the Creative Commons Attribution 4.0 License.
the Creative Commons Attribution 4.0 License.
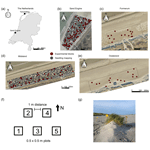
Narrowing down dune establishment drivers on the beach
Jan-Markus Homberger
Sasja van Rosmalen
Michel Riksen
Juul Limpens
Coastal dune development is typically initiated by the interaction between recently established dune-building vegetation and sediment transport processes. Narrowing down the biotic and environmental conditions needed for vegetation establishment could improve predictions of dune initiation, but obtaining such data on a meaningful spatial scale has proven to be challenging.
We investigated the establishment of grasses and the initiation of dunes across a range of environmental conditions on four beach sections in the Netherlands. To understand spatial patterns of spontaneous establishment, we mapped the occurrence of grass seedlings in 1899 plots in July 2021. To explore the role of environmental drivers of grass establishment and the ensuing dune initiation, we conducted an establishment experiment using 750 plots. We introduced seeds and rhizome pieces of Elytrigia juncea and Ammophila arenaria and monitored establishment success, dune initiation, and environmental conditions (soil moisture, salinity, and the change in beach bed level) between March 2022 and February 2023.
Field observations in 2021 showed that 69 %–84 % of the seedlings occurred close to adult dune-building grasses, suggesting limited dispersal of diaspores or, alternatively, strong positive biotic feedback during the seedling stage. The establishment of introduced seeds and rhizomes peaked in locations with high soil moisture (at 15 %–20 %), low salinity (<340 mS m−1), and low sediment dynamics (−2 to +5 cm change in bed level). Here, also the highest probabilities of dune initiation were found, with the highest probability associated with substantial shoot emergence (330 shoots m−2). In addition, dune initiation was associated with the middle section of the beach, characterized by moderate slopes and elevation and sufficient beach width.
Our findings indicate that the conditions required for the initiation of dunes are more restrictive than those for plant establishment, as they depend not only on the arrival of plant material but also on favorable environmental growing conditions. Our results can be applied to better predict the onset and development of coastal dunes.
- Article
(3697 KB) - Full-text XML
-
Supplement
(1241 KB) - BibTeX
- EndNote
Climate change is projected to affect sandy shorelines around the world, which cover approximately 31 % of the ice-free globe (Luijendijk et al., 2018; Ranasinghe, 2016). In fact, approximately 24 % of the sandy beaches around the world have been showing a receding trend over the last 30 years (1984–2016) (Luijendijk et al., 2018), likely partly due to increased storm frequencies and sea level rise (Vousdoukas et al., 2020). For anthropogenic beaches, where the space for coastal retreat is limited, beach nourishments are often used (Arens et al., 2013; Keijsers et al., 2015b) to create a positive sediment budget, allowing beach recovery, which may also stimulate new dune development while improving the climate resilience of dunes. Although vegetation re-establishment can be a decisive factor for dune initiation and beach recovery (Castelle et al., 2017; Hesp, 1989, 2002, 1981; Hilton and Konlechner, 2011; Houser et al., 2015; Snyder and Boss, 2002), little is known about the environmental conditions necessary to support vegetation establishment and the initiation of dunes.
As coastal vegetation establishes and grows over time, dunes may develop as a result of interactions between plant attributes (e.g., cover, density, height) and eolian sediment transport (Hesp, 2002, 1989). Here, perennial dune-building grasses play an important role, as their shoots remain present during the winter season (Hesp, 2002). Dune-building grasses establish from seeds and rhizome fragments or through clonal expansion (Bonte et al., 2021; Davy and Figueroa, 1993). Although the latter is a localized process (Keijsers et al., 2015a; Reijers et al., 2021), seeds and rhizome fragments can be important for recolonization of the beach and recovery of the dune topography after severe storm disturbances (Harris and Davy, 1986a; Snyder and Boss, 2002). In regions affected by storms, management efforts often focus on restoring dunes by planting dune-building species, while in other regions removal of vegetation is prioritized to recover sediment dynamics and restore original habitats (Martínez et al., 2013). Ammophila arenaria (European marram grass) is an excellent example, as it is planted as a dune-stabilizing species on European coastlines (Provoost et al., 2011) yet also removed as an invasive species in countries such as South Africa or New Zealand (Martínez et al., 2008; Hilton and Konlechner, 2010; Thomas et al., 2018). Understanding the environmental conditions that influence vegetation establishment could help optimizing both of these management strategies.
In dune ecosystems, changes to the bed level (i.e., erosion or accretion) are considered key drivers of plant success. Too much sediment burial can limit shoot emergence and may cause plant mortality, especially under complete burial of shoot and plant material (Bonte et al., 2021; Harris and Davy, 1987; Ievinsh and Andersone-Ozola, 2020; Konlechner et al., 2013; Lim, 2011). Conversely, slight amounts of burial may be beneficial (Ievinsh and Andersone-Ozola, 2020; Lammers et al., 2024b; Maun, 1994). Although the impact of burial appears to be well established, much less is known about erosion, soil moisture, and salinity. Erosion is thought to strongly limit seedling survival (Maun, 1994; Huiskes, 1977), although there may be some tolerance for it in adult plants (Konlechner et al., 2019). Lack of soil moisture or rainfall is often mentioned as another important limiting factor for establishment (Chergui et al., 2013; Konlechner et al., 2013; Maun, 1994); similarly, salinity appears to particularly influence seed germination or emergence of shoots from rhizome fragments (Chergui et al., 2013; Konlechner et al., 2013; Walmsley and Davy, 1997).
Although the importance of initial plant establishment for dune initiation is widely recognized, there are still a limited number of studies investigating both simultaneously (van Puijenbroek et al., 2017b; Costas et al., 2024). Even less is known about vegetation establishment during the earliest phase of dune formation, which is difficult to observe on a large spatial scale. However, the strong spatial dependencies of abiotic conditions along a beach topography are important. Salinity decreases with distance from the ocean and may not be a limiting factor for plant performance unless certain levels are reached (van Puijenbroek et al., 2017c). During periods without considerable wave action, changes in bed level are typically the result of wind transport, with most of the sediments being picked up close to the high tide line (Strypsteen et al., 2024) and deposited at the dune toes (Hesp, 2002; Keijsers et al., 2015a). At lower beach elevations, groundwater can form a stable source of moisture as opposed to rainfall-dependent locations (Homberger et al., 2024). This may contribute to seedling recruitment (Huiskes, 1977). Identifying the best conditions for plant establishment and their occurrence on the beach will help translate results to guidelines for management.
The aim of this study was to narrow down drivers of plant establishment and dune initiation by focusing on three major abiotic factors: salinity, soil moisture, and changes in bed level. It has been well established that both environmental conditions and dispersal limitations can influence plant establishment in coastal dune systems (Maun, 2009). In order to separate the impacts of dispersal and environment on the establishment of dune-building grasses, we combined field mapping of spontaneous seedlings with a field establishment experiment. We expected that (1) establishment is dispersal limited; (2) establishment success (i.e., the net effect of germination, shoot emergence, survivorship, and growth) is driven by environmental conditions, primarily bed level change; and (3) dune initiation is a function of the number of emerged shoots, depending on both dispersal patterns and environment. In the establishment experiment, we introduced seeds and rhizome fragments of two common dune-building grasses: Ammophila arenaria (L.) Link (European marram grass) and Elytrigia juncea (L.) Nevski (sand couch). Given the strong spatial zonation of vegetation patterns on the beach, we focused on capturing spatial variation in the experiment, which we covered by monitoring environmental conditions and vegetation establishment in 750 plots across four study sites.
2.1 Study areas
The research was conducted at four beach sections – three of which are located on the Dutch island of Terschelling (52°24′19.4′′ N, 5°16′10.9′′ E) near the villages of Midsland, Formerum, and Oosterend, and one is located on the Sand Engine (52°02′51.0′′ N, 4°10′59.0′′ E) (Fig. 1). All study areas share limited management interventions (e.g., none of the beaches are mechanically cleaned), a beach width that supports vegetation and dune development, and a wide range of expected environmental conditions.
Terschelling is a barrier island on the northern coast of the Netherlands. The northern coast of Terschelling has comparably wide beaches, with widths ranging from 200 to 300 m for the studied beach sections. The predominant wind direction is southwest (Galiforni-Silva et al., 2020). The median grain size (d50) in the intertidal zone ranges from 220–260 µm (Ruessink and Kroon, 1994).
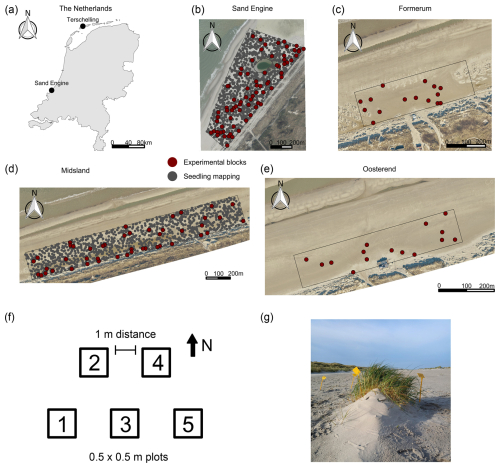
Figure 1Study areas and experimental setup. (a) Map of the Netherlands with study areas. (b) Sand Engine beach section. (c–e) Beach sections on Terschelling. Aerial images in panels (b)–(e) are sourced from https://www.beeldmateriaal.nl/, last access: 22 November 2023. Distributed under the Creative Commons Attribution 4.0 License. Note that the beach sections were delineated to encompass adult vegetation on the beach. As a landward limit, we used the foredune toe, and as a seaward limit we used a buffer from existing vegetation extending up to 100 m (Sand Engine) or 40 m seaward (Terschelling). (f) Blocks with plot arrangement. (g) Plot with a small (embryo) dune (Photo by Myrthe Bouma).
The Sand Engine is a mega-nourishment constructed in 2011 by supplying the foreshore with a volume of 21.5 Mm3 of sediment (Stive et al., 2013). Since the Sand Engine is artificially wide (up to approx. 600 m) and high (up to approx. 7 m a.m.s.l. (meters above mean sea level) in 2021), it provides a wide accommodation space for dune development (Nolet and Riksen, 2019). The dominant wind direction is south to southwest (Hoonhout and de Vries, 2017). Prominent landscape features on the Sand Engine include a dune lake and an extensive embryo dune field. The median grain size on the Sand Engine is 335 µm (Hoonhout and de Vries, 2017). The precipitation and temperature conditions during the experimental setup were comparable between the two study areas, and there were no storm occurrences (Table 1).
Table 1Mean precipitation (mm), mean temperature (°C), and maximum recorded hourly wind speed (m s−1) during the monitoring period.
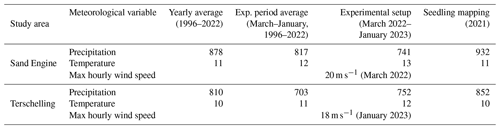
Note: retrieved from the Royal Netherlands Meteorological Institute (KNMI) weather stations Hoek van Holland (WMO code 06330, about 7 km from the Sand Engine) and Terschelling Hoorn (WMO code 06251, about 4 to 5 km from the beach sections).
