the Creative Commons Attribution 4.0 License.
the Creative Commons Attribution 4.0 License.
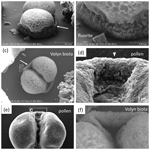
Reply to Head's comment on “The Volyn biota (Ukraine) – indications of 1.5 Gyr old eukaryotes in 3D preservation, a spotlight on the `boring billion' ” by Franz et al. (2023)
Gerhard Franz
Vladimir Khomenko
Peter Lyckberg
Vsevolod Chournousenko
Ulrich Struck
Head et al. (2024) emphasize the importance of the Volyn biota for evolution, especially in the so-called “boring billion”, in a detailed outline about the biological and geological context. However, they question the idea that the Volyn biota represent Precambrian fossils and instead argue that they contain young contaminants of “museum dust”. In addition, they question their biotic origin. We present here a detailed discussion of their points of concern based on presented data, including some additional information. Their points of concern were as follows:
-
One object, shown by Franz et al. (2023a), is similar to a pollen grain, and another object is similar to trichomes; we show indications of fossilization and summarize our arguments against “museum dust”.
-
They question the fossil character of the biota and argue for a biomineralization; we show that the biomineralization in trichomes is distinct from the mineralization of the biota.
-
They missed information about the internal structure; we repeat the presented information about the internal structure in more detail, which is also indicative of fossil material and inconsistent with trichomes.
-
They argue that we did not compare the biota with recent fungi via infrared spectroscopy; since the biota experienced temperatures near 300 °C, we think that a comparison with thermally degraded chitosan is more appropriate.
-
They question the use of strongly negative δ13C as an argument for biotic origin, but we show that, in combination with positive δ15N values and the geological situation, a biotic origin is more likely than abiotic synthesis.
In addition, Popov (2023) questioned the age of the Volyn biota, which we postulated as between approximately 1.5 and 1.7 Ga. He argues that the fossils could be Phanerozoic. We will also outline our arguments for the minimum age of 1.5 Ga.
- Article
(10397 KB) - Full-text XML
- Comment
- BibTeX
- EndNote
We thank Head et al. (2024) for stimulating the discussion about the Volyn biota. They question the fact that these are fossils and instead argue that at least some of them are young contaminants by plant hairs and pollen. This could have occurred during storage in the form of what they called “museum dust” or during sampling. Furthermore, they question the biogenicity and argue for an abiotic origin. We appreciate their comment because this question of contamination was not raised before, either in our papers from 2017, 2022, and 2023 or in any of the previous publications about kerite from Volyn.
1.1 Review of kerite formation
The words “kerite” and “kerogen” are derived from the Greek word “κηρς” (kērós), meaning “wax”. Kerogen is the insoluble residue of organic matter in sedimentary rocks that is left after its treatment by common organic solvents (Durand, 1980); the soluble fraction is called bitumen. With increasing temperature, solid oil bitumens range from asphaltite over kerite to anthraxolite. Kerite (high and low) has a density of 1.05–1.3; an atomic C (C + H + N + O) of 0.39–0.62; an H C (at) of 0.59–1.44; and a composition (in wt %) of 69–91 C, 4.5–9 H, 0.5–2 N, and 1–12 O (Moroz et al., 1998). Moroz et al. (1998) and Ciarniello et al. (2019) also regarded kerite as an analog for extraterrestrial organic matter.
Kerite from the Volyn occurrence was first described as an abiogenic material (Ginzburg et al., 1987; Luk'yanova et al., 1992) and as a prime example of protein synthesis by inorganic processes (Yushkin, 1996). Its composition is given as (wt %) C 76.51, H 5.02, O + N 17.46, S 0.42, Cl 0.24, Fe 0.06, and Cu 0.15 with a chemical formula C491H386O87S(N). Among many minor impurities, Yushkin (1996) lists Si, Al, Na, K, and Mg and mentions very light isotopic δ13C of −40 ‰. However, kerite from Volyn was later reinterpreted as fossilized cyanobacteria (Gorlenko et al., 2000; Zhmur, 2003), transported in a geyser system from ponds on the surface of the Earth into their depths, where it is found now in cavities of pegmatitic rocks.
1.2 Discussion points
The main points of discussion are (i) the possible contamination during or after sampling; (ii) the type of the kerite organic matter, including its chemical composition and its structure; (iii) the morphology of the different objects, as observed under the scanning electron microscope (SEM), and a comparison to what Head et al. (2024) described as contamination; (iv) the available age constraints of the fossils and further possibilities for dating; and, finally, (v) a summary with open questions.
The following information is based on logbooks from the mine used by Vsevolod Chournousenko, a mine geologist in the area since 1990, who also collected the material for our study together with Peter Lyckberg. The samples of kerite occur in situ underground in several, but not all, shafts of the Volyn pegmatite district. Within the large miarolitic cavities (“chambers” in the original literature), kerite is also found in the mineral matrix (feldspar, mica, clay minerals) on the floor of the pegmatite and also hangs from the walls or the ceiling. However, kerite in visible amounts is not preserved in most chambers. It was either destroyed during cleaning and gemstone extraction, or it was already collected. In those chambers which were explored by drilling, it was completely destroyed by drilling fluids mixed with clay that covered the entire ground. Large, well-preserved amounts of kerite were found only in new pockets opened by miners underground without drilling. In January 2013, kerite was found (Peter Lyckberg) in a 5 mm wide zone around topaz crystals on the wall of a 15 m tall chamber in shaft 3. Kerite was observed growing at the base of dark-lilac to black fluorite crystals and in larger fiber masses around large topaz crystals; in larger fiber masses in clay along the lower walls; and in large masses on well-crystallized feldspars, mica, quartz, and topaz high on the walls in two chambers.
