the Creative Commons Attribution 4.0 License.
the Creative Commons Attribution 4.0 License.
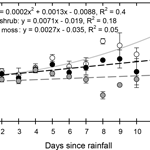
Response of hydrology and CO2 flux to experimentally altered rainfall frequency in a temperate poor fen, southern Ontario, Canada
Danielle D. Radu
Predicted changes to the precipitation regime in many parts of the world include intensifying the distribution into lower frequency, large magnitude events. The corresponding alterations to the soil moisture regime may affect plant growth and soil respiration, particularly in peatlands, where large stores of organic carbon are due to gross ecosystem productivity (GEP) exceeding ecosystem respiration (ER). This study uses lab monoliths corroborated with field measurements to examine the effect of changing rainfall frequency on peatland moisture controls on CO2 uptake in an undisturbed cool temperate poor fen. Lab monoliths and field plots containing mosses, sedges, or shrubs received either 2.3, 1, or 0.5 precipitation events per week, with total rainfall held constant. Decreasing rain frequency led to lower near-surface volumetric moisture content (VMC), water table (WT), and soil tension for all vegetation types, with minimal effect on evapotranspiration. The presence of sedges in particular led to soil tensions of ≥100 cm of water for a sizeable duration (37 %) of the experiment. Altered rainfall frequencies affected GEP but had little effect on ER; overall, low-frequency rain led to a reduced net CO2 uptake for all three vegetation types. VMC had a strong control on GEP and net ecosystem exchange (NEE) of the Sphagnum capillifolium monoliths, and decreasing rainfall frequency influenced these relationships. Overall, communities dominated by mosses became net sources of CO2 after 3 days without rain, whereas sedge communities remained net sinks for up to 14 days without rain. The results of this study demonstrate the hydrological controls of peatland CO2 exchange dynamics influenced by changing precipitation frequency; furthermore, they suggest these predicted changes in frequency will lead to increased sedge GEP but limit the carbon-sink function of peatlands.
- Article
(2514 KB) - Full-text XML
-
Supplement
(1482 KB) - BibTeX
- EndNote
Northern peatlands cover less than 3 % of the Earth's land surface but have sequestered approximately one-third of the global store of terrestrial carbon (Gorham, 1991; Yu, 2012), serving an important role in the global carbon cycle (Roulet et al., 2007; Limpens et al., 2008). However, this accumulated carbon is expected to be severely affected by climate change due to changes in temperature and precipitation patterns (Kettles and Tarnocai, 1999; Li et al., 2007; Gallego-Sala and Prentice, 2013). The net uptake of carbon in peatlands is due to relatively cool and moist conditions that promote the slower release of CO2 by ecosystem respiration (ER) than uptake by gross ecosystem productivity (GEP) (Frolking et al., 2002; Rydin and Jeglum, 2006; Frolking et al., 2010). Maintenance of the carbon sink function of peatlands is dependent on a high water table (WT) to minimize respiration (Alm et al., 1999; Strack et al., 2006; Riutta et al., 2007) and high near-surface soil moisture to enable high rates of Sphagnum moss photosynthesis (Waddington and Roulet, 2000; Moore et al., 2002; Adkinson and Humphreys, 2011), both of which are controlled by the precipitation regime. Changing climate and weather patterns are leading to an intensification of the precipitation regime (Easterling et al., 2000; Cao and Ma, 2009; Diffenbaugh and Field, 2013), with increasingly longer periods without rain that threaten the sink function of northern peatlands (Nijp et al., 2017).
Climate projections for boreal and temperate regions of the Northern Hemisphere suggest larger but less frequent precipitation events, with limited to no increase in absolute seasonal and annual precipitation (Trenberth, 2011; IPCC, 2013; Sillmann et al., 2013; Wang et al., 2014; Westra et al., 2014). This redistribution of the precipitation regime, especially during the growing season, is expected to lead to decreases in near-surface soil moisture, increased soil moisture variability, and deeper WTs(Knapp et al., 2002; Gerten et al., 2008; Vervoort and van der Zee, 2008; Wu et al., 2012). Lower WT levels and near-surface soil moisture due to seasonal drought or temperature-induced increased evapotranspiration (ET) have been shown to lead to reduced net carbon uptake and/or greater rates of CO2 emission from peatlands (Carroll and Crill, 1997; Alm et al., 1999; Tuittila et al., 2004; Strack et al., 2006; Riutta et al., 2007). Additionally, changes to peatland hydrology through drought have led to changes in vegetation biomass and community composition, which in turn can alter peatland CO2 exchange depending on the photosynthesis to respiration ratio of the newly established communities (Buttler et al, 2015; Potvin et al., 2015; Churchill et al., 2015; Dieleman et al., 2015). However, these experimental and monitoring studies typically reduce total precipitation input during the study period, while much less research has focussed on the redistribution of rainfall whilst maintaining precipitation totals.
Most studies examining the impact of precipitation frequency on ecosystems have focussed on grassland systems (Hoover et al., 2014; Knapp et al., 2015; Wilcox et al., 2015; Didiano et al., 2016), with comparatively fewer studies in wetland environments. Riparian marsh species' biomass accumulation is negatively affected by month-long periods without added precipitation (Garssen et al., 2014). In peatland systems, shifts in rainfall frequency have been shown to affect net primary production from Sphagnum moss (Robroek et al., 2009; Nijp et al., 2014) and methane emission (Radu and Duval, 2018a). Sphagnum moss photosynthesis, in particular, responds quickly to trace amounts of precipitation input (Strack and Price, 2009; Adkinson and Humphries, 2011); however, precipitation inputs are only available to these peat-building species for 2–3 days before it is evaporated (Ketcheson and Price, 2014). In the absence of precipitation, Sphagnum depends on capillary rise from the saturated zone to the photosynthesizing capitula (Clymo and Hayward, 1982). When WTs are too low, and precipitation is absent, soil water tensions increase and hyaline cells drain, causing desiccation and reduced photosynthesis (Thompson and Waddington, 2008; Strack et al., 2009; McCarter and Price, 2014).
In addition to the non-vascular Sphagna species, peatlands can be dominated by shrub and graminoid plant functional types, primarily ericaceous shrubs and sedges, respectively (Rydin and Jeglum, 2006). Vascular plants photosynthesize as long as the component cells retain turgor pressure, and are less susceptible to periods of low precipitation and WT drawdowns (Malmer et al., 1994; Vile et al., 2011). Peatland shrubs typically have increased productivity when WTs are lowered (Weltzin et al., 2001; Murphy et al., 2009; Bragazza et al., 2013; Munir et al., 2015). Sedges have deep roots (40–100 cm below peat surface) aided by aerenchyma that transport oxygen to lower depths (Silvan et al., 2004); thus, they are tolerant of high WT conditions, but have also been documented to perform well under low WT conditions (Fenner et al., 2007; Dieleman et al., 2015). Additionally, plant and soil respiration rates differ between moss, sedge, and shrub communities (Chimner, 2004; Juszczak et al., 2012; Duval and Radu, 2018). Since vascular plants can comprise a significant portion of peatland productivity and respiration (Szumigalski and Bayley, 1996; Moore et al., 2002; Riutta et al., 2007; Korrensalo et al., 2017), their responses to climate change in concert with Sphagnum must be taken into account when assessing peatland carbon cycling.
Previous studies of the effect of lowered water tables on different plant functional types to study the impacts of climate change on peatland carbon cycling have not included the importance of precipitation frequency (Riutta et al., 2007; Churchill et al., 2015; Potvin et al., 2015). Recent research on the interaction between peatland WT position, carbon cycling, and precipitation frequency has focussed on Sphagnum moss (Nijp et al., 2014, 2015, 2017). We have recently extended on this research by showing that decreasing rainfall frequency increased vascular plant cover in a cool temperate poor fen in southern Ontario. This consequently led to contracting responses of the seasonal CO2 balance between community types, with greater flux of CO2 to the atmosphere from the mosses but greater CO2 sequestration in sedge and shrub communities (Radu and Duval, 2018b). However, natural variability and logistical constraints in the field prevented us from making a formal assessment of the hydrological controls on the observed differences in NEE. A research gap exists at the intersection of precipitation frequency and its effect on peatland hydrology and CO2 for a variety of peatland community types. Therefore, the objectives of this study are as follows: (i) to investigate the effect of changing precipitation frequency on peatland hydrology, comprising WT position, VMC, soil water tension, and evapotranspiration (ET), in three common peatland vegetation communities – Sphagnum moss only, sedge with Sphagnum, and Sphagnum with ericaceous shrubs; and (ii) to determine the relationship between hydrologic conditions under the different rainfall frequency treatments and GEP, ER, and net ecosystem CO2 exchange of those communities. We altered precipitation frequency without changing total precipitation amount in both in situ field plots and lab monoliths through commensurate changes to event magnitudes.
