the Creative Commons Attribution 4.0 License.
the Creative Commons Attribution 4.0 License.
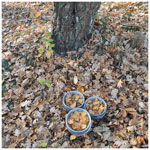
Reviews and syntheses: VOC emissions from soil cover in boreal and temperate natural ecosystems of the Northern Hemisphere
Andrej A. Zaitsev
Plant litter decomposition is a biogeochemical process underlying the carbon cycle in terrestrial ecosystems and between the biosphere and the atmosphere. For the latter, it serves as one of the most important sources of not only carbon dioxide but also volatile organic compounds (VOCs), which have not yet been taken into account in atmospheric models for various purposes and scales, from local to regional and global. This review owes its appearance to the growing interest in decaying leaf litter and living forest floor cover as a hitherto unaccounted for source of photochemically active components of the Earth's atmosphere. This interest is understandable if we take into account the size of this source: for terrestrial ecosystems, the global production of litter is 10 × 1016 g dry matter. The living vegetation cover of the soil on the forest floor, mainly comprising mosses and small shrubs, should also be regarded as a potentially significant source of atmospheric VOCs, as its total biomass may be comparable to or even exceed that of canopy foliage, which is considered the main source of these compounds. This implies a need to integrate these sources into biogenic VOC emission models, which in turn requires extensive research on these sources to understand the conditions and factors that influence VOC emissions. The decomposition of leaf litter, accompanied by the release of VOCs, is a very complex process that depends on a number of biological, chemical and physical environmental factors, but little information is currently available on the role each plays. Equally limited is information on the chemical composition and emission rates of VOCs from these sources.
The review focuses on the main gaps in our knowledge of the sources of biogenic VOCs under the forest canopy, and we are confident that filling them will make a significant contribution to solving such an important task as closing the global organic carbon budget.
- Article
(887 KB) - Full-text XML
-
Supplement
(917 KB) - BibTeX
- EndNote
- Included in Encyclopedia of Geosciences
Terrestrial living vegetation is the main source of atmospheric volatile organic compounds (VOCs) that significantly affects chemical processes in the boundary layer (Isidorov, 1990; Fehsenfeld et al., 1992; Guenther et al., 1995; Navarro et al., 2014; Sindelarova et al., 2014). These emissions have received considerable attention due to the fact that most of them are highly reactive and thus affect atmospheric chemistry to a greater extent than comparable amounts of organic pollutants from industrial and motor vehicle emissions (Bell and Ellis, 2004). In the atmosphere, VOCs undergo gas-phase oxidation (Atkinson and Arey, 2003) or photo-stimulated oxidation on the surface of solid atmospheric aerosols (Isidorov, 1992: Isidorov et al., 1997). Their reactions with •OH radicals and NOx result in the production of secondary photooxidants, such as ozone, H2O2, ROOH and peroxyacetyl nitrate (PAN). Furthermore, the oxidation of biogenic VOCs initiates the production of secondary atmospheric aerosols, which influence the radiative budget of the troposphere (Makkonen et al., 2012; Kulmala et al., 2001, 2013; Faiola et al., 2014; Kourtchev et al., 2016; Lee et al., 2019). An increase in the tropospheric ozone concentration can also affect the climate by perturbing the Earth's radiation budget, as O3 is the third-most important greenhouse gas (Intergovernmental Panel on Climate Change, IPCC, 2013; Kim et al., 2015).
Exposure to elevated surface ozone concentration has detrimental impacts on human health, natural vegetation and crop yield (Chuwah et al., 2015; Zhou et al., 2017; De Marco et al., 2019). In particular, ground-level ozone pollution has become one of the top issues in China and India. According to Feng et al. (2015), throughout China current and future O3 levels will induce a wheat yield loss of 6.4 %–14.9 % and 14.8 %–23.0%, respectively. Moreover, economic losses from wheat and rice in India attributable to ozone are estimated at USD 6.5 billion per year (Sharma et al., 2019). For these reasons, tropospheric ozone production, temporal variations in its concentrations over different regions and examinations of future near-surface air quality changes have been the subjects of numerous recent investigations and publications (Vingarzan, 2004; Curci et al., 2009; Derwent et al., 2015; Dolwick et al., 2015; Gaudel et al., 2015; Kim et al., 2015; Santurtún et al., 2015; Zong et al., 2018; Masiol et al., 2019; Strode et al., 2019; Archibald et al., 2020; Zhang et al., 2021).
To examine the contribution of biogenic organic volatiles to ozone and other secondary pollutant formations, various chemistry transport models have been developed, with VOC and NOx emissions as the input data. Accurate and reliable emission inventories are essential for good model performance; any underestimation of VOCs in the emissions inventory could cause an underestimation of ozone levels by photochemical models (Bell and Ellis, 2004; Mogensen et al., 2015; Zhou et al., 2017). However, it is well known that the estimation of biogenic VOC emissions is still characterised by considerable uncertainty, with a factor of 3−5 for key species such as isoprene (Curci et al., 2009), even though research in this area has been continuously conducted for many years (Guenther et al., 2006; Karl et al., 2009). This uncertainty can be partially connected with systematic errors in the VOC budget, e.g. inadequate emission factors or omission of significant biological sources. Recent work on the determination of the total reactivity of hydroxyl radicals, total OH reactivity emission in different types of forests also indicates the existence of unaccounted for sinks of this radical (Di Carlo et al., 2004; Sinha et al., 2010; Nölscher et al., 2013; Yang et al., 2016; Praplan et al., 2020), that is, the presence of unaccounted sources of reactive biogenic VOCs in the atmosphere. This implies the need to identify and quantitatively characterise sources of unknown reactive VOCs in the atmosphere (Hellén et al., 2021).
Emissions from leaf litter and non-arboreal vegetation under the forest canopy – such as mosses, lichens, grasses and small shrubs, as well as the dead remains of grass in vast steppes (steppe mat), prairies and savannahs – belong to this category of “lost” or “omitted” natural VOC sources (Isidorov, 1992, 1994; Isidorov et al., 1994; Tang et al., 2019). The potential importance of this source of biogenic VOCs evidently results from its gigantic scale: for land ecosystems, the litter mass is estimated to be 6×1016 g C (Bolin, 1983; Zlotin and Bazilevich, 1993), and the global estimate of litter production is in the range (9–10) ×1016 g yr−1 dry matter (Matthews, 1997). According to Liu et al. (2003) the annual leaf litter production in the boreal forests of Eurasia is (1.49 ± 0.61) ×1015 g yr−1, with a total aboveground litter production of (2.07 ± 0.85) ×1015 g yr−1. Depending on different factors, the litter layer can develop to significant thicknesses, exemplified by the ∼40 mm reported from a 20-year unburned Eucalyptus marginata forest in western Australia or the ∼ 60 mm reported under Pinus pinaster in France. For five types of forest in seven southern states of the USA, an average litter depth of ∼ 37 mm was reported. Larger litter thicknesses have also been reported, including a mean of 86 mm under Cryptomeria japonica in Japan (Dunkerley, 2015, and references therein).
Dead plant material contains substantial amounts of volatile organic compounds. A recent study of the chemical composition of green, senescent leaves and partially decomposed silver birch (Betula pendula) leaves in southern Finland showed that although the concentration of many secondary metabolites is significantly reduced, almost all of them are found in both senescent leaves and fresh litter (Paaso et al., 2017). Furthermore, Wood et al. (1995) did not detect statistically significant differences in monoterpene yields in green, yellow or newly fallen brown leaves of the hardwood tree Umbellularia californica and concluded that there is a lack of catabolism of monoterpenes prior to leaf senescence. However, the results of these isolated studies do not give grounds to state that ageing leaves enter the soil with almost the same yield of monoterpene as on the tree. Indeed, in one study, the total monoterpene content was higher in the fresh needles of Pinus monophylla (5.6 ± 2.2 mg g−1 extracted air dry weight, d.w.) than in senescent needles (3.6 ± 1.8 mg g−1 d.w.), regardless of whether they were still attached to the tree or formed the freshest layer of the understorey litter (Wilt et al., 1993). In another study, samples of Scots pine (Pinus sylvestris L.) needles and needle litters collected in low-productive forests in eastern Siberia contained, respectively, 7.9 ± 1.2 and 3.9 ± 0.2 mg g−1 (d.w.) of essential oils, mainly consisting of volatile monoterpene hydrocarbons (Stepen et al., 1987). About 15 % of the total store of essential oils in these forests is contained in litter, with a biomass of 12–15 t ha−1. According to Klemmedson et al. (1990), litter needle biomass comprises 20 % of the live foliar biomass in Canadian ponderosa pine forest. More productive forests of the Northern Hemisphere can accumulate enormous amounts of “dead” organic material. For example, total litter mass in a 100-year-old maritime pine (P. pinaster) forest in southern France was 43.1 ± 12.0 t ha−1, and the depth of the needle compartment of the floor averaged 70 mm (Kurz et al., 2000). The chemical composition of the litter was not determined by the authors, but it can be supposed that the fallen needles in this maritime pine forest contain considerable amounts of terpenes. Under the influence of external factors (both abiogenic and biogenic), decomposition of the tissues and the cell structures of the needles takes place, resulting in the formation of volatile compounds and their evolution into the gas phase.
The first author of this review encountered this phenomenon in 1976 while studying the composition of VOCs in the atmosphere of a city (Leningrad, former USSR), using gas chromatography–mass spectrometry (GC-MS). Air samples were collected in a small public garden at the end of October, i.e. when the vegetation period had finished. For this reason, it was an absolute surprise to identify α-pinene, camphene and limonene in the air samples (their peaks were among the more intensive peaks on the chromatograms) and six other less abundant monoterpene hydrocarbons (Ioffe et al., 1977). In later investigations, it was determined that fresh leaf litter of black poplar (Populus nigra) was the source of terpenes at this sampling site. This was the second surprise because the authors' 1976 summer GC-MS investigations of the volatiles emitted by the living foliage of this plant had demonstrated the intensive evolution of isoprene into the gas phase but the complete absence of any terpene hydrocarbons among other volatiles. However, the author's attention was drawn to leaf litter as a source of atmospheric VOCs, as reported by Zimmerman et al. (1978), who were the first to pay attention to leaf litter and report the magnitude of the VOC release rate from this source. According to their estimation, this averages 162 µg m−2 h−1 at 30 ∘C. In spite of this publication being cited more than 300 times (i.e. well known to the atmospheric chemistry community), this natural source of reactive VOCs has remained unaccounted for and unquantified (it was “a hitherto unrecognised atmospheric source”, Warneke et al., 1999). The same is true for another component of forest ecosystems, namely living soil cover.
The purpose of this review is to draw the closer attention of atmospheric chemists to the VOC emissions from these important components of terrestrial natural ecosystems. To our mind, careful estimation of steppe- and forest-floor-derived VOCs will help improve the reliability of estimates of biogenic emissions so that they can be incorporated into chemistry transport models, as well as improve our understanding of land–atmosphere exchanges and interactions.
In this review, we will focus only on forest floor emissions of non-methane VOCs from boreal and temperate natural ecosystems in the Northern Hemisphere. This limitation is due to the fact that at present there is almost no corresponding data for subtropical and tropical forests in both hemispheres. The vegetation of boreal and sub-boreal forests (whose area represents approximately 16 780 ×103 km2 or about 11.2 % of the terrestrial area of our globe) accumulates a substantial proportion of the planet's living matter: about 700×106 t (d.w.). Biomass in different types of forests varies from 10 000 to 40 000 t km−2. Biomass production in coniferous forests in the north taiga zone is equal to ca. 450 t km−2, whereas in the mixed and broad-leaved forests in the south taiga zone it reaches 800 and 900 t × km−2, respectively (Dobrovolsky, 1998).
Dying parts of plants fall off and form litter, the quantity of which is not proportional to vegetation biomass. For example, south taiga forests with considerable biomass (more than 30 000 t km−2 d.w.) deposit approximately 500 t km−2 (d.w.) of litter in the soil yearly, whereas meadow steppe vegetation with significantly lower amounts of biomass (ca. 2500 t km−2) forms nearly 1500 t km−2 of litter (Dobrovolsky, 1998).
Leaf litter is defined here as the uppermost layer of the remains of dead leaves on the soil surface of a forest, meadow or steppe, decomposed to varying degrees. Living soil cover (LSC), as we understand it, refers to forest floor vegetation formed by understorey dwarf shrubs, mosses, lichens, herbs and ferns. As a rule, in boreal and temperate regions, litterfall is associated with the coming of cold, autumnal weather. However, we should also take into account the transitory but intensive spring falling of bud scales, petals and flowers. This material is characterised by significantly higher N and P levels but has lower concentrations of lignin and cellulose compared to leaf litter (Wang et al., 2016). For this reason, fallen flowers decompose much faster (within 1–1.5 months) than leaves. Many bud scales (for example, protecting buds of different poplar species) are covered with a resinous or gummy substance, mainly consisting of volatile terpenoids. Defoliation of deciduous trees can also occur during the summer, as in July 2015 in the north-eastern region of Poland and in the summer months of 2018 in Germany. Intensive summer litterfall (more than 15 % of the foliage store) is the result of an acute soil water deficit. Besides, many evergreen coniferous trees shed their leaves all year round.
The following chapters will give an overview of the main processes governing litter decomposition and litter-derived VOC emissions, their chemical composition, the participation of litter-destroying microorganisms in their formation, and available information regarding the emission rates of separate VOCs. The Supplementary material to this article provides data on litter and living soil biomass stocks in boreal and temperate forests of the Northern Hemisphere that are useful for estimating the magnitude of VOC emissions.
2.1 The role of biological processes
The evolution of volatile organic compounds from dead plant tissues mostly takes place after their more or less advanced decomposition. Two groups of factors controlling this process in terrestrial ecosystems can be distinguished: abiotic and biotic ones (Swift et al., 1979; Berg, 2014; Krishna and Mohan, 2017). The latter group includes complex interactions between litter-inhabiting saprotrophic (invertebrate and vertebrate) animals and microorganisms.
Leaf-eating insects can influence decomposition processes by modifying the quality of litter. Many studies have demonstrated that soil fauna such as isopods, gastropods, arthropods and other detritophages significantly affect the decomposition rate (Coulis et al., 2013; David, 2014; Frouz et al., 2015; Hassall et al., 1987; Kozlov et al., 2016; Lukowski et al., 2021; Pokhylenko et al., 2020; Wang et al., 2015; Zimmer et al., 2003). Macroarthropods are believed to enhance the decomposition rate of leaf litter and increase VOC emissions both directly and indirectly. Their direct contribution includes the fragmenting of dead plant material and increasing the surface area available for microbial colonisation, while their indirect contribution consists in ingesting saprotrophic litter-colonising microbiota and egesting their faeces in surface-increased substrate, thereby enhancing microbial activity (Zimmer et al., 2003).
