the Creative Commons Attribution 4.0 License.
the Creative Commons Attribution 4.0 License.
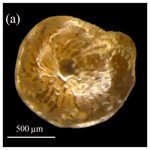
Foraminiferal holobiont thermal tolerance under future warming – roommate problems or successful collaboration?
Doron Pinko
Sigal Abramovich
Danna Titelboim
Understanding the response of marine organisms to expected future warming is essential. Large benthic foraminifera (LBF) are symbiont-bearing protists considered to be major carbonate producers and ecosystem engineers. We examined the thermal tolerance of two main types of LBF holobionts characterized by different algal symbionts and shell types (resulting from alternative biomineralization mechanisms): the hyaline diatom-bearing Amphistegina lobifera and the porcellaneous-dinoflagellate-bearing Sorites orbiculus. In order to assess the holobiont thermal tolerance we separately evaluated foraminiferal calcification rates and symbionts' net photosynthesis under present-day and future warming scenarios. Our results show that both holobionts exhibit progressive loss-of-life functions between 32 and 35 ∘C. This sensitivity differs in the magnitude of their response: calcification of A. lobifera was drastically reduced compared with S. orbiculus. Thus, future warming may significantly shift the relative contribution of the two species as carbonate producers. Moreover, A. lobifera exhibited a synchronous response of calcification and net photosynthesis. In contrast, in S. orbiculus the symbionts decreased net photosynthesis prior to calcification. This implies that algal symbionts limit the resilience of the halobiont.
- Article
(1518 KB) -
Supplement
(358 KB) - BibTeX
- EndNote
Since the beginning of the industrial revolution, anthropogenic activity has led to rapid ocean warming. This negatively affects marine ecosystems and specifically symbiont-bearing calcifiers (Kawahata et al., 2019). The observed rate of global sea surface temperature (SST) rise stands at 0.11 ∘C per decade, and future scenarios predict a similar rate until the end of the century (IPCC, 2014). Therefore, the Mediterranean can be presented in biogeographic models as a “miniature ocean”, providing indications of global patterns in marine ecosystems in a warmer world (Lejeusne et al., 2010). Warming in the Eastern Mediterranean is expected to rise almost 4 times more rapidly than the global forecast (Macias et al., 2013). Thus, the Eastern Mediterranean is expected to be one of the regions most affected by global warming.
Symbiont-bearing large benthic foraminifera (LBF) are single-celled ecosystem engineers. Their carbonate production is estimated to be at least 5 % of the annual production in reef and carbonate shelf environments (Langer, 2008; Langer et al., 1997). Temperature is a major factor in the distribution of LBF that exhibits distinct thresholds for reproduction, survival, bleaching, and calcification (Evans et al., 2015; Hallock et al., 2006a; Langer et al., 2012; Langer and Hottinger, 2000; Schmidt et al., 2011; Titelboim et al., 2019; Weinmann et al., 2013). The symbiont composition of LBF was suggested to be controlled by temperature (Momigliano and Uthicke, 2013; Prazeres, 2018; Prazeres et al., 2017; Prazeres and Renema, 2019; Schmidt et al., 2018), which explains the observation that species-specific thermal tolerance is associated with more diverse algal symbionts (Stuhr et al., 2018).
Many LBF species are Lessepsian invaders, which often comprise over 90 % of the foraminiferal population in the Eastern Mediterranean (Hyams-Kaphzan et al., 2014; Titelboim et al., 2016). Their invasion and successful establishment are facilitated by rising temperatures, as in the case of other Lessepsian organisms (Por, 1978, 2010; Zenetos et al., 2010, 2012). However, some of these species currently live very close to their upper thermal thresholds, and, consequently, their presence will be impeded in the relatively near future (Titelboim et al., 2016). The thermal sensitivity of some LBF species has already been investigated (Schmidt et al., 2011, 2016b; Stuhr et al., 2018; Titelboim et al., 2019). However, the relative contribution (positive or negative) of the holobiont components to cope with rising temperatures has not been fully constrained.
