the Creative Commons Attribution 4.0 License.
the Creative Commons Attribution 4.0 License.
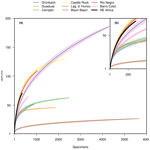
The emergence of the tropical rainforest biome in the Cretaceous
Clément Coiffard
Johan Renaudie
Robert Bussert
Dieter Uhl
Modern tropical rainforests (TRFs) are one of the most ecologically important and species-rich biomes on the planet. However, the origin of modern TRFs is still debated, especially due to the incongruence between the fossil record and molecular data. Here, we test whether Campanian assemblages from northeastern Africa could represent fossil TRF vegetation. In so doing, we compare the investigated assemblages with other assemblages known to represent temperate forests, subtropical forests, and TRF in terms of leaf physiognomy, species richness, and taxonomic composition. We conclude based on fossil leaves that modern-looking TRFs already existed since 80 Ma during the Campanian in northeastern Africa in an area corresponding to 10 % of the modern Amazonian or 25 % of the Congolese TRF. The apparent conflict between the fossil record and phylogenetic evidence is due to the nearly complete absence of published tropical floras for the Cretaceous.
- Article
(6267 KB) - Full-text XML
-
Supplement
(553 KB) - BibTeX
- EndNote
Modern tropical rainforests (TRFs) are one of the most ecologically important and species-rich biomes on the planet. Rainforest environments have an enormous impact on global water and heat circulation and therefore play a major role in water distribution and rainfall patterns. This implies that the closed canopy is stratified, when the closed canopy is one of several layers, including the emergent and lower strata. The presence of woody angiosperms, abundant rainfall, and equable temperatures are also characteristic. The origin of modern TRFs is still debated (Eiserhardt et al., 2017). Molecular phylogenetic studies of modern plants suggest that the earliest appearance of the TRF dated back to the Cenomanian (93.9–100.5 Ma). In contrast, fossil TRFs, characterized by its (leaf) physiognomy, climate proxies, and high diversity, have not been identified so far before the Maastrichtian (66.0–72.1 Ma) (Carvalho et al., 2021). An alternative scenario implies an earlier origin of TRFs at mid-latitudes (Morley, 2000; Davis et al., 2005). However, mid-latitude floras from the Dakota Formation (ca. 100 Ma) (Upchurch and Wolfe, 1987) and from the Olmos Formation (ca. 72 Ma) (Estrada-Ruiz et al., 2008) correspond to a paratropical/subtropical climate, the paratropical (20–25 ∘C) being slightly cooler than truly tropical climate (>25 ∘C) and exhibiting higher seasonal temperature amplitude.
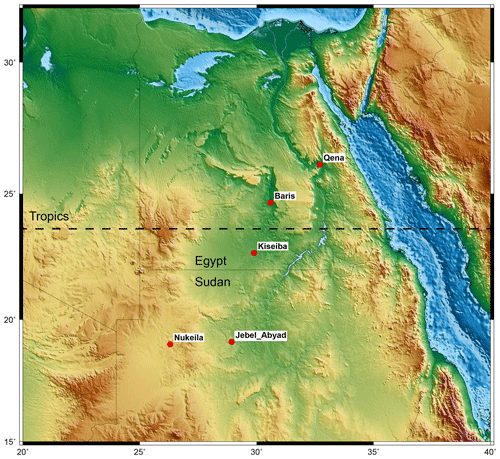
Figure 1Locations of the fossil assemblages studied. The location map shows the studied areas. The topographic map was created using the generic mapping tools of Wessel and Luis (2017) and the topographic data set ETOPO1 of Amante and Eakins (2009).