The two species studied are main perennial dune-building grasses, native to the coasts of northwestern Europe (Bakker, 1976; Huiskes, 1979; Reijers et al., 2020; Lammers et al., 2023). Both species often co-occur within the same dune habitat (Bakker, 1976; Huiskes, 1979), though Elytrigia typically establishes closer to the sea at lower elevations compared to Ammophila (Reijers et al., 2020; van Puijenbroek et al., 2017c) and is therefore considered the main pioneer species during dune succession (Harris and Davy, 1986a). Ammophila is associated with later succession stages, occurring from mobile embryo dunes to fixed gray dunes (Huiskes, 1979; Bakker, 1976). Both species develop vast belowground rhizome systems and are able to grow upward (Harris and Davy, 1986a; Huiskes, 1979), allowing them to effectively trap wind-blown sediments and develop dunes several meters in height (Bakker, 1976). Although the species share many similarities, there are some important differences in their growth form, leading to differences in dune development. Elytrigia tends to grow sparse and dispersed (Lammers et al., 2023), leading to the development of wide and low dunes (van Puijenbroek et al., 2017a, b). Ammophila, on the other hand, grows clumped to patchy and at high densities (Lammers et al., 2023), leading to the formation of narrower and higher dunes (van Puijenbroek et al., 2017a, b). For both species, the main establishment strategy is believed to be clonal expansion (Huiskes, 1979; Reijers et al., 2020). However, both have also been observed to establish from seeds and rhizome fragments (Harris and Davy, 1986a; van der Putten, 1990).
On Terschelling, embryo dunes in the investigated areas are dominated by Elytrigia, but occasionally Ammophila is found closer to the foredunes. On the Sand Engine, both species are also co-occurring, with Ammophila occurring most frequently (Huisman et al., 2021).
2.2 Study design
Coastal vegetation establishment in dune systems is influenced by environmental conditions and dispersal limitations (Maun, 2009). Since separating the impacts of dispersal and the environment can be challenging, we used two complementary approaches to account for them: first, we mapped the occurrence of spontaneously established seedlings, and second, we conducted a field establishment experiment to assess establishment across environmental gradients. Both approaches were carried out on a large spatial scale.
2.2.1 Seedling mapping: spontaneous establishment
To investigate patterns in spontaneous grass seedling establishment, we mapped seedling occurrence in 920 plots on the Sand Engine and 979 at Midsland. The plots were allocated using a random number generator, covering about 1.5 % of the area of each beach section. The minimum distance between the plots was set to 10 m. All plots were visited in July 2021, and their location was recorded with a real‐time kinematic positioning system (RTK, Topcon Positioning Systems, Inc.) with reference to NAP (Normaal Amsterdams Peil, located around mean sea level). Within a radius of 2.5 m from the recorded point, the occurrence of grass seedlings and adult grasses was recorded. Grass seedlings were identified as grass plants shorter than approximately 15 cm with slender green leaves growing isolated from adults and not growing in a line as shoots from clonal expansion do (Fig. S1). We distinguished seedlings from rhizome fragments by noting the stout stems of rhizome shoots and the visibility of rhizome fragments on the soil surface (Fig. S1). Moreover, in case of doubts, we occasionally gently removed the top grains to confirm the presence of the seed.
2.2.2 Design establishment experiment
In order to cover a wide range of environmental conditions in our establishment experiment, we implemented a spatially guided experimental design. We combined a stratified random design (strata represent areas with similar environmental conditions) and spatially balanced probability sampling to determine the location for each experimental block. The strata were used to ensure that all typical environmental variations within each beach section were represented during the sampling process. The doubly balanced sampling algorithm was used to reduce spatial autocorrelation and improve the estimate of plant establishment success, while covering a wide range of environmental conditions (Grafström and Tillé, 2013).
The strata and balancing variables were derived from digital terrain models (DTMs) of the beach sections (source: https://downloads.rijkswaterstaatdata.nl, last access: 22 November 2023). We used the DTMs to derive covariates, which we expected to correlate with the environmental conditions measured in the field (salinity, moisture, and change in bed level). The covariates included the topographic wetness index (TWI), the elevation (DTM of 2021), and the average yearly change in beach bed level (from 2016–2021). Based on the DTM of 2021, the elevation was determined and the TWI was calculated with the SAGA GIS wetness algorithm (Conrad et al., 2015). The average yearly change in bed level was derived by calculating the difference in heights of the DTMs for consecutive years and then taking the average:
Strata were created by applying the k-means clustering algorithm (Hartigan and Wong, 1979) with five clusters across the three covariates (Table 2; see also Fig. S2).
Subsequently, 15 block locations were allocated per strata with the doubly balanced random sampling algorithm (Grafström and Tillé, 2013). We used the center coordinates of the individual raster pixels (x and y) as spreading variables and the same covariates that were used to create the strata as balancing variables. If needed, upon creation in the field, the block locations were moved away from existing vegetation (at least 1 m) and pathways to further ensure similar conditions within each block.
2.2.3 Plant material
We collected inflorescences with seeds of Elytrigia and Ammophila on the Sand Engine in August 2021 and stored them at ambient outside temperatures and humidity. To mimic the natural dispersal process as described by Huiskes (1979), the seeds in the husks were removed from the panicle of Ammophila by means of mechanical threshing and manual sieving. For Elytrigia, the rachis was removed, leaving only spikelets attached to the pedicels. The rhizomes of both species were collected locally during the experimental setup and cut into smaller pieces, leaving two nodes per piece (see Fig. S3). We assumed that each rhizome node and each seed could potentially turn into a shoot (Table 3).
Table 3Overview of species and introduced plant material per plot. To determine the potential number of emerging shoots from seeds, we repeatedly counted the number of seeds in 1 g of seeds for Ammophila and 10 g for Elytrigia. The seed length was determined by measuring the longest axis of the floret repeatedly with a pair of calipers.

* Elytrigia seeds were introduced as spikelets with an average length of 3.8±0.67 cm.
At each block, we created five plots (50×50 cm) (see Figs. 1f and S4). Between each of the individual plots, we maintained a minimum distance of at least 1 m to minimize impacts from shielding and encroachment of dune tails into other plots. Keeping a higher distance was not feasible without exposing some plots to very different conditions (e.g., by placing them on top of existing vegetation or on pathways). The close proximity of the plots implies that vegetation in upwind plots can potentially influence wind velocity and sediment transport, which may alter changes in the bed level in downwind plots, even when vegetation establishment is sparse (Wolfe and Nickling, 1993, 1996; Hesp et al., 2019). To account for this effect, we oriented all plots with respect to geographic north to standardize the wind exposure. We also assigned all four treatments (see Table 3) and the control plot randomly, ensuring that no treatment was systematically placed downwind or upwind. The control plot was created and left untreated to account for spontaneous establishment. The rhizomes and seeds were covered by 2 cm of sand. At this depth, plant establishment is possible, and the material is not immediately carried away (Bonte et al., 2021; Harris and Davy, 1986a; Lammers et al., 2024b). To avoid human disturbance, the plots were not marked but their positions were recorded with the RTK.
2.3 Measurements
2.3.1 Shoot numbers and dune initiation
The number of emerged shoots per plot was monitored during four monitoring moments: in May/June, August, and October of 2022, with the final monitoring round at the end of January and the beginning of February 2023 (referred to as the onset of winter). During each monitoring moment, shoots were counted in all of the plots (i.e., 750 plots). Due to time constraints, an exception was made for the last monitoring moment. Here, only blocks for which shoots had been recorded previously were revisited (i.e., 635 plots). To assess the potential impact of this block exclusion, we performed a sensitivity analysis (see Supplement Sect. S5). This analysis suggested that the block exclusion may have led to a slight overestimation of the predicted average establishment success, but the relative differences in treatments, significance of explanatory variables, and the patterns in the smoother predictions would likely have all remained very similar to the reported model (see Tables S7–S8 and Fig. S11).
To account for spontaneous shoot emergence, we corrected shoot numbers by subtracting the number of shoots that emerged in control plots from treatment plots, setting treatment shoot numbers to 0 for negative numbers. Dune initiation was assessed visually by observing the level of the sand surface within the plot relative to the surroundings. Given a visual confirmation, we measured the highest point within the plot with the RTK. We expressed plant establishment success as the corrected number of shoots present in a plot in the last monitoring round relative to the amount of plant material introduced in March 2022. Given the large spatial scale of our experiment, it was not feasible to explicitly separate the stages of plant development. Therefore, our definition of establishment success includes several ecological processes such as germination from seeds, emergence from rhizome fragments as well as subsequent shoot emergence from the soil, survivorship, and growth.
2.3.2 Bed level change, moisture, and salinity
In parallel with recording shoot numbers, abiotic conditions were measured for each plot during each monitoring moment (i.e., 750 plots and 635 plots at the last monitoring moment). In both beach sections, the wind predominantly comes from the southwest (Hoonhout and de Vries, 2017; Galiforni-Silva et al., 2020); therefore, we always recorded the heights of the southeast and northwest corners by means of the RTK. These two plot corners were always staked using the RTK system, ensuring consistent measurement of the same corners during each monitoring moment. We defined the change in bed level as the difference between the average plot height between two consecutive monitoring moments. Soil moisture and salinity were measured by carefully inserting a WET2 sensor into the soil of each plot and recording with an HH2 moisture meter (Delta‐T Devices Ltd.). The WET‐2 sensor measures the first 6.8 cm of the soil at a volume of 500 mL and 3 % accuracy, while salinity is measured for a soil moisture content greater than 15 % (Delta-T Devices Ltd., 2019).
2.4 Data analysis
2.4.1 Arrival limitations
To explore whether seedlings occurred close to adult vegetation, we calculated the number of plots that contained seedlings and adults relative to the total number of all plots containing seedlings. To visualize arrival limitations, we interpolated the presence/absence of the seedlings on the study area scale using bilinear interpolation for irregularly spaced data points. The result of this interpolation is a continuous estimate of seedling occurrence across the study area, with values ranging from 0 to 1. For visualization purposes, we converted the resulting rasters into polygons using a criterion of an interpolated seedling occurrence of more than 50 %. To compare the seedling mapping approach with our establishment experiment, we also interpolated the presence/absence of shoots per block. Here, we considered seedling presence when shoots of Ammophila and/or Elytrigia seeds were recorded in summer. To compare natural and introduced establishment, we used the monitoring moments in July 2021 and August 2022, as they occurred at a similar point in the growing season. Since both approaches greatly differed in the design (e.g., a larger number of plots with larger areas in the seedling mapping), we did not apply additional spatial analysis beyond the visualization of presence and absence.
2.4.2 Establishment success
To investigate how the establishment of dune-building grasses is influenced by key abiotic drivers, we related plant establishment success with the abiotic conditions measured in the plot. The experiment was designed to capture spatial variability on a timescale relevant for dune building. Describing smaller-scale temporal dynamics was beyond the scope of the study. Therefore, we averaged abiotic conditions over time (moisture, salinity, and bed level change) to relate broader trends with the resulting establishment success at the last monitoring moment.
