the Creative Commons Attribution 4.0 License.
the Creative Commons Attribution 4.0 License.
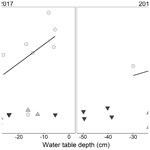
Wildfire overrides hydrological controls on boreal peatland methane emissions
Scott J. Davidson
Christine Van Beest
Richard Petrone
Maria Strack
Boreal peatlands represent a globally important store of carbon, and disturbances such as wildfire can have a negative feedback to the climate. Understanding how carbon exchange and greenhouse gas (GHG) dynamics are impacted after a wildfire is important, especially as boreal peatlands may be vulnerable to changes in wildfire regime under a rapidly changing climate. However, given this vulnerability, there is very little in the literature on the impact such fires have on methane (CH4) emissions. This study investigated the effect of wildfire on CH4 emissions at a boreal fen near Fort McMurray, Alberta, Canada, that was partially burned by the Horse River Wildfire in 2016. We measured CH4 emissions and environmental variables (2017–2018) and CH4 production potential (2018) in two different microform types (hummocks and hollows) across a peat burn severity gradient (unburned (UB), moderately burned (MB), and severely burned (SB)). Results indicated a switch in the typical understanding of boreal peatland CH4 emissions. For example, emissions were significantly lower in the MB and SB hollows in both years compared to UB hollows. Interestingly, across the burned sites, hummocks had higher fluxes in 2017 than hollows at the MB and SB sites. We found typically higher emissions at the UB site where the water table was close to the surface. However, at the burned sites, no relationship was found between CH4 emissions and water table, even under similar hydrological conditions. There was also significantly higher CH4 production potential from the UB site than the burned sites. The reduction in CH4 emissions and production in the hollows at burned sites highlights the sensitivity of hollows to fire, removing labile organic material for potential methanogenesis. The previously demonstrated resistance of hummocks to fire also results in limited impact on CH4 emissions and likely faster recovery to pre-fire rates. Given the potential initial net cooling effect resulting from a reduction in CH4 emissions, it is important that the radiative effect of all GHGs following wildfire across peatlands is taken into account.
Please read the corrigendum first before continuing.
-
Notice on corrigendum
The requested paper has a corresponding corrigendum published. Please read the corrigendum first before downloading the article.
-
Article
(1207 KB)
- Corrigendum
-
Supplement
(256 KB)
-
The requested paper has a corresponding corrigendum published. Please read the corrigendum first before downloading the article.
- Article
(1207 KB) - Full-text XML
- Corrigendum
-
Supplement
(256 KB) - BibTeX
- EndNote
Northern peatlands are an important component of the global carbon (C) cycle, acting as long-term sinks of atmospheric carbon dioxide (CO2). They are also large sources of methane (CH4) (Bridgham et al., 2013), with northern peatlands contributing approximately 40–155 Tg to global CH4 emissions (Neef et al., 2010; Turetsky et al., 2014). CH4 dynamics in peatlands results from a combination of various biogeochemical processes (Lai, 2009). Controls on CH4 production, oxidation, and emissions include microtopography (Cresto Aleina et al., 2016), water table depth (Bubier et al., 1995; Granberg et al., 1997), soil temperature (Granberg et al., 1997; Saarnio et al., 1998), substrate quality and availability (Granberg et al., 1997; Segers, 1998; Joabsson et al., 1999), and vegetation cover (Ström et al., 2005; Strack et al., 2017).
However, disturbances such as wildfire can have a significant impact on the magnitude of C fluxes across peatlands (fire can release between 10 and 85 kg C m−2 through combustion and smouldering; Turetsky et al., 2011), potentially causing a negative feedback to the climate (Randerson et al., 2006). Western boreal Canada is undergoing increasing pressure from wildfire, with fire extent and frequency expected to double by the end of this century (Benscoter et al., 2005; Flannigan et al., 2008). Understanding how ecosystem C cycling and greenhouse gas (GHG) dynamics are impacted after a wildfire is important, especially as boreal peatlands may be vulnerable to changes in wildfire regime under a rapidly changing climate (Flannigan et al., 2008). Fire can remove surface vegetation, increasing net radiation at the ground surface (Brown et al., 2015), and can “re-set” vegetation communities back to the primary succession stage (Johnstone, 2006; Benscoter and Vitt, 2008). Fire can alter soil organic matter quality in the soil column (Neff et al., 2005; Olefeldt et al., 2013a) and reduce belowground C stores in peatlands (Wilkinson et al., 2018). Overall, wildfire can lead to a decrease in C accumulation rate through combustion loss, reduction in vegetation productivity, and increased organic matter decomposition post-fire (Ingram et al., 2019; Robinson and Moore, 2000; Wieder et al., 2009). Furthermore, increased ash deposition after wildfire can increase soil pH (Molina et al., 2007; Davies et al., 2013) and change the physical characteristics of the soil, including blocking of peat macropores and altering hydrology (Noble et al., 2017). Reduction in vegetation cover and soil organic matter can also lead to drier conditions across peatlands (Tarnocai, 2009; Thompson and Waddington, 2013; Kettridge et al., 2015), with the drop in water table level causing an increase in the aerobic zone (Waddington et al., 2015). This could lead to a reduction in CH4 emissions or even uptake of CH4 via oxidation (Strack et al., 2004; Turetsky et al., 2008; Moore et al., 2011). Conversely, high water tables can occur post-fire (Kettridge et al., 2015), although they are often associated with low surface moisture contents due to hydrophobicity of the peat (Doerr et al., 2000). Low soil moisture rates can also occur under increased ash deposition after fire, with increased closure of soil pores by ash causing reduced capacity to hold water and increased runoff (Heydari et al., 2017).
