the Creative Commons Attribution 4.0 License.
the Creative Commons Attribution 4.0 License.
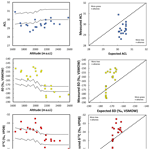
Locally produced leaf wax biomarkers in the high-altitude Areguni Mountains outweigh downstream transport
Alex Brittingham
Michael T. Hren
Samuel Spitzschuch
Phil Glauberman
Yonaton Goldsmith
Boris Gasparyan
Ariel Malinsky-Buller
Sedimentary records of lipid biomarkers such as leaf wax n-alkanes are influenced by not only ecosystem turnover and physiological changes in plants but also earth surface processes integrating these signals into the sedimentary record, though the effect of these integration processes is not fully understood. To determine the depositional constraints on biomarker records in a high-altitude small catchment system, we collected both soil and stream sediments along a 1000 m altitude transect (1500–2500 m a.s.l.) in the Areguni Mountains, a subrange of the Lesser Caucasus Mountains in Armenia. We utilize a treeline at ∼ 2000 m a.s.l., which separates alpine meadow above from deciduous forest below, to assess the relative contribution of upstream biomarker transport to local vegetation input in the stream. We find that average chain length (ACL), hydrogen isotope (δD) and carbon isotope (δ13C) values of n-alkanes are significantly different in soils collected above and below the treeline. However, samples collected from the stream sediments do not integrate these signals quantitatively. As the stream drops below the treeline, the ACL, δD and δ13C values of n-alkanes preserved in streambed sediments reflect a bias toward n-alkanes sourced from trees. This suggests that either (1) there is minimal transportation of organic matter from the more open vegetation at higher elevations or (2) greater production of target biomarkers by trees and shrubs found at lower elevations results in overprinting of stream signals by local vegetation. Though these observations may preclude using n-alkanes to measure past treeline movement in these mountains, δD values of biomarkers in fluvial deposits in these settings are more likely to record local hydrological changes rather than reflect fractionation changes due to turnover in the upstream vegetation structure.
- Article
(2196 KB) - Full-text XML
-
Supplement
(743 KB) - BibTeX
- EndNote
Mountain regions are important hubs for biodiversity and can provide refuge for a number of endemic species of flora and fauna (Antonelli et al., 2018). However, these high-altitude environments are often particularly vulnerable to climate change (Guisan and Theurillat, 2000). Therefore, gaining an understanding of the sensitivity of these regions to past climate change is important for projecting the effects of future climate change on fragile ecosystems. The Caucasus region, covering the republics of Armenia, Georgia and Azerbaijan and parts of the Russian Federation, Türkiye and Iran, in particular has been identified as a biodiversity hotspot that supports a wide variety of plant and animal species (Zazanashvili, 2009; Gasparyan and Glauberman, 2022). To better understand climate and environmental change in both the past and the present, it is necessary to refine our understanding and interpretation of paleoclimate records in this region. Plant wax biomarkers have been used in this region in both geological and archeological contexts to reconstruct past climates; therefore understanding modern variability and transport processes will help refine these interpretations (Brittingham et al., 2019a; Glauberman et al., 2020; Malinsky-Buller et al., 2021, 2024; Trigui et al., 2019). Specifically, we are interested in understanding the sedimentary processes involved in the formation, transport, recycling and accumulation of organic biomarkers in sedimentary archives and assessing whether these archives record a local environmental signal or are a mix of local and transported organic material.
Normal alkanes (n-alkanes) are an important component of the epicuticular wax in terrestrial plants. This waxy coating on plants protects against ultraviolet damage, water loss and predation (Jetter et al., 2006). Specific compounds in this wax, such as n-alkanes, are a useful tool for reconstructing past environmental changes through the analysis of the distribution of alkane homologues as well as their stable hydrogen (δD) and carbon (δ13C) isotope values. Previous research in the Greater and Lesser Caucasus Mountains has documented the applicability of the average chain length (ACL) of leaf wax biomarkers as a tool for differentiating between grassy and deciduous vegetation (Bliedtner et al., 2018; Trigui et al., 2019), although on a global scale ACL does not differentiate well between vegetation types (Bush and McInerney, 2013a).
The carbon isotope (δ13C) value of plant tissue is primarily determined by the photosynthetic pathway of the plant (Diefendorf and Freimuth, 2017). C3 plants, which thrive in areas with cooler growing season temperatures, have more negative δ13C values than do C4 plants, which thrive in warmer growing season temperatures (Ehleringer et al., 1977). C3 vegetation is further influenced by water use efficiency, as water stress influences the intercellular-to-ambient ratio of CO2 in plants (Farquhar et al., 1982). δ13C values in lipids generally follow the same trends, and C3 plants have more negative δ13C lipid values than C4 plants (Diefendorf and Freimuth, 2017). However, the carbon fractionation of lipids is not consistent in different classes of plants (Diefendorf et al., 2011; Pedentchouk et al., 2008; Sikes et al., 2013). Currently, C4 vegetation makes up around 3 % of plant species in Armenia (Rudov et al., 2020) and was present in the Kalavan region during the Holocene (Tornero et al., 2016).