Further decomposition of crushed litter is performed by microorganisms, bacteria, actinomycetes and fungi (Trowbridge et al., 2020). The last constitute a major component of soil biota and represent the primary decomposers in most environments. Indeed, fungi produce the enzymes (including extracellular enzymes) necessary to decompose the lignocellulose matrix of leaf litter and wood (Cox et al., 2001; Fioretto et al., 2007). Participating in the decomposition of organic matter of plant origin, these saprotrophic organisms produce their own, inherent metabolites; as part of these secondary products are volatile organic compounds, they can be transferred into the gas phase (Svendsen et al., 2018).
2.2 Abiotic processes
The microbial–zoological interactions mentioned above stimulate intense abiotic processes such as the evaporation of volatiles and the leaching of water-soluble compounds. Another abiotic process aiding the evolution of volatiles from plant tissues is their mechanical destruction, brought about by the freezing−thawing of dead organic material (Porchikalov and Karelin, 2015). In some studies thermo- and photochemical reactions caused by direct solar radiation are described as abiotic destruction processes; their role is much more pronounced in forest-steppe and steppe landscapes than under the forest canopy.
The relative proportion of biotic and abiotic processes varies greatly, primarily depending on climatic conditions, yet there is only scarce corresponding information available in the literature (Kravchenko et al., 2019). According to Bazilevich et al. (1993), leaching predominates among other abiotic factors in humid north taiga forests. To assess the role of various natural factors in the decomposition of plant fall, these authors conducted field experiments in different regions of Russia. The general conclusion was that there are two principal factors limiting the intensity of organic matter destruction: in tundra-forest and north taiga zones the key factor is a lack of heat, while in semi-arid steppe ecosystems it is a lack of moisture. These authors' experiments, while supporting the phenomenon of the biological decomposition of dead organic material, also highlight the important role of abiotic factors in decomposition. The authors conducted experiments in two neighbouring ecosystems in the central European part of Russia, finding that the proportions of biotic and abiotic factors differ in forest and meadow steppe (Table 1). As can be seen, the contribution of abiotic processes in the case of open landscapes (meadow steppe) significantly exceeds that in the oak forest, and this difference is associated with different levels of solar radiation. Litter-bag experiments with five levels of solar light intensity (controlled by special screens) showed that the decomposition rate of dead aspen and grass leaves declines gradually with decreases in solar radiation intensity and short wavelength radiation (Bazilevich et al., 1993).
Table 1Rate of decomposition of dead plant parts (%) during the warm season in two ecosystems of the forest-steppe zone of Russia (Bazilevich et al., 1993).
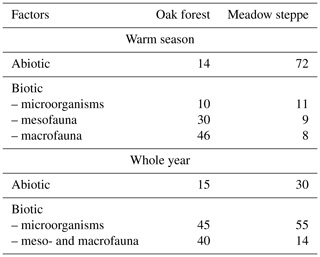
Bosco et al. (2016) found additive effects of ultraviolet (UV) radiation and soil water on litter mass loss. Recent laboratory experiments have shown that the photodegradation of plant litter driven by UV radiation is important in influencing mass loss, nutrient release and the carbon balance in a broad range of terrestrial ecosystems (Austin et al., 2016; Li et al., 2016). It can be assumed that the loss of litter mass under the action of solar radiation (light and/or its thermal component) is at least partially related to the emission of VOCs, but at present it is difficult to draw definite conclusions in this regard due to the limited number of studies of this phenomenon.
Karl et al. (2005) did not see a significant influence of photosynthetically active radiation (PAR) on emissions of VOCs from senescing grass crops. However, shortwave radiation can stimulate different types of photochemical processes. UV-induced C2–C5 hydrocarbon emissions have been measured by Derendorp et al. (2011a, b) from the leaf litter of some plant species, with the influence of experimental conditions determined. In the absence of oxygen, no emissions of C2–C5 hydrocarbons were observed. When the litter was placed in humid air, the emission rates approximately tripled compared with the emissions from leaf litter in dry air. According to the authors, UV-induced hydrocarbon emissions from leaf litter may have a small influence on atmospheric chemistry at the local scale but do not contribute significantly to their global budgets. However, it seems that this conclusion is premature because our knowledge about the influence of shortwave solar radiation on the decomposition of dead plant material and VOC emissions is very limited.
Further field and laboratory measurements are needed to investigate the impact of UV-B radiation (280–310 nm), together with UV-A (315–400 nm) and visible radiation (400–700 nm). It is well worth remembering that senescent leaf matter photoproduces carbon monoxide, which has an important effect on atmospheric composition (Tarr et al., 1995; Derendorp et al., 2011c). In Tarr et al.'s (1995) study, leaf litter photoproduced CO at rates that ranged from 1.3 to 5.4 times higher per unit area than living leaves did. According to Bornman et al. (2015), UV radiation can also stimulate VOC emissions from both living plant and leaf litter, although the magnitude, rates and spatial patterns of these (supposed) emissions remain unknown at present.
On the other hand, there are experimental confirmations of the de novo synthesis of partially oxidised volatile organic compounds in dead plant material under the action of the heat component of solar radiation. Warneke et al. (1999) conducted experiments involving the alternate “roasting” (up to 60–100 ∘C) and wetting of dead beech leaves, demonstrating multiple increases in the emission rates of oxidised VOCs such as methanol, acetone, acetaldehyde, ethanol and butan-2-one. Moreover, these authors showed that even at room temperature, considerable amounts of these volatiles are released over a period of days and weeks. According to the authors' previous estimation, the decay of 1 g of leaf matter produces at least 100 µg of acetone and 300–500 µg of methanol. Global annual emissions of acetone and methanol from dead leaf matter may be at least 6–8 and 18–40 Tg, respectively. Considering the temperature dependence of the emission rates of the VOCs, Warneke et al. (1999) predicted the largest emission rates to be in the late spring and early autumn.
Evidently, similar thermochemical processes can take place more intensively in felted dead grass litter in semi-arid steppe ecosystems, as well as on the outskirts of forests and in open-canopy forests (Hewins et al., 2019). The summer soil surface temperature can reach 60–80 ∘C in these landscapes.
Paradoxically, the abiotic strengthening of VOC emissions can also be stimulated by the process exactly opposite to “roasting”, namely freezing. Frequent freezing and thawing events, especially characteristic in the last two decades for high-latitude regions, can positively influence the decomposition rate (Porchikalov and Karelin, 2015; Jiang et al., 2016). Some authors have presented the idea that a source of reactive VOCs is the wounding processes that occur after leaves undergo freeze–thaw damage. Fukuj and Doskey (1998) measured VOC emissions from a grassland site before and after frost events and reported morning frost-enhanced emissions of acetaldehyde, acetone, methanol and ethanol. Fall et al. (2001) detected the emissions of low-molecular-weight oxygenated VOCs during leaf drying, senescence and following freeze–thaw damage. Leaves of beech, larch, clover, bluegrass and fern wounded in this way released 1-penten-3-ol, 2- and 3-methylbutanal, 2(E)-penten-1-ol, 1-penten-3-one, hexanal and some other minor compounds (supposedly isomeric pentenals) into the gas phase. Moreover, frozen and thawed C5 and C6 compounds were detected by proton transfer reaction mass spectrometry (GC-PTR-MS) and PTR-MS techniques in mid-November 1999 at the Sonnblick Observatory (Austrian Alps, 3106 m a.s.l., above sea level). After a hard freeze had occurred in the central alpine valleys of Austria, unprecedented levels of highly reactive C5 and C6 biogenic VOCs were registered in ambient air transported from these valleys: isoprene, 2- and 3-methylbutanal, 1-penten-3-ol, 1-penten-3-one, hexanal, and 3(Z)- and 2(E)-hexenal. These were the same VOCs seen in the freeze−thaw wounding experiments. Fall et al. (2001) speculated that VOCs were released from freeze-damaged local vegetation, larch and fir trees. This seems doubtful because living frost-hardy trees are quite well protected from such kinds of wounding. However, saturated by rainwater, dead plant matter (for example fallen larch and fir needles) is deprived of this biological protection. After autumn rains, the litter can remain damp for a long time (Wood et al., 1995). In frosty weather, the crystallisation of surplus water takes place, damaging cell and tissue structures. Besides, freezing has a destructive effect on the resin canals in the litter (Wu et al., 2010). All these forms of damage can increase the release and evolution of volatiles.
At present, it is not possible to determine how various time or spatial features affect the proportions of abiogenic and biogenic processes in the emissions of VOCs from dead plant material. According to some authors (Zhang et al., 2008; Gray et al., 2010), abiotic factors play an additional role compared to litter microbial degradation and VOC emissions on a global scale. The predominance of the microbiological production of volatile organics has been demonstrated by Leff and Fierer (2008), who discovered a correlation between respiration, microbial biomass and level of VOC emissions. However, the information presented in this chapter allows us to conclude that substantial quantities of low molecular weight C5−C6 VOCs can be formed as the result of the abiotic decomposition of plant litter. Due to its importance in atmospheric chemistry, it can be concluded that much more research should be devoted to this natural source of atmospheric VOCs. In particular, UV radiation, heat and frost must be regarded as important triggers for seasonal emissions of these VOCs by plant litter.
The second conclusion is related to the qualitative composition of leaf litter emissions: one can expect among these emissions not only plant-derived compounds but also VOCs of microbial and animal origin.
Over the last few decades, the chemical composition of volatile emissions from some thousands of plant species – including practically all forest-forming trees of the Northern Hemisphere – has been investigated. For many hundreds of species, the emission rates of principal biogenic VOCs, isoprene and monoterpenes have also been determined (Kesselmeier and Staudt, 1999). The available information on the chemical composition of litter-derived compounds is much poorer. This chapter provides an overview of the current knowledge, which is based on only a few publications.
Table 2 contains a list of 164 volatile organic compounds detected in emissions from the leaf litter of 30 plants, including 25 arboreal species. The largest group is made up of hydrocarbons (79 compounds), including mono- and sesquiterpenes (63 compounds), which are more active in atmospheric processes. Isoprene, ethanol, acetaldehyde, acetone and methanol belong to the more frequently detected litter-derived components. With the exception of isoprene, these short-chain volatiles have relatively long atmospheric lifetimes and reach concentrations in the parts per billion range.
Table 2Organic compounds in volatile emissions of leaf litter. Plant species: 1 – oriental beech (Fagus orientalis); 2 – sequoia (Sequoiadendron giganteum); 3 – cherry prinsepia (Prinsepia sinenis); 4 – aspen (Populus tremula); 5 – English oak (Quercus robur); 6 – maple (Acer rubrum); 7 – pine (Pinus taeda); 8 – poplar (Populus balsamifera); 9 – birch (Betula pendula); 10 – willow (Salix sp.); 11 – common beech (Fagus sylvatica); 12 – broad beech fern (Phegopteris hexagonoptera); 13 – northern red oak (Q. rubra); 14 – bur oak (Q. macrocarpa); 15 – lodgepole pine (P. contorta); 16 - ponderosa pine (P. ponderosa); 17 – eastern cottonwood (Populus deltoides); 18 – quaking aspen (Populus tremuloides); 19 – green ash (Fraxinus pennsylvanica); 20 – great laurel (Rhododendron maximum); 21 – eucalyptus (Eucalyptus sp.); 22 – spotted knapweed (Centaurea maculosa); 23 – miscanth (Miscanthus sp.); 24 – wheatgrass (Thinopyrum intermedium); 25 – Scots pine (P. sylvestris); 26 – Norway spruce (Picea abies); 27 – European larch (Larix decidua); 28 - clover (Trifolium repens); 29 – bluegrass (Poa pratensis); 30 – European silver fir (Abies alba). References: a – Derendorp et al. (2011a, b); b – Isidorov and Jdanova (2002); c – Ramirez et al. (2010); d – Warneke et al. (1999); e – Fall et al. (2001); f – Gray et al. (2010); h – Isidorov et al. (2003); i – Isidorov et al. (2010); j – Isidorov et al. (2005); k – Kesselmeier and Hubert (2002).

It is plausible that α-pinene is widespread within litter-derived VOCs; however, the accurate identification of this important monoterpene is impossible in certain cases. In some of the investigations cited in Table 2, instead of “traditional” GC-MS analyses, a relatively novel and, in many aspects, convenient proton transfer reaction mass spectrometry (PTR-MS) technique was used (Gray et al., 2010; Ramirez et al., 2010; Stoy et al., 2021). This method has the undoubted advantage of being a tool for the simultaneous online registration of VOC emissions. However, PTR-MS has limited identification possibilities as only a single protonated ion, with a molecular weight corresponding to that of the neutral analyte molecule plus 1 amu, [M+1]+, is used as the sole analytical parameter. This hinders the precise identification of isomers and the distinguishing of isobaric compounds, for example C10H16 monoterpenes and the numerous aromatic C9H12O compounds, nor-terpenoids, and furans with a molecular weight of 136 amu. For this reason, in some cases, the identification of monoterpenes (Gray et al., 2010; Ramirez et al., 2010; Greenberg et al., 2012) or isomeric pentenols, methyl butanols and pentenals (Fall et al., 2001) has to be recognised as tentative. The speciation (i.e. determination of the exact chemical structure) of VOCs is highly important for atmospheric chemistry because even closely related compounds differ in their reactivity (Atkinson and Arey, 2003). In recent years, a more advanced version of the method, based on the use of time-of-flight mass spectrometer reaction of proton transfer (PTR-ToF-MS), has become more frequently used, which makes it possible to determine the mass of a registered ion with an accuracy of 0.01 amu (Viros et al., 2020; Crocker 2021). This significantly increased the identification capability of the method; however, the much more time-consuming and work-intensive GC-MS method (which uses two independent analytical parameters for identification: full mass spectrum and chromatographic retention index) maintains its significance.
Another component of forest floor cover worthy of attention as a source of atmospheric VOCs is non-arboreal vegetation. Small shrubs, grasses, lichens and mosses considerably contribute to the overall green biomass of many types of Northern Hemisphere forests (Isidorov, 1994; Isidorov et al., 1994; Jagodzinski et al., 2016) and play an important role in the photosynthetic production of boreal forests (Kolari et a., 2006). These plants are adapted to low photosynthetically active radiation (PAR) and begin photosynthesis as early as at a PAR level above 3 µmol m−2 s−1 (Morén and Lindroth, 2000). In boreal forests, different feather moss, peat moss and foliose lichen species often form a continuous layer on the forest floor (Hageman and Moroni, 2015). Moreover, a significant fraction of the whole Earth's land surface is dominated by bryophytes (Lindo and Gonzalez, 2010). Hence, mosses, lichens and other forest floor plants can make up a principal part of total green biomass and VOC emissions in forests, at least in subarctic and temperate regions of the Northern Hemisphere (Isidorov, 1992; Isidorov et al., 1999; Mäki et al., 2017).