Microtopography (microforms) across peatlands can be impacted through fire by increasing the prominence of hollows (low-lying areas close to the water table; Belyea and Clymo, 1998) on the landscape through altering elevation (Benscoter et al., 2015), and often hollows will have a higher severity of burn compared to other areas across the landscapes (Mayner et al., 2018; Benscoter et al., 2005). Conversely, hummocks (mounded microtopography, approximately 0.2 m or higher above the water table; Belyea and Clymo, 1998) are generally resistant to fire, namely due to moisture retention differences between the different moss species present at both microform types, as Sphagnum spp. is much more resilient to fire than feather moss (Kettridge et al., 2015).
Despite the increasing pressures from wildfires across northern peatlands, a knowledge gap still persists on CH4 emissions after wildfire, especially in boreal regions. In a study on the impact of wildfire on methanotrophic communities from an ombrotrophic peat bog, Danilova et al. (2015) found a reduction in the activity of the methanotrophs in burned sites 7 years post-fire. This reduction following wildfire could therefore lead to a potential increase in CH4 emissions from bog systems. Grau-andrés et al. (2019) also showed an increase in CH4 emissions at an ombrotrophic bog in the UK 1 year after a prescribed fire, most likely due to increased graminoid coverage. Conversely, studies at other bog sites in the UK report a decrease in emissions after fire (Ward et al., 2007; Davies et al., 2013). In non-peatland ecosystems across boreal regions, wildfire has been shown to cause an increase in CH4 uptake (Burke et al., 1997; Song et al., 2017, 2018). However, in permafrost zones, wildfires can often typically lead to substantial permafrost thaw and increasing moisture levels across the landscape (Gibson et al., 2018), potentially leading to an increase in CH4 emissions (Kim and Tanaka, 2003; Turetsky et al., 2008; Olefeldt et al., 2013b; Helbig et al., 2017). However, Köster et al. (2017, 2018) found an increase in CH4 uptake across continuous permafrost sites in both Canada and Russia after fire. To date, we cannot find any reference on the impact of fire on CH4 emissions across fens, despite being the dominant peatland type in western boreal Canada (Vitt et al., 2000).
Therefore, the objectives of this study are to (i) determine the impact of wildfire on fen CH4 emissions across a peat burn severity gradient; (ii) evaluate the controls on CH4 emissions within each site; and (iii) examine CH4 production potential across a peat burn severity gradient. We hypothesize that CH4 emissions and production will be lower at burned sites due to lowering of the water table, changes in substrate availability, and reduction in vegetation cover.
2.1 Study site and collar locations
The study was undertaken in a treed moderate-rich fen (hereafter referred to as Poplar Fen), 20 km north of Fort McMurray, Alberta, Canada (56∘56.330′ N, 111∘32.934′ W), which was partially burned by the Horse River Wildfire in 2016. The mean annual temperature (1981–2010) is 1 ∘C and the mean annual precipitation is approximately 420 mm (Environment Canada, 2017). This treed fen is dominated by Larix laricina (Du Roi) K.Koch, Picea mariana (Mill.) Britton, Betula pumila (L.), Equisetum fluviatile (L.), Smilacina trifolia (L.) Sloboda, Carex spp., and Sphagnum fuscum (Schimp.) Klinggr and brown mosses, largely Tomenthypnum nitens (Hedwig) Loeske. All vegetation was identified to the species level, with nomenclature for vascular plants and mosses as per the Flora of North America Editorial Committee (1993). Average peat depth ranges between 1 and 1.5 m. The landscape consists of approximately 47 % hummocks and 53 % hollows within the fen area (Gabrielli, 2016). This site was split into three sections along a peat burn severity gradient as assessed by depth of burn (DOB). The unburned (UB) site was situated within the fen interior and was not affected by the wildfire. The moderately burned (MB) site had a DOB of approximately 9–11 cm and the severely burned (SB) site had a DOB of approximately 14.5–17 cm. Both burned sites were situated on the peatland margin, closer to the adjacent upland at a slightly higher elevation. The DOB was determined following the protocols used by Lukenbach et al. (2015a). In summary, this method assumes a pre-fire flat surface between multiple reference points across the site, including adventitious roots in the burned sites and unburned reference points. A string is attached between two reference points and 10 measurements were taken along the length, from string to burned ground surface, giving an estimate of the depth of the burn. At each site, PVC collars (height 15 cm × diameter 20 cm) were placed in both hollows and hummocks. A total of four replicate collars were placed at each microform location at each site to a depth of approximately 15 cm in spring 2017, totaling eight collars at the UB site, eight collars at the MB site, and eight collars at the SB site. The height of the collar was measured from the soil surface in order to have the correct chamber headspace volume for CH4 emission calculations.
2.2 Environmental conditions
Water table (WT) depth (relative to the ground surface) was measured adjacent to each pair of collars at all three sites. A PVC pipe (4 cm (diameter) × 100 cm (length)) fully slotted along the full length and covered in mesh was used. A soil temperature (ST) profile was collected at each collar during each CH4 measurement at −30, −25, −20, −15, −10, −5, and −2 cm from the ground surface. Percentage cover of plant functional type (bryophyte and overstory graminoid and dwarf shrub) as well as bare ground, burned material, and standing water were estimated from photographs taken at peak growing season during 2018 to produce percentage cover estimates of the flux collars.