Early descriptions in the drilling logbooks mention in some cases that chambers were full of kerite, up to 25 kg of kerite in the rather small pegmatite body from shaft 3, which has access to several pegmatite bodies (consistent with reports in the literature, e.g., Ginzburg et al., 1987). Material from this shaft was distributed to museums in the former Soviet Union. The chambers are now at a depth of up to 96 m; some were mined in open pits, but the crystallization depth of the pegmatites was at a depth corresponding to 2–3 kbar. Thus, significant uplift had occurred since intrusion at 1.76 Ga, but there is no indication from the geological literature of the area that the chambers were directly on or beneath the surface and buried again later. Therefore, contamination within the chambers by plant roots going down to 96 m is less likely. In any case, we have no doubt that kerite is part of the deep biosphere. Most trichomes (plant hairs) are known from plants on the surface, not from the deep biosphere.
Samples kerite 1 to kerite 7 were sampled underground by Peter Lyckberg and Vsevolod Chournousenko, put into firmly closed plastic sample bags (double ones with labels on the outer ones), transported first to Luxembourg, and then sent to Berlin. There was no need to separate kerite from the rocks and from the soil, as the material could be picked up. Sample bags were opened only in the electron microscopy laboratory of TU Berlin, which is a special building for electron microscopy with the appropriate arrangements to prevent contamination by dust. All rooms are equipped with airlocks for climatization, and, in addition, water-cooled ceilings minimize airstream and dust movement in the rooms. Samples were prepared in an exhaust hood. Of course, we cannot completely rule out that some objects are contaminants, but the overwhelming majority of objects on the aluminum sample holders for SEM are original as recovered from underground. The only kerite sample which could have been contaminated in a museum is our sample “kerite 0”.
The beryl crystal sample V2008 was collected from the mine tailings in 2008 by Gerhard Franz and stored at TU Berlin in a common wooden rock cabinet. For this sample, contamination on the mine tailings or later is possible.
The breccia with the beryl pseudomorph was also collected from the mine tailings in 2008 by Gerhard Franz, stored at TU Berlin in a common wooden rock cabinet, and consolidated with epoxy for preparation of thin sections and polished blocks for the Ar–Ar determination of muscovite.
3.1 Organic matter in the beryl pseudomorph
We start the discussion with the OM in the pseudomorph. For this, a later contamination can safely be excluded, as it was discovered in thin sections. It is closely surrounded and intergrown with C–H-bearing opal that is brown in thin sections but macroscopically black (Franz et al., 2017; see their Fig. 6). The chemical composition of the OM is characterized by high amounts of Zr, Y, Sc, and REE. These high-field-strength elements (HFSEs) are positively correlated with O, and increasing O contents are correlated with decreasing C contents. The N content is between 2 at % and 4 at %, much lower than the original kerite (see their Fig. 7), which has nearly 8 at % to 9 at % (Ginzburg et al., 1987; Yushkin, 1996). Mobilization of HFSEs is possible with an F-rich fluid (Loges et al., 2023), and a high F content in the system is likely because the pegmatites themselves belong to the Nb–Y–F type and contain a high amount of topaz. In addition, the muscovite in the breccia is F-rich, and fluorite is a common mineral associated with kerite (see below). For further details, such as transmission electron microscopy of the border zone of OM to opal and about opal itself, the reader is referred to our original publication.
We postulated that the low N content was caused by the decay of kerite, producing NH4, which was responsible for K–NH4 exchange reactions in K feldspar and in muscovite, forming buddingtonite and tobelite. There is no doubt that, before the formation of the breccia and the pseudomorph, OM was present in the system. Buddingtonite is not a rare mineral in the Volyn pegmatite field (Proshko, 1987), and the high activity ratio for NH K+ required to transform K feldspar into buddingtonite (Mäder et al., 1996) indicates a large amount of decayed OM. This is not consistent with the concern of Head et al. (2024) that the OM in the pegmatite field is a result of late-stage contamination. Also, the chemical composition of the OM is completely incompatible with anything like museum dust or plant hairs.
3.2 Fossil or non-fossilized OM
Head et al. (2024) question the fossil character of kerite. Here we want to summarize the presented information about the metamorphic, mature character of kerite.
After the occurrence of OM in the pegmatitic environment, the temperatures again reached approximately 300 °C (Franz et al., 2017). This estimate is based on the phase equilibria with bertrandite and muscovite in the pseudomorph. Furthermore, within beryl we observed fluid inclusions with C–H, which occur on cracks sealed by secondary beryl (Voznyak et al., 2012). This implies that temperatures were above the lower thermal stability of beryl, which is at low pressure near 300 °C (Barton and Young, 2002). These temperatures are consistent with our observation on decomposition of chitin to chitosan described in detail in Franz et al. (2023a); see below the discussion about Fourier transform infrared (FTIR) data.
All kerite samples were investigated by open-system pyrolysis. They do not differ significantly, and all spectra show characteristics of mature to very mature OM (Fig. 13 in Franz et al., 2022b, and in the Supplement). This excludes young contamination by plant hairs. Similarly, the light microscopic investigations in cross-sections with white and UV light show clear indications by different reflectivity and fluorescence that are not consistent with young OM. We described the brittle behavior of kerite, which is also not compatible with young unmetamorphosed OM. Brittle behavior was also noted by Yushkin (1996). Luk'yanova et al. (1982) described X-ray diffraction investigations with a diffuse peak at 8° θ indicating OM with some graphite-like sheets.
Head et al. (2024) refer to mineralized trichomes (Mustafa et al., 2017, 2018; Ensikat et al., 2017) and take this as an argument against fossilization. These plant hairs are biomineralized with Ca carbonate, Ca phosphate, and silica, especially at the tip of the trichomes. This biomineralization is quite different from what we interpreted as fossilized and mineralized rims of the Volyn kerite. We wrote that the most conspicuous feature is the common occurrence of Si–Al–O, interpreted as Al silicates. In the quoted investigations, Al was never observed. Furthermore, Ca phosphate was observed in kerite only in some places in nano-sized crystals (see, e.g., Fig. 11 in Franz et al., 2022b), at variance with a continuous biomineralization on the tips. Kerite is completely surrounded by a mineralized rim, whereas trichomes are only mineralized at their tips. All different kerite morphologies are mineralized in the same way.
