGT2 / isoGDGT3) (Kontakiotis et al., 2022), which increases with isoGDGTs input from subsurface-dwelling archaea (Schouten et al., 2002); and (e) BIT (branched and isoprenoid tetraether) index values which reflect the source of organic matter (Butiseacă et al., 2022).
2.5 Statistical analyses
In order to test for the response of the fishes to significant changes in paleoceanographic parameters, we used the Sequential T-test Analysis of Regime Shifts (STARS) algorithm (Rodionov, 2004) to identify regime shifts based on the organic biomarker and foraminifera isotopic data. Regime shifts are abrupt changes between two natural states of climate or of an ecosystem, where each state can have its own internal variability (Kerr, 1992; Scheffer, 2020). The STARS algorithm allows for the robust detection of regime shifts, even at the ends of a time series (Rodionov, 2004). Because the STARS algorithm can only be applied to variables that have a normal distribution, we first tested for normality using the Shapiro–Wilk test. For those variables that did not have a normal distribution (here, δ13CB. albyi, δ18OL. friesii, BIT and δ13CC. ung), we used the L method instead (Lanzante, 1996), which is based on the Mann–Whitney U test. In STARS, we set the p value at 0.05, Huber's weight parameter at 1 and the window size at 10. The selection of the window size is generally arbitrary and can strongly affect the sensitivity of the algorithm. For this reason, we confirmed that the identified regime shifts were also detected when using window size values from 5 to 20.
We investigated the existence of size-dependent patterns in the δ18Ooto and δ13Coto values of the otoliths of the two species by testing for statistical correlation between the otolith size and the δ18Ooto and δ13Coto values, as otolith size is linked to fish size through species-specific functions (Edelist, 2014). For these tests, we used the Spearman rank correlation coefficient (95 % confidence level).
Furthermore, we examined possible covariance between the δ18O and δ13C values of the fish otoliths and foraminifera (reflecting the responses of these marine organisms) and the paleoenvironmental variables, specifically the SST and SSS values (Kontakiotis et al., 2022) and the BIT index (Butiseacă et al., 2022). For these tests, we used the Spearman rank correlation coefficient (95 % confidence level). We conducted these analyses across the entire study interval of the Agios Myron section. Considering the observed lithological cyclicity, we repeated the analyses for the sapropel levels only. Additionally, we used the results of the regime shifts detection, and we repeated the tests once more within each identified regime. The length of the time series does not permit searching for lagged responses.
The above analyses were performed in R (version 4.3.1) (R Development Core Team, 2023), and we used the “dplyr” (Wickham et al., 2023) and “rshift” (Room et al., 2020) packages.
3.1 Isotopic analysis results and size correlations
The δ18OB. albyi values range from −0.28 ‰ to 0.41 ‰ in the homogeneous marls, from −1.47 ‰ to 2.64 ‰ in the laminated marls and from −0.63 ‰ to 0.05 ‰ in the silty marls in the lower part of the section. The δ18OG. obliquus values range from −0.84 ‰ to 0.44 ‰ in the homogeneous marls, from −1.36 ‰ to 0.79 ‰ in the laminated marls and from −0.74 ‰ to 0.23 ‰ in the silty marls. The δ18OL. friesii values range from 2.14 ‰ to 4.30 ‰ in the homogeneous marls, from 2.32 ‰ to 4.11 ‰ in the laminated marls and from 2.07 ‰ to 2.2 ‰ in the silty marls (Agiadi et al., 2024).
The δ13CL. friesii values range from −5.80 ‰ to −4.20 ‰ in the homogeneous marls, from −5.81 ‰ to −4.19 ‰ in the laminated marls and from −5.41 ‰ to −4.68 ‰ in the silty marls in the lower part of the section. The δ13CG. obliquus values range from −0.18 ‰ to 0.46 ‰ in the homogeneous marls, from −0.35 ‰ to 1.49 ‰ in the laminated marls and from 0.29 ‰ to 1.13 ‰ in the silty marls. The δ13CB. albyi values range from −5.92 ‰ to −5.23 ‰ in the homogeneous marls, from −9.02 ‰ to −3.71 ‰ in the laminated marls and from −5.44 ‰ to −5.19 ‰ in the silty marls (Agiadi et al., 2024). There were no Lesueurigobius friesii otoliths in samples younger than 6.653 Ma.
No correlation was detected between the isotopic values and otolith length at the 95 % confidence level, with Spearman's ρ values of 0.13 (p=0.58) for δ13CB. albyi, 0.11 (p=0.70) for δ13CL. friesii, −0.04 (p=0.88) for δ18OB. albyi and −0.17 (p=0.56) for δ18OL. friesii.
For the entire Agios Myron record, there is a strong correlation between δ18OG. obliquus and SSS (N=24, ρ = 0.78, ), but no correlation between δ18OB. albyi and SSS (N=20, ρ = 0.12, p=0.61) or δ18OC. ung and SSS (N=22, ρ = 0.30, p=0.17). However, we found a moderate correlation between δ18OB. albyi and δ18OL. friesii values (N=10, ρ = 0.69, p=0.04).
Examining the laminated marls only, we found a moderate correlation between δ18OB. albyi and δ18OG. obliquus (N=20, ρ = 0.55, p = 0.05) and between δ18OG. obliquus and SST (N= 24, ρ = 0.60, p = 0.03). A strong correlation was found for the sapropel levels between δ18OG. obliquus and SSS (N= 14, ρ = 0.93, ). No correlation was found between δ13CB. albyi and δ13CG. obliquus (N= 14, ρ = 0.06, p = 0.84), between δ18OB. albyi and SST (N= 14, ρ = −0.09, p = 0.77), between δ18OC. ung and SST (N= 14, ρ = 0.24, p = 0.41), between δ18OB. albyi and SSS (N= 14, ρ = 0.39, p = 0.17), or between δ18OC. ung and SSS (N= 14, ρ = 0.24, p = 0.41). Combining the laminated marls with the silty marl levels in the analysis, we found moderate correlations between δ18OB. albyi and δ18OG. obliquus (N= 16, ρ = 0.50, p = 0.05) and between δ18OL. friesii and δ18OC. ung (N= 7, ρ = 0.78, p = 0.05).