2.3 Measurements of field CH4 emissions
Methane fluxes were measured using the closed chamber method, 8 times between 7 May and 16 August 2017 and 14 times between 11 May and 16 August 2018. A cylindrical opaque chamber (20 cm × 50 cm) was placed on the collar, with water poured around the collar edge to create a seal. A battery-powered fan was used to mix the chamber headspace. A thermocouple located within the chamber, attached to a thermometer, was used to measure temperature during sampling. A 20 mL syringe was used to collect gas samples at intervals of 7, 15, 25, and 35 min following chamber closure and injected into Exetainers (Labco, UK). A gas chromatograph (GC; Shimadzu GC2014, Mandel Scientific, Canada) with a flame ionization detector (250 ∘C), helium gas carrier, and standards of 5 and 50 ppm was used to determine CH4 concentration of the gas samples collected during the field seasons. The emissions were determined from the linear change in concentration over time, which includes corrections for temperature and volume of the chamber. Any small negative or positive values in which the change in concentration did not exceed 10 % (precision of concentration analysis) were assigned a zero-emission value. Large negative emissions ( mg CH4 m2 d−1) were removed from the analysis as it is unlikely this system would have consumption rates as large as this and were likely caused by disturbance during chamber placement. These procedures resulted in a total loss of 6 % of the data across both years. In order to determine whether emissions measured from the UB site were representative of emissions from Poplar Fen as a whole, we also compared our fluxes to a previous study of CH4 emissions collected between 2011 and 2014 at the fen.
2.4 Potential CH4 production
Peat samples were collected adjacent to each collar (2–3 m away to avoid disturbing the collar) on 13 August 2018 and immediately shipped to the laboratory and frozen until analyzed. Peat samples were obtained using a tin can to a depth of 20 cm from the ground surface (two samples were collected per sampling location: 0–10 and 10–20 cm).
Potential CH4 production was determined under anaerobic conditions following a similar methodology to Strack et al. (2004). Peat slurries of approximately 20 g of wet peat were made in 250 mL incubation jars. Distilled water was added to the sample to saturate, without allowing for standing water. Samples were flushed with N2 for 15 min and then sealed. Slurries were incubated at room temperature (approximately 20 ∘C) and sampled at 0, 24, and 48 h and then twice weekly between 16 October 2018 and 2 November 2018 (total incubation length of 17 d). Samples were agitated by hand before sampling commenced to mix the gases within the peat pore spaces and the jar headspace.
Samples (10 mL) were extracted from the jars and injected into a Fast Methane Analyzer (FMA; Los Gatos, USA). A 10 mL sample of N2 was replaced in each jar after the sample was extracted to maintain headspace pressure. Potential methane production was determined from the linear increase in CH4 concentration within the jars over the incubation period after correcting for dilution by N2 (Strack et al., 2017). Gravimetric soil moisture (GWC) was determined by weighing subsamples of peat not used in the incubation, drying the samples at 60 ∘C for 2–3 d, and reweighing. Organic matter content was determined by loss on ignition (LOI), burning samples at 550 ∘C for 4 h.
2.5 Data analysis
All statistical analysis was undertaken in R (R Core Team, 2013) using the package nlme (Pinheiro et al., 2018), and all output and models were inspected for normality and homogeneity of residuals (Zuur et al., 2009). Data were log transformed if required, with a value of 10 being added prior to transformation to account for zeros and negative values in the dataset. Seasonal mean values of CH4 flux and associated environmental variables at each collar were used in all model levels (Treat et al., 2007; Turetsky et al., 2014), with statistical significance considered at α=0.05.
In order to evaluate the effect of burn severity on CH4 flux, a linear mixed effects model (LMM) was used with burn severity, microform, the two-way interactions between these, and year as fixed factors, with collar ID as a random effect to take into account repeated measures (Pinheiro et al., 2018). Another LMM was used to evaluate the environmental controls on CH4 flux, with the effect of burn severity, WT, ST at 30 cm depth, and the two-way interactions between each included as fixed effects. If significant factors were found, Tukey pairwise comparisons were completed using the lsmeans package (Lenth, 2016). Any insignificant factors were removed from the model until the final model was found. An individual insignificant factor was kept in the model if its interaction with another factor was significant. The amount of variance explained by the model () was calculated using the method described by Nakagawa and Schielzith (2013).
A two-way analysis of variance (ANOVA) was used to test for differences between CH4 production rate, burn severity, and microform. No significant difference between sampling depths was found, and thus depths were combined in further analyses. Again, if significant differences were found, Tukey pairwise comparisons were completed.
3.1 Environmental and vegetation variables
Water table depth was linked to microtopographic position, with hollows having the highest WT position across all sites. The deepest WT depth (± standard deviation) during both 2017 and 2018 was found at the SB hummocks, approximately and cm below the surface, respectively (Table 1). The shallowest WT was found at the unburned hollows, being and cm below the surface in 2017 and 2018, respectively (Table 1). Average ST at 30 cm depth (taken as spot measurement during each gas flux measurement) was similar across the burn severity gradient, at approximately 10–12 ∘C (Table 1).
Table 1Environmental characteristics (mean (± standard deviation)) for each microform type across the burn severity gradient.
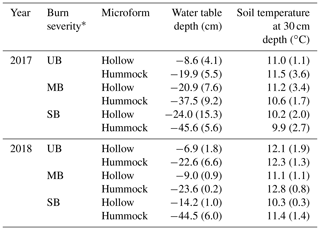
* UB is unburned, MB is moderately burned and SB is severely burned.
