the Creative Commons Attribution 4.0 License.
the Creative Commons Attribution 4.0 License.
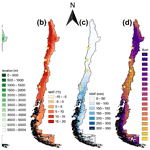
Reviews and syntheses: Composition and characteristics of burrowing animals along a climate and ecological gradient, Chile
Kirstin Übernickel
Jaime Pizarro-Araya
Susila Bhagavathula
Leandro Paulino
Todd A. Ehlers
Although the burrowing activity of some species (e.g., gophers) is well studied, a comprehensive inventory of burrowing animals in adjacent biomes is not yet known, despite the potential importance of burrowing activity on the physical and chemical evolution of Earth's surface. In this study, we review the available information with a focus on the following: (a) an inventory of burrowing vertebrates and invertebrates along the climate and ecological gradient in Chile; (b) the dimensions and characteristics of burrows; and (c) calculation of excavation rates by local species compositions. Methods used include a literature compilation (> 1000 studies) of Chilean burrowing animal species integrated with global, species-specific excavation rates. A field study augments literature findings with quantification of the zoogeomorphic effects on hillslope mass transport at the animal community level and along the arid to humid–temperate climate gradient within the Chilean Coastal Cordillera (27–38∘ S latitude).
The literature review indicates a minimum of 45 vertebrate and 345 invertebrate burrowing species distributed across Chile in different biomes. Burrowing depths for Chilean mammals range between 3 m (e.g., for skunks, Conepatus) and 0.25 m (for rock rats, Aconaemys). For invertebrates, burrowing depths in Chile range between 1 m for scorpions to 0.3 m for spiders. In comparison, globally documented maximum burrow depths reach up to more than 6 m for vertebrates (gopher tortoises and aardvarks) and 4 m for invertebrates (ants).
Minimum excavation rates of local animal communities observed from field sites in Chile are 0.34 m3 ha−1 yr−1 for the arid site, 0.56 m3 ha−1 yr−1 for the semiarid site, 0.93 m3 ha−1 yr−1 for the mediterranean site and 0.09 m3 ha−1 yr−1 for the humid–temperate site, with the latter likely an underestimation. The calculated minimum Chilean excavation rates are within the large range of globally observed single species rates ranging between 0.01 and 56.20 m3 ha−1 yr−1 for vertebrates and from 0.01 to 37.31 m3 ha−1 yr−1 for invertebrates. Taken together, results not only highlight the diverse and latitudinally varying number of burrowing vertebrates and invertebrates present in different biomes, but also foster the understanding of how burrowing activity changes over a gradient and is influenced by mean annual temperature, mean annual precipitation, slope aspect and latitudinal-related incoming solar energy.
- Article
(5837 KB) - Full-text XML
-
Supplement
(434 KB) - BibTeX
- EndNote
Abiotic processes such as overland flow, creep, rain splash, wind and mass wasting, as well as biotic processes induced by animals or plants, influence the erosion of hillslopes (Amelung et al., 2018; Anderson and Anderson, 2010; Gabet et al., 2003; Smith and Gardner, 1985; Starke et al., 2020; Viles, 2020). Geomorphic processes that result from burrowing animals are called zoogeomorphologic (Butler, 1995; Corenblit et al., 2011) and are a form of bioturbation (Hole, 1981; Wilske et al., 2015). Some of the earliest work documenting zoogeomorphologic processes (e.g., downhill sediment transport by animals) was Darwin's (1881) detailed description of earthworm activity. Burrowing animals in the pedosphere include earthworms; a wide range of insects (Bétard, 2020) including larval stages to adult forms of beetles, bees and ants; spiders and scorpions; crustaceans; and myriapods; and also burrowing vertebrates such as lizards (e.g., Donoso-Barros, 1960) and larger mammals, like rodents, wolves, badgers (Thorp, 1949) and birds (e.g., Figueroa and Stucchi, 2008; Masello et al., 2006; Zavalaga and Alfaro-Shigueto, 2015).
However, despite the potential importance of bioturbation as a geomorphic process, inventories of burrowing vertebrates and invertebrates are limited and hamper our understanding of where and when they might impact hillslope processes. Here we compile an inventory of burrowing species, and we investigate the excavation rates of the animal communities and discuss correlations of their activity with controlling environmental factors. Current knowledge of burrowing activity is limited to very few species within specific biomes. Given this, uncertainty surrounds the importance of animal burrowing for geomorphologic studies. Zoogeomorphologic studies are not only infrequent, but also heterogenous in the methods applied (e.g., Butler et al., 2013; Dietrich and Perron, 2006; Gabet et al., 2003; Platt et al., 2016; Wilkinson et al., 2009). As a result, it is difficult to determine surface downhill sediment transport rates at the ecosystem scale and on timescales relevant to surface processes studies (Dietrich and Perron, 2006; Platt et al., 2016). Furthermore, it remains impossible to directly quantify the amount of sediment moved by all animals. The information for this type of analysis is not available with existing techniques. To determine these rates, an approach is needed to estimate the sum of all animal activity at a locality, and to understand the variation of the burrowing activity depending on the biome characteristics.
Previous studies of animal burrowing have focused on either a single species or, less frequently, on a few dominant species, with the vast majority of other animals remaining underrepresented. The most studied burrowing vertebrates are North American gophers (e.g., Black and Montgomery, 1991; Dixon et al., 2009; Ellison, 1946; Huntly and Inouye, 1988; Kalisz and Stone, 1984; Seabloom et al., 2000; Smallwood and Morrison, 1999b; Thorn, 1978; Winchell et al., 2016), prairie dogs (e.g., Bangert and Slobodchikoff, 2006; Reading and Matchett, 1997), zokors/mole rats (e.g., Zhang et al., 2003), or voles (e.g., Hall et al., 1999). The most studied invertebrate animals are ants, termites and earthworms (e.g., Bétard, 2020; Lobry de Bruyn and Conacher, 1990; Carlson and Whitford, 1991; Darwin, 1881; Lee and Foster, 1991; Viles et al., 2021; Wilkinson et al., 2009). Burrowing depth and excavation rates for more than one species per location have been documented in very few studies. Exceptions to this include work studying the interaction between species such as ants and small mammals (e.g., Eldridge and Whitford, 2014) or communities with similar effects such as rodents (Price, 1986). James et al. (2011) studied four vertebrate species in Australia and focused on their burrowing differences due to their native or non-native origin. Voslamber and Veen (1985) compared behavior of two mammal species (badgers and rabbits) in Belgium. In summary, downhill erosion and sedimentation by an excavating animal community at hillslopes or larger scales remain poorly understood.
Furthermore, existing studies do not cover the variety of biomes. There is a concentration of excavating animal studies in North American desert vertebrates and rodents (Platt et al., 2016). To our knowledge, only a single study compared the chemical effects of burrowing by comparable mammal species in different climate settings including a semi-desert in the Ural region and a spruce forest in the Moscow region (Abaturov, 1972). Studies conducted in different biomes and geographical areas, such as South America, are underrepresented in existing literature (Haussmann, 2017). Thus, the effects of burrowing animals along gradients in temperature, precipitation, and vegetation are seldom studied.
In this study, we build upon the previous global inventories and focus on burrowing vertebrates and invertebrates along the climate and ecological gradient in Chile. Our approach complements recent global compilation on ants as geomorphological agents in Viles et al. (2021) and a global review about insects as such agents (Bétard, 2020). From existing literature, we compile the burrowing taxa of Chile, their distribution, burrow details such as tunnel size, depth and geographic extent, as well as excavation rates (when available). Globally published excavation rates of animals are summarized for comparison. In addition, we present new observations from four Chilean study areas spanning diverse biogeographic zones and document the number of burrow entrances of present taxa for vertebrates and invertebrates. From measurements of burrow entrances and tunnel lengths, taxa-independent excavation rates are calculated as minimum values. With this method it is possible to sample and estimate species-independent zoogeomorphic effects at the hillslope scale, and along a climate gradient. The literature survey with the resulting species list not only includes older studies (in Spanish and English) that may be difficult to find, but also highlights the massive gap of knowledge we have regarding this topic of species-specific burrowing volume quantification. Our results highlight what type of information is missing for which animals. The field study presented, in the Coastal Cordillera of Chile, complements the lack of knowledge with a first estimate of the effect of the local burrowing species.
2.1 Literature compilation on Chilean burrowing animals
To identify burrowing animal species in Chile and compile burrow-related information, as well as global animal excavation rates, a literature search was conducted online in standard search engines and search functions of scientific journal publishers and university libraries. In addition, online databases named in section ”data availability” were consulted. Complementary searches were done in English and in Spanish. Additionally, available information was accessed at the scientific collections of Laboratorio de Entomología Ecológica de la Universidad de La Serena (LEULS), La Serena, Chile, and the División Aracnología del Museo Argentino de Ciencias Naturales “Bernardino Rivadavia” (MACN-Ar), Buenos Aires, Argentina. The species-specific information compiled included species taxonomy, common name(s), alimentation type, special characteristics, group size, density, excavation characteristics (tunnel diameter, (maximum) burrow depths, details about burrow type), preferred habitat and distribution limit by elevation. For vertebrates, body size and weight were also compiled. The data compilation focused on species that excavate in an active manner. Excluded were species that are restricted to the use of burrows made by other species, as well as ground dwellers that hide under rocks or vegetation and also soil-dwelling animals that move through the soil by pushing material aside (“swimming”) (Gabet et al., 2003), as they do not burrow in the sense of making tunnels or exchanging material between the subsurface and the surface. The full list of excavating species identified for Chile is provided in a companion data publication (Übernickel et al., 2020). Hereafter we use the data in this data publication for our figures and analysis. The subset of data regarding the four study sites were summarized as the burrowing species lists of vertebrates (Table S1 in the Supplement) and invertebrates (Table S2). In addition, published excavation rates of animals worldwide were compiled (Table 1).
For visualization of mammals in Chile, their geographic ranges provided by the International Union for Conservation of Nature web page (International Union for Conservation of Nature (IUCN) Red List of Threatened Species, https://www.iucnredlist.org/, last access: 7 December 2018, n of downloaded species = 40), were merged and clipped in QGIS (Version 2.18.26) to generate an overview map of the geographic extent in all of Chile. These results were compiled at a resolution of 0.5 latitudinal degrees (approx. 55 km) (Fig. 1). The topography, mean annual precipitation (MAP) and mean annual temperature (MAT) were also compiled (Fick and Hijmans, 2017). The distribution of invertebrate burrowing species in Chile is not yet available. To estimate the diversity of sympatric species along Chile, we generalized the mostly single study site information of the species to the extent of the respective Chilean region (Übernickel et al., 2020) and plotted the number of species per region (Fig. 2).
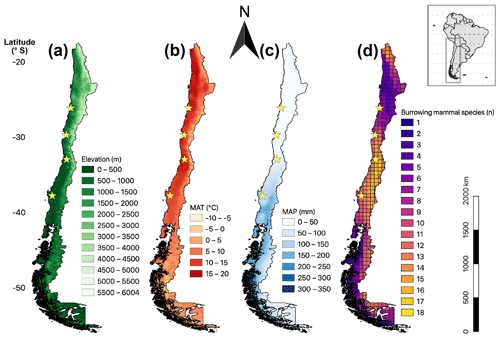
Figure 1Map of mainland Chile showing four parameters: (a) elevation; (b) mean annual temperature (MAT); (c) mean annual precipitation (MAP), MAT, and MAP from Fick and Hijmans (2017); (d) distribution of burrowing mammal species; in map (d) the grid size is 0.5 latitudinal degrees (approx. 55 km × 55 km side lengths). The database for the depicted number of mammal species contains distributional polygons of 40 species (International Union for Conservation of Nature (IUCN) Red List of Threatened Species), downloaded from the web page October 2018; stars: field sites of this study; black edging: border of Chilean mainland and shore (with many small islands in the south).
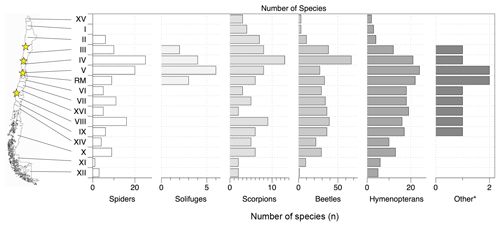
Figure 2Distribution of burrowing invertebrates in Chile per Chilean region. The data compilation is based on the associated data publication to this study (Übernickel et al., 2020). The map source is http://freevectormaps.com, last access: 19 July 2019. Other* refers to isopterans, neuropterans and hemipterans; stars: field sites of this study; black edging: border of Chilean mainland and shore (with many small islands in the south); roman numerals denote Chilean regions from north to south: XV – Región de Arica y Parinacota, I – Región de Tarapacá, II – Región de Antofagasta, III – Región de Atacama, IV – Región de Coquimbo, V – Región de Valparaíso, RM – Región Metropolitana de Santiago, VI – Región del Libertador General Bernardo O'Higgins, VII – Región del Maule, XVI – Región del Ñuble, VIII – Región del Biobío, IX – Región de la Araucanía, XIV – Región de Los Ríos, X – Región de Los Lagos, XI – Región Aysén del General Carlos Ibáñez del Campo, XII – Región de Magallanes y de la Antártica Chilena. Source: Gobierno de Chile, 2017, Nuestro País, https://www.gob.cl/nuestro-pais/, last access: 6 July 2021.
2.2 Field study on animal burrows on hillslopes in Chile
2.2.1 Site description
The study sites are part of the German–Chilean priority program EarthShape (Earth surface shaping by biota; https://www.earthshape.net, last access: 16 March 2021). The sites are located within three national parks (NPs) and one private reserve along the Chilean Coastal Cordillera to minimize anthropogenic disturbances. The latitudinal distance between these areas extends over 1300 km and includes NP Pan de Azúcar (∼ 26∘ S) in the arid north, the semiarid Private Reserve Santa Gracia (∼ 30∘ S), the mediterranean climate NP La Campana (∼ 33∘ S), and the humid–temperate NP Nahuelbuta (∼ 38∘ S) in the south (Fig. 3). For detailed information concerning site descriptions, soil analysis, as well as local vegetation, see Bernhard et al. (2018), Oeser et al. (2018) and Schaller et al. (2018). In the following we summarize the climate, soil classification and topographic characteristics per location (Tables S1 and S2).