The hydrogen isotope (δD) values of n-alkanes in terrestrial plants record the δD values of environmental water (Sachse et al., 2012). This is typically reflective of δD values in precipitation, though precipitation δD values can also undergo positive shifts due to soil evaporation. The δD values of plant waxes are also influenced by fractionation during the biological synthesis of lipids, which imparts a strong negative fractionation on δD values, as well as transpiration of leaf water (Gamarra et al., 2016). The fractionation between meteoric water and lipids is typically larger in gymnosperms than in angiosperms (Oakes and Hren, 2016; Pedentchouk et al., 2008).
Despite the benefits of measuring δD and δ13C values in n-alkanes for understanding environmental and hydrological processes, not all the processes modifying isotope values from plant to n-alkane deposition are well understood. Sedimentary integration is one of the most poorly understood aspects of this process (Sachse et al., 2012). A number of studies on the integration of leaf waxes in catchments have been published in recent years which help clarify these processes (Alewell et al., 2016; Feakins et al., 2018; Häggi et al., 2016; Hemingway et al., 2016; Ponton et al., 2014; Suh et al., 2019). Previous studies on the integration of organic biomarkers have produced mix results, with some demonstrating spatial integration of catchment signals (Alewell et al., 2016; Feakins et al., 2018; Hemingway et al., 2016), whereas others did not observe this (Häggi et al., 2016; Ponton et al., 2014). However, these previous studies typically focused on very large river systems, which undergo transport processes different than those of the first-order streams analyzed in this study. A number of these studies (Alewell et al., 2016; Feakins et al., 2018; Hemingway et al., 2016; Ponton et al., 2014) also observed seasonal differences in biomarker load in river sediments.
Thus, the sedimentary processes involved in the formation, transport, recycling and accumulation of organic biomarkers in first- and second-order streams are not well understood. One challenge in assessing these processes in small streams is that the environment and plant communities are often homogenous, and thus it is not possible to differentiate between local and upstream transported organic material. To better understand transport processes affecting organic material in small catchments, we studied a set of streams in the Dany River, a tributary of the Barepat River, located in the Areguni Mountains in the Lesser Caucasus range. This stream system is divided into two distinct ecological regions by the treeline (at ∼ 2000 m a.s.l.), which separates alpine meadow above the treeline (2000–2500 m a.s.l.) from deciduous forest below it (1500–2000 m a.s.l.). To evaluate the input of n-alkanes from upstream transported organic material relative to vegetation near the stream, we collected soil samples on the slopes of the mountains from both above and below the treeline throughout the watershed and sediments deposited in the streambed along an elevation transect. Comparison of the hillside and streambed sedimentary n-alkanes allows for assessment of the input of n-alkanes locally produced by vegetation compared to those transported in stream sediments within the catchment.
An additional motivation of this research is that treelines are a vulnerable feature of higher-altitude environments. Previous research in the Areguni Mountains study area has assessed the relationship between treeline dynamics and climate forcing in the past (Ghukasyan et al., 2010; Malinsky-Buller et al., 2021; Montoya et al., 2013; Tornero et al., 2016). Pleistocene sediments uncovered at archeological sites in the village of Kalavan within this area have the potential to reconstruct this relationship through the analysis of plant wax biomarkers deposited in fluvial sediments. However, in order to reconstruct these systems in the past it is important to understand modern biomarker integration processes in the first- and second-order streams and their potential effects on the sedimentary archives of the Areguni Mountains.
2.1 Sample collection and extraction
Hillslope soil samples were collected in September 2018 along an altitude transect (1500–2500 m a.s.l.) above the Dany River watershed, a first-order tributary of the Barepat River in the Areguni Mountains, Armenia (Fig. 1), which traverses the treeline at ∼ 2000 m a.s.l. Forest vegetation is predominantly oak (Quercus macranthera), beech (Fagus orientalis) and hornbeam (Carpinus orientalis), while the above-treeline alpine meadow is comprised of Heracleum sp. and Senecio sp. (Joannin et al., 2022; Volodicheva, 2002). Soil samples were collected by first clearing the top ∼ 10 cm of soil to remove roots. Streambed sediment samples were collected from the Dany River throughout the altitude transect at intervals of ∼ 100 m in altitude. In all cases, roughly 100 g of sediment were collected for the extraction of n-alkanes. Samples were extracted using a Soxhlet apparatus with 2:1 dichloromethane : methanol for 48 h. Following lipid extraction, n-alkanes were separated from the total liquid extract by passing samples through a column of activated silica gel (1.25 g) in baked Pasteur pipettes with 2 mL hexane (non-polar fraction), 4 mL dichloromethane (slightly polar fraction) and 4 mL methanol (polar fraction). n-alkanes were quantified through the analysis of the hexane fraction. We quantified n-alkanes using a BP-5 column (30 m × 0.25 mm i.d., 0.25 µm film thickness) with He as the carrier (1.5 mL min−1). The oven temperature was set at 50 °C for 1 min, ramped to 180 °C at 12 °C min−1, then ramped to 320 °C at 6 °C min−1 and held for 4 min (Brittingham et al., 2017; Smolen and Hren, 2023). We measured a standard mixture of n-alkanes (C20–C33) of known concentration to correct for mass-dependent response decreases in longer-chain n-alkanes. Odd-over-even predominance (OEP) (Eq. 1) and average chain length (ACL) (Eq. 2) were used to evaluate distributions of n-alkanes (Bush and McInerney, 2013b). We also calculated Paq, an n-alkane proxy to evaluate the possible biomarker contribution of aquatic and emergent plants (Eq. 3) (Ficken et al., 2000).