According to investigations performed in northern European Russia, the LSC biomass in 20-year-old pine forests is (on average) 1200–2500 t km−2, increasing to 7600–8100 t km−2 in mature forests; for the same time interval, pine needle biomass decreased from 5600–6700 t km−2 to 3200–4700 t km−2 (Zyabchenko, 1984). The LSC composition of young (20–40-year-old) pine forests in northern Russia is, as a rule, dominated by grasses and ferns (Melampyrum sylvaticum, M. pratense, Maianthemum bifolium, Avenella flexuosa, Dryopteris filix-mas, etc.) and small shrubs (Vaccinium vitis-idaea, V. myrtillus, Calluna vulgaris, Ledum palustre, Empetrum nigrum, Erica sp., etc.), but in the oldest forests, they are replaced by green mosses and lichens (Pleurozium schreberi, Polytrichum commune, Lycopodium clavatum, Cetraria islandica, Sphagnum sp., etc.). The share of LSC in the current accretion of biomass in pine forests reaches 30 % in north taiga and 50 % in middle taiga zones (Zyabchenko, 1984). On a level with arboreal vegetation, LSC forms a litter mass which can reach 1.8–2.3 t ha−1. In spite of its importance as a component of forest biomass, LSC has not been studied sufficiently as a source of atmospheric VOCs. Table 3 presents a list of volatile organics registered in gas emissions from a limited number of these plants. It contains 206 C2–C19 compounds belonging to different classes. The compounds listed in the table are divided into 11 groups, the most numerous of which are monoterpenoids (45 compounds) and sesquiterpenoids (42 compounds). These VOCs are among the compounds with the highest potential for secondary aerosol formation. Carbonyls form the third largest group, consisting of 33 aldehydes and ketones.
Table 3VOC composition of some LSC plants (forests and bog forests). Plants: 1 – lichen (Cetraria islandica); 2 – heather (Calluna vulgaris); 3 – moss (Pleurozium schreberi); 4 – club moss (Licopodium clavatum); 5 – red bilberry (Vaccinium vitis-idaea); 6 – bilberry (Vaccinium myrtillus); 7 – moss (Polytrichum commune); 8 – marsh tea (Ledum palustre); 9 – male fern (Dryopteris filix-mas); 10 – soft tree fern (Dicksonia antarctica); 11 – Japanese beech fern (Thelypteris decursive-pinnata); 12 – southern shield fern (Thelypteris kunthii); 13 – cottongrass (Eriophorum sp.); 14 – red raspberry (Rubus idaeus); 15 – Labrador tea (Rhododendron groenlandicum); 16 - bog heather (Erica tetralix); 17 – sphagnum (Sphagnum fuscum); 18 – thick stemmed wood fern (Dryopteris crassirhizoma); 19 – marsh andromeda (Andromeda polifolia); 20 – lichen (Cladonia arbuscular); 21 – moss (Dicranum polysetum); 22 – moss (Hylocomium splendens); 23 – moss (Leucobryum glaucum); 24 – moss (Sphagnum fallax); 25 – pipsissewa (Chimaphilla umbellata); 26 – European clefthoof (Asarum europaeum); 27 – catsfoot (Antennaria dioica); 28 – fern (Pteridium aquilinum). References: a – Isidorov et al. (1994); b – Isidorov (1992); c – Isidorov et al. (1985); d – Isisorov et al. (2022); e – Tingey et al. (1987); f – Drewitt et al. (1998); g – Helmig et al. (1999); h – Mochizuki et al. (2014); i – Rinnan et al. (2005).

Not only the chemical composition of leaf-litter-derived VOCs but also how it changes during litter decomposition is of great interest. Such changes have been registered in a laboratory via the use of head-space solid-phase microextraction (HS-SPME) combined with GC-MS (Isidorov et al., 2003) and since then in long-term litterbag field experiments (Isidorov et al., 2010). This study determined that during the decomposition of Scots pine (Pinus sylvestris) and Norway spruce (Picea abies) litter, substantial changes in the number of compounds emitted into the gas phase take place (Fig. 1).
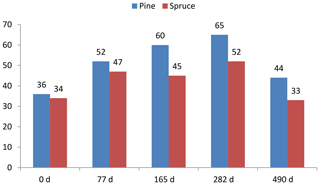
Figure 1Changes in the number of different VOCs registered in emissions from decomposing Scots pine and Norway spruce needles (Isidorov et al., 2010).
Over the entire observation period, mono- and sesquiterpene hydrocarbons were the main components of volatile emissions, but the ratio of these VOC groups varied greatly (Fig. 2). During decomposition, new compounds appeared in the composition of VOCs, mainly represented by monoterpenoids, the oxidation products of α- and β-pinene (pinocarvone, pinocampon, myrtenal, myrtenol, cis-3-pinanone, verbenone), and aliphatic alcohols, carbonyls and esters (Isidorov et al., 2010). Kainulainen and Hopolainen (2002) have also reported the emission of verbenone and verbenol in decomposing pine needle litter. Most of these “additional” compounds were either not detected in the volatiles emitted by living pine and spruce needles and in their essential oils or were present as minor and trace components. For example, substantial quantities of acetone, butan-2-one, hexan-2-one, hexan-3-one, octan-3-one and octan-4-one, as well as C2–C5 aliphatic alcohols and C5–C9 aldehydes, were only registered in volatile emissions from pine and spruce needle litter after an experimental period of 4 weeks. It has been proposed that all these volatiles are secondary products of litter-destroying microorganisms (Isidorov et al., 2003). Therefore, the observed emission of VOCs from leaf litter are the blend of volatiles stored in dead plant tissues, such as monoterpene hydrocarbons and metabolites newly enzymatically synthesised by saprotrophic microbial communities. In particular, C3–C8 carbonyl compounds, lower alcohols and their esters are the main products of the enzymatic oxidative decomposition of unsaturated aliphatic acids (Wurzenberg and Grosch, 1984).
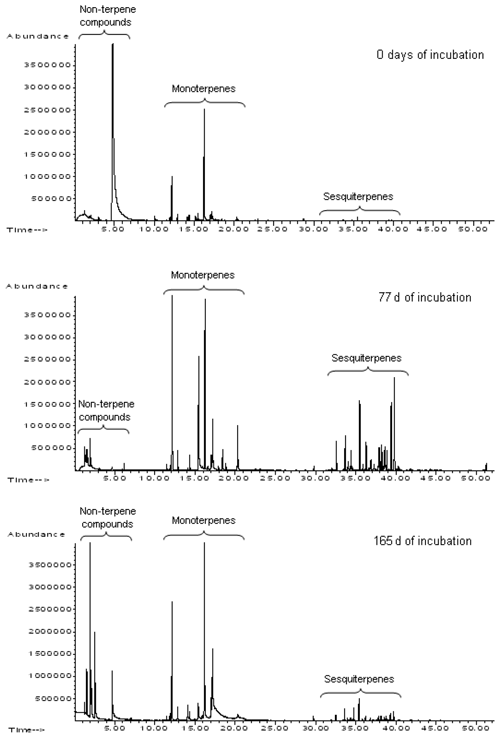
Figure 2Changes in the profiles of volatile emissions of pine litter at the first stages of decomposition in natural conditions. HS-SPME–GC-MS chromatograms from pine needle litter emissions concentrated on polydimethylsiloxane (PDMS) fibre (Isidorov et al., 2010).
It has long been known that diverse microorganisms grown on various nutritive artificial media emit a broad range of VOCs into the gas phase (Norrman, 1969; Babich and Stotzky, 1974; Sunesson et al., 1995; Nilsson et al., 1996). Although the most labile primary and secondary metabolites, such as mono- and oligosaccharides, amino acids, and other organic acids, are withdrawn by trees during leaf senescence, falling foliage still contains considerable amounts of both volatile and non-volatile but easily degradable substances that can be substrates for microorganisms (Tian et al., 2000; Isidorov et al., 2005, 2010). In Gray et al.'s (2010) study, the cell-soluble fraction of the litter from 12 plant species was in the range of 28.9 %–74.6 % of the litter carbon fraction. This material and more stable biopolymers (cellulose, hemicelluloses, lignocellulose and lignin) can be used by various bacteria and fungi. It can be assumed that these groups of microorganisms participate in the assimilation of diverse substrates during the different stages of litter destruction; moreover, the composition of microbial VOCs is species-specific. It is known that the relationship between bacteria and fungi is characterised by antagonism. In most of the fungal cultures studied to date, the biosynthesis of metabolites with bactericidal properties has been found, while many types of bacteria demonstrate fungicidal effects. These competitive relationships can manifest themselves in the progressive decomposition of litter and determine the composition of VOCs of microbial origin.
During leaf litter decomposition, microbial succession takes place, defined as a directed change in the relative abundance and spatial pattern of species comprising communities (Frankland, 1998). Undecomposed fallen leaves act as a carbon source for primary saprotrophs, belonging to bacteria and/or fungi (Bonanomi et al., 2017). After use by the primary colonisers of simple sugars, oligosaccharides, amino acids and other easy-assimilating substances, litter is naturally colonised by other microbes, fungi and cellulolytic bacteria (López-Mondéjar et al., 2016; Starke et al., 2016; Shao et al., 2017; Štursová et al., 2020; Tennakoon et al., 2021). It is believed that more recalcitrant lignified hemicelluloses and lignin are destroyed only by basidiomycetes, fungi capable of producing extracellular lignolytic enzymes (Hobbie et al., 2012; Kirker et al., 2020).
The successional nature of fungal decomposition of the herbaceous litter of the longleaf pine savanna ecosystem was noted by Lodato et al. (2021). Sequencing of fungi isolated by the authors from the litter of Schizachyrium scoparium (little bluestem or beard grass) and S. tenerum (slender bluestem) revealed the participation in the decomposition of several phylotypes, among which representatives of Ascomycota (Dothideomycetes) and Basidiomycota (Agaricomycetes) prevailed.
However, some groups of bacteria, which also have the ability to synthesise enzymes suitable for these purposes, may be involved in the decomposition of recalcitrant biopolymers. In particular, high lignolytic activity has been found in Staphylococcus saprophyticus, Pseudomonas aeruginosa and certain bacteria from the genus Bacillus (Nahrowi et al., 2018). According to Frankland (1998), fungal activity had almost ceased after 5 to 6 years with mycelial bacteria capable of degrading resistant substrates. Due to their slow growth, these microorganisms are unable to compete with non-mycelial bacteria for readily available substances, in the assimilation of which actinobacteria are actively involved (Mahajan et al., 2016). Three-year observations of litter-associated microbial organisms in northern Japan, in a pioneer forest dominated by birch and a climax forest dominated by oak, confirmed that the succession of microorganisms in the litter occurs from fungi to bacteria. In addition, in both forest types, Gram-negative bacteria were replaced by Gram-positive bacteria (Otaki and Tsuyuzaki, 2019). Noteworthy is the observation of the role of microbes associated with deciduous litter in the rotting of aboveground wood: contact with litter leads to an increase in the moisture content of the wood and its visually observed faster decomposition (Kirker et al., 2020).
A propelling power and predominant regulator of the above microbial succession is the change in litter quality and its chemical composition (Bray et al., 2012; Ge et al., 2017). Therefore, it might be expected that the successive assimilation of different substrates by different groups of microbes is accompanied by changes in the chemical composition of the microbial emission of volatiles. Unfortunately, temporal changes in microbial VOC emission profiles during litter decomposition remain uninvestigated. Recent single studies in this direction are cited below.
During the course of a 690 d litterbag experiment in decaying pine (P. sylvestris) and spruce (P. abies) needle litters, 92 fungus species were identified: 54 species in pine and 73 in spruce litter (Isidorov et al., 2016). The composition of the fungal communities was substantially specific, as only 38 % of the species were found in both litter types. At the early stage (30 d of the experiment), the typical primary colonisers from the orders of Capnodiales, Xylariales, Dothideales, Pleosporales, Eurotiales, Thelebolales and Hypocreales dominated. After 7 months, none of the primary colonisers were found in pine litter, and only two (Cladosporium herbarum and Pseudeurotium ovale) were detected in spruce needles. The observed disappearance of these fungi can be linked to the sharp decrease in simple carbohydrates in the early stages of decomposition during the decay of leaf litter (Kainulainen and Hopolainen, 2002; Osono and Takeda, 2005; Isidorov et al., 2010) due to both use by microbes and leaching.
At the middle stage (280 d), Penicillium purpurogenum dominated in pine litter and Chloridium botryoides in spruce litter, whereas the late stage (490–690 d) was characterised by the absolute predominance of Trichoderma species (T. polysporum, T. koningii and T. viride). These filamentous fungi are producers of large amounts of cellulolytic enzymes. Remarkably, in the late stage of the experiment, the recurrence of some primary colonisers was also observed, which can be explained by the action of cellulolytic fungi producing readily available sugars released from the hemicelluloses. The recurrence of the primary colonisers after approximately 350 d of Pinus pinea needle incubation was previously documented by Virzo De Santo et al. (2002). Further degradation of more recalcitrant lignified materials demands the participation of lignolytic fungi.
It can be noted that unlike the case of VOC emissions from living plants, there is little knowledge regarding such emissions from litter-destroying microbes. The composition of VOCs emitted into the gas phase by selected fungi isolated from Scots pine and Norway spruce needles was determined after 490 d of incubation in one study (Isidorov et al., 2016). Based on the results of HS-SPME–GC-MS analyses, as many as 75 C2–C15 volatile organic compounds were identified. Table 4 presents the group composition of these VOCs and the principal representatives of separate groups. As can be seen, VOC emissions are species-specific: grown on the same cultivation media, the fungi differ in the qualitative and quantitative composition of the volatiles produced. Even a representative of the same genus (for example, Trichoderma polysporum and T. koningii, or Penicillium minioluteum and P. purpurogenum) demonstrates considerable distinctions in VOC emissions. Striking differences have also been observed by Nilsson et al. (1996): only 3 of 58 registered VOCs were common for four Penicillium species.
Table 4Relative composition (% of TIC, total ion current) of volatile compound emissions from “fresh” pine and spruce litter and isolated from litter fungi after 490 d of the needle incubation.
