the Creative Commons Attribution 4.0 License.
the Creative Commons Attribution 4.0 License.
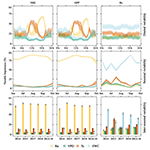
Radiation, soil water content, and temperature effects on carbon cycling in an alpine swamp meadow of the northeastern Qinghai–Tibetan Plateau
Junqi Wei
Torben Røjle Christensen
Zhiyun Jiang
Yujun Ma
Xiuchen Wu
Hongyun Yao
Efrén López-Blanco
Predicted intensified climate warming will likely alter the ecosystem net carbon (C) uptake of the Qinghai–Tibetan Plateau (QTP). Variations in C sink–source responses to climate warming have been linked to water availability; however, the mechanisms by which net C uptake responds to soil water content in saturated swamp meadow ecosystems remain unclear. To explore how soil moisture and other environmental drivers modulate net C uptake in the QTP, field measurements were conducted using the eddy covariance technique in 2014, 2015, 2017, and 2018. The alpine swamp meadow presented in this study was a persistent and strong C sink of CO2 (−168.0 ± 62.5 g C m−2 yr−1, average ± standard deviation) across the entire 4-year study period. A random forest machine-learning analysis suggested that the diurnal and seasonal variations of net ecosystem exchange (NEE) and gross primary productivity (GPP) were regulated by temperature and net radiation. Ecosystem respiration (Re), however, was found mainly regulated by the variability of soil water content (SWC) at different temporal aggregations, followed by temperature, the second contributing driver. We further explored how Re is controlled by nearly saturated soil moisture and temperature comparing two different periods featuring almost identical temperatures and significant differences on SWC and vice versa. Our data suggest that, despite the relatively abundant water supply, periods with a substantial decrease in SWC or increase in temperature produced higher Re and therefore weakened the C sink strength. Our results reveal that nearly saturated soil conditions during the growing seasons can help maintain lower ecosystem respiration rates and thus enhance the overall C sequestration capacity in this alpine swamp meadow. We argue that soil respiration and subsequent ecosystem C sink magnitude in alpine swamp meadows could likely be affected by future changes in soil hydrological conditions caused by permafrost degradation or accelerated thawing–freezing cycling due to climate warming.
- Article
(5594 KB) -
Supplement
(358 KB) - BibTeX
- EndNote
Wetlands play a significant role in the global carbon (C) cycle due to a large amount of C stored in their soils. The Qinghai–Tibetan Plateau (QTP), with an average altitude of over 4000 m a.s.l., has approximately 10 × 104 km2 of natural wetlands, of which ∼ 50 % (4.9 × 104 km2) are alpine swamp meadows. These ecosystems are predominantly located in permafrost areas and are typically soil-nutrient-rich and water-logged (Bai et al., 2019; Zhao et al., 2005). Climate change and human disturbance can have profound consequences on permafrost regions (Biskaborn et al., 2019) and significantly impact their hydrological regimes (Lafrenière and Lamoureux, 2019). Hydrological regimes have an important role in controlling wetland functioning (Bohn et al., 2007; Christensen et al., 2003), and the changes of hydrological regimes may put the wetland functioning of the QTP under pressure (Hruby, 1999; Woodward and Wui, 2001; Foti et al., 2013).
The QTP is forecasted to be warmer and wetter in the future (Chen et al., 2015; Cheng et al., 2011). Warming may accelerate the microbial breakdown of alpine soil organic C and subsequently increase CO2 emissions (X. Zhu et al., 2015). Warming could also improve C sequestration capacity by enhancing the photosynthetic inputs and growth rates of alpine plants (Fu et al., 2015). Therefore, the potential increase in CO2 emissions due to warming in alpine regions could be partially offset by enhanced C uptake (Schuur et al., 2009), triggering different net C uptake responses to climate warming. For example, an increase in temperature in the QTP has been associated with net C sinks in the Zoige alpine wetlands (Kang et al., 2014) but also with net C sources in the Damxung alpine swamp meadow (Niu et al., 2017).
According to recent studies in QTP alpine grasslands, water conditions such as soil water content (SWC) can be a key factor that changes water-use patterns and ecophysiological characteristics of alpine plants (Wu et al., 2019) and modulates the warming-mediated increase in ecosystem C uptake (Ganjurjav et al., 2016; Peng et al., 2014). Ecosystem C processes such as net C uptake and soil respiration may increase with SWC in dry environments but decrease with SWC in water-logged environments (Quan et al., 2019; Taylor et al., 2017). Warming in conjunction with increased precipitation can turn an ecosystem from net source to a sink of C (J. Zhao et al., 2019), increasing both photosynthesis and respiration rates during warmer and wetter years (López-Blanco et al., 2017, 2018). However, when warming occurs in soils associated with low moisture, soil drought can change ecosystems from C sinks to sources (Ganjurjav et al., 2018). Studies in QTP alpine meadows have indicated that warming significantly stimulates ecosystem net C uptake in wet years but does not affect ecosystem net C uptake in dry years, because the positive effects of warming on net C uptake are compensated for by the negative effects of lower soil moisture (Peng et al., 2014).
However, the existing studies concerning ecosystem C dynamics on the QTP mainly focused on alpine meadows (Saito et al., 2009; Zhao et al., 2005, 2010; Z. Zhu et al., 2015); only a few analyses have been conducted to specifically characterize C dynamics in alpine swamp meadows (Zhao et al., 2010; Qi et al., 2021; Liu et al., 2020; Zhu et al., 2020). The magnitudes and interannual variations of net ecosystem exchange (NEE) in alpine wetlands from the QTP are proven to be closely related to radiation, precipitation, and temperature (Cao et al., 2017; Niu et al., 2017). Temperature has been identified as an important driver for ecosystem respiration (Re) in alpine swamp meadows, and Zhao et al. (2005, 2010) found that Re follows an exponential relationship with soil temperature. Other studies also noticed that rainfall is an important determinant of the interannual C sink–source strength in alpine swamp meadows (Liu et al., 2019; Zhu et al., 2020). For example, CO2 emissions were reported to decrease notably after rain events (Zhao et al., 2010). Even though alpine swamp meadow ecosystems are characterized by high SWC, the role of SWC on C cycling has often been neglected or assumed to be less important. Compared to other factors, the effects of SWC on the net C uptake in alpine swamp meadows are still unclear. Climate warming and the associated enhanced evapotranspiration and permafrost degradation may change soil hydrology dramatically (Andresen et al., 2020; L. Zhao et al., 2019). Considering the critical role that SWC played in regulating C uptake and soil respiration of other ecosystem types (Ganjurjav et al., 2016; Peng et al., 2014; Quan et al., 2019; Taylor et al., 2017; Wu et al., 2019), it is important to understand whether the change of SWC would aggravate the saturated water stress or trigger drought effects on net C uptake in the alpine swamp meadow ecosystem under future climate warming.
These uncertainties require a detailed investigation to understand wetland C source–sink processes and their potential future C sink strength variations (sign and magnitude). In addition, as compared with alpine meadows, long-term continuous observations for the alpine swamp meadow are still needed to investigate C dynamics during dry and wet years. So a 4-year field observation dataset is provided in this study to characterize and quantify the importance of SWC in addition to temperature and net radiation on the C sink strength of an alpine swamp meadow. Therefore, the objectives of this study are to (i) quantify the diurnal and seasonal variations of net ecosystem exchange (NEE), gross primary productivity (GPP), and ecosystem respiration (Re); (ii) identify and quantify the relative importance of different key environmental drivers contributing to the variability observed of NEE, Re, and GPP; and (iii) analyse how these C fluxes respond to soil water availability, temperature, and radiation variation in a QTP alpine swamp meadow. This study would provide new insights into a better understanding of the complex C cycle dynamics in the Tibetan Plateau driven by the almost certain future intensified climate warming.
2.1 Site description
The study site (37∘35.75′ N, 100∘00.47′ E, 3571 m a.s.l.) is located in Gangcha County, Qinghai Province, in the north-eastern part of the QTP (Fig. 1a). The mean annual temperature and precipitation measured at the Gangcha National Weather Station were 0.1∘ and 389.4 mm between 1982 and 2011, respectively (Zhang et al., 2016). The site area has seasonal permafrost featuring frozen soils between January–March and November–December for a total of about 125–135 d (Zhang, 2014). The dominant species of the alpine swamp meadow ecosystem is Kobresia pygmaea, followed by Saussurea pulchra, Polygonum viviparum, and Potentilla saundersiana. The average plant height at the experimental site is 7.4 ± 1.5 cm, with a 97 ± 2 % coverage. Our study defined the growing season as the period between June and September. The early (June–July) and late (August–September) growing seasons, as they will be henceforth referred to, correspond with the early growing season and late growing seasons, respectively.