Concerning the analytical procedure applied by us, there is a misunderstanding in the comment by Head et al. (2024). In lines 146 to 149 they wrote the following: “Had Franz et al. (2023) used EDX in addition to applying EDAX EDS to selected cross-sections, they would have been easily able to determine the elemental distribution for all specimens they imaged using SEM which could have assisted in discriminating extant contaminations from fossil material”. For our element mapping, we used wavelength-dispersive spectroscopy (WDS) with an electron microprobe (EMP), which is much more sensitive than energy-dispersive spectroscopy (EDS) such as EDAX. We showed several element distribution maps of different morphologies in Franz et al. (2022b), and, since all show generally identical features with an Al–Si–Ca rim structure and an internal structure with characteristic N–O–S distribution, we can safely exclude biomineralization but instead include mineralization due to a fossilization process.
3.3 EDS (with SEM)
All spectra of kerite objects show a high amount of oxygen. This excludes fresh organisms but again indicates (highly) mature OM. Minerals on the surface of filamentous kerite (Fig. 1a–d), bulbous kerite (Fig. 1e), and the spherical object, interpreted by Head et al. (2024) as a pollen, are mostly Al silicates, some with K, Na, and Ca. The flaky shape of the minerals indicates clay minerals, and one needle-shaped crystal is a Ti oxide, possibly rutile.
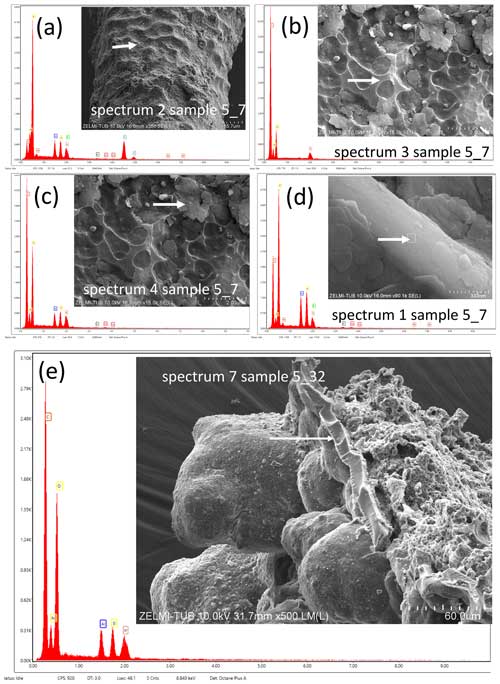
Figure 1EDS spectra obtained with the SEM of filamentous (a, b, c, d) and bulbous (e) kerite objects. (a) Needle-shaped small object with high Ti–O contents (arrow; interpreted as rutile) next to Al silicates with minor amounts of Na, K, and Ca. (b) Spectrum of the clear surface (arrow) of kerite, showing only the kerite composition of C–N–O. (c) Spectrum of platy mineral grains (arrow) with Al–Si and small contents of K and Fe, interpreted as a clay mineral. (e) Base (arrow) of bulbous kerite, with high amounts of Al–Si. Samples are iridium-coated.
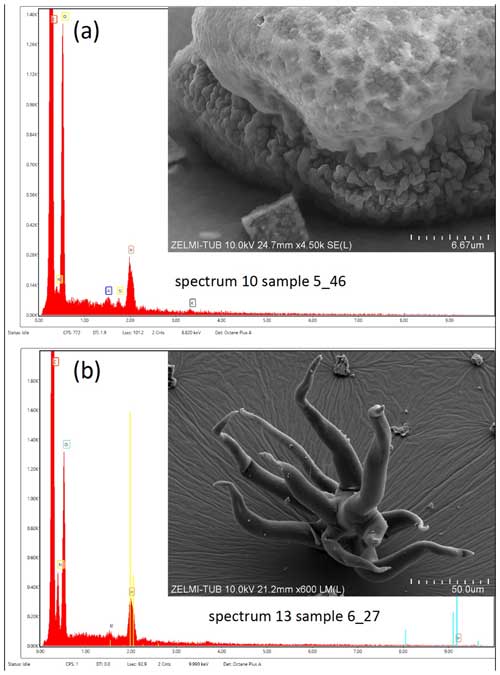
Figure 2EDS spectra of a spherical object (a) and a filamentous object (b). The spherical object shows Al–Si–K peaks, which can be interpreted as illite, whereas the filamentous object shows only the typical composition of kerite with C–N–O; both samples are iridium-coated.
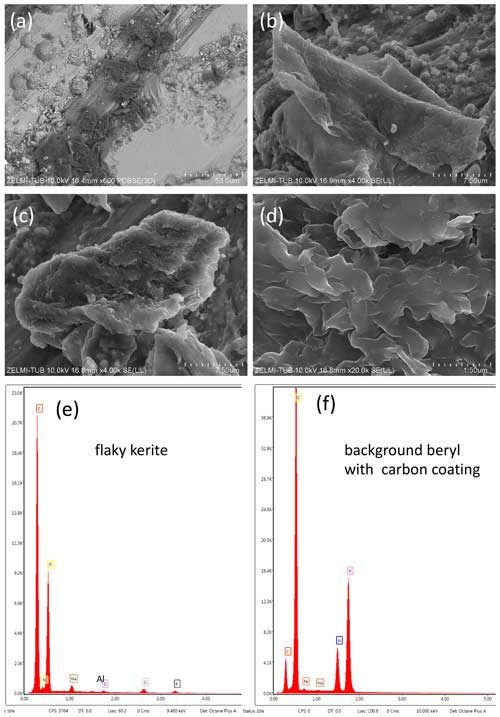
Figure 3EDS data of flaky kerite, observed on sample V2008, a beryl crystal. Panels (a), (b), (c), and (d) show the structure of kerite, with a combined BSE detector for element contrast in panel (a). The dark contrast compared to background beryl and other minerals indicates a low average atomic number. Panel (e) shows the corresponding EDS spectrum, with clear indication of Si, Al, Na, K, and Cl, next to C–N–O of kerite. Panel (f) shows the EDS spectrum of beryl; note the low C peak caused by carbon coating compared to the large C peak of kerite.