In this study, we present the thermal sensitivity of two very dominant and prominent LBF holobiont systems (Fig. 1). Specifically, our study separately assesses the thermal sensitivity of the foraminiferal host calcification rate and algal symbiont net photosynthesis as an indication of their well-being under different warming scenarios. This approach was chosen since calcification is a physiological activity done only by the foraminifera and thus represents a proxy of its well-being (like many organisms, lowering physiological activities that involve high consumption of energy when stressed). The same is true for photosynthesis, which is a primary life process and thus presents an efficient indicator for the tolerance of the symbiont algae. Because of the exclusiveness of each parameter we could use them to disentangle the complex relationship between the two components of the holobiont.
2.1 Specimen collection and handling
In this study, we targeted two LBF species that represent different types of holobiont systems, which differ in their shell construction mechanism and algal symbionts: Amphistegina lobifera (diatom-bearing, hyaline; Larsen, 1976; Prazeres et al., 2017; Schmidt et al., 2015, 2016b) and Sorites orbiculus (dinoflagellate-bearing, porcellaneous; Merkado et al., 2013; Pawlowski et al., 2001; Pochon et al., 2014). Both species have cosmopolitan distributions, are very common in warm shallow marine environments (Langer and Hottinger, 2000), and display different thermal tolerances (Titelboim et al., 2016). Specimens were picked from macro-algal samples that were scraped from beach rocks at Shikmona, on the northern Mediterranean coast of Israel. To reduce variance in growth derived from ontogenetic variability, the specimens were picked between the specific size fractions of 750 and 1000 µm. Live specimens (indicated by their symbiont colour and motility) were cleaned by brushing, divided into groups with an equal number of specimens (40 S. orbiculus and 30 A. lobifera), and transferred into 60 mL airtight Erlenmeyer flasks filled with natural seawater filtered to 0.45 µm, hereafter referred to as “samples”.
2.2 Laboratory manipulative experiments
We conducted temperature manipulative experiments on S. orbiculus and A. lobifera. In these experiments, the well-being of both holobionts was examined by separately determining the responses of the foraminiferal calcification rate and symbiont algae net photosynthesis to elevated temperatures. These are both very accurate quantitative parameters. As such, they were chosen for this study, which aimed to recognize even subtle differences between treatments and between species.
During the experiments, the samples were placed in temperature-controlled water baths, which maintained constant temperatures of ±0.5 ∘C; temperatures were monitored using HOBO data loggers that recorded the temperature every hour. During the cultivating period, the samples were kept under a daily cycle of 12 h light–12 h darkness using a fluorescent light of ∼30 µmol photons m−2 s−1. These are lower than the photosynthetic optimum for A. lobifera (Ziegler and Uthicke, 2011). However, using these light conditions, we were able to produce data comparable to those presented in related published papers (Schmidt et al., 2016a, b, 2018; Titelboim et al., 2019). These light levels should not cause stress, since LBF acclimate rapidly to different light levels (in under 48 h, Ziegler and Uthicke, 2011), and thus the 10 d acclimation is sufficient for them to adjust to the specific light level provided during the experiment.
All samples were acclimated under constant conditions for at least 10 d. Acclimation temperatures were optimal for each species: 27 ∘C for S. orbiculus and 25 ∘C for A. lobifera, and other conditions are as described in Sect. 2.1. Then, the calcification rate and net photosynthesis were measured to establish the performance baselines of the different species and the natural variability between samples, under equal conditions. Two samples (one A. lobifera replicate from 25 ∘C treatment and one S. orbiculus replicate from 30 ∘C) did not exhibit similar values of net oxygen production to the majority of samples and were excluded from the rest of the study to avoid bias. At the end of the acclimation period, seawater was replaced in all samples, and the temperature of each bath was slowly adjusted (1 ∘C h−1). The examined treatments (25, 30, 32, 35 ∘C) represent current and future temperatures expected in the Eastern Mediterranean until the end of the century (Macias et al., 2013). Each temperature treatment included four replicates, unless reduced to three following the low performance of the symbionts (A. lobifera 25 ∘C and S. orbiculus 30 ∘C). After acclimation, following each week, the water was replaced with fresh natural seawater with a verified pH of 8.0–8.1 and salinity of 38.4–39.2. The replaced water from all the samples was transferred to airtight syringes, and then all oxygen samples were immediately measured. Alkalinity measurements were conducted over the next 2 d. To ensure that no changes occurred in this time frame, standard material was measured before and after the first and last sample of the set, respectively. Calcification rates (µmol CaCO3 per week per specimen) were calculated using the alkalinity anomaly method (Smith and Key, 1975). In this method, the calcification rate is determined from the change in total alkalinity of the seawater caused by the precipitation of CaCO3. These are determined by comparison to a control sample containing no foraminifera. Accuracy was assessed by analyses of the Scripps Institute of Oceanography reference seawater (Batch 180) and an internal standard. The calcification rate involves high energetic consumption and as such is drastically influenced by stress levels of a calcifying organism and was especially shown to be related to thermal stress in benthic foraminifera (Evans et al., 2015; Schmidt et al., 2016b; Titelboim et al., 2019). Net photosynthesis (ΔO2 µg L−1 per specimen) was measured as net oxygen production compared with a control sample containing no foraminifera. Dissolved oxygen was measured using a Eutech DO 450 connected to a rugged dissolved oxygen (RDO) sensor. Accuracy was assessed by calibration of the sensor against Winkler titration.