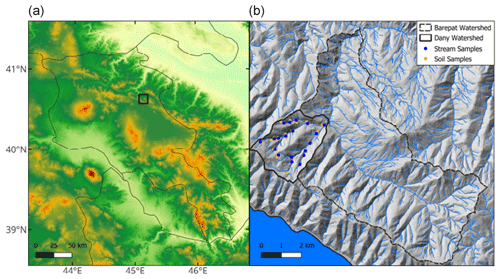
Figure 1(a) Topographic map (Advanced Spaceborne Thermal Emission and Reflection Radiometer Global Digital Elevation Model, ASTER GDEM) of Armenia with an inset map of the sampling location (black box). (b) Inset map of soil (yellow circles) and stream (blue circles) samples collected in the Areguni Mountains, along with the limit of the Barepat (dashed line) and Dany watersheds (solid line).
2.2 Stable isotope analysis
δD and δ13C values of individual n-alkanes were measured with a Thermo Scientific GC IsoLink coupled with a Thermo Scientific MAT 253 isotope ratio mass spectrometer with a BP-5 column (30 m × 0.25 mm i.d., 0.25 µm film thickness). The oven temperature was set at 50 °C for 1 min, ramped to 180 °C at 12 °C min−1, then ramped to 320 °C at 6 °C min−1 and held for 4 min. Internal standards (Mix A5 from Arndt Schimmelman) were run every four samples across a range of concentrations (5–30 V s−1) to correct for size effects. Standard errors were 0.4 ‰ for δ13C and 3 ‰ for δD. Isotope ratio (R) values were converted to δX (δ13C and δD) values (Eq. 3) and are expressed in per mill (‰).
3.1 Alkane abundances
The most abundant alkane homolog in samples collected in the Areguni Mountains is the C29 or C31 alkane, which is typical of terrestrial plants (see Figs. S1–S4 in the Supplement for illustrative chromatograms). Odd-numbered alkanes are significantly more abundant than even-numbered alkanes, and the OEP of all samples is 11.2, with a range from 7.4–18.4. There is no significant difference between the mean OEP of soil (11.1) and stream (11.3) samples in the watershed. These values are similar to those previously measured in the Greater and Lesser Caucasus Mountains (Bliedtner et al., 2018; Trigui et al., 2019).
The mean average chain length (ACL) of all samples averages 29.7, with a range from 28.4 to 31.8 (Fig. 3). In soils above the treeline, the mean ACL value is 30.6 (range of 29.8–31.8). In soils below the treeline, the mean ACL value is 29.5 (range of 28.4–30.4). There is a significant difference between the average ACL values of the n-alkanes in above-treeline and below-treeline soils (Student's t test, p<0.001, n=30). Stream sediments above the treeline have an average ACL value of 29.7 (range of 29.1–30.2), and stream sediments below the treeline have an average ACL value of 29.3 (range of 28.6–30.0). The stream sediments from below the treeline have a significantly (Student's t test, p<0.001, n=21) lower average ACL value than those above the treeline.
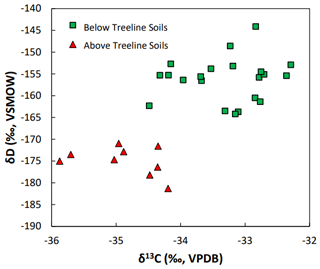
Figure 2The δD and δ13C values of n-alkanes extracted from above-treeline (green squares) and below-treeline (red triangles) sediments. VSMOW: Vienna Standard Mean Ocean Water. VPDB: Vienna Pee Dee Belemnite.
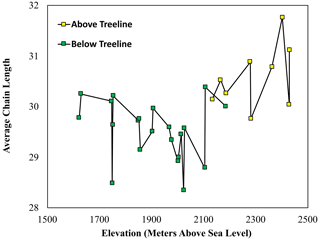
Figure 3The average chain length (ACL) values of n-alkanes extracted from above-treeline (yellow squares) and below-treeline (green squares) sediment across the sampling elevation gradient.
The Paq values of n-alkanes in these samples suggest a mostly terrestrial origin of the organic matter. Higher Paq values indicate contributions of floating and emergent macrophytes. However, we do not find a difference between the Paq values in the stream sediments when compared to the soil samples, indicating that the organic load of the stream sediments is mostly of terrestrial origin. Terrestrial plants have average Paq values of 0.09, with emergent plants averaging 0.25 (Ficken et al., 2000). Only 8 of the 51 samples in this study had Paq values above 0.20, 4 stream and 4 soil samples. This indicates that there was not a significant contribution of aquatic plants in the Dany stream sediments, and the biomarker load is primarily terrestrial in origin.
3.2 δD and δ13C values
The δ13C values in soils and stream sediments collected from the Areguni Mountains reflect a C3 landscape, which is typical in Armenia. δ13C values in all samples ranged from −36.0 ‰ to −32.3 ‰ (Fig. 4). The range is similar for both soil samples (−35.9 ‰ to −32.3 ‰) and stream samples (−36.0 ‰ to −32.5 ‰). However, there is a significant difference in the δ13C values of above- and below-treeline samples, both in the stream and soil samples collected. Above the treeline, δ13C values in soils average −34.9 ‰, and below the treeline soil alkanes average −33.3 ‰ (p<0.0001, Student's t test, n=30; Fig. 2). Stream sediment δ13C values average −35.0 ‰ above the treeline and −33.6 ‰ below the treeline (p<0.0001, Student's t test, n=21). δ13C values in stream samples exhibit a step-like behavior, with a ∼ 2 ‰ shift to more negative values as the stream drops below the treeline.