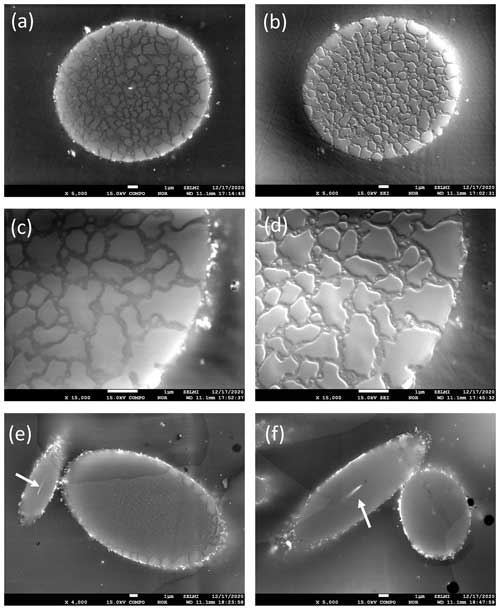
Figure 4BSE (a, c, d, e) and SE (b, d) of cross-sections of filamentous kerite, embedded in epoxy. Note the discontinuous rim of high contrast indicating mineralized parts, also with high contrast (arrows) within the channel (e, f). The mosaic pattern with different contrast in BSE (a, c) is seen in SE images (b, d) as slightly lower areas of approximately 200 nm width.
The EDS spectrum of the object, interpreted as pollen by Head et al. (2024), also shows the presence of Al and Si, together with the typical C–N–O peaks (Fig. 2). The EDS spectrum of the object, interpreted by Head et al. (2024) as trichome “museum dust” (Fig. 3), shows no Al–Si, but the C–N–O ratios are very similar to those of the mineralized filaments; therefore we have no doubts that this is also fossilized OM.
3.4 EMPA data
In BSE images of cross-sections of filamentous kerite, we see a discontinuous mineralized rim (Fig. 4). In combination with the element mappings (see images in Figs. 8–11 in Franz et al. (2022b) and Figs. S6–S7 in the Supplement to Franz et al., 2022b), we can safely conclude that the mineralized rim predominantly consists of Al silicates. Some other minerals, such as Ca phosphate or silica, occur only in isolated spots and do not cover the whole rim. Over a distance of approximately 1 µm, the filament shows a higher-contrast rim in BSE images, indicating a higher average atomic number, consistent with our interpretation that this is caused by a mineralized, impregnated rim of predominantly Al silicates. In the internal structure of the filament, a mosaic pattern can be observed with approximately 200 nm wide channels, also indicated by different element contrast (Fig. 4a, b). In SE images (Fig. 4c, d) a slightly lower position of the channels is seen, caused by different behavior during polishing. This internal structure is compatible with fossilized material, not with fresh cells of trichomes.
3.5 TEM data
In addition to the TEM investigations we presented in Franz et al. (2017), we cut a new focused ion beam (FIB) foil from a filamentous object (Fig. 5). The foil covers the embedding material epoxy (characterized by typical Cl content), the approximately 500 nm wide rim, and kerite (with dominantly C–O and N). The rim consists of a mixture of different minerals, which can be distinguished by different contrast in the HAADF images. EDAX spectra indicate dominantly Al silicates with minor amounts of K, Ca, Fe, and Fe–Ca phosphate. This is different from the type of biomineralization in trichomes shown by Mustafa et al. (2017, 2018) and Ensikat et al. (2017).
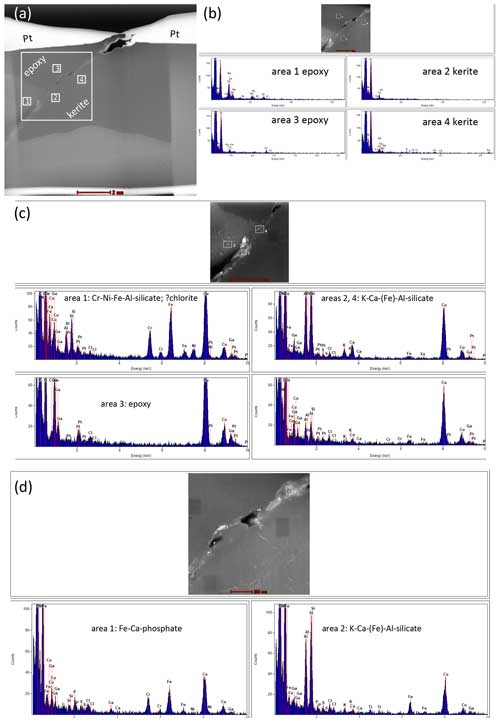
Figure 5Analytical EDAX-TEM results on an FIB from the rim of a filamentous kerite object. Note for all spectra that Ga peaks are due to the Ga ion cutting, Cu peaks originate from the copper grid, and Pt originates from the platinum holder. (a) Overview of the FIB foil; the white frame indicates the position of (b) the high-angle annular dark-field (HAADF) image and EDAX spectra of kerite and the embedding material epoxy. (c) Detail of panel (b) with EDAX spectra of three inclusions, interpreted as possibly chlorite and a complex Al silicate, possibly a clay mineral. (d) Detail of panel (b) with EDAX spectra of two inclusions, an Fe–Ca phosphate and a complex Al silicate.