2.1 Study site
The study was carried out on peat monoliths collected from an undisturbed poor fen in southern Ontario, Canada (44∘15′13.34 N, 80∘20′46.83 W). Vegetation was dominated by Sphagnum moss (particularly S. capillifolium but also S. rubellum, S. fuscum, and S. magellanicum), sedges (Carex oligosperma and Eriophorum vaginatum), and shrubs (mostly Chamaedaphne calyculata, as well as Rhododendron groenlandicum and Vaccinium uliginosum). Moss ground cover is close to 100 % throughout the site, except in areas with mature shrubs, where ground cover averaged 15 %. Average peat depth in the sample area is 2.1 m overlaying a sandy silt till substrate (Burwasser, 1974). The climate near the site is characterized by a mean annual temperature of 6.4∘ C and a mean annual precipitation of 996 mm (1981–2010 normal at Ruskview, ON station, data available: http://climate.weather.gc.ca/climate_normals/, last access: 22 June 2018). Rainfall events >0.2 mm occur on 43 % of the days during the early May – end of September growing season at this climate station. Over the growing season of 2015 at this site we manipulated the rainfall frequency whilst holding total seasonal rain constant; this was undertaken by utilizing rainout shelters over areas dominated by moss, sedge, and shrub communities. We measured plant community changes and CO2 exchange in response to these manipulated rainfall regimes at this site. Details of this field setup and experiment can be found in Radu and Duval (2018b). Here we focus on the hydrological process controls on the effect of rainfall frequency on CO2 dynamics through an experiment under controlled laboratory conditions. We also analyzed the field data in the same manner as the laboratory study.
2.2 Experimental setup
Intact peat cores (30 cm diameter × 40 cm height) were collected from the peatland to investigate how precipitation frequency affects CO2 exchange under controlled climate and WT regimes. Details of core collection and setup can be found in Radu and Duval (2018a). Briefly, cores of each of the three vegetation communities were placed in an environment-controlled chamber (FXC-19 Chamber, BioChambers, Winnipeg, Manitoba, Canada) and water tables were kept at −5 cm for an acclimatization period to the chamber conditions. Climate conditions of the chamber can be found in Table S1 in the Supplement.
We manipulated rainfall frequency over a 4-month study period. Three precipitation frequency treatments were randomly assigned to monoliths of each vegetation community type: 3 events/week (“HiFreq-lab”), 1 event/week (“MedFreq-lab”), and 1 event/2 weeks (“LowFreq-lab”). Simulated rainwater was a diluted Rudolph nutrient solution (Rudolph et al., 1988; Faubert and Rochefort, 2002) to limit detrimental effects to Sphagnum moss growth (Dieleman et al., 2015). The amount of water added for the individual events was adjusted such that the total amount of water was the same between treatments for each 2-week period (see Table S2 for treatment details). At the beginning of the study period, all water levels were set to −5 cm (“high”) and after 2 months were adjusted to −15 cm (“low”). Within each of these two 2-month periods, WT positions were allowed to naturally fluctuate with the addition of rainwater and the loss of water through ET to simulate field conditions.
Perforated PVC wells (1.27 cm diameter) covered with 250 µm Nitex mesh were carefully inserted into peat cores to monitor WT levels; measurements were made three times weekly in all monoliths. VMC was measured with EC-5 soil moisture sensors installed vertically into the peat of each monolith for an integrated depth of 0–5 cm; data were measured at half-hourly intervals with EM50 data loggers. Soil water tension was measured using 15 cm elbow tensiometers (Soil Measurement Systems, Arizona, USA) installed horizontally at 5 cm below moss surface; an INFIELD 7 portable tensiometer (UMS AG, Munich, Germany) was used to measure tension three times per week. Actual evapotranspiration (ET) was measured directly by weighing the monoliths before and after the rainwater additions throughout the study period.
CO2 exchange was measured three times per week in the environmental chamber as above, although under constant atmospheric conditions (Table S1). CO2 fluxes were measured using a clear Plexiglas cylindrical chamber (30 cm diameter × 40 cm height). A fan was mounted on the inside of the chamber to mix the air and homogenize the CO2 concentration during measurements. NEE was measured by fitting the clear chamber to a monolith and sealing it with petroleum jelly to ensure an air-tight seal. The chamber was connected to an infrared gas analyzer (IRGA; EGM-4, PP Systems, Massachusetts, USA). All monoliths were measured sequentially. Following the NEE measurements, ecosystem respiration (ER) was measured with an opaque aluminum cover placed over each chamber to exclude photosynthetically active radiation. Temperatures inside the clear and opaque chambers were monitored during measurements and never found to increase by more than 1.5 ∘C. Gross ecosystem productivity (GEP) was calculated by subtracting ER from NEE.
2.3 Data analysis
The data were checked for homogeneity and normality with a Levene's test and a Shapiro–Wilk test, respectively. Differences in hydrological and CO2 exchange parameters between rainfall frequency treatments and vegetation communities were assessed with linear mixed effects models: WT, VMC, soil tension, ET, GEP, ER, and NPP were response variables; rain fall frequency and water table treatments were fixed effects (with interactions); and the repeated measurements of the monoliths were random effect. Relationships between hydrological variables and CO2 exchange components were examined with linear and nonlinear regression. All statistical analyses were performed using the STATISTICA 8 software package (StatSoft Inc.).
Table 1Mean (± standard deviation) values of volumetric moisture content (VMC), water table (WT), soil matric tension (ψ), and evapotranspiration (ET) for each precipitation, WT, and vegetation community treatment during the laboratory experiment. Different letters indicate significant differences (p<0.05) between precipitation treatments within each water table and vegetation treatment. No letter indicates no significant difference.
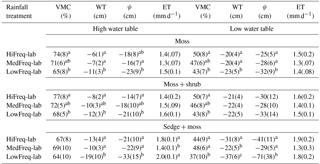
3.1 Peatland hydrology under changing rainfall frequency
Decreasing precipitation frequency generally resulted in decreased WT depth, near-surface VMC, and soil tension for all vegetation types, regardless of the initial WT position (Table 1). Water table fluctuation between rainfall treatments for the different vegetation monoliths are shown in the Supplement (Fig. S1). During the high WT period of the experiment, WT depths were significantly lower in the LowFreq-lab relative to the HiFreq-lab treatment in all vegetation communities (p<0.001; Table 1). In the second phase of the experiment when WTs were reset to −15 cm the WT was significantly lower in the LowFreq-lab relative to the HiFreq-lab treatments in the sedge + moss (p<0.01) and moss (p<0.05) monoliths, but not different in the moss + shrub communities.
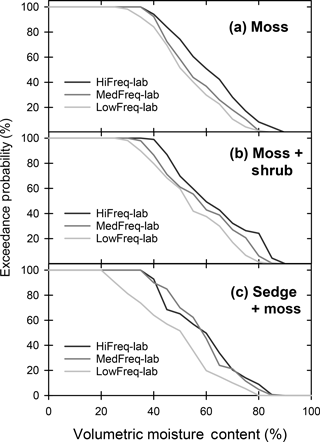
Figure 1Exceedance probability of VMC from the (a) moss, (b) moss + shrub, and (c) sedge + moss peat monoliths subject to Hi-, Med-, and LowFreq-lab precipitation frequency treatments.
Near-surface VMC followed the same pattern of WT fluctuation between rainfall treatments throughout the experiment for all vegetation monoliths (Fig. S2). For all vegetation types VMC was >60 % in the HiFreq-lab monoliths for more than half the experiment (Fig. 1). In comparison, the 50th quantile of VMC in the LowFreq-lab treatment was much lower at 49, 51, and 52 % for the moss (Fig. 1a), moss + shrub (Fig. 1b), and sedge + moss (Fig. 1b), respectively. Overall, VMC was significantly higher in the HiFreq-lab than the LowFreq-lab treatment for the moss and moss + shrub monoliths for both portions of the experiment (p<0.001; Table 1). In the sedge + moss monoliths there were no significant differences in VMC between treatments under the high WT period (p=0.298), but during the low WT period VMC in the LowFreq-lab treatment was significantly lower than either of the more frequent treatments (p<0.005). Similar trends were found during the field experiment (Table S3). There was a significant relationship between VMC and WT for all vegetation communities (, p<0.0001; Fig. S3), with proportionally greater decreases in VMC for a given WT drawdown for low- versus high-frequency treatments for all vegetation types.
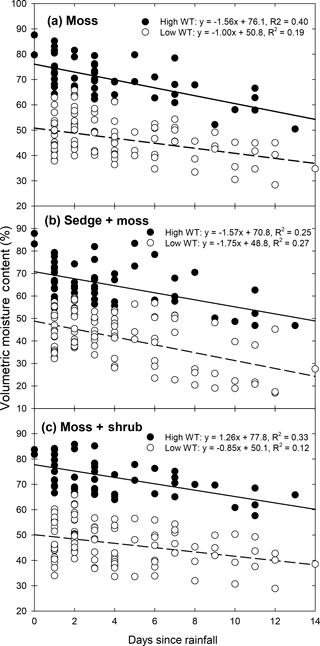
Figure 2Relationship between daily average VMC and days since the last rainfall/irrigation event for (a) moss, (b) sedge + moss, and (c) moss + shrub monoliths. Data for each vegetation type are separated between high WT and low WT portions of the experiment. Data are combined between precipitation frequency treatments. Linear regressions are provided. All regressions were significant (p<0.05).
