the Creative Commons Attribution 4.0 License.
the Creative Commons Attribution 4.0 License.
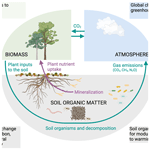
Overview: Global change effects on terrestrial biogeochemistry at the plant–soil interface
Lucia Fuchslueger
Emily Francesca Solly
Alberto Canarini
Albert Carles Brangarí
“Global change” significantly alters organic matter and element cycling, but many of the underlying processes and consequences remain poorly understood. The interface of plants and soil plays a central role, coupling the atmosphere, hydrosphere, biosphere and lithosphere and integrating biological and geochemical processes. The contributions to this special issue address questions on both biotic and abiotic interactions underlying responses of terrestrial biogeochemical cycling to a range of global changes, including increases in atmospheric CO2 concentrations, warming, drought and altered water regimes. In this overview, we synthesize key findings of the contributing empirical, conceptual and modelling-based studies covering responses of plants to elevated CO2; the role of soil organisms in modulating responses to warming; impacts of global change on soil organic carbon, nitrogen, and mineral nutrient availability; and the influence of altered water-table depth caused by global change on greenhouse gas emissions. The showcased studies were conducted in regions from the Arctic to the tropics and highlight the manifold impacts of global change on various ecosystem components controlling biogeochemical processes occurring at the plant–soil interface. This multi-ecosystem interdisciplinary understanding is crucial for deciphering feedbacks of terrestrial ecosystems to the climate system.
- Article
(989 KB) - Full-text XML
- BibTeX
- EndNote
Global change poses manifold threats to different components of ecosystems and can significantly impact biogeochemical cycling. Increased atmospheric carbon dioxide (CO2) concentrations, primarily driven by human activity, have strongly altered the Earth's energy budget, resulting in unprecedented global warming and shifts in precipitation patterns. The last decade has witnessed the hottest years on record, accompanied by intensified droughts and an increased frequency of extreme weather events (Intergovernmental Panel on Climate Change, 2023). These strong environmental changes significantly impact terrestrial ecosystems through a complex interplay of direct and indirect effects (Bardgett et al., 2008). Elevated CO2 and temperatures can directly stimulate plant growth but also trigger a series of indirect effects (e.g. changed carbon supply for soil microbial communities) so that subsequent short- and long-term impacts on soil organic carbon (SOC) sequestration and total ecosystem carbon budgets remain unclear. Likewise, changing moisture and temperature can directly affect soil microorganism activity and SOC decomposition (Kim et al., 2012; Moyano et al., 2013; Schimel, 2018; Verbrigghe et al., 2022) but also indirectly influence soil nutrient availability, SOC stocks and plant feedbacks (Melillo et al., 2011). The magnitude and direction of responses to different global change factors depend largely on the ability of living organisms to tolerate or adapt to changed conditions (Allison, 2023). As greenhouse gas emissions into the atmosphere are driven by autotrophic and heterotrophic organisms, understanding the resistance and resilience of all components in an ecosystem to changing conditions is integral for assessing potential feedbacks on the climate system. Overall, the plant–soil interface stands out as a hotspot for assessing these feedbacks, as it couples the atmosphere, biosphere and lithosphere and integrates biological and geochemical processes across multiple trophic levels. Modelling approaches can help to understand this complex system and to predict future ecosystem feedbacks to environmental and climate change, particularly through altered biogeochemical cycling (e.g. Medlyn et al., 2015). However, the efficacy of models relies on a robust mechanistic understanding of the underlying physiological and biogeochemical processes.
The contributions to this special issue, “Global change effects on terrestrial biogeochemistry at the plant–soil interface”, could be categorized into four functional topics. Studies investigated (1) the ability of plants to regulate resource optimization in response to elevated atmospheric CO2; (2) the role of soil organisms as agents for modulating responses to warming, with a strong focus in particular on arctic ecosystems; (3) the effects of climate change on soil organic carbon, nitrogen and mineral nutrient availability; and (4) the impact of changing soil water tables on greenhouse gas emissions from boreal to tropical ecosystems (Fig. 1). The insights presented in this special issue represent an important step toward comprehensively understanding the susceptibility of Earth's ecosystems to global change.