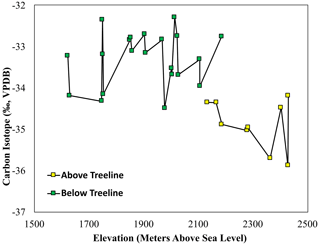
Figure 4The δ13C values of n-alkanes extracted from above-treeline (yellow squares) and below-treeline (green squares) sediment across the sampling elevation gradient.
The δD values measured in soil samples collected in the catchment ranged from −144 ‰ to −185 ‰ (Fig. 5). These values were significantly more negative in above-treeline sediments (−175 ‰) than in below-treeline sediments (−156 ‰) (p<0.001, Student's t test, n=30). δD values were also more negative in stream sediment samples collected above the treeline (−175 ‰) than below the treeline (−158 ‰) (p<0.001, Student's t test, n=21). As with the δ13C values, the δD values of stream sediment samples show a sudden change as the stream drops below the treeline.
4.1 Integration of local and upstream soil n-alkanes into the river sediments
The hillslope soil leaf wax ACL (Fig. 3), δ13C (Fig. 4) and δD (Fig. 5) show a step-like change at the treeline, indicating a significant separation between upstream (above-treeline) and downstream (below-treeline) soils. Using this separation, it is possible to assess the contributions and integration of upstream vs. downstream soils to the streambed sediments along the altitude transect. The step-like transition in streambed δD and δ13C values indicates an overprinting of upstream alkane isotope values by input from deciduous vegetation. Thus, local production largely outweighs upstream transport in this setting. However, to firmly evaluate the upstream and downstream hillslope soil contribution to streambed sediments, there is a need to quantitatively evaluate the area-weighted production of n-alkanes above and below the treeline.
4.2 Modeling n-alkane production and estimating upstream transport and integration
To further evaluate the integration of n-alkanes above and below the treeline, we created a mixing model that calculates the expected δD, δ13C and ACL values at each one of the sampling locations based on the n-alkane production of hillslope sediments above each streambed sampling point (Fig. 6). Our mixing model assumes that the n-alkanes in the river are a function of the weighted n-alkane production above the sampling location.
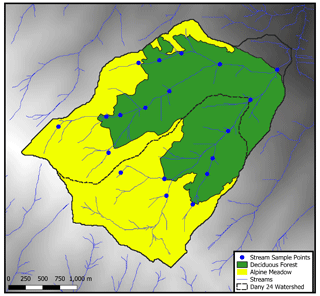
Figure 6Mixing model used to calculate expected values of stream sample points. Upstream watershed area covered by deciduous forest (green) and alpine meadow (yellow) was calculated at each sample location (blue dots). Point watershed for sample 24 (dashed line) is shown here as an example.
The parameters we used for our mixing model are (1) satellite images (Google Earth) to map the areas covered by alpine meadow and forest vegetation throughout the Dany River catchment; (2) an estimate of net primary productivity of organic material production in grasses and trees (grams per area) (Brun et al., 2022); (3) estimates of n-alkane production in grasses and trees in the Greater and Lesser Caucasus Mountains (grams of n-alkane per gram of organic material) (Bliedtner et al., 2018; Trigui et al., 2019); and (4) end-member values of δD, δ13C and ACL derived from the average hillslope soils above and below the treeline. At each sample point within the catchment, we first calculated the upstream area covered by the two dominant vegetation types within the catchment (deciduous forest and alpine meadow) (Fig. 6). This area was then multiplied by the previously mentioned constants (Table 1). By multiplying these terms (area × organic mass production × n-alkane production × end-member soil value), we created an n-alkane production map for the Dany River catchment. Using this method, we calculated the number of grass and tree n-alkanes produced on the hillslopes above the sampling locations and the expected δD, δ13C and ACL values for each stream sampling location (Fig. 7a, c, e).
Table 1Constants used for the mixing model.
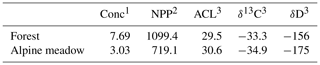
1 n-Alkane concentration in grasses and trees in the Caucasus Mountains (grams of n-alkane per gram of organic material) (Bliedtner et al., 2018; Trigui et al., 2019). 2 Net primary productivity for forest and grassland areas (grams per area) (Brun et al., 2022). 3 Mean values for ACL, δ13C and δD (this study).
We compared the results of our mixing model with the measured δD, δ13C and ACL in the streams. Stream sediment samples collected above the treeline (from ∼ 2000–2600 m a.s.l.) fall within the range of expected values; however, samples below the treeline consistently oversample n-alkanes sourced from below-treeline vegetation. Measured δD, δ13C and ACL values do not have a linear relationship with the expected values based on vegetation area (Fig. 7b, d, f). These measured values would produce underestimates of the upstream area of alpine grasses, yielding incorrect reconstructions of paleovegetation in sedimentary records. Comparing the mixing model with the observations indicates that an area-weighted mixing process is not an adequate model for explaining the n-alkanes signal in the streambed sediments. A simple and straightforward way to interpret this discrepancy is that an area-weighted quantitative integration of n-alkanes is not a good model for describing this catchment system and that local production is much larger than transported organic material.