Increasing the duration since the last precipitation event led to significant decreases in VMC for all vegetation communities (Fig. 2). The number of consecutive dry days had a greater effect on VMC declines during the high WT phase of the experiment for both the moss and moss + shrub monoliths. The VMC rate of decline decreased in the moss monoliths by 50 % between the two phases of the experiment, from 1.6 % d−1 during the high WT period (R2=0.40; p<0.001) to 0.8 % d−1 (R2=0.19; p<0.001) during the low WT phase (Fig. 2a). The decrease in rate of VMC decline was ∼ 31 % between high and low WT portions of the experiment for the moss + shrub monoliths (Fig. 2c). In contrast the rate of VMC decline with increasing consecutive dry days increased slightly in the sedge + moss monoliths as the WT was lowered, from 1.6 % d−1 during the high phase (R2=0.25; p<0.001) to 1.8 % d−1 during the low WT phase (R2=0.27; p<0.001; Fig. 2b).
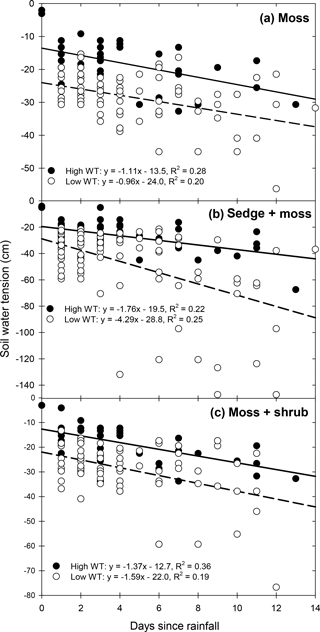
Figure 3Relationship between daily soil water tension (centimetre of water) and days since the last rainfall/irrigation event for (a) moss, (b) sedge + moss, and (c) moss + shrub monoliths. Data for each vegetation type are separated between high WT and low WT portions of the experiment. Data are combined between precipitation frequency treatments. Linear regressions are provided. All regressions were significant (p<0.05).
Average near-surface soil tension increased (became more negative) with decreasing rain frequency in all vegetation treatments (Table 1). These tensions rarely reached −100 cm, the critical level for Sphagnum capillary water supply, except in the sedge + moss monoliths subject to the LowFreq-lab treatment during the low WT period, where soil tension frequently was −150 cm. The monoliths experienced linear increases in tension of ≃1.1, −1.8, and −1.4 cm d−1 of no rainfall under high WT levels for mosses (R2=0.28; p<0.001), sedge + moss (R2=0.22; p<0.001), and moss + sedge (R2=0.36; p<0.001), respectively (Fig. 3). These rates of tension increase generally remained the same in the low WT portion of the experiment for the moss and moss + shrub monoliths (Fig. 3a, c). Conversely, soil tension increased at a rate of −4.3 cm d−1 without rain in the sedge + moss monoliths during the low WT phase, more than double the rate under high WT (Fig. 3b).
Sedge + moss evapotranspiration (ET) was significantly higher under HiFreq-lab and LowFreq-lab than MedFreq-lab treatments during both the high- and low WT phases of the lab experiment (p<0.05; Table 1). There were no significant differences in ET in the moss and moss + shrub monoliths. There was also no clear trend between ET and the number of days since rainfall; however, ET exceeded 3.5 mm d−1 for up to 2 days after rainfall in all vegetation communities, and generally remained below 3 mm d−1 for periods up to 14 days without rain.
Table 2Results of the mixed effects models on CO2 exchange parameters from the monolith experiment. Numerator degrees of freedom for rainfall frequency, water table treatment fixed effects, and their interaction were 2, 1, and 2, respectively, in all models. The denominator degrees of freedom for all models was 222.
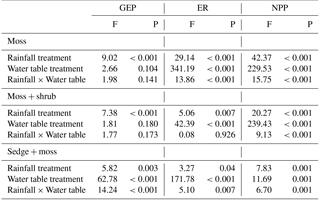
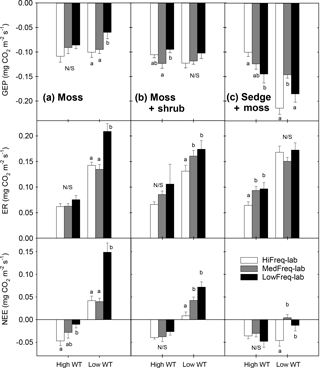
Figure 4Comparison of mean GEP, ER, and NEE between rainfall treatments within each WT treatment for each vegetation community: (a) moss, (b) moss + shrub, and (c) sedge + moss. Error bars represent the standard error of the mean. Negative NEE represents net CO2 uptake. Different letters indicate significant differences (p<0.05) between treatments within each WT period.
3.2 CO2 exchange dynamics
The rainfall frequency treatments had an effect on CO2 exchange dynamics for all vegetation communities, with low-frequency rain eliciting a response for GEP, ER, and NEE (Table 2). The effect of the high versus low WT treatments was also very strong (Table 2). Figure 4 illustrates this rainfall treatment's effect on CO2 exchange in greater detail. Gross ecosystem productivity from the moss monoliths decreased with decreasing rainfall frequency under low WT, with rates nearly twice as high in the HiFreq-lab treatment (−0.101 ± 0.010 mg CO2 m2 s−1) compared to the LowFreq-lab treatment (−0.056 ± 0.014 mg CO2 m2 s−1; p=0.017; Fig. 4a). While there were no differences in moss ER between rainfall treatments during high WT, the LowFreq-lab treatment led to significantly more respiration during the low WT period than either higher-frequency treatments (p=0.004). Moss NEE significantly decreased (less CO2 uptake/more CO2 release) with decreasing precipitation frequency in both WT treatments. Under the high WT period, moss monoliths were net CO2 sinks, with a NEE 3.5 times lower in the LowFreq-lab (−0.010 ± 0.008 mg CO2 m2 s−1) relative to the HiFreq-lab treatment (−0.038 ± 0.006 mg CO2 m2 s−1; p=0.013). During the low WT treatment, the mosses switched to net CO2 sources, with the LowFreq-lab treatment emitting between three and four times more CO2 (0.123 ± 0.016 mg CO2 m2 s−1) than the Med-Freq-lab (0.040 ± 0.007 mg CO2 m2 s−1) and HiFreq-lab (0.031 ± 0.004 mg CO2 m2 s−1) treatments (p<0.001; Fig. 4a).
The presence of shrubs with the moss increased GEP in the LowFreq-lab treatment during the low WT phase, such that there were no differences in GEP due to rainfall treatment at this time (Fig. 4b). Additionally, during high WT the MedFreq-lab treatment for the moss + shrub monoliths led to more CO2 uptake than the LowFreq-lab treatment. There were no differences in ER between vegetation treatments for the high WT portion of the experiment for the moss + shrub monoliths, but lower frequency rain led to greater ER than the HiFreq-lab treatment during the low WT portion. The pattern of NEE in response to rainfall frequency for moss + shrub monoliths was similar that observed with moss – under high WT all three treatments were CO2 sinks, with a shift to sources under low WT (Fig. 4b). While there were no differences in rates of NEE between frequency treatments under high WT (p=0.412), under low WT the MedFreq-lab (0.042 ± 0.007 mg CO2 m2 s−1) and LowFreq-lab (0.07 ± 0.011 mg CO2 m2 s−1) were nearly 6 and 10 times greater sources of CO2 than the HiFreq-lab treatment (0.007 ± 0.008 mg CO2 m2 s−1; p=0.019), respectively.
There was a trend of greater CO2 uptake with decreasing rainfall frequency under high WT in the sedge + moss monoliths, with LowFreq-lab resulting in greater GEP (−0.155 ± 0.008 mg CO2 m2 s−1) than HiFreq-lab (−0.098 ± 0.002 mg CO2 m2 s−1; p=0.03; Fig. 4c). Under low WT, GEP more than doubled in the HiFreq-lab treatment to −0.214 ± 0.012 mg CO2 m2 s−1, which was significantly higher than the MedFreq-lab treatment (−0.146 ± 0.007 mg CO2 m2 s−1; p=0.002). Under high WT conditions the lower-frequency treatments had higher rates of ER than HiFreq-lab, but this pattern disappeared with lower WT conditions. Overall, under high WT there was no effect of rain frequency on the NEE for the sedge + moss monoliths; however, under low WT there was significantly greater NEE (more carbon uptake) during frequent rain (−0.045 ± 0.012 mg CO2 m2 s−1; p=0.002) as compared to MedFreq-lab (0.008 ± 0.007 mg CO2 m2 s−1) and LowFreq-lab (−0.009 ± 0.012 mg CO2 m2 s−1), both of which were not statistically different from zero (Fig. 4c).