3.2 Regime shifts and responses
The regime shift index (RSI) has positive values, which indicate the presence of regime shifts (95 % confidence level), at (1) 6.951 Ma for δ18OG. obliquus (RS1; RSI = 0.23), (2) 6.882 Ma for SST (RS2; RSI = 0.70), (3) 6.847 Ma for SSS (RS3; RSI = 0.85) and (4) 6.814 Ma for δ13CG. obliquus (RS4; RSI = 0.33). There is no correlation between any of the biogeochemical proxies used here from 7.166 to 6.951 Ma (RS1). After 6.951 Ma (RS1), a very strong correlation was indeed found between δ18OG. obliquus and SSS (N= 15, ρ = 0.85, ). Examining the laminated marls, there is only a weak correlation between δ18OB. albyi and δ18OG. obliquus (N= 12, ρ = 0.56, p = 0.06) after 6.951 Ma, whereas there is a very strong correlation between δ18OG. obliquus and SSS (N= 12, ρ = 0.88, ). From 7.166 to 6.882 Ma (RS2), a moderate correlation was found between δ18OG. obliquus and SST (N= 11, ρ = −0.63, p = 0.04), but only a weak correlation was found between δ18OG. obliquus and SSS (N= 11, ρ = 0.58, p = 0.07). After 6.882 Ma (RS2), there is only a very strong correlation between δ18OG. obliquus and SSS (N= 13, ρ = 0.93, ). From 7.166 until 6.847 Ma (RS3), δ18OG. obliquus is strongly correlated with SST (N= 12, ρ = –0.62, p = 0.03) but weakly correlated with SSS (N= 12, ρ = 0.54, p = 0.07); in contrast, after 6.847 Ma, there is only a very strong correlation between δ18OG. obliquus and SSS (N= 12, ρ = 0.94, ). From 7.166 until 6.814 Ma (RS4), a moderate correlation is found between δ18OG. obliquus and SSS (N= 14, ρ = 0.69, p = 0.006); after 6.814 Ma (RS4), the correlation between δ18OG. obliquus and SSS (N= 10, ρ = 0.94, ) becomes very strong.
4.1 Paleoceanographic conditions
Our results evidence a significant correlation between otolith and foraminifera δ18O values in surface and bottom waters, collected from the laminated and the silty marls at the base of the section. This result confirms δ18Ooto as a promising proxy for paleoceanographic studies (as anticipated by, e.g., Radtke et al., 1996; Thorrold et al., 1997; and Darnaude et al., 2014), supporting the combined use of δ18O measured on both otoliths and foraminifera to reveal changes in the life history and migration patterns of teleost fishes in deep time.
As expected, the δ18O measurements of the bottom-dwellers (δ18OL. friesii and δ18OC. ung) have more positive values than those of the surface-dwellers (δ18OB. albyi and δ18OG. obliquus), as the benthic fish and foraminifera occupy the bottom of the water column, which is colder and more saline than the surface water (Fig. 2).
Sea surface salinity fluctuations in the eastern Mediterranean were the dominant factor controlling the biomineral isotopic composition of zooplankton and fishes as early as 6.882 Ma (RS2) and until 6.5 Ma, as seen by the δ18OG. obliquus–SSS and δ18OB. albyi–SSS correlations. Between 7.166 and 6.951 Ma (RS1), surface conditions at Agios Myron were mostly controlled by SST, as shown by the correlation between δ18OG. obliquus and SST. For the interval between 6.951 (RS1) and 6.882 Ma (RS2), both SSS and SST drive the zooplankton and fish responses.
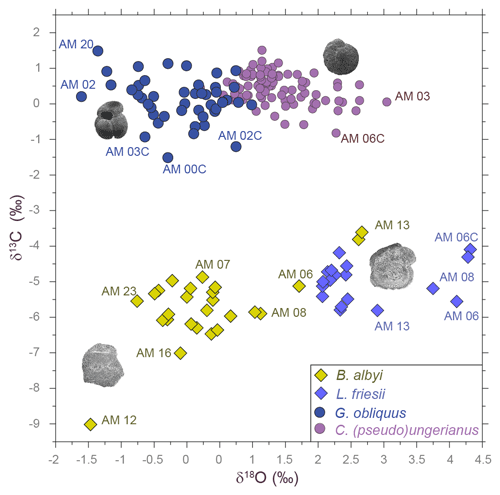
Figure 2The δ18O–δ13C plot of the fish otolith and foraminifer samples analyzed from the Agios Myron section. The four species clusters are clearly distinguished in the plot. Labels of several atypical samples are displayed.
The regime shift in δ13CG. obliquus values at 6.814 Ma (RS4; Fig. 3) takes place in coincidence with a high-amplitude disturbance of δ18OB. albyi, mimicking the change in the Archaea community (isoGDGT2 / isoGDGT3 index). Considering that δ18OL. friesii does not show a similar disturbance, we interpret the δ18OB. albyi pattern to reflect the short-lived event of an intermittently remarkably negative water budget at 6.824 Ma (Butiseacă et al., 2022). Subsequently, from 6.814 Ma until 6.5 Ma, δ18OL. friesii and δ18OC. ung values exhibit increasing trends, in contrast to δ18OB. albyi and δ18OG. obliquus, suggesting that the event at 6.824 Ma triggered persistent stratification and increased bottom-water salinity. This interpretation is also corroborated by the increase in abundance of neogloboquadrinids (Zachariasse et al., 2021), which are indicators of high nutrient availability in a colder, stratified water column (Sierro et al., 2003). The presence of high-salinity-tolerant benthic foraminifera and the concomitant Mn/Al depletion at this time interval suggest decreased bottom-water oxygenation throughout the Mediterranean after a gateway restriction step at 6.8 Ma (Kouwenhoven et al., 2003; Sierro et al., 2003; Lyu et al., 2022). As diagenesis has been shown to affect the otoliths of both pelagic and benthic fishes in a similar way, at least in a continental shelf environment (Agiadi et al., 2022), this benthic–pelagic decoupling after 6.824 Ma is considered to indeed reflect paleoenvironmental change. In contrast, a small, positive shift in δ18OB. albyi is observed at 6.68 Ma, which co-occurs with a positive shift in δ18OL. friesii, possibly reflecting similar changes in both surface and bottom waters, most likely due to an SST drop to 22 °C rather than an SSS decrease that would bring the δ18Ooto towards lower values (Kontakiotis et al., 2022).
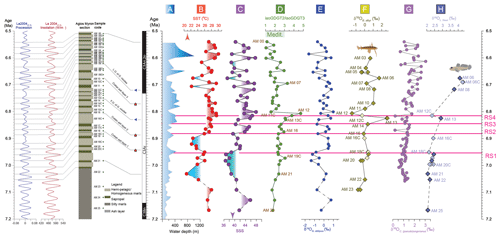
Figure 3The Agios Myron lithostratigraphic column, major bioevents and chronostratigraphy, in the precession and insolation framework for the time interval of the study (Laskar et al., 2004): (a) paleobathymetric curve based on foraminifera (Zachariasse et al., 2021); (b) sea surface temperature (SST) calculated based on TEX; (c) sea surface salinities (SSSs) based on SST-TEX and δ18OG. obliquus (Kontakiotis et al., 2022); (d) the ratio of isoprenoidal glycerol dialkyl glycerol tetraethers 2 and 3 (isoGDGT2 / isoGDGT3) (Butiseacă et al., 2022); (e) δ18O of the shells of the planktonic foraminifer Globigerinoides obliquus; (f) δ18O of the otoliths of the pelagic fish Bregmaceros albyi; (g) δ18O of the shells of the benthic foraminifer Cibicides (pseudo)ungerianus (Zachariasse et al., 2021); and (h) δ18O of the otoliths of the benthic fish Lesueurigobius friesii. RS1–RS4 indicate the four regime shifts found in this study at 6.951 Ma for δ18OG. obliquus, 6.882 Ma for SST, 6.847 Ma for SSS and 6.814 Ma for δ13CG. obliquus.