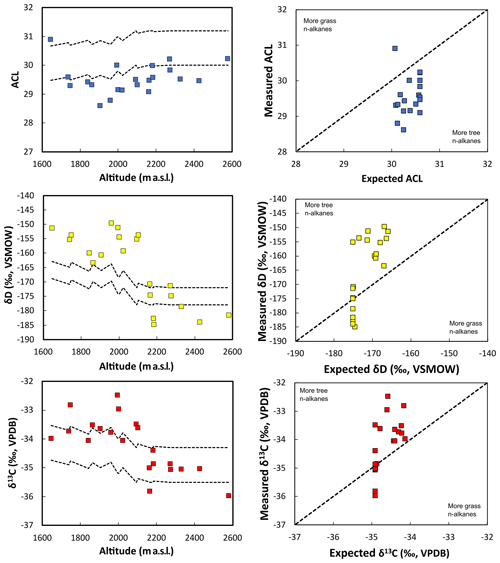
Figure 7Comparison of the measured ACL, δD and δ13C values against expected values of stream sediments. Dashed line represents the range of expected values from stream sediments if vegetation was integrated equally by area.
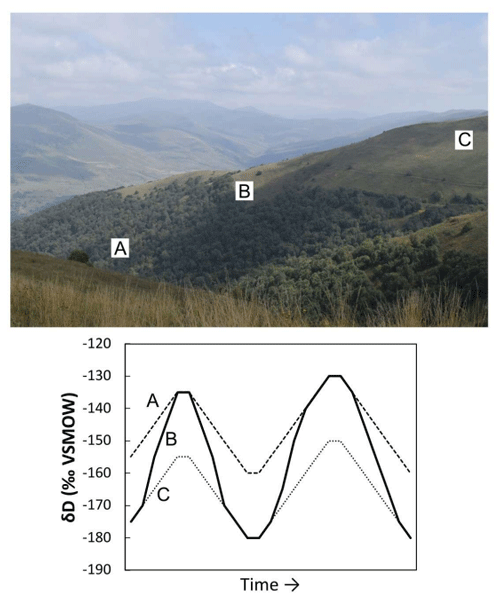
Figure 8A photograph of the Dany watershed with a hypothetical paleoclimate record from three locations: (A, dashed line) below the treeline, (B, solid line) near the treeline with fluctuations in treeline altitude and (C, dotted line) above the treeline.
However, there are still other factors that may be driving this process that our mixing model does not account for. First, the average slope of forested areas in the Dany watershed is higher than those in grassy areas. These steeper slopes would cause more sediment transport into the streambed. Second, although production of n-alkanes in grasses and trees is not significantly different in the Greater and Lesser Caucasus Mountains, concentrations are higher in soils in deciduous areas (Bliedtner et al., 2018; Trigui et al., 2019). This retention of more biomarkers in forest soils would also increase the contribution of deciduous alkanes into the streambed. Third, stream downcutting into older sediments has the potential to re-mobilize stored organic carbon, which may contain a greater load of deciduous n-alkanes. However, analysis of pollen from a lake core nearby (∼ 5 km from the Dany catchment) in the Areguni Mountains shows a gradual shift over the last 4000 years from a grass-dominated landscape to the deciduous forest present today (Joannin et al., 2022b). Therefore, stored biomarkers are more likely to be grass-dominant, and this is unlikely to explain the measured bias to deciduous alkanes.
Since n-alkanes in the first-order stream in this study do not quantitatively integrate n-alkanes based on the upstream area of different vegetation types, this likely precludes the use of n-alkanes as a tool to reconstruct vertical treeline movement in this setting. However, this is a benefit for attempts to reconstruct hydrological changes through the analysis of δD values in n-alkanes. Given the ∼ 20 ‰ difference in apparent fractionation (ε) values for above- and below-treeline sediments, changes in upstream vegetation cover would alter measured δD values in n-alkanes in sedimentary archives. Without this quantitative integration, n-alkanes measured in the Pleistocene sediments found in this watershed are more likely to reflect changes in δD values of precipitation and therefore would serve to reconstruct hydrological cycles, rather than changes in upstream vegetation cover. Since δ13C and the ACL of n-alkanes are also different in above- and below-treeline sediments, these other analyses would also be useful in identifying periods with large changes in the treeline that might complicate interpretation of δD values.
In order to illustrate this point, we present hypothetical records of biomarker δD values from three points in the Dany watershed (Fig. 8), documenting 20 ‰ and 30 ‰ shifts in precipitation δD values. We use δD values of precipitation from water samples collected at the nearest meteorological station with isotope data in Armenia (Dilijan; Brittingham et al., 2019b). Given the lack of quantitative integration in the catchment, a paleoclimate record from either above (A) or below (C) the treeline would record the shift in precipitation δD values. Below-treeline sedimentary records, with the stream organic biomarker load overprinted by local vegetation production, would likely provide a means to reconstruct the δD precipitation values. However, records near the treeline (B) would be influenced by changes in apparent fractionation values associated with changes in vegetation around the stream. Co-occurring climate forcing of shifts in δD values of precipitation and changes in treeline altitude would cause paleoclimate records in this zone to overestimate the magnitude of precipitation δD value shifts.