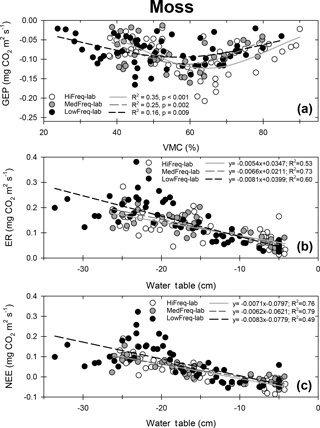
Figure 5Hydrologic controls on CO2 exchange in S. capillifolium-dominated monoliths, depicting relationships in (a) GEP, (b) ER, and (c) NEE between rainfall frequency treatments. Relationships in (a) are unimodal with indicated correlation coefficients and significance. Relationships in (b) are linear and are indicated with correlation coefficients. All regressions in (b) were statistically significant (p<0.001). Relationships for HiFreq-lab and MedFreq-lab treatments in (c) are second-order polynomial; relationship for LowFreq-lab is third-order polynomial with indicated correlation coefficients and significance.
3.3 Controls on CO2 exchange between rainfall frequency treatments
There were significant unimodal relationships between moss GEP and VMC from the monoliths, and these relationships varied between the rain frequency treatments (Fig. 5a). The relationship was strongest for the high frequency rain treatments, with peak rates of GEP occurring at 65 % VMC. The strength of the relationship decreased with decreasing rain frequency, as did the VMC at which peak GEP occurred. There were no significant relationships between GEP and WT. The position of the WT was highly correlated with moss ER for all of the rain frequency treatments (p<0.001; Fig. 5b). As rain frequency decreased, WT declines led to proportionally greater rates of ER, with the rate of increase in LowFreq-lab ER being 1.5 times higher than the HiFreq-lab treatment (Fig. 5b). Moss NEE was strongly controlled by near-surface VMC, with significant quadratic correlations for the HiFreq-lab and MedFreq-lab treatments, and a third-order polynomial relationship for the LowFreq-lab rainfall treatment (Fig. 5c). The switch from net CO2 uptake to a net source occurred in the moss monoliths at VMCs of 60, 62, and 64 % in the HiFreq-lab, MedFreq-lab, and LowFreq-lab, respectively. VMC was below these levels for 49 % of the study in the HiFreq-lab treatment, 66 % in the MedFreq-lab, and 73 % in the LowFreq-lab treatment (Fig. 1). Similar relationships between moss carbon flux and peatland hydrology between rain frequency treatments were found in the field experiment (Fig. S5); however, positive NEE was observed at higher VMC, which occurred for much less of the study period (20 % in the LowFreq treatment).
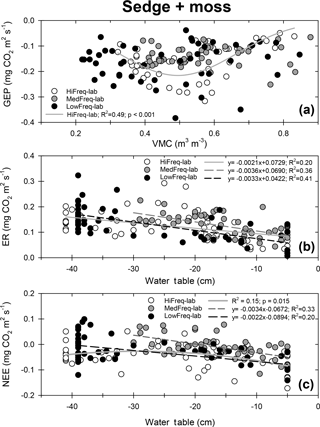
Figure 6Hydrologic controls on CO2 exchange in C. oligisperma -dominated monoliths, depicting relationships in (a) GEP, (b) ER, and (c) NEE between rainfall frequency treatments. Relationship in (a) is unimodal with indicated correlation coefficients and significance. Relationships in (b) and (c) are linear and are indicated with correlation coefficients. All regressions in (b) and (c) were statistically significant (p<0.001).
Under the HiFreq-lab treatment VMC had a unimodal control on rates of GEP from the sedge + moss monoliths, with peak gross production occurring at a VMC of 49 % (Fig. 6a). Decreasing rain frequency rendered this relationship insignificant. Ecosystem respiration was influenced by WT position in the sedge + moss monoliths, with rates increasing between 57 and 71 % faster with WT drawdown in the LowFreq-lab and MedFreq-lab, respectively, than for the HiFreq-lab conditions (Fig. 6b). There were moderate but highly significant (p<0.001) linear correlations between WT and sedge + moss NEE for the lower-frequency treatments, and a quadratic relationship existed between NEE and WT in the HiFreq-lab treatment (Fig. 6c). The trends with the moss + shrub monoliths were similar to the moss-only monoliths (data not shown). There were no significant relationships between WT or VMC with GEP or NEE from the shrub communities in the field, although WT did control ER in these communities (p<0.001).
Overall, increasing the number of days since the last rainfall event led to a strong increase in NEE (CO2 efflux to the atmosphere) for the moss monoliths (R2=0.31, p<0.01), a moderate increase for the moss + shrub monliths (R2=0.18, p<0.001), but almost no increase in the sedge + moss monoliths (R2=0.05, p=0.044; Fig. 7). This relationship was linear for the vascular plant communities, and quadratic for the moss-only monoliths. The moss and moss + shrub monoliths had a net uptake of CO2 when the duration since the last event was under 3.5 days; however, after this threshold, there was net emission of CO2. Conversely, sedge + moss monoliths were largely sinks of CO2 for up to 2 weeks without rainfall, and were predicted to have a net emission of CO2 after 15 days between events.
4.1 Plant community-mediated response of peat hydrology to precipitation frequency
It is expected that a shift in the precipitation to larger, more infrequent events will lead to lower WT levels and drier surface conditions (Knapp et al., 2008; Piao et al., 2009). We found that decreasing the precipitation frequency while holding total seasonal rain constant resulted in lower average WT positions, lower VMC, and higher soil tension in all vegetation communities (Tables 1, S3; Fig. S1, S2). On one hand, the smaller, more frequent precipitation events were able to buffer against seasonal WT declines and maintain more moisture in the near-surface peat layer (Fig. S3). On the other hand, the larger, less frequent events contributed moisture to deeper layers, which led to the observed increased rates of VMC decline with concomitant WT decline (Fig. S3). Therefore, in addition to climate change leading to increased ET and lower WT positions in peatlands (Whittington and Price, 2006; Munir et al., 2015), the frequency of rainfall events is likely to contribute to even drier surface conditions than is currently considered.
Decreasing rainfall frequency allowed for continued near-surface soil moisture decreases (Fig. 2) and tension increases (Fig. 3). Delivering rain every 3 days, the current average for the studied peatland (Table S2), prevented these large soil moisture declines in communities dominated by Sphagnum moss (Table S3; Fig. S2). However, the presence of sedges increased ET relative to the moss-only communities. Vascular plant abundance increases peatland ET, even during periods of limited rainfall (Takagi et al., 1999; Petrone et al., 2004; Admiral and Lafleur, 2007; Wu et al., 2013; Takashi et al., 2016). During the first portion of the experiment when WTs were high, the rate of drying was the same between moss and sedge + moss monoliths; however, during the low WT portion the rate of drying in the sedge community became 75 % higher than in the moss-only community (Fig. 2a, b). This led to over a four-fold greater rate of tension increase in the presence of sedges (Fig. 3a, b). The low-frequency rain treatment in the sedge + moss community led to near-surface tensions ≤100 cm, sometimes after only 4 days without rain. This tension threshold is generally considered the point at which Sphagnum can no longer effectively photosynthesize (Price, 1997, Thompson and Waddington, 2008).
In our study −100 cm of tension was reached at a VMC of 37 %, similar to thresholds found in other peatlands (Price and Whitehead, 2004; Cagampan and Waddington, 2008). The high- and medium-frequency treatments for all vegetation communities maintained soil moisture above this value for at least 92 % of the experiment; however, deviations below this threshold increased considerably in the low-frequency rain treatment (Fig. 1). The moss and moss + shrub monoliths subject to low-frequency rain experienced moss-level VMC <37 % for ∼ 10 % of the experiment, whereas the sedge + moss monoliths were below this threshold for 38 % of the experiment. Overall, our results suggest that the interaction between deeper WTs and less-frequent precipitation regimes expected with climate change will lead to high tensions that may limit moisture uptake by Sphagnum mosses in peatlands dominated by sedges.
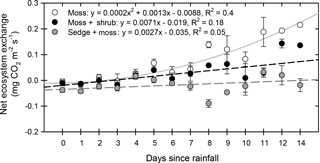
Figure 7Relationships between NEE and the number of consecutive dry days since rainfall for each vegetation community. Negative NEE represents CO2 uptake. Error bars represent the standard deviation of the mean. The relationships for the moss and moss + shrub communities are significant at the p<0.001 level ; the relationship for the sedge + moss communities is significant at the p=0.045 level.
4.2 Precipitation frequency – peatland hydrology interactive effects on CO2 exchange
The precipitation regimes we imposed affected the CO2 exchange of the vegetation communities. Overall, decreasing rainfall frequency led to decreased NEE (less storage/greater flux to the atmosphere) from the peatland communities tested, with differences in NEE for four of the six vegetation–WT combinations we tested (Fig. 4). Nijp et al. (2014) also found NEE decreased from three Sphagnum species as precipitation frequency decreased. Field measurements of CO2 fluxes from peatlands have also documented decreased GEP and increased ER during extended rainless periods, switching peatlands to net sources of CO2 during these short periods (Alm et al., 1999; Lund et al., 2012). Seasonal estimates from our study site confirm increased flux of CO2 to the atmosphere from moss communities during low-frequency rain; however, the abundant shrub communities we were able to test in the field reduced losses to the atmosphere under low-frequency rain, due to higher GEP from the mature shrubs (Radu and Duval, 2018b).