4.2 Fish metabolic response to paleoceanographic change
Carbon in otoliths originates from two sources: seawater and diet. Therefore, δ13Coto values reflect changes in the seawater δ13C, which depends on pH, and in the fish's diet and metabolic rate. The δ13Coto values here are more negative than those of the foraminifera (Fig. 2), which is expected because δ13Cdiet is more negative than δ13CDIC. Here, considering δ13CG. obliquus and δ13CC. ung values as reflecting δ13CDIC in surface and bottom waters, respectively (Mackensen and Schmiedl, 2019), we evaluate the δ13Coto changes as indicative of changes in the metabolic responses of the two fish species. Considering the foraminifera δ13C as a proxy for seawater δ13C, the δ13Coto values are mostly controlled by fish metabolism (Fig. 4e, h). Notably, the δ13Coto and the Δ13C records (expressed as Δ13C and Δ13C) exhibit the same trends for both surface (Fig. 4e) and bottom (Fig. 4h) waters.
For fish feeding on phytoplankton and zooplankton, such as those examined in this study, δ13Coto is negatively correlated with food availability and, therefore, with net primary productivity (Burton et al., 2011): under conditions of high (low) food availability, individual fishes with high (low) metabolic rates are more resilient and would exhibit lower (higher) δ13Coto values, as they would be consuming more and, consequently, acquiring more 12C from their diet. In addition, δ13Coto values may be positively, negatively, or not at all correlated with temperature, depending on the species, oceanographic conditions and setting (Kalish, 1991; Martino et al., 2019). In the case of Agios Myron, no significant correlation is found between δ13CB. albyi (or δ18OB. albyi) and SST or SSS values. Both δ13CB. albyi and δ18OB. albyi show much wider ranges in the laminated than in the homogenous marls, but these are not accompanied by greater ranges in SST or SSS in the sapropelitic levels (Kontakiotis et al., 2022). δ13CB. albyi alone could be explained by wider ranges in primary productivity and nutrient supply among the laminated marls. In that case, the δ18OB. albyi range would mean that the fishes also experienced larger ranges in salinities and/or temperatures (compared with the homogeneous marl levels). However, such a hypothesis is not supported by the SST and SSS records (Fig. 3). Alternatively, the δ13CB. albyi and δ18OB. albyi ranges (Figs. 3, 4) could be attributed to shifts in the depth distribution of B. albyi to include deeper, even anoxic, parts of the water column, as is common for the modern B. nectabanus (FishBase, 2024).
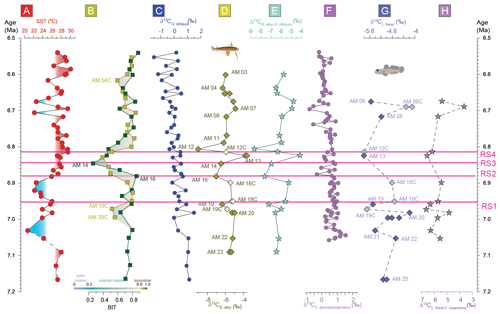
Figure 4The Agios Myron (a) sea surface temperature (SST) calculated based on TEX (Kontakiotis et al., 2022); (b) branched and isoprenoid tetraether (BIT) index (Butiseacă et al., 2022); (c) δ13C of the shells of the planktonic foraminifer Globigerinoides obliquus; (d) δ13C of the otoliths of the pelagic fish Bregmaceros albyi; (e) difference between δ13CB. albyi and δ13CG. obliquus; (f) δ13C of the shells of the benthic foraminifer Cibicides (pseudo)ungerianus (Zachariasse et al., 2021); (g) δ13C of the otoliths of the benthic fish Lesueurigobius friesii; and (h) difference between δ13CL. friesii and δ13CC. ung. RS1–RS4 indicate the four regime shifts found in this study at 6.951 Ma for δ18OG. obliquus, 6.882 Ma for SST, 6.847 Ma for SSS and 6.814 Ma for δ13CG. obliquus.
The foraminifera δ13C records (Fig. 4c, f) follow the global decreasing trend. Both surface DIC and bottom-water DIC become increasingly depleted in 13C from 7.2 to 6.5 Ma in the Agios Myron section (as both δ13CG. obliquus and δ13CC. ung show negative trends; Fig. 4). In the absence of a similar trend in the BIT index (i.e., a change in the terrestrial versus aquatic source of organic matter), these trends reflect decreased productivity and ventilation of bottom waters, in line with the North Atlantic and western Mediterranean records (Hodell et al., 2001; van der Schee et al., 2016; Drury et al., 2018; Bulian et al., 2022, 2023). In contrast, otoliths cannot be used as proxies for the δ13C of seawater, because they are strongly influenced by the δ13C of the fishes' diet, which varies greatly. For example, Bregmaceros spp. feed on zooplanktonic invertebrates (mostly copepods), but some species are also known to eat phytoplankton (FishBase, 2024). As the exact composition of the Late Miocene fish diet in Agios Myron cannot be determined, we cannot attribute the observed δ13Coto shifts to changes in the abundance of particular organisms (e.g., copepods). Ultimately, δ13Coto is a proxy for the average fish metabolism (Wurster and Patterson, 2003; Solomon et al., 2006; Trueman et al., 2016; Chung et al., 2019a, b; Martino et al., 2020; Smoliński et al., 2021; Trueman et al., 2023; Jones et al., 2023). Both δ13CB. albyi and δ13CL. friesii show negative peaks at 6.959, 6.899 and 6.806 Ma, which coincide with changes in the composition of the Achaea community (isoGDGT2 / isoGDGT3; Fig. 3) and fall around the times of peaks in the BIT index. The statistical testing did not show a correlation between δ13CB. albyi, δ13CL. friesii, BIT and isoGDGT2 / isoGDGT3, and the time interval of this study does not permit an investigation of any lagged responses. Additionally, we consider it highly unlikely that diagenetic effects would produce coincident peaks in the carbon isotopic ratio of fish otoliths and BIT: the carbon isotopic composition of fish otoliths reflects mostly the ratio of their diet items (in this case, phytoplankton and zooplankton), whereas BIT is the ratio of terrigenous/aquatic GDGTs that come predominately from terrestrial soils, peats, lakes and rivers (Hopmans et al., 2004; Peterse et al., 2012) or from Thaumarchaeota (mostly sourced by marine archaeol picoplankton; Sinninghe Damsté et al., 2002). Therefore, we consider that these negative δ13CB. albyi and δ13CL. friesii peaks reflect abrupt, regional events related to food availability crises (source and content) for surface and bottom-water fishes, which impacted fish metabolism.
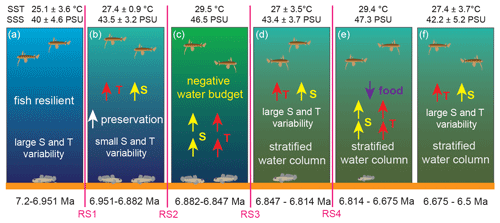
Figure 5Schematic model of the response of surface- and bottom-dwelling marine fishes to the paleoceanographic and paleoclimatic changes taking place from 7.2 to 6.5 Ma in the eastern Mediterranean. RS1–RS4 indicate the four regime shifts found in this study at 6.951 Ma for δ18OG. obliquus, 6.882 Ma for SST, 6.847 Ma for SSS and 6.814 Ma for δ13CG. obliquus.