We chose to fit generalized additive models (GAMs) to our data, since we anticipated nonlinearity in the growth responses of the dune-building grasses (Nolet et al., 2018). Given that our experimental design was not fully random, we corrected for the design by including random effects in the model structure. Since plots within the same block were assigned at a distance of 1 m, we expected their conditions to be similar and correlated (Zuur et al., 2009). Furthermore, each beach section may have its own environmental conditions, leading to site-specific differences (Zuur et al., 2009). Describing site- or block-specific effects was beyond the scope of the study; therefore, we treated blocks and beach sections as random effects in all GAMs, using random smoothers to model them (Wood, 2017). This approach assumes that blocks and beach sections come from a common distribution in the population, with the effects observed in our experiment viewed as independent samples from that distribution (Wood, 2017). By modeling these random components, inferences about the entire study population can be made (e.g., the overall establishment success) (Zuur et al., 2009; Wood, 2017).
Since the salinity measurements were restricted to soil moisture contents greater than 15 %, less data were available to model the impact of salinity on plant establishment (N=173 vs. N=508, model with moisture and change in bed level). Consequently, we fit two separate generalized additive models: one with all continuous data (salinity, moisture, and bed level change) but lower structural complexity (no treatment-specific effects) and another with higher model complexity and treatment-specific effects, excluding salinity (see also Table 4).
In both GAMs, we used a zero-inflated Poisson distribution with a log link to account for zero inflation (i.e., higher presence of zero shoots than predicted by a standard Poisson model). Moreover, we used shoot numbers as a response variable and introduced plant material as an offset to account for differences in the amount of plant material introduced initially (see Table 3), effectively modeling the plant response as the ratio between the number of shoots and the plant material (i.e., establishment success).
The relationship between establishment success and environmental predictors was modeled using thin plate regression spline smoothers, while interactions between continuous variables were modeled using tensor product interaction since the main effects and lower interactions were also present (Wood, 2017). Both approaches allow for nonlinear relationships and nonlinear interactive effects between continuous variables and the response (establishment success) (Wood, 2017). In addition, we also added a fixed treatment effect to explore the overall average impact of treatments on establishment success. Finally, in the model with the highest complexity (without salinity), we also varied smoothers per treatment group to investigate treatment-specific responses to environmental predictors.
2.4.3 Dune initiation
To investigate the response of dune initiation to biotic and abiotic conditions, we used a similar GAM structure as in the establishment success models and included the same random effects (block and study site) and the same smoother types for two continuous variables (soil moisture and bed level change) and their interactions. As the response variable, we used the presence or absence of a dune (0 and 1) and applied a binomial error distribution. In contrast to the plant establishment success models, we included the number of observed shoots as a continuous variable to test whether the number of emerged shoots could explain the probability of finding a dune. Therefore, no offset- or treatment-specific effects were included in this model.
To highlight interactions between conditions leading to plant establishment and dune formation, we combined predictions from two GAMs. First, we predicted the number of shoots over a range of bed level changes and two moisture levels. Second, we predicted the probability of the occurrence of dunes from the number of predicted shoots under the same conditions.
Finally, for all GAMs, parameters were selected using the double penalty approach (Marra and Wood, 2011), and smoothness was determined from restricted maximum likelihood (REML) (Wood, 2011). We also checked the validity of the model assumptions using a simulated residual diagnostic. More details on GAM structures and assumptions can be found in Supplement Sects. S3–4.
2.4.4 Additional analysis and packages
We identified plant establishment and dune-building and promoting landscape features using the k-means clustering algorithm. Variables included plant establishment success, dune height relative to the surroundings, height above sea level, slope, and distance to the coastline at average high tide (all measured at the onset of winter). The distance between plots and the coastline was determined from satellite imagery. The slopes were calculated as the difference in height between two plot corner points divided by their distance.
Statistical analysis and data processing were conducted in R version 4.2.3 (R Core Team, 2023). The experimental sampling design was created with BalancedSampling (Grafström et al., 2024). GAMs were fitted using the mgcv package (Wood, 2017). The adequacy of the model assumptions was evaluated using simulated residual diagnostics with the DHARMa package (Hartig, 2022). Post hoc Wald tests for differences between plant material treatments were performed using the itsadug package (van Rij et al., 2022). Figures and maps were generated using ggplot2 and sf (Pebesma, 2018; Wickham, 2016). For spatial interpolations of plant presence/absence and shoot numbers, we used the akima package (Akima and Gebhardt, 2022).
3.1 Arrival limitations
Mapped spontaneous seedling occurrences showed distinct spatial patterns for both beach sections (Fig. 2). The majority of seedlings occurred near adult grasses, with 69 % (Sand Engine) and 84 % (Midsland, Terschelling) observed within a radius of 2.5 m from adults. The seedling occurrence was generally lower near the sea. On the Sand Engine, the occurrence was also concentrated around the dune lake, with a notable presence of seedlings even at considerable distances from existing adult vegetation.
The shoot and seedling emergence patterns in the establishment experiment showed less spatial variation than the natural seedling occurrences. Shoot emergence was also observed in areas with only a few naturally occurring seedlings, suggesting that dispersal of plant material is likely a limiting factor under natural conditions.
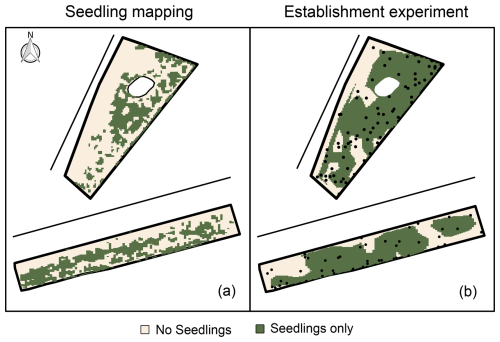
Figure 2Spatial patterns in dune‐building grass occurrence (not vegetation cover) on the Sand Engine (upper) and at Midsland (lower). The lines mark the approximate coastline at average high tide. (a) Natural occurrence from seedling mapping (July 2021). (b) Presence of shoots from seeds per block from the establishment experiment (August 2022). The black points show the locations of the experimental blocks.
3.2 Establishment success
During the course of the establishment experiment, grass shoots were observed in 36 % of plots, with significantly higher shoot numbers in plots with introduced plant material compared to control plots (average treated: 48±130 shoots m−2 vs. average control: 6±30 shoots m−2, pairwise comparison with Wilcoxon rank-sum test, p<0.001). The number of shoots over time increased with precipitation, but decreased with high burial rates. Shoot numbers generally increased in summer but decreased during periods of low precipitation and at the last monitoring moment (see Fig. S12).
The establishment experiment covered a wide range of environmental conditions and variability. The change in bed level ranged from −52 to +84 cm (average: cm) with an average burial amount of 6.7±7.9 cm and an average erosion amount of 5.4±5.6 cm. Soil moisture ranged from 1 % to 49 % (average: 9.3±9.4 %), and salinity ranged from 0 to 7001 mS m−1 (average: 299±613 mS m−1). Shoots were observed almost across the entire range of measured soil moisture (1 %–46.1 %), in lower salinity ranges (0–2042 mS m−1), and in lower ranges of bed level change (−27 cm to +27 cm).
Establishment success was influenced by the average abiotic conditions recorded during the monitoring period, both of which showed clear spatial dependencies (Fig. 3a). Specifically, establishment success was notably higher at locations below 2.4 m elevation, reaching an average of 10 % compared to 5.6 % at higher elevations (Fig. 3a). These low elevations coincided with high average soil moisture (15.4 % ± 12.6 % vs. 5.9 % ± 4 % at elevations above 2.4 m) and limited changes in bed level. Furthermore, no establishment was recorded for average changes in bed level of ± 10 cm (Fig. 3a).
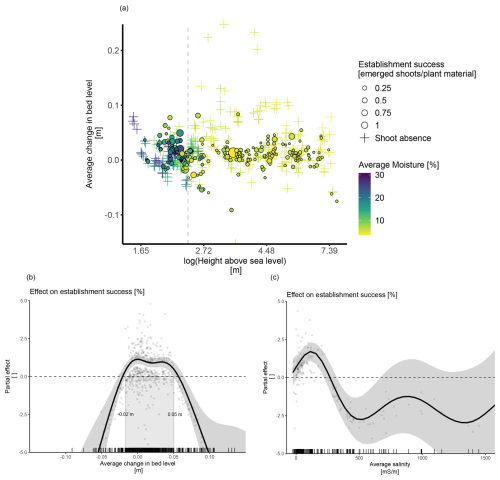
Figure 3The effect of abiotic conditions on plant establishment success at the onset of winter (January–February 2023). (a) Establishment success across an elevation gradient in relation to average soil moisture and bed level change. The dashed gray line marks a change from dry to wet conditions which occurs at approximately 2.4 m. (b) The model‐predicted average partial effect of the change in bed level on plant establishment success. (c) The model-predicted average partial effect of average salinity on plant establishment success. Note that the salinity effect was modeled for a conditional dataset with fewer observations (N=173).
The statistical analysis confirmed a significant effect of the average change in bed level on establishment success, with the success being highest between 2 cm of erosion and 5 cm of burial, regardless of the species (Fig. 3 b). We also found a significant generalized effect of average salinity on establishment success, with success being negatively affected by salinity above 340 mS m−1 (Table 4 and Fig. 3c).
Table 4Statistical model results of the relationship between establishment success (shoot number/introduced plant material) and biotic and average abiotic predictors.
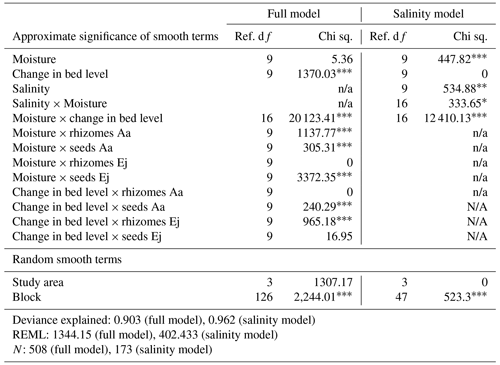
Note: We used the average bed level change and average recorded moisture per plot recorded during the monitoring period (March 2022–January 2023). The complexity of the model which includes average salinity was reduced as less salinity data were available. Ej represents Elytrigia juncea, and Aa represents Ammophila arenaria. p<0.001 significant level. p<0.01 significant level. * p<0.05 significant level.
We did not find a significant generalized effect for moisture, but significant interactions with bed level and treatment were found (Table 4). However, the nature of these interactions was not always clear, probably because of limited amounts of data in specific ranges. Nevertheless, it might be interesting to note that the seeds of Elytrigia and the rhizomes of Ammophila showed a positive response, with a suggested optimum within the range of 15 %–20 % of average moisture.
The establishment success also differed between the plant species and diaspore. In our establishment experiment, seeds of Elytrigia showed the highest average establishment success (13.4 %), followed by rhizome pieces of Ammophila (9.0 %) and then rhizomes of Elytrigia (6.6 %), though none significantly differed from the other (W(2)=0–0.471, p>0.1). In contrast, seeds of Ammophila had a significantly lower establishment success than the other treatments (1 %, W(2)=102–158.6, p<0.001).
3.3 Dune initiation
Dune initiation was first observed during the second monitoring moment in August 2022 on all four beach sections. In subsequent campaigns, dune formation was recorded in 11 %–12 % of all plots, during which the newly developed dunes showed an average trend of accretion. Most dunes were initiated from plots with introduced Elytrigia seeds (57 %), while 12 %–19 % formed in plots where rhizomes and seeds of Ammophila had been introduced, respectively. Rhizomes of Elytrigia had the smallest contribution, with 7 %–8 % of dunes occurring depending on the monitoring moment. The average height of the dunes reached 7.4 ± 3.4 cm relative to the surroundings and did not exceed 19 cm during the course of the experiment.