Understanding the early development of TRFs has been hampered by the fact that most previous information originated from mid- to high-latitude fossil sites reflecting more temperate climates, while low-latitude sites have hardly been studied. However, relevant material including Campanian fossil plants from Egypt and Sudan, which were located near or on the Equator in palaeogeographic reconstructions for the Campanian, was collected by the Sonderforschungsbereich 69 (a collaborative research centre located in Berlin funded by the DFG) in the 1970s to 1990s (Fig. 1). The studied assemblages were preliminarily studied by Klitzsch and Lejal-Nicol (1984), Lejal-Nicol (1987), and Kahlert et al. (2009). However, these contributions focused only on a few selected taxa and did not attempt to estimate the palaeoclimate or the palaeodiversity. The studied collection, from Baris Oasis in Egypt, comes from the Quseir Formation (Fm.) of early to middle Campanian age (ca. 84–76 Ma) (Mahmoud, 2003). In addition, seven smaller assemblages from lateral and temporal equivalents of the Quseir Fm. were also studied. To test whether these assemblages correspond to fossil TRF vegetation, or at least a habitat suitable for stem lineages of modern TRF taxa, we compared them with contemporary and younger fossil assemblages known to represent temperate forests, subtropical forests, and TRFs considering three aspects:
-
leaf physiognomy, dominated by entire margined taxa, reflecting mean annual temperature >24 ∘C, and mesophyllous or larger leaves, implying rainfall >1500 mm, corresponding to the limit of TRFs in Africa (White, 1983);
-
a high species richness, comparable to modern assemblages (Wing et al., 2009); and
-
a taxonomic composition similar to modern TRFs (Wing et al., 2009).
1.1 Palaeoclimate background during the Campanian of NE Africa
1.1.1 Sedimentological evidence
In southern Egypt and northern Sudan, the shallow marine Campanian sediments are rich in kaolinite and sometimes contain phosphorites (Schwarz and Germann, 1995). However, equivalent continental facies comprise stacked palaeosols consisting of quartz, kaolinite, iron hydroxides, and oxides. These palaeosols are interpreted as laterites formed in situ or eroded and redeposited (Schwarz and Germann, 1995). The dominance of kaolinite in the clay mineral fraction in the sediments indicates a perennial warm and humid tropical palaeoclimate that promoted the hydrolysis of aluminosilicate minerals (Chamley, 1989; Thiry, 2000). Phosphorite formation has also been ascribed to intense chemical weathering on the African continent due to a humid tropical palaeoclimate and fluvial transport of the weathering59). Laterites are typically formed by intense tropical or subtropical weathering, although preferably in a warm climate with pronounced dry seasons (Widdowson, 2007).
1.1.2 Palaeobotanical evidence
Records of gymnospermous charcoal, in sediments of the Quseir Fm., although rare, indicate the presence of wildfires during the Campanian in the surroundings of Baris (El Atfy et al., 2016). Although the occurrence of wildfires is often used as an indication of somewhat drier conditions, it is not clear whether such conditions occurred seasonally or just during an occasional dry spell (El Atfy et al., 2016). Even in modern rainforests such occasional dry spells can promote the ignition and spread of wildfires (Scott et al., 2013). Under conditions of higher than present oxygen concentrations in the atmosphere, which have been reconstructed by a number of models for the Cretaceous (Brown et al., 2012), it is even more likely that wildfires could also be ignited within ever-wet TRFs and spread easily (Belcher et al., 2010).
Palynological data from the Quseir Fm. also point to a flora dominated by angiosperms, although pteridophytes and gymnosperms are present, but not diverse (Mahmoud, 2003). Based on the composition and diversity of the palynoflora, as well as data from palynofacies analysis, Mahmoud (2003) interpreted the source habitats of the recovered palynomorphs as a fluviolacustrine landscape with abundant moist and aquatic habitats, as well as subordinate drier habitats (i.e. with Araucaria, ephedroids, and other gymnosperms) in the hinterland, in which the plants grew under a warm and humid, tropical to subtropical palaeoclimate.
The studied materials comprise fossil leaves collected by members of the Sonderforschungsbereich 69 in 1984 and 1987 that are housed now at the Natural History Museum of Berlin. They comprise collection B (Baris), which encompasses the material described here that was collected east of Baris (24∘33′N, 30∘43′E) in southern Egypt from the Quseir Fm., which has been dated as early to middle Campanian (Mahmoud, 2003). In addition, further eight assemblages were also studied; among them, four assemblages come from Egypt and three from the Bir Kiseiba area (pf12–81, pf3/82 and pf13/82) (Klitzsch and Lejal-Nicol, 1984; Lejal-Nicol, 1987) that were collected from the Kiseiba Fm., an equivalent to the Quseir Fm. (Klitzsch and Hermina, 1989) and only one from the Qena area (Schrank, 1992), also from the Quseir Fm. Moreover, four assemblages come from Sudan, one from the Jebel Abyad Plateau (pf82/23S) (Lejal-Nicol, 1987; Barazi, 1985) and three from the Bir Atrun-Nukheila area (pf8–84, pf9/84, pf10/84) (Lejal-Nicol, 1987; Barazi, 1985; Klitzsch and Wycisk, 1987), all of which are from the Kababish Fm. and its equivalents (Barazi, 1985).