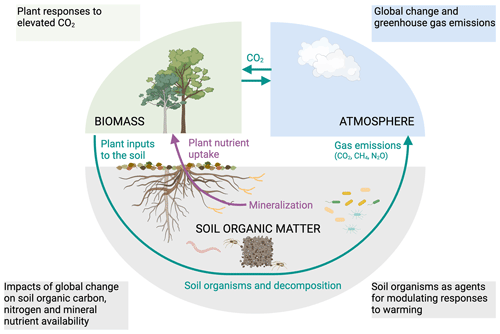
Figure 1Global change affects all ecosystems, with interconnected feedbacks and links across its different components. For instance, altered environmental conditions have the potential to influence plant growth and plant carbon allocation to maintain resource and nutrient acquisition, consequently changing both the quality and the quantity of carbon inputs to the soil. Similarly, global change can modify the community structure and activity of soil organisms, and subsequently soil organic matter decomposition and mineral weathering, overall affecting soil nutrient availability. Finally, greenhouse gas emissions into the atmosphere respond sensitively to global change, thereby feeding back on the climate system and affecting all other ecosystem components. The figure was created with BioRender.com.
Plants are a major component in terrestrial carbon cycling, taking up CO2 from the atmosphere and converting it into biomass. However, the extent and mechanisms of plant resistance to and recovery from global change depend on many biotic and abiotic factors. For instance, responses can strongly differ between vegetation types (e.g. crop plants vs. forest stands), physiological traits (e.g. wet- vs. drought-adapted species), and plant ecological strategies and flexibility (e.g. specialists vs. generalists) and depend on the magnitude of the environmental change. In general, the exposure to changing environmental conditions, such as increased CO2 concentrations, warming or drought episodes, can induce both short- (e.g. physiological) and longer-term (e.g. vegetation composition change) responses, with implications for biogeochemical cycling at the ecosystem level. Trade-offs between plant resource acquisition (water use and nutrient uptake) and biomass growth can in particular yield a substantial variation in climate change feedback. Using different modelling approaches, Manzoni et al. (2022) explored whether elevated atmospheric CO2 concentrations could lead to reduced transpiration rates from vegetation, potentially reducing water loss and facilitating ecosystem “greening” in dry regions. However, they found small variations in canopy-level transpiration with rising atmospheric CO2 due to the close coordination of physiological (reduced stomatal conductance) and morphological (increased leaf area) characteristics of plants to maximize water use efficiency. Yet, the responses to elevated atmospheric CO2 are also closely modulated by nutrient resource availability (Walker et al., 2021). In a free-air CO2-enrichment field experiment in a mature eucalyptus forest, Pihlblad et al. (2023) observed a faster nutrient recycling, particularly of available phosphorus forms, but not an additional availability of nutrients through soil organic matter (SOM) decomposition. In the long term, this could lead to plant nutrient limitations and reduce the potential of mature forest ecosystems to fix carbon as biomass in response to elevated CO2. Both studies (Manzoni et al., 2022; Pihlblad et al., 2023) highlight the importance of accounting for resource limitations of plants in response to elevated CO2; however, they also emphasize that adaptions can profoundly differ between ecosystems or plant communities. It is thus essential to improve our understanding of the variability in short- and long-term plant responses to accurately predict ecosystem behaviour under climate change.