We found dune initiation significantly explained by the number of emerged shoots (p<0.001, χ2=77.8). A significant positive effect was observed when the number of shoots reached 42 shoots m−2 with the probability of dune formation increasing almost exponentially, reaching the highest probability at 330 shoots m−2 (Fig. 4a). Furthermore, the interaction between shoot number and bed level change was significant (p<0.01, χ2=17.46), with higher shoot numbers and accretion leading to the highest probability of dune formation and erosion leading to a reduced probability of dune initiation (Fig. 4b).
Neither moisture (p=0.06, χ2=30.61) nor interactions with the moisture term were statistically significant (for more details, see Table S6). However, when looking at the combined model predictions of establishment success and dune formation probabilities, we found that the probability of dune formation is highest when soil moisture is high and the change in bed level is within the tolerance level of the dune‐building plants (Fig. 4c).
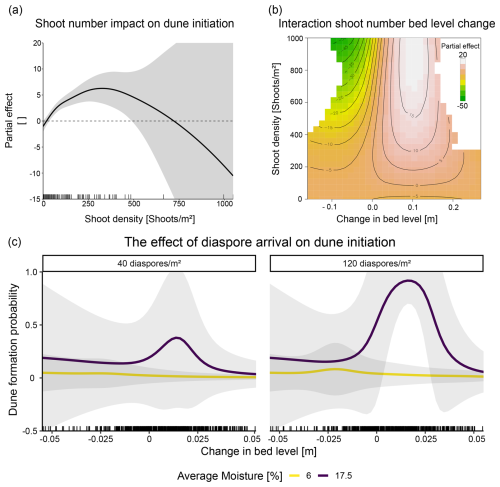
Figure 4Dune initiation probability in relation with abiotic and biotic conditions. (a) The effect of shoot numbers on the dune initiation probability. (b) The interaction effect between shoot number and change in bed level (as measured in two plot corners) on dune initiation probabilities. Areas with insufficient data amounts were excluded based on a distance threshold of 0.3 (blank regions of the plot). (c) Bed level change and moisture effects on dune initiation. For the predictions, shoot numbers were averaged over all treatment combinations. For the moisture levels, we used 6 % (average moisture at locations higher than 2.4 m) and conditions at 17.5 % moisture for which the statistical model and the raw data suggest optimal conditions.
3.4 Hot spots for dune development on the beach
Dunes that persisted until the onset of winter (January/February 2023) tended to occur at locations where a lot of shoots also persisted (Fig. 5). Spatial patterns of dune formation further showed that at Midsland and Formerum, more dunes persisted closer toward the land side, though such a trend was not visible on the Sand Engine or Oosterend. Although the patterns of dune formation until the onset of winter largely coincided with high shoot numbers, some of the observed dunes occurred as remnants of earlier dune formation where the shoots had already died off.
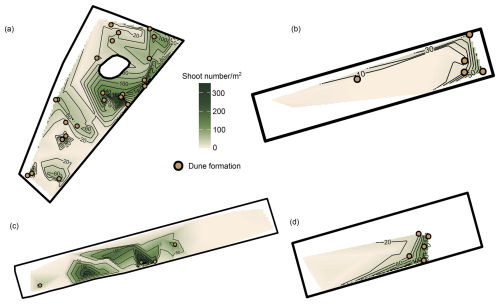
Figure 5Patterns of dune development and shoot numbers at the onset of winter on four beach sections. (a) Sand Engine. (b) Oosterend. (c) Midsland. (d) Formerum. Note that for the interpolation, shoot numbers were summed up per block location. Orange points show locations with observed dune development; darker green colors indicate higher shoot numbers.
Dune development and high shoot numbers coincided with an average distance from the coastline of 279 m (SD ± 100 m), moderate heights above sea level (avg. 2.92 m, SD ±1.05 m), and moderate slopes (avg. 6 %, SD ± 6.4 %).
In this study, our aim was to narrow down the drivers of the of establishment of two major dune-building grasses (Ammophila arenaria and Elytrigia juncea) and subsequent dune initiation. Given the expectation that arrival limitations and environmental conditions can act simultaneously (Maun, 2009), we introduced plant material at multiple beach sites on a large scale. This approach uniquely allowed us to isolate environmental constraints from arrival limitations, enabling a clearer assessment of establishment success.
In accordance, we found dispersal to new locations to be a first important barrier, limiting spontaneous establishment to locations downwind from the sea near existing vegetation. In turn, average bed level changes between −2 cm and 5 cm had a positive impact on the success of plant establishment (ca. −9.3 to +23.25 cm yr−1), with salinity limiting establishment success and moisture playing a key role for subsequent shoot growth. Finally, the initiation of a dune was most probable when the shoot densities reached 330 shoots m−2, suggesting that conditions for dune initiation are more restrictive than for plant establishment. The optimal locations for dune initiation were typically restricted to the middle of the beach, with high establishment success being associated with an average distance to the sea of 279 m for both study areas. Our results provide valuable insights for coastal management strategies. In the following, we discuss our results in more detail.
4.1 Arrival and success of plant material
Our results indicate that the arrival of plant material to new locations can be an important limiting factor for seedling establishment; 69 %–84 % of the spontaneous seedlings occurred in close proximity to existing adult vegetation. Although seedling establishment may occasionally have been mistaken for establishment from rhizome fragments, it is important to note that both seedling and rhizome fragment establishment often occurred side by side within the same plot. Therefore, arrival limitations may also be similar for the rhizome pieces. In fact, we did not observe any rhizome fragments or adult vegetation at certain sections of both beaches. Another reason for finding many seedlings close to adults could be positive biotic feedbacks. However, grass adults may also inhibit seedling recruitment through habitat modifications (Lammers et al., 2024b), which contrasts with biotic facilitation. Although simply recording the occurrence of seedlings does not allow us to draw conclusions about environmental limiting conditions, pathways for arrival, or the amount of plant material arrived, the presence of arrival limitations was further supported by results from the establishment experiment. We found more establishment in plots with introduced plant material compared to control plots, indicating that the amount of plant material that arrives in certain areas is smaller under natural conditions.
It is important to differentiate dispersal mechanisms for both types of diaspores. Wind, capable of carrying seeds over long distances, offers a high potential for colonization of new areas. On the Sand Engine, we observed considerable colonization near the dune lake, even at larger distances from adult plants (Fig. 2), likely due to prevailing southwest winds. However, unfavorable wind directions may limit seed arrival, dispersing them landward instead of onto the beach (Hilton et al., 2019; Lammers et al., 2024b). While wind dispersal is important for seeds (Huiskes, 1979) and for rhizome pieces, storms are crucial as they detach rhizome fragments from adult plants. The seeds and rhizome pieces may then be dispersed by seawater to new areas over large distances and especially deposited along wrack lines, facilitating plant establishment and dune formation within areas of accumulated wrack material (Aptekar and Rejmánek, 2000; de la Peña et al., 2011; Davy and Figueroa, 1993; Hesp, 1989; Costas et al., 2024). Moreover, the dispersal potential may vary between species. Ammophila seeds are typically dispersed as single spikelets each containing only one floret (Huiskes, 1979). Although Elytrigia also disperses its seeds in spikelets, they typically contain three to five florets attached together. These spikelets have a higher weight and length (Table 3) compared to spikelets of Ammophila. On the other hand, Ammophila generally has larger rhizome pieces compared to Elytrigia (see Fig. S3). These differences in size and weight may potentially affect their transport by wind and water. However, the extent to which diaspore size influences arrival limitations requires further research.
Differences in size may also explain differences in overall establishment success between treatments. For instance, seeds with a higher mass tend to produce larger seedlings that establish more successfully (Maun, 2009). In fact, the observed average establishment success followed the order of the size of the plant material, with the larger rhizome fragments of Ammophila having a higher average establishment success compared to Elytrigia rhizomes, and bigger seeds of Elytrigia having a higher establishment success compared to seeds of Ammophila. Compared individually, seeds of Ammophila performed significantly worse compared to rhizome fragments, while seeds of Elytrigia performed similarly compared to rhizome fragments or even slightly better. This aligns with patterns found in previous studies of these two species (Harris and Davy, 1986a; van der Putten, 1990). This direct comparison reveals an advantage of the pioneer species Elytrigia over Ammophila. Although both species can rely on rhizome fragments and clonal expansion for establishment (Reijers et al., 2020; Keijsers et al., 2015a), seed-based establishment is rare for Ammophila (van der Putten, 1990), and based on our findings we expect it to occur much less frequently than in Elytrigia. The ability to establish spontaneously from seeds may give Elytrigia a competitive advantage in highly disturbed environments, possibly partly explaining its closer distribution to the sea compared to Ammophila (Reijers et al., 2020; van Puijenbroek et al., 2017c). This may also contribute to its initial dominance during dune initiation compared to later successional species (Costas et al., 2024), which often show lower seed establishment success (Maun, 2009).
Finally, as seed size interacts with both establishment success and dispersal potential, it remains unclear how these factors might confer establishment advantages. Future studies tracking seed or rhizome fragment dispersal within the dune system could clarify these dynamics and their impact on species distribution and subsequent dune initiation.
4.2 Establishment success in response to environmental drivers
We showed that key environmental factors, such as salinity, soil moisture, and bed level changes, significantly influence plant establishment success after the arrival of seeds and rhizome fragments.
The change in bed level appears to be very important, showing both a generalized and a treatment-specific effect (see Table 4). The average change in bed level had a positive impact on the establishment success within a range between 2 cm of erosion and 5 cm of burial (see Fig. 3b). At higher average bed level changes, establishment success was negatively influenced, and shoots were absent when the average bed level change reached more than ± 10 cm (ca. ± 46.5 cm yr−1) (see Fig. 3a). The patterns we observed in the field are similar to previous studies that report the impact of partial or complete burial on the establishment of Elytrigia and Ammophila. Although these studies found that limited amounts of burial have a positive effect on shoot emergence, survival, and growth (Konlechner et al., 2013; Ievinsh and Andersone-Ozola, 2020; Lammers et al., 2024b; Harris and Davy, 1986b), establishment may cease when certain amounts of burial are reached. No more shoot emergence occurred for Ammophila seeds at burial depths of 9–10 cm (Lammers et al., 2024b; Bonte et al., 2021; Lim, 2011), and shoot emergence was restricted to a burial depth of 12.7 cm for Elytrigia (Harris and Davy, 1986b). Rhizome fragments were reported to be more tolerant to complete burial. Shoot emergence occurred at a maximum burial depth of 35 cm for Ammophila (Konlechner et al., 2013) and of 17.8 cm for Elytrigia (Harris and Davy, 1986b). After shoot emergence, burial reportedly also affects survival. Survival of Ammophila shoots (emerged from the rhizomes) was markedly reduced when buried by 80 % of their height (ca. 4–13.6 cm) (Konlechner et al., 2013); similarly, survival was reduced when shoots of Ammophila that had emerged from seeds were buried by 60 % (Ievinsh and Andersone-Ozola, 2020). In contrast to studies under controlled conditions, field studies reported changes in bed level greater than 5–10 cm to restrict the establishment of Elytrigia and Ammophila from seeds and rhizome fragments (Lammers et al., 2024b; Harris and Davy, 1986a; Lammers, 2024), showing similarities to the numbers found in this study. Moreover, our results suggest erosion to be a stronger limiting factor in establishing dune-building grasses compared to burial. A negative effect of erosion occurred already at 2 cm, which coincides with the initial depth of plant material burial. This indicates that, following the dispersal of plant material, some burial may be needed for successful establishment. However, it appears that early-establishing plants may also withstand limited amounts of erosion beyond this level (see Fig. 3a and b). This limited tolerance of juvenile dune-building grasses to erosion has not been previously described to the best of our knowledge.
