the Creative Commons Attribution 4.0 License.
the Creative Commons Attribution 4.0 License.
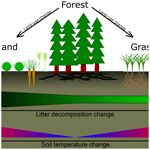
Deforestation for agriculture leads to soil warming and enhanced litter decomposition in subarctic soils
Tino Peplau
Christopher Poeplau
Edward Gregorich
Julia Schroeder
The climate-change-induced poleward shift of agriculture could lead to enforced deforestation of subarctic forest. Deforestation alters the microclimate and, thus, soil temperature, which is an important driver of decomposition. The consequences of land-use change on soil temperature and decomposition in temperature-limited ecosystems are not well understood. In this study, we buried tea bags together with soil temperature loggers at two depths (10 and 50 cm) in native subarctic forest soils and adjacent agricultural land in the Yukon Territory, Canada. A total of 37 plots was established on a wide range of different soils and resampled after 2 years to quantify the land-use effect on soil temperature and decomposition of fresh organic matter. Average soil temperature over the whole soil profile was 2.1 ± 1.0 and 2.0 ± 0.8 ∘C higher in cropland and grassland soils compared to forest soils. Cumulative degree days (the annual sum of daily mean temperatures > 0 ∘C) increased significantly by 773 ± 243 (cropland) and 670 ± 285 (grassland). Litter decomposition was enhanced by 2.0 ± 10.4 % and 7.5 ± 8.6 % in cropland topsoil and subsoil compared to forest soils, but no significant difference in decomposition was found between grassland and forest soils. Increased litter decomposition may be attributed not only to increased temperature but also to management effects, such as irrigation of croplands. The results suggest that deforestation-driven temperature changes exceed the soil temperature increase that has already been observed in Canada due to climate change. Deforestation thus amplifies the climate–carbon feedback by increasing soil warming and organic matter decomposition.
- Article
(2770 KB) -
Supplement
(454 KB) - BibTeX
- EndNote
© His Majesty the King in Right of Canada, as represented by the Minister of Agriculture and Agri-Food Canada, 2022. The co-author Edward Gregorich is an employee of the Canadian Government and therefore claims Crown copyright for the respective contributions.
The poleward shift of agriculture due to climate change (Franke et al., 2022) will alter the land cover of vast areas in subarctic regions. As the global mean temperature rises, permafrost soils of the boreal forest region thaw (Biskaborn et al., 2019) and agriculture in high latitudes expands to regions that had previously been less suitable for agriculture (Tchebakova et al., 2011). Climate change warms the subarctic more strongly than the global average (IPCC, 2013). So, subarctic soils are especially prone to soil organic carbon (SOC) loss. Subarctic soils store large amounts of SOC (Hugelius et al., 2014) that are easily decomposable (Mueller et al., 2015). Moreover, the conversion of pristine subarctic forests into agricultural land has been reported to cause large losses of SOC (Grünzweig et al., 2004; Karhu et al., 2011; Peplau et al., 2022a), which in turn fosters climate change. The mechanisms behind deforestation-induced loss of SOC may be manifold and are not understood in detail. This hampers process-based modelling to extrapolate land-use change effects in space and time.
Besides alterations in species composition and net primary productivity, the replacement of forests by open landscapes has a strong impact on the microclimate, particularly on the temperature regime. Due to missing canopy upon deforestation, the ground is exposed to more direct sunlight and airflow is favoured, leading to more variable near-surface temperatures in open landscapes compared to closed forests (De Frenne et al., 2021). The more rapid intra-day temperature changes of the near-surface air have unclear implications for soil temperature. As Lembrechts et al. (2022) showed, there is an offset between air and soil temperature, which depends on the climatic conditions, and soils are around 3.6 ∘C warmer than the air in boreal forests. Surface air temperature may decrease (Lee et al., 2011), but, regardless of the intensity and direction of air temperature changes, little is known about the effects of land-use change on soil temperature. This applies particularly in the context of subarctic agriculture, since the removal of pristine vegetation and management techniques may have opposing effects on soil temperature. Consequently, potential feedbacks between land-use change, soil temperature and soil organic matter decomposition are also unclear.
Temperature is the most important driver for the decomposition of fresh organic matter (Gregorich et al., 2017), along with moisture (Petraglia et al., 2019) and substrate quality (Fierer et al., 2005). There has been extensive research about the mechanisms behind the effects of soil warming on organic matter decomposition: at first hand, depolymerization of complex organic structures, microbial enzyme production, sorption processes and aggregate turnover are key for temperature-induced changes in soil organic matter decomposition (Conant et al., 2011). The effect of warming on soil organic matter cycling is indirectly influenced by various site properties, such as evapotranspiration, mineralogy or plant litter chemistry (Davidson et al., 2000) and is, therefore, regionally highly variable (Carey et al., 2016). In subarctic forests, losses of SOC due to accelerated decomposition exceed the warming-induced gain in SOC due to enhanced net primary productivity (Karhu et al., 2010), as the large share of labile SOC is quickly decomposed upon warming (Peplau et al., 2021). Despite a different composition of SOC in grasslands than in forests (Grünzweig et al., 2004), it has been shown that subarctic grasslands are also highly prone to SOC loss upon warming (Poeplau et al., 2017).
The objectives of this study were (1) to quantify changes in soil temperature when subarctic forest is converted into agricultural land (i.e. grassland and cropland), (2) to elucidate the influence of various soil properties on such temperature changes, and (3) to compare the decomposition of fresh organic matter in forest and agricultural soils. It was hypothesized that the removal of insulating vegetation by deforestation is shifting the soil temperature regime from relatively moderate temperatures in forest soils to more extreme temperatures in agricultural soils, with warmer summer and colder winter temperatures in agricultural soils than in forest soils. Furthermore, it was hypothesized that warmer summer temperatures encourage the decomposition of soil organic matter in agricultural soils compared to forest soils.
2.1 Research area
A paired-plot litter decomposition experiment was set up in the Yukon Territory in northwestern Canada, at the southern edge of the northern circumpolar permafrost region. The experiment compared litter decomposition and soil temperature in forest and agricultural land (cropland and/or grassland). Since the Klondike gold rush at the end of the 19th century, the Yukon has had an established agricultural sector which allows for studying the effects of land-use change from forest to grassland and forest to cropland in the subarctic. Farms were considered to be suitable for studying the effects of land-use change from forest to grassland or cropland when they (1) originated from forest; (2) were located on mineral soils; and (3) had a remaining native forest adjacent to the agricultural land, i.e. within a distance of approximately 100 m. Furthermore, both forest and agricultural land needed to be located on flat terrain with comparable soil properties. This was checked in an auger-based pre-assessment in consultation with the farmers, focussing on soil texture and the proportion of rock fragments and on bands of organic material as well as on the visibility of hydromorphic properties. A total of 15 farms, covering pairs of forest and cropland and of forest and grassland and triplets with forest, cropland and grassland, were included in this study (for details see Table S1). These farms provided 21 pairs of forest and cropland (n=12) or forest and grassland (n=9) (Fig. 1). As described in detail in Peplau et al. (2022a), forests in the research area were mixed-wood forests of the boreal cordillera ecoregion and croplands were small-scale fields with grains, potatoes or vegetables, and herbs and greens, whereas grasslands were used as pasture for livestock grazing or for hay production.