Lower WTs, characteristic of drought periods, can decrease productivity from Sphagnum-dominated peatlands through reduced VMC creating a moisture stress (Alm et al., 1999; Chivers et al., 2009; Potvin et al., 2015), as well as increase aerobic respiration through increased oxygen diffusion and a greater depth of aerated soil (Carroll and Crill, 1997; Tuittila et al., 2004); this results in an overall net loss of carbon to the atmosphere. Our study demonstrates that low WTs exacerbate the effect of low rain frequency through greater rates of ER, causing the moss and moss + shrub communities to switch from net sinks of CO2 to net sources (Fig. 4a, b). The combination of low WT and low-frequency rain led to greater rates of near-surface drying (Fig. 2), which would stimulate soil respiration through increased aeration (Silvola et al., 1996). Additionally, low WTs resulted in the sedge + moss monoliths, subject to the two lower frequency treatments, switching from sinks to becoming carbon neutral (Fig. 4c). The lack of NEE response to a low WT from the sedge + moss community subject to frequent rain was due to increased GEP, presumably because the high frequency rain maintained moss photosynthesis, while the sedges increased production with lower WT (Wu et al., 2013; Potvin et al., 2015).
Carbon assimilation by S. capillifolium was sensitive to rainfall frequency-induced changes in VMC. As rainfall frequency decreased, peak GEP rates were lower and occurred at lower VMC, while the strength of the relationship between GEP and VMC weakened (Fig. 5). Repeated drying and wetting of mosses has been found to result in lower photosynthetic capacity due to damage to moss cellular integrity, including degradation of chlorophyll and rupture of cell membranes (Schipperges and Rydin, 1998). The low-frequency rain events allowed for greater near-surface VMC variability, which may have contributed to lower moss GEP at any given VMC. Gerdol et al. (1996) found Sphagnum species, including the dominant species in our study, S. capillifolium, were unable to resume photosynthesis after an 11-day period without new water. In our study, the mosses continued to photosynthesize, albeit at lower rates, after 13 days without rain, as soil water tensions were weak enough (≥100 cm) to allow water uptake. Mosses subject to a regime of 6 days between rainfall events had GEP rates 25 % lower than under a regime of 2 days between events.
The observed increase in the VMC threshold at which the Sphagnum capillifolium monoliths switched from NEE sinks to sources as precipitation frequency decreased (Fig. 5a) was related to the concomitant lower WTs (Fig. S3a). Therefore, decreasing rain frequency not only lowered near-surface soil moisture but also created an additional stress for the mosses by reducing the availability of capillary water due to the lower WT. During the high WT portion of the experiment VMC was found to drop below these thresholds after 10–11 days without rain; however, the low WT period was characterized by a near-surface VMC almost always less than 60 % (Fig. 2a). Strack et al. (2009) found a sink–source threshold for S. rubellum of ∼ 45 % VMC in the near-surface peat, and Nijp et al. (2014) found that a VMC of 48 % corresponded to a switch from sink to source for S. balticum. All three species are lawn- or small hummock-forming species capable of withstanding low water availability, yet they differ greatly in their response to VMC. The physiological mechanisms driving this range in moisture content threshold to NEE among Sphagna species were beyond the scope of our study, but these differences in species-specific responses to soil water content are very important for parameterizing peatland ecosystem models (le Roux et al., 2013; Nijp et al., 2017).
In our study, moss-dominated communities became sources of CO2 to the atmosphere after less than a week without rain (Fig. 7). This switch from carbon sink to source was primarily driven by decreased VMC between events limiting GEP (Figs. S2, 5a). The monoliths dominated by vascular plants were less affected by rainfall-induced decreases in VMC due to their deeper roots (Silvan et a., 2004), with the sedge communities maintaining uptake or carbon neutrality for the maximum 14 consecutive dry days of our experiment (Fig. 7). In our study region, southern Ontario, seven consecutive dry days occur quite regularly during the growing season, with one or two 14-day dry periods typical within a growing season. The frequency of extreme precipitation events is increasing in the region (Cao and Ma, 2006; Soulis et al., 2016); however, little research has been conducted on the dynamics of the intervening dry periods. Most climate models project these dry periods to increase in both length and recurrence during growing seasons (Orlowsky and Seneviratine, 2012; Sillmann et al., 2013; Walsh et al., 2014).
4.3 Implications for peatland plant functional types and climate change
Our study demonstrates that plant functional types will be differentially affected as rainfall becomes less frequent, particularly if accompanied by lower WTs as a result of climate change. Sphagnum-only communities experienced a significant decrease in GEP with less frequent rainfall, while GEP in the communities with vascular plants was generally unaffected. Additionally, decreased precipitation frequency had stronger negative implications for S. capillifolium in the presence of sedges, with near-surface tensions of ≥100 cm after only 3 days without rainfall when the WT was deep (≥15 cm). Although we did not measure S. capillifolium productivity separate from the sedges in the same communities, white, desiccated S. capillifolium capitula were observed in sedge + moss monoliths receiving less frequent rainfall when the water table was low. In a companion study we found that low frequency rain led to significantly more seasonal sedge and ericaceous shrub cover and GEP than high frequency rain (Radu and Duval, 2018b). Ericaceous shrubs in particular seem to thrive, with rates of GEP under LowFreq exceeding HiFreq rates 75 % of the time, generally at multiples of 2–7 under high light conditions (Fig. S4).
Our results show that decreased precipitation frequency will decrease net CO2 uptake in peatland plant communities dominated by Sphagnum, both in the presence and absence of sedges and juvenile shrubs. With the lower water table positions expected as a result of increased ET, we showed that these communities may switch to CO2 sources, and decreased precipitation frequency may increase net CO2 release. Furthermore, increased shrub dominance (Hedwall et al., 2017) is likely to shift the CO2 balance towards increased CO2 efflux to the atmosphere. Therefore, we found that decreasing precipitation frequency is likely to lead to a positive feedback for climate change due to increased net CO2 release to the atmosphere caused by drier surface conditions and a possible shift to sedge- and shrub-dominated communities.
Over the longer term, decreased precipitation frequency is likely to affect the net carbon sink function of temperate peatlands. The reduced NEE of the Sphagnum-dominated monoliths with decreasing precipitation frequency (Fig. 4) will progressively limit the storage of carbon in the peat on the annual and decadal scales. We have recently shown that decreased growing season precipitation frequency increases sedge and shrub growth (Radu and Duval, 2018b). The incorporation of vascular plant litter into the peat matrix should increase rates of respiration over and above the short-term results of the present study due to the greater rates of decomposition relative to Sphagnum peat (Leifeld et al., 2012; Dieleman et al., 2015; Duval and Radu, 2018). Predicted lower WT position as a consequence of global warming will result in lower long-term rates of carbon accumulation in peatlands (Strack et al., 2006; Peichl et al., 2014; Carlson et al., 2015). Therefore, the combined effects of lowered WTs, increased vascular plant cover, and decreased VMC due to decreased precipitation frequency (Fig. 2) are likely to reduce long-term rates of carbon storage, potentially switching temperate peatlands to carbon sources.
Our study has demonstrated that decreasing rainfall frequency results in a lower average VMC, WT, and soil water tension, and increases their variability. In turn, these changes in peat hydrology lead to changes in CO2 exchange dynamics, with significant effects on GEP and NEE. Moss-dominated communities in particular were strongly affected by changes in rainfall frequency, displaying decreasing gross and net CO2 uptake with deceasing rain frequency. In contrast, sedge-dominated communities were able to better withstand the longer periods without rain, and actually increased GEP with low-frequency rain when the WT was close to the surface. The empirical relationships between CO2 exchange and near-surface VMC and WT showed clear differences between rainfall treatments, demonstrating the influence of rain frequency on these couplings. The presence of a low WT was found to have very strong effects on CO2 exchange, shifting the moss and moss + shrub communities from net CO2 sinks to net sources while rendering the sedge + moss communities CO2 neutral under the lower-frequency treatments.
We show the maintenance of near-surface moisture is very important to moss productivity, and as few as four consecutive dry days was sufficient to illicit a net CO2 efflux response from the moss monoliths. However, the deeper rooting system of the sedges prevented these monoliths from becoming sources for the extent of our experiment (14 consecutive days without rain). Most climate change projections predict increases in the number and recurrence of consecutive dry days during the growing season for many peat-forming areas throughout the globe. Our results suggest this will limit net CO2 uptake in peatlands. We show that the presence of a lower WT, as predicted due to rising temperatures, increases the effect of lowered precipitation frequency. These rainfall-induced moisture and CO2 dynamics should be included in peatland ecosystem and climate models.
Data are available from the corresponding author upon request.
The supplement related to this article is available online at: https://doi.org/10.5194/bg-15-3937-2018-supplement.
DDR and TPD developed the idea and methodology for the study, and led the experimental setup. DDR led the data acquisition. TPD and DDR performed the data analysis. Both authors wrote the manuscript.