In the establishment experiment, average salinity negatively affected establishment success when 340 mS m−1 was exceeded and reached the lowest establishment success at around 500 mS m−1 of average salinity. Shoots were not observed when the salinity exceeded 2000 mS m−1. Similar to the studies on burial, only a limited number of field studies have been carried out on the effect of salinity on the establishment of Elytrigia and Ammophila. Insights from controlled experiments suggest that both species are vulnerable to salinity during early establishment phases, although slightly higher tolerances to salinity have been reported compared to our field study. Germination experiments reveal Elytrigia to negatively respond to salinity values of 500 mS m−1 (El-Katony et al., 2015), yet a considerable reduction only occurs when salinity exceeds about 1250–2000 mS m−1 with less than 10 % of seeds germinating at 2500–3000 mS m−1 (El-Katony et al., 2015; Del Vecchio et al., 2020). Similarly, Ammophila responds negatively to salinity values of about 500–1000 mS m−1 with less than 15 % germinating at salinity values of approximately 2050 mS m−1 (Chergui et al., 2013). Much less appears to be known about the effect of salinity on Elytrigia and Ammophila during later establishment phases, although the shoots of Ammophila established from rhizome fragments tended not to survive when submerged in seawater (Konlechner et al., 2013).1
Soil moisture influenced establishment success in the form of treatment-specific responses (Table 4). Moreover, we found higher average establishment successes in locations with higher soil moisture. These locations coincided with lower elevations. According to Lammers et al. (2024b), locations below 2.4 m above sea level offered both higher establishment success and soil moisture, suggesting a positive influence of groundwater and limited susceptibilities to fluctuations in precipitation (Homberger et al., 2024).
Even though soil moisture can be expected to especially influence the early establishment of dune vegetation (Schat and Van Beckhoven, 1991), only a few studies quantified this response for juvenile Ammophila plants (Chergui et al., 2013; Lammers et al., 2024b; Konlechner et al., 2013), and we were unable to find any reference study for juvenile Elytrigia, indicating a need for further studies. Germination experiments revealed seeds of Ammophila to be quite vulnerable to hydric stress (Chergui et al., 2013). Rhizome fragments, on the other hand, appear to offer some resistance to drought conditions. In a controlled experiment, they were shown to no longer produce shoots when dried for 3 d, while shoots that emerged from rhizome fragments were able to survive for 1–2 weeks even when the soil moisture content reached 0 % (Konlechner et al., 2013). As mature species, Elytrigia and Ammophila respond similarly to soil moisture (Homberger et al., 2024), both showing considerable resistance to drought (Dixon et al., 2004; Homberger et al., 2024). Even younger Ammophila plants (about 8 months old) are reportedly resistant to drought (Lammers et al., 2024a). However, mortality has been observed in mature Ammophila and Elytrigia plants when soil moisture remains below a critical threshold (3.3 %–4.8 %) for prolonged periods of 48 to 90 d (Homberger et al., 2024). Since juvenile grasses may be more vulnerable, it could therefore be expected that establishment may be inhibited when soil moisture reaches certain low thresholds, as well. However, during our field measurements average soil moisture tended to stay above 5 %. Furthermore, rainfall occurred throughout the study period for both study areas, making it unlikely that the plants experienced long periods of drought (see Fig. S12).
Although we found many similarities between our study and previous studies when it comes to species responses to bed level changes, salinity, and soil moisture, a comparison is not straightforward. Only a few studies on Elytrigia and Ammophila establishment have been carried out under field conditions (Lammers et al., 2024b; Konlechner et al., 2013; Harris and Davy, 1986a; Lammers et al., 2024a). Therefore, much of the knowledge about species responses comes from controlled conditions at specific life stages of the species (see Chergui et al., 2013; El-Katony et al., 2015; Del Vecchio et al., 2020; Lim, 2011; Ievinsh and Andersone-Ozola, 2020; Harris and Davy, 1986b; Bonte et al., 2021, and also partly Lammers et al., 2024b; Konlechner et al., 2013). In this study, we did not separate the life stages of the species but rather described establishment success as a result of patterns observed over a longer time in the field. As a result, the plants were exposed to natural conditions that led to realistic spatial patterns in establishment, but some challenges remained. For instance, despite observing treatment-specific responses to environmental drivers (see Table 4), narrowing down the actual tolerance ranges using statistical models and raw data proved challenging despite using a sampling design aimed at covering a wide range of environmental conditions. Although some similarities in species responses can be expected (see somewhat similar responses to salinity during germination, e.g., El-Katony et al., 2015; Del Vecchio et al., 2020; Chergui et al., 2013; similar responses to moisture as adults, e.g., Homberger et al., 2024; and similar responses for seed burial, e.g., Lammers et al., 2024b; Bonte et al., 2021; Lim, 2011; Harris and Davy, 1986b), it may be interesting to further separate species tolerances during different life stages, which may give further insight into their commonly observed distributions. For example, a somewhat higher tolerance to average salinity may be expected when including later development stages as both species are reportedly tolerant to salinity as mature plants, with Elytrigia even responding positively to it (van Puijenbroek et al., 2017c). Here, studies at higher temporal resolution could help untangle different plant life stages.
Although a higher temporal resolution may require reducing the spatial scale compared to this study, future research could also benefit from more frequent monitoring of environmental conditions. In natural settings, sedimentation often fluctuates with time, and previously buried plants can sometimes become uncovered, making it difficult to draw conclusions about plant survival in plots with large fluctuations in sedimentation (Maun, 1994; Harris and Davy, 1986a). Although some of our plots showed consistent trends of accretion or erosion, the temporal resolution of our setup may have been too low to accurately capture the dynamics of plots with large fluctuations. Although we averaged bed level changes to capture broader trends, this may not have addressed all uncertainties, and more detailed, frequent measurements could offer clearer insights into these fluctuations and their effects on plant establishment.
Additionally, plot placement within blocks may have affected wind flow and sediment transport to the downwind plots, as even sparse vegetation can alter shear stress and deposition patterns (Wolfe and Nickling, 1993, 1996). Therefore, some changes in bed level could have resulted from shielding. However, because the width, density, and height of the plants often remained minimal, the influence of the upwind plots on the downwind plots likely remained small (Hesp, 1981; Hesp et al., 2019). Indeed, no encroachment of dune tails into other plots was observed, suggesting that establishment was not hindered in downwind plots. In fact, shielding may have facilitated establishment when sediment burial stayed between 0 to 5 cm on average (see Fig. 3b). Although at the scale of this study a greater separation between plots of a block was not possible, future studies with varying plot distances could better isolate the effect of established upwind plants on downwind plants. If limited amount of burial can be caused by the presence of newly established vegetation and if this indeed increases establishment success of downwind vegetation as suggested here, then this facilitation effect seems to disappear as dune vegetation matures and deposition becomes too high (Lammers et al., 2024b). Although in this study we controlled for these effects through treatment randomization and statistical adjustments, future studies could further investigate facilitation through sedimentation across life stages by taking these interactions into account in the plot design.
Finally, while we looked into three commonly mentioned establishment drivers for dune-building vegetation additional factors have been shown to influence plant establishment such as biotic interactions, nutrient availability and human disturbance (van der Putten et al., 1988; Andersen, 1995; Willis, 1965). Human impacts may especially be interesting as recreation and coastal management regimes can also substantially influence coastal dune development and therefore represents an important avenue for future coastal dune research (Farrell et al., 2023). Moreover, as seen in this study, the combination of multiple stressors may have an interactive effect on establishment. For instance, we found a significant interactive effect of moisture and the change in bed level on the establishment success (see Table 4). Surface moisture can limit the amount of eolian-caused bed level change (Hallin et al., 2023). Indeed, our findings suggest that stable wet conditions (lower locations) had smaller ranges in bed level change, resulting in higher establishment success (see Fig. 3a). However, in lower-lying areas close to the sea, the benefits of wet conditions may be outweighed by increased salinity from the sea, negatively impacting shoot emergence. Future studies that focus on interactions in greater depth could help disentangle the combined effects of various environmental and anthropogenic factors, providing a more comprehensive understanding of what drives successful establishment of dune plants.
4.3 Conditions for dune initiation
Past research on the development of dunes generally focused on more established dunes, with fewer studies focusing on the initiation of the dunes itself (Charbonneau et al., 2021). Although it has been well established that vegetation plays a crucial role in the initiation and development of dunes, previous studies tended to investigate dune initiation with already established (naturally or artificially) plants (Hesp et al., 2019; Charbonneau et al., 2021; Hesp et al., 2021; van Puijenbroek et al., 2017b), which does not allow one to draw conclusions about the conditions that lead to successful establishment. This study aimed to narrow down these conditions, seeking to explain how successful plant establishment can lead to dune initiation.
In accordance, we were able to demonstrate that dune initiation depends on plant material arrival, the characteristics of the arrived plant material (rhizome vs. seeds and species identity), and the environmental conditions the plants encounter after initial establishment. It is important to note that in general, a sufficient supply of sediment is also necessary to initiate the dunes (Hesp et al., 2021; Costas et al., 2024). This study did not focus on sediment supply, yet due to the high number of developed embryo dunes at all four study sections, it can be assumed that the sediment supply was sufficient (Hesp et al., 2021). However, in many plots the amount of observed establishment was too low for the dunes to actually develop. Only when the number of shoots reached 42 shoots m−2 (comparable to a plant cover of 5 %; Hesp et al., 2019) did the probability of dune initiation increase significantly. An optimum was reached at 330 shoots m−2 (a cover of about 40 %; Hesp et al., 2019). Those findings are in line with previous studies that demonstrated the influence of vegetation cover on dune initiation, showing more enduring sedimentation at higher covers (Hesp et al., 2019; Charbonneau et al., 2021; Costas et al., 2024).
Although these relationships between cover and dune initiation seem well established (Hesp et al., 2019; Charbonneau et al., 2021; Costas et al., 2024), factors that lead to a high plant cover development appear to be less understood. As previously suggested, environmental conditions such as stable soil moisture, low salinity, and moderate levels of burial can all favor the emergence of shoots. When these conditions align, the likelihood of successful dune initiation could be expected to increase. However, the role of burial may actually be more complex. On the one hand, sediment burial can stimulate positive growth responses (Ievinsh and Andersone-Ozola, 2020; Nolet et al., 2018; Reijers et al., 2021), while, on the other hand, dense vegetation can improve sediment trapping (Hesp et al., 2019; Charbonneau et al., 2021). As plants grow and mature, their capacity for active sediment trapping may therefore increase, which, in turn, could further stimulate growth, creating a positive feedback loop between sediment trapping and plant development.