2.2 Litter decomposition experiment
In order to investigate the effects of land-use change on soil temperature regime and litter decomposition, tea bags and temperature loggers were buried at the chosen farms in summer 2019. Tea bags with green tea (“Bio Grüner Tee”, Paulsen Tee, Fockbek, Germany, charge no. 187896FC) as a standard litter material were weighed, tagged and buried at depths of 10 and 50 cm from the soil surface (n=3 per depth). Before the start of the experiment, 12 tea bags were opened and weighed to determine the weight of the bag material without the tea. This methodology is based on the work of Keuskamp et al. (2013) but is slightly modified in terms of burial depth (10 and 50 cm instead of 5 cm), choice of tea (only green tea instead of green and rooibos tea) and duration of burial (2 years instead of 3 months), as these modifications were necessary to meet the objectives of our study. Temperature loggers (Tinytag Plus 2 TGP-4017, Gemini Data Loggers Ltd) were buried at the same depths (n=1 per depth) and set up to record the soil temperature every 2 h. The tea bags were buried at spots considered representative of the given plots by placing them approximately 30 cm apart from each other around the temperature loggers. After 2 years, the tea bags and temperature loggers were dug out in September 2021. The tea bags were cleaned of roots and soil, dried at 60 ∘C, opened in order to manually pick out fine roots that grew into the tea bag, and weighed again to determine mass loss as a proxy for decomposition. The tea bags from very clayey sites were additionally washed prior to opening to remove clay particles from the tea bag material.
In total, 209 out of 216 tea bags and all 72 temperature loggers were recovered. After downloading the data from the loggers, measurements were checked for plausibility (no abrupt changes that would exceed normal hourly fluctuations) and completeness (no missing data) before further processing.
2.3 Soil parameters
In addition to the burial of tea bags and temperature loggers, soil samples were taken from every plot to characterize the soils of the sites investigated. The sampling was done in summer 2019, at the same time as tea bag burial. Details about the soil sampling and laboratory analyses can be found in Peplau et al. (2022a). Soil was sampled from depth increments of 0–10 and 40–60 cm, matching the depth of the buried sensors and tea bags. Five field replicates of every depth increment were pooled to a mixed sample and analysed for organic and inorganic carbon (C) and total nitrogen (N) content (Byers et al., 1978), pH (ISO 10390), plant-available phosphorus (Olsen et al., 1954), SOC fractions (Zimmermann et al., 2007), and texture (Köhn, 1929). The soils in the research area were Cambisols and Cryosols (Jones et al., 2009) with pH values between 5.5 and 8.9 (mean: 7.4) and clay contents between 49 and 578 g kg−1 (mean: 178 g kg−1). Soil parameters and values of SOC stocks were obtained from an earlier study at the same sites (Peplau et al., 2022a). In this earlier study, soils were sampled from 0–80 cm, with depth increments of 0–10, 10–20, 20–40, 40–60 and 60–80 cm. The organic C was measured with an elemental analyser (LECO TruMac CN). To distinguish between organic C and inorganic C, samples with pH > 6.2 were heated in a muffle furnace at 440 ∘C overnight before the measurement. The heating removes organic C, and the following measurement thus provides an estimate of inorganic C.
2.4 Statistics
The descriptive variables of annual mean temperature, minimum temperature, maximum temperature, temperature amplitude, number of frost days (i.e. days with a mean temperature lower than 0 ∘C) and cumulative degree days (temperature sum of days with a mean temperature above 0 ∘C) were calculated from the original 2-year temperature dataset. The number of frost days and cumulative degree days were divided by 2 to obtain the average of both years.
To test for significant differences in litter decomposition and soil temperature parameters between forest, cropland and grassland, linear mixed-effects models were used with land-use type and depth as fixed effects, allowing for an interaction, and site as a random effect, allowing for a random intercept. Homoscedasticity, normality of the residuals and linearity of the dataset were given, and no transformation of the data was necessary. Since cropland and grassland soils did not have the identical reference forests, separate models were used for cropland–forest and grassland–forest pairs. After performing the linear mixed-effects models, estimated marginal means were used to obtain pairwise comparisons of all groups of the linear mixed-effects model (confidence level = 0.95).
In order to identify variables that are driving the decomposition of the buried tea bags, Pearson's correlation coefficient was calculated separately for the complete dataset and for every land-use type and depth.
All statistical analyses were conducted using R version 4.0.4 (R Core Team, 2021) with the packages readxl (Wickham and Bryan, 2019), tidyverse (Wickham et al., 2019), dplyr (Wickham et al., 2020), purrr (Henry and Wickham, 2020), ggplot2 (Wickham, 2016), ggpubr (Kassambra, 2020), ggthemes (Arnold, 2021), ggpmisc (Aphalo, 2021), corrplot (Wei and Simko, 2021), lme4 (Bates et al., 2015), lmerTest (Kuznetsova et al., 2017), multcomp (Horthon et al., 2008), multcompView (Graves et al., 2019) and emmeans (Lenth, 2021). Values are given as mean ± standard deviation if not indicated otherwise. The level of significance for all statistical analyses was selected as α=0.05. All data used for this study are openly available at https://doi.org/10.5281/zenodo.7219753 (Peplau et al., 2022b).
3.1 Soil temperature as affected by land use
Forest soils were cooler and had smaller intra-day variations in temperature than cropland and grassland soils (Fig. 2). During winter, the soil temperature of all land uses did not exceed 0 ∘C and showed very little short-term variations within a couple of days. With the beginning of spring in April, soil temperature at 10 cm depth increased sharply to above 0 ∘C and short-term variations in temperature became larger. At 50 cm, the spring soil temperature increase was visible but less pronounced than at 10 cm. The sharp increase in soil temperature to above 0 ∘C was visible at all sites at the same time, independently of how low the soil temperature was beforehand. On average, grasslands soils were 2.2 ± 0.9 and 1.8 ± 0.5 ∘C warmer than forest soils at 10 and 50 cm and cropland soils were 2.1 ± 1.1 and 2.0 ± 0.9 ∘C warmer than forest soils at 10 and 50 cm (Fig. 3), which was significant with p<0.001 in all four cases. The linear mixed-effects model showed no interaction between land use and depth (p=0.91 and p=0.26 in cropland and grassland), indicating that soil temperature is affected similarly by land-use change at both depths. Moreover, significantly higher cumulative degree days indicated warmer soils under agricultural use than under forest. Cumulative degree days at 10 and 50 cm were elevated by 606 ± 222 and 733 ± 338 in grassland soils and by 768 ± 262 and 779 ± 237 in cropland soils, with p<0.001 in all four cases. This roughly corresponds to a doubling of cumulative degree days upon land-use change. Detailed information about the soil temperature for every site, land-use type and depth can be found in Table S1.
Significant differences between forest and grassland at both depth increments were found in mean temperature, minimum temperature, maximum temperature, total amplitude and cumulative degree days but not in the number of frost days (Table 1). The comparison between forest and cropland resulted in significant differences at 10 and 50 cm for mean temperature, maximum temperature, total amplitude, cumulative degree days and frost days. In contrast to grasslands, croplands did not have different minimum temperature but had fewer frost days than forests. Temperature differences between forest and grassland were smaller in soils with high clay content, while there was no such correlation observed in cropland soils (Fig. 4).