3.6 IR spectra
Head et al. (2024) criticize our IR spectra and argue that we should have used modern fungal chitin standards for comparison and a more detailed comparison with sub-fossil and fossil fungi. Since we knew that the Volyn biota experienced temperatures near 300 °C, comparison with modern fungi did not seem appropriate. Instead, we followed the procedure by Loron et al. (2019) and the thermal degradation studies of chitosan (Wanjun et al., 2005; Zawadzki and Kaczmarek, 2010; Vasilev et al., 2019). These are clearly consistent with our conclusion that chitosan is a constituent of the kerite material.
Head et al. (2024) present evidence for the strong similarity of one object in our sample collection to pollen of an extinct conifer. The similarity is indeed striking, but we want to stress important differences (Fig. 6).
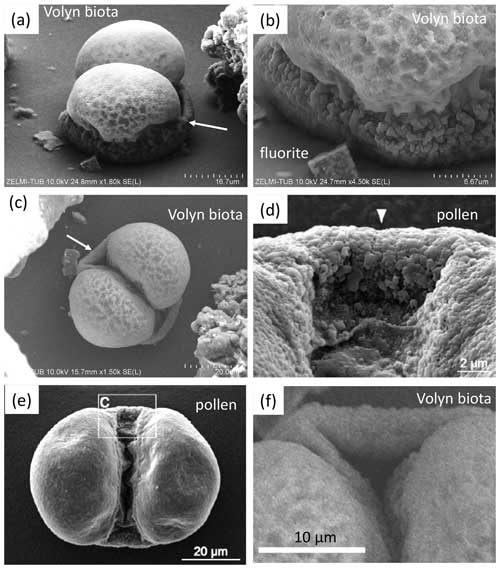
Figure 6SEM images for direct comparison of a kerite object from the Volyn biota (a, b, c, f) and Pinus pollen (d, e) from Head et al. (2024). Note that the kerite object sits firmly on a base consisting of OM (a, b), whereas pollen consists of free objects. The surface of kerite is characterized by dents (b), whereas pollen shows a microrugulate surface (d). The objects described in pollen as air sacs (d, e) sit at a similar height to the pollen grain itself (d), whereas what we described as a sheath comes from the base of the kerite object (arrows in panels a and c). This sheath shows some inward folding (f), which is not seen in the air sac of the pollen.
The kerite object sits firmly on a base consisting of OM (Fig. 6a, b), whereas pollen consists of free objects. The surface of kerite is characterized by dents (Fig. 6b), whereas the pollen shows a microrugulate surface (Fig. 6d). The objects described in pollen as air sacs (Fig. 6d, e) sit at a similar height to the pollen grain itself, whereas what we described as a sheath comes from the base of the kerite object (arrows in Fig. 6a, c). This sheath shows some inward folding (Fig. 6f), which is not seen in the air sac of the pollen.
The other object, which Head et al. (2024) interpret as a plant hair (Fig. 3j, k, l in Franz et al., 2023a) due to its similarity to “museum dust”, also sits firmly on a base. If such delicate objects as unfossilized trichomes were transported down into the chamber (where they were sampled), it is difficult to imagine that they survived the transport.
Head et al. (2024) restrict their criticism to these two objects but do not mention the fact that the large majority of objects we presented have a different morphology, with filaments up to the millimeter size, bulbous objects, objects with irregular shape, etc. None of these objects are similar to trichomes. Also, they do not mention the internal structure with a channel, which we documented in detail (Fig. 11 in Franz et al., 2023a) and which is also obvious in BSE images (Fig. 4). They also do not mention the presence of Bi(Te,S) biomineralization, which we documented (Fig. 10 in Franz et al., 2023a). To the best of our knowledge, this type of biomineralization was not observed in trichomes.
Popov (2023) questioned the minimum age of the organic matter, which we proposed as 1.5 Ga, based on the Ar–Ar laser ablation data (Franz et al., 2022a) of muscovite in a pseudomorph after beryl. He proposed a sequence of events, starting with the intrusion of granites and pegmatites at approximately 1.76 Ga (Shumlyanskyy et al., 2017, 2021); then cooling and pseudomorph formation due to a hydrothermal event at 1.5 Ga; then cooling again; then the introduction of organic matter; and finally a second hydrothermal event, which converted muscovite into tobelite and K feldspar into buddingtonite. The age of the second event could have been early Phanerozoic, based on our data (Franz et al., 2022b) of dating attempts of the kerite itself, which produced, in the wording of Popov (2023), an isochrone of 493 ± 98 (1s) Ma but which we regarded only as a reference line due to the large uncertainty. In this sequence of events, the breccia formation is missing, but this event is important: it fractionated feldspar and quartz into irregular centimeter-sized pieces, including a large piece of pegmatitic beryl. This event must have occurred before the pseudomorph formation because the delicate pseudomorph, consisting of a rather loose framework of muscovite and bertrandite, would not have survived the brecciation. However, the breccia is cemented by black opal (pigmented by hydrocarbons), and OM must have been present before the precipitation of opal. Therefore, the sequence of events after the intrusion must have been as follows: organic matter, brecciation, and pseudomorph formation at 1.5 Ga in one event, first with muscovite formation; then the decay of the kerite and production of NH tobelite and buddingtonite (including formation of secondary C–H-bearing fluid inclusions in low-T beryl); then further cooling. It was made clear in our text that “the fluid composition changed during the pseudomorph formation, starting with F-dominated K-rich fluids producing pure F-muscovite, followed by alternating NH4-rich and K-rich compositions, producing oscillatory growth zones in buddingtonite (Fig. 5e) and ending with a late K-rich fluid (producing some outer K-rich zones in buddingtonite; Fig. 5d).” This is the same conclusion as in our first analysis of the pseudomorph's texture (Franz et al., 2017) and is clear from the summary in Fig. 13, illustrating the sequence of processing in one single geological event. We feel misinterpreted by Popov (2023), who wrote that in our second study we had changed our minds.