Sediment and stream samples from the Areguni Mountains, a subrange of the Lesser Caucasus Mountains in Armenia, demonstrate that there is a significant difference in hillslope soil δD, δ13C and ACL values above and below the treeline. n-Alkanes in sediments in the Areguni Mountains can be used to differentiate between the above- and below-treeline sediments. However, n-alkanes extracted from stream sediments reflect their local area rather than demonstrate transport from the higher-altitude alpine meadow. These results complicate attempts to reconstruct changes in past treeline in this mountain range, given that the biomarker load in the stream does not reflect the relative area of different upstream vegetation types. However, these results simplify the interpretation of past n-alkane δD values, as apparent fractionation differences between grasses and trees are less likely to impart a significant influence on δD values in streambed n-alkanes.
All data for this publication can be found in the Supplement.
The supplement related to this article is available online at https://doi.org/10.5194/bg-22-831-2025-supplement.
AB and SS contributed to data acquisition and sample collection. AMB, PG, BG, MTH and AB acquired funding. AB, MTH, YG, AMB and PG contributed to data analysis. All authors contributed to the writing of this manuscript.
The contact author has declared that none of the authors has any competing interests.
Publisher's note: Copernicus Publications remains neutral with regard to jurisdictional claims made in the text, published maps, institutional affiliations, or any other geographical representation in this paper. While Copernicus Publications makes every effort to include appropriate place names, the final responsibility lies with the authors.
We would like to thank the villagers of Kalavan for their help, support and hospitality, especially the Ghukasyan family for providing us a home away from home. We also thank Suren Kesejyan, Hovhannes Partevyan and Vardan Stepanyan. We would like to thank Joseph B. Novak and an anonymous reviewer for their helpful comments on the manuscript.
The research project in Kalavan was funded by the support of the Gerda Henkel Stiftung (grant nos. AZ 10_V_17 and no. AZ 23/F/19) and the Leakey Foundation. Alex Brittingham received funding from the Lady Davis Foundation, the Fritz Thyssen Foundation (grant awarded for the project Pleistocene Hunter-Gatherer Lifeways and Population Dynamics in the Ararat (paleo-lake) Depression, Armenia) and the European Research Council (Investigating Pleistocene population dynamics in the Southern Caucasus; grant no. 948015 awarded to Ariel Malinsky-Buller). Further support was provided by the Areni-1 Cave Consortium (Areni-1 Cave Scientific-Research Foundation, Armenia; Gfoeller Renaissance Foundation, USA), as well as the Institute of Archaeology and Ethnography of the National Academy of Sciences of the Republic of Armenia (supported by the Higher Education and Science Committee, Republic of Armenia; grant no. 21AG-6A080).
This paper was edited by Petr Kuneš and reviewed by Joseph B. Novak and one anonymous referee.
Alewell, C., Birkholz, A., Meusburger, K., Schindler Wildhaber, Y., and Mabit, L.: Quantitative sediment source attribution with compound-specific isotope analysis in a C3 plant-dominated catchment (central Switzerland), Biogeosciences, 13, 1587–1596, https://doi.org/10.5194/bg-13-1587-2016, 2016.
Antonelli, A., Kissling, W. D., Flantua, S. G. A., Bermúdez, M. A., Mulch, A., Muellner-Riehl, A. N., Kreft, H., Linder, H. P., Badgley, C., Fjeldså, J., Fritz, S. A., Rahbek, C., Herman, F., Hooghiemstra, H., and Hoorn, C.: Geological and climatic influences on mountain biodiversity, Nat. Geosci., 11, 718–725, https://doi.org/10.1038/s41561-018-0236-z, 2018.
Bliedtner, M., Schäfer, I. K., Zech, R., and Von Suchodoletz, H.: Leaf wax n-alkanes in modern plants and topsoils from eastern Georgia (Caucasus) – Implications for reconstructing regional paleovegetation, Biogeosciences, 15, 3927–3936, https://doi.org/10.5194/bg-15-3927-2018, 2018.
Brittingham, A., Hren, M., and Hartman, G.: Microbial alteration of the hydrogen and carbon isotopic composition of n-alkanes in sediments, Org. Geochem., 107, 1–8, https://doi.org/10.1016/j.orggeochem.2017.01.010, 2017.
Brittingham, A., Hren, M. T., Hartman, G., Wilkinson, K. N., Mallol, C., Gasparyan, B., and Adler, D. S.: Geochemical Evidence for the Control of Fire by Middle Palaeolithic Hominins, Sci. Rep., 9, 15368, https://doi.org/10.1038/s41598-019-51433-0, 2019a.
Brittingham, A., Petrosyan, Z., Hepburn, J. C., Richards, M. P., Hren, M. T., and Hartman, G.: Influence of the North Atlantic Oscillation on δD and δ18O in meteoric water in the Armenian Highland, J. Hydrol., 575, 513–522, https://doi.org/10.1016/j.jhydrol.2019.05.064, 2019b.
Brun, P., Zimmermann, N. E., Hari, C., Pellissier, L., and Karger, D. N.: Global climate-related predictors at kilometer resolution for the past and future, Earth Syst. Sci. Data, 14, 5573–5603, https://doi.org/10.5194/essd-14-5573-2022, 2022.
Bush, R. T. and McInerney, F. A.: Leaf wax n-alkane distributions in and across modern plants: Implications for paleoecology and chemotaxonomy, Geochim. Cosmochim. Ac., 117, 161–179, https://doi.org/10.1016/j.gca.2013.04.016, 2013.