Global warming is one of the most pronounced climate change factors driving large ecosystem transformations. This is particularly evident in cold ecosystems, with the Arctic containing 50 %–70 % of the total carbon stock (Hugelius et al., 2014) being crucial for terrestrial carbon cycling. Predictions suggest that arctic regions will experience greater temperature increases compared to other ecosystems, underscoring the importance of understanding the unique challenges faced by these regions (Intergovernmental Panel on Climate Change, 2023). Soil (micro-)organisms are key regulators of matter and energy cycling between the soil and the atmosphere via their catabolic and anabolic processes (Ågren and Wetterstedt, 2007). Given the temperature sensitivity of enzymatic reactions, warming mostly has a stimulating effect on microbial activity in cold ecosystems, which can potentially destabilize carbon stored in Arctic soils and contribute to further climate change. Thus, investigating warming effects on soil microbes in cold ecosystems is particularly relevant. Rijkers et al. (2023) identified strong differences in the temperature adaptation of microbial communities in soils from the subarctic to the high Arctic region. They predicted that summer warming can increase the community-level optimal growth temperature but found no evidence supporting temperature adaptation of the soil bacterial community structure. SOM decomposition is strongly affected not only by microbial communities, but also by the presence of soil fauna. Blume-Werry et al. (2023) proposed that, in Arctic ecosystems, climate warming can create new niches for soil organisms migrating both laterally and vertically belowground. This may introduce new soil- and fauna-associated functions that can strongly affect decomposition processes and challenges the way we simulate carbon losses under climate change scenarios. Indeed, Monteux et al. (2022) showed that introducing a model microarthropod (Collembola Folsomia candida) species can influence the bacterial diversity and prime respiration of the native soil community. These studies highlight the importance of investigating and understanding biotic interactions in soil as well as the need to further explore potential additive or interactive effects of warming and other climate change factors on the soil food web.
Warming is expected to boost net primary production and potentially enhance ecosystem carbon sequestration (Melillo et al., 2017). However, warming also has a stimulating effect on microbial activity and SOM turnover (e.g. Classen et al., 2015; Davidson and Janssens, 2006), which can lead to significant decreases in soil organic carbon stocks (Verbrigghe et al., 2022). Soil microbes release enzymes transforming SOM into available carbon and nutrients, ultimately releasing CO2 via intracellular metabolism (Allison et al., 2007). Many studies have examined the temperature sensitivity of SOM decomposition via CO2 emission or mass loss of carbon substrate (e.g. Kirwan et al., 2014; Wang et al., 2013). However, differentiating contributions by extracellular and intracellular processes are key for a mechanistic understanding of warming. Adekanmbi et al. (2023) investigated these sensitivities and found that depolymerization of high-molecular-weight carbon was more sensitive to temperature changes at higher temperatures, whereas the respiration of the generated monomers was more sensitive to temperature changes at moderate temperatures. Hence, this study suggested that global warming may shift the importance from extracellular depolymerization to intracellular metabolic processes as the rate-limiting step of SOM mineralization.
The stimulating effect of warming on plant productivity can be constrained by low nutrient availability, for instance by nitrogen (Melillo et al., 2011) and cations (Jonard et al., 2015). In cold ecosystems, the addition of nutrients has been found to stimulate plant aboveground growth (Bergh et al., 2008) but to reduce the allocation of carbon to roots and associated microorganisms (Wallander et al., 2011). Almeida et al. (2022) investigated the impact of forest fertilization on the soil ectomycorrhizal fungal community in an experimental spruce forest in Norway. The authors observed changes in the fungal community composition after 3 years of N additions, potentially affecting hydrophobic SOM formation and reducing soil carbon sequestration. Myers-Pigg et al. (2023) explored the net response of SOC composition to climate change, assessing its diagenetic state based on lignin-phenol biomarkers. Their biomarker-based approach revealed a coupled increase in carbon and N cycling rates, which may support forest productivity and help maintain SOC stocks. Finally, in a modelling study using the ForSAFE model on different forest systems distributed across Sweden, Kronnäs et al. (2023) found that warming and longer growing seasons for plants could enhance soil weathering rates, inducing substantial changes in soil texture and mineral nutrient stocks. This emphasizes the importance of including soil texture and seasonal climate variations for predicting forest responses to climate change in future studies and highlights that understanding the interactions between primary production, carbon sequestration and limiting factors is crucial for predicting and managing the impacts of climate change on terrestrial ecosystems.