Concerning the dating of the studied assemblage, a lower age boundary is defined by a regional hiatus associated with a major unconformity between the Santonian and the Campanian, which has been dated palynologically (Wycisk, 1994, and references therein).
An upper age boundary is defined by a transgression that covered the whole area. This transgression has been dated in Egypt as late Campanian based on palynology (El Beialy, 1995) and ammonites (Dominik and Schaal, 1984).
In addition to plant megafossils, the ecosystem in the Quseir Fm. is characterized by the presence of fragmented bones and other vertebrate remains of lungfishes, sawfishes, turtles, and crocodiles, as well as dinosaurs (Abu El-Kheir, 2020).
3.1 Climate proxies and diversity analyses
For the diversity analyses and climate proxies, the leaves were grouped into morphotypes without considering formerly proposed taxa (a literature survey was done afterwards). Each morphotype differs from all others by at least one of the criteria described in the manual of leaf architecture described by Ellis et al. (2009). Although the biggest leaves are often fragmentary, all broken slabs are still recognized, e.g. by their tertiary venation (e.g. M-17 has horizontal tertiaries, while in M-16 they are clearly at an obtuse angle to the midvein) or their shape (e.g. M-18 and M-19 are very coriaceous but differ in the base shape, which is cordate and rounded respectively). Considering the high proportion of simple, entire margined leaves, the most important distinguishing characters are found in the higher orders of venation.
Climate data were estimated using two kinds of climate proxies; the first is simple linear regressions between a physiognomic and a climate parameter, and the second is an artificial neural network approach based on 31 leaf characters and 13 palaeoclimate variables (CLANN). Each morphotype was evaluated for the physiognomic characters used (see Supplement).
As simple linear regressions between a physiognomic and a climate parameter, we used the regression by Kowalski and Dilcher (2003) and Miller et al. (2006) to calculate temperature estimates based on leaf margin analyses. For the rainfall estimates based on leaf size, we employed the regression by Wilf et al. (1998).
Climate proxies were also checked using the CLANN method (i.e. an artificial neural network approach to CLAMP) (Li et al., 2016) calibrated using the CLAMP global data set, which considers 378 sites worldwide (Spicer et al., 2009). As in Li et al. (2016), the spread for each climatic variable was calculated using 10-fold cross-validation, repeated 1000 times. The scoresheet for the Baris flora is given in the Supplement (Data Sect. S1) as well as the code used.
The rarefied diversity, i.e. the diversity expected for a subsample of the collection, was estimated for individual localities (single collection point) and for the bulk floras. Concerning possible collection biases, it is worth noting that the material was collected very carefully by sedimentologists who collected almost everything, including tiny unidentifiable plant fragments, which abound in the collection. To test whether our assemblages are more similar to TRFs than to other kinds of vegetation, we compared the diversity of fossil samples from NE Africa with tropical and warm temperate associations from the Cretaceous, Paleocene, and Eocene. The data from Cerrejón, Castle Rock, the Bison Basin, Rio Negro, and Barro Colorado were taken from Wing et al. (2009) and compiled with data from Laguna del Hunco (Wilf et al., 2005) and Grünbach (Herman and Kvaček, 2010). The data from Grünbach are based on the number of specimens of each taxon (see Supplement). Only the bulk flora diversity could be estimated for Grünbach due to the absence of data concerning individual collection points. The rarefaction curves were produced using PAST (Krebs, 1989; Hammer et al., 2001).
4.1 Morphotypes
Previous works focused on the investigated material (Klitzsch and Lejal-Nicol, 1984; Lejal-Nicol, 1987; Kahlert et al., 2009) recorded only 37 taxa, while our current revision based on 361 specimens resulted in the identification of 70 morphotypes: 54 non-monocotyledon (dicots) angiosperms, 11 monocotyledons, 4 ferns, and a single conifer (Fig. 2). The presence of some morphotypes present in several assemblages (Table S1 in the Supplement), especially monocotyledons, suggests homogeneous vegetation for the whole area and supports their contemporaneity.