A single regime shift in δ13CG. obliquus is found at 6.814 Ma (RS4) and coincides with a change in the Archaea community (isoGDGT2 / isoGDGT3 index; Fig. 4; Butiseacă et al., 2022). The event at 6.82–6.81 Ma has a strong impact on fish metabolism, as is clearly seen first in the negative peak in δ13CB. albyi and then in the positive shifts in δ13CB. albyi and δ13CL. friesii, which are accompanied by a BIT shift to an increased relative contribution of terrestrial organic matter (Butiseacă et al., 2022) and to the maximum of isoGDGT2 / isoGDGT3. Along with the positive δ18OB. albyi shift at 6.824 Ma that is attributed to a negative water-budget event (see Sect. 4.1), we propose a temporary influx of high-nutrient terrestrial material at 6.814 Ma, leading to a phytoplankton bloom and a consequent increase in zooplankton abundance, providing more food for the fish population in both surface and bottom waters. After this point, δ13CL. friesii increases and peaks at 6.690 Ma (Fig. 4), indicating a corresponding decrease in metabolic rate, and then abruptly drops at 6.675 Ma before the disappearance of L. friesii from Agios Myron and other Mediterranean sites (Karakitsios et al., 2017; Schwarzhans et al., 2020). At 6.690 Ma, there is a pronounced drop in δ13CC. ung but no corresponding change in δ13CG. obliquus, δ18OC. ung or δ18OG. obliquus, suggesting that decreased bottom-water ventilation, combined with basin deepening at the Agios Myron location (Zachariasse et al., 2021), resulted in the local extirpation of L. friesii.
Evolutionary adaptation is expected to reduce the effects of environmental change on physiology; however, when multiple environmental stressors act contemporaneously, their interactions largely determine the net effect (Petitjean et al., 2019). In the early Messinian, temperature and salinity in the Mediterranean Sea (Vasiliev et al., 2019; Kontakiotis et al., 2019, 2022) as well as the source and amount of organic matter available (including from primary production; Kontakiotis et al., 2020; Butiseacă et al., 2022) showed large-amplitude variability. Temperature and salinity would be expected to act synergistically, as they both increase metabolism (Clarke and Johnston, 1999; Gillooly et al., 2001; Komoroske et al., 2016), but the roles of primary productivity and organic matter type are unclear. At the evolutionary scale, to survive, species would have to adapt to the prolonged and compound effects of these multiple stressors, considering also that the frequency of shifts between warm and/or higher-salinity and cold and/or lower-salinity intervals increased after 6.72 Ma (Kontakiotis et al., 2022, Butiseacă et al., 2022). Our results suggest that the pelagic fish species indeed adapted well to the combined effects of temperature and salinity changes, whereas their benthic counterparts could not adapt after 6.814 Ma (RS4). After this tipping point, a change in the source and amount of organic matter also took place, which we believe led to the local maladaptation and finally extirpation of the benthic fish communities.
In the pre-MSC Messinian, due to the restriction of the Mediterranean–Atlantic gateway, the Mediterranean experienced intense variability in temperature and salinity as well as changes in primary productivity and terrestrial influx, which resulted in increased stratification of the water column and decreased ventilation of the bottom waters (Fig. 5). Our results evidence turning points in the Mediterranean paleoceanographic conditions between 6.951 and 6.882 Ma, when salinity and its variation amplitude increase were probably related to stratification of the water column, and at 6.814 Ma, when there is a major disturbance in the influx amount and source of organic material to the basin driving a change in primary production. For fishes, the most important event is the regime shift at 6.814 Ma (RS4), after which we see a pelagic–benthic decoupling with δ18OL. friesii expressing the higher salinity of the lower part of the water column, as well as a rapid increase and then drop in the metabolic rate of the benthic fish (δ13CL. friesii), ultimately leading to the local extirpation of the species.
The raw data used in this paper are publicly available from https://doi.org/10.5281/zenodo.10602427 (Agiadi et al., 2024).
KA and IV: conceptualization, funding acquisition, visualization and writing – original draft preparation; IV and GK: data curation; KA: formal analysis and methodology; IV, GB, GK, DT, EB, SZ, EK, AA and AM: investigation; GB, GK, DT, EB, SZ, EK, AA and AM: writing – review and editing.
The contact author has declared that none of the authors has any competing interests.
Publisher’s note: Copernicus Publications remains neutral with regard to jurisdictional claims made in the text, published maps, institutional affiliations, or any other geographical representation in this paper. While Copernicus Publications makes every effort to include appropriate place names, the final responsibility lies with the authors.
The authors would like to thank Clive Trueman, for his insightful comments on an early version of this paper, and the anonymous reviewers, for their very constructive comments. This research was funded in whole, or in part, by the Austrian Science Fund (FWF; grant no. DOI 10.55776/V986, available via https://www.fwf.ac.at/en/discover/research-radar, last access: 28 August 2024; PI: Konstantina Agiadi). For the purpose of open access, the author has applied a CC BY public copyright license to any author-accepted manuscript version arising from this submission. This work was also supported by Greek national funds and the European Social Fund through the “Postdoctoral Research Fellowships” action of the Greek State Scholarships Foundation (“Comparative study of the Messinian Salinity Crisis effect on the eastern Ionian and northern Aegean ichthyofauna” project, 2017–2019; Konstantina Agiadi) and by the Greek–German collaboration project (IKYDA-DAAD) “Quantification of the environmental changes in the eastern Mediterranean at the onset of the Messinian Salinity Crisis (Crete-Greece)” (QUANTMES; Iuliana Vasiliev). Collaboration for this project was facilitated by the COST Action CA15103 “Uncovering the Mediterranean salt giant” MEDSALT (2016–2020).
This research was funded in whole, or in part, by the Austrian Science Fund (FWF; grant no. DOI 10.55776/V986, available via https://www.fwf.ac.at/en/discover/research-radar; PI: Konstantina Agiadi).
This paper was edited by Andrew Thurber and reviewed by two anonymous referees.
Agiadi, K. and Albano, P. G.: Holocene fish assemblages provide baseline data for the rapidly changing eastern Mediterranean, Holocene, 30, 1438–1450, https://doi.org/10.1177/0959683620932969, 2020.
Agiadi, K. and Karakitsios, V.: Quaternary climatic variability modulates Bregmaceros Mediterranean distribution range, Proc. 10th Hell. Symp. Oceanogr. Fish. Athens, 7–11 May 2012, Athens, Greece, Hellenic Centre for Marine Research, 1–6, 2012.
Agiadi, K., Koskeridou, E., Triantaphyllou, M., Girone, A., and Karakitsios, V.: Fish otoliths from the Pliocene Heraklion Basin (Crete Island, Eastern Mediterranean), Geobios, 46, 461–472, https://doi.org/10.1016/j.geobios.2013.07.004, 2013.