There might have been additional hydrothermal events since 1.5 Ga, caused, e.g., by the Neoproterozoic Volyn Large Igneous Province at approximately 600 Ma or by later Devonian rifting of the Pripyat aulacogen (Shumlyanskyy et al., 2016), but none of these events are documented up to now in the pegmatites of the Volyn field. We fully agree with Popov (2023) that the late-stage development of pegmatites, including later overprinting by hydrothermal events, may point to a protracted history. However, for the Volyn locality, the late-stage development is documented in Lazarenko et al. (1973) and in a study of dissolution of Volyn beryl crystals with the formation of typical and diagnostic etching (Franz et al., 2023b).
Head et al. (2024) conclude their discussion with the following: “We have doubts whether any of the in situ Volyn `biota' is organic in origin”, based on references to the low δ13C values obtained via experiments with Fischer–Tropsch (FT) synthesis under hydrothermal conditions in the presence of metallic Fe. From a starting composition with an assumed value for δ13C of −20 ‰, different organic compounds were obtained with a rather uniform composition of −50 ‰ (McCollom and Seewald, 2006). Abiotic synthesis of nitrogen-bearing organic carbon species, such as amino acids, is thermodynamically favored by molecular H2, which is produced by serpentinization of Fe-rich mantle-derived rocks (Ménez et al., 2018). The presence of Fe-rich minerals as a catalyst for the production of abiotic carbonaceous material in serpentinites (Nan et al., 2021) is not a good analogue for the granitic environment, in which Fe-rich minerals are generally scarce. An FT process is unlikely in the current geological setting of an Fe-poor granite–pegmatite system.
The source of carbon for abiotic synthesis in Volyn should be the mantle, with a uniform δ13C value of −5 ‰ (Marty et al., 2013, and references therein), because the Korosten pluton is composed mainly of mantle-derived granitic, gabbroic, and anorthositic rocks (Shumlyanskyy et al., 2017, 2021). Assuming a similar fractionation of −30 ‰ for a source with δ13C of −5 ‰, a composition of abiotic kerite should have values of −35 ‰, but many kerite bulk samples have much lower values between −40 ‰ and −48 ‰. According to the model of abiotic origin, mantle-derived fluids should also be the source for nitrogen. The N-isotopic signature of the mantle scatters from −25 ‰ to +15 ‰, with most values around −5 ± 3 ‰ Cartigny (2005); therefore a mantle source is less likely for the Volyn locale, with positive δ15N values up to 10 ‰ throughout. A more detailed description of the isotopic composition of the kerite organic matter might be possible with in situ methods. Such methods are currently not available for us, but we will explore the possibility of cooperation with other laboratories.
An alternative source might be the country rocks of the Korosten pluton, but this would require large amounts of C- and N-rich fluids, and there is no geological evidence for such fluid–rock interactions. They should also have left their signature within the granites, which are the hosts of the pegmatites. Yushkin (1996) presented analyses of different proteins in kerite and used them to argue that abiotic synthesis is possible. However, he starts from the assumption of abiotic origin and did not consider the possibility of fossil material. The large amounts of kerite of several kilograms recovered from the mine (Ginzburg et al., 1987) are, in our view, more consistent with biomass accumulation; what has been described as abiotic formation of carbonaceous material was observed in small amounts in thin sections only (e.g., Nan et al., 2021; Ménez et al., 2018).
Although the morphology of two objects selected by Head et al. (2024) shows a striking similarity to recent organisms, the combination of all observations is much more in favor of fossil organisms due to the following: the occurrence in the mine as part of the deep biosphere; a large variety of morphologically different objects, which nevertheless all have the same type of rim mineralization; their brittle behavior; the internal structure with a channel in the filaments; and the presence of biomineral inclusions of Bi(Te,S).
Further studies on the molecular composition, i.e., certain biomarkers, will help to characterize kerite in more detail and give information about the type of organisms. However, this requires more material, which is not available due to the current situation in Ukraine.
We are aware that our single age determination of 1.5 Ga for the hydrothermal overprinting of the pegmatites should be verified or falsified by ages in different minerals and/or different isotope systems. If more and better data were available, we would be happy to change the interpretation, but, with the currently available data, the presented interpretation seems to be the best one. We are currently working on Rb–Sr data with the laser ablation system from the same sample, which was determined by Ar–Ar and can be applied to both minerals, muscovite and feldspar. Additional sample material with white mica and feldspar is also available and will be studied.
Fluid inclusion studies might further help to clarify the origin of kerite (Voznyak et al., 2012, and references therein; Kalyuzhnyi et al., 1971). Liu et al. (2022) observed whewellite (CaC2O4.H2O) in the CO2–N2–CH4 vapor of fluid inclusions in topaz, thought to be a product of oxidation of organic material with an alkaline fluid. In situ determination of C and N isotopes, and possibly other stable isotopes (e.g., O, S), might also help to further clarify the type of organisms, their internal structure, and their origin.
All data appear as figures in the text or in the cited references.
GF: concept, figures, and writing. VK: writing and FTIR. VC and PL: information about the sampling and occurrence.
The contact author has declared that none of the authors has any competing interests.
Publisher’s note: Copernicus Publications remains neutral with regard to jurisdictional claims made in the text, published maps, institutional affiliations, or any other geographical representation in this paper. While Copernicus Publications makes every effort to include appropriate place names, the final responsibility lies with the authors.
We thank Anja Schreiber and Richard Wirth for permission to use unpublished TEM data, Cindy De Jonge for editorial handling, and the two anonymous reviewers for their helpful comments.
This research has been supported by the Alexander von Humboldt Foundation.
This paper was edited by Cindy De Jonge and reviewed by two anonymous referees.