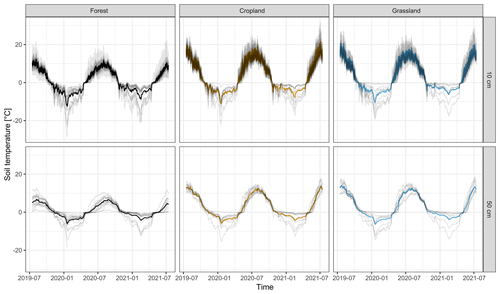
Figure 2Soil temperature profile in forest, cropland and grassland at 10 and 50 cm. Grey lines show the temperature of the individual sites; coloured lines indicate average temperatures. The date format is year-month.
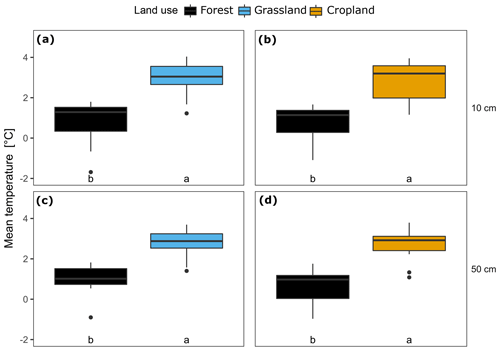
Figure 3Tukey-style boxplot of the mean temperature in grassland–forest pairs (n=9) (a, c) and cropland–forest pairs (n=12) (b, c) at 10 (a, b) and 50 cm (c, d) depth. Different letters at the bottom of each panel indicate statistically significant differences in mean temperature based on estimated marginal means at a level of significance of α=0.05 (p<0.05). Interactive effects between land use and depths were not significant.
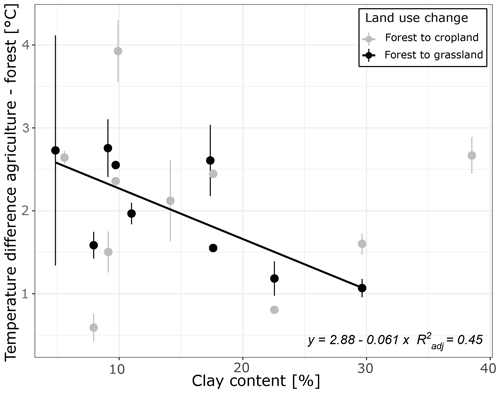
Figure 4Relationship between clay content [%] and soil temperature difference between agricultural land and forest. Points indicate mean values between 10 and 50 cm depth; vertical lines indicate standard deviation of the mean temperature between 10 and 50 cm. The thick line (black) and the formula show the linear regression for the given relationship for the conversion from forest to grassland (p<0.05).
3.2 Litter mass loss
We observed significant differences in litter mass loss between cropland and forest soils but not between grassland and forest soils (Fig. 5). The linear mixed-effects model showed no significant interaction between land use and depth (p=0.11 and p=0.43 for cropland and grassland), indicating that litter mass loss is affected similarly by land-use change at both depths. In forest–cropland pairs, the mean proportional loss of added litter was lower in forest soils (70 ± 7 % and 52 ± 4 % at 10 and 50 cm) than in cropland soils (73 ± 8 % and 61 ± 8 % at 10 and 50 cm), with p=0.321 and p=0.003. In forest–grassland pairs, mean decomposition of added litter in forest soils was 70 ± 4 % and 60 ± 12 % at 10 and 50 cm, while it was 67 ± 7 % and 53 ± 18 % in grassland soils at 10 and 50 cm, with p=0.517 and p=0.085. Detailed information about mass loss for every site, land-use type and depth can be found in Table S1.
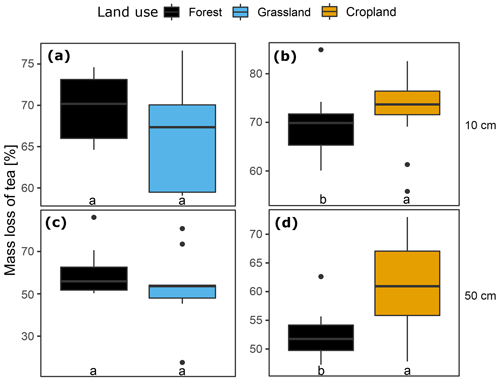
Figure 5Tukey-style boxplot, comparing mean decomposition of the buried tea bags in grassland–forest pairs (n=9) (a, c) and cropland–forest pairs (n=12) (b, d) at 10 (a, b) and 50 cm (c, d) depth. Different letters at the bottom of each panel indicate statistically significant differences in mass loss based on estimated marginal means at a level of significance of α=0.05 (p<0.05). Interactive effects between land use and depths were not significant.
3.3 Soil properties and microclimate explaining tea mass loss
The correlation between litter mass loss and soil temperature, site characteristics and soil properties strongly differed between agricultural land and forest. In forest topsoils, only minimum temperature was significantly negatively correlated with mass loss of tea, while mass loss in subsoils was significantly correlated with minimum temperature, number of frost days and total temperature amplitude (Fig. 6). In cropland soils, significant correlations were only observed in topsoils. In contrast to forest soils, mass loss in cropland soil was positively correlated with minimum temperature. Furthermore, there was a significant negative correlation between mass loss and silt content. In grassland soils, tea mass loss was only correlated with temperature parameters (mean temperature, maximum temperature, amplitude, number of frost days and cumulative degree days). In contrast to croplands, there was no significant correlation between decomposition and SOC fractions in grassland soils. Across all land-use types and depths, mass loss correlated significantly with soil temperature parameters, except for mean temperature and number of frost days. Weaker, yet significant, correlations were observed between mass loss and soil organic matter (C and N content as well as SOC fractions). This was not observed when separating the sample set into the different land-use types and depths, except for forest subsoils (soil organic matter parameters) and grassland subsoils (temperature parameters). Besides elevated mean and maximum temperature, which may be biased by single extreme values, cumulative degree days also increased in cropland and grassland soils compared to forest soils. This increase had a highly significant effect on litter decomposition (Fig. 7) (p<0.001). Furthermore, there was a good correlation between SOC stocks and mean soil temperature in forest soils with higher SOC stocks in colder soils, something that was not observed in agricultural land (Fig. 8).
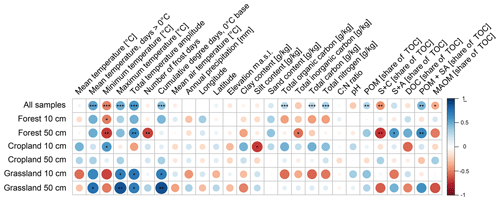
Figure 6Correlogram of Pearson's correlation coefficient, showing the correlation of mean potential litter decomposition and the most important soil temperature parameters, site characteristics, soil properties and fractions of organic soil matter (POM: particulate organic matter; S+C: silt and clay; S+A: sand and stable aggregates; DOC: dissolved organic carbon; MAOM: mineral-associated organic matter). The size and colour of the points represent the direction and the value of the correlation coefficient. Asterisks indicate statistical significance with * p<0.05, p<0.01 and p<0.001.
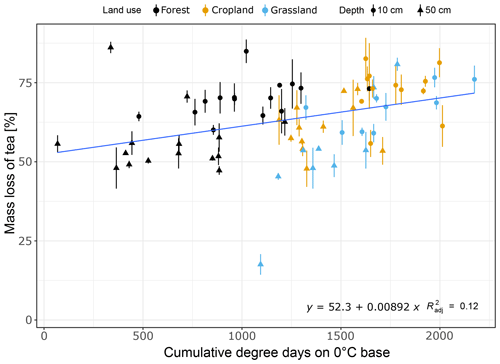
Figure 7Point range and regression line (p<0.05) of tea decomposition over cumulative degree days at 10 cm depth (circles) and 50 cm depth (triangles). Shapes indicate mean values; vertical lines indicate standard deviation of the decomposition. The regression line was fitted using all data points.