2.3 Statistical analysis
To examine whether differences in calcification rates and net photosynthesis are significant between temperature treatments and between weeks, statistical analyses were performed using STATISTICA10 software. For each set of data, we tested assumptions of normality of the residuals and homogeneity of variances, and a statistical test was chosen accordingly. If both assumptions were valid, ANOVA was performed, and in cases where normality was valid and homogeneity was violated, Welch's ANOVA test was applied. In cases where normality was violated, the non-parametric Kruskal–Wallis test was applied. Each was followed by the proper post hoc test. All statistical analyses are summarized in Table 1.
Our experimental design takes biological variability in calcification rates and net photosynthesis between different species into consideration. This notion is based on previous observations that different species, even from the same genus, and different populations of the same species display different calcification rates under the same conditions (i.e. baseline; Titelboim et al., 2019). Specifically, among our experiments the activity baselines of both calcification and net photosynthesis are significantly different between A. lobifera and S. orbiculus (one-way ANOVA: p value <0.001; Fig. 2; Tables S1 and S2 in the Supplement). Hence, the thermal tolerance of the two holobionts was separately evaluated for each experiment.
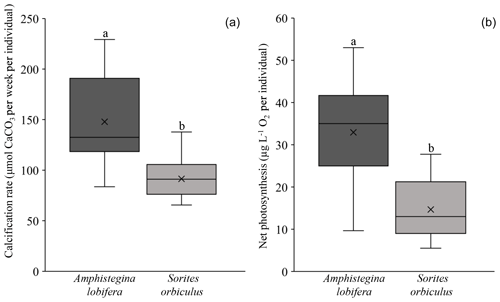
Figure 2Activity baseline of the foraminiferal calcification rate (a) and symbionts' net photosynthesis (b) of A. lobifera (n=14, 15) and S. orbiculus (n=15, 15). Note the significant differences in baseline values of both calcification rates (p<0.001) and photosynthetic activity (p<0.001) between the two holobionts. Error bars represent minimum and maximum values.
3.1 Sorites orbiculus (porcellaneous-dinoflagellate holobiont system)
Calcification rates of S. orbiculus under the different temperature treatments exhibited the highest values at 25, 30, and 32 ∘C. A small decrease was observed at 35 ∘C, but this was not significant during the first week (Fig. 3; Tables S3.1 and S3.2). Net photosynthesis shows positive values under 25, 30, and 32 ∘C. At 35 ∘C, net photosynthesis was negative (Fig. 3; for statistical analyses, see Tables S4.1 and 4.2). Unaccountably, in week 3, one sample exhibited an abnormally high value (i.e. extreme in Fig. 3) with respect to previous weeks as well as to other replicates and thus was neither included in the average and error calculations nor in the statistical analysis.
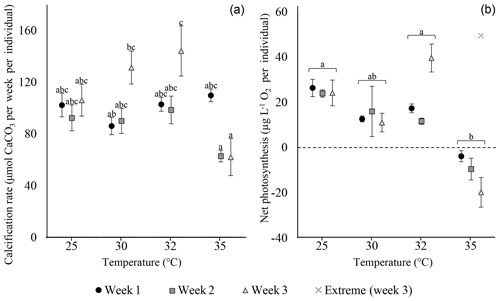
Figure 3Calcification rates (a) and net photosynthesis (b) of S. orbiculus. Letters represent the results of the post hoc tests (Tables S3.2 and S4.2). Error bars are SE. A single abnormal measurement, obtained at week 3, is marked as extreme and is not calculated as part of the average, error, and statistical analysis.