One of the most effective and accessible approaches for evaluating effects of global changes on soil biogeochemical cycling is the measurement of gaseous emissions, such as carbon dioxide (CO2), methane (CH4) and nitrous oxide (N2O). From soils, CO2 is released by plant roots and SOM decomposition by soil organisms under aerobic conditions (autotrophic and heterotrophic respiration), CH4 under anaerobic conditions by methanogenic microorganisms, and N2O through nitrification and denitrification processes in soils. All gas fluxes are highly responsive to changes in soil water conditions controlling whether ecosystems are sources or sinks for greenhouse gases (Jungkunst and Fiedler, 2007). Zhao and Zhuang (2023) modelled greenhouse gas flux responses of northern peatlands in response to warming, revealing increases in both CO2 and CH4 emissions due to deeper water-table levels and enhanced permafrost thawing. They demonstrated that warming had a limited impact on net primary production due to shifts in plant functional types and nitrogen limitations, which could turn ecosystems into carbon sources. Their estimates proposed that this shift might occur as early as 2050, notably earlier than previously anticipated (e.g. Gallego-Sala et al., 2018; McGuire et al., 2018). Zhang et al. (2022) further explored the effect of water-table levels and nitrogen availability on greenhouse gas emissions in alpine peatlands by conducting a mesocosm experiment. They found that a moderate increase in nitrogen deposition and rising water-table levels stimulated CH4 emissions, whereas only nitrogen deposition consistently increased N2O emissions. Finally, in a field study, Castellón et al. (2022) quantified the emissions of CO2 and CH4 in a natural mangrove forest experiencing daily and seasonal variations in water-table depths. Higher-topography areas generally exhibited increased CO2 emissions and decreased CH4 emissions, likely induced by reduced flooding frequency. The study highlighted the role of microtopography, seasonality and soil physicochemical properties in influencing greenhouse gas emissions. Changes in water-table depth thus exert a major influence on greenhouse gas emissions, creating feedback loops that further impact the climate system.
The studies contributing to this special issue, “Global change effects on terrestrial biogeochemistry at the plant–soil interface”, reveal pronounced impacts of global change on biogeochemical processes driven by different trophic levels, extending from autotrophic plants to heterotrophic soil fauna and microbial populations. Both empirical and modelling studies show that global change can affect small soil-scale fluxes, as well as large ecosystem-scale fluxes of C and nutrients, inducing substantial feedback loops on the climate system. The diverse methodologies employed in the contributing studies highlight that a combination of techniques is essential for a mechanistic and holistic understanding of the complex interactions at the plant–soil interface. Future research should then prioritize the combination of approaches and focus on regions hosting the largest organic matter pools and exhibiting the highest sensitivity to climate. This enhanced understanding of multi-scale across-temporal responses is crucial for improving predictions of the resistance and resilience of terrestrial ecosystems to global change, informing effective strategies for mitigating and adapting to future environmental challenges.
No data sets were used in this article.
LF, ES, AC and AB contributed to the conceptualization, as well as to writing the original draft, and to the review and editing of the final version of this manuscript.
At least one of the (co-)authors is a member of the editorial board of Biogeosciences. The peer-review process was guided by an independent editor, and the authors also have no other competing interests to declare.
Publisher’s note: Copernicus Publications remains neutral with regard to jurisdictional claims made in the text, published maps, institutional affiliations, or any other geographical representation in this paper. While Copernicus Publications makes every effort to include appropriate place names, the final responsibility lies with the authors.
This article is part of the special issue “Global change effects on terrestrial biogeochemistry at the plant–soil interface”. It is not associated with a conference.
We want to thank Michael Bahn for initiating and supporting this special issue.
This research has been supported by the Horizon 2020 research and innovation programme (grant no. REWIRE 847693), the Schweizerischer Nationalfonds zur Förderung der Wissenschaftlichen Forschung (grant no. Ambizione PZ00P2_180030), the Japan Society for the Promotion of Science (Postdoctoral Fellowships for Research in Japan (Standard) project), and the Svenska Forskningsrådet Formas (grant no. 2022-01478).