The vegetation survey of the collars undertaken in 2018 indicated that bryophytes dominated across all collars at all sites, regardless of microtopographic position. UB hollows were dominated by the moss T. nitens, while the hummocks were dominated by S. fuscum. The SB hummocks and hollows both had the highest percentage of bare ground at ∼49 % and ∼55 % cover, respectively, indicating vegetation (mostly T. nitens) was completely removed during the fire. It was noted that Polytrichum strictum Bridel, J. Bot (Schrader) moss was beginning to colonize these bare areas. The MB hummocks had the highest percentage of burned material (∼40 % cover; predominantly singed S. fuscum) (Fig. 1).
3.2 CH4 emissions
The average CH4 flux (± standard deviation) at unburned hollows was 126.5±80.5 and 56.3±18.9 mg CH4 m−2 d−1 in 2017 and 2018, respectively (Fig. 2). CH4 emissions were much lower in the MB and SB hollows in both years, with the average flux being and mg CH4 m−2 d−1 in 2017 and 0.21±1.7 and 0.62±2.5 mg CH4 m−2 d−1 in 2018 (Fig. 2), respectively. Interestingly, across the burned sites, hummocks had higher fluxes in 2017 than hollows, with the average flux being 1.10±2.04 mg CH4 m−2 d−1 at the MB site and 4.53±9.3 mg CH4 m−2 d−1 at the SB site (Fig. 2). During 2018, fluxes were lower, with hummocks being a slight sink of CH4 with average fluxes of mg CH4 m−2 d−1 at the MB site and 0.43±1.6 mg CH4 m−2 d−1 at the SB site (Fig. 2). No significant difference in emissions was found (t(22.5)=1.5, p=0.154) between the present study and the study undertaken between 2011 and 2014 (Fig. S1 in the Supplement).
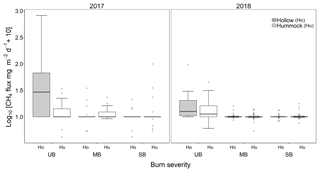
Figure 2Methane (CH4) emissions at each microform type across the peat burn severity gradient for 2017 and 2018. UB is unburned, MB is moderately burned and SB is severely burned. Note that CH4 values were log transformed +10; therefore, a value of 1 represents the measured value zero.
Results of the LMM illustrate that there was a significant effect of burn on CH4 flux (Table 2) but no significant effect of microform or year (Table 2). The second LMM considering environmental controls explained 63 % of the variance in CH4 emissions and found a significant interaction between burn severity and WT depth (Table 2; Fig. 3) but no significant relationship between CH4 flux with WT depth, ST (Fig. S2) or burn severity alone.
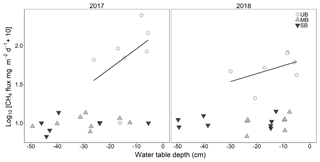
Figure 3Seasonal mean methane (CH4) flux at each collar across the peat burn severity gradient plotted against seasonal mean water table (WT) depth. UB is unburned, MB is moderately burned and SB is severely burned. Note that CH4 values were log transformed +10; therefore, a value of 1 represents the measured value zero.
Table 2Statistical results of the linear mixed effects modela.
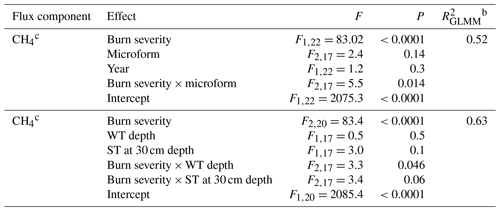
a All models have a random factor of collar ID to take into account the repeated measures across both years. b We report the marginal accounting for variance explained by fixed factors only. c The model was calculated using log10 CH4 values.
3.3 Potential CH4 production
Measured potential CH4 production was highest in the unburned hollows ranging from between 0.006 and 0.13 µg g−1 peat h−1 (Fig. 4); however, there was no significant effect of burn severity (ANOVA, F=2.959, p=0.065). CH4 production was much lower across the burned sites, ranging between 0.0001 and 0.004 µg g−1 peat h−1. The MB hummocks followed a similar pattern to the field measurements of CH4 flux, having higher potential CH4 production than the hollows. No significant difference in organic matter content or gravimetric water content was found between sites or microform types (Table S1 in the Supplement).
Fire had a strong effect on CH4 emissions in this study, causing a large decrease in CH4 flux in the MB and SB hollows in comparison to the UB hollows. Conversely, this study also highlights the resistance of hummocks to fire (Wieder et al., 2009; Benscoter et al., 2015), with hummocks across the burned sites maintaining higher CH4 emissions after the fire compared to hollows. Methane production in the laboratory followed a similar trend to the field study, with the highest production in the UB hollows and virtually no production in the burned hollows, again highlighting this reversal of typical peatland CH4 emissions. These results contrast with other studies looking at CH4 emissions post-fire at peatland sites, with Danilova et al. (2015) indicating that fire across an ombrotrophic bog could decrease CH4 oxidation due to removal of the methanotrophic community, while Grau-andrés et al. (2019) note a potential increase in CH4 emissions due to increased graminoid cover. We did not specifically measure CH4 oxidation in this study.
We hypothesized that the lower CH4 emissions at the burned sites could be due to the intensity of the burn, reducing substrate availability (labile carbon) and minimizing the methanogenesis community, resulting in lower emissions. An increase in fire frequency has the potential to reduce organic matter quality and change vegetation communities in peatlands (Lukenbach et al., 2015b). Associated with a change in vegetation communities is the potential change in biogeochemical cycling and microbial processes (Ward et al., 2007). For example, the slight recovery in CH4 emissions in 2018 (2 years post-fire) could be due to vegetation recovery (Ward et al., 2007), providing more available substrate through root exudates for CH4 production (Greenup et al., 2000; Robroek et al., 2015). The presence of graminoids in the SB hollows post-fire was also found, which could also lead to increasing CH4 emissions in the future, as plant-mediated transport of CH4 is well documented across peatland ecosystems (Ström et al., 2005).