Barton, M. D. and Young, S.: Non-pegmatitic deposits of beryllium: Mineralogy, geology, phase equilibria and origin, Rev. Mineral. Geochem., 50, 591–691, 2002.
Cartigny, P.: Stable isotopes and the origin of diamond, Elements, 1, 79–84, 2005.
Ciarniello, M., Moroz, L. V., Poch, O., Vinogradoff, V., Beck, P., Rousseau, B., Istiqomah, I., Sultana, R., Raponi, A., Schroeder, S., Kappel, D., Quirico, E., Filacchione, G., Pommerol, A., Mennella, V., and Pilorget, C.: VIS-IR spectroscopy of mixtures of ice, organic matter and opaque minerals in support of minor bodies remote sensing observations, EPSC Abstracts, 13, 1467–1468, 2019.
Durand, B.: Sedimentary organic matter and kerogen, Definition and qualitative importance of kerogen, in: Kerogen – Insoluble Organic Matter from Sedimentary Rocks, edited by: Durand, B., 13–34, Editions Technip, Paris, 1980.
Ensikat, H.-J., Mustafa, A., and Weigend, M.: Complex patterns of multiple biomineralization in single-celled plant trichomes of the Loasaceae, Amer. J. Bot., 104, 195–206, 2017.
Franz, G., Khomenko, V., Vishnyevskyy, A., Wirth, R., Nissen, J., and Rocholl, A.: Biologically mediated crystallization of buddingtonite in the Paleoproterozoic: Organic-igneous interactions from the Volyn pegmatite, Ukraine, Amer. Mineral. 102, 2119–2135, 2017.
Franz, G., Sudo, M., and Khomenko, V.: 40Ar 39Ar dating of a hydrothermal pegmatitic buddingtonite–muscovite assemblage from Volyn, Ukraine, Eur. J. Mineral., 34, 7–18, https://https://doi.org/10.5194/ejm-34-7-2022, 2022a.
Franz, G., Lyckberg, P., Khomenko, V., Chournousenko, V., Schulz, H.-M., Mahlstedt, N., Wirth, R., Glodny, J., Gernert, U., and Nissen, J.: Fossilization of Precambrian microfossils in the Volyn pegmatite, Ukraine, Biogeosciences, 19, 1795–1811, https://https://doi.org/10.5194/bg-19-1795-2022, 2022b.
Franz, G., Khomenko, V., Lyckberg, P., Chornousenko, V., Struck, U., Wirth, R., Gernert, U., and Nissen, J.: The Volyn biota (Ukraine) – indications for 1.5 Gyr old eucaryotes in 3D-preservation, a spotlight on the `boring billion', BioGeosciences, 20, 1901–1924, https://doi.org/10.5194/bg-20-1901-2023, 2023a.
Franz, G., Vyschnevskyi, O. A., Khomenko, V. M., Lyckberg, P., and Gernert, U.: Etch pits in heliodor and green beryl from the Volyn pegmatites, Northwest Ukraine: A diagnostic feature, Gems Gemol., 59, 324–339, 2023b.
Ginzburg, A. I., Bulgakov, V. S., Vasilishin, I. S., Luk'yanova, V. T., Solntseva, L. S., Urmenova, A. M., and Uspenskaya, V. A.: Kerite from pegmatites of Volyn, Dokl. Akad. Nauk SSSR, 292, 188–191, 1987 (in Russian).
Gorlenko, V. M., Zhmur, S. I., Duda, V. I., Osipov, G. A., Suzina, N. E., and Dmitriev, V. V.: Fine structure of fossilized bacteria in Volyn kerite, Orig. Life Evol. Biosph., 30, 567–577, 2000.
Head, M. J., Riding, J. B., O'Keefe, J. M. K., Jeiter, J., and Gravendyck, J.: Comment on “The Volyn biota (Ukraine) – indications of 1.5 Gyr old eukaryotes in 3D preservation, a spotlight on the `boring billion' ” by Franz et al. (2023), Biogeosciences, 21, 1773–1783, https://doi.org/10.5194/bg-21-1773-2024, 2024.
Kalyuzhnyi V. A., Voznyak, D. K., and Gigashvili, G. M.: Mineral-forming fluids and mineral paragenesis of chamber pegmatites of Ukraine, Kyiv: Naukova Dumka, 216 pp., 1971 (in Ukrainian).
Lazarenko, E. K., Pavlishin, V. J., Latysh, V. T., and Sorokon, Ju. G.: Mineralogy and genesis of the chamber pegmatites from Volyn, Lvov, Vysskaja shkola, 360 pp., 1973 (in Russian).
Liu, Y., Schmidt, C., and Li, J.: Peralkalinity in peraluminous granitic pegmatites, I. Evidence from whewellite and hydrogen carbonate in fluid inclusions, Amer. Mineral, 107, 233–238, 2022.
Loges, A., Manni, M., Louvel, M., Wilke, M., Jahn, S., Welter, E., Borchert, M., Qiao, S., Klemme, S., and Keller, B. G.: Complexation of Zr and Hf in fluoride-rich hydrothermal aqueous fluids and its significance for high field strength element fractionation, Geochim. Cosmochim. Ac., 366, 167–181, https://doi.org/10.1016/j.gca.2023.12.013, 2023.
Loron, C. C., François, C., Rainbird, R. H., Turner, E. C., Borensztajn, S., and Javaux, E. J.: Early fungi from the Proterozoic era in Arctic Canada, Nature, 570, 232–235, 2019.
Lu'kyanova, V. T., Lobzova, R. V., and Popov, V. T.: Filaceous kerite in pegmatites of Volyn, Izvestiya Ross. Akademii Nauk Ser, Geologicheskaya, 5, 102–118, 1992 (in Russian).