3.2 Amphistegina lobifera (hyaline diatom holobiont system)
Both calcification and net photosynthesis are synchronous throughout the experiment. After the first and second weeks, calcification rates and net photosynthesis exhibited the highest values under 25, 30, and 32 ∘C. At 35 ∘C calcification and net photosynthesis were both severely reduced and net photosynthesis was negative (Fig. 4; Tables S5 and S6). Between the second and third weeks, many specimens exhibit massive bleaching that occurred in different treatments between 25 and 32 ∘C in similar proportions (Tukey HSD post hoc test: p values > 0.1; Table S7.2) and thus was clearly not related to the different temperature. Bleaching in the 35 ∘C treatment did not exceed two specimens per replicate (Table S11). For this reason, measurements of the third week are excluded from the results.
Our study separately describes the thermal sensitivity of the foraminifera and the algal symbionts in two types of holobiont systems: A. lobifera-hosting diatoms, mostly from the order Fragilariales (Barnes, 2016; Prazeres et al., 2017; Schmidt et al., 2016b, 2018), and S. orbiculus-hosting dinoflagellates, Symbiodiniaceae (Merkado et al., 2013; Pawlowski et al., 2001; Pochon et al., 2007). Both species are considered to be prominent calcifiers based on their massive occurrences and widespread distribution (Langer and Hottinger, 2000), and both record a graduate decline in physiological performance between 32 and 35 ∘C (Figs. 3–4). Both holobionts show thermal resilience up to 32 ∘C and a negative response at 35 ∘C (Figs. 3 and 4). However, they differ with respect to the magnitude of their responses: A. lobifera and its diatom symbionts share similar thermal sensitivity, with near inhibition of calcification and negative net photosynthesis at 35 ∘C, whereas in S. orbiculus calcification is less dramatically reduced at 35 ∘C, indicating that it is more resilient to extreme SST than A. lobifera. Moreover, the Symbiodiniaceae symbionts exhibit stress earlier (already after the first week) than calcification, which was not reduced at the first week and only slightly reduced after. The different thermal sensitivity exhibited by calcification rate and by symbionts of S. orbiculus implies that they might be a limiting factor for the host to cope with future warming. A similar apparent discordance was previously observed in Amphistegina (Prazeres et al., 2017; Stuhr et al., 2017; Schmidt et al., 2016; Hallock et al., 2006b). Hallock et al. (2006b) suggested that the ectoplasm of bleached specimens is “preprogrammed” to continue calcification. Possible explanations for the synchronized response of the A. lobifera holobiont in this study are either that (1) the symbiont and host have similar thermal sensitivity or (2) the weekly resolution of measurements may not capture a short discordance time between the responses of the symbiont and host.
It was previously shown that coral's ability to cope with elevated temperatures is related to its partnering with functionally diverse symbionts (Baker et al., 2004; Howells et al., 2012; Jones et al., 2008; Poquita-Du et al., 2020; Rowan, 2004), although its symbiosis is limited to dinoflagellate from the Symbiodiniaceae “clades” (LaJeunesse et al., 2018; Silverstein et al., 2015). LBF are known to host different kinds of symbionts (Pochon et al., 2007), which include dinoflagellates, diatoms, unicellular chlorophytes, unicellular rhodophytes, and/or cyanobacteria (reviewed in Lee, 2006). Whereas the general types of the symbiont (algal genus) seem to be phylogenetically fixed, there appears to be considerable flexibility in symbiont infestation even within one individual (Lee, 2006). This versatile symbiont partnership may control the holobionts' thermal tolerance and provide one of the key factors in their response to future warming. For example, a mechanism to cope with thermal stress was suggested in Pararotalia calcariformata, an extremely heat-tolerant symbiont-bearing foraminifera, that host a diverse symbiont community of diatoms. In case of thermal stress, functionally relevant members of the symbiont community can become more dominant and magnify the ability of the holobiont to tolerate elevated temperatures (Schmidt et al., 2018). This might also explain the observation that species-specific differences in the thermal tolerance of Amphistegina species are correlated to different symbiont assemblages. Specifically, larger diversity of algal symbionts was associated with the more tolerant species (Stuhr et al., 2018).