The authors declare that they have no conflict of interest.
We thank the entire Stream and Wetland Ecohydrology Research Group at UTM
for their assistance with the field portion of this study. In particular, we
thank Ana Banjavcic and Michael Harris for help with core collection and
William Ng for lab analysis. This research was funded by NSERC Discovery
Grant #418197 to TPD and a NSERC CGS-M and UTM-Graduate Expansion Fund to
DDR. The constructive comments of three anonymous reviewers are greatly
appreciated.
Edited by: Paul Stoy
Reviewed by: three anonymous referees
Adkinson, A. C. and Humphreys, E. R.: The response of carbon dioxide exchange to manipulations of Sphagnum water content in an ombrotrophic bog, Ecohydrology, 4, 733–743, https://doi.org/10.1002/eco.171, 2011.
Admiral, S. W. and P. M. Lafleur.: Partitioning of latent heat flux at a northern peatland, Aquat. Botany, 86, 107–116, https://doi.org/10.1016/j.aquabot.2006.09.006, 2007.
Alm, J., Schulman, L., Walden, J., Nykanen, H., Martikainen, P. J., and Silvola, J.: Carbon balance of a boreal bog within a year with an exceptionally dry summer, Ecology, 80, 161–174, https://doi.org/10.1890/0012-9658(1999)080[0161:CBOABB]2.0.CO;2, 1999.
Bragazza, L., Parisod, J., Buttler, A., and Bardgett, R. D.: Biogeochemical plant-soil microbe feedback in response to climate warming in peatlands, Nat. Clim. Change, 3, 273–277, https://doi.org/10.1038/NCLIMATE1781, 2013.
Burwasser, C. J.: Quaternary geology of the collingwood-nottawasaga area, southern ontario; ontario div. mines, prelim, Map P. 919 Geol. Ser., scale 1:50,000, 1974.
Buttler, A., Robroek, B. J. M., Laggoun-Défarge, F., Jassey, V. E. J., Pochelon, C., Bernard, G., Delarue, F., Gogo, S., Mariotte, P., Mitchell, E. A. D., and Bragazza, L.: Experimental warming interacts with soil moisture to discriminate plant responses in an ombrotrophic peatland, J. Veget. Sci., 26, 964–974, https://doi.org/10.1111/jvs.12296, 2015.
Cagampan, J. P. and Waddington, J. M.: Moisture dynamics and hydrophysical properties of a transplanted acrotelm on a cutover peatland, Hydrol. Proc., 22, 1776–1787, https://doi.org/10.1002/hyp.6802, 2008.
Cao, Z. and Ma, J.: Summer severe-rainfall frequency trend and variability over Ontario, Canada, J. Appl. Meteorol. Climatol., 48, 1955–1960, https://doi.org/10.1175/2009JAMC2055.1, 2009.
Carlson, K. M., Goodman, L. K., and May-Tobin, C. C.: Modeling relationships between water table depth and peat soil carbon loss in Southeast Asian plantations, Environ. Res. Lett., 10, 074006, https://doi.org/10.1088/1748-9326/10/7/074006, 2015.
Carroll, P. and Crill, P.: Carbon balance of a temperate poor fen, Glob. Biogeochem. Cy., 11, 349–356, https://doi.org/10.1029/97GB01365, 1997.
Chimner, R. A.: Soil respiration rates of tropical peatlands in Micronesia and Hawaii, Wetlands, 24, 51–56, https://doi.org/10.1672/0277-5212(2004)024[0051:SRROTP]2.0.CO;2, 2004.
Chivers, M. R. Turetsky, M. R., Waddington, J. M., Harden, J. W., and McGuire, A. D.: Effects of experimental water table and temperature manipulations on ecosystem CO2 fluxes in an Alaskan rich fen, Ecosystems, 12, 1329–1342, https://doi.org/10.1007/s10021-009-9292-y, 2009.
Churchill, A. C., Turetsky, M. R., McGuire, A. D., and Hollingsworth, T. N.: Response of plant community structure and primary productivity to experimental drought and flooding in an Alaskan fen, Can. J. Forest Res., 45, 185–193, https://doi.org/10.1139/cjfr-2014-0100, 2015.
Clymo, R. S. and Hayward, P. M.: The ecology of Sphagnum, Bryophyte Ecology, edited by: Smith, A. J. E., Chapman & Hall: New York, 229–289, 1982.
Didiano, T. J., Johnson, M. T. J., and Duval, T. P.: Disentangling the effects of precipitation amount and frequency on the performance of 14 grassland species, PLoS One, 11, e0162310, https://doi.org/10.1371/journal.pone.0162310, 2016.
Dieleman, C. M., Branfireun, B. A., McLaughlin, J. W., and Lindo, Z.: Climate change drives a shift in peatland ecosystem plant community: Implications for ecosystem function and stability, Glob. Change Biol., 21, 388–395, https://doi.org/10.1111/gcb.12643, 2015.
Diffenbaugh, N. S. and Field, C. B.: Changes in ecologically critical terrestrial climate conditions, Science, 341, 486–492, https://doi.org/10.1126/science.1237123, 2013.
Duval, T. P. and Radu, D. D.: Effect of temperature and soil organic matter quality on greenhouse-gas production from temperate poor and rich fen soils. Ecol. Eng., 114, 66–75, https://doi.org/10.1016/j.ecoleng.2017.05.011, 2018.
Easterling, D. R., Meehl, G. A., Parmesan, C., Changnon, S. A., Karl, T. R., and Mearns, L. O.: Climate extremes: Observations, modeling, and impacts, Science, 289, 2068–2074, https://doi.org/10.1126/science.289.5487.2068, 2000.
Faubert, P. and Rochefort, L.: Response of peatland mosses to burial by wind-dispersed peat, The Bryologist, 105, 96–103, https://doi.org/10.1639/0007-2745(2002)105[0096:ROPMTB]2.0.CO;2, 2002.
Fenner, N., Freeman, C., Lock, M. A., Harmens, H., Reynolds, B., and Sparks, T.: Interactions between elevated CO2 and warming could amplify DOC exports from peatland catchments, Environ. Sci. Tech., 41, 3146–3152, https://doi.org/10.1021/es061765v, 2007.
Frolking, S., Roulet, N. T., Moore, T. R., Lafleur, P. M., Bubier, J. L., and Crill, P. M.: Modeling seasonal to annual carbon balance of Mer Bleue Bog, Ontario, Canada, Glob. Biogeochem. Cy., 16, 1030, https://doi.org/10.1029/2001GB001457, 2002.
Frolking, S., Roulet, N. T., Tuittila, E., Bubier, J. L., Quillet, A., Talbot, J., and Richard, P. J. H.: A new model of Holocene peatland net primary production, decomposition, water balance, and peat accumulation, Earth Syst. Dynam., 1, 1–21, https://doi.org/10.5194/esd-1-1-2010, 2010.
Gallego-Sala, A. V. and Prentice, C.: Blanket peat biome endangered by climate change, Nat. Clim. Change 3, 152–155, https://doi.org/10.1038/NCLIMATE1672, 2013.
Garssen, A. G., Verhoeven, J. T. A., and Soons, M. B.: Effects of climate-induced increases in summer drought on riparian plant species: a meta-analysis, Freswater Biol., 59, 1052–1063, doi.10.1111/fwb.12328, 2014.
Gerdol, R., Bonora, A., Gualandri, R., and Pancaldi, S.: CO2 exchange, photosynthetic pigment composition, and cell ultrastructure of Sphagnum mosses during dehydration and subsequent rehydration, Can. J. Botany, 74, 726–734, 1996.
Gerten, D., Luo, Y., LeMaire, G., Partons, W. J., Keoughs, C., Weng, G., Beier, C., Ciais, P., Cramer, W., Dukes, J. S., Hanson, P. J., Knapp, A. A. K., Linder, S., Nepstad, D., Rustad, L., and Sowerby, A.: Modelled effects of precipitation on ecosystem carbon and water dynamics in different climatic zones, Glob. Change Biol., 14, 2365–2379, https://doi.org/10.1111/j.1365-2486.2008.01651.x, 2008.
Gorham, E.: Northern peatlands: Role in the carbon cycle and probable responses to climatic warming, Ecol. Appl., 1, 182–195, https://doi.org/10.2307/1941811, 1991.
Hedwall, P.-O., Brunet, J., and Rydin, H.: Peatland plant communities under global change: negative feedback loops counteract shifts in species composition, Ecology, 98, 150-161, https://doi.org/10.1002/ecy.1627, 2017.
Hoover, D. L., Knapp, A. K., and Smith, M. D.: Resistance and resilience of a grassland ecosystem to climate extremes, Ecology, 95, 2646–2656, https://doi.org/10.1890/13-2186.1, 2014.
IPCC 2013: Climate Change 2013: The Physical Science Basis, in: Contribution of Working Group I to the Fifth Assessment Report of the Intergovernmental Panel on Climate Change, edited by: Stocker, T. F., Qin, D., Plattner, G.-K., Tignor, M., Allen, S. K., Boschung, J., Nauels, A., Xia, Y., Bex, V., and Midgley, P. M., Cambridge University Press, Cambridge, UK and New York, NY, USA, 1535 pp., 2013.