Agiadi, K., Antonarakou, A., Kontakiotis, G., Kafousia, N., Moissette, P., Cornée, J.-J., Manoutsoglou, E., and Karakitsios, V.: Connectivity controls on the late Miocene eastern Mediterranean fish fauna, Int. J. Earth Sci., 106, 1147–1159, https://doi.org/10.1007/s00531-016-1355-7, 2017.
Agiadi, K., Vasileiou, G., Koskeridou, E., Moissette, P., and Cornee, J.-J.: Coastal fish otoliths from the early Pleistocene of Rhodes (eastern Mediterranean), Geobios, 55, 1–15, https://doi.org/10.1016/j.geobios.2019.06.006, 2019.
Agiadi, K., Azzarone, M., Hua, Q., Kaufman, D. S., Thivaiou, D., and Albano, P. G.: The taphonomic clock in fish otoliths, Paleobiology, 48, 154–170, https://doi.org/10.1017/pab.2021.30, 2022.
Agiadi, K., Vasiliev, I., Butiseacă, G., Kontakiotis, G., Thivaiou, D., Besiou, E., Zarkogiannis, S., Koskeridou, E., Antonarakou, A., and Mulch, A.: Dataset for Agiadi et al._Coupled otolith and foraminifera oxygen and carbon stable isotopes evidence paleoceanographic changes and fish metabolic responses, Zenodo [data set], https://doi.org/10.5281/zenodo.10602427, 2024.
Antonarakou, A., Kontakiotis, G., Vasilatos, C., Besiou, E., Zarkogiannis, S., Drinia, H., Mortyn, P. G., Tsaparas, N., Makri, P., and Karakitsios, V.: Evaluating the Effect of Marine Diagenesis on Late Miocene Pre-Evaporitic Sedimentary Successions of Eastern Mediterranean Sea, IOP Conf. Ser. Earth Environ. Sci., 221, 012051, https://doi.org/10.1088/1755-1315/221/1/012051, 2019.
Bulian, F., Sierro, F. J., Ledesma, S., Jiménez-Espejo, F. J., and Bassetti, M.-A.: Messinian West Alboran Sea record in the proximity of Gibraltar: Early signs of Atlantic-Mediterranean gateway restriction, Mar. Geol., 434, 106430, https://doi.org/10.1016/j.margeo.2021.106430, 2021.
Bulian, F., Kouwenhoven, T. J., Jiménez-Espejo, F. J., Krijgsman, W., Andersen, N., and Sierro, F. J.: Impact of the Mediterranean-Atlantic connectivity and the late Miocene carbon shift on deep-sea communities in the Western Alboran Basin, Palaeogeogr. Palaeocl., 589, 110841, https://doi.org/10.1016/j.palaeo.2022.110841, 2022.
Bulian, F., Jiménez-Espejo, F. J., Andersen, N., Larrasoaña, J. C., and Sierro, F. J.: Mediterranean water in the Atlantic Iberian margin reveals early isolation events during the Messinian Salinity Crisis, Glob. Planet. Change, 231, 104297, https://doi.org/10.1016/j.gloplacha.2023.104297, 2023.
Burton, T., Killen, S. S., Armstrong, J. D., and Metcalfe, N. B.: What causes intraspecific variation in resting metabolic rate and what are its ecological consequences?, P. R. Soc. B, 278, 3465–3473, https://doi.org/10.1098/rspb.2011.1778, 2011.
Butiseacă, G. A., van der Meer, M. T. J., Kontakiotis, G., Agiadi, K., Thivaiou, D., Besiou, E., Antonarakou, A., Mulch, A., and Vasiliev, I.: Multiple Crises Preceded the Mediterranean Salinity Crisis: Aridification and Vegetation Changes Revealed by Biomarkers and Stable Isotopes, Glob. Planet. Change, 217, 103951, https://doi.org/10.1016/j.gloplacha.2022.103951, 2022.
Campana, S. E.: Chemistry and composition of fish otoliths: pathways, mechanisms and applications, Mar. Ecol. Prog. Ser., 188, 263–297, 1999.
Chung, M.-T., Trueman, C. N., Godiksen, J. A., Holmstrup, M. E., and Grønkjær, P.: Field metabolic rates of teleost fishes are recorded in otolith carbonate, Commun. Biol., 2, 1–10, https://doi.org/10.1038/s42003-018-0266-5, 2019a.
Chung, M.-T., Trueman, C. N., Godiksen, J. A., Grønkjær, P., Chung, M.-T., Trueman, C. N., Godiksen, J. A., and Grønkjær, P.: Otolith δ13C values as a metabolic proxy: approaches and mechanical underpinnings, Mar. Freshw. Res., 70, 1747–1756, https://doi.org/10.1071/MF18317, 2019b.
Clarke, A., and Johnston, N. M.: Scaling of metabolic rate with body mass and temperature in teleost fish, J. Anim. Ecol., 68, 893–905, https://doi.org/10.1046/j.1365-2656.1999.00337.x, 1999.
Cook, P. K., Languille, M.-A., Dufour, E., Mocuta, C., Tombret, O., Fortuna, F., and Bertrand, L.: Biogenic and diagenetic indicators in archaeological and modern otoliths: Potential and limits of high definition synchrotron micro-XRF elemental mapping, Chem. Geol. 414, 1–15, https://doi.org/10.1016/j.chemgeo.2015.08.017, 2015.
Cornée, J.-J., Quillévéré, F., Moissette, P., Fietzke, J., López-Otálvaro, G. E., Melinte-Dobrinescu, M., Philippon, M., Hinsbergen, D. J. J. van, Agiadi, K., Koskeridou, E., and Münch, P.: Tectonic motion in oblique subduction forearcs: insights from the revisited Middle and Upper Pleistocene deposits of Rhodes, Greece, J. Geol. Soc., 176, 78–96, https://doi.org/10.1144/jgs2018-090, 2019.
Darnaude, A. M., Sturrock, A., Trueman, C. N., Mouillot, D., EIMF, Campana, S. E., and Hunter, E.: Listening in on the past: What can otolith δ18O values really tell us about the environmental history of fishes?, PLOS ONE, 9, e108539, https://doi.org/10.1371/journal.pone.0108539, 2014.
Dietl, G. P., Kidwell, S. M., Brenner, M., Burney, D. A., Flessa, K. W., Jackson, S. T., and Koch, P. L.: Conservation Paleobiology: leveraging knowledge of the Past to inform conservation and restoration, Ann. Rev. Earth Planet. Sci., 43, 79–103, https://doi.org/10.1146/annurev-earth-040610-133349, 2015.
Donelson, J. M., Sunday, J. M., Figueira, W. F., Gaitan-Espitia, J. D., Hobday, A. J., Johnson, C. R., Leis, J. M., Ling, S. D., Marshall, D., Pandolfi, J. M., Pecl, G., Rodgers, G. G., Booth, D. J., and Munday, P. L.: Understanding interactions between plasticity, adaptation and range shifts in response to marine environmental change, Philos. T. R. Soc. B, 374, 1768, 20180186, https://doi.org/10.1098/rstb.2018.0186, 2019.