Higher emissions at the UB site could result from overall shallower WT at this location compared to the MB and SB sites (Table 1), which were located at the fen margins. Poplar Fen has a highly variable connection to groundwater (Elmes et al., 2018) and the hydrogeologic setting of Poplar Fen likely contributed to the limited effect of the wildfire at this location, but could also result in higher CH4 emissions than would have occurred naturally at the burned sites prior to the fire. However, the comparison of our results to emissions measured between 2011 and 2014 at another location in Poplar Fen burned during the fire indicates there was no significant difference in CH4 emissions. Interestingly, we see no relationship with CH4 emissions and WT depth at the burned sites. This switch in the typical understanding of the relationship between CH4 emissions and WT further strengthens our argument on the overriding influence of fire. Even under suitable hydrological conditions, there is a lack of CH4 production, as shown in the incubation study. Removal of vegetation and soil organic matter can lead to drier conditions (Thompson and Waddington, 2013), with a lower water table creating a larger aerobic zone, potentially leading to lower rates of CH4 production and potentially greater rates of CH4 consumption (Strack et al., 2004; Moore et al., 2011). However, fire can also cause a higher water table, which could potentially lead to larger anaerobic zones and potentially higher CH4 emissions. However, this is dependent on the severity of the burn, where a low-severity fire which only removes vegetation and does not impact the microbial community and organic matter content of the soil may still allow for CH4 production. Conversely, a high-severity burn which potentially has removed these communities and organic matter may no longer allow for CH4 production, even with suitable hydrological conditions.
The higher CH4 production found at the MB hummocks is likely due to the small methanogen community surviving the fire, due to the resistance of S. fuscum to fire (Benscoter et al., 2011). After fire, there could be chemical changes in the soil substrate, such as an increase in availability of terminal electron acceptors, that could contribute to the reduction in CH4 production and emissions (Wilson et al., 2017). Therefore, there is a potential long-term impact on the biogeochemical processes of peatlands (Danilova et al., 2015), and in order to fully understand the overall impact of wildfire on CH4 emissions, additional studies at other sites encompassing the full range of boreal peatland types would be key. This is especially true given the conflicting results in the literature regarding the overall impact of fire across a variety of peatland sites. Continuous monitoring of the recovery of the ecosystem over time could help evaluate the amount of time required for CH4 emissions to return to similar levels to the undisturbed site.
This study investigated the impact of wildfire on CH4 emissions at a treed, moderate-rich fen in northern Alberta. We believe this is the first study to investigate the impact of wildfire on CH4 emissions at a non-permafrost boreal fen. The results showed a significant impact of fire on the magnitude of CH4 flux, with a significant reduction in flux observed at the burned sites in comparison to the unburned (UB) site. No relationship was found with water table at the burned sites, contrasting with the significant relationship at the UB site, further illustrating that methanogenesis was limited following fire. This was further supported by a lower rate of CH4 production from peat collected at burned sites compared to UB, likely linked to reduced methanogen population and/or substrate availability due to the resistance of Sphagnum spp. hummocks reducing burn severity. With the expected increase in wildfire frequency across western boreal Canada, it is vital we fully understand the impact of fire on CH4 dynamics. If fire is to reduce CH4 emissions and production across these peatland ecosystems, there is a potential initial net cooling effect; therefore, it is important to take into account the radiative effect of all GHGs following wildfire.
The data that support the findings of this study are available from the corresponding author upon reasonable request.
The supplement related to this article is available online at: https://doi.org/10.5194/bg-16-2651-2019-supplement.
MS and RP secured the funding; SJD, CVB, RP, and MS designed the study; SJD performed the research; SJD analyzed the data with input from MS; and SJD, CVB, RP, and MS wrote the paper.
The authors declare that they have no conflict of interest.
Funding for this project was provided by a Natural Sciences and Engineering Research Council of Canada (NSERC) Collaborative Research and Development (CRD) grant to Maria Strack and Richard Petrone co-funded by Suncor Energy Inc., Imperial Oil Resources Limited, Teck and Shell Canada Energy, and an NSERC Discovery Grant awarded to Maria Strack. The authors would like to acknowledge Canada's Oil Sands Innovation Alliance (COSIA) for its support of this project. We thank James Michael Waddington and Manuel Helbig for helpful comments on an earlier version of the manuscript. Finally, we would like to thank Matthew Coulas, Mariah Smith, Dryden Miller, and Emily Prystupa for their help in the field.
This research has been supported by the Natural Sciences and Engineering Research Council of Canada (grant nos. 418557 and 342020).
This paper was edited by Paul Stoy and reviewed by two anonymous referees.
Belyea, L. R. and Clymo, R. S.: Do hollows control the rateof peat bog growth?, in: Patterned mires and mire pools, edited by: Standen, V., Tallis, J. H., and Meade, R., 55–65, British Ecological Society, London, 1998.
Benscoter, B. W. and Vitt, D. H.: Spatial patterns and temporal trajectories of the bog ground layer along a post-fire chronosequence, Ecosystems, 11, 1054–1064, 2008.
Benscoter, B. W., Vitt, D. H., and Wieder, R. K.: Association of postfire peat accumulation and microtopography in boreal bogs, Can. J. Forest Res., 35, 2188–2193, 2005.