2.2 Field measurements
An eddy covariance (EC) system was installed at the study site (Fig. 1b) to measure the CO2 fluxes at a sampling frequency of 10 Hz from 2014 to 2018. Data for 2016 were missing due to equipment malfunction. The EC system included an open-path infrared gas analyser, which quantified fluctuations in water vapour and CO2 fluxes. A 3-D sonic anemometer was installed at a 2.0 m height above ground to directly measure horizontal and vertical wind velocity components (u, v, and w). C flux data were recorded with a data logger (Campbell Scientific Inc.). An automated meteorological station was installed near the EC station to measure meteorological variables such as air temperature (Ta; ∘C), precipitation (P; mm), net radiation (Rn; W m−2), wind speed (WS; m s−1), wind direction (WD; ∘), relative humidity (RH; %), and vapour pressure deficit (VPD; hPa). The meteorological data were collected at 1 min intervals and subsequently resampled at 30 min time steps to keep pace with the EC data. More details on the in situ instrument specifications are summarized in Table 1.
Soil water content (SWC; %) was measured at depths of 10, 20, 40, 60, and 100 cm from the soil surface with EC-H2O sensors (Decagon Devices, USA) at a 10 min recording frequency. The precision of the EC-H2O sensors for soil moisture measurements was ± 0.03 m3 m−3. As the roots of Kobresia meadows are mainly distributed within the top 20 cm of soil, we focused only on the variation of SWC in the top 20 cm of the soil.
2.3 Eddy covariance data processing
The half-hourly NEE data were calculated using the EddyPro software (version 5.2, LI-COR) from the 10 Hz raw data. During the calculation, three-dimensional rotation was used to correct the data by removing the effects of instrument tilt irregularity on airflow (Wilczak et al., 2001). Webb, Pearman, and Leuning (WPL) (Webb et al., 1980) correction was applied to calculate the averages of CO2 covariance, rectifying the air density variations induced by heat and water vapour. The half-hourly flux data were quality-checked based on several filtering algorithms, including (1) the rejection of outliers in sonic temperature, water vapour density, and CO2 density (Li et al., 2008; Liu et al., 2011); (2) the elimination of data 1 h before and after precipitation events (Li et al. 2008; Liu et al., 2011); (3) the removal of negative NEE during the non-growing season (from November to March) (Cao et al., 2017; Qi et al., 2021) attributed to the self-heating effect from EC instruments (Cao et al., 2017); and (4) the exclusion of measurements with weak turbulence conditions at night-time. The weak turbulence periods were identified by bootstrapping friction velocity (u∗) thresholds, as described by Papale et al. (2006). This approach effectively divided the data into 4-year and seven-temperature subsets with similar micro-meteorological conditions (except for u∗). The u∗ thresholds (5 %, 50 %, and 95 % of bootstrapping) were calculated specifically per year and temperature subset.
Based on those different subsets, we gap-filled and partitioned NEE (into GPP and Re) to spread the uncertainty variability that emerged from the different u∗ thresholds, similar to López-Blanco et al. (2017). All missing data were marked as −9999 (no data). Negative and positive NEE values represent the sink and source of C, respectively. Additionally, a standardized mechanism to fill NEE gaps is needed for adequate data processing (Moffat et al., 2007). Therefore, this study adopted the method described by López-Blanco et al. (2020) using the marginal distribution sampling (MDS) algorithm in the REddyProc gap-filling tool (Reichstein et al., 2016), which was readapted from Reichstein et al. (2005). Finally, NEE was separated into GPP and Re by applying the REddyProc partitioning algorithm (Reichstein et al., 2016) for further analyses. This partitioning method is based on the exponential regression of night-time respiration with temperature using the Lloyd–Taylor function (Lloyd and Taylor, 1994). Night-time periods were selected via current combined solar radiation and potential radiation thresholds based on the exact solar time, latitude, and longitude. REddyProc estimates the temperature sensitivity from a short-term period, and based on this short-term temperature sensitivity, it estimates the reference temperature in the continuous period of the entire dataset. These estimates were then used to calculate Re for daytime and night-time, while GPP was estimated based on the difference between NEE and Re.
2.4 Identifying the importance of environmental drivers
In order to characterize how environmental conditions impact diurnal and seasonal variability of NEE, GPP and Re at this alpine swamp meadow, we used a novel method based on machine learning. Random forest (Breiman, 2001) is a machine-learning technique that can be used to quantify and interpret the contribution of environmental drivers (covariates) to the variability of different C fluxes (response variables) by combining multiple individual regression trees. This technique has been increasingly utilized to upscale global C fluxes from eddy covariance data (Zeng et al., 2020) but also to evaluate controls on C cycle processes (Zhang et al., 2017; López-Blanco et al., 2017, 2020). Here, we calculate the relative importance of air temperature (Ta), net radiation (Rn), soil water content (SWC), and vapour pressure deficit (VPD) controlling the C sink strength, photosynthesis, and respiration variability. This random forest algorithm constructs multiple (1000 in this analysis) decision trees during training time with different random subsamples (with replacement) from the same input training dataset. In each cluster classified by random forest, the algorithm generates a multiple linear regression to characterize different C fluxes as a function of environmental drivers (López-Blanco et al., 2017, 2020). This algorithm version (Pedregosa et al., 2011) estimates the relative importance of each covariate between 0 % and 100 %, which correspond to the fraction of decision participating during data clustering. We used the random forest algorithm to evaluate the diurnal and seasonal patterns of the relative importance of Ta, Rn, SWC, and VPD responsible for the variability of C fluxes. We used data from the June–September period aggregated per hour, and we ran multiple random forests with growing season data binned per hour of the day, day of the year, and yearly, respectively (Table S1 in the Supplement).
Since C fluxes are affected by plant phenology and climate factors, including temperature, soil moisture, and radiation simultaneously, to analyse the effect of a single factor, ideally, other factors need to be identical or at least close (no significant differences). In each comparison, data of the same period in each year were selected to exclude the influence of plant phenology. To further analyse the effect of soil moisture, radiation, and temperature on C fluxes, we selected a specific group of data for further evaluation other than the entire observation time. The group of data contains two late growing season periods: periods with a significant difference in SWC but almost identical Ta and Rn (i.e. late growing season of 2014 vs. 2015) and periods with a significant difference in Ta but almost identical SWC and Rn (i.e. late growing season of 2014 vs. 2018). Additionally, in order to analyse the effect of annual temperature on C fluxes, we selected a group of time stamps with significant differences in Ta but almost identical SWC and Rn (i.e. 2017 vs. 2014 and 2018 vs. 2014). The magnitude of the differences between C fluxes in the same group was analysed by the independent-sample t test method.
3.1 Meteorological variability
Mean daily meteorological variables (including Ta, P, SWC, and Rn) exhibited evident seasonal variability except for VPD; these variables increased progressively in the early growing seasons, reached their maximum in July, and decreased gradually afterwards (Fig. 2a, b, c, d). Air temperature during the growing season was 7.7 ± 2.6, 7.4 ± 2.6, 8.5 ± 2.9, and 9.2 ± 3.3∘C in 2014, 2015, 2017, and 2018, respectively, while precipitation totalled 662.8, 521.4, 661.2, and 624.3 mm for the same years, falling primarily during the growing season. The precipitation measured during the late growing season of 2015 was only half of the amount measured in 2014, 2017, and 2018. The lower precipitation regime led to a marked decline in SWC, making the late growing season of 2015 the driest period among all growing seasons during the study period. The greatest difference in SWC occurred in the late growing season of 2014 and 2015, when Ta values at the same period were very close (Ta was 6.8 ± 2.6 and 6.8 ± 2.5 ∘C in 2014 and 2015, respectively). Compared to 2014, SWC decreased by 15.4% in the late growing season of 2015 (Fig. 2b; Table S2). Meanwhile, SWC in the late growing season of 2014 and 2018 was almost identical (80.7 ± 4.1 and 80.8 ± 3.8, respectively), but the temperature difference was the largest (25 %) compared to any other years (Fig. 2a; Table S2).