Juszczak, R., Humphreys, E., Manual, A., Michalak-Galczewska, M., Kayzer, D., and Olejnik, J.: Ecosystem respiration in a heterogeneous temperate peatland and its sensitivity to peat temperature and water table depth, Plant and Soil, 366(1-2), 505–520, https://doi.org/10.1007/s11104-012-1441-y, 2012.
Ketcheson, S. J. and Price, J. S.: Characterization of the fluxes and stores of water within newly formed Sphagnum moss cushions and their environment, Ecohydrology, 7, 771–782, https://doi.org/10.1002/eco.1399, 2014.
Kettles, I. M. and Tarnocai, C.: Development of a model for estimating the sensitivity of Canadian peatlands to climate warming, Geographie physique et quaternaire, 53, 323–338, 1999.
Knapp, A. K., Fay, P. A., Blair, J. M., Collins, S. L., Smith, M. D., Carlisle, J. D., Harper, C. W., Danner, B. T., Lett, M. S., and McCarron, J. K.: Rainfall variability, carbon cycling, and plant species diversity in a mesic grassland, Science, 298, 2202–2205, https://doi.org/10.1126/science.1076347, 2002.
Knapp, A. K., Beier, C., Briske, D. D., Classen, A. T., Luo, Y., Reichstein, M., Smith, M. D., Smith, S. D., Bell, J. E., Fay, P. A., Heisler, J. L., Leavitt, S. W., Sherry, R., Smith, B., and Weng, E.: Consequences of more extreme precipitation regimes for terrestrial ecosystems, BioScience, 58, 811–821, https://doi.org/10.1641/B580908, 2008.
Knapp, A. K., Hoover, D. L., Wilcox, K. R., Avolio, M. L., Koerner, S. E., La Pierre, K. J., Loik, M. E., Luo, Y. Q., Sala, O. E. and Smith, M. D., Characterizing differences in precipitation regimes of extreme wet and dry years: implications for climate change experiments, Glob. Change Biol., 21, 2624–2633, https://doi.org/10.1111/gcb.12888, 2015
Korrensalo, A., Alekseychik, P., Hájek, T., Rinne, J., Vesala, T., Mehtätalo, L., Mammarella, I., and Tuittila, E.-S.: Species-specific temporal variation in photosynthesis as a moderator of peatland carbon sequestration, Biogeosciences, 14, 257–269, https://doi.org/10.5194/bg-14-257-2017, 2017.
Leifeld, J., Steffens, M., and Galego-Sala, A.: Sensitivity of peatland carbon loss to organic matter quality, Geophys. Res. Lett., 39, L14704, https://doi.org/10.1029/2012gl051856, 2012.
le Roux, P. C., Aalto, J., and Luoto, M.: Soil moisture's underestimated role in climate change impact modelling in low-energy systems, Glob. Change Biol., 19, 2965–2975, https://doi.org/10.1111/gcb.12286, 2013.
Li, W., Dickenson, R. E., Fu, R., Niu, G.-Y., Yang, Z.-L., and Canadell, J. G.: Future precipitation changes and their implications for tropical peatlands, Geophys. Res. Lett., 34, L01403, https://doi.org/10.1029/2006GL028364, 2007.
Limpens, J., Berendse, F., Blodau, C., Canadell, J. G., Freeman, C., Holden, J., Roulet, N., Rydin, H., and Schaepman-Strub, G.: Peatlands and the carbon cycle: from local processes to global implications – a synthesis, Biogeosciences, 5, 1475–1491, https://doi.org/10.5194/bg-5-1475-2008, 2008.
Lund, M., Christensen, T. R., Lindroth, A., and Schubert, P.: Effects of drought conditions on the carbon dioxide dynamics in a temperate peatland, Environ. Res. Lett., 7, 045704, https://doi.org/10.1088/1748-9326/7/4/045704, 2012.
Malmer, N., Svensson, B., and Wallén, B.: Interactions between Sphagnum mosses and field layer vascular plants in the development of peat-forming systems, Folia Geobot. Phytotx., 29, 483–496, 1994.
McCarter, C. P. R. and Price, J. S., Ecohydrology of Sphagnum moss hummocks: mechanisms of capitula water supply and simulate effects of evaporation, Ecohydrology, 7, 33–44, https://doi.org/10.1002/eco.1313, 2014.
Moore, T. R. and Dalva, M.: The influence of temperature and water table position on carbon dioxide and methane emissions from laboratory columns of peatland soils, Europ. J. Soil Science, 44, 651–664, 1993.
Moore, T. R., Bubier, J. L., Frolking, S. E., Lafleur, P. M., and Roulet, N. T.: Plant biomass and production and CO2 exchange in an ombrotrophic bog, J. Ecol., 90, 25–36, https://doi.org/10.1046/j.0022-0477.2001.00633.x, 2002.
Munir, T. M., Perkins, M., Kaing, E., and Strack, M.: Carbon dioxide flux and net primary production of a boreal treed bog: Responses to warming and water-table-lowering simulations of climate change, Biogeosciences, 12, 1091–1111, https://doi.org/10.5194/bg-12-1091-2015, 2015.
Murphy, M. T., McKinley, A., and Moore, T. R.: Variations in above- and below-ground vascular plant biomass and water table on a temperate ombrotrophic peatland, Botany, 87, 845–853, https://doi.org/10.1139/B09-052, 2009.
Nijp, J. J., Limpens, J., Metselaar, K. M., van der Zee, S. E. A. T. M., Berendse, F., and Robroek, B. J. M.: Can frequent precipitation moderate the impact of drought on peatmoss carbon uptake in northern peatlands?, New Phytol., 203, 70–80, https://doi.org/10.1111/nph.12792, 2014.
Nijp, J. J., Limpens, J., Metselaar, K., Peichl, M., Nilsson, M. B., van der Zee, S. E. A. T. M., and Berendse, F.: Rain events decrease boreal peatland net CO2 uptake through reduced light availability, Glob. Change Biol., 21, 2309–2320, https://doi.org/10.1111/gcb.12864, 2015.
Nijp, J. J., Metselaar, K., Limpens, J., Teutschbein, C., Peichl, M., Nilsson, M. B., Berendse, F., and van der Zee, S. E. A. T. M.: Including hydrological self-regulating processes in peatland models: Effects on peatmoss drought projections, Sci. Tot. Environ., 580, 1389–1400, https://doi.org/10.1016/j.scitotenv.2016.12.104, 2017.
Orlowsky, B. and Seneviratne, S. I.: Global changes in extreme events: regional and seasonal dimension, Clim. Change, 110, 669–696, https://doi.org/10.1007/s10584-011-0122-9, 2012.
Peichl, M., Oquist, M., Lofvenius, M.O., Ilstedt, U., Sagerfors, J., Grelle, A., Lindroth, A., and Nilsson, M. B.: A 12-year record reveals pre-growing season temperature and water table level threshold effects on the net carbon dioxide exchange in a boreal fen, Environ. Res. Lett., 9, 055006, https://doi.org/10.1088/1748-9326/9/5/055006, 2014.
Petrone, R. M., Price, J. S., Waddington, J. M., and von Waldow, H.: Surface moisture and energy exchange from a restored peatland, Québec, Canada, J. Hydrol., 295, 198–210, https://doi.org/10.1016/j.jhydrol.2004.03.009, 2012.
Piao, S., Yin, L., Wang, X., Ciais, P., Peng, S., Shen, Z., and Seneviratne, S. I.: Summer soil moisture regulated by precipitation frequency in China, Environ. Res. Lett., 4, 044012, https://doi.org/10.1088/1748-9326/4/4/044012, 2009.
Potvin, L. R., Kane, E. S., Chimner, R. A., Kolka, R. K., and Lilleskov, E. A.: Effects of water table position and plant functional group on plant community, aboveground production, and peat properties in a peatland mesocosm experiment (PEATcosm), Plant Soil, 387, 277–294, https://doi.org/10.1007/s11104-014-2301-8, 2015.
Price, J. S.: Soil moisture, water tension, and water table relationships in a managed cutover bog, J. Hydrol., 202, 21–32, https://doi.org/10.1016/S0022-1694(97)00037-1, 1997.
Price, J. S. and Whitehead, G. S.: Developing hydrologic thresholds for Sphagnum recolonization on an abandoned cutover bog, Wetlands, 21, 32–40, https://doi.org/10.1672/0277-5212(2001)021[0032:DHTFSR]2.0.CO;2, 2001.
Radu, D. D. and Duval, T. P.: Impact of rainfall regime on methane flux from a cool temperate fen depends on vegetation cover, Ecol. Eng, 114, 76–87, https://doi.org/10.1016/j.ecoleng.2017.06.047, 2018a.
Radu, D. D. and Duval, T. P.: Precipitation frequency alters peatland ecosystem structure and CO2 exchange: contrasting effects on moss, sedge, and shrub communities, Glob. Change Biol., 24, 2051–2065, https://doi.org/10.1111/gcb.14057, 2018b.