Diefendorf, A. F. and Freimuth, E. J.: Extracting the most from terrestrial plant-derived n-alkyl lipids and their carbon isotopes from the sedimentary record: A review, Org. Geochem., 103, 1–21, https://doi.org/10.1016/j.future.2015.08.005, 2017.
Diefendorf, A. F., Freeman, K. H., Wing, S. L., and Graham, H. V.: Production of n-alkyl lipids in living plants and implications for the geologic past, Geochim. Cosmochim. Ac., 75, 7472–7485, https://doi.org/10.1016/j.gca.2011.09.028, 2011.
Ehleringer, J., Björkman, O., and Bjorkman, O.: Quantum yields for CO2 uptake in C3 and C4 plants, Plant Physiol., 59, 86–90, 1977.
Farquhar, G. D., O'Leary, M. H., and Berry, J. A.: On the Relationship between Carbon Isotope Discrimination and the Intercellular Carbon Dioxide Concentration in Leaves, Aust. J. Plant Physiol., 9, 121–137, 1982.
Feakins, S. J., Wu, M. S., Ponton, C., Galy, V., and West, A. J.: Dual isotope evidence for sedimentary integration of plant wax biomarkers across an Andes-Amazon elevation transect, Geochim. Cosmochim. Ac., 242, 64–81, https://doi.org/10.1016/j.gca.2018.09.007, 2018a.
Ficken, K. J., Li, B., Swain, D. L., and Eglinton, G.: An n -alkane proxy for the sedimentary input of submerged foating freshwater aquatic macrophytes, Org. Geochem., 31, 745–749, 2000.
Gamarra, B., Sachse, D., and Kahmen, A.: Effects of leaf water evaporative 2H-enrichment and biosynthetic fractionation on leaf wax n-alkane δ2H values in C3 and C4 grasses, Plant Cell Environ., 39, 2390–2403, https://doi.org/10.1111/pce.12789, 2016.
Gasparyan, B. and Glauberman, P.: Beyond European boundaries: Neanderthals in the Armenian Highlands and the Caucasus, in: Updating Neanderthals, edited by: Romagnoli, F., Rivals, F., and Benazzi, S., 275–301, Academic Press, ISBN 978-0128214282, 2022.
Ghukasyan, R., Colonge, D., Nahapetyan, S., Ollivier, V., Gasparyan, B., Monchot, H., and Chataigner, C.: Kalavan-2 (North of Lake Sevan, Armenia): A new late middle paleolithic site in the Lesser Caucasus, Archaeology, Ethnol. Anthropol. Eur., 38, 39–51, https://doi.org/10.1016/j.aeae.2011.02.003, 2010.
Glauberman, P., Gasparyan, B., Sherriff, J., Wilkinson, K., Li, B., Knul, M., Brittingham, A., Hren, M. T., Arakelyan, D., Nahapetyan, S., Raczynski-henk, Y., Haydosyan, H., and Adler, D. S.: Barozh 12: Formation processes of a late Middle Paleolithic open-air site in western Armenia, Quaternay Sci. Rev., 236, 106276, https://doi.org/10.1016/j.quascirev.2020.106276, 2020.
Guisan, A. and Theurillat, J.-P.: Assessing alpine plant vulnerability to climate change: a modeling perspective, Integrated Ass., 1, 307–320, https://doi.org/10.1023/A:1018912114948, 2000.
Häggi, C., Sawakuchi, A. O., Chiessi, C. M., Mulitza, S., Mollenhauer, G., Sawakuchi, H. O., Baker, P. A., Zabel, M., and Schefuß, E.: Origin, transport and deposition of leaf-wax biomarkers in the Amazon Basin and the adjacent Atlantic, Geochim. Cosmochim. Ac., 192, 149–165, https://doi.org/10.1016/j.gca.2016.07.002, 2016.
Hemingway, J. D., Schefuß, E., Dinga, B. J., Pryer, H., and Galy, V. V.: Multiple plant-wax compounds record differential sources and ecosystem structure in large river catchments, Geochim. Cosmochim. Ac., 184, 20–40, https://doi.org/10.1016/j.gca.2016.04.003, 2016.
Jetter, R., Kunst, L., and Samuels, A. L.: Composition of plant cuticular waxes, in: Biology of the Plant Cuticule, edited by: Riederer, M. and Miller, C., Blackwell Publishing Ltd, Oxford, 145–181, https://doi.org/10.1002/9780470988718.ch4, 2006.
Joannin, S., Capit, A., Ollivier, V., Bellier, O., Brossier, B., Mourier, B., Tozalakian, P., Colombié, C., Yevadian, M., Karakhanyan, A., Gasparyan, B., Malinsky-Buller, A., Chataigner, C., and Perello, B.: First pollen record from the Late Holocene forest environment in the Lesser Caucasus, Rev. Palaeobot. Palynol., 304, 104713, https://doi.org/10.1016/j.revpalbo.2022.104713, 2022a.
Malinsky-Buller, A., Glauberman, P., Ollivier, V., Lauer, T., Timms, R., Frahm, E., Brittingham, A., Triller, B., Kindler, L., Knul, M. V., Krakovsky, M., Joannin, S., Hren, M. T., Bellier, O., Clark, A. A., Blockley, S. P. E., Arakelyan, D., Marreiros, J., Paixaco, E., Calandra, I., Ghukasyan, R., Nora, D., Nir, N., Adigyozalyan, A., Haydosyan, H., and Gasparyan, B.: Short-Term occupations at high elevation during the Middle Paleolithic at Kalavan 2 (Republic of Armenia), PLoS One, 16, e0245700, https://doi.org/10.1371/journal.pone.0245700, 2021.