3.2 Diurnal and seasonal variability of CO2 fluxes
During the growing season, NEE (Fig. 3a1–d1) and GPP (Fig. 3a3–d3) featured a clear peak of the diurnal variations; both fluxes reached their summit between 12:00 and 14:00 local time. Re, however, presented a lower daily variability. The rates of NEE, Re, and GPP during the growing season averaged −2.3 ± 0.3, 3.2 ± 1.0, and 5.5 ± 0.9 µmol m−2 s−1, respectively, for the entire study period (2014, 2015, 2017, and 2018). For the late growing seasons, the lowest rate of net C uptake was measured in 2015 (−10.0 µmol m−2 s−1), whereas 2014 (−12.4 µmol m−2 s−1), 2017 (−12.2 µmol m−2 s−1), and 2018 (−12.5 µmol m−2 s−1) exhibited more negative NEE values (i.e. stronger net C uptake rate). Between the late growing season in 2015 and the late growing seasons in 2014, 2017, and 2018, there was a significant difference in the rates of Re (p<0.01), while no significant difference was found in GPP variability (p>0.05), suggesting that Re may be the component causing the difference observed in NEE. Specifically, the rates of Re in the late growing season of 2014 and 2015 were 2.4 ± 0.2 and 3.0 ± 0.2 µmol m−2 s−1, respectively, which indicated that the drier conditions in 2015 generated a 25 % higher Re compared to 2014. In addition, the rate of Re in the late growing season was significantly higher in warmer 2018 (3.5 ± 0.2 µmol m−2 s−1) than in 2014 (2.4 ± 0.2 µmol m−2 s−1).
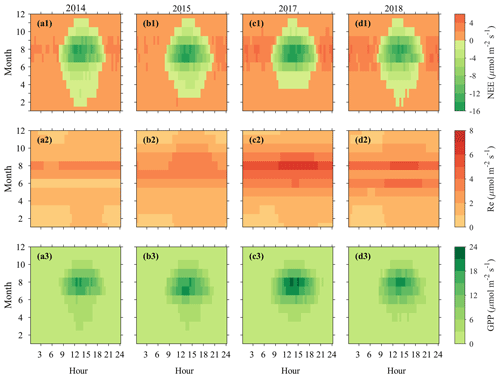
Figure 3Diurnal variability of gap-filled NEE and partitioned Re and GPP in 2014, 2015, 2017, and 2018.
NEE, Re, and GPP also exhibited a strong seasonal variability; the C fluxes gradually increased from low values in early June to the maximum in the middle of the growing season (late July to early August on average), followed by a decrease towards the end of the growing season (Fig. 4a, b, c). The C sink strength across the growing season was found lowest in 2018, followed by 2017, whereas 2014 and 2015 exhibited relatively higher values (Fig. 4a). Notably, the accumulated Re in the late growing season was significantly higher in 2015 compared to 2014 (P<0.05), while there was no significant difference in GPP (Fig. 4b; Table S2). Moreover, the late growing season of 2015 witnessed the lowest SWC while keeping the same Ta compared to the same period in 2014 (Fig. 2b; Table S2). The substantial decline of SWC observed in 2015 appeared to be responsible for the weaker observed C sink strength. On the other hand, Ta in the late growing season of 2018 was the highest for the same period among all the years, while the SWC remained the same as 2014 (Fig. 2a). The late growing season of 2018 showed overall higher GPP and Re but lower net C uptake than 2014. Significantly higher Re in 2018 caused by higher temperatures eventually led to a decrease in the C sink capacity (Fig. 4a, b, c; Table S2).
3.3 The importance of environmental forcing controlling C fluxes
Our data processed by a machine-learning technique suggest that the relative importance of the primary environmental drivers (Rn, Ta, VPD, and SWC) regulating C fluxes varies on different timescales (i.e. diurnal and seasonal scales) in this swamp meadow of the QTP (Fig. 5a, b, c). The diurnal variability of NEE and GPP was mostly driven by Ta (Fig. 5a), especially in the central hours of the day between 11:00 and 15:00, while Re showed a fairly lower temperature dependence compared to NEE and GPP (Fig. 5a). SWC was relatively more important than air temperature controlling the diurnal variability of respiration (Fig. 5a). The seasonal variability shaping the terrestrial C fluxes are regulated not only by meteorological variables but also by plant phenology. To separate the role of meteorological variables from phenology, we carried out a random forest analysis every fortnight and assumed that plant phenology changed little during this time span (Fig. 5b; seasonal variability). The analyses based on random forest revealed a distinct seasonal pattern for different C fluxes from June to September, pointing to a marked contribution of net radiation over NEE and GPP (Fig. 5b). Re, however, was found mostly regulated by SWC. The contribution patterns of each environmental driver to the variations of C fluxes on an inter-seasonal scale (Fig. 5c) are similar to the ones found at the seasonal scale (Fig. 5b). The inter-seasonal variability of NEE and GPP was explained clearly by Rn, while SWC revealed a stronger relative importance over Re. Overall, SWC dynamics seem to be the most important variable explaining the variability observed in the Re data, suggesting that soil moisture plays an essential role on a diurnal and seasonal basis in this cold swamp meadow ecosystem. Note also that Ta played a secondary role in regulating Re at all assessed timescales (Fig. 5a, b, c).
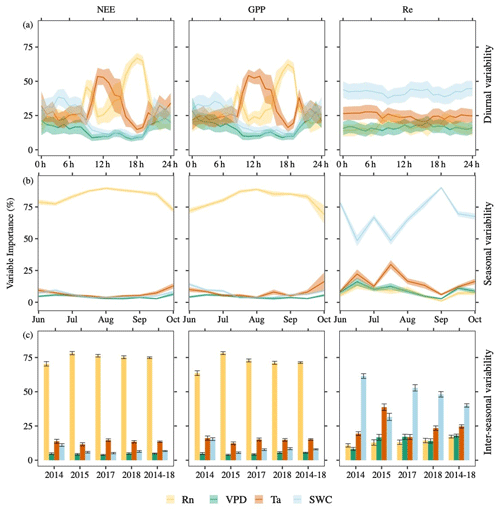
Figure 5Contribution to diurnal and seasonal variation of NEE, GPP, and Re from different environmental drivers (Rn (yellow), Ta (orange), SWC (blue), and VPD (green)). Solid lines with shades (diurnal and seasonal variability) and bars with error bars (inter-seasonal variability) both illustrate the average ± standard deviation of the importance across 1000 decision trees. Inter-seasonal variability refers to the variability of the integrated growing season of 2014, 2015, 2017, and 2018.
Since NEE is the difference between Re and GPP, environmental variables affecting Re and GPP could affect NEE indirectly (Song et al., 2011). Radiation affects the magnitude of plant photosynthesis and controls temperature, one of the key factors related to C fluxes. Abundant radiation benefits photosynthesis and respiration and thus directly affects the C sink strength of an alpine wetland ecosystem in the Qinghai Lake basin (Cao et al., 2017). In this study, radiation has been identified as the most important factor in regulating diurnal and seasonal variations of GPP and NEE, but soil moisture contributed the most to the variation of Re. On an annual scale, Niu et al. (2017) show that 99 % of the interannual variation of NEE in an alpine swamp meadow can be well explained by temperature conditions, precipitation, and radiation. The results of this study demonstrate that ecosystem C sequestration is regulated not only by radiation and temperature but also by soil moisture in the alpine swamp meadow site studied herein. Given there were no significant differences in net radiation among the 4 years we studied, the effects of SWC and temperature on C fluxes on diurnal and seasonal scales are therefore discussed in detail below.
4.1 Low soil moisture is associated with enhanced ecosystem respiration
A previous study in alpine meadow ecosystems found that water stress may be the key limiting factor leading to a decline in the photosynthetic rate at noon with a low SWC of 6 %–21 % (Zhang et al., 2018). In this alpine swamp meadow, the soil layer maintained a relatively high SWC due to the frequent precipitation during the growing season. SWC was always greater than 70 % during the entire study period (Fig. 2b). Therefore, microbial activity and thus heterotrophic respiration were likely suppressed by the anaerobic environment due to saturated soil water condition (Chimner and Cooper, 2003; Sun et al., 2021). At this site, SWC was found to be a more important factor than temperature in controlling the variability of Re at different time aggregations (Fig. 5a, b, c). This result suggests that even under water-saturated conditions, the C dynamics of this alpine swamp meadow are still highly sensitive to changes in soil moisture and could therefore be significantly influenced by future changes in water supply (Li et al., 2015). In fact, previous studies have stressed that soil moisture will likely interact with temperature to affect Re (Han et al., 2013) and therefore modify the overall C sink strength.
To better understand the underlying mechanisms around how SWC interacts with the C fluxes in the studied alpine swamp meadow ecosystem, we selected two late growing season periods, which have significant differences in SWC but no significant difference in Ta (Fig. 6a; Table S2).