Riutta, T., Laine, J., and Tuittila, E.-S.: Sensitivity of CO2 exchange of fen ecosystem components to water level variation, Ecosystems, 10, 718–733, https://doi.org/10.1007/s10021-007-9046-7, 2007.
Robroek, B. J. M., Schouten, M. G. C., Limpens, J., Berendse, F., and Poorter, H.: Interactive effects of water table and precipitation on net CO2 assimilation of three co-occuring Sphagnum mosses differing in distribution above the water table, Glob. Change Biol., 13, 397–411, https://doi.org/10.1111/j.1365-2486.2008.01724.x, 2009.
Roulet, N. T., Lafleur, P. M., Richard, P. J. H., Moore, T. R., Humphreys, E. R., and Bubier, J.: Contemporary carbon balance and late Holocene carbon accumulation in a northern peatland, Glob. Change Biol., 13, 397–411, https://doi.org/10.1111/j.1365-2486.2006.01292.x, 2007.
Rudolph, H., Kirchhoff, M., and Gliesmann, S.: Sphagnum culture techniques, eduted by: Glime, J. M., Methods in Bryology, Proceedings of the Bryological Methods Workshop, Mainz, Hattori Botanical Laboratory, Nichinan, 25–34, 1988.
Rydin, H. and Jeglum, J.: The Biology of Peatlands, Oxford University Press, Oxford, 2006.
Schipperges, B. and Rydin, H. Response of photosynthesis of Sphagnum species from contrasting microhabitat to tissue water content and repeated desiccation, New Phytol., 140, 677–684, https://doi.org/10.1046/j.1469-8137.1998.00311.x, 1998.
Sillmann, J., Kharin, V. V., Zwiers, F. W., Zhang, X., and Bronaugh, D.: Climate extremes indices in the CMIP5 model ensemble: Part 2: Future climate projections, J. Geophys. Res.-Atmos, 118, 2473–2493, https://doi.org/10.1002/jgrd.50188, 2013.
Silvan, N., Tuittila, E.-S., Kitunen, V., Vasander, H., and Laine, J.: Nitrate uptake by Eriophorum vaginatum controls N2O production in a restored peatland, Soil Biol. Biochem., 37, 1519–1526, https://doi.org/10.1016/j.soilbio.2005.01.006, 2004.
Silvola, J., Alm, J., Ahlholm, U., Nykanen, H., and Martikainen, P. J.: CO2 fluxes from peat in boreal mires under varying temperature and moisture conditions, J. Ecology, 84, 219–228, 1996.
Soulis, E. D., Sarhadi, A., Tinel, M., and Suthar, M.: Extreme precipitation time trends in Ontario, 1960–2010, Hydrol. Proc., 30, 4090–4100, 2016.
Strack, M., Waddington, J. M., Rochefort, L., and Tuittila, E.: Response of vegetation and net ecosystem carbon dioxide exchange at different peatland microforms following water table drawdown, J. Geophys. Res.-Biogeo., 111, G02006, https://doi.org/10.1029/2005JG000145, 2006.
Strack, M. and Price, J. S.: Moisture controls on carbon dioxide dynamics of peat-Sphagnum monoliths, Ecohydrol., 2, 34–41, https://doi.org/10.1002/eco.36, 2009.
Strack, M., Waddington, J. M., Lucchese, M. C., and Cagampan, J. P.: Moisture controls on CO2 exchange in a Sphagnum-dominated peatland: results from an extreme drought field experiment, Ecohydrol., 2, 454–461, https://doi.org/10.1002/eco.68, 2009.
Strack, M., Keith, A. M., and Xu, B.: Growing season carbon dioxide and methane exchange at a restored peatland on the Western Boreal Plain, Ecol. Eng., 64, 231–239, https://doi.org/10.1016/j.ecoleng.2013.12.013, 2014.
Szumigalski, A. R. and Bayley, S. E.: Net above-ground production along a bog-rich fen gradient in central Alberta, Canada, Wetlands, 16, 467–476, 1996.
Takagi, K., Tsuboya, T., Takahashi, H., and Inoue, T.: Effect of the invasion of vascular plants on heat and water balance in the Sarobetsu mire, northern Japan, Wetlands, 19, 246–254, 1999.
Takashi, H., Hiroyuki, Y., Masayuki, T., Yoshiyasu, F., Hiroko, F., and Hidenori, T.: Effects of the expansion of vascular plants in Sphagnum-dominated bog on evapotranspiration, Agricult. Forest Meteorol., 220, 90–100, https://doi.org/10.1016/j.agrformet.2016.01.039, 2016.
Thompson, D. K. and Waddington, J. M.: Sphagnum under pressure: towards an ecohydrological approach to examining Sphagnum productivity, Ecohydrol., 1, 299–308, https://doi.org/10.1002/eco.31, 2008.
Trenberth, K. E.: Changes in precipitation with climate change, Climate Res., 47, 123–138, https://doi.org/10.3354/cr00953, 2011.
Tuittila, E.-S., Vasander, H., and Laine, J.: Sensitivity of C sequestration in reintroduced Sphagnum to water-level variation in a cutaway peatland, Restor. Ecol., 12, 483–493, https://doi.org/10.1111/j.1061-2971.2004.00280.x, 2004.
Vervoort, R. W. and van der Zee, S. E. A. T. M.: Simulating the effect of capillary flux on the soil water balance in a stochastic ecohydrological framework, Water Resour. Res., 44, W08425, https://doi.org/10.1029/2008WR006889, 2008.
Vile, M. M., Scott, K. D., Brault, E., Wieder, R. K., and Vitt, D. H.: Living on the edge: the effects of drought on Canada's western boreal peatlands, edited by: Tuba, Z. and Slack, N. G., Bryophyte Ecology and Climate Change, Cambridge: Cambridge University Press, 277–297, 2011.
Waddington, J. M. and Roulet, N. T.: Carbon balance of a boreal patterned peatland, Glob. Change Biol., 6, 87–97, https://doi.org/10.1046/j.1365-2486.2000.00283.x, 2000.
Walsh, J., Wuebbles, D., Hayhoe, K., Kossin, J., Kunkel, K., Stephens, G., Thorne, P., Vose, R., Wehner, M., Willis, J., Anderson, D., Kharin, V., Knutson, T., Landerer, F., Lenton, T., Kennedy, J., and Somerville, R.: Appendix 3: Climate Science Supplement. Climate Change Impacts in the United States: The Third National Climate Assessment, Melillo, J. M., Terese (T.C.) Richmond, and Yohe, G. W., Eds., U.S. Global Change Research Program, 735–778, 2014.
Wang, X., Huang, G., and Liu, J.: Projected increases in intensity and frequency of rainfall extremes through a regional climate modeling approach, J. Geophys. Res.-Atmos., 119, 13271–13286, https://doi.org/10.1002/2014JD022564, 2014.
Weltzin, J. F., Harth, C., Bridgham, S. D., Pastor, J., and Vonderharr, M.: Production and microtopography of bog bryophytes: response to warming and water-table manipulations, Oecologia, 128, 557–565, https://doi.org/10.1007/s004420100691, 2001.
Westra, S., Fowler, H. J., Evans, J. P., Alexander, L. V., Berg, P., Johnson, F., Kendon, E. J., Lenderink, G., and Roberts, N. M.: Future changes to the intensity and frequency of short-duration extreme rainfall, Rev. Geophys., 52, 522–555, https://doi.org/10.1002/2014RG000464, 2014.
Whittington, P. N. and Price, J. S.: The effects of water table draw-down (as a surrogate for climate change) on the hydrology of a fen peatland, Canada, Hydrol. Proc., 20, 3589–3600, https://doi.org/10.1002/hyp.6376, 2006.
Wilcox, K. R., von Fischer, J. C., Muscha, J. M., Petersen, M. K., and Knapp, A. K.: Contrasting above- and belowground sensitivity of three Great Plains grasslands to altered rainfall regimes, Glob. Change Biol., 21, 335–344, https://doi.org/10.1111/gcb.12673, 2015.
Wu, C., Chen, J. M., Pumpanen, J., Cescatti, A., Marcolla, B., Blanken, P. D., Ardö, J., Tang, Y., Magiulo, V., Georgiadis, T., Soegaard, H., Cook, D. R., and Harding, R. J.: An underestimated role of precipitation frequency in regulating summer soil moisture, Environ. Res. Lett., 7, 024011, https://doi.org/10.1088/1748-9326/7/2/024011, 2012.
Wu, J., Roulet, N. T., Sagerfors, J., and Nilsson, M. B.: Simulation of six years of carbon fluxes for a sedge-dominated oligotrophic minerogenic peatland in northern Sweden using the McGill Wetland model (MWM), J. Geophys. Res.-Biogeo., 118, 795–807, https://doi.org/10.1002/jgrg.20045, 2013.
Yu, Z. C.: Northern peatland carbon stocks and dynamics: a review, Biogeosciences, 9, 4071–4085, https://doi.org/10.5194/bg-9-4071-2012, 2012.