Benscoter, B. W., Thompson, D. K., Waddington, J. M., Flannigan, M. D., Wotton, B. M., De Groot, W. J., and Turetsky, M. R.: Interactive effects of vegetation, soil moisture and bulk density on depth of burning of thick organic soils, Int. J. Wildland Fire, 20, 418–429, 2011.
Benscoter, B. W., Greenacre, D., and Turetsky, M. R.: Wildfire as a key determinant of peatland microtopography, Can. J. Forest Res., 45, 1132–1136, 2015.
Bridgham, S. D., Cadillo-Quiroz, H., Keller, J. K., and Zhuang, Q.: Methane emissions from wetlands?: biogeochemical, microbial, and modeling perspectives from local to global scales, Glob. Change Biol., 19, 1325–1346, 2013.
Brown, L. E., Palmer, S. M., Johnston, K., and Holden, J.: Vegetation management with fire modifies peatland soil thermal regime, J. Environ. Manage., 154, 166–176, 2015.
Bubier, J. L., Moore, T. R., Bellisario, L., Comer, N. T., and Crill, M.: Ecological controls on methane emissions from a northern peatland complex in the zone of discontinuous permafrost, Manitoba, Canada, Global Biogeochem. Cy., 9, 455–470, 1995.
Burke, R. A., Zepp, R. G., Tarr, A., Miller, L., and Stocks, J.: Effect of fire on soil-atmosphere exchange of methane and carbon dioxide in Canadian boreal forest sites, J. Geophys. Res., 102, 289–300, 1997.
Cresto Aleina, F., Runkle, B. R. K., Brücher, T., Kleinen, T., and Brovkin, V.: Upscaling methane emission hotspots in boreal peatlands, Geosci. Model Dev., 9, 915–926, https://doi.org/10.5194/gmd-9-915-2016, 2016.
Danilova, O. V., Belova, S. E., Kulichevskaya, I. S., and Dedysh, S. N.: Decline of activity and shifts in the methanotrophic community structure of an ombrotrophic peat bog after wildfire, Microbiology, 84, 624–629, 2015.
Davies, G. M., Gray, A., Rein, G., and Legg, C. J.: Forest Ecology and Management, Peat consumption and carbon loss due to smouldering wildfire in a temperate peatland, Forest Ecol. Manage., 308, 169–177, 2013.
Doerr, S. H., Shakesby, R. A., and Walsh, R. P. D.: Soil water repellency: Its causes, characteristics and hydro-geomorphological significance, Earth Sci. Rev., 51, 33–65, 2000.
Elmes, M. C., Thompson, D. K., Sherwood, J. H., and Price, J. S.: Hydrometeorological conditions preceding wildfire, and the subsequent burning of a fen watershed in Fort McMurray, Alberta, Canada, Nat. Hazards Earth Syst. Sci., 18, 157–170, https://doi.org/10.5194/nhess-18-157-2018, 2018.
Environment Canada: Canadian Climate Normals 1981–2010 Station Data, Government of Canada, Ottawa, available at: http://climate.weather.gc.ca/climate_normals (last access: 30 March 2019), 2017.
Flannigan, M. D., Stocks, B., Turetsky, M. R., and Wotton, M.: Impacts of climate change on fire activity and fire management in the circumboreal forest, Glob. Change Biol., 15, 1–12, 2008.
Flora of North America Editorial Committee: Flora of North America North of Mexico, 19+ Vols., New York, NY, Flora of North America, 1993.
Gabrielli, E. C.: Partitioning Evapotranspiration in Forested Peatlands within the Western Boreal Plain, Fort McMurray, Alberta, Canada, MSc thesis, Wilfrid Laurier University, 2016.
Gibson, C. M., Chasmer, L. E., Thompson, D. K., Quinton, W. L., Flannigan, M. D., and Olefeldt, D.: Wildfire as a major driver of recent permafrost thaw in boreal peatlands, Nat. Commun., 9, 3041, https://doi.org/10.1038/s41467-018-05457-1, 2018.
Granberg, G., Catharina, M., Ingvar, S., Svensson, B. H., and Mats, N.: Sources of spatial variation in methane emission from mires in northern Sweden: A mechanistic approach in statistical modelling, Global Biogeochem. Cy., 11, 135–150, 1997.
Grau-andrés, R., Gray, A., Davies, G. M., Scott, E. M., and Waldron, S.: Burning increases post-fire carbon emissions in a heathland and a raised bog, but experimental manipulation of fire severity has no effect, J. Environ. Manage., 233, 321–328, 2019.
Greenup, A. L., Bradford, M. A., Mcnamara, N. P., Ineson, P., and Lee, J. A.: The role of Eriophorum vaginatum in CH4 flux from an ombrotrophic peatland, Plant Soil, 227, 265–272, 2000.
Heydari, M., Rostamy, A., Najafi, F., and Dey, D. C.: Effect of fire severity on physical and biochemical soil properties in Zagros oak (Quercus brantii Lindl.) forests in Iran, J. Forest Res., 28, 95–104, 2017.
Helbig, M., Chasmer, L. E., Kljun, N., and Quinton, W. L.: The positive net radiative greenhouse gas forcing of increasing methane emissions from a thawing boreal forest-wetland landscape, Glob. Change Biol., 23, 2413–2427, 2017.
Ingram, R. C., Moore, P. A., Wilkinson, S., Petrone, R. M., and Waddington, J. M.: Post-fire soil carbon accumulation does not recover boreal peatland combustion loss in some hydrogeological settings, J. Geophys. Res.-Biogeo., 124, 775–788, https://doi.org/10.1029/2018JG004716, 2019.
Joabsson, A., Christensen, T. R., and Wallen, B.: Vascular plant controls on methane emissions from northern peatforming wetlands, Trends Ecol. Evol., 14, 385–388, 1999.