The most significant difference in C fluxes between the late growing season of 2014 and 2015 was observed in Re (p<0.05). Additionally, on both diurnal and seasonal scales, a 15.4 % decrease in SWC in the late growing season of 2015 resulted in a 25.7 % increase in Re and a 19.4 % decrease in net C uptake compared to 2014 (Fig. 6a, b; Table S2). According to Jansson and Hofmockel (2020), the intensification of anaerobic conditions due to water saturated soil can be responsible for weaker respiratory losses. This finding suggests that drier conditions in 2015 likely prevented this alpine swamp meadow from water-logged states, thereby strengthening soil respiration due to improved soil aeration (Wang et al., 2014).
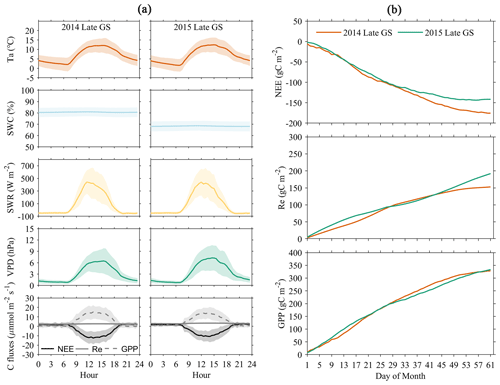
Figure 6(a) Comparisons of the diurnal variations of environmental drivers (Ta, SWC, Rn, and VPD) and C fluxes (NEE, Re, and GPP) between the late growing season of 2014 and 2015. The shading represents the mean ± standard deviation of the presented variables. (b) Comparisons of the daily accumulated C fluxes (NEE, Re, and GPP) between the late growing season of 2014 and 2015. Note thats late GS represents late (August–September) growing season.
Although the SWC in September 2015 was much greater than the 6 %–21 % range reported by Zhang et al. (2018), our data suggest that a 22.2 % reduction in SWC in September 2015 resulted in a 51.6 % decline in net C uptake compared to September 2014 (Fig. S1; Table S3). There is evidence from the literature that the rates of net C uptake in alpine wetlands during the growing season can be lower under drier conditions (Hao et al., 2011), indicating that this alpine swamp meadow ecosystem may be adapted to high levels of SWC (Li et al., 2015). Higher SWC may limit the diffusion of oxygen from the atmosphere to the soil, inhibiting the activity of microorganisms and reducing the decomposition rate of soil organic matter (Chimner and Cooper, 2003). Our comparisons suggest that drying can weaken the overall C sink strength in this alpine swamp meadow ecosystem. Wetlands are predicted to experience lower water tables due to permafrost degradation in the Tibetan Plateau, and, therefore, permafrost thaw-induced wetland drying could enhance the response of C emissions to climate warming (Yu et al., 2020).
4.2 Temperature increase leads to higher C losses
The important role played by temperature controlling C exchange has been extensively found in alpine marshland across the QTP (Qi et al., 2021). For example, Zhao et al. (2010) and Zhao et al. (2005) show that Re follows the exponential variation of soil temperature. Zhu et al. (2020) also suggested that soil temperature plays the most important role in the change of monthly Re in the alpine wetland at Luanhaizi, northeastern Qinghai–Tibetan Plateau. We therefore explored another comparison between the late growing season of 2014 and 2018 (Fig. 7a, b; Table S2) when phenology, radiation, and SWC were almost identical at both periods but temperature differed. Compared to 2014, a 25 % increase in Ta in the late growing season of 2018 led to joint larger GPP and Re fluxes (Fig. 7a, b; Table S2). Although both GPP and Re increased, the intensification in Re was greater than GPP, indicating that warmer temperatures had a stronger impact on Re, resulting in a decrease in the net C uptake (Fig. 7b; Table S2). To evaluate if this finding is also consistent at an annual scale, we further analysed annual aggregated data. An annual comparison was made between 2014, 2017, and 2018 when SWC was found insignificantly different while temperatures in 2017 and 2018 were 44.4 % higher than in 2014 (Table S4). Again, this 44.4 % increase in Ta in 2017 and 2018 both led to stronger GPP and Re (Table S4). Although both GPP and Re increased, the intensity in Re was greater than GPP, indicating that warmer temperatures have a stronger impact on Re at this site, resulting in an approximately 50 % decrease in the net C uptake (Table S4).
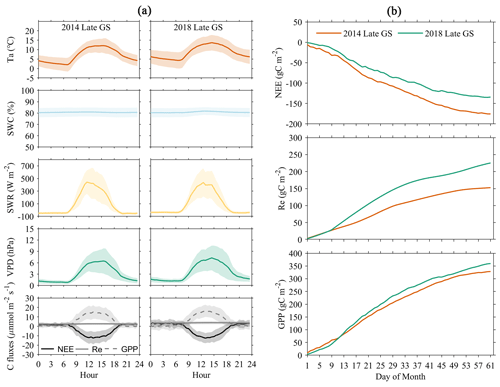
Figure 7(a) Comparisons of the diurnal variations of environmental drivers (Ta, SWC, Rn, and VPD) and C fluxes (NEE, Re, and GPP) between the late growing season of 2014 and 2018. The shading represents the mean ± standard deviation of the presented variables. (b) Comparisons of the daily accumulated C fluxes (NEE, Re, and GPP) between the late growing season of 2014 and 2018. Note that late GS represents late (August–September) growing season.
This comparison suggests that future warming could weaken the overall C sink strength in this alpine swamp meadow ecosystem. Similar climate sensitivities have also been found in recent studies. For example, a study performed by Niu et al. (2017) in an alpine swamp meadow on the central Tibetan Plateau suggests Re was more sensitive to increased temperature than GPP. This suggests that global warming may exacerbate future C releases in the alpine wetlands of the QTP (Gao et al., 2019; Niu et al., 2017; Zhu et al., 2020). Liu et al. (2018) concluded that warming has a significant inhibitory effect on GPP and a minor effect on Re, resulting in a weaker carbon sequestration capacity of their studied alpine wetland ecosystem. However, other researchers have also reached different conclusions. For instance, Qi et al. (2021) found that GPP is consistently more sensitive than Re to changes of temperature at daily, seasonal, and annual scales, suggesting that cold condition can act as a strong constraint on C uptake in alpine marshlands. Wei et al. (2021) also found that the uptake of C by plants will exceed the amount of C release under warmer and wetter climate conditions based on manipulative experiments and model simulations for the Tibetan Plateau. Their study is based on a longer-term trend while our study only covers 4 years of year-round observations; thus, site-specific differences in time and space scales may explain this variability. These inconsistent ecosystem responses suggest that there are still large uncertainties regulating the responses of C fluxes to temperature variation, and further work is still crucial.
4.3 Combined effects of temperature and soil moisture on C exchange dynamics
The QTP experienced a higher rate of temperature increase than that of the Northern Hemisphere average (Zhang et al., 2013). The effects triggered by climate-induced warming over NEE in this area have been argued to either increase or decrease the net C balance NEE or even have no effect whatsoever (Ganjurjav et al., 2018; Li et al., 2020; Wu et al., 2011; Zhu et al., 2017). These inconsistent responses could be due to water limitations offsetting the C balance or even reversing the effect of elevated temperatures, which change the decomposition and photosynthetic processes (Wu et al., 2011; Yu et al., 2013; J. Zhao et al., 2019). Alpine swamp meadows of the QTP have recently attracted much attention because they hold 5.9 % (∼ 1.98 Pg C) of the total grassland soil organic C (∼ 33.52 Pg C). Such ecosystems have the highest organic C density (∼ 50 kg C m−2) and play an important role in the global C cycle (Niu et al., 2017). To test whether the observed SWC effects in this study were representative of other sites on the QTP and put it into a broader perspective, we examined the temperature and precipitation (as a proxy for SWC) impacts on NEE (Liu et al., 2016).
Table 2Comparison of annual NEE (g C m−2 yr−1) at different sites in the QTP.
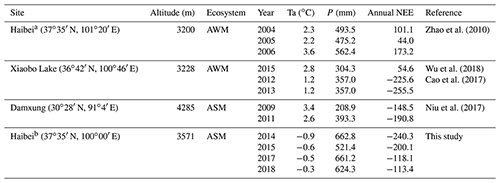
Note that P denotes precipitation, AWM denotes alpine wetland meadow, and ASM denotes alpine swamp meadow; Haibeia and Haibeib denote different sites.