Considering the role of LBF in the carbon cycle and as ecosystem engineers, their future with expected warming is a major concern. Previous studies modelled the predicted changes in the distribution of LBF and their contribution to carbonate production (e.g. Langer, 2008; Langer et al., 2013; Weinmann et al., 2013; Weinmann and Langer, 2017). However, our results highlight the need for species-specific considerations for more accurate predictions on the fate of LBF.
Our study shows that LBF have different thermal tolerances that are limited by the sensitivity of their eukaryotic algal symbionts. Considering recent findings on the significant role of the prokaryotic microbiome in the physiological performance of LBF (Prazeres, 2018; Prazeres et al., 2017), it will be highly valuable also to explore, in future studies, their specific contribution to the thermal tolerance of the holobiont.
All data related to the paper are available in the Supplement.
The supplement related to this article is available online at: https://doi.org/10.5194/bg-17-2341-2020-supplement.
The study was designed by DT and DP. Sampling and culturing experiments were carried out by DP and DT using facilities provided by SA. Interpretation of data and writing of the paper were done by all authors: DP, DT, and SA.
The authors declare that they have no conflict of interest.
We thank Yahel Eshed and Neta Gershon for their assistance in the fieldwork.
This research has been supported by the Israel Science Foundation (grant no. 941/17) and the BMBF–MOST (grant nos. 3-15275 and 3-15274).
This paper was edited by Jack Middelburg and reviewed by two anonymous referees.
Baker, A. C., Starger, C. J., McClanahan, T. R., and Glynn, P. W.: Corals' adaptive response to climate change, Nature, 430, 741–741, https://doi.org/10.1038/430741a, 2004.
Barnes, K. H.: Diversity and Distribution of Diatom Endosymbionts in Amphistegina spp. (Foraminifera) Based on Molecular and Morphological Techniques, Grad. Theses Diss., available at: https://scholarcommons.usf.edu/etd/6177 (last access: 24 December 2019), 2016.
Evans, D., Erez, J., Oron, S., and Müller, W.: Mg/Ca-temperature and seawater-test chemistry relationships in the shallow-dwelling large benthic foraminifera Operculina ammonoides, Geochim. Cosmochim. Ac., 148, 325–342, https://doi.org/10.1016/j.gca.2014.09.039, 2015.
Hallock, P., Williams, D., Fisher, E., and Toler, S.: Bleaching in foraminifera with algal symbionts: implications for reef monitoring and risk asessment, Anuário do Inst. Geociências, 29, 108–128, 2006a.
Hallock, P., Williams, D. E., Toler, S. K., Fisher, E. M., and Talge, H. K.: Bleaching in reef-dwelling foraminifers: implications for reef decline, Proceedings of 10th International Coral Reef Symposium, 28 June–2 July 2004, Okinawa, Japan, 729–737, 2006b.
Howells, E. J., Beltran, V. H., Larsen, N. W., Bay, L. K., Willis, B. L., and van Oppen, M. J. H.: Coral thermal tolerance shaped by local adaptation of photosymbionts, Nat. Clim. Change, 2, 116–120, https://doi.org/10.1038/nclimate1330, 2012.
Hyams-Kaphzan, O., Grossowicz, L. P., and Almogi-Labin, A.: Characteristics of benthic foraminifera inhabiting rocky reefs in northern Israeli Mediterranean shelf, Geological Survey of Israel Report GSI/36/2014, No. ES-20-14, Geological Survey of Israel, Jerusalem, 2014.
IPCC: Climate Change 2013 – The Physical Science Basis, Intergovernmental Panel on Climate Change, Cambridge University Press, Cambridge, 2014.
Jones, A. M., Berkelmans, R., Van Oppen, M. J. H., Mieog, J. C., and Sinclair, W.: A community change in the algal endosymbionts of a scleractinian coral following a natural bleaching event: Field evidence of acclimatization, P. Roy. Soc. B-Biol. Sci., 275, 1359–1365, https://doi.org/10.1098/rspb.2008.0069, 2008.