Johnstone, J. F.: Response of boreal plant communities to variations in previous fire-free interval, Int. J. Wildland Fire, 15, 497–508, 2006.
Kettridge, N., Turetsky, M. R., Sherwood, J. H., Thompson, D. K., Miller, C. A., Bensocter, B. W., Flannigan, M. D., Wotton, B. M., and Waddington, J. M.: Moderate drop in water table increases peatland vulnerability to post-fire regime shift, Sci. Rep.-UK, 5, 8063, https://doi.org/10.1038/srep08063, 2015.
Kim, Y. and Tanaka, N.: Effect of forest fire on the fluxes of CO2, CH4 and N2O in boreal forest soils, interior Alaska, J. Geophys. Res., 108, 8154, https://doi.org/10.1029/2001JD000663, 2003.
Köster, E., Köster, K., Berninger, F., Aaltonen, H., Zhou, X., and Pumpanen, J.: Carbon dioxide, methane and nitrous oxide fluxes from a fire chronosequence in subarctic boreal forests of Canada, Sci. Total Environ., 601–602, 895–905, 2017.
Köster, E., Köster, K., Berninger, F., Prokushkin, A., and Aaltonen, H.: Changes in fluxes of carbon dioxide and methane caused by fire in Siberian boreal forest with continuous permafrost, J. Environ. Manage., 228, 405–415, 2018.
Lai, D. Y. F.: Methane Dynamics in Northern Peatlands?: A Review, Pedosphere, 19, 409–421, 2009.
Lenth, R. V.: Least-Squares Means: The R Package lsmeans, J. Stat. Softw., 69, 1–33, https://doi.org/10.18637/jss.v069.i01, 2016.
Lukenbach, M. C., Hokanson, K. J., Moore, P. A., Devito K. J., Kettridge, N., Thompson, D. K., Wotton, B. M., Petrone, R. M., and Waddington, J. M.: Hydrological controls on deep burning in a northern forested peatland, Hydrol. Process., 29, 4114–4124, 2015a.
Lukenbach, M. C., Devito, K. J., Kettridge, N., Petrone, R. M., and Waddington, J. M.: Hydrogeological controls on post-fire moss recovery in peatlands, J. Hydrol., 530, 405–418, 2015b.
Mayner, K. M., Moore, P. A., Wilkinson, S. L., Petrone, R. M., and Waddington, J. M.: Delineating boreal plains bog margin ecotones across hydrogeological settings for wildfire risk management, Wetl. Ecol. Manag., 26, 1037–1046, 2018.
Molina, M., Fuentes, R., and Calderón, R., Escudey M., Avendaño, K., Gutiérrez, M., and Chang, A.: Impact of forest fire ash on surface charge characteristics of Andisols, Soil Sci., 172, 820–834, 2007.
Moore, T. R., Young, A., Bubier, J. L., Humphreys, E. R., Lafleur, P. M., and Roulet, N. T.: A Multi-Year Record of Methane Flux at the Mer Bleue Bog, Southern Canada, Ecosystems, 14, 646–657, 2011.
Nakagawa, S. and Schielzeth, H.: A general and simple method for obtaining R2 from generalized linear mixed-effects models, Methods Ecol. Evol., 4, 133–142, 2013.
Neef, L., Van Weele, M., and Van Velthoven, P.: Optimal estimation of the present-day global methane budget, Global Biogeochem. Cy., 24, 1–10, 2010.
Neff, J. C., Harden, J. W., and Gleixner, G.: Fire effects on soil organic matter content , composition , and nutrients in boreal interior Alaska, Can. J. Forest Res., 2187, 2178–2187, 2005.
Noble, A., Palmer, S. M., Glaves, D. J., Crowle, A., and Holden, J.: Impacts of peat bulk density, ash deposition and rainwater chemistry on establishment of peatland mosses, Plant Soil, 419, 41–52, 2017.
Olefeldt, D., Devito, K. J., and Turetsky, M. R.: Sources and fate of terrestrial dissolved organic carbon in lakes of a Boreal Plains region recently affected by wildfire, Biogeosciences, 10, 6247–6265, https://doi.org/10.5194/bg-10-6247-2013, 2013a.
Olefeldt, D., Turetsky, M. R., Crill, P. M., and Mcguire, A. D.: Environmental and physical controls on northern terrestrial methane emissions across permafrost zones, Glob. Change Biol., 19, 589–603, 2013b.
Pinheiro, J., Bates, D., DebRoy, S., Sarkar, D., and R Core Team: nlme: Linear and Nonlinear Mixed Effects Models. R package version 3.1-137, available at: https://CRAN.R-project.org/package=nlme (last access: 30 March 2019), 2018.
Randerson, J. T., Liu, H., Flanner, M. G., Chambers, S. D., Jin, Y., Hess, P. G., Pfister, F., Mack, M. C., Treseder, K. K., Welp, L. R., Chapin, F. S., Harden, J. W., Goulden, M. L., Lyons, E., Neff, J. C., Schuur, E. A. G., and Zender, C. S.: The Impact of Boreal Forest Fire on Climate Warming, Science, 314, 1130–1132, 2006.
R Core Team: R: A language and environment for statistical computing. R Foundation for Statistical Computing, Vienna, Austria, available at: http://www.R-project.org/ (last access: 5 July 2019), 2013.
Robinson, S. D. and Moore, T. R.: The Influence of Permafrost and Fire upon Carbon Accumulation in High Boreal Peatlands, Northwest Territories, Canada, Arct. Antarct. Alp. Res., 32, 155–166, 2000.