Malinsky-Buller, A., Edeltin, L., Ollivier, V., Joannin, S., Peyron, O., Lauer, T., Frahm, E., Brittingham, A., Hren, M. T., Sirdeys, N., Glauberman, P., Adigyozalyan, A., and Gasparyan, B.: The environmental and cultural background for the reoccupation of the Armenian Highlands after the Last Glacial Maximum: The contribution of Kalavan 6, J. Archaeol. Sci. Rep., 56, 104540, https://doi.org/10.1016/j.jasrep.2024.104540, 2024.
Montoya, C., Balasescu, A., Joannin, S., Ollivier, V., Liagre, J., Nahapetyan, S., Ghukasyan, R., Colonge, D., Gasparyan, B., and Chataigner, C.: The Upper Palaeolithic site of Kalavan 1 (Armenia): An Epigravettian settlement in the Lesser Caucasus, J. Hum. Evol., 65, 621–640, https://doi.org/10.1016/j.jhevol.2013.07.011, 2013.
Oakes, A. M. and Hren, M. T.: Temporal variations in the δD of leaf n-alkanes from four riparian plant species, Org. Geochem., 97, 122–130, https://doi.org/10.1016/j.orggeochem.2016.03.010, 2016.
Pedentchouk, N., Sumner, W., Tipple, B., and Pagani, M.: δ13C and δD compositions of n-alkanes from modern angiosperms and conifers: An experimental set up in central Washington State, USA, Org. Geochem., 39, 1066–1071, https://doi.org/10.1016/j.orggeochem.2008.02.005, 2008.
Ponton, C., West, A. J., Feakins, S. J., and Galy, V.: Leaf wax biomarkers in transit record river catchment composition, Geophys. Res. Lett., 41, 6420–6427, https://doi.org/10.1002/2014GL061184, 2014.
Rudov, A., Mashkour, M., Djamali, M., and Akhani, H.: A Review of C4 Plants in Southwest Asia: An Ecological, Geographical and Taxonomical Analysis of a Region With High Diversity of C4 Eudicots, Front. Plant Sci., 11, 546518, https://doi.org/10.3389/fpls.2020.546518, 2020.
Sachse, D., Billault, I., Bowen, G. J., Chikaraishi, Y., Dawson, T. E., Feakins, S. J., Freeman, K. H., Magill, C. R., McInerney, F. a., van der Meer, M. T. J. J., Polissar, P., Robins, R. J., Sachs, J. P., Schmidt, H.-L., Sessions, A. L., White, J. W. C., West, J. B., and Kahmen, A.: Molecular paleohydrology: interpreting the hydrogen-isotopic composition of lipid biomarkers from photosynthesizing organisms, Annu. Rev. Earth Planet Sc., 40, 221–249, https://doi.org/10.1146/annurev-earth-042711-105535, 2012.
Sikes, E. L., Medeiros, P. M., Augustinus, P., Wilmshurst, J. M., and Freeman, K. R.: Seasonal variations in aridity and temperature characterize changing climate during the last deglaciation in New Zealand, Quaternary Sci. Rev., 74, 245–256, https://doi.org/10.1016/j.quascirev.2013.01.031, 2013.
Smolen, J. D. and Hren, M. T.: Differential effects of clay mineralogy on thermal maturation of sedimentary n-alkanes, Chem. Geol., 634, 121572, https://doi.org/10.1016/j.chemgeo.2023.121572, 2023.
Suh, Y. J., Diefendorf, A. F., Bowen, G. J., Cotton, J. M., and Ju, S. J.: Plant wax integration and transport from the Mississippi River Basin to the Gulf of Mexico inferred from GIS-enabled isoscapes and mixing models, Geochim. Cosmochim. Ac., 257, 131–149, https://doi.org/10.1016/j.gca.2019.04.022, 2019.
Tornero, C., Balasse, M., Bălăşescu, A., Chataigner, C., Gasparyan, B., and Montoya, C.: The altitudinal mobility of wild sheep at the Epigravettian site of Kalavan 1 (Lesser Caucasus, Armenia): Evidence from a sequential isotopic analysis in tooth enamel, J. Hum. Evol., 97, 27–36, https://doi.org/10.1016/j.jhevol.2016.05.001, 2016b.
Trigui, Y., Wolf, D., Sahakyan, L., Hovakimyan, H., Sahakyan, K., Zech, R., Fuchs, M., Wolpert, T., Zech, M., and Faust, D.: First calibration and application of leaf wax n-alkane biomarkers in loess-paleosol sequences and modern plants and soils in Armenia, Geosciences, 9, 263, https://doi.org/10.3390/geosciences9060263, 2019.
Volodicheva, N.: The Caucasus, The physical geography of northern Eurasia, 350–376, ISBN 9780198233848, 2002.
Zazanashvili, N.: The Caucasus hotspot, 15–25, in: Status and protection of globally threatened species in the Caucasus, edited by: Zazanashvili, N. and Mallon, D., Tbilisi, CEPF, WWF. Contour Ltd., 232 pp., ISBN 978-9941-0-2203-6, 2009.