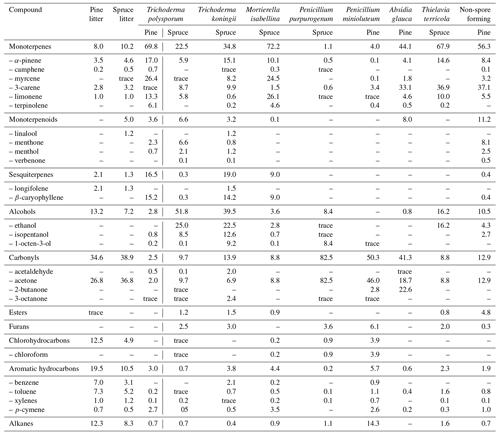
Even less is known about VOC production by litter-destroying bacteria. However, the emission of photochemical significant organic volatiles, including isoprene, by bacilli and actinomycetes was discovered (Kuzma et al., 1995; Fall and Copley, 2000; Wilkins, 1996; Wagner et al., 2000; Schöller et al., 2002). At present, the important role of bacteria in leaf litter and coarse woody debris destruction is well documented (Kim et al., 2014; Urbanová et al., 2015; López-Mondéjar et al., 2016; Mahajan et al., 2016; Purahong et al., 2016; Bonanomi et al., 2017; Dolan et al., 2017; Hu et al., 2017). However, further studies are required to understand their role in the litter-derived emission of VOCs into the atmosphere.
In terrestrial environments, algae represent the main component of the microbial flora. Forest litter is also the place of the active development of microscopic algae, which form a specific element of the living soil cover (Maltsev et al., 2017a, b). They participate in all biogenic processes in leaf litter, together with other organisms. However, data on the algae of leaf litter are scanty. Maltsev et al. (2017a) have recently studied the structural and dynamic parameters of the algal communities of the anthropogenic and natural forests in the steppe zone of Ukraine and found that the composition of communities varies by season and forest-forming tree species. The authors identified 119 algae species belonging to six divisions: Chlorophyta (68 species), Xanthophyta (21 species), Cyanoprocaryota (17 species), Bacillariophyta (7 species), Eustigmatophyta and Charophyta (3 species each). With respect to species number and abundance, coniferous forest litter was predominated by green (Chlorophyta) and yellow-green (Xanthophyta) algae, whereas leaf forest litter (Q. robur and Robinia pseudoacacia plantations) was characterised by the significant participation of oxygenic photosynthesising cyanobacteria Cyanoprocaryota. In the available literature, we were unable to find information on the emission of VOCs by this group of photosynthetic organisms of the soil cover.
In the past three and a half decades, many measurements of VOC emission rates have been carried out both for the living foliage of individual plants and for various plant communities. In particular, in a review covering the period from 1979 to 1997, 314 values of the emission rates of isoprene and monoterpenes published in 52 articles are given. Another 126 values of the emission rates of 39 non-terpene compounds from more than 50 plant species were published from 1992 to 1999 (Kesselmeier and Staudt, 1999). Among the plants listed in this review, only one species from the genus Ericaceae, which is part of the living soil cover of boreal forests, is mentioned: blueberry (Vaccinium uliginosum). This shrub is one of the weak sources of isoprene (emission rate 0.009 µg g−1 h−1). However, two other species of shrub from the same genus – cassandra (Chamaedaphne calyculata) and Labrador tea (Ledum groenlandicum) – are strong monoterpene emitters, with a release rate of more than 3 µgC g−1 h−1 (Isebrands et al., 1999).
Also limited are data in the literature on rates of VOC emissions from forest leaf litter in natural environments or even laboratory experiments. However, the few observations made confirm the importance of the contribution of the forest floor to total VOC emissions with regard to some forest types (Petersson, 1988; Janson, 1993; Isidorov et al., 1994; Stepanov, 1999; Hayward et al., 2001). In addition to these early studies, several investigations have been carried out in recent years as part of the SMEAR II (Station for Measuring Relationships between Forest Ecosystems and the Atmosphere) research programme (Hari and Kulmala, 2005).
The information presented in Table 5 is remarkable in that almost all measurements were carried out in boreal coniferous forests or in mixed forests with a predominance of conifers. Meanwhile, there is no reason to believe that the decomposition of litter of small- or broad-leaved species prevailing in the more southern forests contributes less to the total emissions of biogenic VOCs than the northern, predominantly coniferous forests. However, we were unable to find any publications on VOC emissions from deciduous forests. Besides, plants of live soil cover were considered as a source of VOCs, along with litter, only in some studies (Mäki et al., 2017, 2019a, b). It is also noteworthy that all but two studies were conducted in forests of the Eastern Hemisphere (Hayward et al., 2001; Greenberg et al., 2012).
Although this review focuses on the problems associated with VOC emissions from the forest floor of the boreal and temperate zones of the Northern Hemisphere, Table 5 provides the only information we know of these processes in tropical forests (Drewer et al., 2021). The 2-year exploration was carried out in a logged tropical forest and an oil palm plantation in Malaysia on the island of Borneo. The amount of litter on the soil determined the level of monoterpene fluxes, of which the main ones, as in the case of the floor of northern forests, were α- and β-pinene, as well as limonene (all other measured monoterpenoids, such as 3-carene, camphene and eucalyptol, were emitted at lower rates). Fluxes from oilseed plantations, which were practically devoid of litter, were low and increased only where litter was present.
The results of the field experiments carried out to date and their conclusions can be contradictory even within the same working group. For instance, Janson (1993) recorded a fairly high rate (up to 580 µgC m−2 h−1) of terpene emission by litter in a mature pine forest in southern Sweden, with significantly higher values in autumn than in summer. According to the author's estimate based on these measurements, the flux of terpenes from the pine forest litter accounted for 30 % of the crown emissions. However, subsequent measurements (Janson et al., 1999) in coniferous forests and wetlands in summer and early autumn yielded considerably lower emission rates of monoterpenes (19–90 µg C m−2 h−1); it was concluded that the flux of biogenic VOCs from the forest floor only accounts for a few percentage points of the total forest flow. According to data obtained at the same time in western Russia (Stepanov, 1999), the emission rates of monoterpenes by spruce litter were higher (21–402 µg m−2 h−1), and the highest values were recorded not in July–August at air temperatures of 22–25 ∘C but in the second half of September at temperatures of 6–8 ∘C. In both summer and autumn, temporarily increased emission levels were observed immediately after rain events. The strong effect of rain on VOC emissions from litter has been confirmed in a recent study (Crocker, 2021). In the author's opinion, a short-term increase in emission by leaf litter of Clitoria fairchildiana after its moistening occurs in accordance with Henry's law. The concentration profile of monoterpenes, measured by Stepanov (1999) under the canopy of an 80-year-old spruce forest in summer in calm weather, showed the presence of two maxima, one of which was located approximately at half the height of the crown tree and the other near the surface covered with coniferous litter at a height of 0.8 m.
In another study, monoterpene emissions from the boreal pine forest floor in Finland varied from 0 to 373 µg m−2 h−1 (Hellén et al., 2006); that is, its upper value was consistent with that obtained by Stepanov (1999). According to the authors, emissions of terpenes from the forest floor can be a significant source for the atmosphere, especially in spring.
Kivimäenpää et al. (2018) conducted a latitudinal experiment in Finland, involving a 1000 km north–south transect, and concluded that emissions from the floor of pine forests have a noticeable additive effect on total VOC emissions. The authors of an earlier study (Räisänen et al., 2009), who measured the fluxes of monoterpenes in a pine forest in eastern Finland, were inclined to the same conclusion. According to their estimates, the total flux of monoterpenes in June-September was 502 mg m−2, whereas the flux from the forest canopy was only 374 mg m−2. According to the authors, the difference of more than 25 % can be partially explained by the emission of terpenes from the forest floor.
The results of recent measurements – carried out mainly by Scandinavian researchers (Mäki et al., 2017, 2019a, b; Kivimäenpää et al., 2018; Wang et al., 2018) – and their conclusions about the important role of VOC emissions from forest litter (primarily photochemically active terpenes and sesquiterpenes with high potential for secondary aerosol formation) are at odds with the earlier conclusion (Janson et al., 1999; Greenberg et al., 2012) that they only slightly contribute to the total ecosystem flux of VOCs. To clarify the nature of such discrepancies, it is necessary to continue research with a wider coverage of geographical and climatic conditions and, first of all, to include forests formed by deciduous trees in the research programme.
Table 5 also includes chamber measurements of mono- and sesquiterpene fluxes from wet scrubland in northern Sweden, including an experiment that adds birch litter to the chambers (Faubert et al., 2010). The relatively low emissions of terpenoids were doubled in response to an air temperature increment of only 1.9–2.5 ∘C. This suggests that the predicted warming of the climate in the subarctic and arctic regions is likely to lead to a significant increase in the flux of reactive VOCs from plants of the living ground cover of the tundra. The same conclusion was reached by the authors who studied the release of terpenes by plants of the living soil cover of the high and low Arctic heaths and subarctic peatland (Lindwall et al., 2015).
In addition to these field observations, the results of the small number of laboratory measurements of the rates of VOC release by the litter of some species of woody and shrub plants have been published. For example, the rates of terpene emission by fresh litter of European larch, as well as fresh and decaying litter of Norway spruce (P. abies) and Scots pine (P. sylvestris), have been reported (Table 6). At the early stages of decomposition (2.5–5.5 months from the beginning of the experiment), the rates of terpene emission from pine and spruce litter exceeded those observed for freshly fallen needles. Over the entire observation period, in the case of spruce litter, camphene and α-pinene were released into the gas phase at the highest rates, whereas in the case of pine litter, the main VOCs were α-pinene and 3-carene.
Table 6Laboratory measurements of emission rates of monoterpene hydrocarbons from fresh and decaying litter of Norway spruce and Scots pine (Isidorov et al., 2010), as well as from fresh litter of European larch, Larix decidua (Isidorov et al., 2005).
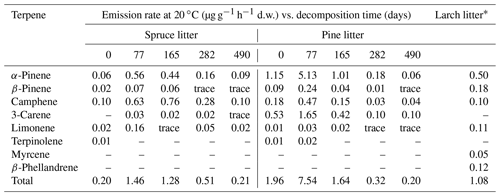
* Emission at 22 ∘C.
In a recent study, the results of laboratory measurements of the rates of VOC release by fresh (not decomposed) deciduous litter of 16 species of woody and shrub plants typical of the Mediterranean region are presented (Viros et al., 2020). These species are divided by the authors into two groups depending on the presence of terpene storage structures. A total of 87 VOCs were identified in the isolations of all the examined plants by GC-MS. The emission rates of various VOCs at a temperature of 30 ∘C ranged greatly. In the case of nine plants of the terpene-storing species (Eucalyptus globulus, Juniperus oxycedrus, Pinus pinea, P. halepensis, Rosmarinus officinalis, Thymus vulgaris, Cistus albidus, C. salviifolius, Cotinus coggygria), the total emissions of monoterpenes, sesquiterpenes and non-terpene compounds were in the range of <0.01–4.62, 0.04–0.92 and <0.01–0.23 µg g−1 h−1, respectively (Table 7). Emissions of seven non-terpene-storing species via litter (Acer monspessulanum, Erica arborea, Quercus ilex, Q. coccifera, Q. pubescens, Q. suber, Ulex parviflorus) consisted mainly of linear alkanes and carbonyl compounds and ranged from 0.01 to 0.61 µg g−1 h−1 (Table 8).
Table 7Emission rates of volatile organic compounds (ng g−1 h−1) from litter of nine terpene-storing species. Values are mean ± SE (n=3 to 10) (Viros et al., 2020).
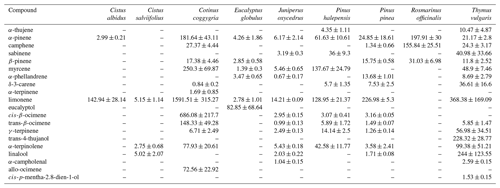
Table 8Emission rates of compounds (ng g−1 h−1) from litter of seven non-terpene-storing species. Values are mean ± SE (n= 3 to 10) (Viros et al., 2020).
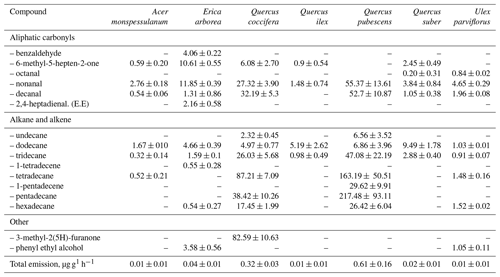
A 15-month litterbag experiment was conducted to study the composition of VOCs released by the decaying pine needles of Pinus halepensis, one of the main coniferous trees in the Mediterranean (Viros et al., 2021). Material for laboratory studies was sampled every 3 months, and VOC emissions were determined both online (using the PTR-ToF-MS instrument) and offline (GC-MS). During the observation period, 58 compounds were registered in the composition of the fallen needle excretions. The maximum emission of 9.18 µg g−1 h−1 was observed 3 months after the beginning of the experiment, with the main components being α-pinene, terpinolene, 3-carene, limonene, sabinene and myrcene, whereas the main sesquiterpenes were β-caryophyllene, α-humulene and copaene. In addition, at this stage, the emission of highly volatile non-terpene compounds took place, with the main ones being methanol, acetic acid and acetone. The maximum emission of oxidised monoterpenes and sesquiterpenes occurred between the third and sixth months of the experiment. Comparison of data on VOC emissions from green and decaying fallen needles of P. halepensis led the authors of the cited work to the conclusion that the contribution of litter to the total emission in forests from this pine species (their area in the western Mediterranean is about 3.5 × 106 ha) can be extremely high.
The data presented in this section indicate that VOC emissions from the forest floor are not negligible. Moreover, during the spring and autumn seasons, the forest floor can be the main source of these reactive components. However, to establish its effect on air quality, additional studies are needed to determine the emission factors for different ecosystems and biomes.
It is recognised that a critical challenge for atmospheric chemistry is closing the atmospheric organic carbon budget. This review shows that deciduous litter and plants of living forest soil cover are an unaccounted source of large amounts of VOCs, for many of which a high potential for the formation of ozone and other atmospheric photooxidants and/or secondary aerosols has been postulated. The results of many studies indicate that these elements of forest ecosystems are not negligible sources of atmospheric VOCs, especially in spring and autumn. Based on their origin, they are a mixture of a variable composition of products released as a result of various insufficiently studied physical and biological processes.
There are serious knowledge gaps that prevent a quantitative assessment of the role of VOC emissions from the forest floor in biospheric processes. These include the lack of information on the composition and emission rates of litter VOCs in various forest types, primarily those formed by deciduous tree species: the overwhelming majority of the measurements were made in the coniferous forests of the northern belt. This applies to an even greater extent to the dead parts of herbaceous plants of steppe and related ecosystems, although the biomass of grass felt per unit area in them can significantly exceed that of deciduous litter in forests. The participation of soil cover plants in the balance of these compounds has hardly been studied, and it is unclear whether they serve as sources of VOCs or as their absorbers (Mäki et al., 2017; Stoy et al., 2021). In some pioneering works, an important influence on the rate of decomposition of litter of solar radiation, including in the visible part of the spectrum, has been shown (Austin et al., 2016; Keiser et al., 2021). However, the chemical composition of the resulting compounds, both volatile and easily accessible for microbiological assimilation, has not been studied. Insufficient research has focused on the impact of global changes on litter decomposition and VOC emissions by ecosystems. There is also a lack of data on both litter and plants of the living ground cover of forests in different geographic zones necessary for an inventory of VOC emissions on a regional and global scale.