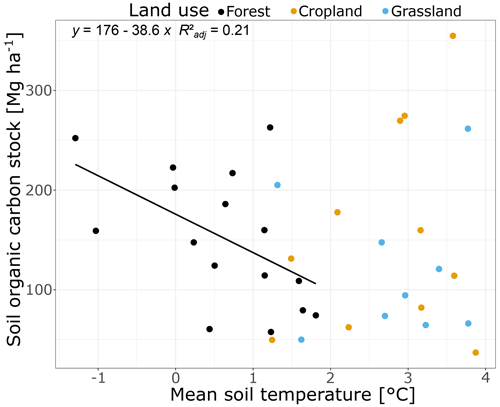
Figure 8Soil organic carbon (SOC) stocks between 0–80 cm depth [Mg ha−1] and mean soil temperature [∘C] of forest, grassland and cropland soils with a significant (p<0.05) correlation (solid line) between SOC stocks and soil temperature in forest soils. Mean temperature was calculated from soil temperatures at 10 and 50 cm.
4.1 Land-use change alters soil temperature in subarctic soils
The higher temperatures, higher cumulative degree days and greater amplitude that we measured in grassland and cropland soils supported our hypothesis that deforestation is shifting the soil temperature regimes from a moderate temperature amplitude, with relatively low summer temperatures in forest soils, to more extreme amplitudes in agricultural soils, with particularly warm summer temperatures. The observed soil warming upon deforestation is in line with results from earlier studies, reporting temperature increases of 2.0 ∘C in the tropics (Jiménez et al., 2007), between 2.5 and 3.3 ∘C in the temperate zone (Morecroft et al., 1998), and up to 5.0 ∘C during summer in boreal Alaska (Grünzweig et al., 2003). Similarly to our results, forest soils in all of the three studies mentioned were on average cooler in summer compared to agricultural land, which is due to shading by the forest canopy. During summer, we observed that forest soils were on average 4.0 ∘C cooler in topsoil and subsoil than croplands and 3.8 and 4.2 ∘C cooler than grasslands, which is slightly less than observed by Grünzweig et al. (2003). Also, cumulative degree days increased between 600 and 800, which is almost a doubling of cumulative degree days upon land-use change. This is a similar increase to that reported by Grünzweig et al. (2003), which was between 500 and 650 annually. As shown in a modelling study for all of Canada, warmer winter soil temperatures can be related to thicker snow cover in deforested land compared to forest (Zhang et al., 2005). Snow cover, along with vegetation, is the most important factor determining soil temperature patterns (Qian et al., 2011; Zhang et al., 2005). Warmer winter soil temperatures in agricultural land compared to forest as a consequence of thicker snow cover on open land than on forests were also reported by Grünzweig et al. (2003). Agricultural soils of the Yukon were as cold as (cropland) and slightly warmer than (grassland) the forest soils during winter. This emphasizes the importance of vegetation for the soil temperature. Snow cover on bare soil might insulate the soil in a similar way to natural forest vegetation, but the combination of dense grasses and overlaying snow cover adds an additional insulation effect.
The temperature difference between forest and agricultural land appeared to be influenced not only by insulation of the soil by vegetation or snow but also by inherent soil thermal properties, which are regulated by soil texture, and therefore indirectly soil moisture. Measurements of soil moisture have not been conducted in this study, but since the study region has only little precipitation and farmers irrigate their croplands regularly, it is likely that the irrigated land is on average wetter than the forest (Peplau et al., 2022a). Clayey grassland sites had smaller temperature differences upon deforestation than sandy sites. This can be related to the differences in the thermal properties of air, water and different minerals, with clayey sites having the lowest and sandy sites having the highest thermal conductivity (Dong et al., 2015). Overall, clayey soils have a larger pore volume and are, therefore, more buffered thermally than sandy soils if the pore space is not water filled but contains a lot of air. Under wet conditions, heat exchange between the soil and the atmosphere is increased and soils cool down more strongly than under dry conditions. In cropland soils, no relationship between soil texture and temperature was supported statistically. Since croplands in the Yukon are irrigated regularly, the thermal insulation of the soils might be reduced at all cropland sites, independently of soil texture, which was not the case in unirrigated grassland sites. Moreover, the effects of soil properties on the soil temperature regime might be masked by differences in vegetation.
Table 1Mean, minimum and maximum temperature; temperature amplitude; number of frost days; and cumulative degree days on 0 ∘C base in forest, grassland and cropland soils. Values are means with standard deviation. Asterisks indicate significant difference (p<0.05).
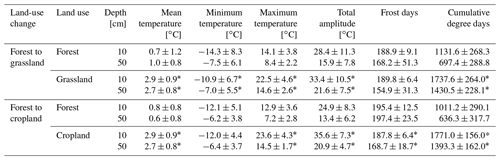
As we have shown, land-use change has a soil warming effect of around 2.1 ∘C. Due to climate change, Canadian soils warmed by 0.6 ∘C during the 20th century, with regional differences of between −2 and +5 ∘C, underlining the great importance of spatially distributed soil temperature measurements (Zhang et al., 2005). Climate-change-related alterations in the temperature of Canadian soils have been observed to be greatest in spring (0.26–0.30 ∘C per decade since the 1950s), while winter soil temperatures have not changed significantly (Qian et al., 2011). In contrast, air temperatures in Canada have increased most strongly in winter (2.3 ∘C between 1950 and 2010) and less so in spring (1.7 ∘C between 1950 and 2010). The annual mean air temperature increased by 1.5 ∘C between 1950 and 2010 or 0.25 ∘C per decade (Vincent et al., 2012), which is slightly less than the increase in soil temperature within the same time. Our results imply that land-use change from pristine forest to agriculture exceeds the effect of climate change on soil temperature and is particularly strong during summer, when biological activity is highest in subarctic ecosystems. Common models of SOC turnover are fed by air temperature instead of soil temperature (Balesdent et al., 2018; Kaczynski et al., 2017; Crowther et al., 2016). Thus, one of the most important drivers of microbial activity and SOC mineralization, that is temperature, is assumed to be independent of the vegetation cover. The present study highlights that this might be a severe shortcoming in such models, which often fail to capture land-use change effects (Boysen et al., 2021; Gottschalk et al., 2010).