Drury, A. J., Westerhold, T., Hodell, D., and Röhl, U.: Reinforcing the North Atlantic backbone: revision and extension of the composite splice at ODP Site 982, Clim. Past, 14, 321–338, https://doi.org/10.5194/cp-14-321-2018, 2018.
Dufour, E., Cappetta, H., Denis, A., Dauphin, Y., and Mariotti, A.: La diagenese des otolithes par la comparaison des donnees microstructurales, mineralogiques et geochimiques: Application aux fossiles du Pliocene du Sud-Est de la France, Bull. Soc. Geol. France, 171, 521–532, 2000.
Edelist, D.: New length–weight relationships and Lmax values for fishes from the Southeastern Mediterranean Sea, J. Appl. Ichthyol., 30, 521–526, https://doi.org/10.1111/j.1439-0426.2012.02060.x, 2014.
Elderfield, H., Vautravers, M., and Cooper, M.: The relationship between shell size and , , δ18O, and δ13C of species of planktonic foraminifera, Geochem. Geophy. Geosy., 3, 1–13, https://doi.org/10.1029/2001GC000194, 2002.
Flecker, R., Krijgsman, W., Capella, W., de Castro Martíns, C., Dmitrieva, E., Mayser, J. P., Marzocchi, A., Modestu, S., Ochoa, D., Simon, D., Tulbure, M., van den Berg, B., van der Schee, M., de Lange, G., Ellam, R., Govers, R., Gutjahr, M., Hilgen, F., Kouwenhoven, T., Lofi, J., Meijer, P., Sierro, F. J., Bachiri, N., Barhoun, N., Alami, A. C., Chacon, B., Flores, J. A., Gregory, J., Howard, J., Lunt, D., Ochoa, M., Pancost, R., Vincent, S., and Yousfi, M. Z.: Evolution of the Late Miocene Mediterranean-Atlantic gateways and their impact on regional and global environmental change, Earth-Sci. Rev., 150, 365–392, https://doi.org/10.1016/j.earscirev.2015.08.007, 2015.
Gauldie, R. W.: Biological factors controlling the carbon isotope record in fish otoliths: Principles and evidence, Comp. Biochem. Physiol. B, 115, 201–208, https://doi.org/10.1016/0305-0491(96)00077-6, 1996.
Gillooly, J. F., Brown, J. H., West, G. B., Savage, V. M., and Charnov, E. L.: Effects of size and temperature on metabolic rate, Science, 291, 2248–2251, 2001.
Girone, A., Nolf, D., and Cavallo, O.: Fish otoliths from the pre-evaporitic (Early Messinian) sediments of northern Italy: Their stratigraphic and palaeobiogeographic significance, Facies, 56, 399–432, https://doi.org/10.1007/s10347-010-0212-6, 2010.
Herbert, T. D., Lawrence, K. T., Tzanova, A., Peterson, L. C., Caballero-Gill, R., and Kelly, C. S.: Late Miocene global cooling and the rise of modern ecosystems, Nat. Geosci., 9, ngeo2813, https://doi.org/10.1038/ngeo2813, 2016.
Hilgen, F. J., Krijgsman, W., Langereis, C. G., Lourens, L. J., Santarelli, A., and Zachariasse, W. J.: Extending the astronomical (polarity) time scale into the Miocene, Earth Planet. Sc. Lett., 136, 495–510, https://doi.org/10.1016/0012-821X(95)00207-S, 1995.
Hilgen, F. J., Krijgsman, W., and Wijbrans, J. R.: Direct comparison of astronomical and ages of ash beds: Potential implications for the age of mineral dating standards, Geophys. Res. Lett., 24, 2043–2046, https://doi.org/10.1029/97GL02029, 1997.
Hodell, D., Curtis, J., Sierro, F., and Raymo, M.: Correlation of late Miocene to early Pliocene sequences between the Mediterranean and North Atlantic, Paleoceanography, 16, 164–178, https://doi.org/10.1029/1999PA000487, 2001.
Hopmans, E. C., Weijers, J. W. H., Schefuss, E., Herfort, L., Sinninghe Damsté, J. S., and Schouten, S.: A novel proxy for terrestrial organic matter in sediments based on branched and isoprenoid tetraether lipids, Earth Planet. Sc. Lett. 224, 107–116, https://doi.org/10.1016/j.epsl.2004.05.012, 2004.
Hsü, K. J., Ryan, W. B. F., and Cita, M. B.: Late Miocene Desiccation of the Mediterranean, Nature, 242, 240–244, https://doi.org/10.1038/242240a0, 1973.
Ilyina, L. B., Shcherba, I. G., and Khondkarian, S. O.: Map 8: Middle late Miocene (late Tortonian – early Messinian – early Maeotian – late Pannonian) in Lithological-Paleogeographic maps of Paratethys, in: Cour. Forschungsinstitut Senckenberg, edited by: Popov, S. V., Rögl, F., Rozanov, A. Y., Steininger, F. F., Shcherba, I. G., and Kovac, M., Schweizerbart Science Publishers, ISBN: 978-3-510-61370-0, 250 pp., 2004.
Jones, J., Hunter, E., Hambach, B., Wilding, M., and Trueman, C. N.: Individual variation in field metabolic rates of wild living fish have phenotypic and ontogenetic underpinnings: insights from stable isotope compositions of otoliths, Front. Ecol. Evol., 11, 1161105, https://doi.org/10.3389/fevo.2023.1161105, 2023.
Kalish, J. M.: Oxygen and carbon stable isotopes in the otoliths of wild and laboratory-reared Australian salmon (Arripis trutta), Mar. Biol., 110, 37–47, https://doi.org/10.1007/BF01313090, 1991.
Karakitsios, V., Roveri, M., Lugli, S., Manzi, V., Gennari, R., Antonarakou, A., Triantaphyllou, M., Agiadi, K., Kontakiotis, G., Kafousia, N., and de Rafelis, M.: A record of the Messinian salinity crisis in the eastern Ionian tectonically active domain (Greece, eastern Mediterranean), Basin Res., 29, 203–233, https://doi.org/10.1111/bre.12173, 2017.
Kerr, R. A.: Unmasking a Shifty Climate System, Science, 255, 1508–1510, https://doi.org/10.1126/science.255.5051.1508, 1992.
Komoroske, L. M., Jeffries, K. M., Connon, R. E., Dexter, J., Hasenbein, M., Verhille, C., and Fangue, N. A.: Sublethal salinity stress contributes to habitat limitation in an endangered estuarine fish, Evol. Appl., 9, 963–981, 2016.
Kontakiotis, G., Besiou, E., Antonarakou, A., Zarkogiannis, S., Kostis, A., Mortyn, P. G., Moissette, P., Cornée, J. J., Schulbert, C., Drinia, H., Anastasakis, G., and Karakitsios, V.: Decoding sea surface and paleoclimate conditions in the eastern Mediterranean over the Tortonian-Messinian Transition. Palaeogeogr. Palaeocl., 534, 109312, https://doi.org/10.1016/j.palaeo.2019.109312, 2019.