Mäder, U. K., Ramseyer, K., Daniels, E. J., and Althaus, E.: Gibbs free energy of buddingtonite (NH4AlSi3O8) extrapolated from experiments and comparison to natural occurrences and polyedral estimation, Eur. J. Mineral., 8, 755–766, 1996.
Marty, B., Alexander, O'D., and Raymond, S. N.: Primordial origins of Earth's carbon, Rev. Mineral. Geochem., 75, 149–181, 2013.
McCollom, T. M. and Seewald, J. S.: Carbon isotope composition of organic compounds produced by abiotic synthesis under hydrothermal conditions, Earth Planet. Sc. Lett., 243, 74–84, https://doi.org/10.1016/j.epsl.2006.01.027, 2006.
Ménez, B., Pisapia, C., Andreani, M., Jamme, F., Vanbellingen, Q., Brunelle, A., Richard, L., Dumas, P., and Réfrégiers, M.: abiotic synthesis of amino acids in the recesses of the oceanic lithosphere, Nature, 564, 59–63, https://doi.org/10.1038/s41596-018-0684-z.hal-02111638, 2018.
Moroz, L. V., Arnold, G., Korochantsev, A. V., and Wäsch, R.: Natural solid bitumens as possible analogs for cometary and asteroid organics: 1. Reflectance spectroscopy of pure bitumens, ICARUS, 134, 253–268, https://doi.org/10.1006/icar.1998.5955, 1998.
Mustafa, A., Ensikat, H.-J., and Weigend, M.: Ontogeny and the process of biomineralization in the trichomes of Laosaceae, Amer. J. Bot., 104, 367–378, 2017.
Mustafa, A., Ensikat, H.-J., and Weigend, M.: Mineralized trichomes in Boraginales: complex microscale heterogeneity and simple phylogenetic patterns, Ann. Botany, 121, 741–751, https://doi.org/10.1093/aob/mcx191, 2018.
Nan, J., King, H. E., Delen, G., Meirer, F., Weckhuysen, B. M., Guo, Z., Peng, Z., and Plümper, O.: The nanogeochemistry of abiotic carbonaceous matter in serpentinites from the Yap Trench, western Pacific Ocean, Geology, 49, 330–334, https://doi.org/10.1130/G48153.1, 2021.
Popov, D. V.: Do pegmatites crystallise fast? A perspective from petrologically-constrained isotopic dating, Geosciences, 13, 297, https://doi.org/10.3390/geosciences13100297, 2023.
Proshko, V. Ya., Bagmut, N. N., Vasilishin, I. S., and Panchenko, V. I.: Ammonium feldspars from Volyn pegmatites and their radiospectroscopic properties, Mineral. J., 9, 67–71, 1987 (in Russian).
Shumlyanskyy, L., Nosova, A., Billström, K., Söderlund, U., Andréasson, P.-G., and Kuzmenkova, O.: The U–Pb zircon and baddeleyite ages of the Neoproterozoic Volyn Large Igneous Province: implication for the age of the magmatism and the nature of a crustal contaminant, Gff-Upsala, 138, 17–30, 2016.
Shumlyanskyy, L., Hawkesworth, C., Billström, K., Bogdanova, S., Mytrokhyn, O., Romer, R., Dhuime, B., Claesson, S., Ernst, R., Whitehouse, M., and Bilan, O.: The origin of the Palaeoproterozoic AMCG complexes in the Ukrainian Shield: new U-Pb ages and Hf isotopes in zircon, Precam. Res., 292, 216–239, 2017.
Shumlyanskyy, L., Franz, G., Glynn, S., Mytrokhyn, O., Voznyak, D., and Bilan, O.: Geochronology of granites of the western Korosten AMCG complex (Ukrainian Shield): implications for the emplacement history and origin of miarolitic pegmatites, Eur. J. Mineral., 33, 703–716, https://doi.org/10.5194/ejm-33-703-2021, 2021.
Vasilev, A., Efimov, M., Bondarenko, G., Kozlov, V., Dzidziguri, E., and Karpacheva, G.: Thermal behavior of chitosan as a carbon material precursor under IR radiation, IOP Conf. Ser.-Mater. Sci. Eng., 693, 012002, https://doi.org/10.1088/1757-899X/693/1/012002, 2019.
Voznyak, D. K., Khomenko, V. M., Franz, G., and Wiedenbeck, M.: Physico-chemical conditions of the late stage of Volyn pegmatite evolution: Fluid inclusions in beryl studied by thermobarometry and IR-spectroscopy methods, Mineral. J., 34, 26–38, 2012 (in Ukrainian).
Wanjun T., Cunxin W., and Donghua, C.: Kinetic studies on the pyrolysis of chitin and chitosan, Polym. Degrad. Stabil., 87, 389–394, 2005.
Yushkin, N. P.: Natural polymer crystals of hydrocarbons as models of prebiological organisms, J. Crystal Growth, 167, 237–247, 1996.
Zawadzki, J. and Kaczmarek, H.: Thermal treatment of chitosan in various conditions, Carbohydr. Polym., 80, 394–400, 2010.
Zhmur, S. I.: Origin of Cambrian fibrous kerites of the Volyn region, Lithol. Mineral Resour., 38, 55–73, 2003
- Abstract
- Introduction
- Occurrence of kerite and sampling
- Composition and structure of kerite
- Comparison of kerite morphology
- Age of the fossils
- Origin of kerite – biotic or abiotic
- Summary and open questions
- Data availability
- Author contributions
- Competing interests
- Disclaimer
- Acknowledgements
- Financial support
- Review statement
- References
- Abstract
- Introduction
- Occurrence of kerite and sampling
- Composition and structure of kerite
- Comparison of kerite morphology
- Age of the fossils
- Origin of kerite – biotic or abiotic
- Summary and open questions
- Data availability
- Author contributions
- Competing interests
- Disclaimer
- Acknowledgements
- Financial support
- Review statement
- References