The NEE observations from this study were within the NEE ranges of previous studies in similar ecosystems located across the QTP (−255.5 to 173.2 g C m−2 yr−1) (Table 2). According to Wei et al. (2021), there are six observational studies about C flux around our study site, and three of them are focused on alpine swamp meadows. Among them, one study had a 1-year dataset (Zhang et al., 2008), and the other two characterized the same location (Zhao et al., 2005, 2010). The three studies were reported as a net C source, while our 4-year dataset revealed that this alpine swamp meadow functioned as a net C sink of −168.0 ± 62.5 g C m−2 yr−1 at a 3571 m a.s.l. The different directions of C exchange suggest that there are still uncertainties in our understanding of C exchange in alpine swamp meadows, and further efforts are still needed to improve our projection of C balance change of this ecosystem under changing climate.
In addition, the NEE estimates of this alpine swamp meadow show a stronger C sink strength than those from alpine meadows (−161.3 to 85.4 g C m−2 yr−1) (Chai et al., 2017; Wang et al., 2017; Wu et al., 2020), alpine steppes (−30 to 21.8 g C m−2 yr−1) (Wang et al., 2018; D. Wang et al., 2020; Y. Wang et al., 2020; Wu et al., 2010), and alpine shrublands (−14 to −67 g C m−2 yr−1) (Zhao et al., 2005, 2006). This is likely a result of the inhibiting effects of the nearly saturated soil condition over soil respiration (Sun et al., 2021). In permanently or seasonally inundated swamp meadows, high SWC may have triggered lower C loss rates and further benefited C preservation. At our site, the higher C sink strength was likely attributed to higher precipitation (and therefore higher SWC) and lower temperature, which created colder and more humid conditions than other sites (Table 2). It has been demonstrated that cold and humid conditions favour stronger C sinks in alpine meadow ecosystems (Fu et al., 2009).
The interannual comparison of the sites presented in Table 2 shows that under low annual precipitation conditions (∼ 300 mm), the joint effects of warming and reduced precipitation weakened the net C uptake at Damxung (Niu et al., 2017) and even turned the C sink of Xiaobo Lake wetland into a C source when comparing 2015 with 2012 and 2013 (Cao et al., 2017; Wu et al., 2018). Under relatively high annual precipitation (∼ 500 mm), the joint effects of warming and increased precipitation enhanced C release in the Haibeia site (see Table 2) when comparing 2006 to 2004 and 2005 (Zhao et al., 2010). This indicates that net C uptake under warming conditions can be weakened even under high annual precipitation rates.
The alpine swamp meadow from the QTP presented in this study has been found to act as a consistent and strong sink of CO2 (−168.0 ± 62.5 g C m−2 yr−1). The results from a novel machine-learning technique revealed that air temperature is the most important variable driving NEE and GPP on a diurnal scale, while net radiation has a stronger importance controlling the seasonal variability of the same fluxes. Soil moisture, however, has the largest influence over Re variability on diurnal and seasonal scales, suggesting that SWC is a key control on Re. In addition, air temperature played a less important role in regulating Re. This study reveals that both drying and warming can suppress net C uptake in water-saturated alpine swamp meadow ecosystems by enhancing Re. The response of net C uptake to climate warming further indicates that the forecasted warming in the QTP will not always increase the net C sink strength. Our results not only highlight the contributions of soil moisture in regulating C sequestration under high water conditions but also support future process-based modelling initiatives focusing on alpine swamp meadow ecosystem C dynamics.
Post-processed data and scripts used in this paper are available from the authors upon request (xyli@bnu.edu.cn).
The supplement related to this article is available online at: https://doi.org/10.5194/bg-19-861-2022-supplement.
JW, XL, LL, TRC, XW, and ELB designed the research. JW, LL, and ELB processed the data and performed the analyses. YM and HY helped collect the data. JW and ELB created the visualization of the outputs. JW, XL, and ELB prepared the manuscript with contributions (writing, review, and editing) from LL, TRC, and ZJ.
The contact author has declared that neither they nor their co-authors have any competing interests.
Publisher’s note: Copernicus Publications remains neutral with regard to jurisdictional claims in published maps and institutional affiliations.
We thank Trevor Keenan and two anonymous reviewers for their insightful comments, which significantly improved the paper. We also thank Viola Zierenberg for the effort in proofreading the paper.
The study was financially supported by the National Natural Science Foundation of China (NSFC 41730854 and 41971029), the Second Tibetan Plateau Scientific Expedition and Research Program (STEP 2019QZKK0306), the Strategic Priority Research Program of Chinese Academy of Sciences (XDA20100102), and projects from the State Key Laboratory of Earth Surface Processes and Resource Ecology. This study also received financial support from the China Scholarship Council (no. 201906040130) and the Faculty of Technical Sciences from Aarhus University. Additionally, Efrén López-Blanco was funded by the Greenland Research Council, grant number 80.35, financed by the Danish Program for Arctic Research.
This paper was edited by Trevor Keenan and reviewed by two anonymous referees.
Andresen, C. G., Lawrence, D. M., Wilson, C. J., McGuire, A. D., Koven, C., Schaefer, K., Jafarov, E., Peng, S., Chen, X., Gouttevin, I., Burke, E., Chadburn, S., Ji, D., Chen, G., Hayes, D., and Zhang, W.: Soil moisture and hydrology projections of the permafrost region – a model intercomparison, The Cryosphere, 14, 445–459, https://doi.org/10.5194/tc-14-445-2020, 2020.
Bai, W., Xi, J., and Wang, G.: Effects of short-term warming and nitrogen addition on CO2 emission during growing season in an alpine swamp meadow ecosystem of Qinghai-Tibetan Plateau, Chin. J. Ecol., 38, 927–936, https://doi.org/10.13292/j.1000-4890.201904.001, 2019.
Biskaborn, B. K., Smith, S. L., Noetzli, J., Matthes, H., Vieira, G., Streletskiy, D. A., Schoeneich, P., Romanovsky, V. E., Lewkowicz, A. G., Abramov, A., Allard, M., Boike, J., Cable, W. L., Christiansen, H. H., Delaloye, R., Diekmann, B., Drozdov, D., Etzelmuller, B., Grosse, G., Guglielmin, M., Ingeman-Nielsen, T., Isaksen, K., Ishikawa, M., Johansson, M., Johannsson, H., Joo, A., Kaverin, D., Kholodov, A., Konstantinov, P., Kroger, T., Lambiel, C., Lanckman, J. P., Luo, D., Malkova, G., Meiklejohn, I., Moskalenko, N., Oliva, M., Phillips, M., Ramos, M., Sannel, A. B. K., Sergeev, D., Seybold, C., Skryabin, P., Vasiliev, A., Wu, Q., Yoshikawa, K., Zheleznyak, M., and Lantuit, H.: Permafrost is warming at a global scale, Nat. Commun., 10, 264, https://doi.org/10.1038/s41467-018-08240-4, 2019.
Bohn, T. J., Lettenmaier, D. P., Sathulur, K., Bowling, L. C., Podest, E., McDonald, K. C., and Friborg, T.: Methane emissions from western Siberian wetlands: heterogeneity and sensitivity to climate change, Environ. Res. Lett., 2, 045015, https://doi.org/10.1088/1748-9326/2/4/045015, 2007.
Breiman, L.: Random forests, Mach. Learn., 45, 5–32, https://doi.org/10.1023/a:1010933404324, 2001.
Cao, S. K., Cao, G. C., Feng, Q., Han, G. Z., Lin, Y. Y., Yuan, J., Wu, F. T., and Cheng, S. Y.: Alpine wetland ecosystem carbon sink and its controls at the Qinghai Lake, Environ. Earth Sci., 76, 210, https://doi.org/10.1007/s12665-017-6529-5, 2017.
Chai, X., Shi, P. L., Zong, N., Niu, B., He, Y. T., and Zhang, X. Z.: Biophysical regulation of carbon flux in different rainfall regime in a northern Tibetan alpine meadow, J. Resour. Ecol., 8, 30–41, https://doi.org/10.5814/j.issn.1674-764x.2017.01.005, 2017.
Chen, D., Xu, B., Yao, T., Guo, Z., Cui, P., Chen, F., Zhang, R., Zhang, X., Zhang, Y., Fan, J., Hou, Z., and Zhang, T.: Assessment of past, present and future environmental changes on the Tibetan Plateau, Chinese Sci. Bull., 60, 3025–3035, https://doi.org/10.1360/n972014-01370, 2015.
Cheng, Z., Liu, X., Fan, G., Bai, A., and Wang, B.: Spatiotemporal Distribution of Climate Change over the Qinghai-Tibetan Plateau in 21st Century, Arid Zone Res., 28, 669–676, 2011.