4.2 Litter decomposition and its implications for SOC dynamics in subarctic soils
Using tea bags instead of naturally occurring litter had the advantage that the material was standardized, eliminating effects on decomposition caused by differences in litter material between sites or within the soil profile. However, the decomposition values obtained can only be interpreted as potential litter decomposition. It was hypothesized that warmer summer temperatures foster the decomposition of fresh soil organic matter in agricultural soils compared to forest soils. Indeed, there was a greater mass loss of tea in cropland soils than in forest soils. Particularly in subsoils, mass loss was around 8.7 % higher in croplands than in forests, while it was 2.9 % in topsoil, which might be related to the fact that the temperature effect was more masked by agricultural management in the topsoil, where tilling, harvesting and other practices led to regular soil disturbance. However, the hypothesis must be rejected for grassland soils, since there was no significant difference in litter decomposition between forest and grassland soils, despite warmer soil temperatures in grasslands than in forests. This might suggest that not only did the deforestation induce soil warming but also agricultural management controlled litter decomposition. In a global study, Djukic et al. (2018) reported that precipitation is the most important climatic factor for litter decomposition and temperature appeared to be less important. However, their study was conducted only during summer, where water availability, not temperature, was the limiting factor. Our results suggest that, in temperature-limited regions, the temperature may play an important role in litter decomposition, as shown by the relationship between cumulative degree days and litter mass loss. However, missing evidence for this relationship in grassland–forest pairs might underline the importance of water availability as a prerequisite for decomposition. Soil moisture was not quantified in this study, but we can assume that croplands had higher soil moisture than grasslands, as croplands are irrigated regularly due to the dry climate in the research area (on average, 262 mm annual precipitation; Environment Climate Change Canada, 2020) and grasslands remain rainfed, according to a farmers' questionnaire conducted in Peplau et al. (2022a). However, litter decomposition might be underestimated in grassland plots, since there were more fine roots potentially growing into the tea bags, which might not have been removed entirely before weighing. Furthermore, despite the fact that no soil particles were visible within the tea bags after opening the bags after burial, there might be a slight underestimation of the decomposition caused by small clay particles sticking between the remaining tea leaves.
Various studies have reported losses of SOC after land-use change (Grünzweig et al., 2003, 2004; Guo and Gifford 2002; Wei et al., 2014; Poeplau, 2011). A certain proportion of these losses can be assigned to deforestation-induced removal of the uppermost soil layers, including litter and parts of the topsoil (Grünzweig et al., 2003). C input quantity (Luo et al., 2017) and quality (Cotrufo et al., 2019) as well as frequent soil disturbances and changes in aggregate stability (Six et al., 2000) can add to the alterations in SOC stocks driven by land-use change. Here, we were able to show that microclimatic changes and their effect on litter decomposition are another relevant driver of SOC stock change after deforestation. Under natural conditions, as represented by the forest sites, SOC stocks were related to mean soil temperature to some extent. The coldest forest soils stored significantly more SOC than the warmest forest soils, with a linear decrease in SOC of 38.6 ± 17.2 Mg C ha−1 ∘C−1 (p<0.05) warming (Fig. 8). This slope is 1 order of magnitude higher than values observed in warming experiments (Peplau et al., 2021: 1.9 Mg C ha−1 ∘C−1; Verbrigghe et al., 2022: 2.8 Mg C ha−1 ∘C−1). This might indicate that the observed range in forest SOC stocks cannot solely be explained by a direct temperature effect. Instead, it has been observed that the coldest sites, which were also characterized by shallow permafrost (detectable ground ice in summer within the upper 50 cm), were rather wet sites with thick, C-rich A horizons. Farmers reported that the waterlogging ceased after deforestation, i.e. with the deepening of the permafrost layer (Peplau et al., 2022a). Despite a high general variability in SOC stocks across forest sites, our data suggest that, in the presence of permafrost, warming might have a much more severe effect than in non-permafrost soils. This is because water infiltration is hampered by underlying ice layers and soils remain wetter during summer than in sites without permafrost. The linear relationship between soil temperature and SOC stocks was not apparent in agricultural soils, although SOC stocks were reduced significantly in cropland soils (Peplau et al., 2022a). The decoupling of SOC stocks from soil temperature in agricultural soils shows that the natural, climate-driven balance between C input, mineralization and C storage is heavily disturbed by agricultural activity. Depending on agricultural practices (i.e. cultivated crops, soil management, irrigation), the amount and quality of C input vary greatly from natural habitats and also between sites. This makes the quantification of alterations of C mineralization induced by land-use change more complicated. Given that land-use change will increase soil temperatures in subarctic soils, the future spread of agriculture on permafrost soils may therefore additionally accelerate the climate–carbon feedback, causing more SOC loss than already caused by climate change and the warming of pristine forests. The observed different effects of land-use change on soil temperature and decomposition in croplands and grasslands indicate that changes in the soil water regime might be essential for prospective land-use–carbon feedbacks. Since warmed soils have a deeper (or entirely thawed) active layer, soils naturally dry upon warming due to drainage effects (Andresen et al., 2020).
The aim of this study was to quantify the effect of land-use change from subarctic forest to agricultural land on soil temperature and decomposition of fresh soil organic matter. Using tea as a standardized litter material which is easy to compare under varying site properties allowed us to couple soil temperature as influenced by land-use type with litter decomposition in diverse soils under subarctic agriculture. Overall, the effect of land-use change on soil temperature exceeded the effect of past climate change and thus strongly enhanced the climate–carbon feedback. Deforestation resulted in soil warming, but the consequences for litter decomposition depended on the subsequent management. Cropland soils, which are more often disturbed by field operations and irrigation, had a greater decomposition of fresh organic matter than forests. This was not the case in grasslands, even though they exhibited a greater difference in soil temperature. Therefore, future climate mitigation strategies and modelling efforts need to consider the effect of land cover on soil temperature changes, additionally to air temperature changes.
All data used for this study are openly available at https://doi.org/10.5281/zenodo.7219753 (Peplau et al., 2022b).
The supplement related to this article is available online at: https://doi.org/10.5194/bg-20-1063-2023-supplement.
CP and JS designed the tea bag and temperature experiments and were responsible for the field setup. JS and TP finalized the experiments. TP was responsible for writing the original draft, including figures and statistical analysis, and all co-authors contributed by reviewing and editing. EG provided additional language editing.
The contact author has declared that none of the authors has any competing interests.
Publisher’s note: Copernicus Publications remains neutral with regard to jurisdictional claims in published maps and institutional affiliations.
We would like to thank all the farmers who participated in this study and allowed us to use their land for our experiments. We are also grateful to Yukon's First Nations, who granted us permission to study their traditional land, without which this study would not have been possible. This study was part of the project “Breaking the Ice”, funded by the German Research Foundation. Funding for Edward Gregorich was provided by the Science and Technology Branch of Agriculture and Agri-Food Canada (Project “Biological Soil Carbon Stabilization”).
This research has been supported by the Deutsche Forschungsgemeinschaft (grant no. 401106790) and Agriculture and Agri-Food Canada (Project J-001756).
This paper was edited by Sara Vicca and reviewed by two anonymous referees.
Andresen, C. G., Lawrence, D. M., Wilson, C. J., McGuire, A. D., Koven, C., Schaefer, K., Jafarov, E., Peng, S., Chen, X., Gouttevin, I., Burke, E., Chadburn, S., Ji, D., Chen, G., Hayes, D., and Zhang, W.: Soil moisture and hydrology projections of the permafrost region – a model intercomparison, The Cryosphere, 14, 445–459, https://doi.org/10.5194/tc-14-445-2020, 2020.
Aphalo, P. J.: ggpmisc: Miscellaneous Extensions to “ggplot2”, Version 0.4.0, https://CRAN.R-project.org/package=ggpmisc (last access: 24 February 2023), 2021.
Arnold, J. B.: ggthemes: Extra Themes, Scales and Geoms for “ggplot2”, Version 4.2.4, https://CRAN.R-project.org/package=ggthemes (last access: 24 February 2023), 2021.
Bates, D., Mächler, M., Bolker, B., and Walker, S.: Fitting Linear Mixed-Effects Models Using lme4, J. Stat. Softw., 67, 1–48, https://doi.org/10.18637/jss.v067.i01, 2015.