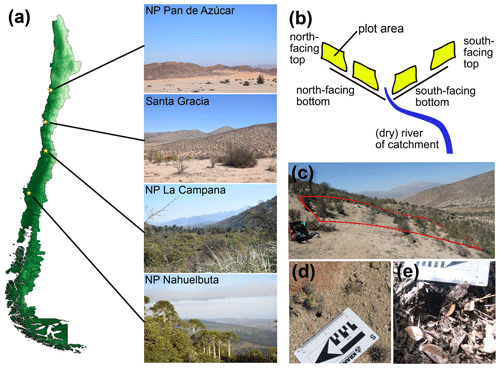
Figure 3Overview of study sites in Chile. (a) Indication of study site locations (yellow stars) and example images; (b) plot setup on opposing slopes with four plots per study site; (c) example image of plot in Santa Gracia; (d–e) examples of measured burrow entrances (d: on bare ground, Santa Gracia; e: within leaf litter, NP La Campana); NP – National Park.
NP Pan de Azúcar is the northernmost arid site with plot sites on hillslopes near 26.1098∘ S, 70.5502∘ W. The NP was established in 1985 (Rundel et al., 1996). It has a MAP of 12 mm and a MAT of 16.8 ∘C (Fick and Hijmans 2017). The elevation is 330 m a.s.l., the vegetation cover is < 10 %, and the slopes range between 25 and 40∘ (Oeser et al., 2018), with the plots located on several slopes. The soil is classified as a Regosol. Bulk density varies between 1.3 Mg m−3 at the north-facing slope and 1.5 Mg m−3 at the south-facing slope, and the texture is sandy loam in both slopes (Bernhard et al., 2018).
The Private Reserve Santa Gracia contains plots on hillslopes near 29.759∘ S, 71.166∘ W. It has a MAP of 66 mm and a MAT of 13.7 ∘C (Fick and Hijmans 2017). The elevation is around 680 m a.s.l., and vegetation cover is 30 %–40 %. The north-facing slope has an angle of 15∘ and the south-facing slope of 25∘ (Oeser et al., 2018). The soil is classified as Cambisol due to a differentiation between surface horizons and subsoil. Bulk densities are homogenous in the first 20 cm depth and 1.5 Mg m−3 for both slopes, but texture predominates as sandy loam in the north-facing slope, while the south-facing slope has a loamy sand texture (Bernhard et al., 2018). Known disturbances of this ecosystem are goats feeding on disturbed (reduced) vegetation (Armesto et al., 2007).
NP La Campana is the mediterranean site and contains plots at the sector Ocoa, around 32.9562∘ S, 71.0637∘ W. The NP was established in 1967 with the boundaries legalized in 1985 (Macdonald et al., 1988). It has a MAP of 367 mm and a MAT of 14.1 ∘C (Fick and Hijmans 2017). The elevation is around 730 m a.s.l.; with a vegetation cover of close to 100 %, the north-facing slope has an angle of 12∘ and the south-facing slope of 23∘ (Oeser et al. 2018). The soil is classified as Cambisol. Bulk densities vary between 1.5 Mg m−3 at the north-facing slope and 1.1 Mg m−3 at the south-facing slope, and the general soil texture is sandy loam for the first 20 cm of depth (Bernhard et al., 2018). Known disturbances of the ecosystem are (illegally) grazing cows (Rundel and Weisser, 1975).
NP Nahuelbuta is the southernmost site, with plots located near 37.8087∘ S, 73.0137∘ W. This NP was established in 1936 (Servicio Agrícola y Ganadero, 1970). It has a MAP of 1,469 mm and a MAT of 6.6 ∘C (Fick and Hijmans 2017). The elevation is around 1240 m a.s.l., and vegetation cover is ∼ 100 %. The north-facing slope has an angle of 13∘ and the south-facing slope of 15∘ (Oeser et al. 2018). The soil is classified as an Umbrisol. The bulk density is 0.9 Mg m−3 at the north-facing slope and 0.8 Mg m−3 at the south-facing slope, and the texture is sandy-clay loam (Bernhard et al., 2018). The ecosystem is disturbed by (illegally) grazing cows.
2.2.2 Data compilation
Data acquisition took place during three field campaigns in November 2016, May 2017 and March 2018. Each site was visited during every field campaign. The plots per site were selected with the criteria to be located in east-to-west oriented valleys, two plots per opposing ∼ north- and ∼ south-facing slopes with one plot near the top and one plot near the bottom of the hillslope (Fig. 3b). The size of the plots was 10 × 10 m in 2016 and 2018; in May 2017 5 m × 5 m sized plots were analyzed and numbers later scaled for comparability.
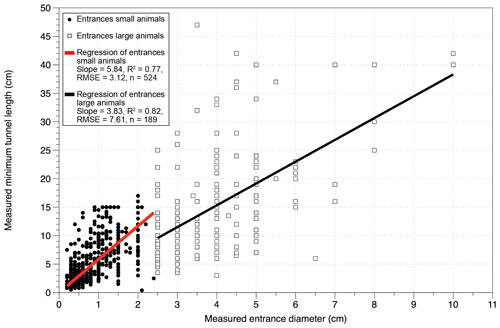
Figure 4Linear regressions of the correlation between measured entrance diameter and measured minimum tunnel lengths for the groups of large animals and small animals. The regressions are forced through 0 and are calculated from the data of 2018. Entrances of large animals are defined as entrances ≥ 2.5 cm diameter; entrances of small animals are of diameters < 2.5 cm.
The burrow measurement procedure was to walk uphill within the plot in approximately 50 cm separated tracks, measuring the detectable burrow entrances (diameters ≥ 0.1 cm) along the path and marking them to avoid duplicate measurements. The procedure and the parameters taken were optimized during the three field campaigns. First, the diameter of burrow entrances was digitally measured from photographs using ImageJ (v1.51m9). When the entrance was oval the major and minor axes were measured. In 2017 and 2018, the time effort was reduced by counting burrow entrances and taking measurements on site. From 2017 onwards minimum tunnel length was determined as well, measuring manually from the entrance to the reachable maximum end of the tunnel. Furthermore, the direction of the tunnel into the ground was labeled as either vertical, horizontal or 45∘. In 2018 the ground cover of the plots was estimated (Table S3). Note that for NP Pan de Azúcar the two plots per hillslope scheme was not completely fulfilled, as slopes at this site were too short. Plots were selected as similar to the original setup as possible.
Minimum burrowed depths (Fig. 6) were approximated from the 2018 data, calculating the depths using the law of sines and the measured minimum tunnel length and the approximate angle of the tunnel (vertical, horizontal or 45∘). The minimum excavation rate in m3 ha−1 yr−1 (Fig. 7) was estimated based on tunnel parameters measured in March 2018. March is late summer in Chile and represents the closest estimate of burrowing activity within a year before autumn and winter rainfall reset the surface. For each entrance the minimum excavated volume was calculated, dependent on the measured minimum tunnel length and the entrance's size and approximate shape. For round and oval entrances, the volume was calculated using the entrance radius, or major and minor axis, respectively. Crevice-shaped entrances were treated as ovals for conservative volume approximation. The volumes were converted from values per plot to m3 ha−1 yr−1. Note that for tunnel volumes and subsequently the excavation rate we can only report the minimum values, because additional volume behind a first curve or obstacle in the tunnel could not be measured.
2.2.3 Data analysis
From the collected data we correlated entrance diameter and minimum tunnel lengths (Fig. 4), measured the distribution of burrow entrances regarding the plot position on the top or bottom of the slope, distribution of entrances relative to the slope aspect (north- or south-facing), and distribution of entrances along the climate gradient (Fig. 5), and calculated minimum burrowing depth (Fig. 6) and minimum excavation rates (Fig. 7). Note that the approach to evaluate the number of burrow entrances is a proxy to burrowing activity, as the actual density of the individuals remains unknown. Also, by counting the burrow entrances, the number of burrows is not exactly registered, as one burrow may have several entrances. Statistical analysis on the burrow entrance data was not applicable as no replicates were collected. Finally, ground cover impeded the collection of sufficient data at the southernmost site (NP Nahuelbuta).
In addition, the solar energy input and its effects may influence the distribution of the burrow entrances as they vary with the slope aspect (Gallardo-Cruz et al., 2009). To detect a link of solar energy input and the quantity of burrow entrances on north- and south-facing slopes, the diurnal course of potential direct radiation was calculated (Fig. 8). We assumed cloud-free conditions and the potential direct radiation on an inclined surface using the terrain angle, based on the coordinates of the study sites following Bendix (2004). The calculations were conducted for 21 June and 21 December, the period of lowest and highest solar radiation, respectively. For simplicity, atmospheric transmission is fixed to 0.8 and potentially occurring shadows of neighboring topography and/or vegetation were neglected. Plotted values are for midday.
3.1 Literature compilation on Chilean burrowing animals
The literature survey included > 1000 references and revealed 45 vertebrate and 345 invertebrate species in Chile with excavating activity. The full species list is provided in the associated data publication (Übernickel et al., 2020). Of the 45 burrowing vertebrate species identified for Chile, 40 are mammals, 2 are birds and 3 are reptiles. Most burrowing species are rodents, i.e., mice, (mole) rats, rabbits, cavies (guinea pigs), chinchillas and nutrias. Other excavating mammals present include foxes, skunks, armadillos and some insectivores. Regarding burrowing Chilean invertebrates, information beyond the name and taxonomic description from museum specimens was scarce, and many species remain unobserved in their habitat. Also, excavating activity in the literature was frequently only indirect. For example, only adaptations of body parts for burrowing activity were described. The 345 burrowing invertebrate species are composed as follows: within the basal group of diverse spiders, there are 83 species with 44 trap door spiders (3 Actinopodidae, 6 Migidae, 14 Nemesiidae, 21 Pycnothelidae), 8 funnel web spiders (Hexathelidae), 4 goblin spiders (Oonopidae), 4 sand recluse spiders (Sicariidae), 13 tarantulas (Theraphosidae) and 10 ant spiders (Zodariidae); the closest relatives to the spiders are sun spiders (Solifugae), with 10 burrowing species within the families Mummuciidae, Daesiidae and Ammotrechidae. Related to these basal taxa are scorpions, with 50 burrowing species (most belonging to the genera Bothriurus sp. and Brachistosternus spp.). Higher taxonomic groups with burrowing species are beetles, with 157 species including 63 darkling beetles (Tenebrionidae), 13 ground beetles, (Carabidae), 11 trogids (Trogidae), 29 dung beetles and 41 scarab beetles (both of Scarabaeidae); 13 bees (Colletidae); 5 wasps (Crabronidae and Sphecidae); 23 ants (Formicidae); 1 ant lion (Myrmeleontidae); 1 termite (Rhinotermitidae) and 1 cicada (Cicadidae) species (Übernickel et al., 2020). Bétard (2020) mentions additional orders and families of insects that burrow on a global scale. However, of these (Blattodea, Dermaptera, Diptera, Embioptera, Ephemeroptera, Lepidoptera, Mecoptera, Megaloptera, Odonata, Orthoptera, Plecoptera, Trichoptera), we did not find supporting literature for equivalent Chilean species. The following sections provide details concerning the findings summarized separately for vertebrates and invertebrates in Chile, subdivided into the geographic distribution in Chile, composition and spatial occurrence at study sites, and their burrow characteristics.
3.1.1 Burrowing vertebrates
The distribution of Chilean burrowing mammal species varies with latitude and altitude along Chile (Fig. 1d), with most species having restricted distributions in distinct parts of the country. A maximum of 18 sympatric species were identified around 35∘ S. Most diversity is present at the mediterranean zone (central Chile) with commonly 11 to 16 species per grid cell. Trends in the species distribution are gradual with latitude, and fewer species are present at higher elevations, i.e., the Andes (Fig. 1a). Similarly, species number decreases with decreasing mean annual temperature, i.e., Patagonia in the south (MAT, Fig. 1b). Finally, species numbers decrease with decreasing mean annual precipitation, i.e., northern arid lowlands (MAP, Fig. 1c).
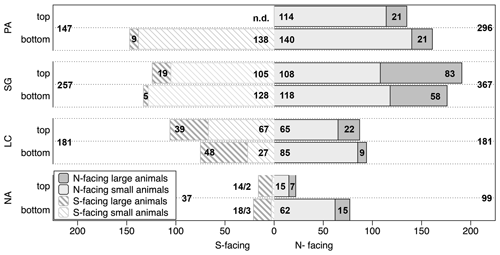
Figure 5Overview of number of measured burrow entrances per plot specification across study sites. The data are presented per top/bottom position on slopes as well as N(orth)- or S(outh)-facing aspect. The numbers within the bars indicate the number of entrances found at the respective slope position per animal group, the numbers outside of the bars are total values for the respective slope; the depicted data are pooled from all three field campaigns: 2016, 2017 and 2018. PA – NP Pan de Azúcar, SG – Santa Gracia, LC – NP La Campana, NA – NP Nahuelbuta, n.d. – not determined. (Note that the spatial distribution of the plots in NP Pan de Azúcar was not as uniform as at the other sites, and the numbers contain three plots north-facing (one top, two bottom) and one plot south-facing (bottom).)
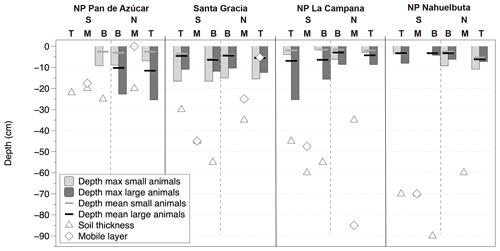
Figure 6Animal burrowing depth as mean and maximum values from measurements in an arid to humid–temperate climatic gradient; for comparison the following are included: the depth of mobile layer following Schaller et al. (2018) and soil thickness following Oeser et al. (2018). NP – National Park, N – north-facing slope, S – south-facing slope. T, M and B are indications of the position on a slope: T – top slope, M – mid-slope, B – bottom slope.