Since we did not distinguish between plant life stages, part of the observed establishment success in relation to burial may reflect the growth triggered by sediment trapping rather than burial alone. Nonetheless, other factors appear to be similarly critical for dune initiation. For instance, plots with Elytrigia seeds not only showed the highest establishment success but also the most frequent dune initiation. Given the randomization of treatments, these higher initiation frequencies appear to be more closely related to the high establishment success of this treatment than to preferential sediment accumulation. Thus, while a feedback loop between sediment trapping and growth possibly develops over time, the initial successful establishment of vegetation remains crucial for triggering dune initiation. Future studies with a higher temporal resolution could help more clearly separate early growth responses from the onset of sediment trapping.
Our results show that the initiation and thus recovery of dunes depend on specific plant–environment interactions that promote peaks in establishment. It is important to recognize that sufficient accommodation space is also needed for dune development (Nolet and Riksen, 2019). In fact, this space for the establishment of plants and the development of dunes may be reduced in the future, since sea level rise caused by future climate change may lead to substantial beach erosion (Hesp, 2002; Vousdoukas et al., 2020). Due to their proximity to the sea, coastal dune-building species are very vulnerable to these changes (Garner et al., 2015), and the strong negative response to erosion found in this study would further support this.
Although erosion of beaches and dunes may lead to a natural landward propagation of dune systems (Hesp, 2002; Carter, 1991; Davidson-Arnott, 2005; Davidson-Arnott and Bauer, 2021), the space for such a retreat is often restricted due to the presence of infrastructure (Lansu et al., 2024). In this light, many countries have adopted management strategies to offset problems of sea level rise and beach erosion (Staudt et al., 2021). Foreshore nourishments and similar interventions may explain why globally 74 % of sandy beaches are either stable or accreting despite sea level rise (Gao et al., 2020; Luijendijk et al., 2018). The Netherlands is not an exception here, showing a seaward dune propagation trend at a faster rate than sea level rise (van IJzendoorn et al., 2021), which can be explained by its dynamic coastline preservation strategy with extensive nourishment activities (Luijendijk et al., 2018; Keijsers et al., 2015c; Rijkswaterstaat, 2024).
Although artificially created, these adopted management strategies may favor plant establishment and dune initiation. Nonetheless, insights into arrival limitations and conditions leading to high establishment success could be better integrated to formulate more targeted strategies if dune initiation is desired. For instance, coastal managers could focus on designing landscape features that facilitate establishment and dune formation. Such a design may include creating suitable conditions in the middle section of a wide beach with limited slopes and heights above mean sea level. Wet landscape features such as the dune lake on the Sand Engine can similarly contribute to dune development, as this provides a stable source of plant-available moisture with a lower susceptibility to fluctuations in weather.
On the other hand, coastal managers could incorporate our findings into strategies that promote storm resilience. Following large storm disturbances, dunes and their entire vegetation communities can be removed (Harris and Davy, 1986a), and the recovery of dunes after storms is largely dependent on vegetation recolonization (Houser et al., 2015; Snyder and Boss, 2002). Recovery may take longer without diaspore arrival (e.g., if the dominant wind direction limits seed dispersal on to the beach), when dune-building grasses have to rely on growing back from the foredunes through clonal expansion, which is a localized process (Keijsers et al., 2015a; Reijers et al., 2021). On the other hand, in line with our findings, if favorable environmental conditions arise after storms (e.g., a wet summer), vegetation colonization and dune recovery may be expedited. To help with the recovery and restoration of dunes, vegetation is often planted, though typically focusing on the foredunes and using only one key species (in Europe, typically Ammophila). Since our results showed that Elytrigia is superior in establishing from seeds and tends to initiate more dunes, it might be worthwhile to consider including it for restoration projects, especially if the objective is to accelerate dune formation after storms. Since our study had only a limited timescale and storms were mostly absent, future studies on longer timescales could provide further insight into the relationship between plant establishment and beach/dune recovery after storms, providing additional recommendations for coastal management.
Finally, our results may also inform management interventions with other purposes, such as enhancing embryo dune habitat development or limiting the establishment of dune stabilizers. The latter may be especially interesting for efforts to recover sediment dynamics by creating blowouts or international invasive species eradication programs.
The experimental data that support the main findings of this study can be found on the Data Archiving and Networked Services (DANS)-EASY website (https://doi.org/10.17026/PT/EEZGNY, Homberger et al., 2024).
The supplement related to this article is available online at https://doi.org/10.5194/bg-22-1301-2025-supplement.
JMH: formal analysis, conceptualization, methodology, investigation, visualization, writing (original draft). SvR: conceptualization, methodology, investigation, writing (review and editing). MR: conceptualization, methodology, supervision, writing (review and editing), funding acquisition. JL: conceptualization, methodology, supervision, writing (review and editing), project administration, funding acquisition.
The contact author has declared that none of the authors has any competing interests.
Publisher’s note: Copernicus Publications remains neutral with regard to jurisdictional claims made in the text, published maps, institutional affiliations, or any other geographical representation in this paper. While Copernicus Publications makes every effort to include appropriate place names, the final responsibility lies with the authors.
The authors express their gratitude for the contributions made by Yvonne Feijen, Peter van Rosmalen, Femke van Beersum, Sten Tokens, Elise Poldervaart, and Ke Chen in setting up the establishment experiment. Additionally, they thank Myrthe Bouma and Rosanne Erhardt for their assistance with additional data collection. The authors also thank Dries Bonte and two additional anonymous reviewers for their valuable feedback and constructive comments, which greatly improved the quality of the paper.
This research has been supported by the Nederlandse Organisatie voor Wetenschappelijk Onderzoek (grant no. 17064).
This paper was edited by Sara Vicca and reviewed by Dries Bonte and two anonymous referees.
Akima, H. and Gebhardt, A.: akima: Interpolation of Irregularly and Regularly Spaced Data, CRAN [code], https://CRAN.R-project.org/package=akima (last access: 19 November 2024), 2022. a
Andersen, U. V.: Resistance of Danish coastal vegetation types to human trampling, Biol. Conserv., 71, 223–230, https://doi.org/10.1016/0006-3207(94)00031-K, 1995. a
Aptekar, R. and Rejmánek, M.: The effect of sea-water submergence on rhizome bud viability of the introducedAmmophila arenaria and the nativeLeymus mollis in California, J. Coast. Conserv., 6, 107–111, https://doi.org/10.1007/BF02730474, 2000. a
Arens, S. M., Mulder, J. P., Slings, Q. L., Geelen, L. H., and Damsma, P.: Dynamic dune management, integrating objectives of nature development and coastal safety: Examples from the Netherlands, Geomorphology, 199, 205–213, https://doi.org/10.1016/j.geomorph.2012.10.034, 2013. a
Bakker, J. P.: Phytogeographical Aspects of the Vegetation of the Outer Dunes in the Atlantic Province of Europe, J. Biogeogr., 3, 85–104, https://doi.org/10.2307/3038138, 1976. a, b, c, d
Bonte, D., Batsleer, F., Provoost, S., Reijers, V., Vandegehuchte, M. L., Van De Walle, R., Dan, S., Matheve, H., Rauwoens, P., Strypsteen, G., Suzuki, T., Verwaest, T., and Hillaert, J.: Biomorphogenic Feedbacks and the Spatial Organization of a Dominant Grass Steer Dune Development, Front. Ecol. Evol., 9, 761336, https://doi.org/10.3389/fevo.2021.761336, 2021. a, b, c, d, e, f
Carter, R. W. G.: Near-future sea level impacts on coastal dune landscapes, Landscape Ecol., 6, 29–39, https://doi.org/10.1007/BF00157742, 1991. a
Castelle, B., Bujan, S., Ferreira, S., and Dodet, G.: Foredune morphological changes and beach recovery from the extreme 2013/2014 winter at a high-energy sandy coast, Mar. Geol., 385, 41–55, https://doi.org/10.1016/j.margeo.2016.12.006, 2017. a
Charbonneau, B. R., Dohner, S. M., Wnek, J. P., Barber, D., Zarnetske, P., and Casper, B. B.: Vegetation effects on coastal foredune initiation: Wind tunnel experiments and field validation for three dune-building plants, Geomorphology, 378, 107594, https://doi.org/10.1016/j.geomorph.2021.107594, 2021. a, b, c, d, e
Chergui, A., El Hafid, L., and Melhaoui, M.: The effects of temperature, hydric and saline stress on the germination of marram grass seeds (Ammophila arenaria L.) of the SIBE of Moulouya embouchure (Mediterranean – North-eastern Morocco), Research Journal of Pharmaceutical, Biological and Chemical Sciences, 4, 1333–1339, 2013. a, b, c, d, e, f, g
Conrad, O., Bechtel, B., Bock, M., Dietrich, H., Fischer, E., Gerlitz, L., Wehberg, J., Wichmann, V., and Böhner, J.: System for Automated Geoscientific Analyses (SAGA) v. 2.1.4, Geosci. Model Dev., 8, 1991–2007, https://doi.org/10.5194/gmd-8-1991-2015, 2015. a