Balesdent, J., Basile-Doelsch, I., Chadoeuf, J., Cornu, S., Derrien, D., Fekiacova, Z., and Hatté, C.: Atmosphere-soil carbon transfer as a function of soil depth, Nature, 559, 599–602, https://doi.org/10.1038/s41586-018-0328-3, 2018.
Biskaborn, B. K., Smith, S. L., Noetzli, J., Matthes, H., Vieira, G., Streletskiy, D. A., Schoeneich, P., Romanovsky, V. E., Lewkowicz, A.G., Abramov, A., Allard, M., Boike, J., Cable, W. L., Christiansen, H. H., Delaloye, R., Diekmann, B., Drozdov, D., Etzelmüller, B., Grosse, G., Guglielmin, M., Ingeman-Nielsen, T., Isaksen, K., Ishikawa, M., Johansson, M., Johannsson H., Joo, A., Kaverin, D., Kholodov, A., Konstantinov, P., Kröger, T., Lambiel, C., Lanckman, J.-P., Luo, D., Malkova, G., Meiklejohn, I., Moskalenko, N., Oliva, M., Phillips, M., Ramos, M., Sannel, A. B. K., Sergeev, D., Seybold, C., Skryabin, P., Vasiliev, A., Wu, Q., Yoshikawa, K., Zheleznyak, M., and Lantuit, H.: Permafrost is warming at a global scale, Nat. Commun., 10, 264–275, https://doi.org/10.1038/s41467-018-08240-4, 2019.
Boysen, L. R., Brovkin, V., Warlind, D., Peano, D., Lanso, A. S., Delire, C., Burke, E., Poeplau, C., and Don, A.: Evaluation of soil carbon dynamics after forest cover change in CMIP6 land models using chronosequences, Environ. Res. Lett., 16, 1–12, https://doi.org/10.1088/1748-9326/ac0be1, 2021.
Byers, S. C., Mills, E. L., and Steward, P. L.: A comparison of methods of determining organic carbon in marine sediments, with suggestions for a standard method, Hydrobiologia, 58, 43–47, 1978.
Carey, J. C., Tang, J., Templer, P. H., Kroeger, K. D., Crowther, T. W., Burton, A. J., Dukes, J., Emmet, B., Frey, S. D., Heskel, M. A., Jiang, L., Machmuller, M. B., Mohan, J., Panetta, A. M., Reich, P. B., Reinsch, S., Wang, X., Allison, S. D., Bamminger, C., Bridgham, S., Collins, S. L., Dato, G. d., Eddy, W. C., Enquist, B. J., Estiarte, M., Harte, J., Henderson, A., Johnson, B. R., Larsen, K., S., Yiqi, L., Marhan, S., Melillo, J. M., Penuelas, J., Pfeifer-Meister, L., Poll, C., Rastetter, E., Reinmann, A. B., Reynolds, L. L., Schmidt, I. K., Shaver, G. R., Strong, A. L., Suseela, V., and Tietema, A.: Temperature response of soil respiration largely unaltered with experimental warming, P. Natl. Acad. Sci. USA, 113, 13797–13802, https://doi.org/10.1073/pnas.1605365113, 2016.
Conant, R. T., Ryan, M. G., Ågren, G. I., Birge, H. E., Davidson, E. A., Eliasson, P. E., Evans, S. E., Frey, S. D., Giardina, C. P., Hopkins, F. M., Hyvönen, R., Kirschbaum, M. U. F., Lavallee, J. M., Leifeld, J., Parton, W. J., Steinweg, J. M., Wallenstein, M. D., Wetterstedt, J. A. M., and Bradford, M. A.: Temperature and soil organic matter decomposition rates – synthesis of current knowledge and a way forward, Glob. Change Biol., 17, 3392–3404, https://doi.org/10.1111/j.1365-2486.2011.02496.x, 2011.
Cotrufo, M. F., Ranalli, M. G., Haddix, M. L., Six, J., and Lugato, E.: Soil carbon storage informed by particulate and mineral-associated organic matter, Nat. Geosci., 12, 989–994, https://doi.org/10.1038/s41561-019-0484-6, 2019.
Crowther, T. W., Todd-Brown, K. E. O., Rowe, C. W., Wieder, W. R., Carey, J. C., Machmuller, M. B., Snoek, B. L., Fang, S., Zhou, G., Allison, S. D., Blair, J. M., Bridgham, S. D., Burton, A. J., Carrilla, Y., Reich, P. B., Clark, J. S., Classen, A. T., Dijkstra, F. A., Elberling, B., Emmet, B. A., Estiarte, M., Frey, S. D., Guo, J., Harte, J., Jiang, L., Johnson, B. R., Kröel-Dulay, G., Larsen, K. S., Laudon, H., Lavallee, J. M., Luo, Y., Lupascu, M., Ma, L. N., Marhan, S., Michelson, A., Mohan, J., Niu, S., Pendall, E., Penuelas, J., Pfeifer-Meister, L., Poll, C., Reinsch, S., Reynolds, L. L., Schmidt, I. K., Sistla, S., Sokol, N. W., Templer, P. H., Treseder, K. K., Welker, J. M., and Bradford, M. A.: Quantifying global soil carbon losses in response to warming, Nature, 540, 104–108, https://doi.org/10.1038/nature20150, 2016.
Davidson, E. A., Trumbore, S. E., and Amundson, R.: Soil warming and organic carbon content, Nature, 408, 789–790, 2000.
De Frenne, P., Lenoir, J., Luoto, M., Scheffers, B. R., Zellweger, F., Aalto, J., Ashcroft, M., B., Christianse, D. M., Decocq, G., Pauw, K. d., Govaert, S., Greiser, C., Gril, E., Hampe, A., Jucker, T., Klinges, D. H., Koelemeijer, I. A., Lembrechts, J. J., Marrec, R., Meeussen, C., Ogée, J., Tyystjärvi, V., Vangansbeke, P., and Hylander, K.: Forest microclimates and climate change: Importance, drivers and future research agenda, Glob. Change Biol., 27, 2279–2297. https://doi.org/10.1111/gcb.15569, 2021.
Djukic, I., Kepfer-Rojas, S., Schmidt, I. K., Larsen, K. S., Beier, C., Berg, B., and Verheyen, K.: Early stage litter decomposition across biomes, Sci. Total Environ., 628/629, 1369–1394, https://doi.org/10.1016/j.scitotenv.2018.01.012, 2018.
Environment Climate Change Canada: Canadian climate normal 1982–2010 station data, Temperature and Precipitation Graph for 1981 to 2010 Canadian Climate Normals WHITEHORSE A, Government of Canada, https://climate.weather.gc.ca/climate_normals/results_1981_2010_e.html?stnID=1618&autofwd=1 (last access: 24 February 2023), 2020.
Fierer, N., Craine, J. M., McLauchlan, K., and Schimel, J. P.: Litter quality and the temperature sensitivity of decomposition, Ecology, 86, 320–326, 2005.
Franke, J. A., Müller, C., Minoli, S., Elliott, J., Folberth, C., Gardner, C., Hank, T., Izaurralde, R., C., Jägermeyr, J., Jones, C. D., Liu, W., Olin, S., Pugh, T. A. M., Ruane, A. C., Stephens, H., Zabel, F., and Moyer, E. J.: Agricultural breadbaskets shift poleward given adaptive farmer behavior under climate change, Glob. Change Biol., 28, 167–181, https://doi.org/10.1111/gcb.15868, 2022.