Other burrowing vertebrates in addition to mammals include birds and reptiles. Among the burrowing birds only the burrowing owl (Athene cunicularia) and the parrot “Tricahue” (Cyanoliseus patagonus) are identified. These species have distributions at the Chilean mainland; Chilean seabirds, e.g., burrowing penguins and petrels, were excluded from the list, as in general they are exclusively present on islands (Chester, 2008; Figueroa and Stucchi, 2008; Reyes Arriagada et al., 2013; Zavalaga and Alfaro-Shigueto, 2015). Of the three burrowing reptile species, two are Liolaemus species. Chile has 99 known species of Liolaemus sp. (Ruiz de Gamboa, 2020), but only for the two Liolaemus sp. the burrowing behavior was found documented. The third burrowing reptile is the Chilean Racerunner Callopistes maculatus (Übernickel et al., 2020) with a distribution from just north of Antofagasta to Cauquenes (Contreras et al., 2020).
The species in the four study sites are composed of 27 out of the total 45 Chilean burrowing vertebrates (Table S1). The NP Pan de Azúcar study area has 13 documented (and up to 15, including burrowing species potentially present) burrowing vertebrate species, 13 (up to 17) are present in Santa Gracia, 14 (up to 17) in NP La Campana and 11 (up to 15) in NP Nahuelbuta. Very few species are present along the entire studied gradient: two foxes (Lycalopex griseus, L. culpaeus), two grass mice (Abrothrix olivaceus, A. longipilis), Darwin's leaf-eared mouse (Phyllotis darwini) and the introduced common house mouse (Mus musculus).
The knowledge of the geographic distribution and density of burrowing vertebrates in Chile is sparse for most species, with no further details other than the morphometric description of the animals available (Übernickel et al., 2020). Most details are available for some rodents, especially for the cururo (Spalacopus cyanus) and degu (Octodon degus). Within the known burrowing species, the density of individuals varies considerably on a local level, with most species occurring in densities usually fewer than one individual per hectare, up to an approximate 300 individuals per hectare under favorable, time-restricted circumstances associated with (for example) El Niño–Southern Oscillation (ENSO) events (Cepeda-Pizarro et al., 2005; Lima et al., 1999; Meserve et al., 1995; Previtali et al., 2010). Mammal group sizes vary from a solitary lifestyle, usual for armadillos, skunks and foxes, to groups of more than 15 to 20 individuals for most rodents. These rodent colonies generally inhabit multi-entrance burrows (Iriarte, 2007; Kolb, 1985; Pearson, 1988; Redford and Eisenberg, 1992; Schmid-Holmes et al., 2001; Shepherd and Ditgen, 2013). The burrow entrances are usually clumped in a small area (Iriarte, 2007; Pearson, 1984, 1988, 1951), with larger spacing between complex burrows. There are usually fewer than two colonies per hectare (Cofré and Marquet, 1999; Patton et al., 2015; Redford and Eisenberg, 1992).
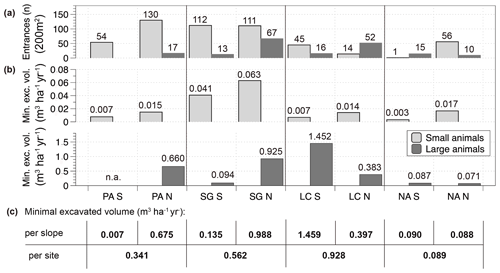
Figure 7Burrow entrance numbers and minimum excavated volumes per slope in an arid to humid–temperate climatic gradient. (a) Quantity of burrow entrances by animals separated by site, slope and animal group (large and small animals), n of entrances are sums of two plots per slope (composed of 2 10 m × 10 m plots, total surface 10 × 20 m2); (b) minimum excavated volume of plots upscaled to m3 ha−1 yr−1 separated by slope; (c) Minimum excavated volume amounts per slope and site summed for all animals; the figure is based on the data of the 2018 field campaign. PA – NP Pan de Azúcar, SG – Santa Gracia, LC – NP La Campana, NA – NP Nahuelbuta, S – south-facing, N – north-facing, n.a. – not applicable. (Note that in PA the sum of the south-facing plot entrances is one plot, and the north-facing entrance sum is three plots.)
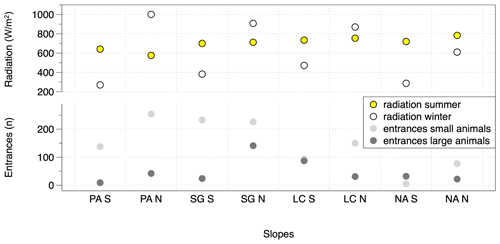
Figure 8Calculated solar radiation and sum of animal burrow entrances separated per animal group (large and small animals) for the slopes along the studied climate gradient; the solar radiation calculation follows Bendix (2004); the slopes are oriented N(orth)-facing and S(outh)-facing; the depicted data are pooled from all three field campaigns: 2016, 2017 and 2018. PA – Pan de Azúcar, SG – Santa Gracia, LC – La Campana, NA – Nahuelbuta. (Note that in PA the sum of the south-facing plot entrances is one plot, and the north-facing entrance sum is three plots.)
Mammal burrow dimensions are highly variable, and for most species the areal extent of burrows is not reported. The following provides an overview of the known basic dimensions available for Chilean species (Übernickel et al., 2020). The largest reported burrows are of armadillos and skunks, with tunnel lengths of up to 4 m and tunnel diameters of up to 30 cm. The longest reported tunnel lengths are of up to 49 m and maximum burrow depths of 1.2 m for the Maule tuco-tuco (Ctenomys maulinus brunneus). The areal extent of burrow systems varies between 20–30 m2 for the mountain mole rat (Chelemys macronyx) and over 200 m2 for the Magellan tuco-tuco (Ctenomys magellanicus), or 300 m burrow lengths for the Puna tuco-tuco (Ctenomys opimus). Known burrowing depths for Chilean mammals reach 3 m for skunks (Conepatus), 1.5 m for armadillos (Chlamyphoridae), at least 80 cm for rabbits (Oryctolagus), 75 cm for tuco-tucos (Ctenomys), 60 cm for mole rats (Chelemys), degus (Octodon) and cururos (Spalacopus), and 28 cm for cavies and 25 cm for rock rats (Aconaemys).
A large group of burrowing small mammals, including rodents and insectivores, vary in their tunnel diameter between 2 and 11 cm. By diameter the entrances are roughly classifiable to distinct groups. The largest rodent-made entrances with diameters of 7 to 10 cm are made by tuco-tucos (Ctenomys sp.), rats (Rattus sp.), rock rats (Aconaemys sp.) and degu (Octodon sp.). Intermediate entrances with diameters of 4 to 7 cm are made by mole rats (Chelemyx sp.), the rabbit rat (Reithrodon sp.), the cururo (Spalacopus cyanus), and mice of several genera (Eligmodontia, Euneomys, Geoxus, Phyllotis), with specific tunnel diameters of 6 cm only reported for G. valdivianus. The smallest entrance diameters among mammals of 2.5 to 4 cm are made by grass mice (Abrothrix sp. and Akodon sp.), the mouse Loxodontomys sp. and the house mouse (Mus musculus).
For non-mammal burrowing vertebrates, information on burrow characteristics is scarce. Concerning lizards, field observations reveal that burrows of the lizard Callopistes vary considerably in size, and frequently they occupy burrows made by Spalacopus cyanus or other rodents. The observed entrance diameter is 10 to 15 cm and 0.6 to 2 m for tunnel lengths, and they have also been observed to shelter under rocks (Arturo Cortés Maldonado, Juan Pablo Castillo Peña, personal communication, 2019). Regarding birds, burrowing parrots make entrances that vary largely in size, 14–49 cm horizontally and 8–25 cm vertically.
3.1.2 Burrowing invertebrates
The distribution of burrowing invertebrate species is heterogeneous along the north to south extent of Chile (Fig. 2). To summarize, the burrowing animal diversity is highest in the semiarid “Norte Chico” (small north) of Chile, followed by the mediterranean area. The humid–temperate area adjacent to the mediterranean area towards the south also contains considerable diversity. Further to the north (central Atacama Desert) and further to the south (Patagonia) comparatively small numbers of burrowing species are registered. In detail, of the total 345 species identified, the largest number (131) is present in the region of Coquimbo. Other regions with high diversity in burrowing invertebrates are the regions of Valparaíso (87), Biobío (81), Metropolitana (75) and Atacama (71, Fig. 2). An intermediate diversity is present at the regions of Maule (69), Araucanía (66), Ñuble (63), Los Lagos (57), L.G.B. O'Higgins (56) and Los Ríos (41). In the following, we summarize the species with the respective information available (Übernickel et al., 2020).
The distribution of the basal taxa, spiders and scorpions, covers nearly all Chile (Fig. 2). Generally, burrowing spiders (Arachnida) are distributed from the region of Antofagasta (II) southwards; most are distributed in central to southern Chile. Trap door spiders and tarantulas are more common in mediterranean and humid–temperate sites, being more of an exception in arid and semiarid sites. Ant and camel (sun) spiders have distribution over most of Chile. Of the Nemesiidae (family of trap door spiders), the genus Lycinus spp., consisting of eight species, has a distributional range in the northern half of Chile, from the regions Antofagasta to Valparaíso (II–V). Solifuges are present only in the semiarid and adjacent regions. Scorpions as a group have wide elevational distributions, from the coastal desert to the high Cordillera of the Andes (0 up to 4500 m a.s.l.). For individual scorpion species the pattern differs: single species exist either at (semi)arid ranges, or at mediterranean to humid–temperate ranges. The scorpion with the broadest distribution in Chile, from the region of Antofagasta (II) to the region of Valparaíso (V), is Caraboctonus keyserlingi.
The distribution of the taxonomically higher and (mostly) volant groups of beetles and hymenopterans covers all of Chile, but subgroups are restricted to specific climatic areas. Here we summarize for beetles, bees and ants; for other subgroups information is not available. Darkling beetles (Gyriosomus) inhabit arid and semiarid sites only, whereas ground beetles, scarab beetles and trogids have wider distributions. Dung beetles are present in humid areas. Praocis (Mesopraocis) beetles inhabit mainly the Chilean Coastal Desert. Burrowing bees are generally distributed from the north to the central region, such as the genus Caupolicana (Colletidae): it is distributed from Arica to the Araucanía. Ants, represented in Chile by ca. 65 species (Cuezzo, 2007; Johnson and Moreau, 2016; Snelling and Hunt, 1975) are distributed over all Chilean biomes from sea level to 2500 m a.s.l. (Ipinza-Regla et al., 1983); a maximum of 47 sympatric species is present in central Chile (antmaps.org, also for genus distributions). Of burrowing species, the large-sized “hormigones” (Camponotus) are one of the most abundant and widely distributed genus in Chile (Snelling and Hunt, 1975). The fire ant genus Solenopsis includes S. gayi, the most common native ant in Chile with the widest distribution. Of the neuropterans, antlions are present in northern (Meserve et al., 2016; Miller and Stange, 2016) and central Chile.
The species of burrowing invertebrates are unknown for most parts of Chile. We approximated the local compositions by counting all burrowing species with published presence within the regions of the study sites. “Likely present” species according to current knowledge were also included. We figure the following species diversity (Table S2): in NP Pan de Azúcar there are 58 burrowing invertebrate species (10 spiders, 2 solifuges, 8 scorpions, 26 beetles, 3 bees, 3 wasps, 5 ants, 1 cicada), the lowest diversity across the four research sites. Compared to the other sites this arid site hosts notably the fewest spider, beetle and ant species. In Santa Gracia the highest number of burrowing invertebrates is present, with 93 species (27 spiders, 3 solifuges, 3 scorpions, 40 beetles, 7 bees, 3 wasps, 9 ants, 1 cicada). Compared to the northern arid site, at this semiarid site the numbers of spider species, beetles, bees and ants are nearly doubled. Towards the south, the overall number of burrowing species remains similar, but with shifts within the taxonomic groups. In NP La Campana 101 burrowing species are likely present, including 32 spiders, 5 solifuges, 6 scorpions, 28 beetles, 7 bees, 5 wasps, 15 ants, 1 termite, 1 antlion and 1 cicada. Compared to the previous semiarid site, here again an increase in the number of spider species number is observable. The scorpion diversity is doubled, beetle diversity is halved and there is noticeably more ant diversity. At the southernmost and humid–temperate site NP Nahuelbuta, 99 burrowing species are estimated presently, including 26 spiders, 10 scorpions, 41 beetles, 3 bees, 1 wasp, 17 ants and 1 cicada. Compared to the previous mediterranean site NP La Campana, the total number is very similar, but there are shifts towards more scorpion and beetle diversity. Burrowing termites and antlions are not known to occur this far south.
Known details of the spatial distribution of burrowing Chilean invertebrates are very scarce. Habitat preferences (e.g., specific substrates species are associated with) are registered for very few species (Übernickel et al., 2020). Details of densities are only recorded for tarantulas (Theraphosidae); outside of Chile they are estimated to have a density of 0.07 to 0.65 m−2 (Pérez-Miles et al., 2005). Some Chilean genera of tarantulas (e.g., Euathlus, Grammostola and Paraphysa) are likely to have very reduced densities, compared to undisturbed conditions, as their trade for the pet industry was banned only in 2018 (Servicio Agrícola y Ganadero, 2018).