Chimner, R. A. and Cooper, D. J.: Influence of water table levels on CO2 emissions in a Colorado subalpine fen: an in-situ microcosm study, Soil Biol. Biochem., 35, 345–351, https://doi.org/10.1016/s0038-0717(02)00284-5, 2003.
Christensen, T. R., Ekberg, A., Strom, L., Mastepanov, M., Panikov, N., Oquist, M., Svensson, B. H., Nykanen, H., Martikainen, P. J., and Oskarsson, H.: Factors controlling large scale variations in methane emissions from wetlands, Geophys. Res. Lett., 30, 1414, https://doi.org/10.1029/2002gl016848, 2003.
Foti, R., del Jesus, M., Rinaldo, A., and Rodriguez-Iturbe, I.: Signs of critical transition in the Everglades wetlands in response to climate and anthropogenic changes, P. Natl. Acad. Sci. USA, 110, 6296–6300, https://doi.org/10.1073/pnas.1302558110, 2013.
Fu, G., Shen, Z.-X., Sun, W., Zhong, Z.-M., Zhang, X.-Z., and Zhou, Y.-T.: A Meta-analysis of the Effects of Experimental Warming on Plant Physiology and Growth on the Tibetan Plateau, J. Plant Growth Regul., 34, 57–65, https://doi.org/10.1007/s00344-014-9442-0, 2015.
Fu, Y., Zheng, Z., Yu, G., Hu, Z., Sun, X., Shi, P., Wang, Y., and Zhao, X.: Environmental influences on carbon dioxide fluxes over three grassland ecosystems in China, Biogeosciences, 6, 2879–2893, https://doi.org/10.5194/bg-6-2879-2009, 2009.
Ganjurjav, H., Gao, Q., Gornish, E. S., Schwartz, M. W., Liang, Y., Cao, X., Zhang, W., Zhang, Y., Li, W., Wan, Y., Li, Y., Danjiu, L., Guo, H., and Lin, E.: Differential response of alpine steppe and alpine meadow to climate warming in the central Qinghai-Tibetan Plateau, Agr. Forest Meteorol., 223, 233–240, https://doi.org/10.1016/j.agrformet.2016.03.017, 2016.
Ganjurjav, H., Hu, G., Wan, Y., Li, Y., Danjiu, L., and Gao, Q.: Different responses of ecosystem carbon exchange to warming in three types of alpine grassland on the central Qinghai-Tibetan Plateau, Ecol. Evol., 8, 1507–1520, https://doi.org/10.1002/ece3.3741, 2018.
Gao, Y., Quan, M. Y., Shen, W. D., and Ren, X. D.: Research on experimental warming effects of CO2 emissions and environmental response mechanisms in an alpine swamp meadow ecosystem on the Qinghai-Tibet Plateau, J. Guangxi Uni. (Nat. Sci. Ed.), 44, 580–586, https://doi.org/10.13624/j.cnki.issn.1001-7445.2019.0580, 2019.
Han, G., Yang, L., Yu, J., Wang, G., Mao, P., and Gao, Y.: Environmental Controls on Net Ecosystem CO2 Exchange Over a Reed (Phragmites australis) Wetland in the Yellow River Delta, China, Estuar. Coast, 36, 401–413, https://doi.org/10.1007/s12237-012-9572-1, 2013.
Hao, Y. B., Cui, X. Y., Wang, Y. F., Mei, X. R., Kang, X. M., Wu, N., Luo, P., and Zhu, D.: Predominance of Precipitation and Temperature Controls on Ecosystem CO2 Exchange in Zoige Alpine Wetlands of Southwest China, Wetlands, 31, 413–422, https://doi.org/10.1007/s13157-011-0151-1, 2011.
Hruby, T.: Assessments of Wetland Functions: What They Are and What They Are Not, Environ. Manage., 23, 75–85, https://doi.org/10.1007/s002679900168, 1999.
Jansson, J. K. and Hofmockel, K. S.: Soil microbiomes and climate change, Nat. Rev. Microbiol., 18, 35–46, https://doi.org/10.1038/s41579-019-0265-7, 2020.
Kang, X., Wang, Y., Chen, H., Tian, J., Cui, X., Rui, Y., Zhong, L., Kardol, P., Hao, Y., and Xiao, X.: Modeling Carbon Fluxes Using Multi-Temporal MODIS Imagery and CO2 Eddy Flux Tower Data in Zoige Alpine Wetland, South-West China, Wetlands, 34, 603–618, https://doi.org/10.1007/s13157-014-0529-y, 2014.
Lafrenière, M. J. and Lamoureux, S. F.: Effects of changing permafrost conditions on hydrological processes and fluvial fluxes, Earth-Sci. Rev., 191, 212–223, https://doi.org/10.1016/j.earscirev.2019.02.018, 2019.
Li, C., He, H., Liu, M., Su, W., Fu, Y., Zhang, L., Wen, X., and Yu, G.: The design and application of CO2 flux data processing system at ChinaFLUX, Geo-Info. Sci., 10, 557–565, https://doi.org/10.3969/j.issn.1560-8999.2008.05.002, 2008.
Li, C., Yang, Y., Li, X., Chen, Q., and Zhou, H.: Effects of Simulated Climate Warming and Grazing on Photosynthesis and Respiration of Permafrost Meadow Plant Community, Russ. J. Ecol+, 51, 224–232, https://doi.org/10.1134/s1067413620030042, 2020.
Li, H., Nicotra, A. B., Xu, D., and Du, G.: Habitat-specific responses of leaf traits to soil water conditions in species from a novel alpine swamp meadow community, Conserv. Physiol., 3, cov046, https://doi.org/10.1093/conphys/cov046, 2015.
Liu, D., Li, Y., Wang, T., Peylin, P., MacBean, N., Ciais, P., Jia, G., Ma, M., Ma, Y., Shen, M., Zhang, X., and Piao, S.: Contrasting responses of grassland water and carbon exchanges to climate change between Tibetan Plateau and Inner Mongolia, Agr. Forest Meteorol., 249, 163–175, https://doi.org/10.1016/j.agrformet.2017.11.034, 2018.
Liu, L., Wang, X., Lajeunesse, M. J., Miao, G., Piao, S., Wan, S., Wu, Y., Wang, Z., Yang, S., Li, P., and Deng, M.: A cross-biome synthesis of soil respiration and its determinants under simulated precipitation changes, Glob. Change Biol., 22, 1394–1405, https://doi.org/10.1111/gcb.13156, 2016.
Liu, S. M., Xu, Z. W., Wang, W. Z., Jia, Z. Z., Zhu, M. J., Bai, J., and Wang, J. M.: A comparison of eddy-covariance and large aperture scintillometer measurements with respect to the energy balance closure problem, Hydrol. Earth Syst. Sci., 15, 1291–1306, https://doi.org/10.5194/hess-15-1291-2011, 2011.
Liu, X. W., Zhu, D., Zhan, W., Chen, H., Zhu, Q. A., Hao, Y. B., Liu, W. J., and He, Y. X.: Five‐year measurements of net ecosystem CO2 exchange at a fen in the Zoige peatlands on the Qinghai‐Tibetan Plateau, J. Geophys. Res.-Atmos., 124, 11803–11818, https://doi.org/10.1029/2019JD031429, 2019.
Liu, Y., Geng, X., Tenzintarchen, Wei, D., Dai, D., and Xu, R.: Divergence in ecosystem carbon fluxes and soil nitrogen characteristics across alpine steppe, alpine meadow and alpine swamp ecosystems in a biome transition zone, Sci. Total Environ., 748, 142453, https://doi.org/10.1016/j.scitotenv.2020.142453, 2020.
Lloyd, J. and Taylor, J. A.: On the Temperature-Dependence of Soil Respiration, Funct. Ecol., 8, 315–323, https://doi.org/10.2307/2389824, 1994.
López-Blanco, E., Lund, M., Williams, M., Tamstorf, M. P., Westergaard-Nielsen, A., Exbrayat, J.-F., Hansen, B. U., and Christensen, T. R.: Exchange of CO2 in Arctic tundra: impacts of meteorological variations and biological disturbance, Biogeosciences, 14, 4467–4483, https://doi.org/10.5194/bg-14-4467-2017, 2017.
López-Blanco, E., Lund, M., Christensen, T. R., Tamstorf, M. P., Smallman, T. L., Slevin, D., Westergaard-Nielsen, A., Hansen, B. U., Abermann, J., and Williams, M.: Plant Traits are Key Determinants in Buffering the Meteorological Sensitivity of Net Carbon Exchanges of Arctic Tundra, J. Geophys. Res.-Biogeo., 123, 2675–2694, https://doi.org/10.1029/2018jg004386, 2018.