Gottschalk, P., Bellarby, J., Chenu, C., Foereid, B., Smith, P., Wattenbach, M., Zingore, S., and Smith, J.: Simulation of soil organic carbon response at forest cultivation sequences using 13C measurements, Org. Geochem., 41, 41–54, https://doi.org/10.1016/j.orggeochem.2009.04.017, 2010.
Graves, S., Piepho, H.-P., Selzer, L, and Dorai-Raj, S.: multcompView: Visualizations of Paired Comparisons, https://CRAN.R-project.org/package=multcompview (last access: 24 February 2023), 2019.
Gregorich, E. G., Janzen, H., Ellert, B. H., Helgason, B. L., Qian, B., Zebarth, B. J., Angers, D. A., Beyaert, R. P., Drury, C. F., Duguid, S., May, W. E., McConkey, B. G., and Dyck, M. F.: Litter decay controlled by temperature, not soil properties, affecting future soil carbon, Glob. Change Biol., 23, 1725–1734, https://doi.org/10.1111/gcb.13502, 2017.
Grünzweig, J. M., Sparrow, S. D., and Chapin, F. S.: Impact of forest conversion to agriculture on carbon and nitrogen mineralization in subarctic Alaska, Biogeochemistry, 64, 271–296, https://doi.org/10.1023/A:1024976713243, 2003.
Grünzweig, J. M., Sparrow, S. D., Yakir, D., and Chapin, F. S.: Impact of Agricultural Land-use Change on Carbon Storage in Boreal Alaska, Glob. Change Biol., 10, 452–472, 2004.
Guo, L. B. and Gifford, R. M.: Soil carbon stocks and land use change: a meta analysis, Glob. Change Biol., 8, 345–360, https://doi.org/10.1046/j.1354-1013.2002.00486.x, 2002.
Henry, L. and Wickham, H.: purrr: Functional Programming Tools, Version 0.3.4, https://CRAN.R-project.org/package=purrr (last access: 24 February 2023), 2020.
Horthon, T., Bretz, F., and Westfall, P.: Simultaneous Inference in General Parametric Models, Biometrical J., 50, 246–363, https://doi.org/10.1002/bimj.200810425, 2008.
Hugelius, G., Strauss, J., Zubrzycki, S., Harden, J. W., Schuur, E. A. G., Ping, C.-L., Schirrmeister, L., Grosse, G., Michaelson, G. J., Koven, C. D., O'Donnell, J. A., Elberling, B., Mishra, U., Camill, P., Yu, Z., Palmtag, J., and Kuhry, P.: Estimated stocks of circumpolar permafrost carbon with quantified uncertainty ranges and identified data gaps, Biogeosciences, 11, 6573–6593, https://doi.org/10.5194/bg-11-6573-2014, 2014.
IPCC: Climate Change 2013: The Physical Science Basis, Contribution of Working Group I to the Fifth Assessment Report of the Intergovernmental Panel on Climate Change, edited by: Stocker, T. F., Qin, D., Plattner, G.-K., Tignor, M., Allen, S. K., Boschung, J., Nauels, A., Xia, Y., Bex, V., and Midgley, P. M., Intergovernmental Panel on Climate Change, Cambridge, United Kingdom and New York, NY, USA, Cambridge University Press, ISBN 978-1-107-66182-0, 2013.
Jiménez, C., Tejedor, M., and Rodríguez, M.: Influence of land use changes on the soil temperature regime of Andosols on Tenerife, Canary Islands, Spain, Eur. J. Soil Sci., 58, 445–449, https://doi.org/10.1111/j.1365-2389.2007.00897.x, 2007.
Jones, A., Stolbovoy, V., Tarnocai, C., Broll, G., Spaargaren, O., and Montanarella, L. (Eds.): Soil Atlas of the Northern Circumpolar Region, European Commission, Office for Official Publications of the European Communities, Luxembourg, 142 pp., ISBN 978-92-79-09770-6, 2009.
Kaczynski, R., Siebielec, G., Hanegraaf, M. C., and Hein, K.: Modelling soil carbon trends for agriculture development scenarios at regional level, Geoderma, 286, 104–115, https://doi.org/10.1016/j.geoderma.2016.10.026, 2017.
Karhu, K., Fritze, H., Hämäläinen, K., Vanhala, P., Jungner, H., Oinonen, M., Sonninen, E., Tuomi, M., Spetz, P., Kizunen, V., and Liski, J.: Temperature sensitivity of soil carbon fractions in boreal forest soil, Ecology, 91, 370–376, 2010.
Karhu, K., Wall, A., Vanhala, P., Liski, J., Esala, M., and Regina, K.: Effects of afforestation and deforestation on boreal soil carbon stocks – Comparison of measured C stocks with Yasso07 model results, Geoderma, 164, 33–45, https://doi.org/10.1016/j.geoderma.2011.05.008, 2011.
Kassambra, A.: ggpubr: “ggplot2” Based Publication Ready Plots, https://CRAN.R-project.org/package=ggpubr (last access: 24 February 2023), 2020.
Keuskamp, J. A., Dingemans, B. J. J., Lehtinen, T., Sarneel, J. M., and Hefting, M. M.: Tea Bag Index: a novel approach to collect uniform decomposition data across ecosystems, Methods Ecol. Evol., 4, 1070–1075, https://doi.org/10.1111/2041-210X.12097, 2013.
Köhn, M.: Korngrößenanalyse vermittels Pipettanalyse, Tonindustrie-Zeitung, 53, 729–731, 1929.
Kuznetsova, A., Brockhoff, P. B. M., and Christensen, R. H. B.: lmerTest Package: Tests in Linear Mixed Effects Models, J. Stat. Softw., 82, 1–26, https://doi.org/10.18637/jss.v082.i13, 2017.
Lee, X., Goulden, M. L., Hollinger, D. Y., Barr, A., Black, T. A., B., Bohrer, G., Bracho, R., Drake, B., Goldstein, A., Gu, L., Katul, G., Kolb, T., Law, B. E., Margolis, H., Meyers, T., Monson, R., Munger, W., Oren, R., Paw, K. T., Richardson, A. D., Schmidt, H. P., Staebler, R., Wofsy, S., and Zhao, L.: Observed increase in local cooling effect of deforestation at higher latitudes, Nature, 479, 384–387, https://doi.org/10.1038/nature10588, 2011.
Lembrechts, J. J., van den Hoogen, J., Aalto, J., Ashcroft, M. B., De Frenne, P., Kemppinen, J., Kopecký, M., Luoto, M., Maclean, I. M. D., Crowther, T. W., Bailey, J. J., Haesen, S., Klinges, D. H., Niittynen, P., Scheffers, B. R., Van Meerbeek, K., Aartsma, P., Abdalaze, O., Abedi, M., and Lenoir, J.: Global maps of soil temperature, Glob. Change Biol., 28, 3110–3144, https://doi.org/10.1111/gcb.16060, 2022.
Lenth, R. V.: emmeans: Estimated Marginal Means, aka Least-Square Means, https://CRAN.R-project.org/package=emmeans (last access: 24 February 2023), 2021.