Kawahata, H., Fujita, K., Iguchi, A., Inoue, M., Iwasaki, S., Kuroyanagi, A., Maeda, A., Manaka, T., Moriya, K., Takagi, H., Toyofuku, T., Yoshimura, T., and Suzuki, A.: Perspective on the response of marine calcifiers to global warming and ocean acidification – Behavior of corals and foraminifera in a high CO2 world “hot house”, Prog. Earth Planet. Sci., 6, 5, https://doi.org/10.1186/s40645-018-0239-9, 2019.
LaJeunesse, T. C., Parkinson, J. E., Gabrielson, P. W., Jeong, H. J., Reimer, J. D., Voolstra, C. R., and Santos, S. R.: Systematic Revision of Symbiodiniaceae Highlights the Antiquity and Diversity of Coral Endosymbionts, Curr. Biol., 28, 2570–2580, https://doi.org/10.1016/j.cub.2018.07.008, 2018.
Langer, M. R.: Assessing the Contribution of Foraminiferan Protists to Global Ocean Carbonate Production, J. Eukaryot. Microbiol., 55, 163–169, https://doi.org/10.1111/j.1550-7408.2008.00321.x, 2008.
Langer, M. R. and Hottinger, L.: Biogeography of selected “larger” foraminifera, Micropaleontology, 46, 105–126, 2000.
Langer, M. R., Silk, M. T., and Lipps, J. H.: Global ocean carbonate and carbon dioxide production; the role of reef Foraminifera, J. Foraminifer. Res., 27, 271–277, https://doi.org/10.2113/gsjfr.27.4.271, 1997.
Langer, M. R., Weinmann, A. E., Lötters, S., and Rödder, D.: “Strangers” in paradise: Modeling the biogeographic range expansion of the foraminifera amphistegina in the mediterranean sea, J. Foramin. Res., 42, 234–244, https://doi.org/10.2113/gsjfr.42.3.234, 2012.
Langer, M. R., Weinmann, A. E., Lötters, S., Bernhard, J. M., and Rödder, D.: Climate-Driven Range Extension of Amphistegina (Protista, Foraminiferida): Models of Current and Predicted Future Ranges, PLoS One, 8, e5444, https://doi.org/10.1371/journal.pone.0054443, 2013.
Larsen, A. R.: Studies of Recent Amphistegina, Taxonomy and some Ecological Aspects, Israel J. Earth Sci., 25, 1–26, 1976.
Lee, J. J.: Algal symbiosis in larger foraminifera, Symbiosis, 42, 63–75, 2006.
Lejeusne, C., Chevaldonné, P., Pergent-Martini, C., Boudouresque, C. F., and Pérez, T.: Climate change effects on a miniature ocean: the highly diverse, highly impacted Mediterranean Sea, Trends Ecol. Evol., 25, 250–260, https://doi.org/10.1016/J.TREE.2009.10.009, 2010.
Macias, D., Garcia-Gorriz, E., and Stips, A.: Understanding the Causes of Recent Warming of Mediterranean Waters. How Much Could Be Attributed to Climate Change?, PLoS One, 8, e81591, https://doi.org/10.1371/journal.pone.0081591, 2013.
Merkado, G., Holzmann, M., Apothéloz-Perret-Gentil, L., Pawlowski, J., Abdu, U., Almogi-Labin, A., Hyams-Kaphzan, O., Bakhrat, A., and Abramovich, S.: Molecular evidence for lessepsian invasion of soritids (larger symbiont bearing benthic foraminifera), PLoS One, 8, e77725, https://doi.org/10.1371/journal.pone.0077725, 2013.
Momigliano, P. and Uthicke, S.: Symbiosis in a giant protist (Marginopora verte bralis, Soritinae): Flexibility in symbiotic partner ships along a natural temperature gradient, Mar. Ecol.-Prog. Ser., 491, 33–46, https://doi.org/10.3354/meps10465, 2013.
Pawlowski, J., Holzmann, M., Fahrni, J. F., Pochon, X., and Lee, J. J.: Molecular Identification of Algal Endosymbionts in Large Miliolid Foraminifera: 2. Dinoflagellates, J. Eukaryot. Microbiol., 48, 368–373, https://doi.org/10.1111/j.1550-7408.2001.tb00326.x, 2001.