The study of the decomposition of leaf litter, as well as the chemical composition and emission rates of volatile organic compounds, is of interest not only from the point of view of atmospheric chemistry. Biogenic VOCs are involved in competitive interactions as both allelochemicals and as neighbour detection signals (Kegge and Pierik, 2009). Volatile organic compounds from leaf litter decomposition alter soil microbial communities and carbon dynamics (McBride et al., 2020). However, it should be noted that this is not the only process linking deciduous litter and soil biota. When litter decomposes, both labile and more stable forms of dissolved organic matter are formed, significantly affecting the binding and consumption of carbon in the soil (Hensgens et al., 2021); however, the chemical composition of compounds leached from the litter and the very scale of this phenomenon remain unexplored.
This review is limited to boreal and temperate natural ecosystems of the Northern Hemisphere because of the almost complete absence of literature data on VOC emissions from forest soil cover from other botanical-geographical zones. In particular, only a few works are devoted to VOC emissions in subtropical and tropical forests (Drewer et al., 2021; Crocker, 2021). We would like our review to serve as a stimulus for expanding the geography of research and for a deeper study of the role of both “dead” and living forest soil cover in the release of organic compounds into the environment, which play an important role in many ecological processes under the forest canopy and in the global atmosphere.
All research data presented in this review are published in the cited literature and are publicly available.
The supplement related to this article is available online at: https://doi.org/10.5194/bg-19-4715-2022-supplement.
Both authors contributed equally to the preparation and writing of the article.
The contact author has declared that neither of the authors has any competing interests.
Publisher's note: Copernicus Publications remains neutral with regard to jurisdictional claims in published maps and institutional affiliations.
This research has been supported by the Narodowe Centrum Nauki (grant no. 2019/35/B/ST10/02252).
This paper was edited by Paul Stoy and reviewed by Arnaud P. Praplan and two anonymous referees.
Aaltonen, H., Pumpanen, J., Pihlatie, M., Hakola, H., Helén, H., Kulmala, L., Vesala, T., and Bäk, J.: Boreal pine forest floor biogenic volatile organic compound emissions peak in early summer and autumn, Agr. Forest Meteorol., 151, 682–691, https://doi.org/10.1016/j.agrformet.2010.12.010, 2011.
Archibald, A. T., Neu, J. L., Elshorbany, Y. F., Cooper, O. R., Young, P. J., Akiyoshi, H., Cox, R. A., Coyle, M., Derwent, R. G., Deushi, M., Finco, A., Frost, G. J., Galbally, I. E., Gerosa, G., Granier, C., Griffiths, P. T., Hossaini, R., Hu, L., Jöckel, P., Josse, B., Lin, M. Y., Mertens, M., Morgenstern, O., Naja, M., Naik, V., Oltmans, S., Plummer, D. A., Revell, L. E., Saiz-Lopez, A., Saxena, P., Shin, Y. M., Shahid, I., Shallcross, D., Tilmes, S., Trickl, T., Wallington, T. J., Wang, T., Worden, H. M., and Zeng, G.: Tropospheric Ozone Assessment Report: A critical review of changes in the tropospheric ozone burden and budget from 1850 to 2100, Elementa-Science for Anthropocene, 8, 034, https://doi.org/10.1525/elementa.2020.034, 2020.
Asensio, D., Peñuelas, J., Ogaya, R., and Llusià, J.: Seasonal soil VOC exchange rates in a Mediterranean holm oak forest and their responses to drought conditions, Atmos. Environ., 41, 2456–2466, https://doi.org/10.1016/j.atmosenv.2006.05.007, 2007.
Atkinson, R. and Arey, J.: Gas-phase tropospheric chemistry of biogenic volatile organic compounds: A review, Atmos. Environ., 31, 197–219, https://doi.org/10.1016/S1352-2310(03)00391-1, 2003.
Austin, A. T., Mendez, M. S., and Ballaré, C. L.: Photodegradation alleviates the lignin bottelneck for carbon turnover in terrestrial ecosystems, P. Natl. Acad. Sci. USA, 113, 4392–4397, https://doi.org/10.1073/pnas.1516157113, 2016.
Babich, H. and Stotzky, G.: Air pollution and microbial ecology, CRC Crit. Rev. Microbiol., 4, 353–421, https://doi.org/10.1080/10643387409381619, 1974.
Bazilevich, N. I., Drozdov, A. V., and Zlotin, R. I.: Geographical peculiarities of the production-destruction-processes in Northern Eurasia, in: Geography of organic matter production and decay, SCOPE Conf. Pap. 18., Szymbark, 11–18 September 1991, Warszaw, 51–79, 1993.
Bell, M. and Ellis, H.: Sensitivity analysis of tropospheric ozone to modified biogenic emissions for the Mid–Atlantic region, Atmos. Environ., 38, 1879–1889, https://doi.org/10.1016/j.atmosenv.2004.01.012, 2004.
Berg, B.: Decomposition patterns for foliar litter – A theory for influencing factors, Soil Biol. Biochem., 78, 222–232, https://doi.org/10.1016/j.soilbio.2014.08.005, 2014.
Bolin, B.: The carbon cycle, in: SCOPE 21 – The Major Biogeochemical Cycles and Their Interactions, edited by: Bolin, B. and Cook, R. B., Wiley, Chichester, https://doi.org/10.1016/0016-7037(84)90418-6, 1983.
Bonanomi, G., Cesarano, G., Lombardi, N., Motti, R., Scala, F., Mazzoleni, S., and Incerti, G.: Litter chemistry explains contrasting feeding preferences of bacteria, fungi, and higher plants, Sci. Rep., 7, 9208, 1–13, https://doi.org/10.1038/s41598-017-09145-w, 2017.
Bornman, J. F., Barnes, P. W., Robinso, S. A., Ballaré, C. L., Flint, S. D., and Caldwell, M. M.: Solar ultraviolet radiation and ozone depletion-driven climate change: effects on terrestrial ecosystems, Photochem. Photobiol. Sci., 14, 88–107, https://doi.org/10.1039/C4PP90034K, 2015.
Bosco, T., Bertiller, M. B., and Carrera A. L.: Combined effects of litter features, UV radiation, and soil water on litter decomposition in denuded areas of the arid Patagonian Monte, Plant Soil, 406, 71–82, https://doi.org/10.1007/s11104-016-2864-7, 2016.
Bray, S. R., Kitajima, K., and Mack, M. C.: Temporal dynamics of microbial communities on decomposing leaf litter of 10 plant species in relation to decomposition rate, Soil Biol. Biochem., 49, 30–37, https://doi.org/10.1016/j.soilbio.2012.02.009, 2012.
Chuwah, C., van Noije, T., van Vuuren, D. P., Stehfest, E., and Hazeleger, W.: Global impact of surface ozone changes on crop yields and land use, Atmos. Environ., 49, 11–13, https://doi.org/10.1016/j.atmosenv.2015.01.062, 2015.
Coulis, M., Hättenschwiler, F. N., and David, J. F.: Macroarthropod–microorganism interaction during the decomposition of Mediterranean shrub litter at different moisture levels, Soil Biol. Biochem. 64, 114–121, https://doi.org/10.1016/j.soilbio.2013.04.012, 2013.
Cox, P., Wilkinson, S. P., and Anderson, J. M.: Effects of fungal inocula on the decomposition of lignin and structural polysaccharides in Pinus sylvestris litter, Biol. Fertil. Soils, 33, 246–251, https://doi.org/10.1007/s003740000315, 2001.
Crocker, L. N.: The Composition and Diversity of Volatile Organic Compounds (VOCs) from Leaf Litter in the Biosphere 2 Tropical Rainforest, The University of Arizona, ProQuest Dissertations Publishing, 28870239, https://repository.arizona.edu/handle/10150/663219 (last access: 29 September 2022), 2021.
Curci, G., Beekmann, M., Vautard, R., Smiatek, G., Steinbrecher, R., Theloke, J., and Friedrich, R.: Modelling study of the impact of isoprene and terpene biogenic emissions on European ozone levels, Atmos. Environ., 43, 1444–1455, https://doi.org/10.1016/j.atmosenv.2008.02.070, 2009.
David, J. F.: The role of litter-feeding macroarthropods in decomposition process: A reappraisal common views, Soil Biol. Biochem., 76, 109–118, https://doi.org/10.1016/J. SOILBIO.2014.05.009, 2014.
De Marco, A., Proietti, C., Anav, A., Ciancarella, L., D'Elia, I., Fares, S., Fornasier, M. F., Fusaro, L., Gualtieri, M., Manes, F., Marchetto, A., Mircea, M., Paoletti, E., Piersanti, A., Rogora, M., Salvati, L., Salvatori, E., Screpanti, A., Vialetto, G., and Leonardi, C.: Impacts of air pollution on human and ecosystem health, and implications for the National Emission Ceilings Directive: Insights from Italy, Environ. Intern., 125, 320–333, https://doi.org/10.1016/j.envint.2019.01.064, 2019.
Derendorp, L., Holzinger, R., Wishkerman, A., Keppler, F., and Röckmann, T.: Methyl chloride and C2–C5 hydrocarbons emissions from dry leaf litter and their dependence on temperature, Atmos. Environ., 45, 3112–3119, https://doi.org/10.1016/j.atmosenv.2011.03.016, 2011a.
Derendorp, L., Holzinger R., and Röckmann, T.: UV induced emissions of C2–C5 hydrocarbons from leaf litter, Environ. Chem., 8, 622–611, https://doi.org/10.1071/EN11024, 2011b.
Derendorp, L., Quist, J. B., Holzinger R., and Röckmann, T.: Emissions of H2 and CO from leaf litter of Sequoidendron giganteum, and their dependence on UV radiation and temperature, Atmos. Environ., 45, 7520–7524, https://doi.org/10.1016/j.atmosenv.2011.09.044, 2011c.
Derwent, R. G., Utembe, S. R., Jenkin, M. E., and Shallcross, D. E.: Tropospheric ozone production regions and the intercontinental origins of surface ozone over Europe, Atmos. Environ., 112, 216–224, https://doi.org/10.1016/j.atmosenv.2015.04.049, 2015.
Di Carlo, P., Brune, W.H., Martinez, M., Harder, H., Lesher, R., Ren, X., Thornberry, T., Carroll, M. A., Young, V., Shepson, P. B., Riemer, D., Apel, E., and Campbell, C.: Missing OH reactivity in a forest: Evidence for unknown reactive biogenic VOCs, Science, 304, 722–725, https://doi.org/10.1126/science.1094392, 2004.
Dobrovolsky, V. V.: Elements of Biogeochemistry, Moscow, High School Publ., ISBN: 5-06-003112-8, 1998 (in Russian).
Dolan, K. L., Peña, J., Allison, S. D., and Martiny, J. B. H.: Phylogenetic conservation of substrate use specialization in leaf litter bacteria, PLoS ONE 12, e0174472, https://doi.org/10.1371/journal.pone.0174472, 2017.
Dolwick, P., Akhtar, F., Baker, K.R., Possiel, N., Simon, H., and Tonnesen, G.: Comparison of background ozone estimates over the western United States based on two separate model methodologies, Atmos. Environ., 109, 282–296, https://doi.org/10.1016/j.atmosenv.2015.01.005, 2015.
Drewer, J., Leduning, M. M., Purser, G., Cash, J. M., Sentian, J., and Skiba, U. M.: Monoterpenes from tropical forest and oil palm plantation floor in Malaysian Borneo/Sabah: emission and composition, Environ. Sci. Pollut. Res., 28, 31792–31802, https://doi.org/10.1007/s11356-021-13052-z, 2021.
Drewitt, G. B., Curren, K., Steyn, D. G., Gillespie, T. J., and Niki, H.: Measurement of biogenic hydrocarbon emissions from vegetation in the Lower Fraser Valley, British Columbia, Atmos. Environ., 32, 3457–3466, https://doi.org/10.1016/S1352-2310(98)00043-0, 1998.
Dunkerley, D.: Percolation through leaf litter: What happens during rainfall events of varying intensity?, J. Hydrol., 525, 737–746, https://doi.org/10.1016/j.jhydrol.2015.04.039, 2015.
Faiola, C. L., VanderSchelden, G. S., Wen, M., Elloy, F. C., Cobos, D. R., Watts, R. J., Jobson, B. T., and VanReken, T. M.: SOA formation potential of emissions from soil and leaf litter, Environ. Sci. Technol., 48, 938–946, https://doi.org/10.1021/es4040045, 2014.
Fall, R., and Copley, S. D.: Bacterial sources and sinks of isoprene, a reactive atmospheric hydrocarbon, Environ. Microbiol., 2, 123–130, https://doi.org/10.1046/j.1462-2920.2000.00095.x, 2000.
Fall, R., Karl, T., Jordan, A., and Lindinger, W.: Biogenic C5 VOCs: release from leaves after freeze–thaw wounding and occurrence in air at a high mountain observatory, Atmos. Environ., 35, 3905–3916, https://doi.org/10.1016/S1352-2310(01)00141-8, 2001.
Faubert, P., Tiiva, P., Rinnan, Å., Michelsen, A., Holopainen, J. K., and Rinnan, R.: Doubled volatile organic compound emissions from subarctic tundra under simulated climate warming, New Phytol., 187, 199–208, https://doi.org/10.1111/J.1469-8137.2010.03270.X, 2010.
Fehsenfeld, F. C., Calvert, J., Fall, R., Goldan, P., Guenther, A. B., Hewitt, C. N., Lamb, B., Liu, S., Trainer, M., Westberg, H., and Zimmerman, P.: Emissions of volatile organic compounds from vegetation and the implications for atmospheric chemistry, Glob. Biogeochem. Cy., 6, 398–420, https://doi.org/10.1029/92GB02125, 1992.
Feng, Z., Hu, E., Wang, X., Jiang, L., and Liu, X.: Ground–level O3 pollution and its impacts on food crops in China: A review, Atmos. Environ., 199, 42–48, https://doi.org/10.1016/j.envpol.2015.01.016, 2015.
Fioretto, A., Papa, S., Pellegrino, A., and Fuggi, A.: Decomposition dynamics of Myrtus communis and Quercus ilex leaf litter: Mass loss, microbial activity and quality change, Appl. Soil Ecol., 36, 32–40, https://doi.org/10.1016/j.apsoil.2006.11.006, 2007.