Here, the dimensions of invertebrate burrows in Chile are presented and grouped for the basal taxa first, followed by the volant species' details. Trap door spiders make simple tunnels with diameters of 0.5 to 2 cm. Of the trap door spider family Actinopodidae, Plesiolena bonneti burrows in 45∘ inclination to horizontal. A sister species, P. jorgelina, produces burrow tunnels with diameters of 2 cm and to depths of 25 cm. The trap door spider family Nemesiidae burrow with diameters between 0.7 and 1.6 cm and to a maximum depth of 30 cm. Of this family Lycinus sp. excavate tunnels of approximately 1.0–1.5 cm in diameter with more than 10 cm depth, and the burrow walls are coated with silk. Of the fourth family of trap door spiders, Pycnothelidae (ex-Nemesiidae), Acanthognathus sp. make round burrows with estimated entrance diameters of 2 to 2.5 cm, also coated with very fine silk. Tarantulas usually burrow vertically approximately 1 m in depth and coat the inside of their burrows with silk (Perafán and Pérez-Miles, 2014). Among scorpions, species of the family Bothriuridae construct simple burrows of 25 to 50 cm depth, reaching up to 1 m depth occasionally. Female solifuges at locations outside of Chile are described to burrow down to 10 to 20 cm for oviposition.
Burrow dimensions of higher taxa and (mostly) volant groups of beetles, bees, wasps, ants and cicadas are summarized in the following. For other subgroups information is not available. Chilean dung beetles reach burrow depths of 40 to 60 cm. The females of Gyriosomus (Tenebrionidae) and trogids reach burrow depths of 20 cm. Praocis (Mesopraocis) sp. are usually found within the uppermost 30 cm of the substrate, but they have also been found close to 2 m depth (Jaime Pizarro-Araya, personal communication, 2012–2014). They lay eggs on surfaces or at depths between 5 and 10 cm. Both burrowing bee genera, Caupolicana and Cadeguala, burrow their nests in the soil to around 50 cm depth. The burrows of Cadeguala occidentalis reach extensions of 120×170 cm with over 150 entrances. The “digger wasp” Sphex sp. burrows reach 33 cm depth in regions outside of Chile (Brockmann, 1980). The burrows of the cicada Tettigades chilensis reach depths of 45 cm.
3.1.3 Published excavation rates of burrowing animals
Globally, excavation rates of single vertebrate and invertebrate species are published for specific sites (Table 1). Among vertebrates, the globally published excavation rates vary over several orders of magnitude, with the largest excavation rate reported as 56.2 m3 ha−1 yr−1 for the Botta's pocket gopher in a study in the USA (Gabet, 2000). Intermediate excavation rates of 16 to 25 m3 ha−1 yr−1 are more commonly reached by vertebrates, with the highest values by Botta's pocket gopher (23.8 m3 ha−1 yr−1), the house mouse (20.6 m3 ha−1 yr−1), and moles and voles together (20 m3 ha−1 yr−1). A range of excavation rates from 10 to 16 m3 ha−1 yr−1 is reached by the European mole in Russia and the Arctic ground squirrel. Excavation rates up to 10 m3 yr−1 are reported for badgers, bettongs (rat kangaroos), cururos (rodents), porcupines and rabbits. Similarly, the globally published excavation rates for invertebrates also vary (Table 1) with the highest excavation rates reached by earthworms with 37.3 m3 ha−1 yr−1 and ants at 14.4 m3 ha−1 yr−1. Ants and earthworms reach the same order of magnitude as vertebrates' intermediate excavation rates (see above). In comparison, low maximum volumes are published for beetles, with 0.12 m3 ha−1 yr−1.
Table 1Global animal excavation rates from the literature. Data in original work were given in either V – volume or M – mass. When the original data were provided as mass without determined bulk density, a standard value was applied for the calculation.
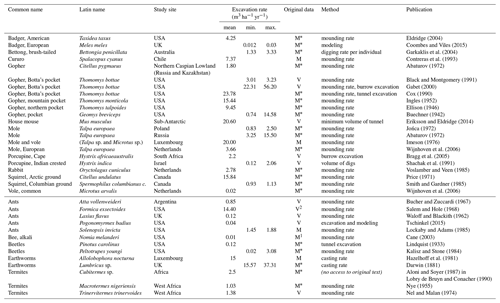
1 Bulk density in original work was extraordinarily high. 2 Mound growth of 0.06 m3 colony−1 yr−1 (Andrews, 1925) was used in combination with notably high number of colonies encountered of n=240 ha−1. * Bulk density not provided; calculated with 1.2 g cm−3 following Amelung et al. (2018).
3.1.4 Maximum burrow depths of vertebrates and invertebrates
Globally, the documented maximum depth of animal burrows is 6 m (gopher tortoises and aardvark dens; Platt et al., 2016). Among invertebrates, the basal taxa of scorpions and spiders reach burrow depths of 70 and 60 cm, respectively (Framenau and Hudson, 2017; Talal et al., 2015). Ant nests are documented up to depths of 4 m, and cicada nests reach depths of 2.5 m (Bétard, 2020; Tschinkel, 2004).
Chilean burrowing mammals are described to reach maximum burrow depths of 3 m for skunks, 1.5 m for armadillos, > 80 cm for rabbits, < 75 cm for tuco-tucos, ∼ 60 cm for mole rats (Chelemys), degus and cururos, ∼ 28 cm for cavies, and < 25 cm for rock rats (Aconaemys). For invertebrates maximum burrowing depths are estimated to range from 100 cm for scorpions to 30 cm for spiders. Higher-taxa burrow depths are 60 cm for beetles, 52 cm for bees and 45 cm for cicadas.
3.2 Field study on animal burrows on hillslopes in Chile
A total of 1590 burrow entrances were found within the plots and measured throughout the three field seasons in 2016 to 2018 (yellow stars, Fig. 1, Fig. 3). During all field visits animal burrow entrances were identified at all sites and in all plots. In general, there are two types of animal burrows: single and small entrances, like those of most invertebrates, or clumped multiple and larger entrances, typical of most rodent burrows. To simplify analysis, burrow entrances were separated into two groups using a diameter of 2.5 cm as a threshold. The threshold is based on the relation of a burrow entrance diameter to the hosts' body width (e.g., Gabet et al., 2003; Vleck, 1981). The smallest entrance diameter reported for mammals is 2–3 cm for Mus musculus (house mouse, Table S1). Here we follow the approach of Kelt et al. (2004) and define a threshold diameter of 2.5 cm to include grass mice. Given this, entrance diameters < 2.5 cm were classified as from “small animals”, mostly invertebrates, and ≥ 2.5 cm for “large animals”, mostly mammals (Fig. 9).
Field observations in Santa Gracia revealed the presence of the lizard Callopistes maculatus identified by characteristic feces adjacent to burrow entrances, located next to a dry riverbed. As for burrowing birds, the owl Athene cunicularia and the parrot Cyanoliseus patagonus were observed. For invertebrates, burrow entrances of approx. 1.5 cm in diameter and the characteristic exuviae of the nymphs of the cicada Tettigades chilensis were found (Kirstin Übernickel and Jaime Pizarro-Araya, personal communication in Santa Gracia, 2019). In Quebrada de Talca, near Santa Gracia, a large ground nest of hymenopterans with a surface extension of approx. 1 m2 was observed. In NP La Campana, burrowing behavior of a female Mummuciidae was observed.
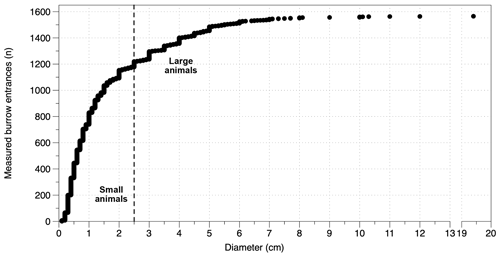
Figure 9Number of measured entrances sorted by diameter. The dashed vertical line indicates the 2.5 cm diameter value of classification between small animals and large animals. ntotal=1565; nsmall animal=1177; nlarge animal=388.
3.2.1 Burrow entrance distribution
The number of entrances found varies per plot between 1 and 108 (median: 24, Fig. 5). The majority of burrow entrances, approximately three-quarters of all entrances, are originated by small animals. Large-animal entrances were found on all slopes. Our observations do not indicate a pattern across top and bottom positions of the plots. Across slopes, more entrances are found on the north-facing slopes than on the south-facing slopes in the two northern sites. In the mediterranean climate of the NP La Campana, the number of registered entrances is the same across both slopes. For the southernmost NP Nahuelbuta, available data are too sparse to allow any conclusion from visual inspection. Along the climate gradient, more entrances are found in arid (n=443) or semiarid sites (n=624), compared to the mediterranean (n=362) or humid–temperate study areas (n=136).
3.2.2 Relationship between burrows and climate
The number of entrances per slope and per study site along the climate gradient could be related to incoming solar radiation. The number of entrances is plotted together with the calculated summer and winter radiation energy on north- and south-facing slopes (Fig. 8). The maximum of the solar radiation at noon throughout a year varies with latitude, and the amount decreases from 26∘ S (PA) to 38∘ S (NA). During the summer, at latitudes > 30∘ S (Santa Gracia) the radiation is higher on north-facing slopes than on south-facing slopes. During winter, there is higher solar radiation on all north-facing slopes, compared to south-facing slopes. The number of burrow entrances decreases from the semiarid site (Santa Gracia) towards the south. Within each site, the majority of the small-animal entrances is located in accordance with the higher radiation on north-facing slopes. The number of large-animal burrow entrances is also higher on north-facing slopes at the arid and semiarid sites. In contrast, at the mediterranean and humid–temperate sites, large animal entrance numbers are higher at the south-facing slopes. Similar to the incoming solar energy, MAT is high at the arid site, peaks at the semiarid Santa Gracia site, and then decreases towards the south (Fig. 10). The MAT in Chile varies from desert maximum values of 20 ∘C in the north to temperatures around 0 ∘C or below in the south of Chile. Small-animal and large-animal entrances follow the same pattern decreasing towards the south with decreasing MAT. MAP counteracts this pattern (Fig. 10). The MAP on the studied climate gradient in Chile varies from 12 mm in the north to 1469 mm in the humid south.
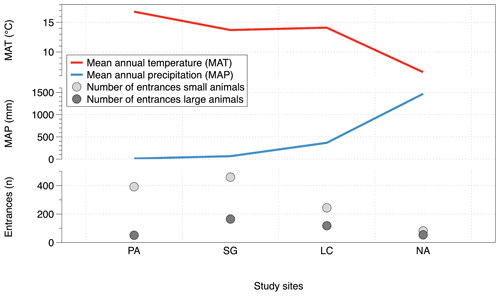
Figure 10Mean annual temperature (MAT) and mean annual precipitation (MAP) and animal burrow entrance distribution along the climatic gradient; the depicted data are pooled from all three field campaigns: 2016, 2017 and 2018; MAT and MAP from Fick and Hijmans (2017). PA – NP Pan de Azúcar, SG – Santa Gracia, LC – NP La Campana, NA – NP Nahuelbuta. (Note that in PA the sum of the south-facing plot entrances is one plot, and the north-facing entrance sum is three plots.)
3.2.3 Burrow dimensions
The burrow dimensions measured were entrance diameter, minimum tunnel length and tunnel orientation. The correlation of the diameter and the minimum tunnel lengths are positive for both groups (Fig. 4), steeper for small-animal entrances (slope: 5.84) and shallower for large-animal entrances (slope: 3.83). The longest straight tunnel length was 47 cm with a diameter of 3.5 cm. For invertebrates the longest detected tunnel length was 16 cm with 2 cm diameter. The minimum burrowed depth, calculated from minimum tunnel length and tunnel orientation, has a mean value of < 6 cm depth (range of mean values for small and large animals per plot: 1.6–11.6 cm, Fig. 6). Maximum values of calculated burrowing depth are < 26 cm for all sites and slopes. For reference, we plotted soil thickness (Oeser et al. 2018) and mobile layer thicknesses from Schaller et al. (2018) from the same sites (Fig. 6). Both the soil and mobile layer thicknesses have values around 20 cm depth in the north and increase towards the south reaching values around 60 to 90 cm depth.
3.2.4 Minimum excavation rates
Minimum excavation rates were calculated from tunnel volumes (Fig. 7). The species-independent minimum excavation rates increase from north to south (calculated including all animals summed for both slopes: PA: 0.34 m3 ha−1 yr−1; SG: 0.56 m3 ha−1 yr−1; LC: 0.93 m3 ha−1 yr; NA: 0.09 m3 ha−1 yr−1), except for the southernmost site. Even through the number of entrances generated by small animals is always larger, the excavation volumes generated by large animals is about 1.5 magnitudes larger than small-animal entrances on the same slope.
Comparing across slopes in each study area the sum of minimum excavation rates (Fig. 7) is larger on the north-facing slope in NP Pan de Azúcar (south-facing: 0.007 m3 ha−1 yr−1; north-facing 0.675 m3 ha−1 yr−1) and also in Santa Gracia (south-facing: 0.135 m3 ha−1 yr−1; north-facing: 0.988 m3 ha−1 yr−1). In NP La Campana, the contrary pattern is visible, i.e., a larger excavation rate on the south-facing slope (south-facing: 1.459 m3 ha−1 yr−1; north-facing 0.397 m3 ha−1 yr−1). At the southernmost site, NP Nahuelbuta, the excavation rate is similar on both slopes (south-facing: 0.090 m3 ha−1 yr−1; north-facing: 0.088 m3 ha−1 yr−1). Focusing on the subgroup of small-animal minimum excavation rates, they are larger on the north-facing slopes at the three northern sites. For large-animal minimum excavation rates a clear pattern is not observed with the available data.
4.1 Literature compilation on Chilean burrowing animals
In this literature review we focus on an inventory of burrowing vertebrates and invertebrates along the climate and ecological gradient in Chile. Currently known burrowing species vary considerably along Chile. Mammal diversity was highest for the mediterranean biome, while invertebrates peaked in their diversity in the semiarid areas.
The compilation of the data was challenging, as the primary aim of most of the included studies was not to quantify rates of sediment transport by burrowing. Moreover, the older literature in Spanish was usually not available through searchable platforms on the internet, or it was not yet digitalized. The compiled data may have a bias towards the authors and their scientific networks contacted during the search of the compiled information. Furthermore, the knowledge of Chilean species, especially invertebrates, is still very limited and may also be biased by many unknown natural histories and distributions of Chilean species. Concerning the quantity of generated knowledge there may be a bias in our compilation towards the central zone of Chile, as it has the most demographic development and longest historic development of zoologic collections at research institutions in the cities of Santiago and Concepción. Nevertheless, currently there are at least 12 universities with zoology research programs ranging from Arica (16∘ S) to Punta Arenas (53∘ S).