López-Blanco, E., Jackowicz-Korczynski, M., Mastepanov, M., Skov, K., Westergaard-Nielsen, A., Williams, M., and Christensen, T. R.: Multi-year data-model evaluation reveals the importance of nutrient availability over climate in arctic ecosystem C dynamics, Environ. Res. Lett., 15, 094007, https://doi.org/10.1088/1748-9326/ab865b, 2020.
Moffat, A. M., Papale, D., Reichstein, M., Hollinger, D. Y., Richardson, A. D., Barr, A. G., Beckstein, C., Braswell, B. H., Churkina, G., Desai, A. R., Falge, E., Gove, J. H., Heimann, M., Hui, D., Jarvis, A. J., Kattge, J., Noormets, A., and Stauch, V. J.: Comprehensive comparison of gap-filling techniques for eddy covariance net carbon fluxes, Agr. Forest Meteorol., 147, 209–232, https://doi.org/10.1016/j.agrformet.2007.08.011, 2007.
Niu, B., He, Y., Zhang, X., Du, M., Shi, P., Sun, W., and Zhang, L.: CO2 Exchange in an Alpine Swamp Meadow on the Central Tibetan Plateau, Wetlands, 37, 525–543, https://doi.org/10.1007/s13157-017-0888-2, 2017.
Papale, D., Reichstein, M., Aubinet, M., Canfora, E., Bernhofer, C., Kutsch, W., Longdoz, B., Rambal, S., Valentini, R., Vesala, T., and Yakir, D.: Towards a standardized processing of Net Ecosystem Exchange measured with eddy covariance technique: algorithms and uncertainty estimation, Biogeosciences, 3, 571–583, https://doi.org/10.5194/bg-3-571-2006, 2006.
Pedregosa, F., Varoquaux, G., Gramfort, A., Michel, V., Thirion, B., Grisel, O., Blondel, M., Prettenhofer, P., Weiss, R., Dubourg, V., Vanderplas, J., Passos, A., Cournapeau, D., Brucher, M., Perrot, M., and Duchesnay, E.: Scikit-learn: Machine Learning in Python, J. Mach. Learn. Res., 12, 2825–2830, 2011.
Peng, F., You, Q., Xu, M., Guo, J., Wang, T., and Xue, X.: Effects of Warming and Clipping on Ecosystem Carbon Fluxes across Two Hydrologically Contrasting Years in an Alpine Meadow of the Qinghai-Tibet Plateau, Plos One, 9, 109319, https://doi.org/10.1371/journal.pone.0109319, 2014.
Qi, Y., Wei, D., Zhao, H., and Wang, X.: Carbon Sink of a Very High Marshland on the Tibetan Plateau, J. Geophys. Res.-Biogeo., 126, e2020JG006235, https://doi.org/10.1029/2020jg006235, 2021.
Quan, Q., Tian, D., Luo, Y., Zhang, F., Crowther, T. W., Zhu, K., Chen, H. Y. H., Zhou, Q., and Niu, S.: Water scaling of ecosystem carbon cycle feedback to climate warming, Sci. Adv., 5, eaav1131, https://doi.org/10.1126/sciadv.aav1131, 2019.
Reichstein, M., Falge, E., Baldocchi, D., Papale, D., Aubinet, M., Berbigier, P., Bernhofer, C., Buchmann, N., Gilmanov, T., Granier, A., Grunwald, T., Havrankova, K., Ilvesniemi, H., Janous, D., Knohl, A., Laurila, T., Lohila, A., Loustau, D., Matteucci, G., Meyers, T., Miglietta, F., Ourcival, J. M., Pumpanen, J., Rambal, S., Rotenberg, E., Sanz, M., Tenhunen, J., Seufert, G., Vaccari, F., Vesala, T., Yakir, D., and Valentini, R.: On the separation of net ecosystem exchange into assimilation and ecosystem respiration: review and improved algorithm, Glob. Change Biol., 11, 1424–1439, https://doi.org/10.1111/j.1365-2486.2005.001002.x, 2005.
Reichstein, M., Moffat, A. M., Wutzler, T., and Sickel, K.: REddyProc: Data processing and plotting utilities of (half-)hourly eddy-covariance measurements, R package version 0.8-2/r14, https://R-Forge.R-project.org/projects/reddyproc/ (last access: 6 February 2022), 2016.
Saito, M., Kato, T., and Tang, Y.: Temperature controls ecosystem CO2 exchange of an alpine meadow on the northeastern Tibetan Plateau, Glob. Change Biol., 15, 221–228, https://doi.org/10.1111/j.1365-2486.2008.01713.x, 2009.
Schuur, E. A. G., Vogel, J. G., Crummer, K. G., Lee, H., Sickman, J. O., and Osterkamp, T. E.: The effect of permafrost thaw on old carbon release and net carbon exchange from tundra, Nature, 459, 556–559, https://doi.org/10.1038/nature08031, 2009.
Song, C. C., Sun, L., Huang, Y., Wang, Y. S., and Wan, Z. M.: Carbon exchange in a freshwater marsh in the Sanjiang Plain, northeastern China, Agr. Forest Meteorol., 151, 1131–1138, https://doi.org/10.1016/j.agrformet.2011.04.001, 2011.
Sun, S., Che, T., Gentine, P., Chen, Q., Wang, L., Yan, Z., Chen, B., and Song, Z.: Shallow groundwater inhibits soil respiration and favors carbon uptake in a wet alpine meadow ecosystem, Agr. Forest Meteorol., 297, 108254, https://doi.org/10.1016/j.agrformet.2020.108254, 2021.
Taylor, P. G., Cleveland, C. C., Wieder, W. R., Sullivan, B. W., Doughty, C. E., Dobrowski, S. Z., and Townsend, A. R.: Temperature and rainfall interact to control carbon cycling in tropical forests, Ecol. Lett., 20, 779–788, https://doi.org/10.1111/ele.12765, 2017.
Wang, D., Wang, K., Zheng, X., Butterbach-Bahl, K., Diaz-Pines, E., and Chen, H.: Applicability of a gas analyzer with dual quantum cascade lasers for simultaneous measurements of N2O, CH4 and CO2 fluxes from cropland using the eddy covariance technique, Sci. Total Environ., 729, 138784, https://doi.org/10.1016/j.scitotenv.2020.138784, 2020.
Wang, H., Yu, L., Chen, L., Wang, C., and He, J.: Responses of soil respiration to reduced water table and nitrogen addition in an alpine wetland on the Qinghai-Xizang Plateau, Chin. J. Plant Ecol., 38, 619–625, https://doi.org/10.3724/SP.J.1258.2014.00057, 2014.
Wang, L., Liu, H., Sun, J., and Shao, Y.: Biophysical effects on the interannual variation in carbon dioxide exchange of an alpine meadow on the Tibetan Plateau, Atmos. Chem. Phys., 17, 5119–5129, https://doi.org/10.5194/acp-17-5119-2017, 2017.
Wang, L., Liu, H., Shao, Y., Liu, Y., and Sun, J.: Water and CO2 fluxes over semiarid alpine steppe and humid alpine meadow ecosystems on the Tibetan Plateau, Theor. Appl. Climatol., 131, 547–556, https://doi.org/10.1007/s00704-016-1997-1, 2018.
Wang, Y., Zhu, Z., Ma, Y., and Yuan, L.: Carbon and water fluxes in an alpine steppe ecosystem in the Nam Co area of the Tibetan Plateau during two years with contrasting amounts of precipitation, Int. J. Biometeorol., 64, 1183–1196, https://doi.org/10.1007/s00484-020-01892-2, 2020.
Webb, E. K., Pearman, G. I., and Leuning, R.: Correction of Flux Measurements for Density Effects Due to Heat and Water-Vapor Transfer, Q. J. Roy. Meteor. Soc., 106, 85–100, https://doi.org/10.1002/qj.49710644707, 1980.
Wei, D., Qi, Y. H., Ma, Y. M., Wang, X. F., Ma, W. Q., Gao, T. G., H, L., Zhao, H., Zhang, J. X., and Wang, X. D.: Plant uptake of CO2 outpaces losses from permafrost and plant respiration on the Tibetan Plateau, P. Natl. Acad. Sci. USA, 118, e2015283118, https://doi.org/10.1073/pnas.2015283118, 2021.
Wilczak, J. M., Oncley, S. P., and Stage, S. A.: Sonic anemometer tilt correction algorithms, Bound-Lay. Meteorol., 99, 127–150, https://doi.org/10.1023/a:1018966204465, 2001.