Kontakiotis, G., Karakitsios, V., Cornée, J. J., Moissette, P., Zarkogiannis, S. Z., Pasadakis, N., Koskeridou, E., Manoutsoglou, E., Drinia, H., and Antonarakou, A.: Preliminary results based on geochemical sedimentary constraints on the hydrocarbon potential and depositional environment of a Messinian sub-salt mixed siliciclastic-carbonate succession onshore Crete (Plouti section, eastern Mediterranean), Med. Geosc. Rev., 2, 247–265, https://doi.org/10.1007/s42990-020-00033-6, 2020.
Kontakiotis, G., Butiseacă, G. A., Antonarakou, A., Agiadi, K., Zarkogiannis, S. D., Krsnik, E., Besiou, E., Zachariasse, W. J., Lourens, L., Thivaiou, D., Koskeridou, E., Moissette, P., Mulch, A., Karakitsios, V., and Vasiliev, I.: Hypersalinity accompanies tectonic restriction in the eastern Mediterranean prior to the Messinian Salinity Crisis, Palaeogeogr. Palaeocl., 592, 110903, https://doi.org/10.1016/j.palaeo.2022.110903, 2022.
Kouwenhoven, T. J., Hilgen, F. J., and van der Zwaan, G. J.: Late Tortonian–early Messinian stepwise disruption of the Mediterranean–Atlantic connections: constraints from benthic foraminiferal and geochemical data, Palaeogeogr. Palaeocl., 198, 303–319, https://doi.org/10.1016/S0031-0182(03)00472-3, 2003.
Krijgsman, W., Hilgen, F. J., Langereis, C. G., Santarelli, A., and Zachariasse, W. J.: Late Miocene magnetostratigraphy, biostratigraphy and cyclostratigraphy in the Mediterranean, Earth Planet. Sc. Lett., 136, 475–494, https://doi.org/10.1016/0012-821X(95)00206-R, 1995.
Krijgsman, W., Hilgen, F. J., Raffi, I., Sierro, F. J., and Wilson, D. S.: Chronology, causes and progression of the Messinian salinity crisis, Nature, 400, 652–655, https://doi.org/10.1038/23231, 1999.
Kroopnick, P. M.: The distribution of 13C of ΣCO2 in the world oceans, Deep-Sea Res. Pt. A, 32, 57–84, https://doi.org/10.1016/0198-0149(85)90017-2, 1985.
Landini, W. and Sorbini, C.: Evolutionary dynamics in the fish faunas of the Mediterranean basin during the Plio-Pleistocene, Quaternary Int., 140/141, 64–89, https://doi.org/10.1016/j.quaint.2005.05.019, 2005.
Lanzante, J. R.: Resistant, Robust and Non-Parametric Techniques for the Analysis of Climate Data: Theory and Examples, Including Applications to Historical Radiosonde Station Data, Int. J. Climatol., 16, 1197–1226, https://doi.org/10.1002/(SICI)1097-0088(199611)16:11<1197::AID-JOC89>3.0.CO;2-L, 1996.
Laskar, J., Robutel, P., Joutel, F., Gastineau, M., Correia, A. C. M., and Levrard, B.: A long-term numerical solution for the insolation quantities of the Earth, Astron. Astrophys., 428, 261–285, https://doi.org/10.1051/0004-6361:20041335, 2004.
Leonhard, I. and Agiadi, K.: Addressing challenges in marine conservation with fish otoliths and their death assemblages, Geol. Soc. London Sp. Publ., 529, SP529-2022-132, https://doi.org/10.1144/SP529-2022-132, 2023.
Lopes, M. S., Dufour, E., Sabadini-Santos, E., Gaspar, M. D., Macario, K., Neto, B., Tombret, O., Fiorillo, D., Lemoine, M., Pessoa, L. A., Grouad, S., and Aguilera, O.: Stable isotopic analysis and radiocarbon dating of micropogonias furnieri otoliths (Sciaenidae) from southeastern Brazilian coast: seasonal palaeoenvironmental insight, Radiocarbon, 64, 1109–1137, https://doi.org/10.1017/RDC.2022.57, 2022.
Lyu, J., Kouwenhoven, T. J., Calieri, R., and Lourens, L. J.: Foraminifera of the Faneromeni section (Crete, Greece) reflect the palaeoenvironmental development towards the Messinian salinity crisis, Mar. Micropaleontol., 172, 102107, https://doi.org/10.1016/j.marmicro.2022.102107, 2022.
Mackensen, A. and Schmiedl, G.: Stable carbon isotopes in paleoceanography: atmosphere, oceans, and sediments, Earth-Sci. Rev., 197, 102893, https://doi.org/10.1016/j.earscirev.2019.102893, 2019.
Mancini, A. M., Gennari, R., Lozar, F., Natalicchio, M., Della Porta, G., Bernasconi, D., Pellegrino, L., Dela Pierre, F., Martire, L., and Negri, A.: Sensitivity of the thermohaline circulation during the Messinian: Toward constraining the dynamics of Mediterranean deoxygenation, Deep-Sea Res. Pt. I, 203, 104217, https://doi.org/10.1016/j.dsr.2023.104217, 2024.
Martino, J. C., Doubleday, Z. A., and Gillanders, B. M.: Metabolic effects on carbon isotope biomarkers in fish, Ecol. Indic., 97, 10–16, https://doi.org/10.1016/j.ecolind.2018.10.010, 2019.
Martino, J. C., Doubleday, Z. A., Chung, M.-T., and Gillanders, B. M.: Experimental support towards a metabolic proxy in fish using otolith carbon isotopes, J. Exp. Biol., 223, jeb217091, https://doi.org/10.1242/jeb.217091, 2020.
Mirasole, A., Gillanders, B. M., Reis-Santos, P., Grassa, F., Capasso, G., Scopelliti, G., Mazzola, A., and Vizzini, S.: The influence of high pCO2 on otolith sha pe, chemical and carbon isoto pe com position of six coastal fish s pecies in a Mediterranean shallow CO2 vent, Mar. Biol., 164, 191, https://doi.org/10.1007/s00227-017-3221-y, 2017.
Moissette, P., Cornée, J.-J., Antonarakou, A., Kontakiotis, G., Drinia, H., Koskeridou, E., Tsourou, T., Agiadi, K., and Karakitsios, V.: Palaeoenvironmental changes at the Tortonian/Messinian boundary: A deep-sea sedimentary record of the eastern Mediterranean Sea, Palaeogeogr. Palaeocl., 505, 217–233, https://doi.org/10.1016/j.palaeo.2018.05.046, 2018.
Peterse, F., van der Meer, J., Schouten, S., Weijers, J. W. H., Fierer, N., Jacckson, R. B., Kim, J.-H., and Sinninghe Damsté, J. S.: Revised calibration of the MBT-CBT paleotemperature proxy based on branched tetraether membrane lipids in surface soils, Geochim. Cosmochim. Ac., 96, 215–229, https://doi.org/10.1016/j.gca.2012.08.011, 2012.