Frankland, J. C.: Fungal succession – unraveling the unpredictable, Mycol. Res., 102, 1–15, https://doi.org/10.1017/S0953756297005364, 1998.
Frouz, J., Roubíčková, A., Hedĕnec, P., and Tajovský, K.: Do soil fauna really hasten litter decomposition? A meta-analysis of enclosure studies, Eur. J. Soil Biol., 68, 18–24, https://doi.org/10.1016/j.ejsobi.2015.03.002, 2015.
Fukuj, Y. and Doskey, P. V.: Air–surface exchange of non-methane organic compounds as a grassland site: seasonal variations and stressed emissions, J. Geophys. Res., 103, 13153–13168, https://doi.org/10.1029/98JD00924, 1998.
Gaudel, A., Ancellet, G., and Godin–Beekmann, S.: Analysis of 20 years of tropospheric ozone vertical profiles by lidar and ECC at Observatoire dr Haute Provence (OHP) at 44∘ N, 6.7∘ E, Atmos. Environ., 113, 78–89, https://doi.org/10.1016/j.atmosenv.2015.04.028, 2015.
Ge, J., Xie, Z., Xu, W., and Zhao, Ch.: Controls over leaf litter decomposition in a mixed evergreen and deciduous broad-leaved forests, Central China, Plant Soil, 412, 345–355, https://doi.org/10.1007/s11104-016-3077-9, 2017.
Gray, C. M., Monson, R. K., and Fierer, N.: Emission of volatile organic compounds during the decomposition of plant litter, J. Geophys. Res., 115, G03015, https://doi.org/10.1029/2010JG001291, 2010.
Greenberg, J. P., Asensio, D., Turnipseed, A., Guenther, A. B., Karl, T., and Gochin, D.: Contribution of leaf and needle litter to whole ecosystem BVOC fluxes, Atmos. Environ., 59, 302–311, https://doi.org/10.1016/j.atmosenv.2012.04.038, 2012.
Guenther, A., Hewitt, C. N., Erickson, D., Fall, R., Geron, C., Graedel, T., Harley, P., Klinger, L., Lerdau, M., McKay, W. A., Pierce, T., Scholes, B., Steinbrecher, R., Tallamrajan, R., Tailor, J., and Zimmerman, P. R.: A global model of natural volatile organic compounds emission, J. Geophys. Res., 100, 8873–8892, https://doi.org/10.1029/94JD02950, 1995.
Guenther, A., Karl, T., Harley, P., Wiedinmyer, C., Palmer, P. I., and Geron, C.: Estimates of global terrestrial isoprene emissions using MEGAN (Model of Emissions of Gases and Aerosols from Nature), Atmos. Chem. Phys., 6, 3181–3210, https://doi.org/10.5194/acp-6-3181-2006, 2006.
Hageman, U. and Moroni, M. T.: Moss and lichen decomposition in old-growth and harvested high-boreal forests estimated using the litterbag and minicontainer methods, Soil Biol. Biochem., 87, 10–24, https://doi.org/10.1016/j.soilbio.2015.04.002, 2015.
Hari, P. and Kulmala, M.: Station for Measuring Ecosystem-Atmosphere Relations (SMEAR II), Boreal Environ. Res., 10, 315–322, 2005.
Hassall, M., Turner, J. G., and Rands, M. R. W.: Effects of terrestrial isopods on the decomposition of woodland leaf litter, Oecologia, 72, 597–604, https://doi.org/10.1007/BF00378988, 1987.
Hayward, S., Muncey, R. J., James, A. E., Halsall, C. J., and Hewitt, C. N.: Monoterpene emissions from soil in Sitka spruce forest, Atmos. Environ., 35, 4081–4087, https://doi.org/10.1016/S1352-2310(01)00213-8, 2001.
Hellén, H., Hakola, H., Pystynen, K.-H., Rinne, J., and Haapanala, S.: C2-C10 hydrocarbon emissions from a boreal wetland and forest floor, Biogeosciences, 3, 167–174, https://doi.org/10.5194/bg-3-167-2006, 2006.
Hellén, H., Praplan, A. P., Tykkä, T., Helin, A., Schallhart, S., Schiestl-Aalto, P. P., Bäck, J., and Hakola, H.: Sesquiterpenes and oxygenated sesquiterpenes dominate the VOC (C5–C20) emissions of downy birches, Atmos. Chem. Phys., 21, 8045–8066, https://doi.org/10.5194/acp-21-8045-2021, 2021.
Helmig, D., Klinger, L. F., Guenther, A., Vierling, L., Geron, Ch., and Zimmerman, P.: Biogenic volatile organic compound emissions (BVOCs). I. Identifications from three continental sites in the U.S., Chemosphere, 38, 2163–2187, https://doi.org/10.1016/S0045-6535(98)00425-1, 1999.
Hensgens, G., Lechtenfeld, O. J., Guillemette, F., Laudon, H., and Berggren, M.: Impacts of litter decay on organic leachate composition and reactivity, Biogeochemistry, 154, 99–117, https://doi.org/10.1007/s10533-021-00799-3, 2021.
Hewins D. B., Lee, H., Barnes, P. W., McDowell, N. G., Pockman, W. T., Rahn, T., and Throop, H. L.: Early exposure to UV radiation overshadowed by precipitation and litter quality as drivers of decomposition in the northern Chihuahuan Desert, PLOS ONE, 14, e0210470, https://doi.org/10.1371/journal.pone.0210470, 2019.
Hobbie, S. E., Eddy, W. C., Buyarsku, C. R., Carol Adair, E., Ogdahl, M. L., and Weisenhorn, P.: Response of decomposing litter and its microbial community to multiple forms of nitrogen enrichment, Ecol. Monogr., 82, 389–405, https://doi.org/10.1890/11-1600.1, 2012.
Hu, Z., Xu, C., McDowell, N. G., Jonson, D. J., Wang, M., Luo, Y., Zhou, X., and Huang, Z.: Linking microbial community composition to C loss rates during wood decomposition, Soil Biol. Biochem., 104, 108–116, https://doi.org/10.1016/j.soilbio.2016.10.017, 2017.
Ioffe, B. V., Isidorov, V. A., and Zenkevich, I. G.: Gas chromatographic–mass spectrometric determination of volatile organic compounds in an urban atmosphere, J. Chromatogr., 142, 787–795, https://doi.org/10.1016/S0021-9673(01)92086-2, 1977.
IPCC: Climate Change 2013: the Physical Science Basis. Contribution of working group in to the fifth assessment report of the intergovernmental panel on climate change, Cambridge University Press, Cambridge, 2013.
Isebrands, J. D., Guenther, A. B., Harley, P., Helmig, D., Klinger, L., Vierling, L., Zimmerman, P., and Geron, C.: Volatile organic compound emission rates from mixed deciduous and coniferous forests in Northern Wisconsin, USA, Atmos. Environ., 33, 2527–2536, https://doi.org/10.1016/S1352-2310(98)00250-7, 1999.
Isidorov, V. A.: Organic Chemistry of the Earth's Atmosphere, Springer, Berlin, ISBN 0-387-51731-6, 1990.
Isidorov, V. A.: Non-methane hydrocarbons in the atmosphere of boreal forests: composition, emission rates, estimation of regional emission and photocatalytic transformation, Ecol. Bull., 42, 71–76, https://www.jstor.org/stable/20113107 (last access: 29 September 2022), 1992.
Isidorov, V. A.: Volatile Emissions of Plants: Composition, Emission Rate, and Ecological Significance, Alga Publ., St. Petersburg, ISBN: 5-87290-071-1, 1994.
Isidorov, V. and Jdanova, M.: Volatile organic compounds from leaves litter, Chemosphere 48, 975–979, https://doi.org/10.1016/s0045-6535(02)00074-7, 2002.
Isidorov, V. A., Zenkevich, I. G., and Ioffe, B.V.: Volatile organic compounds in the atmosphere of forests. Atmos. Environ.. 19, 1–8, https://doi.org/10.1016/0004-6981(85)90131-3, 1985.
Isidorov, V. A., Povarov, V. G., Klokova, E. M., Prilepsky, E. B., and Churilova, Y.: Estimation of photochemically active VOC emission by forests of the European part of the former USSR, in: Physico-Chemical Behaviour of Atmospheric Pollutants, edited by: Angeletti, G. and Restelli, G., ECSC-EC-EAEC, Brussels, Luxembourg, 31–40, ISBN: 92-826-7923-3, 1994.
Isidorov, V. A, Klokova, E. M., Povarov, V. G., and Kolkova, S.: Photocatalysis on atmospheric aerosols: Experimental studies and modelling, Catal. Today, 39, 233–242, https://doi.org/10.1016/S0920-5861(97)00104-1, 1997.
Isidorov, V., Povarov, V., and Stepanov, A.: Forest soil cover: VOC sink or source?, in: Proc. EUROTRAC Symp., edited by: Borrell, P. M. and Borrel, P., WIT Press, Southampton, 158–162, ISSN-1743-3541, 1999.
Isidorov, V. A., Vinogorova, V. T., and Rafałowski, K.: HS–SPME analysis of volatile organic compounds of coniferous needle litter, Atmos. Environ., 37, 4645–4650, https://doi.org/10.1016/j.atmosenv.2003.07.005, 2003.
Isidorov, V., Vinogorova, V., and Rafałowski, K.: Gas chromatographic determination of extractable compounds composition and emission rate of volatile terpenes from larch needle litter, J. Atmos. Chem., 50, 263–278, https://doi.org/10.1007/s10874-005-5078-6, 2005.
Isidorov, V. A., Smolewska, M., Purzyńska-Pugacewicz, A., and Tyszkiewicz, Z.: Chemical composition of volatile and extractive compounds of pine and spruce leaf litter in the initial stages of decomposition, Biogeosciences, 7, 2785–2794, https://doi.org/10.5194/bg-7-2785-2010, 2010.
Isidorov, V., Tyszkiewicz, Z., and Pirożnikow, E.: Fungal succession in relation to volatile organic compounds emissions from Scots pine and Norway spruce leaf litter–destroying fungi, Atmos. Environ., 131, 301–316, https://doi.org/10.1016/j.atmosenv.2016.02.015, 2016.
Isidorov, V. A., Pirożnikow, E., Spirina, V. L., Vasyanin, A. N., Kulakova, S. A., Abdulmanova, I. F., and Zaitsev, A. A: Emission of volatile organic compounds by plants of the floor of boreal and mid-latitude forests, J. Atmos. Chem., 79, 153–166, https://doi.org/10.1007/s10874-022-09434-3, 2022.
Jagodzinski, A. M., Dyderski, M. K., Rawlik, K., and Katna, B.: Seasonal variability of biomass, total leaf area and specific leaf area of forest understory herbs reflects their life strategies, Forest Ecol. Manage., 374, 71–81, https://doi.org/10.1016/j.foreco.2016.04.050, 2016.
Janson, R. W.: Monoterpene emissions from Scots pine and Norwegian spruce, J. Geophys. Res., 98, 2839–2850, https://doi.org/10.1029/92JD02394, 1993.
Janson, R., De Serves C., and Romero R.: Emission of isoprene and carbonyl compounds from a boreal forest and wetland in Sweden, Agr. Forest Meteorol., 98–99, 671–681, https://doi.org/10.1016/S0168-1923(99)00134-3, 1999.
Jiang, L. P., Yue, K., Yang, Y. L., and Wu, Q. G.: Leaching and freeze-thaw events contribute to litter decomposition – A review, Sains Malays., 4, 1041–1047, 2016.
Kainulainen, P. and Holopainen, J. K.: Concentration of secondary compounds in Scots pine needles at different stages of decomposition, Soil Biol. Biochem., 34, 37–42, https://doi.org/10.1016/S0038-0717(01)00147-X, 2002.
Karl, M., Guenther, A., Köble, R., Leip, A., and Seufert, G.: A new European plant-specific emission inventory of biogenic volatile organic compounds for use in atmospheric transport models, Biogeosciences, 6, 1059–1087, https://doi.org/10.5194/bg-6-1059-2009, 2009.
Karl, T., Harren,F., Warneke, C., de Gouw, J., Grayless, C., and Fall, R.: Senescing grass crops as regional sources of reactive volatile organic compounds, J. Geophys. Res., 110, D15302, https://doi.org/10.1029/2005JD005777, 2005.
Kegge, W. and Pierik, R.: Biogenic volatile organic compounds and plant competition, Trends Plant Sci., 15, 1263–132, https://doi.org/10.1016/j.tplants.2009.11.007, 2009.
Keiser, A. D., Warren, R., Filley, T., and Bradford, M. A.: Signatures of an abiotic decomposition pathway in temperate forest leaf litter, Biogeochemistry, 153, 177–190, https://doi.org/10.1007/s10533-021-00777-9, 2021.
Kesselmeier, J. and Hubert, A.: Exchange of reduced volatile sulfur compounds between leaf litter and the atmosphere, Atmos. Environ., 36, 4679–4686, https://doi.org/10.1016/S1352-2310(02)00413-2, 2002.
Kesselmeier, J. and Staudt, M.: Biogenic volatile organic compounds (VOC): An overview on emission, physiology and ecology, J. Atmos. Chem., 33, 23–88, https://doi.org/10.1023/A:1006127516791, 1999.
Kim, M. J., Park, R. J., Ho, C.-H., Choi, K.-C., Song, C.-K., and Lee, J.-B.: Future ozone and oxidants change under RCP scenarios, Atmos. Environ., 101, 103–115, https://doi.org/10.1016/j.atmosenv.2014.11.016, 2015.
Kim, M., Kim, W.-S., Tripathi, B. M., and Adams, J.: Distinct bacterial communities dominate tropical and temperate zone leaf litter, Microb. Ecol., 67, 837–848, https://doi.org/10.1007/s00248-014-0380-y, 2014.
Kirker, G. T., Bishell, A., Cappellazzi, J., Palmer, J., Bechle, N., Lebow, P., and Lebow, S.: Role of leaf litter in above-round wood decay, Microorganisms, 8, 696, https://doi.org/10.3390/microorganisms8050696, 2020.
Kivimäenpää M., Markkanen J.-M., Ghimire R. P., Holopainen T., Vuorinen M., and Holopainen J. K.: Scots pine provenance affects the emission rate and chemical composition of volatile organic compounds of forest floor, Can. J. For. Res., 48, 1373–1381, https://doi.org/10.1139/cjfr-2018-0049, 2018.
Klemmedson, J. O., Meier, C. E., and Campbell, R. E.: Litter fall transfers of dry matter and nutrients in ponderosa pine stand, Canadian J. For. Res., 20, 1105–1115, https://doi.org/10.1139/x90-146, 1990.