Woodward, R. T. and Wui, Y. S.: The economic value of wetland services: a meta-analysis, Ecol. Econ., 37, 257–270, https://doi.org/10.1016/s0921-8009(00)00276-7, 2001.
Wu, F., Cao, S., Cao, G., Han, G., Lin, Y., and Cheng, S.: Variation of CO2 Flux of Alpine Wetland Ecosystem of Kobresia tibetica Wet Meadow in Lake Qinghai, J. Ecol. Rural Environ., 34, 124–131, https://doi.org/10.11934/j.issn.1673-4831.2018.02.004, 2018.
Wu, H., Zhao, G., Li, X. Y., Wang, Y., He, B., Jiang, Z., Zhang, S., and Sun, W.: Identifying water sources used by alpine riparian plants in a restoration zone on the Qinghai-Tibet Plateau: Evidence from stable isotopes, Sci. Total Environ., 697, 134092, https://doi.org/10.1016/j.scitotenv.2019.134092, 2019.
Wu, J., Wu, H., Ding, Y., Qin, J., and Zeng, D.: Interannual and seasonal variations in carbon exchanges over an alpine meadow in the northeastern edge of the Qinghai-Tibet Plateau, China, PloS one, 15, e0228470, https://doi.org/10.1371/journal.pone.0228470, 2020.
Wu, L., Gu, S., Zhao, L., Xu, S., Zhou, H., Feng, C., Xu, W.-X., Li, Y., Zhao, X., and Tang, Y.: Variation in net CO2 exchange, gross primary production and its affecting factors in the planted pasture ecosystem in Sanjiangyuan Region of the Qinghai-Tibetan Plateau of China, Chin. J. Plant Ecol., 34, 521–528, https://doi.org/10.3724/SP.J.1142.2010.40521, 2010.
Wu, Z., Dijkstra, P., Koch, G. W., Penuelas, J., and Hungate, B. A.: Responses of terrestrial ecosystems to temperature and precipitation change: a meta-analysis of experimental manipulation, Glob. Change Biol., 17, 927–942, https://doi.org/10.1111/j.1365-2486.2010.02302.x, 2011.
Yu, G. R., Zhu, X. J., Fu, Y. L., He, H. L., Wang, Q. F., Wen, X. F., Li, X. R., Zhang, L. M., Zhang, L., Su, W., Li, S. G., Sun, X. M., Zhang, Y. P., Zhang, J. H., Yan, J. H., Wang, H. M., Zhou, G. S., Jia, B. R., Xiang, W. H., Li, Y. N., Zhao, L., Wang, Y. F., Shi, P. L., Chen, S. P., Xin, X. P., Zhao, F. H., Wang, Y. Y., and Tong, C. L.: Spatial patterns and climate drivers of carbon fluxes in terrestrial ecosystems of China, Glob. Change Biol., 19, 798–810, https://doi.org/10.1111/gcb.12079, 2013.
Yu, L., Wang, H., Wang, Y., Zhang, Z., Chen, L., Liang, N., and He, J.-S.: Temporal variation in soil respiration and its sensitivity to temperature along a hydrological gradient in an alpine wetland of the Tibetan Plateau, Agr. Forest Meteorol., 282–283, 107854, https://doi.org/10.1016/j.agrformet.2019.107854, 2020.
Zeng, J., Matsunaga, T., Tan, Z. H., Saigusa, N., Shirai, T., Tang, Y., Peng, S., and Fukuda, Y.: Global terrestrial carbon fluxes of 1999–2019 estimated by upscaling eddy covariance data with a random forest, Sci. Data, 7, 313, https://doi.org/10.1038/s41597-020-00653-5, 2020.
Zhang, G., Zhang, Y., Dong, J., and Xiao, X.: Green-up dates in the Tibetan Plateau have continuously advanced from 1982 to 2011, P. Natl. Acad. Sci. USA, 110, 4309–4314, https://doi.org/10.1073/pnas.1210423110, 2013.
Zhang, H., Wu, P., Yin, A., Yang, X., Zhang, M., and Gao, C.: Prediction of soil organic carbon in an intensively managed reclamation zone of eastern China: A comparison of multiple linear regressions and the random forest model, Sci. Total Environ., 592, 704–713, https://doi.org/10.1016/j.scitotenv.2017.02.146, 2017.
Zhang, S. Y.: Energy partitioning and evapotranspiration over the typical ecosystems in the Qinghai Lake watershed, PhD thesis, College of Resources Science and Technology, Beijing Normal University, China, 115 pp., 2014 (n Chinese with English abstract).
Zhang, S.-Y., Li, X.-Y., Zhao, G.-Q., and Huang, Y.-M.: Surface energy fluxes and controls of evapotranspiration in three alpine ecosystems of Qinghai Lake watershed, NE Qinghai-Tibet Plateau, Ecohydrology, 9, 267–279, https://doi.org/10.1002/eco.1633, 2016.
Zhang, T., Zhang, Y., Xu, M., Zhu, J., Chen, N., Jiang, Y., Huang, K., Zu, J., Liu, Y., and Yu, G.: Water availability is more important than temperature in driving the carbon fluxes of an alpine meadow on the Tibetan Plateau, Agr. Forest Meteorol., 256, 22–31, https://doi.org/10.1016/j.agrformet.2018.02.027, 2018.
Zhao, J., Luo, T., Wei, H., Deng, Z., Li, X., Li, R., and Tang, Y.: Increased precipitation offsets the negative effect of warming on plant biomass and ecosystem respiration in a Tibetan alpine steppe, Agr. Forest Meteorol., 279, 107761, https://doi.org/10.1016/j.agrformet.2019.107761, 2019.
Zhao, L., Li, Y. N., Zhao, X. Q., Xu, S. X., Tang, Y. H., Yu, G. R., Gu, S., Du, M. Y., and Wang, Q. X.: Comparative study of the net exchange of CO2 in 3 types of vegetation ecosystems on the Qinghai-Tibetan Plateau, Chinese Sci. Bull., 50, 1767–1774, https://doi.org/10.1360/04wd0316, 2005.
Zhao, L., Li, Y., Xu, S., Zhou, H., Gu, S., Yu, G., and Zhao, X.: Diurnal, seasonal and annual variation in net ecosystem CO2 exchange of an alpine shrubland on Qinghai-Tibetan plateau, Glob. Change Biol., 12, 1940–1953, https://doi.org/10.1111/j.1365-2486.2006.01197.x, 2006.
Zhao, L., Li, J., Xu, S., Zhou, H., Li, Y., Gu, S., and Zhao, X.: Seasonal variations in carbon dioxide exchange in an alpine wetland meadow on the Qinghai-Tibetan Plateau, Biogeosciences, 7, 1207–1221, https://doi.org/10.5194/bg-7-1207-2010, 2010.
Zhao, L., Hu, G., Zou, D., Wu, X., Ma, L., Sun, Z., Yuan, L., Zhou, H., and Liu, S.: Permafrost changes and its effects on hydrological processes on Qinghai-Tibet Plateau, Bull. Chin. Acad. Sci. (Chinese Version), 34, 1233–1246, https://doi.org/10.16418/j.issn.1000-3045.2019.11.006, 2019.
Zhu, J., Zhang, Y., and Jiang, L.: Experimental warming drives a seasonal shift of ecosystem carbon exchange in Tibetan alpine meadow, Agr. Forest Meteorol., 233, 242–249, https://doi.org/10.1016/j.agrformet.2016.12.005, 2017.
Zhu, J., Zhang, F., Li, H., He, H., Li, Y., Yang, Y., Zhang, G., Wang, C., and Luo, F.: Seasonal and Interannual Variations of CO2 Fluxes Over 10 Years in an Alpine Wetland on the Qinghai-Tibetan Plateau, J. Geophys. Res.-Biogeo., 125, e2020JG006011, https://doi.org/10.1029/2020jg006011, 2020.
Zhu, X., Song, C., Swarzenski, C. M., Guo, Y., Zhang, X., and Wang, J.: Ecosystem-atmosphere exchange of CO2 in a temperate herbaceous peatland in the Sanjiang Plain of northeast China, Ecol. Eng., 75, 16–23, https://doi.org/10.1016/j.ecoleng.2014.11.035, 2015.
Zhu, Z., Ma, Y., Li, M., Hu, Z., Xu, C., Zhang, L., Han, C., Wang, Y., and Ichiro, T.: Carbon dioxide exchange between an alpine steppe ecosystem and the atmosphere on the Nam Co area of the Tibetan Plateau, Agr. Forest Meteorol., 203, 169–179, https://doi.org/10.1016/j.agrformet.2014.12.013, 2015.