Pochon, X., Garcia-Cuetos, L., Baker, A. C., Castella, E., and Pawlowski, J.: One-year survey of a single Micronesian reef reveals extraordinarily rich diversity of Symbiodinium types in soritid foraminifera, Coral Reefs, 26, 867–882, https://doi.org/10.1007/s00338-007-0279-x, 2007.
Pochon, X., Putnam, H. M., and Gates, R. D.: Multi-gene analysis of Symbiodinium dinoflagellates: A perspective on rarity, symbiosis, and evolution, PeerJ, 2014, e394, https://doi.org/10.7717/peerj.394, 2014.
Poquita-Du, R. C., Huang, D., Chou, L. M., and Todd, P. A.: The contribution of stress-tolerant endosymbiotic dinoflagellate Durusdinium to Pocillopora acuta survival in a highly urbanized reef system, Coral Reefs, https://doi.org/10.1007/s00338-020-01902-0, online first, 2020.
Por, F. D.: Lessepsian migration – the influx of Red Sea biota into the Mediterranean by way of the Suez Canal, Springer-Verlag, Berlin, 1978.
Por, F. D.: Climate Optimum rejuvenates the Mediterranean marine world, Integr. Zool., 5, 112–121, https://doi.org/10.1111/j.1749-4877.2010.00194.x, 2010.
Prazeres, M.: Bleaching-associated changes in the microbiome of large benthic foraminifera of the Great Barrier Reef, Australia, Front. Microbiol., 9, 1–13, https://doi.org/10.3389/fmicb.2018.02404, 2018.
Prazeres, M. and Renema, W.: Evolutionary significance of the microbial assemblages of large benthic Foraminifera, Biol. Rev., 94, 828–848, https://doi.org/10.1111/brv.12482, 2019.
Prazeres, M., Ainsworth, T., Roberts, T. E., Pandolfi, J. M., and Leggat, W.: Symbiosis and microbiome flexibility in calcifying benthic foraminifera of the great Barrier Reef, Microbiome, 5, 1–11, https://doi.org/10.1186/S40168-017-0257-7, 2017.
Rowan, R.: Thermal adaptation in reef coral symbionts, Nature, 430, 742, https://doi.org/10.1038/430742a, 2004.
Schmidt, C., Heinz, P., Kucera, M., and Uthicke, S.: Temperature-induced stress leads to bleaching in larger benthic foraminifera hosting endosymbiotic diatoms, Limnol. Oceanogr., 56, 1587–1602, https://doi.org/10.4319/lo.2011.56.5.1587, 2011.
Schmidt, C., Morard, R., Almogi-Labin, A., Weinmann, A. E., Titelboim, D., Abramovich, S., and Kucera, M.: Recent Invasion of the Symbiont-Bearing Foraminifera Pararotalia into the Eastern Mediterranean Facilitated by the Ongoing Warming Trend, PLoS One, 10, e0132917, https://doi.org/10.1371/journal.pone.0132917, 2015.
Schmidt, C., Titelboim, D., Brandt, J., Herut, B., Abramovich, S., Almogi-Labin, A., and Kucera, M.: Extremely heat tolerant photo-symbiosis in a shallow marine benthic foraminifera, Sci. Rep.-UK, 6, 1–9, https://doi.org/10.1038/srep30930, 2016a.
Schmidt, C., Morard, R., Prazeres, M., Barak, H., and Kucera, M.: Retention of high thermal tolerance in the invasive foraminifera Amphistegina lobifera from the Eastern Mediterranean and the Gulf of Aqaba, Mar. Biol., 163, 228, https://doi.org/10.1007/s00227-016-2998-4, 2016b.
Schmidt, C., Morard, R., Romero, O., and Kucera, M.: Diverse Internal Symbiont Community in the Endosymbiotic Foraminifera Pararotalia calcariformata: Implications for Symbiont Shuffling Under Thermal Stress, Front. Microbiol., 9, 2018, https://doi.org/10.3389/fmicb.2018.02018, 2018.
Silverstein, R. N., Cunning, R., and Baker, A. C.: Change in algal symbiont communities after bleaching, not prior heat exposure, increases heat tolerance of reef corals, Glob. Change Biol., 21, 236–249, https://doi.org/10.1111/gcb.12706, 2015.