Petitjean, Q., Jean, S., Gandar, A., Côte, J., Laffaille, P., and Jacquin, L.: Stress responses in fish: from molecular to evolutionary processes, Sci. Total Env., 684, 371–380, 2019.
R Development Core Team: R: A language and environment for statistical computing, 2023.
Radtke, R. L., Lenz, P., Showers, W., and Moksness, E.: Environmental information stored in otoliths: insights from stable isotopes, Mar. Biol., 127, 161–170, https://doi.org/10.1007/BF00993656, 1996.
Rodionov, S. N.: A sequential algorithm for testing climate regime shifts, Geophys. Res. Lett., 31, L09204, https://doi.org/10.1029/2004GL019448, 2004.
Room, A., Franco-Gaviria, F., and Urrego, D.: rshift: paleoecology and regime shift analysis, https://CRAN.R-project.org/package=rshift (last access: 12 December 2023), 2020.
Sabino, M., Dela Pierre, F., Natalicchio, M., Birgel, D., Gier, S., and Peckmann, J.: The response of water column and sedimentary environments to the advent of the Messinian salinity crisis: insights from an onshore deep-water section (Govone, NW Italy), Geol. Mag., 158, 825–841, https://doi.org/10.1017/s0016756820000874, 2020.
Scheffer, M.: Critical Transitions in Nature and Society, Princeton University Press, 398 pp., ISBN: 9780691122045, 2020.
Schouten, S., Hopmans, E. C., Schefuß, E., and Sinninghe Damsté, J. S.: Distributional variations in marine crenarchaeotal membrane lipids: a new tool for reconstructing ancient sea water temperatures?, Earth Planet. Sc. Lett., 204, 265–274, https://doi.org/10.1016/S0012-821X(02)00979-2, 2002.
Schwarzhans, W., Agiadi, K., and Carnevale, G.: Late Miocene–Early Pliocene evolution of Mediterranean gobies and their environmental and biogeographic significance, Riv. Ital. Paleontol. E, 126, 3, https://doi.org/10.13130/2039-4942/14185, 2020.
Seidenkrantz, M.-S., Kouwenhoven, T. J., Jorissen, F. J., Shackleton, N. J., and van der Zwaan, G. J.: Benthic foraminifera as indicators of changing Mediterranean–Atlantic water exchange in the late Miocene, Mar. Geol., 163, 387–407, https://doi.org/10.1016/S0025-3227(99)00116-4, 2000.
Sierro, F. J., Flores, J. A., Francés, G., Vazquez, A., Utrilla, R., Zamarreño, I., Erlenkeuser, H., and Barcena, M. A.: Orbitally-controlled oscillations in planktic communities and cyclic changes in western Mediterranean hydrography during the Messinian, Palaeogeogr. Palaeocl., 190, 289–316, https://doi.org/10.1016/S0031-0182(02)00611-9, 2003.
Sinninghe Damsté, J. S., Schouten, S., Hopmans, E. C., van Duin, A. C. T., and Geenevasen, J. A. J.: Crenarchaeol: the characteristic core glycerol dibiphytanyl glycerol tetraether membrane lipid of cosmopolitan pelagic crenarchaeota, J. Lipid Res., 43, 1641–1651, https://doi.org/10.1194/jlr.m200148-jlr200, 2002.
Smoliński, S., Denechaud, C., Leesen, G. von, Geffen, A. J., Grønkjær, P., Godiksen, J. A., and Campana, S. E.: Differences in metabolic rate between two Atlantic cod (Gadus morhua) populations estimated with carbon isotopic composition in otoliths, PLOS ONE, 16, e0248711, https://doi.org/10.1371/journal.pone.0248711, 2021.
Solomon, C. T., Weber, P. K., Joseph J Cech, J., Ingram, B. L., Conrad, M. E., Machavaram, M. V., Pogodina, A. R., and Franklin, R. L.: Experimental determination of the sources of otolith carbon and associated isotopic fractionation, Can. J. Fish. Aquat. Sci., 63, 79–89, https://doi.org/10.1139/f05-200, 2006.
Thorrold, S. R., Campana, S. E., Jones, C. M., and Swart, P. K.: Factors determining δ13C and δ18O fractionation in aragonitic otoliths of marine fish, Geochim. Cosmochim. Ac., 61, 2909–2919, 1997.
Trueman, C. N., Chung, M.-T., and Shores, D.: Ecogeochemistry potential in deep time biodiversity illustrated using a modern deep-water case study, Philos. T. R. Soc. B, 371, 20150223, https://doi.org/10.1098/rstb.2015.0223, 2016.
Trueman, C. N., Artetxe-Arrate, I., Kerr, L. A., Meijers, A. J. S., Rooker, J. R., Sivankutty, R., Arrizabalaga, H., Belmonte, A., Deguara, S., Goñi, N., Rodriguez-Marin, E., Dettman, D. L., Santos, M. N., Karakulak, F. S., Tinti, F., Tsukahara, Y., and Fraile, I.: Thermal sensitivity of field metabolic rate predicts differential futures for bluefin tuna juveniles across the Atlantic Ocean, Nat. Commun., 14, 7379, https://doi.org/10.1038/s41467-023-41930-2, 2023.
van der Schee, M., Sierro, F. J., Jiménez-Espejo, F. J., Hernández-Molina, F. J., Flecker, R., Flores, J. A., Acton, G., Gutjahr, M., Grunert, P., García-Gallardo, Á., and Andersen, N.: Evidence of early bottom water current flow after the Messinian Salinity Crisis in the Gulf of Cadiz, Mar. Geol., 380, 315–329, https://doi.org/10.1016/j.margeo.2016.04.005, 2016.
Vasiliev, I., Karakitsios, V., Bouloubassi, I., Agiadi, K., Kontakiotis, G., Antonarakou, A., Triantaphyllou, M., Gogou, A., Kafousia, N., Rafélis, M. de, Zarkogiannis, S., Kaczmar, F., Parinos, C., and Pasadakis, N.: Large Sea Surface Temperature, Salinity, and Productivity-Preservation Changes Preceding the Onset of the Messinian Salinity Crisis in the Eastern Mediterranean Sea, Paleoceanogr. Paleocl., 34, 182–202, https://doi.org/10.1029/2018PA003438, 2019.
Wickham, H., Francois, R., Henry, L., Muller, K., and Vaughan, D.: dplyr: A Grammar of Data Manipulation, https://dplyr.tidyverse.org (last access: 12 December 2023), 2023.
Wurster, C. M. and Patterson, W. P.: Metabolic rate of late Holocene freshwater fish: evidence from δ13C values of otoliths, Paleobiology, 29, 492–505, https://doi.org/10.1666/0094-8373(2003)029<0492:MROLHF>2.0.CO;2, 2003.
Zachariasse, W. J., Kontakiotis, G., Lourens, L. J., and Antonarakou, A.: The Messinian of Agios Myron (Crete, Greece): A key to better understanding of diatomite formation on Gavdos (south of Crete), Palaeogeogr. Palaeocl., 581, 110633, https://doi.org/10.1016/j.palaeo.2021.110633, 2021.