4.1.1 Relationship between burrows and climate
Ideally, our compilation would state which species are dominant in moving sediment under changing conditions along the climate gradient in Chile. Unfortunately, this approach is not possible because for most species we lack not only information about their distribution, but also any data about their density. Limited data from previous work suggested the composition of burrowing animals is related to a specific site's climate (Crawford et al., 1993; Paton et al., 1995). This finding is confirmed in our literature review. For mammals, we found a maximum of 18 sympatric species in central Chile with a mediterranean climate in the lowland. Some burrowing species are adaptable to varying climate conditions such as the Chilean rodent cururo Spalacopus cyanus (Begall and Gallardo, 2000; Contreras, 1986), which adapted its body size and behavior to the local conditions over a larger climatic gradient. According to their known distribution, foxes (Lycalopex griseus, L. culpaeus) and the small mammals Abrothrix olivaceus, A. longipilis, Phyllotis darwini and Mus musculus seem also very flexible in the climate in which they live. For invertebrates, the quantity of sympatric species has a maximum of 131 species in the region of Coquimbo (IV), part of the “Norte Chico” semiarid climate (Fig. 2). Some invertebrate groups are restricted to specific biomes such as darkling beetles in the arid and semiarid regions, solifuges in semiarid regions, trap door spiders and tarantulas in mediterranean areas, or dung beetles in humid settings. Fewer species, mostly ants, ant and camel (sun) spiders, as well as scarab beetles, have a very wide distribution across several biomes.
4.1.2 Burrowing animal excavation rates
The published global species-specific excavation rates reach maximum reported values around 56.2 m3 ha−1 yr−1 (Botta's pocket gopher, Gabet, 2000) (Table 1). More common are rates up to 25 m3 ha−1 yr−1 for vertebrates. Interestingly, values for invertebrates reach the same order of magnitude, especially earthworms at 15-37.1 m3 ha−1 yr−1 (Darwin, 1881; Hazelhoff et al., 1981) or ants with excavation rates of 14.4 m3 ha−1 yr−1 (Salem and Hole, 1968). Of the listed values in Table 1, maximum values for ants should be considered with caution because of possible overestimation in the assumptions. Earthworm excavation rates are about 15 m3 ha−1 yr−1, and ant excavation rates are up to 2 m3 ha−1 yr−1, as found by the majority of authors. For most burrowing invertebrates data about excavated volumes remain unknown. The species-specific values reported in the literature are potentially higher than the average burrowing activity, as most study sites were selected because they had a high number of burrows of the target species present (rather than doing a plot scale inventory as in this study). Study outcomes may also vary to a certain degree by different sampling methods, as, for example, burrow volumes may reach more than 20 times the volume of the mounding at the surface (Bétard, 2020; Price, 1971; Tschinkel, 2015). As a result the mound volume calculations presented (Table 1) should be considered as minimum excavation rates. Another established but less commonly used method to determine the excavation rate of animals is the excavation of entire burrow structures. This may result in more exact volume calculations, but it is much more labor intensive and not always possible. For a more general discussion readers are referred to previous work by Haussmann (2017) and Wilkinson (2009) and Smallwood and Morrison (1999a) on gopher burrows. In our excavation rate approach, including the complete composition of burrowing animals, neither of the approaches was applicable. Recognizable mounds next to the entrances were often missing, and it remains unclear if they were generated by the animal in the first place, as not all species create mounds (Price, 1971). Furthermore, excavation of all structures was not possible because of the extensive labor required, the limited likelihood to be able to excavate all structures of all sizes and complexities, and restrictions on applying this method in protected areas. Given these challenges, our method is a minimally invasive method, allowing inclusion of all burrowing animals with entrance diameters ≥ 1 mm under the given circumstances of limited mound availability. This method, the same as the method of measuring mound volumes, results in minimal excavation rates.
4.2 Field study on animal burrows on hillslopes in Chile
This study consolidated previous results from the literature regarding burrowing animals' composition and spatial occurrence variation across the four studied biomes along a climate gradient. In addition, our field study areas (Fig. 3) documented the zoogeomorphic effects on hillslope mass transport at the animal community level and along the climate gradient. We found the distribution of burrow entrances to be heterogeneous for both vertebrate and invertebrate burrowing species within a biome as well as along the climate gradient. More burrow entrances were found at north-facing slopes than on opposing slopes in two of the four biomes, and the highest excavation rates were found in the semiarid and the mediterranean biomes. Links of burrow activity with soil thickness, MAT, MAP and solar radiation are discussed in the following.
4.2.1 Distribution of burrow entrances
Varying soil thickness at top and bottom slope positions of hillslopes could influence the burrow entrance distribution. A thicker soil layer at bottom slope positions potentially provides more substrate and nutrients for vegetation to grow bulbs and rhizomes in, which in turn may provide more favorable food conditions for burrowing herbivores. The soil thickness measurements on south-facing slopes of our study areas increased from arid to humid–temperate sites from approximately 20 to 90 cm and also increased from top to bottom measurements within the slopes (Oeser et al., 2018, Fig. 6). Despite these findings, we did not find a difference in the distribution of burrow entrances within top and bottom slope positions (Fig. 5). Soil thickness appears not to be a limiting factor for burrow entrance distribution between top and bottom slope.
Burrow entrance distribution could also be influenced by the incoming solar energy, which is higher on north-facing slopes in comparison to south-facing slopes in the Southern Hemisphere and has an effect on the available energy and also on water balances (Gallardo-Cruz et al., 2009). The effect of the hillslope aspect (and hence available solar energy) on animal excavation activity has been addressed in previous work. In the Northern Hemisphere in an alpine region in Canada the total sediment displacement of animals has been highest on east- and south-facing slopes, compared to north- and west-facing ones (Hall and Lamont, 2003). Furthermore, in a Chilean animal trap study at a semiarid north-central site, north-facing slopes have had the doubled abundance of small mammals, mostly burrowing species, compared to south-facing slopes (Jiménez et al., 1992). Our findings of a higher number of entrances on north-facing slopes than on south-facing slopes were consistent with both studies in the arid and semiarid biomes (Fig. 5).
4.2.2 Differences in the distribution of large- and small-animal burrows
We observed small-animal burrows to be mostly distributed evenly over the plot surface areas; large-animal burrows were mostly distributed in a clumped manner. Furthermore, large-animal burrow entrances were present on all slopes but not in all plots. We adopted this distributional difference by separation of the data into two groups by their burrow entrance diameter (see results Sect. 3.2) to gain a better understanding of the patterns. If large-animal burrow entrances were not present in the sampled plot, it would provide no indication about their absence or presence and the resulting effect on a hillslope, as an area between colonially used burrows may have been selected. When large-animal burrow entrances were present in a plot, it resulted in a comparatively high impact, which may overprint all volumes excavated by small animals that were up to three-quarters of locally counted entrances (Fig. 7).
In addition, even single large-animal dens, i.e., fox dens, may overprint all other burrowing activity. In NP Pan de Azúcar, adjacent to the plots within a 90 m radius, we observed larger holes that gave the impression of being dens or burrow entrances. A total of 21 of these structures were measured in 2017, which were oriented in all directions. At the bottom of the slopes they were excavated farther than further uphill. Occasionally feces were found in front of the entrances. The diameter of the structures was on average 15 cm (range: 7–57 cm), height was on average 14 cm (range: 4–45 cm), and the excavated depth was on average 34 cm (range: 10–76 cm).
4.2.3 Excavated depths
Animal groups have different burrowing depths. By burrowing, animals are able to maintain favorable conditions of temperature and humidity compared to above-surface conditions (e.g., Bétard, 2020). Described burrowing depths in the literature for Chilean burrowing animals range for vertebrates from 0.25 to 3 m and for invertebrates between 0.3 and 1.0 m. The observed maximum excavated depth measured at all four study sites was < 26 cm (Fig. 6). A previous study at the same study sites had revealed an increasing depth of the mixed layer from arid to more humid conditions, with a maximum of 85 cm in NP La Campana, followed by 70 cm in NP Nahuelbuta, 45 cm in Santa Gracia and 17.5 cm in NP Pan de Azúcar (Fig. 6) (Schaller et al., 2018). The calculated minimal burrow depth reached the soil mixing depth values from the literature only at the arid site. Based on the maximum burrow depths from the literature for the differing taxa in Chile, we presume that real burrow depths are similar to the mobile layer depths reported in the study of Schaller et al. (2018). The observed result is likely an underestimation caused by the measurements procedure, i.e., the inability to easily determine the full burrow extent deeper than the first curvature after the entrance.
4.2.4 Relationship between burrows, climate and climate variation
A correlation between the burrowing activity and regional climate was hypothesized. As indicators of gradients in climate along the extent of Chile we investigated incoming solar radiation, MAT and MAP. The burrowing activity of both vertebrates and invertebrates along the climatic gradient appeared to be linked to all three variables (Figs. 8, 10). The quantity of animal burrow entrances across the study sites showed the highest activity in the semiarid Santa Gracia study area (Fig. 5), followed by arid NP Pan de Azúcar, NP La Campana and lowest activity for NP Nahuelbuta. Because of the clumped distribution patterns of large-animal entrances (see discussion above), the small-animal entrances resemble a more stable indicator. Previous work has found a temperature gradient to have an effect on arthropod density (Tiede et al., 2017) with fewer species at lower mean temperatures. Our results support this finding.
As previously stated, species groups with the largest zoogeomorphic effects vary with climate. For example, in humid areas dominant bioturbators are earthworms (Lumbricidae), whereas in arid areas the effects of mammals are highest (Paton et al., 1995; Whitford and Kay, 1999; Wilkinson et al., 2009). In our results, the minimum excavation rates reached a maximum for invertebrates in the semiarid Santa Gracia, and for vertebrates the highest impact was found in mediterranean NP La Campana (Fig. 7). Nevertheless, a more detailed understanding of animal activity, especially within the invertebrates, is necessary to assign the impact to specific groups.
4.2.5 Minimum excavation rates
The species-independent excavation rates in four biomes studied in Chile (Fig. 3) were quantified. With current possibilities this approach can only be a minimum estimate. In the future, a more complete dataset of all animals present and their burrowed volumes may provide possibilities to model this type of information. But even then, the information would have to be available species by species.
Although the field observations presented provide only initial estimates, they nevertheless highlight trends and patterns present at the hillslope scale. Largest total sediment volumes were displaced in the south-facing mediterranean slope (1.46 m3 ha−1 yr−1, NP La Campana). Along the climate gradient the minimum excavation rates increased from arid to mediterranean, with a decrease at the humid–temperate site such that values were 0.34 m3 ha−1 yr−1 for the arid site, 0.56 m3 ha−1 yr−1 for the semiarid site, 0.93 m3 ha−1 yr−1 for the mediterranean site, and 0.09 m3 ha−1 yr−1 for the humid–temperate site. Compared to published species-specific excavation rates (Table 1), our Chilean study area estimates are in the range observed for invertebrate species. As discussed above, the direct comparison of calculated excavation rates with species-specific rates from the literature has to be evaluated with caution. On the one hand, the presented animal community values are likely to present a more representative excavation rate than the numbers of species-focused studies. On the other hand, the presented values (0.09 to 1.45 m3 ha−1 yr−1, Fig. 7) are minimum excavation rates limited by the study design. The local species-independent excavation rates are likely to be higher but unquantifiable with available observations.
For the invertebrates, observations resulted in a range of excavation rates between 0.003 and 0.063 m3 ha−1 yr−1 (Fig. 7). The values of identified small-animal burrow entrances were likely more reliable from the arid and semiarid sites, because of the bias detecting the entrances in litter cover at the southern sites. The orders of magnitude for excavation rates by small animals in this study are 2–3 orders below the excavation rates of large animals. Regardless, the number of entrances and excavated volumes of small animals also have important impacts on factors other than downhill erosion, such as soil mixing, water runoff or infiltration, bulk density and a range of further secondary effects of bioturbation (Bétard, 2020; further discussion about this topic in Birkby, 1983; Carlson and Whitford, 1991; Jouquet et al., 2006; Lobry de Bruyn and Conacher, 1990; Thorp, 1949; Whitford, 1996).
4.3 Study caveats and future research needs
In our literature review large gaps of knowledge appeared regarding excavating habits in most species, distributional ranges of species, species' densities, excavated masses or volumes, and burrowing depths. Without better knowledge of these values the quantification of downhill erosion by burrowing animals is limited. The method presented in this study is offered as an approach to deduce minimum excavation rates for different animal burrow entrances, independent of animal taxa. In the following, improvements upon the methods used here are discussed.
One issue encountered is the increasing ground cover by vegetation that occurs towards the south and reduces the detection of entrances in our two southernmost study areas (Fig. 3). In NP La Campana the ground was covered with litter where in the southernmost NP Nahuelbuta dense understory vegetation, mainly bamboo twigs and litter, covered the ground. The number of burrow entrances found is very likely to be reduced, and also the minimum excavated volume is presumably underrepresented. Removal of the ground litter would imply a massive disturbance of the plot surface, which would reduce the probability of repeated observation of undisturbed burrowing behavior.
A second issue for consideration is the seasonality of a species' activity when estimating a species' abundance and effect on burrowing. Individual species' activity patterns in Chilean vertebrates and invertebrates may result from species-specific reproduction cycles (e.g., Yunger et al., 2002). Different groups have activity peaks over different times within seasons, making repeated measurements during a year time span favorable. Furthermore, repeated measurements over several year variations in climate variations (e.g., ENSO cycles) and vegetational change are recommended, and dry periods with low plant abundances, mass seedlings, etc. should also be considered. Repeated measurements could also give insight into arid sites. For example, it currently remains unclear if the burrow entrance number in NP Pan de Azúcar is high because rain does not reset the yearly burrowing activity records, or the density of burrowing individuals is comparatively high, even if caused by fewer known species numbers.