Smith, S. V. and Key, G. S.: Carbon dioxide and metabolism in marine environments1, Limnol. Oceanogr., 20, 493–495, https://doi.org/10.4319/lo.1975.20.3.0493, 1975.
Stuhr, M., Reymond, C. E., Rieder, V., Hallock, P., Rahnenführer, J., Westphal, H., and Kucera, M.: Reef calcifiers are adapted to episodic heat stress but vulnerable to sustained warming, PloS one, 12, e0179753, https://doi.org/10.1371/journal.pone.0179753, 2017.
Stuhr, M., Meyer, A., Reymond, C. E., Narayan, G. R., Rieder, V., Rahnenführer, J., Kucera, M., Westphal, H., Muhando, C. A., and Hallock, P.: Variable thermal stress tolerance of the reef-associated symbiont-bearing foraminifera Amphistegina linked to differences in symbiont type, Coral Reefs, 37, 811–824, https://doi.org/10.1007/s00338-018-1707-9, 2018.
Titelboim, D., Almogi-Labin, A., Herut, B., Kucera, M., Schmidt, C., Hyams-Kaphzan, O., Ovadia, O., and Abramovich, S.: Selective responses of benthic foraminifera to thermal pollution, Mar. Pollut. Bull., 105, 324–336, https://doi.org/10.1016/j.marpolbul.2016.02.002, 2016.
Titelboim, D., Almogi-Labin, A., Herut, B., Kucera, M., Asckenazi-Polivoda, S., and Abramovich, S.: Thermal tolerance and range expansion of invasive foraminifera under climate changes, Sci. Rep.-UK, 9, 1–5, https://doi.org/10.1038/s41598-019-40944-5, 2019.
Weinmann, A. E. and Langer, M. R.: Diverse thermotolerant assemblages of benthic foraminiferal biotas from tropical tide and rock pools of eastern Africa, Rev. Micropaleontol., 60, 511–523, https://doi.org/10.1016/j.revmic.2017.09.002, 2017.
Weinmann, A. E., Rödder, D., Lötters, S., and Langer, M. R.: Heading for New Shores: Projecting Marine Distribution Ranges of Selected Larger Foraminifera, PLoS One, 8, e62182, https://doi.org/10.1371/journal.pone.0062182, 2013.
Zenetos, A., Gofas, S., Verlaque, M., Çinar, M. E., García Raso, J. G., Bianchi, C. N., Morri, C., Azzurro, E., Bilecenoglu, M., Froglia, C., Siokou, I., Violanti, D., Sfriso, A., San Martín, G., Giangrande, A., Katagan, T., Ballesteros, E., Ramos-Esplá, A., Mastrototaro, F., Ocaña, O., Zingone, A., Gambi, M. C., and Streftaris, N.: Alien species in the Mediterranean Sea by 2010. A contribution to the application of European Union's Marine Strategy Framework Directive (MSFD). Part I. Spatial distribution, Mediterr. Mar. Sci., 11, 381–493, 2010.
Zenetos, A., Gofas, S., Morri, C., Rosso, A., Violanti, D., Garcia Raso, J. E., Cinar, M. E., Almogi-Labin, A., Ates, A. S., Azzurro, E., Ballesteros, E., Bianchi, C. N., Bilecenoglu, M., Gambi, M. C., Giangrande, A., Gravili, C., Hyams-Kaphzan, O., Karachle, P. K., Katsanevakis, S., Lipej, L., Mastrototaro, F., Mineur, F., Pancucci-Papadopoulou, M. A., Ramos Espla, A., Salas, C., San Martin, G., Sfriso, A., Streftaris, N., and Verlaque, M.: Alien species in the Mediterranean Sea by 2012. A contribution to the application of European Union's Marine Strategy Framework Directive (MSFD). Part 2. Introduction trends and pathways, Mediterr. Mar. Sci., 13, 328–352, https://doi.org/10.12681/mms.327, 2012.
Ziegler, M. and Uthicke, S.: Photosynthetic plasticity of endosymbionts in larger benthic coral reef Foraminifera, J. Exp. Mar. Biol. Ecol., 407, 70–80, https://doi.org/10.1016/j.jembe.2011.07.009, 2011.