Kolari, P., Pumpanen, J., Kulmala, L., Ilvesniemi, H., Nikinmaa, E., Grönholm, T., and Hari, P.: Forest floor vegetation plays an important role in photosynthetic production of boreal forests, For. Ecol. Manage., 221, 241–248, https://doi.org/10.1016/j.foreco.2005.10.021, 2006.
Kourtchev, I., Giorio, C., Manninen, A., Wilson, E., Mahon, B., Aalto, J., Kajos, M., Venables, D., Ruuskanen, T., Levula, J., Loponen, M., Connors, S., Harris, N., Zhao, D., Kiendler-Scharr, A., Mentel, T., Rudich, Y., Hallquist, M., Doussin, J. F., Maenhaut, W., Bäck, J., Petäjä, T., Wenger, J., Kulmala, M., and Kalberer, M.: Enhanced volatile organic compounds emissions and organic aerosol mass increase the oligomer content of atmospheric aerosols, Sci. Rep., 6, 35038, https://doi.org/10.1038/srep35038, 2016.
Kozlov, M. V., Zverev, V., and Zvereva E. L.: Shelters of leaf-tying herbivores decompose faster than leaves damaged by free-living insects: Implications for nutrient turnover in polluted habitats, Sci. Total Environ., 568, 946–951, https://doi.org/10.1016/j.scitotenv.2016.04.121, 2016.
Kravchenko, I. K., Tikhonova, E. N., Ulanova, R. V., Menko E., and Sukacheva, M. V.: Effect of temperature on litter decomposition, soil microbial community structure and biomass in mixed-wood forest in European Russia, Curr. Sci., 116, 765–772, https://doi.org/10.18520/cs/v116/i5/765-772, 2019.
Krishna, M. P. and Mohan, M.: Litter decomposition in forest ecosystems: a review, Energy Ecol. Environ., 2, 236–249, https://doi.org/10.1007/s40974-017-0064-9, 2017.
Kulmala, M., Hämeri, K., Aalto, P. P., Mäkelä, J. M., Pirjola, L., Nilsson, E., Douglas, E., Buzorius, G., Rannik, Ü., Dal Maso, M., Seidl, W., Hoffmann, T., Janson, R., Hansson, H. C., Viisanen, Y., Laaksonen, A., and O'Dowd, C. D.: Overview of the international project on biogenic aerosol formation in the boreal forest (BIOFOR), Tellus B, 53, 324–343, https://doi.org/10.1034/j.1600-0889.2001.530402.x, 2001.
Kulmala, M., Kontkanen, J., Junninen, H., Lehtipalo, K., Manninen, H. E., Nieminen, T., Petäjä, T., Sipilä, M., Schobesberger, S., Rantala, P., Franchin, A., Jokinen, T., Järvinen, E., Äijälä, M., Kangasluoma, J., Hakala, J., Aalto, P. P., Paasonen, P., Mikkilä, J., Vanhanen, J., Aalto, J., Hakola, H., Makkonen, U., Ruuskanen, T., Mauldin III, R. L., Duplissy, J., Vehkamäki, H., Bäck, J., Kortelainen, A., Riipinen, I., Kurtén, T., Johnston, M. V., Smith, J. N., Ehn, M., Mentel, T. F., Lehtinen, K. E., Laaksonen, A., Kerminen, V. M., and Worsnop, D. R.: Direct observation of atmospheric aerosol nucleation, Science, 339, 943–946, https://doi.org/10.1126/science.1227385, 2013.
Kurz, C., Coûteaux, M.-M., and Thiéry, J. M.: Residence time and decomposition rate of Pinus pinaster needles in a forest floor from direct field measurements under a Mediterranean climate, Soil Biol. Biochem., 32, 1197–1206, https://doi.org/10.1016/S0038-0717(00)00036-5, 2000.
Kuzma, J., Nemecek-Marshall, M., Pollock, W. H., and Fall, R.: Bacteria produce of volatile hydrocarbon isoprene, Curr. Microbiol., 30, 97–103, https://doi.org/10.1007/BF00294190, 1995.
Lee, S. H., Gordon, H., Yu, H., Lehtipalo, K., Haley, R., Li, Y., and Zhang, R.: New particle formation in the atmosphere: From molecular clusters to global climate, J. Geophys. Res.-Atmos., 124, 7098–7146, https://doi.org/10.1029/2018JD029356, 2019.
Leff, J. W. and Fierer, N.: Volatile organic compound (VOC) emissions from soil and litter samples, Soil. Biol. Biochem., 40, 1629–1636, https://doi.org/10.1016/j.soilbio.2008.01.018, 2008.
Lindo, Z. and Gonzalez, A.: The bryosphere: an integral and influential component of the earth's biosphere, Ecosystems 13, 612–627, https://doi.org/10.1007/s10021-010-9336-3, 2010.
Lindwall, F., Faubert, P., and Rinnan, R.: Diel variation of biogenic volatile organic compound emissions - field study in the sub, low and high arctic on the effect of temperature and light, PLoS One 10, e0123610, https://doi.org/10.1371/journal.pone.0123610, 2015.
Liu, C., Ilvesniemi, H., Berg, B., Kutsch, W., Yang, Y., Ma, X., and Westman, C. J.: Aboveground litterfall in Eurasian forests, J. Forest. Res., 14, 27–34, https://doi.org/10.1007/BF02856758, 2003.
Li, Y. L., Huang, H. Y., Wu, G. F., Yan, S. Z. Chang, Z. Z., Bi, J. H., and Chen, L.: The effect of UV-A on dry rice straw decomposition under controlled laboratory conditions, Bioresources, 11, 2568–2582, https://doi.org/10.15376/biores.11.1.2568-2582, 2016.
Lodato, M. B., Boyette, J. S., Smilo, R. A., Jackson, C. R., Halvorson, H. M., and Kuehn, K. A.: Functional importance and diversity of fungi during standing grass litter decomposition, Oekologia, 195, 499–512, https://doi.org/10.1007/s00442-020-04838-y, 2021.
López-Mondéjar, R., Zühlke, D., Becher, D., Riedel, K., and Baldrian, P.: Cellulose and hemicellulose decomposition by forest soil bacteria proceeds by the action of structurally variable enzymatic systems, Sci. Rep., 6, 25279, https://doi.org/10.1038/srep25279, 2016.
Lukowski, A., Giertych, M. J., Zmuda, M., Maderek, E., Adamczyk, D., and Karolewski, P.: Decomposition of herbivore-damaged leaves of understory species growing in oak and pine stands, Forests 12, 304, https://doi.org/10.3390/f12030304, 2021.
Mahajan, R., Nikitina, A., Litti, Y., Nozhevnikova, A., and Goel, G.: Autochthonous microbial community associated with pine needle forest litterfall influences its degradation under natural environmental conditions, Environ. Monit. Assess., 188, 417, https://doi.org/10.1007/s10661-016-5421-1, 2016.
Makkonen, R., Asmi, A., Kerminen, V.-M., Boy, M., Arneth, A., Guenther, A., and Kulmala, M.: BVOC-aerosol-climate interactions in the global aerosol-climate model ECHAM5.5-HAM2, Atmos. Chem. Phys., 12, 10077–10096, https://doi.org/10.5194/acp-12-10077-2012, 2012.
Mäki, M., Heinonsalo, J., Hellén, H., and Bäck, J.: Contribution of understorey vegetation and soil processes to boreal forest isoprenoid exchange, Biogeosciences, 14, 1055–1073, https://doi.org/10.5194/bg-14-1055-2017, 2017.
Mäki, M., Krasnov, D., Hellen, H., Noe, S., and Back, J.: Stand type affects fluxes of volatile organic compounds from the forest floor in hemiboreal and boreal climates, Plant Soil, 441, 363–381, https://doi.org/10.1007/s11104-019-04129-3, 2019a.
Mäki, M., Aalto, J., Hellèn, H., Pihlatie, M., and Bäck, J.: Interannual and seasonal dynamics of volatile organic compounds fluxes from the boreal forest floor, Front. Plant Sci., 10, 191, https://doi.org/10.3389/fpls.2019.00191, 2019b.
Maltsev, Y. I., Pakhomov, A. Y., and Maltseva, I. A.: Specific features of algal communities in forest litter of forest biogeocenoses of the steppe zone, Contemp. Prob. Ecol., 10, 71–76, https://doi.org/10.1134/s1995425517010085, 2017a.
Maltsev, Y. I., Didovich, S. V., and Maltseva, I. A.: Seasonal changes in the communities of microorganisms and algae in the litters of tree plantations in the steppe zone, Euras. Soil Sci., 50, 935–942, https://doi.org/10.1134/S1064229317060059, 2017b.
Masiol, M., Squizzato, S., Chalupa, D., Rich, D. Q., and Hopke, P. K.: Spatial-temporal variations of summertime ozone concentrations across a metropolitan area using a network of low-cost monitors to develop 24 hourly land-use regression models, Sci. Total Environ., 654, 1167–1178, https://doi.org/10.1016/j.scitotenv.2018.11.111, 2019.
Matthews, E.: Global litter production, pools, and turnover times: Estimates from measurement data and regression models, J. Geophys. Res., 102, 18771–18800, https://doi.org/10.1029/97JD02956, 1997.
McBride, S. G., Choudoir, M., Fierer, N., and Strickland, M.: Volatile organic compounds from leaf litter decomposition alter soil microbial communities and carbon dynamics, Ecology 101, e03130, https://doi.org/10.1002/ecy.3130, 2020.
Mochizuki, T., Tani, A., Takahashi, Y., Saigusa N., and Ueyama, M.: Long–term measurement of terpenoid flux above a Larix kaempferi forest using a relaxed eddy accumulation method, Atmos. Environ., 83, 53–61, https://doi.org/10.1016/j.atmosenv.2013.10.054, 2014.
Mogensen, D., Gierens, R., Crowley, J. N., Keronen, P., Smolander, S., Sogachev, A., Nölscher, A. C., Zhou, L., Kulmala, M., Tang, M. J., Williams, J., and Boy, M.: Simulations of atmospheric OH, O3 and NO3 reactivities within and above the boreal forest, Atmos. Chem. Phys., 15, 3909–3932, https://doi.org/10.5194/acp-15-3909-2015, 2015.
Morén, A.-S. and Lindroth A.: CO2 exchange at the floor of boreal forests, Agric. For. Meteorol., 101, 1–14, https://doi.org/10.1016/S0168-1923(99)00160-4, 2000.
Nahrowi, M. B., Susilowati, A., and Setyaningsih, R.: Screening and identification of lignolytic bacteria from the forest at eastern slope of Lawu Mountain, AIP Conf. Proc. 2002, 020028, https://doi.org/10.1063/1.5050138, 2018.
Navarro, J. C. A., Smolander, S., Struthers, H., Zorita, E., Ekman, A. M., Kaplan, J. O., Guenther, A., Arneth, A., and Riipinen, I.: Global emissions of terpenoid VOCs from terrestrial vegetation in the last millennium, J. Geophys. Res.-Atmos., 119, 6867–6885, https://doi.org/10.1002/2013jd021238, 2014.
Nilsson, T., Larsen, T. O., Montanarella, L., and Madsen, J. Ø.: Application of head–space solid–phase microextraction for the analysis of volatile metabolites emitted by Penicillium species, J. Microbiol. Meth., 25, 245–255, https://doi.org/10.1016/0167-7012(95)00093-3, 1996.
Nölscher, A. C., Bourtsoukidis, E., Bonn, B., Kesselmeier, J., Lelieveld, J., and Williams, J.: Seasonal measurements of total OH reactivity emission rates from Norway spruce in 2011, Biogeosciences, 10, 4241–4257, https://doi.org/10.5194/bg-10-4241-2013, 2013.
Norrman, J.: Production of volatile organic compounds by the yeast fungus Dipodascus aggregatus., Arch. Microbiol., 68, 133–149, https://doi.org/10.1007/BF00413873, 1969.
Osono, T. and Takeda, H.: Decomposition of organic chemical components in relation to nitrogen dynamics in leaf litter of 14 tree species in a cool temperate forests, Ecol. Res., 20, 41–49, https://doi.org/10.1007/s11284-004-0002-0, 2005.
Otaki M. and Tsuyuzaki S.: Succession of litter-decomposing microbial organisms in deciduous birch and oak forests, northern Japan, Acta Oecol., 101, 103485, https://doi.org/10.1016/j.actao.2019.103485, 2019.
Paaso, U., Keski-Saari, S., Keinänen, M., Karvinen, H., Silfrer, T., Rousi, M., and Mikola, J.: Interpopulation genotypic variation of foliar secondary chemistry during leaf senescence and litter decomposition in silver birch (Betula pendula), Front. Plant Sci., 8, 1074, https://doi.org/10.3389/fpls.2017.01074, 2017.
Petersson, G.: High ambient concentration of monoterpenes in Scandinavian pine forests, Atmos. Environ., 22, 2617–2619, https://doi.org/10.1016/0004-6981(88)90497-0, 1988.
Pokhylenko, A. P., Didur, O. O., Kulbachko, Y. L., Bandura, L. P., and Chernykh, S. A.: Influence of saprophages (Isopoda, Diplopoda) on leaf litter decomposition under different levels of humidification and chemical loading, Biosyst. Divers., 28, 384–389, https://doi.org/10.15421/012049, 2020.
Porchikalov, A. V. and Karelin, D. V.: A field study of tundra plant litter decomposition rate via mass loss and carbon dioxide emission: The role of biotic and abiotic controls, biotope, season of year, and spatial–temporal scale, Biol. Bull. Rev., 5, 1–16, https://doi.org/10.1134/S2079086415010077, 2015.
Praplan, A. P., Tykkä, T., Schallhart, S., Tarvainen, V., Bäck, J., and Hellén, H.: OH reactivity from the emissions of different tree species: investigating the missing reactivity in a boreal forest, Biogeosciences, 17, 4681–4705, https://doi.org/10.5194/bg-17-4681-2020, 2020.
Purahong, W., Wubet, T., Lentendu, G., Schloter, M., Pecyna, M. J., Kapturska, D., Hofrichter, M., Kruger, D., and Buscot, F.: Life in leaf litter: novel insights into community dynamics of bacteria and fungi during litter decomposition, Mol. Ecol., 26, 4059–4074, https://doi.org/10.1111/mec.13739, 2016.
Räisänen, T., Ryyppö, A., and Kellomäki, S.: Monoterpene emission of a boreal Scots pine (Pinus sylvestris L.) forest, Agric. For. Meteor., 149, 808–819, https://doi.org/10.1016/j.agrformet.2008.11.001, 2009.
Ramirez, K. S., Lauber, C. L., and Fierer, N.: Microbial consumption and production of volatile compounds at the soil–litter interface, Biogeochemistry, 99, 97–107, https://doi.org/10.1007/s10533-009-9393-x, 2010.