A third consideration is the high variation around the positive correlations between diameter and tunnel lengths (Fig. 4). This may be an artifact of measurements limited to the first obstacle (see Methods section). In addition, the excavation rate estimate is only a minimum. With measurements including the curvatures of the tunnels, the slopes of the correlation are expected to be steeper, possibly more similar to the regression shown for small animals. Identification of the burrow builders on species level and known burrow dimensions for the respective species would also be an approach to improve the minimum estimation of excavation rates.
Finally, comprehensive datasets are valuable to improve the conceptual understanding of downhill sediment fluxes. To upscale the conclusions from the hillslope scale to catchment or regional scales, further parameters obtainable with methods from other disciplines would improve the results. Soil texture measurements, as it influences the amount of energy spent, and therefore the overall activity of the respective burrowing species (Price and Podolsky, 1989) could provide improved insights into burrow distributions. Geomorphological techniques (see review by Viles, 2020) would provide additional information, such as the form of sediment traps beneath monitored areas that enable the estimation of total material that moved downhill. Soil mixing rates and differences in mixing rates as dependent on the depth, and over time spans of decades to millennia, could be resolved by luminescence or cosmogenic nuclide techniques applied to depth profiles (Gray et al., 2020; Reimann et al., 2017; Schaller et al., 2018). In addition, photogrammetry of mound modifications over time would be useful for estimating burrow-related sediment fluxes at the surfaces from loose soil patches. UAV remote sensing techniques could serve to more rapidly estimate burrow density in areas without vegetation cover (Lawton et al., 2006). Lidar data measuring vegetation structure could facilitate the identification of the occurrence and abundance of vertebrate (Müller et al., 2010) and invertebrate assemblages (Müller et al., 2018; Vierling et al., 2011).
This review provides a synthesis of existing knowledge and new observations concerning the zoogeomorphic effect of burrowing animals in Chile. This is a first step of quantification of the complete burrowing animals' community at given sites on a hillslope scale and identifies patterns that are some of the principal components that drive differences in animal burrowing effects on hillslopes along a climatic gradient. The quantification of zoogeomorphic effects on hillslope scales is in its infancy, and additional observations are needed. The key findings of this study are highlighted below.
We document 45 burrowing vertebrate and 345 burrowing invertebrate species and species distribution summaries for both groups (Figs. 1d and 2). Most burrowing mammal species are present in the mediterranean climate of Chile. For invertebrates most known burrowing species are present in the semiarid region of Coquimbo (IV) and adjacent regions. A second invertebrate peak of high species diversity is in the humid–temperate area around the region of Biobío (VIII).
For the Chilean study areas, minimum excavation rates are 0.34 m3 ha−1 yr−1 for the arid site, 0.56 m3 ha−1 yr−1 for the semiarid site, 0.93 m3 ha−1 yr−1 for the mediterranean site and 0.09 m3 ha−1 yr−1 for the humid–temperate site, with the latter being vastly underestimated due to vegetation and litter cover. Relative to single species rates from the literature, our calculated excavation rates are in the range of previous invertebrate rates and low for rates of vertebrates.
In this study we discuss the relationships between burrowing animal activity and different metrics such as topographic slope and aspect, as well as latitudinal site-specific variations in solar radiation and climate. We found more burrow entrances on north-facing than on south-facing slopes. On the climate gradient animal burrow entrances showed the highest activity in the semiarid Santa Gracia study area (Fig. 5), a gradual decrease in NP La Campana and lowest activity in NP Nahuelbuta – following decreasing MAT and increasing MAP as well as decreasing incoming solar energy towards the south. Arid NP Pan de Azúcar reflected less activity than the semiarid region, potentially due to the extreme conditions that are more challenging for animals to adapt to.
The following online catalogues were consulted during the literature search:
-
Catalogue of Life, https://www.catalogueoflife.org/ (Catalogue of Life, 2017),
-
the IUCN Red List of Threatened Species, https://www.iucnredlist.org (IUCN, 2017),
-
Antmaps, https://antmaps.org/ (Guénard et al., 2017; Janicki et al., 2016).
The results from the entire literature search for burrowing animals in Chile and the original references have been published as a data publication as Übernickel et al. (2020; https://doi.org/10.5880/fidgeo.2020.042). Additional data for Fig. 1 can be found in the Supplement.
The supplement related to this article is available online at: https://doi.org/10.5194/bg-18-5573-2021-supplement.
KÜ and TAE conceived the study. KÜ designed the experiments and carried them out, with contributions from LP. KÜ, SB and JPA conducted the literature survey. KÜ prepared the manuscript and figures with contributions from TAE and JPA. All co-authors reviewed and improved the final manuscript.
The authors declare that they have no conflict of interest.
Publisher's note: Copernicus Publications remains neutral with regard to jurisdictional claims in published maps and institutional affiliations.
We thank the Chilean National Forestry Corporation (CONAF) for providing access to the sample locations and on-site support for the research. We are grateful for the help with solar radiation calculation to Jörg Bendix (University of Marburg, Germany) and species identification to Jaime R. Rau (ULAGOS, Chile) and Guillermo D'Elía (UACH, Chile). For help with data acquisition we thank the CONAF staff of NP Pan de Azúcar. We thank the International Union for Conservation of Nature (IUCN) for providing the Chilean burrowing vertebrate distributions as shape files online (2018). We thank Nina Farwig for comments on an earlier version of the manuscript. We also acknowledge Andrés A. Ojanguren-Affilastro and Martín J. Ramírez (MACN, Argentina), Elizabeth Chiappa (UPLA, Chile), and Jose Mondaca (SAG, Chile) for natural history of arthropods, and Arturo Cortés Maldonado (ULS, Chile) and Juan Pablo Castillo Peña (ULS, Chile) for natural history of Callopistes. We thank Simon Mudd and an anonymous reviewer for constructive comments.
This research has been supported by the German Research Foundation (DFG) priority research program SPP-1803 “EarthShape: Earth Surface Shaping by Biota”, grant nos. DFG EH329/17-1 and DFG EH329/17-2 to Todd A. Ehlers. Jaime Pizarro-Araya has been supported by the University of La Serena, La Serena, Chile, grant no. DIDULS PR2121210.
This paper was edited by Steven Bouillon and reviewed by Simon Mudd and one anonymous referee.
Abaturov, B. D.: The role of burrowing animals in the transport of mineral substances in the soil, Pedobiologia, 12, 261–266, 1972.
Aloni, K. and Soyer, J.: Cycle des materiaux de construction des termitieres d'humivores en savane au Shaba meridional (zaire), Rev. Zool. Afr., 101, 329–358, 1987.
Andrews, E. A.: Growth of ant mounds, Psyche, 32, 75–87, https://doi.org/10.1155/1925/54079, 1925.
Amelung, W., Blume, H.-P., Fleige, H., Horn, R., Kandeler, E., Kögel-Knabner, I., Kretzschmar, R., Stahr, K., and Wilke, B.-M.: Scheffer/Schachtschabel Lehrbuch der Bodenkunde, 17th Edn., edited by: Scheffer, F. and Schachtschabel, P., Springer Spektrum, Berlin, 749 pp., 2018.
Anderson, R. S. and Anderson, S. P.: Geomorphology: the mechanics and chemistry of landscapes, Cambridge University Press, Cambridge; New York, 637 pp., 2010.
Armesto, J. J., Arroyo, M. T. K., and Hinojosa, L. F.: The mediterranean environment of Central Chile, in: The physical geography of South America, Oxford University Press, New York, 16, 184–199, 2007.
Bangert, R. K. and Slobodchikoff, C. N.: Conservation of prairie dog ecosystem engineering may support arthropod beta and gamma diversity, J. Arid Environ., 67, 100–115, https://doi.org/10.1016/j.jaridenv.2006.01.015, 2006.
Begall, S. and Gallardo, M. H.: Spalacopus cyanus (Rodentia: Octodontidae): an extremist in tunnel constructing and food storing among subterranean mammals, J. Zool., 251, 53–60, https://doi.org/10.1111/j.1469-7998.2000.tb00592.x, 2000.
Bendix, J.: Geländeklimatologie, Gebrüder Borntraeger, Berlin, Stuttgart, 277 pp., 2004.
Bernhard, N., Moskwa, L.-M., Oeser, R., Canessa, R., Bader, M. Y., Baumann, K., Blanckenburg, F. von, Boy, J., Brink, L. van den, Brucker, E., Dippold, M., Ehlers, T. A., Fuentes-Espoz, J. P., Godoy, R., Köster, M., Kuzyakov, J., Leinweber, P., Neidhardt, H., Matus, F., Mueller, C. W., Oelmann, Y., Oses, R., Osses, P., Paulino, L., Schaller, M., Schmid, M., Schmidt, K., Spielvogel, S., Spohn, M., Stock, S., Stroncik, N., Tielbörger, K., Übernickel, K., Scholten, T., Seguel, O., Wagner, D., and Kühn, P.: Pedogenic and microbial response to regional climate and local topography: in-sights from a climate gradient (arid to humid) on granitoid parent material in Chile, Catena, 170, 335–355, https://doi.org/10.1016/j.catena.2018.06.018, 2018.
Bétard, F.: Insects as zoogeomorphic agents: an extended review, Earth Surf. Process. Landf., 46, 89–109, https://doi.org/10.1002/esp.4944, 2020.
Birkby, J. L.: Interaction of western harvester ants with southeastern Montana soils and vegetation, Master of Science in Botany, Montana State University, Bozeman, Montana, USA, 1983.
Black, T. A. and Montgomery, D. R.: Sediment transport by burrowing mammals, Marin County, California, Earth Surf. Process. Landf., 16, 163–172, https://doi.org/10.1002/esp.3290160207, 1991.
Bragg, C. J., Donaldson, J. D., and Ryan, P. G.: Density of Cape porcupines in a semi-arid environment and their impact on soil turnover and related ecosystem processes, J. Arid Environ., 61, 261–275, https://doi.org/10.1016/j.jaridenv.2004.09.007, 2005.
Brockmann, H. J.: The control of nest depth in a digger wasp (Sphex ichneumoneus L.), Anim. Behav., 28, 426–445, https://doi.org/10.1016/S0003-3472(80)80051-0, 1980.
Bucher, E. H. and Zucchardi, R. B.: Significación de los hormigueros de Atta vollenweideri Forel como alteradores del suelo en la Provincia de Tucumán, Acta Zool. Lilloana, 23, 83–96, 1967.
Buechner, H. K.: Interrelationships between the pocket gopher and land use, J. Mammal., 23, 346–348, https://doi.org/10.1093/jmammal/23.3.346a, 1942.
Butler, D. R.: Zoogeomorphology: animals as geomorphic agents, Cambridge University Press, New York, USA, 1995.
Butler, D. R., Whitesides, C. J., Wamsley, J. M., and Tsikalas, S. G.: The geomorphic impacts of animal burrowing and denning, in: Treatise on Geomorphology, Elsevier, 271–280, https://doi.org/10.1016/B978-0-12-374739-6.00334-1, 2013.
Cane, J. H.: Annual displacement of soil in nest tumuli of alkali bees (Nomia melanderi) (Hymenoptera: Apiformes: Halictidae) across an agricultural landscape, J. Kans. Entomol. Soc., 76, 172–176, 2003.
Carlson, S. R. and Whitford, W. G.: Ant mound influence on vegetation and soils in a semiarid mountain ecosystem, Am. Midl. Nat., 126, 125–139, https://doi.org/10.2307/2426157, 1991.
Catalogue of Life: available at: https://www.catalogueoflife.org/, last access: 9 February 2017.
Cepeda-Pizarro, J., Pizarro-Araya, J., and Vásquez, H.: Composición y abundancia de artrópodos epígeos del Parque Nacional Llanos de Challe: impactos del ENOS de 1997 y efectos del hábitat pedológico, Rev. Chil. Hist. Nat., 78, 635–650, https://doi.org/10.4067/S0716-078X2005000400004, 2005.
Chester, S. R.: A wildlife guide to Chile: continental Chile, Chilean Antarctica, Easter Island, Juan Fernandez Archipelago, 1st Edn., Princeton University Press, Princeton, N.J, 391 pp., 2008.
Cofré, H. and Marquet, P. A.: Conservation status, rarity, and geographic priorities for conservation of Chilean mammals: an assessment, Biol. Conserv., 88, 53–68, https://doi.org/10.1016/S0006-3207(98)00090-1, 1999.
Contreras, J. M., Mora-Carreño, M., and Mella Ávila, J. E.: Ampliación del límite norte de distribución de Callopistes maculatus Gravenhorst 1838 (Squamata, Teiidae): registros en la Reserva Nacional La Chimba (Región de Antofagasta, Chile, Bol. Chil. Herpetol., 7, 70–72, 2020.
Contreras, L., Gutiérrez, J. R., Valverde, V., and Cox, G. W.: Ecological relevance of subterranean herbivorous rodents in semiarid coastal Chile, Rev. Chil. Hist. Nat., 66, 357–368, 1993.
Contreras, L. C.: Bioenergetics and distribution of fossorial Spalacopus cyanus (Rodentia): Thermal stress, or cost of burrowing?, Physiol. Zool., 59, 20–28, 1986.
Coombes, M. A. and Viles, H. A.: Population-level zoogeomorphology: The case of the Eurasian badger (Meles meles L.), Phys. Geogr., 36, 215–238, https://doi.org/10.1080/02723646.2015.1026170, 2015.
Corenblit, D., Baas, A. C. W., Bornette, G., Darrozes, J., Delmotte, S., Francis, R. A., Gurnell, A. M., Julien, F., Naiman, R. J., and Steiger, J.: Feedbacks between geomorphology and biota controlling Earth surface processes and landforms: A review of foundation concepts and current understandings, Earth-Sci. Rev., 106, 307–331, https://doi.org/10.1016/j.earscirev.2011.03.002, 2011.