Luo, Z., Feng, W., Luo, Y., Baldock, J., and Wang, E.: Soil organic carbon dynamics jointly controlled by climate, carbon inputs, soil properties and soil carbon fractions, Glob. Change Biol., 23, 4430–4439, https://doi.org/10.1111/gcb.13767, 2017.
Morecroft, M. D., Taylor, M. E., and Oliver, H. R.: Air and soil microclimates of deciduous woodland compared to an open site, Agr. Forest Meteorol., 90, 141–156, 1998.
Mueller, C. W., Rethemeyer, J., Kao-Kniffin, J., Löppmann, S., Hinkel, K. M., and Bockheim, J. G.: Large amounts of labile organic carbon in permafrost soils of northern Alaska, Glob. Change Biol., 21, 2804–2817, https://doi.org/10.1111/gcb.12876, 2015.
Olsen, S. R., Cole, C. V., Watanabe, F. S., and Dean, L. A.: Department of Agriculture, United States: Estimation of available phosphorus in soils by extraction with sodium bicarbonate, Colorado Agricultural Experiment Station Scientific journal series no. 418., https://openlibrary.org/books/OL25604885M/Estimation_of_available_phosphorus_in_soils_by_extraction_with_sodium_bicarbonate (last access: 24 February 2023), 1954.
Peplau, T., Schroeder, J., Gregorich, E., and Poeplau, C.: Long-term geothermal warming reduced stocks of carbon but not nitrogen in a subarctic forest soil, Glob. Change Biol., 27, 5341–5355, https://doi.org/10.1111/gcb.15754, 2021.
Peplau, T., Schroeder, J., Gregorich, E., and Poeplau, C.: Subarctic soil carbon losses after deforestation for agriculture depend on permafrost abundance, Glob. Change Biol., 28, 5227–5242, https://doi.org/10.1111/gcb.16307, 2022a.
Peplau, T., Poeplau, C., Gregorich, E., and Schroeder, J.: Dataset to: Deforestation for agriculture leads to soil warming and enhanced litter decomposition in subarctic soils, Zenodo [data set], https://doi.org/10.5281/zenodo.7219753, 2022b.
Petraglia, A., Cacciatori, C., Chelli, S., Fenu, G., Calderisi, G., Gargano, D., Abeli, T., Orsenigo, S., and Carbognani, M.: Litter decomposition: effects of temperature driven by soil moisture and vegetation type, Plant Soil, 435, 187–200, https://doi.org/10.1007/s11104-018-3889-x, 2019.
Poeplau, C., Don, A., Vesterdal, L., Leifeld, J., van Wesemael, B. A., Schumacher, J., and Gensior, A.: Temporal dynamics of soil organic carbon after land-use change in the temperate zone – carbon response functions as a model approach, Glob. Change Biol., 17, 2415–2427, https://doi.org/10.1111/j.1365-2486.2011.02408.x, 2011.
Poeplau, C., Kätterer, T., Leblans, N. I. W., and Sigurdsson, B. D.: Sensitivity of soil carbon fractions and their specific stabilization mechanisms to extreme soil warming in a subarctic grassland, Glob. Change Biol., 23, 1316–1327, https://doi.org/10.1111/gcb.13491, 2017.
Qian, B., Gregorich, E. G., Gameda, S., Hopkins, D. W., and Wang, X. L.: Observed soil temperature trends associated with climate change in Canada, J. Geophys. Res., 116, D02106, https://doi.org/10.1029/2010JD015012, 2011.
R Core Team: R: A language and environment for statistical computing, Version 4.0.4, R Foundation for Statistical computing, https://www.r-project.org/ (last access: 24 February 2023), 2021.
Six, J., Elliott, E. T., and Paustian, K.: Soil macroaggregate turnover and microaggregate formation: a mechanism for C sequestration under no-tillage agriculture, Soil Biol. Biochem., 32, 2099–2103, 2000.
Tchebakova, N. M., Parfenova, E. I., Lysanova, G. I., and Soja, A. J.: Agroclimatic potential across central Siberia in an altered twenty-first century, Environ. Res. Lett., 6, 45207, https://doi.org/10.1088/1748-9326/6/4/045207, 2011.
Verbrigghe, N., Leblans, N. I. W., Sigurdsson, B. D., Vicca, S., Fang, C., Fuchslueger, L., Soong, J. L., Weedon, J. T., Poeplau, C., Ariza-Carricondo, C., Bahn, M., Guenet, B., Gundersen, P., Gunnarsdóttir, G. E., Kätterer, T., Liu, Z., Maljanen, M., Marañón-Jiménez, S., Meeran, K., Oddsdóttir, E. S., Ostonen, I., Peñuelas, J., Richter, A., Sardans, J., Sigurðsson, P., Torn, M. S., Van Bodegom, P. M., Verbruggen, E., Walker, T. W. N., Wallander, H., and Janssens, I. A.: Soil carbon loss in warmed subarctic grasslands is rapid and restricted to topsoil, Biogeosciences, 19, 3381–3393, https://doi.org/10.5194/bg-19-3381-2022, 2022.
Vincent, L. A., Wang, X. L., Milewska, E. J., Wan, H., Yang, F., and Swail, V.: A second generation of homogenized Canadian monthly surface air temperature for climate trend analysis, J. Geophys. Res., 117, D18110, https://doi.org/10.1029/2012JD017859, 2012.
Wei, T. and Simko, V.: R package “corrplot”: Visualization of a Correlation Matrix, Version 0.90, Github, https://github.com/taiyun/corrplot (last access: 24 February 2023), 2021.
Wei, X., Shao, M., Gale, W., and Li, L.: Global pattern of soil carbon losses due to the conversion of forests to agricultural land, Sci. Rep., 4, 4062, https://doi.org/10.1038/srep04062, 2014.
Wickham, H.: ggplot2: Elegant Graphics for Data Analysis: Springer-Verlag New York, https://ggplot2.tidyverse.org (last access: 24 February 2023), 2016.
Wickham, H. and Bryan, J.: readxl: Read Excel Files, Version 1.3.1, https://CRAN.R-project.org/package=readxl (last access: 24 February 2023), 2019.
Wickham, H., Averick, M., Bryan, J., Chang, W., D'Agostino McGowan, L., Francois, R., Grolemund, G., Hayes, A., Henry, L., Hester, J., Kuhn, M., Pedersen, T. L., Miller, E., Bache, S. M., Müller, K., Ooms, J., Robinsons, D., Seidel, D. P., Spine, V., Takahashi, K., Vaughan, D., Wilke, C., Woo, K., and Yutani, H.: Welcome to the tidyverse, J. Open Sour. Softw., 4, 1686, https://doi.org/10.21105/joss.01686, 2019.
Wickham, H., François, R., Henry, L., and Müller, K.: dplyr: A Grammar of Data Manipulation, Version R package version 1.0.0, https://CRAN.R-project.org/package=dplyr (last access: 24 February 2023), 2020.
Zhang, Y., Chen, W., Smith, S. L., Riseborough, D. W., and Cihlar, J.: Soil temperature in Canada during the twentieth century: Complex responses to atmospheric climate change, J. Geophys. Res., 110, D03112, https://doi.org/10.1029/2004JD004910, 2005.
Zimmermann, M., Leifeld, J., Schmidt, M. W. I., Smith, P., and Fuhrer, J.: Measured soil organic matter fractions can be related to pools in the RothC model, Eur. J. Soil Sci., 58, 658–667, https://doi.org/10.1111/j.1365-2389.2006.00855.x, 2007.