This paper was edited by Jianming Xu and reviewed by three anonymous referees.
Adekanmbi, A. A., Dale, L., Shaw, L., and Sizmur, T.: Differential temperature sensitivity of intracellular metabolic processes and extracellular soil enzyme activities, Biogeosciences, 20, 2207–2219, https://doi.org/10.5194/bg-20-2207-2023, 2023.
Ågren, G. I. and Wetterstedt, J. Å. M.: What determines the temperature response of soil organic matter decomposition?, Soil Biol. Biochem., 39, 1794–1798, https://doi.org/10.1016/J.SOILBIO.2007.02.007, 2007.
Allison, S. D.: Microbial drought resistance may destabilize soil carbon, Trends Microbiol., 31, 780–787, https://doi.org/10.1016/j.tim.2023.03.002, 2023.
Allison, V. J., Condron, L. M., Peltzer, D. A., Richardson, S. J., and Turner, B. L.: Changes in enzyme activities and soil microbial community composition along carbon and nutrient gradients at the Franz Josef chronosequence, New Zealand, Soil Biol. Biochem., 39, 1770–1781, https://doi.org/10.1016/j.soilbio.2007.02.006, 2007.
Almeida, J. P., Rosenstock, N. P., Woche, S. K., Guggenberger, G., and Wallander, H.: Nitrophobic ectomycorrhizal fungi are associated with enhanced hydrophobicity of soil organic matter in a Norway spruce forest, Biogeosciences, 19, 3713–3726, https://doi.org/10.5194/bg-19-3713-2022, 2022.
Bardgett, R. D., Freeman, C., and Ostle, N. J.: Microbial contributions to climate change through carbon cycle feedbacks, ISME J., 2, 805–814, 2008.
Bergh, J., Nilsson, U., Grip, H., Hedwall, P. O., and Lundmark, T.: Effects of frequency of fertilisation on production, foliar chemistry and nutrient leaching in young Norway spruce stands in Sweden, Silva Fenn., 42, 721–733, https://doi.org/10.14214/SF.225, 2008.
Blume-Werry, G., Klaminder, J., Krab, E. J., and Monteux, S.: Ideas and perspectives: Alleviation of functional limitations by soil organisms is key to climate feedbacks from arctic soils, Biogeosciences, 20, 1979–1990, https://doi.org/10.5194/bg-20-1979-2023, 2023.
Castellón, S. E. M., Cattanio, J. H., Berrêdo, J. F., Rollnic, M., Ruivo, M. D. L., and Noriega, C.: Greenhouse gas fluxes in mangrove forest soil in an Amazon estuary, Biogeosciences, 19, 5483–5497, https://doi.org/10.5194/bg-19-5483-2022, 2022.
Classen, A. T., Sundqvist, M. K., Henning, J. A., Newman, G. S., Moore, J. A. M., Cregger, M. A., Moorhead, L. C., and Patterson, C. M.: Direct and indirect effects of climate change on soil microbial and soil microbial-plant interactions: What lies ahead?, Ecosphere, 6, 30, https://doi.org/10.1890/ES15-00217.1, 2015.
Davidson, E. A. and Janssens, I. A.: Temperature sensitivity of soil carbon decomposition and feedbacks to climate change, Nature, 440, 165–173, https://doi.org/10.1038/nature04514, 2006.