Cox, G. W.: Soil mining by pocket gophers along topographic gradients in a mima moundfield, Ecology, 71, 837–843, https://doi.org/10.2307/1937355, 1990.
Crawford, C. S., Mackay, W. P., and Cepeda-Pizarro, J. G.: Detritivores of the Chilean arid zone (27–32∘ S) and the Namib Desert: a preliminary comparison, Rev. Chil. Hist. Nat., 66, 283–289, 1993.
Cuezzo, F.: First record of the ant genus Oxyepoecus (Formicidae: Myrmicinae: Solenopsidini) in Chile, with remarks on its geographical range, Rev. Soc. Entomológica Argent., 66, 165–167, 2007.
Darwin, C.: The formation of vegetable mold through the action of worms, John Murray, London, 326 pp., 1881.
Dietrich, W. E. and Perron, J. T.: The search for a topographic signature of life, Nature, 439, 411–418, https://doi.org/10.1038/nature04452, 2006.
Dixon, J. L., Heimsath, A. M., Kaste, J., and Amundson, R.: Climate-driven processes of hillslope weathering, Geology, 37, 975–978, https://doi.org/10.1130/G30045A.1, 2009.
Donoso-Barros, R.: La familia Teiidae en Chile, Rev. Chil. Hist. Nat., 55, 41–54, 1960.
Eldridge, D. J.: Mounds of the American badger (Taxidea taxus): significant features of North American shrub-steppe ecosystems, J. Mammal., 85, 1060–1067, https://doi.org/10.1644/BEH-105.1, 2004.
Eldridge, D. J. and Whitford, W. G.: Disturbances by desert rodents are more strongly associated with spatial changes in soil texture than woody encroachment, Plant Soil, 381, 395–404, https://doi.org/10.1007/s11104-014-2142-5, 2014.
Ellison, L.: The pocket gopher in relation to soil erosion on mountain range, Ecology, 27, 101–114, https://doi.org/10.2307/1932505, 1946.
Eriksson, B. and Eldridge, D. J.: Surface destabilisation by the invasive burrowing engineer Mus musculus on a sub-Antarctic island, Geomorphology, 223, 61–66, https://doi.org/10.1016/j.geomorph.2014.06.026, 2014.
Fick, S. E. and Hijmans, R. J.: WorldClim 2: new 1-km spatial resolution climate surfaces for global land areas: new climate surfaces for global land areas, Int. J. Climatol., 37, 4302–4315, https://doi.org/10.1002/joc.5086, 2017.
Figueroa, J. and Stucchi, M.: Peruvian diving petrels Pelecanoides garnotii on Lobos de Afuera islands, Peru, Mar. Ornithol., 36, 189–190, 2008.
Framenau, V. W. and Hudson, P.: Taxonomy, systematics and biology of the Australian halotolerant wolf spider genus Tetralycosa (Araneae: Lycosidae: Artoriinae), Eur. J. Taxon., 335, 1–72, https://doi.org/10.5852/ejt.2017.335, 2017.
Gabet, E. J.: Gopher bioturbation: Field evidence for non-linear hillslope diffusion, Earth Surf. Process. Landf., 25, 1419–1428, https://doi.org/10.1002/1096-9837(200012)25:13<1419::AID-ESP148>3.0.CO;2-1, 2000.
Gabet, E. J., Reichman, O. J., and Seabloom, E. W.: The effects of bioturbation on soil processes and sediment transport, Annu. Rev. Earth Planet. Sci., 31, 249–273, https://doi.org/10.1146/annurev.earth.31.100901.141314, 2003.
Gallardo-Cruz, J. A., Pérez-García, E. A., and Meave, J. A.: β-Diversity and vegetation structure as influenced by slope aspect and altitude in a seasonally dry tropical landscape, Landsc. Ecol., 24, 473–482, https://doi.org/10.1007/s10980-009-9332-1, 2009.
Garkaklis, M. J., Bradley, J. S., and Wooller, R. D.: Digging and soil turnover by a mycophagous marsupial, J. Arid Environ., 56, 569–578, https://doi.org/10.1016/S0140-1963(03)00061-2, 2004.
Goudie, A. S.: The geomorphological role of termites and earthworms in the tropics, in: Biogeomorphology, Basil Blackwell, New York, 166–192, 1988.
Gray, H. J., Keen-Zebert, A., Furbish, D. J., Tucker, G. E., and Mahan, S. A.: Depth-dependent soil mixing persists across climate zones, P. Natl. Acad. Sci., 117, 8750–8756, https://doi.org/10.1073/pnas.1914140117, 2020.
Guénard, B., Weiser, M., Gomez, K., Narula, N., and Economo, E. P.: The Global Ant Biodiversity Informatics (GABI) database: a synthesis of ant species geographic distributions, Myrmecological News, 24, 83–89, 2017 (data available at: https://antmaps.org/, last access: 8 March 2021).
Hall, K. and Lamont, N.: Zoogeomorphology in the Alpine: some observations on abiotic–biotic interactions, Geomorphology, 55, 219–234, https://doi.org/10.1016/S0169-555X(03)00141-7, 2003.
Hall, K., Boelhouwers, J., and Driscoll, K.: Animals as erosion agents in the alpine zone: some data and observations from Canada, Lesotho, and Tibet, Arct. Antarct. Alp. Res., 31, 436–446, https://doi.org/10.2307/1552592, 1999.
Haussmann, N. S.: Soil movement by burrowing mammals: A review comparing excavation size and rate to body mass of excavators, Prog. Phys. Geogr., 41, 29–45, https://doi.org/10.1177/0309133316662569, 2017.
Hazelhoff, L., van Hoof, P., Imeson, A. C., and Kwaad, F. J. P. M.: The exposure of forest soil to erosion by earthworms, Earth Surf. Process. Landf., 6, 235–250, https://doi.org/10.1002/esp.3290060305, 1981.
Hole, F. D.: Effects of animals on soil, Geoderma, 25, 75–112, https://doi.org/10.1016/0016-7061(81)90008-2, 1981.
Huntly, N. and Inouye, R.: Pocket gophers in ecosystems: patterns and mechanisms, BioScience, 38, 786–793, https://doi.org/10.2307/1310788, 1988.
Imeson, A. C.: Some effects of burrowing animals on slope processes in the Luxembourg Ardennes, Part 1 The excavation of animal mounds in experimental plots, Geogr. Ann. Ser. Phys. Geogr., 58, 115–125, https://doi.org/10.2307/520748, 1976.
Ingles, L. G.: The ecology of the mountain pocket gopher, Thomomys monticola, Ecology, 33, 87–95, https://doi.org/10.2307/1931254, 1952.
Ipinza-Regla, J., Covarrubias, R., and Fueyo, R.: Distribución altitudinal de Formicidae en los Andes de Chile Central, Folia Entomológica Mex., 5, 123–128, 1983.
Iriarte, J. A.: Mamíferos de Chile, 1st ed., Lynx, Santiago, Chile, 420 pp., 2007.
IUCN: The IUCN Red List of Threatened Species, available at: https://www.iucnredlist.org, last access: 7 December 2018.
James, A. I., Eldridge, D. J., Koen, T. B., and Moseby, K. E.: Can the invasive European rabbit (Oryctolagus cuniculus) assume the soil engineering role of locally-extinct natives?, Biol. Invasions, 13, 3027–3038, https://doi.org/10.1007/s10530-011-9987-9, 2011.
Janicki, J., Narula, N., Ziegler, M., Guénard, B., and Economo, E. P.: Visualizing and interacting with large-volume biodiversity data using client-server web-mapping applications: The design and implementation of antmaps.org, Ecological Informatics, 32, 185–193, 2016 (data available at: https://antmaps.org/, last access: 8 March 2021).
Jiménez, J. E., Feinsinger, P., and Jaksić, F. M.: Spatiotemporal patterns of an irruption and decline of small mammals in northcentral Chile, J. Mammal., 73, 356–364, https://doi.org/10.2307/1382070, 1992.
Johnson, R. A. and Moreau, C. S.: A new ant genus from southern Argentina and southern Chile, Patagonomyrmex (Hymenoptera: Formicidae), Zootaxa, 4139, 1–31, https://doi.org/10.11646/zootaxa.4139.1.1, 2016.
Jońca, E.: Winter denudation of molehills in mountainous areas, Acta Theriol. (Warsz.), 17, 407–412, https://doi.org/10.4098/AT.arch.72-31, 1972.
Jouquet, P., Dauber, J., Lagerlöf, J., Lavelle, P., and Lepage, M.: Soil invertebrates as ecosystem engineers: Intended and accidental effects on soil and feedback loops, Appl. Soil Ecol., 32, 153–164, https://doi.org/10.1016/j.apsoil.2005.07.004, 2006.
Kalisz, P. J. and Stone, E. L.: Soil mixing by scarab beetles and pocket gophers in north-central Florida, Soil Sci. Soc. Am. J., 48, 169–172, https://doi.org/10.2136/sssaj1984.03615995004800010031x, 1984.
Kelt, D. A., Meserve, P. L., Karina Nabors, L., Forister, M. L., and Gutiérrez, J. R.: Foraging ecology of small mammals in semiarid Chile: the interplay of biotic and abiotic effects, Ecology, 85, 383–397, 2004.
Kolb, H. H.: The burrow structure of the European rabbit (Oryctolagus cuniculus L.), J. Zool., 206, 253–262, https://doi.org/10.1111/j.1469-7998.1985.tb05649.x, 1985.
Lawton, K., Robertson, G., Kirkwood, R., Valencia, J., Schlatter, R., and Smith, D.: An estimate of population sizes of burrowing seabirds at the Diego Ramirez archipelago, Chile, using distance sampling and burrow-scoping, Polar Biol., 29, 229–238, https://doi.org/10.1007/s00300-005-0043-z, 2006.
Lee, K. E. and Foster, R. C.: Soil fauna and soil structure, Soil Res., 29, 745–775, https://doi.org/10.1071/sr9910745, 1991.
Lima, M., Marquet, P. A., and Jaksic, F. M.: El Niño events, precipitation patterns, and rodent outbreaks are statistically associated in semiarid Chile, Ecography, 22, 213–218, https://doi.org/10.1111/j.1600-0587.1999.tb00470.x, 1999.
Lindquist: Amounts of dung buried and soil excavated by certain Coprini (Scarabaeidae), J. Kans. Entomol. Soc., 6, 109–125, 1933.
Lobry de Bruyn, L. A. and Conacher, A. J.: The role of termites and ants in soil modification – a review, Aust. J. Soil Res., 28, 55–93, https://doi.org/10.1071/SR9900055, 1990.
Lockaby, B. G. and Adams, J. C.: Pedoturbation of a forest soil by fire ants, Soil Sci. Soc. Am. J., 49, 220–223, https://doi.org/10.2136/sssaj1985.03615995004900010044x, 1985.
Macdonald, I. A. W., Graber, D. M., DeBenedetti, S., Groves, R. H., and Fuentes, E. R.: Introduced species in nature reserves in mediterranean-type climatic regions of the world, Biol. Conserv., 44, 37–66, https://doi.org/10.1016/0006-3207(88)90004-3, 1988.
Masello, J. F., Pagnossin, M. L., Sommer, C., and Quillfeldt, P.: Population size, provisioning frequency, flock size and foraging range at the largest known colony of Psittaciformes: the Burrowing Parrots of the north-eastern Patagonian coastal cliffs, Emu – Austral Ornithol., 106, 69–79, https://doi.org/10.1071/MU04047, 2006.
Meserve, P. L., Yunger, J. A., Gutierrez, J. R., Contreras, L. C., Milstead, W. B., Lang, B. K., Cramer, K. L., Herrera, S., Lagos, V. O., Silva, S. I., Tabilo, E. L., Torrealba, M.-A., and Jaksic, F. M.: Heterogeneous responses of small mammals to an El Niño Southern Oscillation event in northcentral semiarid Chile and the importance of ecological scale, J. Mammal., 76, 580–595, https://doi.org/10.2307/1382367, 1995.
Meserve, P. L., Vásquez, H., Kelt, D. A., Gutiérrez, J. R., and Milstead, W. B.: Patterns in arthropod abundance and biomass in the semiarid thorn scrub of Bosque Fray Jorge National Park, north-central Chile: A preliminary assessment, J. Arid Environ., 126, 68–75, https://doi.org/10.1016/j.jaridenv.2015.11.002, 2016.
Miller, R. B. and Stange, L. A.: A revision of the genus Eremoleon Banks (Neuroptera: Myrmeleontidae: Nemoleontini), Insecta Mundi, 495, 1–111, 2016.
Müller, J., Stadler, J., and Brandl, R.: Composition versus physiognomy of vegetation as predictors of bird assemblages: The role of lidar, Remote Sens. Environ., 114, 490–495, https://doi.org/10.1016/j.rse.2009.10.006, 2010.
Müller, J., Brandl, R., Brändle, M., Förster, B., de Araujo, B. C., Gossner, M. M., Ladas, A., Wagner, M., Maraun, M., Schall, P., Schmidt, S., Heurich, M., Thorn, S., and Seibold, S.: LiDAR-derived canopy structure supports the more-individuals hypothesis for arthropod diversity in temperate forests, Oikos, 127, 814–824, https://doi.org/10.1111/oik.04972, 2018.
Nel, C. and Malan, E. M.: The distribution of the mounds of Trinervitermes trinerviodes in the central Orange Free State, Nel & Malan: Journal of the Entomological Society of Southern Africa, 37, 251–256, 1974.
Nye, P. H.: Some soilforming processes in the humid tropics: IV. The action of the soil fauna, J. Soil Sci., 6, 73–83, https://doi.org/10.1111/j.1365-2389.1955.tb00831.x, 1955.