Robroek, B. J. M., Jassey, V. E. J., Kox, M. A. R., Berendsen, R. L., Mills, R. T. E., Cécillon, L., Puissant, J., Meima-Franke, M., Bakker, P. A. H. M., and Bodelier, P. L. E.: Peatland vascular plant functional types affect methane dynamics by altering microbial community structure, J. Ecol., 103, 925–934, 2015.
Saarnio, S., Alm, J., Martikainen, P. J., and Silvola, J.: Effects of raised CO2 on potential CH4 production and oxidation in, and CH4 emission from, a boreal mire, J. Ecol., 86, 261–268, 1998.
Segers, R.: Methane production and methane consumption: a review of processes underlying wetland methane fluxes, Biogeochemistry, 41 23–51, 1998.
Song, X., Wang, G., Ran, F., Chang, R., Song, C., and Xiao, Y.: Effects of topography and fire on soil CO2 and CH4 flux in boreal forest underlain by permafrost in northeast China, Ecol. Eng., 106, 35–43, 2017.
Song, X., Wang, G., Hu, Z., Ran, F., and Chen, X.: Boreal forest soil CO2 and CH4 fluxes following fire and their responses to experimental warming and drying, Sci. Total Environ., 644, 862–872, 2018.
Strack, M., Waddington, J. M., and Tuittila, E.: Effect of water table drawdown on northern peatland methane dynamics?: Implications for climate change, Global Biogeochem. Cy., 18, 1–7, 2004.
Strack, M., Mwakanyamale, K., Hassanpour Fard, G., Bird, M., Bérubé, V., and Rochefort, L.: Effect of plant functional type on methane dynamics in a restored minerotrophic peatland, Plant Soil, 410, 231–246, 2017.
Ström, L., Mastepanov, M., and Christensen, T. R.: Species-specific effects of vascular plants on carbon turnover and methane emissions from wetlands, Glob. Change Biol., 75, 65–82, 2005.
Tarnocai, C.: The Impact of Climate Change on Canadian Peatlands, Can. Water Resour. J., 34, 453–466, 2009.
Thompson, D. K. and Waddington, J. M.: Peat properties and water retention in boreal forested peatlands subject to wildfire, Water Resour. Res., 49, 3651–3658, 2013.
Treat, C. C., Bubier, J. L., Varner, R. K., and Crill, P. M.: Timescale dependence of environmental and plant-mediated controls of CH4 flux in a temperate fen, J. Geophys. Res.-Biogeo., 112, 1–9, 2007.
Turetsky, M. R., Treat, C. C., Waldrop, M. P., Waddington, J. M., Harden, J. W., and Mcguire, A. D.: Short-term response of methane fluxes and methanogen activity to water table and soil warming manipulations in an Alaskan peatland, J. Geophys. Res., 113, G00A10, https://doi.org/10.1029/2007JG000496, 2008.
Turetsky, M. R., Donahue, W. F., and Benscoter, B. W.: Experimental drying intensifies burning and carbon losses in northern peatland, Nat. Commun., 2, 514–519, 2011.
Turetsky, M. R., Kotowska, A., Bubier, J., and Dise, N. B.: A synthesis of methane emissions from 71 northern, temperate, and subtropical wetlands, Glob. Change Biol., 20, 2183–2197, 2014.
Vitt, D. H., Halsey, L. A., Bauer, I. E., and Campbell, C.: Spatial and temporal trends in carbon storage of peatlands of continental western Canada through the Holocene, Can. J. Earth Sci., 37, 683–693, 2000.
Waddington, J. M., Morris, P. J., Kettridge, N., Granath, G., Thompson, D. K., and Moore, P. A.: Hydrological feedbacks in northern peatlands, Ecohydrology, 8, 113–127, 2015.
Ward, S. E., Bardgett, R. D., McNamara, N. P., Adamson, J. K., and Ostle, N. J.: Long-Term Consequences of Grazing and Burning on Northern Peatland Carbon Dynamics, Ecosystems, 10, 1069–1083, 2007.
Wieder, R. K., Scott, K. D., Kamminga, K., Vile, M. A., Vitt, D. H., Bone, T., Xu, B., Benscoter, B., and Bhatti, J. S.: Postfire carbon balance in boreal bogs of Alberta, Canada, Glob. Change Biol., 15, 63–81, 2009.
Wilkinson, S. L., Moore, P. A., Flannigan, M. D., Wotton, B. M., and Waddington, J. M.: Did enhanced afforestation cause high severity peat burn in the Fort McMurray Horse River wildfire, Environ. Res. Lett., 13, 014018, https://doi.org/10.1088/1748-9326/aaa136, 2018.
Wilson, R. M., Tfaily, M., Rich, V. I., Keller, J. K., Bridgham, S. D., Medvedeff Zalman, C., Meredith, L., Hanson, P. J., Hines, M., Pfeifer-Meister, L., Saleska, S. R., Crill, P., Cooper, W. T., Chanton, J. P., and Kostka, J. E.: Hydrogenation of organic matter as a terminal electron sink sustains high CO2:CH4 production ratios during anaerobic decomposition, Org. Geochem., 112, 22–32, 2017.
Zuur, A. G., Ieno, E. N., Walker, N. J., Saveliev, A. A., and Smith, G. M.: Mixed Effect Models and Extensions in Ecology with R, Springer-Verlag, New York, 547 pp., 2009.
The requested paper has a corresponding corrigendum published. Please read the corrigendum first before downloading the article.
- Article
(1207 KB) - Full-text XML
- Corrigendum
-
Supplement
(256 KB) - BibTeX
- EndNote