Gallego-Sala, A. V., Charman, D. J., Brewer, S., Page, S. E., Prentice, I. C., Friedlingstein, P., Moreton, S., Amesbury, M. J., Beilman, D. W., Björck, S., Blyakharchuk, T., Bochicchio, C., Booth, R. K., Bunbury, J., Camill, P., Carless, D., Chimner, R. A., Clifford, M., Cressey, E., Courtney-Mustaphi, C., De Vleeschouwer, F., de Jong, R., Fialkiewicz-Koziel, B., Finkelstein, S. A., Garneau, M., Githumbi, E., Hribjlan, J., Holmquist, J., Hughes, P. D. M., Jones, C., Jones, M. C., Karofeld, E., Klein, E. S., Kokfelt, U., Korhola, A., Lacourse, T., Le Roux, G., Lamentowicz, M., Large, D., Lavoie, M., Loisel, J., Mackay, H., MacDonald, G. M., Makila, M., Magnan, G., Marchant, R., Marcisz, K., Martínez Cortizas, A., Massa, C., Mathijssen, P., Mauquoy, D., Mighall, T., Mitchell, F. J. G., Moss, P., Nichols, J., Oksanen, P. O., Orme, L., Packalen, M. S., Robinson, S., Roland, T. P., Sanderson, N. K., Sannel, A. B. K., Silva-Sánchez, N., Steinberg, N., Swindles, G. T., Turner, T. E., Uglow, J., Väliranta, M., van Bellen, S., van der Linden, M., van Geel, B., Wang, G., Yu, Z., Zaragoza-Castells, J., and Zhao, Y.: Latitudinal limits to the predicted increase of the peatland carbon sink with warming, Nat. Clim. Change, 810, 907–913, https://doi.org/10.1038/S41558-018-0271-1, 2018.
Hugelius, G., Strauss, J., Zubrzycki, S., Harden, J. W., Schuur, E. A. G., Ping, C. L., Schirrmeister, L., Grosse, G., Michaelson, G. J., Koven, C. D., O'Donnell, J. A., Elberling, B., Mishra, U., Camill, P., Yu, Z., Palmtag, J., and Kuhry, P.: Estimated stocks of circumpolar permafrost carbon with quantified uncertainty ranges and identified data gaps, Biogeosciences, 11, 6573–6593, https://doi.org/10.5194/bg-11-6573-2014, 2014.
Intergovernmental Panel on Climate Change: Climate Change 2021 – The Physical Science Basis Working Group I Contribution to the Sixth Assessment Report of the Intergovernmental Panel on Climate Change, Cambridge University Press, 287–422, https://doi.org/10.1017/9781009157896.004, 2023
Jonard, M., Fürst, A., Verstraeten, A., Thimonier, A., Timmermann, V., Potočić, N., Waldner, P., Benham, S., Hansen, K., Merilä, P., Ponette, Q., de la Cruz, A. C., Roskams, P., Nicolas, M., Croisé, L., Ingerslev, M., Matteucci, G., Decinti, B., Bascietto, M., and Rautio, P.: Tree mineral nutrition is deteriorating in Europe, Glob. Change Biol., 21, 418–430, https://doi.org/10.1111/GCB.12657, 2015.
Jungkunst, H. F. and Fiedler, S.: Latitudinal differentiated water table control of carbon dioxide, methane and nitrous oxide fluxes from hydromorphic soils: feedbacks to climate change, Glob. Change Biol., 13, 2668–2683, https://doi.org/10.1111/J.1365-2486.2007.01459.X, 2007.
Kim, D. G., Vargas, R., Bond-Lamberty, B., and Turetsky, M. R.: Effects of soil rewetting and thawing on soil gas fluxes: A review of current literature and suggestions for future research, Biogeosciences, 9, 2459–2483, https://doi.org/10.5194/bg-9-2459-2012, 2012.
Kirwan, M. L., Guntenspergen, G. R., and Langley, J. A.: Temperature sensitivity of organic-matter decay in tidal marshes, Biogeosciences, 11, 4801–4808, https://doi.org/10.5194/bg-11-4801-2014, 2014.
Kronnäs, V., Lucander, K., Zanchi, G., Stadlinger, N., Belyazid, S., and Akselsson, C.: Effect of droughts and climate change on future soil weathering rates in Sweden, Biogeosciences, 20, 1879–1899, https://doi.org/10.5194/bg-20-1879-2023, 2023.