Oeser, R. A., Stroncik, N., Moskwa, L.-M., Bernhard, N., Schaller, M., Canessa, R., van den Brink, L., Köster, M., Brucker, E., Stock, S., Fuentes, J. P., Godoy, R., Matus, F. J., Oses Pedraza, R., Osses McIntyre, P., Paulino, L., Seguel, O., Bader, M. Y., Boy, J., Dippold, M. A., Ehlers, T. A., Kühn, P., Kuzyakov, Y., Leinweber, P., Scholten, T., Spielvogel, S., Spohn, M., Übernickel, K., Tielbörger, K., Wagner, D., and von Blanckenburg, F.: Chemistry and microbiology of the Critical Zone along a steep climate and vegetation gradient in the Chilean Coastal Cordillera, Catena, 170, 183–203, 2018.
Paton, T. R., Humphreys, G. S., and Mitchell, P. B.: Soils: A new global view, 238 pp., Yale University Press, USA, UCL Press Limited, UK, 1995.
Patton, J. L., Pardiñas, U. F. J., and D'Elía, G.: Mammals of South America, Volume 2: Rodents, University of Chicago Press, 1384 pp., https://doi.org/10.7208/chicago/9780226169606.001.0001, 2015.
Pearson, O. P.: Mammals in the highlands of southern Peru, Bull. Mus. Comp. Zoöl., 106, 117–217, 1951.
Pearson, O. P.: Taxonomy and natural history of some fossorial rodents of Patagonia, southern Argentina, J. Zool., 202, 225–237, https://doi.org/10.1111/j.1469-7998.1984.tb05952.x, 1984.
Pearson, O. P.: Biology and feeding dynamics of a South American herbivorous rodent, Reithrodon, Stud. Neotropical Fauna Environ., 23, 25–39, https://doi.org/10.1080/01650528809360741, 1988.
Perafán, C. and Pérez-Miles, F.: The Andean tarantulas Euathlus Ausserer, 1875, Paraphysa Simon, 1892 and Phrixotrichus Simon, 1889 (Araneae: Theraphosidae): phylogenetic analysis, genera redefinition and new species descriptions, J. Nat. Hist., 48, 2389–2418, https://doi.org/10.1080/00222933.2014.902142, 2014.
Pérez-Miles, F., Costa, F. G., Toscano-Gadea, C., and Mignone, A.: Ecology and behaviour of the “road tarantulas” Eupalaestrus weijenberghi and Acanthoscurria suina (Araneae, Theraphosidae) from Uruguay, J. Nat. Hist., 39, 483–498, https://doi.org/10.1080/00222930410001671282, 2005.
Platt, B. F., Kolb, D. J., Kunhardt, C. G., Milo, S. P., and New, L. G.: Burrowing through the literature: The impact of soil-disturbing vertebrates on physical and chemical properties of soil, Soil Sci., 181, 175–191, https://doi.org/10.1097/SS.0000000000000150, 2016.
Previtali, M. A., Meserve, P. L., Kelt, D. A., Milstead, W. B., and Gutierrez, J. R.: Effects of more frequent and prolonged El Niño events on life-history parameters of the Degu, a long-lived and slow-reproducing rodent, Conserv. Biol., 24, 18–28, https://doi.org/10.1111/j.1523-1739.2009.01407.x, 2010.
Price, L. W.: Geomorphic effect of the Arctic Ground Squirrel in an alpine environment, Geogr. Ann. Ser. Phys. Geogr., 53, 100–106, 1971.
Price, M. V.: Structure of desert rodent communities: A critical review of questions and approaches, Am. Zool., 26, 39–49, https://doi.org/10.1093/icb/26.1.39, 1986.
Price, M. V. and Podolsky, R. H.: Mechanisms of seed harvest by heteromyid rodents: soil texture effects on harvest rate and seed size selection, Oecologia, 81, 267–273, https://doi.org/10.1007/BF00379814, 1989.
Reading, R. P. and Matchett, R.: Attributes of Black-Tailed Prairie Dog colonies in Northcentral Montana, J. Wildl. Manag., 61, 664–673, https://doi.org/10.2307/3802174, 1997.
Redford, K. H. and Eisenberg, J. F.: Mammals of the Neotropics, Volume 2: The Southern Cone: Chile, Argentina, Uruguay, Paraguay, 474 pp., University of Chicago Press, Chicago, 1992.
Reimann, T., Román-Sánchez, A., Vanwalleghem, T., and Wallinga, J.: Getting a grip on soil reworking – Single-grain feldspar luminescence as a novel tool to quantify soil reworking rates, Quat. Geochronol., 42, 1–14, https://doi.org/10.1016/j.quageo.2017.07.002, 2017.
Reyes Arriagada, R., Hiriart-Bertrand, L., Riquelme, V., Simeone, A., Pütz, K., Lüthi, B., and Raya Rey, A. N.: Population trends of a mixed-species colony of Humboldt and Magellanic Penguins in southern Chile after establishing a protected area, Avian Conserv. Ecol., 8, 1–10, 2013.
Ruiz de Gamboa, M.: Estados de conservación y lista actualizada de los reptiles nativos de Chile, Bol. Chil. Herpetol., 7, 1–11, 2020.
Rundel, P. W. and Weisser, P. J.: La Campana, a new national park in central Chile, Biol. Conserv., 8, 35–46, https://doi.org/10.1016/0006-3207(75)90077-4, 1975.
Rundel, P. W., Dillon, M. O., and Palma, B.: Flora and Vegetation of Pan de Azúcar National Park in the Atacama Desert of Northern Chile, Gayana Botánica, 53, 295–315, 1996.
Salem, M. Z. and Hole, F. D.: Ant (Formica exsectoides) pedoturbation in a forest soil, Soil Sci. Soc. Am. J., 32, 563–567, https://doi.org/10.2136/sssaj1968.03615995003200040039x, 1968.
Schaller, M., Ehlers, T. A., Lang, K. A. H., Schmid, M., and Fuentes-Espoz, J. P.: Addressing the contribution of climate and vegetation cover on hillslope denudation, Chilean Coastal Cordillera (26–38∘ S), Earth Planet. Sc. Lett., 489, 111–122, https://doi.org/10.1016/j.epsl.2018.02.026, 2018.
Schmid-Holmes, S., Drickamer, L. C., Robinson, A. S., and Gillie, L. L.: Burrows and burrow-cleaning behavior of house mice (Mus musculus domesticus), Am. Midl. Nat., 146, 53–62, https://doi.org/10.1674/0003-0031(2001)146[0053:BABCBO]2.0.CO;2, 2001.
Seabloom, E. W., Reichman, O. J., and Gabet, E. J.: The effect of hillslope angle on pocket gopher (Thomomys bottae) burrow geometry, Oecologia, 125, 26–34, https://doi.org/10.1007/PL00008888, 2000.
Servicio Agrícola y Ganadero (SAG): Plan de manejo y desarrollo del PN Nahuelbuta, Departamento de patrimonio forestal, 1–87, available at: https://www.conaf.cl/wp-content/files_mf/1382466219PNNahuelbuta.pdf (last access: 7 October 2021), 1970.
Servicio Agrícola y Ganadero (SAG): Legislación Ley No. 19.473 y su Reglamento, 110, 2018.
Shachak, M., Brand, S., and Gutterman, Y.: Porcupine disturbances and vegetation pattern along a resource gradient in a desert, Oecologia, 88, 141–147, https://doi.org/10.1007/BF00328415, 1991.
Shepherd, J. D. and Ditgen, R. S.: Rodent handling of Araucaria araucana seeds, Austral Ecol., 38, 23–32, https://doi.org/10.1111/j.1442-9993.2012.02366.x, 2013.
Smallwood, K. S. and Morrison, M. L.: Estimating burrow volume and excavation rate of pocket gophers (Geomyidae), Southwest. Nat., 44, 173–183, 1999a.
Smallwood, K. S. and Morrison, M. L.: Spatial scaling of pocket gopher (Geomyidae) density, Southwest. Nat., 44, 73–82, 1999b.
Smith, D. J. and Gardner, J. S.: Geomorphic effects of ground squirrels in the Mount Rae Area, Canadian Rocky Mountains, Arct. Alp. Res., 17, 205–210, https://doi.org/10.2307/1550850, 1985.
Snelling, R. R. and Hunt, J. H.: The ants of Chile (Hymenoptera: Formicidae), Rev. Chil. Hist. Nat., 9, 63–129, 1975.
Starke, J., Ehlers, T. A., and Schaller, M.: Latitudinal effect of vegetation on erosion rates identified along western South America, Science, 367, 1358–1361, https://doi.org/10.1126/science.aaz0840, 2020.
Talal, S., Tesler, I., Sivan, J., Ben-Shlomo, R., Muhammad Tahir, H., Prendini, L., Snir, S., and Gefen, E.: Scorpion speciation in the Holy Land: Multilocus phylogeography corroborates diagnostic differences in morphology and burrowing behavior among Scorpio subspecies and justifies recognition as phylogenetic, ecological and biological species, Mol. Phylogenet. Evol., 91, 226–237, https://doi.org/10.1016/j.ympev.2015.04.028, 2015.
Thorn, C. E.: A preliminary assessment of the geomorphic role of pocket gophers in the alpine zone of the Colorado Front Range, Geogr. Ann. Ser. Phys. Geogr., 60, 181–187, https://doi.org/10.2307/520441, 1978.
Thorp, J.: Effects of certain animals that live in soils, Sci. Mon., 68, 180–191, 1949.
Tiede, Y., Schlautmann, J., Donoso, D. A., Wallis, C. I. B., Bendix, J., Brandl, R., and Farwig, N.: Ants as indicators of environmental change and ecosystem processes, Ecol. Indic., 83, 527–537, https://doi.org/10.1016/j.ecolind.2017.01.029, 2017.
Tschinkel, W. R.: The nest architecture of the Florida harvester ant, Pogonomyrmex badius, J. Insect Sci., 4, 1–19, https://doi.org/10.1093/jis/4.1.21, 2004.
Tschinkel, W. R.: The architecture of subterranean ant nests: beauty and mystery underfoot, J. Bioeconomics, 17, 271–291, https://doi.org/10.1007/s10818-015-9203-6, 2015.
Übernickel, K., Pizarro-Araya, J., and Bhagavathula, S.: Burrowing vertebrates and invertebrates and their characteristics in Chile, GFZ Data Serv. [data set], https://doi.org/10.5880/fidgeo.2020.042, 2020.
Vierling, K. T., Bässler, C., Brandl, R., Vierling, L. A., Weiß, I., and Müller, J.: Spinning a laser web: predicting spider distributions using LiDAR, Ecol. Appl., 21, 577–588, https://doi.org/10.1890/09-2155.1, 2011.
Viles, H.: Biogeomorphology: Past, present and future, Geomorphology, 366, 106809, https://doi.org/10.1016/j.geomorph.2019.06.022, 2020.
Viles, H. A., Goudie, A. S., and Goudie, A. M.: Ants as geomorphological agents: A global assessment, Earth-Sci. Rev., 213, 103469, https://doi.org/10.1016/j.earscirev.2020.103469, 2021.
Vleck, D.: Burrow structure and foraging costs in the fossorial rodent, Thomomys bottae, Oecologia, 49, 391–396, 1981.
Voslamber, B. and Veen, A. W. L.: Digging by badgers and rabbits on some wooded slopes in Belgium, Earth Surf. Process. Landf., 10, 79–82, https://doi.org/10.1002/esp.3290100111, 1985.
Waloff, N. and Blackith, R. E.: The growth and distribution of the mounds of Lasius flavus (Fabricius) (Hym: Formicidae) in Silwood Park, Berkshire, J. Anim. Ecol., 31, 421–437, https://doi.org/10.2307/2044, 1962.
Whitford, W. G.: The importance of the biodiversity of soil biota in arid ecosystems, Biodivers. Conserv., 5, 185–195, 1996.
Whitford, W. G. and Kay, F. R.: Biopedturbation by mammals in deserts: a review, J. Arid Environ., 41, 203–230, https://doi.org/10.1006/jare.1998.0482, 1999.
Wijnhoven, S., Thonon, I., Velde, G. V. D., Leuven, R., Zorn, M., Eijsackers, H., and Smits, T.: The impact of bioturbation by small mammals on heavy metal redistribution in an embanked floodplain of the river Rhine, Water. Air. Soil Pollut., 177, 183–210, https://doi.org/10.1007/s11270-006-9148-4, 2006.
Wilkinson, M. T., Richards, P. J., and Humphreys, G. S.: Breaking ground: Pedological, geological, and ecological implications of soil bioturbation, Earth-Sci. Rev., 97, 257–272, https://doi.org/10.1016/j.earscirev.2009.09.005, 2009.
Wilske, B., Eccard, J. A., Zistl-Schlingmann, M., Hohmann, M., Methler, A., Gerde, A., Liesenjohann, T., Dannenmann, M., Butterbach-Bahl, K., and Breuer, L.: Effects of short term bioturbation by common voles on biogeochemical soil variables, PlosOne, 10, 1–19, https://doi.org/10.1371/journal.pone.0126011, 2015.
Winchell, E. W., Anderson, R. S., Lombardi, E. M., and Doak, D. F.: Gophers as geomorphic agents in the Colorado Front Range subalpine zone, Geomorphology, 264, 41–51, https://doi.org/10.1016/j.geomorph.2016.04.003, 2016.
Yunger, J. A., Meserve, P. L., and Gutiérrez, J. R.: Small-mammal foraging behavior: mechanisms for coexistence and implication for population dynamics, Ecol. Monogr., 72, 561–577, https://doi.org/10.1890/0012-9615(2002)072[0561:SMFBMF]2.0.CO;2, 2002.
Zavalaga, C. B. and Alfaro-Shigueto, J.: Unveiling an important Humboldt Penguin (Spheniscus humboldti) breeding colony in Perú and the need for its protection against the potential impact of guano harvest, Waterbirds, 38, 302–307, https://doi.org/10.1675/063.038.0311, 2015.
Zhang, Y., Zhang, Z., and Liu, J.: Burrowing rodents as ecosystem engineers: the ecology and management of plateau zokors Myospalax fontanierii in alpine meadow ecosystems on the Tibetan Plateau, Mammal Rev., 33, 284–294, https://doi.org/10.1046/j.1365-2907.2003.00020.x, 2003.