Costas, S., Bon de Sousa, L., Gallego-Fernández, J. B., Hesp, P., and Kombiadou, K.: Foredune initiation and early development through biophysical interactions, Sci. Total Environ., 940, 173548, https://doi.org/10.1016/j.scitotenv.2024.173548, 2024. a, b, c, d, e, f
Davidson-Arnott, R. G. and Bauer, B. O.: Controls on the geomorphic response of beach-dune systems to water level rise, J. Great Lakes Res., 47, 1594–1612, https://doi.org/10.1016/j.jglr.2021.05.006, 2021. a
Davidson-Arnott, R. G. D.: Conceptual Model of the Effects of Sea Level Rise on Sandy Coasts, J. Coast. Res., 216, 1166–1172, https://doi.org/10.2112/03-0051.1, 2005. a
Davy, A. J. and Figueroa, E.: The colonization of strandlines, Special Publication-British Ecological Society, 12, 113–128, 1993. a, b
de la Peña, E., Vandegehuchte, M. L., Bonte, D., and Moens, M.: Nematodes surfing the waves: long‐distance dispersal of soil‐borne microfauna via sea swept rhizomes, Oikos, 120, 1649–1656, https://doi.org/10.1111/j.1600-0706.2011.19540.x, 2011. a
Del Vecchio, S., Fantinato, E., Roscini, M., Acosta, A. T. R., Bacchetta, G., and Buffa, G.: The germination niche of coastal dune species as related to their occurrence along a sea–inland gradient, J. Veg. Sci., 31, 1112–1121, https://doi.org/10.1111/jvs.12899, 2020. a, b, c, d
Delta-T Devices Ltd.: User Manual for the WET sensor type WET-2, https://delta-t.co.uk/wp-content/uploads/2019/06/WET-User_Manual_v1.6.pdf (last access: 4 March 2025), 2019. a, b
Dixon, P., Hilton, M., and Bannister, P.: Desmoschoenus spiralis displacement by Ammophila arenaria: the role of drought, New Zeal. J. Ecol., 28, 207–213, 2004. a
El-Katony, T. M., Khedr, A.-H. A.-F., and Soliman, N. G.: Nutrients alleviate the deleterious effect of salinity on germination and early seedling growth of the psammophytic grass Elymus farctus, Botany, 93, 559–571, https://doi.org/10.1139/cjb-2015-0096, 2015. a, b, c, d
Farrell, E. J., Delgado Fernandez, I., Smyth, T., Li, B., and Swann, C.: Contemporary research in coastal dunes and aeolian processes, Earth Surf. Proc. Land., 49, 108–116, https://doi.org/10.1002/esp.5597, 2023. a
Galiforni-Silva, F., Wijnberg, K. M., and Hulscher, S. J. M. H.: On the Relation between Beach-Dune Dynamics and Shoal Attachment Processes: A Case Study in Terschelling (NL), J. Mar. Sci. Eng., 8, 541, https://doi.org/10.3390/jmse8070541, 2020. a, b
Gao, J., Kennedy, D. M., and Konlechner, T. M.: Coastal dune mobility over the past century: A global review, Prog. Phys. Geogr., 44, 814–836, https://doi.org/10.1177/0309133320919612, 2020. a
Garner, K. L., Chang, M. Y., Fulda, M. T., Berlin, J. A., Freed, R. E., Soo-Hoo, M. M., Revell, D. L., Ikegami, M., Flint, L. E., Flint, A. L., and Kendall, B. E.: Impacts of sea level rise and climate change on coastal plant species in the central California coast, PeerJ, 3, e958, https://doi.org/10.7717/peerj.958, 2015. a
Grafström, A. and Tillé, Y.: Doubly balanced spatial sampling with spreading and restitution of auxiliary totals, Environmetrics, 24, 120–131, https://doi.org/10.1002/env.2194, 2013. a, b
Grafström, A., Lisic, J., and Wilmer, P.: BalancedSampling: Balanced and Spatially Balanced Sampling, CRAN [code], https://cran.r-project.org/web/packages/BalancedSampling/index.html (last access: 22 November 2023), 2024. a
Hallin, C., van IJzendoorn, C., Homberger, J.-M., and de Vries, S.: Simulating surface soil moisture on sandy beaches, Coast. Eng., 185, 104376, https://doi.org/10.1016/j.coastaleng.2023.104376, 2023. a
Harris, D. and Davy, A. J.: Strandline Colonization by Elymus Farctus in Relation to Sand Mobility and Rabbit Grazing, J. Ecol., 74, 1045, https://doi.org/10.2307/2260232, 1986a. a, b, c, d, e, f, g, h, i, j
Harris, D. and Davy, A. J.: Regenerative Potential of Elymus Farctus From Rhizome Fragments and Seed, J. Ecol., 74, 1057, https://doi.org/10.2307/2260233, 1986b. a, b, c, d, e
Harris, D. and Davy, A. J.: Seedling Growth in Elymus farctus after Episodes of Burial with Sand, Ann. Bot., 60, 587–593, https://doi.org/10.1093/oxfordjournals.aob.a087482, 1987. a
Hartig, F.: DHARMa: Residual Diagnostics for Hierarchical (Multi-Level/Mixed) Regression Models, CRAN [code], https://CRAN.R-project.org/package=DHARMa (last access: 19 December 2023), 2022. a
Hartigan, J. A. and Wong, M. A.: Algorithm AS 136: A K-Means Clustering Algorithm, Appl. Stat., 28, 100–108, https://doi.org/10.2307/2346830, 1979. a
Hesp, P.: Foredunes and blowouts: initiation, geomorphology and dynamics, Geomorphology, 48, 245–268, https://doi.org/10.1016/S0169-555X(02)00184-8, 2002. a, b, c, d, e, f
Hesp, P. A.: The Formation of Shadow Dunes, SEPM J. Sediment. Res., 51, 101–112, https://doi.org/10.1306/212F7C1B-2B24-11D7-8648000102C1865D, 1981. a, b
Hesp, P. A.: A review of biological and geomorphological processes involved in the initiation and development of incipient foredunes, Proceedings of the Royal Society of Edinburgh, Biol. Sci. Sect. B, 96, 181–201, https://doi.org/10.1017/S0269727000010927, 1989. a, b, c
Hesp, P. A., Dong, Y., Cheng, H., and Booth, J. L.: Wind flow and sedimentation in artificial vegetation: Field and wind tunnel experiments, Geomorphology, 337, 165–182, https://doi.org/10.1016/j.geomorph.2019.03.020, 2019. a, b, c, d, e, f, g, h
Hesp, P. A., Hernández-Calvento, L., Hernández-Cordero, A. I., Gallego-Fernández, J. B., Romero, L. G., Miot da Silva, G., and Ruz, M. H.: Nebkha development and sediment supply, Sci. Total Environ., 773, 144815, https://doi.org/10.1016/j.scitotenv.2020.144815, 2021. a, b, c
Hilton, M. and Konlechner, T.: Incipient foredunes developed from marine-dispersed rhizome of Ammophilia arenaria, J. Coast. Res., 64, 288–292, 2011. a
Hilton, M., Konlechner, T., McLachlan, K., Lim, D., and Lord, J.: Long-lived seed banks of Ammophila arenaria prolong dune restoration programs, J. Coast. Conserv., 23, 461–471, https://doi.org/10.1007/s11852-018-0675-0, 2019. a
Hilton, M. J. and Konlechner, T. M.: A review of the marram grass eradication programme (1999–2009), Stewart Island, New Zealand, 17th Australasian Weeds Conference 2009, Stewart Island, New Zealand, https://caws.org.nz/old-site/awc/2010/awc201013861.pdf (last access: 4 March 2025), 2010. a
Homberger, J.-M.: Data from: Dune establishment drivers on the beach: narrowing down the window of opportunity, DANS Data Station Physical and Technical Sciences [data set], https://doi.org/10.17026/PT/EEZGNY, 2024.
Homberger, J.-M., Lynch, A., Riksen, M., and Limpens, J.: Growth response of dune‐building grasses to precipitation, Ecohydrology, 1–16, https://doi.org/10.1002/eco.2634, 2024. a, b, c, d, e, f, g
Hoonhout, B. and de Vries, S.: Aeolian sediment supply at a mega nourishment, Coast. Eng., 123, 11–20, https://doi.org/10.1016/j.coastaleng.2017.03.001, 2017. a, b, c
Houser, C., Wernette, P., Rentschlar, E., Jones, H., Hammond, B., and Trimble, S.: Post-storm beach and dune recovery: Implications for barrier island resilience, Geomorphology, 234, 54–63, https://doi.org/10.1016/j.geomorph.2014.12.044, 2015. a, b
Huiskes, A. H. L.: The Natural Establishment of Ammophila arenaria from Seed, Oikos, 29, 133–136, https://doi.org/10.2307/3543303, 1977. a, b
Huiskes, A. H. L.: Ammophila Arenaria (L.) Link (Psamma Arenaria (L.) Roem. et Schult.; Calamgrostis Arenaria (L.) Roth), J. Ecol., 67, 363–382, https://doi.org/10.2307/2259356, 1979. a, b, c, d, e, f, g, h
Huisman, B. J., Wijsman, J. W. M., Arens, S. M., Vertegaal, C. T. M., van der Valk, L., van Donk, S. C., Vreugdenhil, H. S. I., and Taal, M. D.: Evaluatie van 10 jaar Zandmotor: Bevindingen uit het Monitoringen Evaluatie Programma (MEP) voor de periode 2011 tot 2021, Tech. rep., Deltares, Wageningen University and Research, Vertegaal, Arens, https://publications.deltares.nl/11201431_003_0016.pdf (last access: 4 March 2025), 2021. a
Ievinsh, G. and Andersone-Ozola, U.: Variation in Growth Response of Coastal Dune-Building Grass Species Ammophila Arenaria and Leymus Arenarius to Sand Burial, Botanica, 26, 116–125, https://doi.org/10.2478/botlit-2020-0013, 2020. a, b, c, d, e, f
Keijsers, J., De Groot, A., and Riksen, M.: Vegetation and sedimentation on coastal foredunes, Geomorphology, 228, 723–734, https://doi.org/10.1016/j.geomorph.2014.10.027, 2015a. a, b, c, d
Keijsers, J. G. S., Giardino, A., Poortinga, A., Mulder, J. P. M., Riksen, M. J. P. M., and Santinelli, G.: Adaptation strategies to maintain dunes as flexible coastal flood defense in The Netherlands, Mitig. Adapt. Strat. Gl., 20, 913–928, https://doi.org/10.1007/s11027-014-9579-y, 2015b. a
Keijsers, J. G. S., Giardino, A., Poortinga, A., Mulder, J. P. M., Riksen, M. J. P. M., and Santinelli, G.: Adaptation strategies to maintain dunes as flexible coastal flood defense in The Netherlands, Mitig. Adapt. Strat. Gl., 20, 913–928, https://doi.org/10.1007/s11027-014-9579-y, 2015c. a
Konlechner, T. M., Hilton, M. J., and Orlovich, D. A.: Accommodation space limits plant invasion: Ammophila arenaria survival on New Zealand beaches, J. Coast. Conserv., 17, 463–472, https://doi.org/10.1007/s11852-013-0244-5, 2013. a, b, c, d, e, f, g, h, i, j, k
Konlechner, T. M., Kennedy, D. M., Cousens, R. D., and Woods, J. L.: Patterns of early-colonising species on eroding to prograding coasts; implications for foredune plant communities on retreating coastlines, Geomorphology, 327, 404–416, https://doi.org/10.1016/j.geomorph.2018.11.013, 2019. a
Lammers, C.: A sandy symphony: Unraveling dune grass interactions and their role in landscape formation, Ph.D. thesis, University of Groningen, https://doi.org/10.33612/diss.938857435, 2024. a
Lammers, C., van de Ven, C. N., van der Heide, T., and Reijers, V. C.: Are Ecosystem Engineering Traits Fixed or Flexible: A Study on Clonal Expansion Strategies in Co-occurring Dune Grasses, Ecosystems, 26, 1195–1208, https://doi.org/10.1007/s10021-023-00826-4, 2023. a, b, c
Lammers, C., Berghuis, P. M., Mayor, A. G., Reijers, V. C., Rietkerk, M., and van der Heide, T.: Extreme heat and drought did not affect interspecific interactions between dune grasses, Estuar. Coast. Shelf Sci., 311, 109020, https://doi.org/10.1016/j.ecss.2024.109020, 2024a. a, b
Lammers, C., Schmidt, A., van der Heide, T., and Reijers, V. C.: Habitat modification by marram grass negatively affects recruitment of conspecifics, Oecologia, 204, 705–715, https://doi.org/10.1007/s00442-024-05525-y, 2024b. a, b, c, d, e, f, g, h, i, j, k, l, m