Manzoni, S., Fatichi, S., Feng, X., Katul, G. G., Way, D., and Vico, G.: Consistent responses of vegetation gas exchange to elevated atmospheric CO2 emerge from heuristic and optimization models, Biogeosciences, 19, 4387–4414, https://doi.org/10.5194/bg-19-4387-2022, 2022.
McGuire, A. D., Lawrence, D. M., Koven, C., Clein, J. S., Burke, E., Chen, G., Jafarov, E., MacDougall, A. H., Marchenko, S., Nicolsky, D., Peng, S., Rinke, A., Ciais, P., Gouttevin, I., Hayes, D. J., Ji, D., Krinner, G., Moore, J. C., Romanovsky, V., Schädel, C., Schaefer, K., Schuur, E. A. G., and Zhuang, Q.: Dependence of the evolution of carbon dynamics in the northern permafrost region on the trajectory of climate change, P. Natl. Acad. Sci. USA, 115, 3882–3887, 2018.
Medlyn, B. E., Zaehle, S., De Kauwe, M. G., Walker, A. P., Dietze, M. C., Hanson, P. J., Hickler, T., Jain, A. K., Luo, Y., Parton, W., Prentice, I. C., Thornton, P. E., Wang, S., Wang, Y.-P., Weng, E., Iversen, C. M., McCarthy, H. R., Warren, J. M., Oren, R., and Norby, R. J.: Using ecosystem experiments to improve vegetation models, Nat. Clim. Change, 5, 528–534, https://doi.org/10.1038/nclimate2621, 2015.
Melillo, J. M., Butler, S., Johnson, J., Mohan, J., Steudler, P., Lux, H., Burrows, E., Bowles, F., Smith, R., Scott, L., Vario, C., Hill, T., Burton, A., Zhouj, Y. M., and Tang, J.: Soil warming, carbon-nitrogen interactions, and forest carbon budgets, P. Natl. Acad. Sci. USA, 108, 9508–9512, https://doi.org/10.1073/pnas.1018189108, 2011.
Monteux, S., Mariën, J., and Krab, E. J.: Dispersal of bacteria and stimulation of permafrost decomposition by Collembola, Biogeosciences, 19, 4089–4105, https://doi.org/10.5194/bg-19-4089-2022, 2022.
Moyano, F. E., Manzoni, S., and Chenu, C.: Responses of soil heterotrophic respiration to moisture availability: An exploration of processes and models, Soil Biol. Biochem., 59, 72–85, https://doi.org/10.1016/j.soilbio.2013.01.002, 2013.
Myers-Pigg, A. N., Kaiser, K., Benner, R., and Ziegler, S. E.: Soil organic matter diagenetic state informs boreal forest ecosystem feedbacks to climate change, Biogeosciences, 20, 489–503, https://doi.org/10.5194/bg-20-489-2023, 2023.
Pihlblad, J., Andresen, L. C., Macdonald, C. A., Ellsworth, D. S., and Carrillo, Y.: The influence of elevated CO2 and soil depth on rhizosphere activity and nutrient availability in a mature Eucalyptus woodland, Biogeosciences, 20, 505–521, https://doi.org/10.5194/bg-20-505-2023, 2023.
Rijkers, R., Dekker, M., Aerts, R., and Weedon, J. T.: Maximum summer temperatures predict the temperature adaptation of Arctic soil bacterial communities, Biogeosciences, 20, 767–780, https://doi.org/10.5194/bg-20-767-2023, 2023.
Schimel, J. P.: Life in Dry Soils: Effects of Drought on Soil Microbial Communities and Processes, Annu. Rev. Ecol. Evol. Syst., 49, 409–432, https://doi.org/10.1146/annurev-ecolsys-110617-062614, 2018.
Verbrigghe, N., Leblans, N. I. W., Sigurdsson, B. D., Vicca, S., Fang, C., Fuchslueger, L., Soong, J. L., Weedon, J. T., Poeplau, C., Ariza-Carricondo, C., Wallander, H., and Janssens, I. A.: Soil carbon loss in warmed subarctic grasslands is rapid and restricted to topsoil, Biogeosciences, 19, 3381–3393, https://doi.org/10.5194/bg-19-3381-2022, 2022.