Rinnan, R., Rinnan, Å., Holopainen, T., Holopainen, J. K., and Pasanen, P.: Emission of non-methane volatile organic compounds (VOCs) from boreal peatland microcosms – effects of ozone exposure, Atmos. Environ., 39, 921–930, https://doi.org/10.1016/j.atmosenv.2004.09.076, 2005.
Santurtún, A., González–Hildago, J. C., Sanchez–Lorenzo, A., and Zarrabeitia, M. T.: Surface ozone concentration trends and its relationship with weather type in Spain (2001–2010), Atmos. Environ., 101, 10–22, https://doi.org/10.1016/j.atmosenv.2014.11.005, 2015.
Sharma, A., Ojha, N., Pozzer, A., Gufran Beig, G., Sachin S., and Gunthe, S. S.: Revisiting the crop yield loss in India attributable to ozone, Atmos. Environ., 1, 1–8, https://doi.org/10.1016/j.aeaoa.2019.100008, 2019.
Schöller, C. E. G., Gurtler, H., Pedersen, R., Molin, S., and Wilkins, K. Volatile metabolites from actinomycetes, J. Agric. Food Chem., 50, 2615–2621, https://doi.org/10.1021/jf0116754, 2002.
Shao, S., Zhao, Y., Zhang, W., Hu, G., Xie, H., Yan, J., Han, S., He, H., and Zhang, X.: Linkage of microbial residue dynamics with soil organic carbon accumulation during subtropical forest succession, Soil Biol. Biochem., 114, 114–120, https://doi.org/10.1016/j.soilbio.2017.07.007, 2017.
Sindelarova, K., Granier, C., Bouarar, I., Guenther, A., Tilmes, S., Stavrakou, T., Müller, J.-F., Kuhn, U., Stefani, P., and Knorr, W.: Global data set of biogenic VOC emissions calculated by the MEGAN model over the last 30 years, Atmos. Chem. Phys., 14, 9317–9341, https://doi.org/10.5194/acp-14-9317-2014, 2014.
Sinha, V., Williams, J., Lelieveld, J., Ruuskanen, T., Kajos, M., Patokoski, J., Hellen, H., Hakola, H., Mogensen, D., Boy, M., Rinne, J., and Kulmala, M.: OH reactivity measurements within a boreal forests: Evidence of unknown reactive emissions, Environ. Sci. Technol., 44, 6614–6620, https://doi.org/10.1021/es101780b, 2010.
Starke, R., Kermer, R., Ullmann-Zeunert, L., Baldwin, I. T., Seifert, J., Bastida, F., von Bergen, M., and Jehmlich N.: Bacteria dominate the short-term assimilation of plant-derived N in soil, Soil Biol. Biochem., 96, 30–38, https://doi.org/10.1016/j.soilbio.2016.01.009, 2016.
Stepanov, A.: Formation of concentration fields of phytogenic volatile organic compounds under the canopy of the boreal forest, PhD Thesis, St. Petersburg University, 1999.
Stepen, R. A., Vershnyak, V. M., and Peryshkina, G. I.: The store of essential oil in Yakut pine (Pinus sylvestris L.) forests, Rastit. Res., 1, 81–86, 1987 (in Russian).
Stoy, P. C., Trowbridge, A. M., Siqueira, M. B., Freire, L. S., Phillips, R. P., Jacobs, L., Wiesner, S., Moonson, R. K., and Novick, K. A.: Vapor pressure deficit helps explain biogenic volatile organic compound fluxes from the forest floor and canopy of a temperate deciduous forest, Oecologia, 197, 971–988, https://doi.org/10.1007/s00442-021-04891-1, 2021.
Strode, S. A., Ziemke, J. R., Oman, L. D., Lamsal, L. N., Olsen, M. A., and Liu, J. H.: Global changes in the diurnal cycle of surface ozone, Atmos. Environ., 199, 323–333, https://doi.org/10.1016/j.atmosenv.2018.11.028, 2019.
Štursová, M., Šnajdr, J., Koukol, O., Tláskal, V, Cajthaml, T., and Baldrian, P.: Long-term decomposition of litter in the montane forest and the definition of fungal traits in the successional space, Fungal Ecol., 46, 100913, https://doi.org/10.1016/j.funeco.2020.100913, 2020.
Sunesson, A. L., Vaes, W. H. J., Nilsson, C. A., Blomquist, G., Andersson, B., and Carlson, R.: Identification of volatile metabolites from five fungal species cultivated on two media, Appl. Environ. Microbiol., 61, 2911–2918, https://doi.org/10.1128/AEM.61.8.2911-2918.1995, 1995.
Svendsen, S. H., Prieme, A., Voriskova, J., Kramshoj, M., Schostag, M., Jacobsen, C S., and Rinnan, R.: Emissions of biogenic volatile organic compounds from arctic shrub litter are coupled with changes in the bacterial community composition, Soil Biol. Biochem., 120, 80–90, https://doi.org/10.1016/j.soilbio.2018.02.001, 2018.
Swift, M. J., Heal, O. W., and Anderson, J. M.: Decomposition in Terrestrial Ecosystems, Blackwell Sci. Publ., Oxford, ISBN: 05-200-40015, 1979.
Tang, J., Schurgers, G., and Rinnan, R.: Process understanding of soil BVOC fluxes in natural ecosystems: a review, Rev. Geophys., 57, 966–986, https://doi.org/10.1029/2018rg000634, 2019.
Tarr, M. A., Miller, W. L., and Zepp, R. G.: Direct carbon monoxide photoproduction from plant matter, J. Geophys. Res., 100, 11403–11413, 1995.
Tennakoon, D. S., Gentekaki, E., Jeewon, R., Kuo, C. H., Promputtha, I., and Hyde, K. D.: Life in leaf litter: Fungal community succession during decomposition, Mycosphere, 12, 406–429, https://doi.org/10.5943/mycosphere/12/1/5, 2021.
Tian, X., Takeda, H., and Azuma, J.: Dynamics of organic-chemical components in leaf litters during a 5.5-year decomposition, Eur. J. Soil. Biol., 36, 81–89, https://doi.org/10.1016/S1164-5563(00)01049-9, 2000.
Tingey, D. T., Evans, R. C., Bates, E. H., and Gumpertz, M. L.: Isoprene emissions and photosynthesis in three ferns. The influence of light and temperature, Physiol. Plant., 69, 609–616, https://doi.org/10.1111/J.1399-3054.1987.TB01974.X, 1987.
Trowbridge, A. M., Stoy, P. C., and Phillips, R. P.: Soil biogenic volatile organic compound flux in a mixed hardwood forest: Net uptake at warmer temperatures and the importance of mycorrhizal associations, J. Geophys. Res.-Biogeo., 124, e2019JG005479, https://doi.org/10.1029/2019jg005479, 2020.
Urbanová, M., Šnajdr, J., and Baldrian, P.: Composition of fungal and bacterial communities in forest litter and soil is largely determined by dominant trees, Soil Biol. Biochem., 84, 53–64, https://doi.org/10.1016/j.soilbio.2015.02.011, 2015.
Vingarzan, R.: A review of surface ozone background levels and trends, Atmos. Environ., 38, 3431–3442, https://doi.org/10.1016/j.atmosenv.2004.03.030, 2004.
Viros, J., Fernandez, C., Wortham, H., Gavinet, J., Lecareux, C., and Ormeño, E.: Litter of mediterranean species as a source of volatile organic compounds, Atmos. Environ., 242, 117815, https://doi.org/10.1016/j.atmosenv.2020.117815, 2020.
Viros, J., Santonja, M., Temime-Roussel, B., Wortham, H., Fernandez, C., and Ormeño, E.: Volatilome of Aleppo Pine litter over decomposition process, Ecol. Evol., 11, 6862–6880, https://doi.org/10.1002/ece3.7533, 2021.
Virzo De Santo, A., Rutigliano, F. A., Berg, B., Fioretto, A., Puppi, G., and Alfani, A.: Fungal mycelium and decomposition of needle litter in three contrasting coniferous forests, Acta Oecol., 23, 247–259, https://doi.org/10.1016/S1146-609X(02)01155-4, 2002.
Wagner, W. P., Helmig, D., and Fall, R.: Isoprene biosynthesis in Bacillus subtilis via the methylerythritol phosphate pathway, J. Nat. Prod., 63, 37–40, https://doi.org/10.1021/np990286p, 2000.
Wang, J., Xu, B., Wu, Y., Gao, J., and Shi, F.: Flower litters of alpine plants affect soil nitrogen and phosphorus rapidly in the eastern Tibetan Plateau, Biogeosciences, 13, 5619–5631, https://doi.org/10.5194/bg-13-5619-2016, 2016.
Wang, M., Schurgers, G., Hellèn, H., Lagergren, F., and Holst, T.: Biogenic volatile organic compound emission from a boreal forest floor, Boreal Environ. Res., 23, 249–265, 2018.
Wang, Z., Yin, X., and Li, X.: Soil mesofauna effects on litter decomposition in the coniferous forest of the Chanbai Mountains, China, Appl. Soil Biol., 92, 64–71, https://doi.org/10.1016/j.apsoil.2015.03.010, 2015.
Warneke, C., Karl, T., Judmaier, H., Hansel, A., Jordan, A., Lindinger, W., and Crutzen, P. J.: Acetone, methanol, and other partially oxidized volatile organic emissions from dead plant matter by abiological processes: significance for atmospheric HOx chemistry, Glob. Biogeochem. Cy., 13, 9–17, https://doi.org/10.1029/98GB02428, 1999.
Wilkins, K.: Volatile metabolites from actinomycetes, Chemosphere 32, 1427–1434, https://doi.org/10.1016/0045-6535(96)00051-3, 1996.
Wilt, F. M., Miller, G. C., Everett, R. L., and Hackett, M.: Monoterpene concentrations in fresh, senescent, and decaying foliage of singleleaf pinyon (Pinus monophylla Torr. & Frem.: Pinaceae) from the western Great Basin, J. Chem. Ecol., 19, 185–194, https://doi.org/10.1007/BF00993688, 1993.
Wood, S. E., Gaskin, J. F., and Langenheim, J. H.: Loss of monoterpenes from Umbellularia californica leaf litter, Biochem. System. Ecol., 23, 581–591, https://doi.org/10.1016/0305-1978(95)00087-9, 1995.
Wu, F., Yang, W., Zhang, J., and Deng, R.: Litter decomposition in two subalpine forests during the freeze–thaw season, Acta Oecol., 36, 135–140, https://doi.org/10.1016/j.actao.2009.11.002, 2010.
Wurzenberger, M. and Ghosch, W.: Origin of the oxygen in the products of the enzymatic cleavage reaction of linoleic acid to 1-octen-3-ol and 10-oxo-trans-8-decenoic acid in mushrooms (Psalliota bispora), Biochim. Biophys. Ac., 794, 18–24, https://doi.org/10.1016/0005-2760(84)90292-3, 1984.
Yang, Y., Shao, M., Wang, X., Nölscher, A., Kessel, S., Guenther, A., and Williams, J.: Towards a quantitative understanding of total OH reactivity: A review, Atmos. Environ., 134, 147–161, https://doi.org/10.1016/j.atmosenv.2016.03.010, 2016.
Zhang, D., Hui, D., Luo, Y., and Zhou, G.: Rates of litter decomposition in terrestrial ecosystems: global patterns and controlling factors, J. Plant Ecol., 1, 85–93, https://doi.org/10.1093/jpe/rtn002, 2008.
Zhang, Y. Q., West, J. J., Emmons, L. K., Flemming, J., Jonson, J. E., Lund, M. T., Sekiya, T., Sudo. K., Gaudel, A., Chang, K.-L., Nédélec, P., and Thouret, V.: Contributions of world regions to the global tropospheric ozone burden change from 1980 to 2010, Geophys. Res. Let., 48, e2020GL089184, https://doi.org/10.1029/2020GL089184, 2021.
Zhou, P., Ganzeveld, L., Rannik, Ü., Zhou, L., Gierens, R., Taipale, D., Mammarella, I., and Boy, M.: Simulating ozone dry deposition at a boreal forest with a multi-layer canopy deposition model, Atmos. Chem. Phys., 17, 1361–1379, https://doi.org/10.5194/acp-17-1361-2017, 2017.
Zimmerman, P. R., Chatfield, R. B., Fishmann, J., Crutzen, P. J., amd Hanst, P. L.: Estimation of production of CO and H2 from the oxidation of hydrocarbon emissions from vegetation, Geophys. Res. Lett., 5, 679–682, https://doi.org/10.1029/GL005i008p00679, 1978.
Zimmer, M., Kautz, G., and Topp, W.: Leaf litter–colonizing microbiota: supplementary food source or indicator of food quality for Porcellio scaber (Isopoda: Oniscidea)?, Eur. J. Soil Biol., 39, 209–216, https://doi.org/10.1016/S0038-0717(02)00065-2, 2003.
Zlotin, R. I. and Bazilevich, N. I.: Global maps of organic matter structure in terrestrial ecosystems, in: Geography of organic matter production and decay. SCOPE Conf. Pap. 18, Szymbark, 11–18 September 1991, Warszaw, pp. 7–20, 1993.
Zong, R. H., Yang, X., Wen, L., Xu, C., Zhu, Y., Chen, T., Yao, L., Wang, L., Zhang, J., Yang, L., Wang, L., Shao, M., Zhu, T., Xue, L., and Wang, W.: Strong ozone production at a rural site in the North China Plain: Mixed effects of urban plumes and biogenic emissions, J. Envir. Sci., 71, 261–270, https://doi.org/10.1016/j.jes.2018.05.003, 2018.
Zyabchenko, S. S.: North European Pine Forests, Nauka Publ., Leningrad, 1984 (in Russian).
- Abstract
- Introduction
- Biotic and abiotic controls of leaf litter decomposition
- Qualitative composition of plant litter and LSC emissions
- VOCs from litter-decomposing microbes
- VOC emission rates: laboratory and field measurements
- Conclusions
- Data availability
- Author contributions
- Competing interests
- Disclaimer
- Financial support
- Review statement
- References
- Supplement
- Article
(887 KB) - Full-text XML
main playersin the formation of tropospheric O3 and secondary aerosols, which have a significant impact on climate, human health and crops. A complex mixture of VOCs, formed as a result of physicochemical and biological processes, is released into the atmosphere from the forest floor. This review presents data on the composition of VOCs and contribution of various processes to their emissions.
- Abstract
- Introduction
- Biotic and abiotic controls of leaf litter decomposition
- Qualitative composition of plant litter and LSC emissions
- VOCs from litter-decomposing microbes
- VOC emission rates: laboratory and field measurements
- Conclusions
- Data availability
- Author contributions
- Competing interests
- Disclaimer
- Financial support
- Review statement
- References
- Supplement