Lansu, E. M., Reijers, V. C., Höfer, S., Luijendijk, A., Rietkerk, M., Wassen, M. J., Lammerts, E. J., and van der Heide, T.: A global analysis of how human infrastructure squeezes sandy coasts, Nat. Commun., 15, 432, https://doi.org/10.1038/s41467-023-44659-0, 2024. a
Lim, D.: Marram grass seed ecology: the nature of the seed bank and secondary dispersal, Tech. Rep. May, University of Otago, https://ourarchive.otago.ac.nz/esploro/outputs/graduate/Marram-grass-seed-ecology-the-nature/9926481770801891 (last access: 4 March 2025), 2011. a, b, c, d
Luijendijk, A., Hagenaars, G., Ranasinghe, R., Baart, F., Donchyts, G., and Aarninkhof, S.: The State of the World's Beaches, Sci. Rep., 8, 6641, https://doi.org/10.1038/s41598-018-24630-6, 2018. a, b, c, d
Marra, G. and Wood, S. N.: Practical variable selection for generalized additive models, Computational Statistics and Data Analysis, 55, 2372–2387, https://doi.org/10.1016/j.csda.2011.02.004, 2011. a
Martínez, L. M., Gallego-Fernández, J. B., and Hesp, P. A.: Restoration of coastal dunes, Springer Series on Environmental Management, Springer Berlin, Heidelberg, ISBN: 978-3-642-33444-3, https://doi.org/10.1007/978-3-642-33445-0, 2013. a
Martínez, M. L., Maun, M. A., and Psuty, N. P.: The Fragility and Conservation of the World's Coastal Dunes: Geomorphological, Ecological and Socioeconomic Perspectives, in: Biodiversity and conservation, edited by Martínez, M. L. and Psuty, N. P., vol. 171 of Ecological Studies, 355–369, Springer Berlin Heidelberg, Berlin, Heidelberg, ISBN 978-3-540-74001-8, https://doi.org/10.1007/978-3-540-74002-5_21, 2008. a
Maun, M. A.: Adaptations enhancing survival and establishment of seedlings on coastal dune systems, Vegetatio, 111, 59–70, https://doi.org/10.1007/BF00045577, 1994. a, b, c, d
Maun, M. A.: The Biology of Coastal Sand Dunes, Oxford University Press, New York, ISBN 9780198570356, https://doi.org/10.1093/oso/9780198570356.001.0001, 2009. a, b, c, d, e
Nolet, C. and Riksen, M. J. P. M.: Accommodation space indicates dune development potential along an urbanized and frequently nourished coastline, Earth Surf. Dynam., 7, 129–145, https://doi.org/10.5194/esurf-7-129-2019, 2019. a, b
Nolet, C., van Puijenbroek, M. E. B., Suomalainen, J., Limpens, J., and Riksen, M. J. P. M.: UAV-imaging to model growth response of marram grass to sand burial: Implications for coastal dune development, Aeolian Res., 31, 50–61, https://doi.org/10.1016/j.aeolia.2017.08.006, 2018. a, b
Pebesma, E.: Simple Features for R: Standardized Support for Spatial Vector Data, R J., 10, 439–446, https://doi.org/10.32614/RJ-2018-009, 2018. a
Provoost, S., Jones, M. L. M., and Edmondson, S. E.: Changes in landscape and vegetation of coastal dunes in northwest Europe: a review, J. Coast. Conserv., 15, 207–226, https://doi.org/10.1007/s11852-009-0068-5, 2011. a
R Core Team: R: A Language and Environment for Statistical Computing, R Foundation for Statistical Computing, Vienna, Austria, https://www.R-project.org/ (last access: 4 March 2025), 2023. a
Ranasinghe, R.: Assessing climate change impacts on open sandy coasts: A review, Earth-Sci. Rev., 160, 320–332, https://doi.org/10.1016/j.earscirev.2016.07.011, 2016. a
Reijers, V. C., Lammers, C., de Rond, A. J., Hoetjes, S. C., Lamers, L. P., and van der Heide, T.: Resilience of beach grasses along a biogeomorphic successive gradient: resource availability vs. clonal integration, Oecologia, 192, 201–212, https://doi.org/10.1007/s00442-019-04568-w, 2020. a, b, c, d, e
Reijers, V. C., Hoeks, S., van Belzen, J., Siteur, K., de Rond, A. J. A., van de Ven, C. N., Lammers, C., van de Koppel, J., and van der Heide, T.: Sediment availability provokes a shift from Brownian to Lévy‐like clonal expansion in a dune building grass, Ecol. Lett., 24, 258–268, https://doi.org/10.1111/ele.13638, 2021. a, b, c
Rijkswaterstaat: Kustlijnkaarten 2024, https://open.rijkswaterstaat.nl/open-overheid/onderzoeksrapporten/@269594/kustlijnkaarten-2024/ (last access: 4 March 2025), 2024. a
Ruessink, B. G. and Kroon, A.: The behaviour of a multiple bar system in the nearshore zone of Terschelling, the Netherlands: 1965–1993, Mar. Geol., 121, 187–197, https://doi.org/10.1016/0025-3227(94)90030-2, 1994. a
Schat, H. and Van Beckhoven, K.: Water as a stress factor in the coastal dune system, in: Ecological responses to environmental stresses, 76–91, Kluwer Academic Publishers, Dordrecht, ISBN 9789401067577, https://doi.org/10.1007/978-94-009-0599-3_8, 1991. a
Snyder, R. A. and Boss, C. L.: Recovery and stability in barrier island plant communities, J. Coast. Res., 18, 530–536, 2002. a, b, c
Staudt, F., Gijsman, R., Ganal, C., Mielck, F., Wolbring, J., Hass, H. C., Goseberg, N., Schüttrumpf, H., Schlurmann, T., and Schimmels, S.: The sustainability of beach nourishments: a review of nourishment and environmental monitoring practice, J. Coast. Conserv., 25, 34, https://doi.org/10.1007/s11852-021-00801-y, 2021. a
Stive, M. J., de Schipper, M. A., Luijendijk, A. P., Aarninkhof, S. G., van Gelder-Maas, C., van Thiel de Vries, J. S., de Vries, S., Henriquez, M., Marx, S., and Ranasinghe, R.: A New Alternative to Saving Our Beaches from Sea-Level Rise: The Sand Engine, J. Coast. Res., 290, 1001–1008, https://doi.org/10.2112/JCOASTRES-D-13-00070.1, 2013. a
Strypsteen, G., Delgado‐Fernandez, I., Derijckere, J., and Rauwoens, P.: Fetch‐driven aeolian sediment transport on a sandy beach: A new study, Earth Surf. Proc. Land., 49, 1530–1543, https://doi.org/10.1002/esp.5784, 2024. a
Thomas, Z., Turney, C., Palmer, J., Lloydd, S., Klaricich, J., and Hogg, A.: Extending the observational record to provide new insights into invasive alien species in a coastal dune environment of New Zealand, Appl. Geogr., 98, 100–109, https://doi.org/10.1016/j.apgeog.2018.07.006, 2018. a
van der Putten, W. H.: Establishment of Ammophila arenaria (Marram Grass) from Culms, Seeds and Rhizomes, J. Appl. Ecol., 27, 188-199, https://doi.org/10.2307/2403577, 1990. a, b, c
van der Putten, W. H., van Dijk, C., and Troelstra, S. R.: Biotic soil factors affecting the growth and development of Ammophila arenaria, Oecologia, 76, 313–320, https://doi.org/10.1007/BF00379970, 1988. a
van IJzendoorn, C. O., de Vries, S., Hallin, C., and Hesp, P. A.: Sea level rise outpaced by vertical dune toe translation on prograding coasts, Sci. Rep., 11, 12792, https://doi.org/10.1038/s41598-021-92150-x, 2021. a
van Puijenbroek, M. E. B., Limpens, J., de Groot, A. V., Riksen, M. J. P. M., Gleichman, M., Slim, P. A., van Dobben, H. F., and Berendse, F.: Embryo dune development drivers: beach morphology, growing season precipitation, and storms, Earth Surf. Proc. Land., 42, 1733–1744, https://doi.org/10.1002/esp.4144, 2017a. a, b
van Puijenbroek, M. E. B., Nolet, C., de Groot, A. V., Suomalainen, J. M., Riksen, M. J. P. M., Berendse, F., and Limpens, J.: Exploring the contributions of vegetation and dune size to early dune development using unmanned aerial vehicle (UAV) imaging, Biogeosciences, 14, 5533–5549, https://doi.org/10.5194/bg-14-5533-2017, 2017b. a, b, c, d
van Puijenbroek, M. E. B., Teichmann, C., Meijdam, N., Oliveras, I., Berendse, F., and Limpens, J.: Does salt stress constrain spatial distribution of dune building grasses Ammophila arenaria and Elytrichia juncea on the beach?, Ecol. Evol., 7, 7290–7303, https://doi.org/10.1002/ece3.3244, 2017c. a, b, c, d, e
van Rij, J., Wieling, M., Baayen, R. H., and van Rijn, H.: itsadug: Interpreting Time Series and Autocorrelated Data Using GAMMs, CRAN [code], https://cran.r-project.org/web/packages/itsadug/index.html (last access: 23 November 2023), 2022. a
Vousdoukas, M. I., Ranasinghe, R., Mentaschi, L., Plomaritis, T. A., Athanasiou, P., Luijendijk, A., and Feyen, L.: Sandy coastlines under threat of erosion, Nat. Clim. Change, 10, 260–263, https://doi.org/10.1038/s41558-020-0697-0, 2020. a, b
Walmsley, C. A. and Davy, A. J.: Germination characteristics of shingle beach species, effects of seed ageing and their implications for vegetation restoration, J. Appl. Ecol., 34, 131–142, 1997. a
Wickham, H.: ggplot2: Elegant Graphics for Data Analysis, Springer-Verlag New York, CRAN [code], ISBN 978-3-319-24277-4, https://cran.r-project.org/web/packages/ggplot2/index.html (last access: 28 January 2025), 2016. a
Willis, A. J.: The influence of mineral nutrients on the growth of Ammophila arenaria, J. Ecol., 53, 735–745, 1965. a
Wolfe, S. A. and Nickling, W. G.: The protective role of sparse vegetation in wind erosion, Prog. Phys. Geogr., 17, 50–68, https://doi.org/10.1177/030913339301700104, 1993. a, b
Wolfe, S. A. and Nickling, W. G.: Shear stress partitioning in sparsely vegetated desert canopies, Earth Surf. Proc. Land., 21, 607–619, https://doi.org/10.1002/(SICI)1096-9837(199607)21:7<607::AID-ESP660>3.0.CO;2-1, 1996. a, b
Wood, S. N.: Fast Stable Restricted Maximum Likelihood and Marginal Likelihood Estimation of Semiparametric Generalized Linear Models, J. Roy. Stat. Soc. Ser. B, 73, 3–36, https://doi.org/10.1111/j.1467-9868.2010.00749.x, 2011. a
Wood, S. N.: Generalized additive models: an introduction with R, CRC press, Chapman & Hall/CRC Texts in Statistical Science, ISBN: 978-1498728331, 2017. a, b, c, d, e, f
Zuur, A. F., Ieno, E. N., Walker, N., Saveliev, A. A., and Smith, G. M.: Mixed effects models and extensions in ecology with R, Statistics for Biology and Health, Springer New York, New York, NY, ISBN 978-0-387-87457-9, https://doi.org/10.1007/978-0-387-87458-6, 2009. a, b, c
Salinity values were reported in terms of mmol L−1 NaCl, g L−1 NaCl, or electrical conductivity. Values in g L−1 of NaCl were converted to mmol L−1 by multiplying by a factor of 17.1 (assuming a molar mass of NaCl of 58.44 g mol−1). Seawater is reported to have an electrical conductivity of 5000 mS m−1, equivalent to 500 mmol L−1 NaCl (Del Vecchio et al., 2020; van Puijenbroek et al., 2017c). All literature values in the previous paragraphs have been converted to pore water conductivity as measured by the WET‐2 sensor (Delta-T Devices Ltd., 2019), accordingly.