Walker, A. P., De Kauwe, M. G., Bastos, A., Belmecheri, S., Georgiou, K., Keeling, R. F., McMahon, S. M., Medlyn, B. E., Moore, D. J. P., Norby, R. J., Zaehle, S., Anderson-Teixeira, K. J., Battipaglia, G., Brienen, R. J. W., Cabugao, K. G., Cailleret, M., Campbell, E., Canadell, J. G., Ciais, P., Craig, M. E., Ellsworth, D. S., Farquhar, G. D., Fatichi, S., Fisher, J. B., Frank, D. C., Graven, H., Gu, L., Haverd, V., Heilman, K., Heimann, M., Hungate, B. A., Iversen, C. M., Joos, F., Jiang, M., Keenan, T. F., Knauer, J., Körner, C., Leshyk, V. O., Leuzinger, S., Liu, Y., MacBean, N., Malhi, Y., McVicar, T. R., Penuelas, J., Pongratz, J., Powell, A. S., Riutta, T., Sabot, M. E. B., Schleucher, J., Sitch, S., Smith, W. K., Sulman, B., Taylor, B., Terrer, C., Torn, M. S., Treseder, K. K., Trugman, A. T., Trumbore, S. E., van Mantgem, P. J., Voelker, S. L., Whelan, M. E., and Zuidema, P. A.: Integrating the evidence for a terrestrial carbon sink caused by increasing atmospheric CO2, New Phytol., 229, 2413–2445, https://doi.org/10.1111/nph.16866, 2021.
Wallander, H., Ekblad, A., and Bergh, J.: Growth and carbon sequestration by ectomycorrhizal fungi in intensively fertilized Norway spruce forests, Forest Ecol. Manage., 262, 999–1007, https://doi.org/10.1016/J.FORECO.2011.05.035, 2011.
Wang, Y., Chen, B. C., Wieder, W. R., Leite, M., Medlyn, B. E., Rasmussen, M., Smith, M. J., Agusto, F. B., Hoffman, F. M., and Luo, Y. Q.: Oscillatory behavior of two nonlinear microbial models of soil carbon decomposition carbon decomposition, Biogeosciences, 11, 1817–1831, https://doi.org/10.5194/bg-11-1817-2014, 2013.
Zhang, W., Hu, Z., Audet, J., Davidson, T. A., Kang, E., Kang, X., Li, Y., Zhang, X., and Wang, J.: Effects of water table level and nitrogen deposition on methane and nitrous oxide emissions in an alpine peatland, Biogeosciences, 19, 5187–5197, https://doi.org/10.5194/bg-19-5187-2022, 2022.
Zhao, B. and Zhuang, Q.: Peatlands and their carbon dynamics in northern high latitudes from 1990 to 2300: a process-based biogeochemistry model analysis, Biogeosciences, 20, 251–270, https://doi.org/10.5194/bg-20-251-2023, 2023.
- Abstract
- Introduction
- Plant responses to elevated CO2
- Soil organisms as agents for modulating responses to warming
- Impacts of global change on soil organic carbon, nitrogen and mineral nutrient availability
- Influence of altered water-table depth caused by global change on greenhouse gas emissions
- Concluding remarks
- Data availability
- Author contributions
- Competing interests
- Disclaimer
- Special issue statement
- Acknowledgements
- Financial support
- Review statement
- References
- Abstract
- Introduction
- Plant responses to elevated CO2
- Soil organisms as agents for modulating responses to warming
- Impacts of global change on soil organic carbon, nitrogen and mineral nutrient availability
- Influence of altered water-table depth caused by global change on greenhouse gas emissions
- Concluding remarks
- Data availability
- Author contributions
- Competing interests
- Disclaimer
- Special issue statement
- Acknowledgements
- Financial support
- Review statement
- References