4.2 Climate
The physiognomy of the Baris assemblage is similar to that of a modern TRF. Of the 20 terrestrial non-monocotyledonous angiosperms with a preserved margin, 85 % are entire-margined, yielding estimates of mean annual temperature (MAT) ranging from >25 ∘C (26 to 33 ∘C) corresponding to a tropical climate (Wolfe, 1979). Furthermore, the average leaf size of the 23 terrestrial non-monocotyledonous angiosperms is 69 cm2, yielding an estimate of the mean annual precipitation (MAP) of 2609 mm based on the regression model of Wing et al. (2009) which is comparable to >1500 mm precipitation found in the Guineo-Congolian evergreen rainforests (White, 1983). The CLANN analysis results in slightly lower temperatures (24 ∘C) and precipitation (1795 mm).
The CLANN analysis also favours an equable climate with an annual amplitude of about 6 ∘C (warm month mean temperature, WMMT: 27 ∘C; cold month mean temperature, CMMT: 21 ∘C). This reflects the continuous growing season (12 months). Nevertheless, the precipitation of the three wettest months (Three-Wet: 911 mm/304 mm per month) is about twice the mean monthly precipitation (MMGSP: 150 mm), while the three driest months (131 mm/44 mm per month) indicate a dry season (month with less than 50 mm). This suggests a strong seasonality with a short dry season. Nevertheless, such seasonality is comparable to the Guinean part of the Guineo-Congolian TRF (White, 1983).
Table 1Diversity and composition. Information on the sites is given in the Supplement. Stotal, number of species in all samples; Salpha10, number of species in sample rarefied to 10 specimens; Salpha100, number of species in sample rarefied to 100 specimens; and Stotal100, number of species in all samples rarefied to 100 specimens.
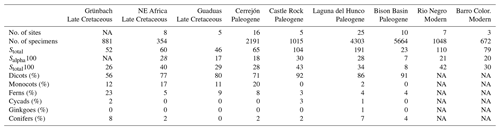
4.3 Diversity
Despite the low number of specimens, the diversity of the Baris flora rarefied at 100 specimens (the averaged diversity of a subsample consisting of 100 specimens) and appears comparable to the richest floras of the Paleogene and modern TRFs, as well as subtropical assemblages, and is richer and more diverse than the Paleocene temperate assemblages (Table 1). Moreover, the assemblages were also rarefied at 20 specimens; in order to include most assemblages, the same applies to the remaining assemblages of NE Africa. The total diversity of the Campanian of NE Africa displays the same pattern (Fig. 3).
4.4 Taxonomic composition
In terms of composition (Table 1), the African Campanian assemblages are characterized by the dominance of angiosperms, which account for 94 % of the flora. Among the mid-latitude floras, the Campanian Grünbach flora has only 68 % angiosperms, and only Paleogene assemblages exhibit more than 85 % angiosperms (e.g. Castle Rock). Even among the angiosperm-dominated assemblages (i.e. >85 % angiosperms), only the tropical assemblages of Guaduas, Cerrejón, and Africa are rich in monocots (11 %, 20 %, and 17 % respectively), while other assemblages, although extremely rich, display no more than 2 % monocots (i.e. Laguna del Hunco and Castle Rock). The high dominance of both monocots and other angiosperms and the near exclusion of other plant groups seem to be typical for both ancient and modern TRFs.
Furthermore, a closer comparison with the contemporaneous subtropical Grünbach flora, the Maastrichtian Guaduas flora, and the Paleocene Cerrejón TRF supports a closer affinity of the NE African vegetation with younger TRFs than with coeval subtropical vegetation.
A thorough comparison with Grünbach based on Herman and Kvaček (2010) reveals the complete absence of taxa common to both assemblages. Of particular importance is the absence of platanoid taxa in NE Africa, although they are very common in northern mid-latitudes floras. Among monocots, Araceae, which are abundant in NE Africa, also occur at Grünbach, but they are represented by Orontioideae, a subfamily that is currently restricted to the northern subtropical to temperate area (Mayo et al., 1997).
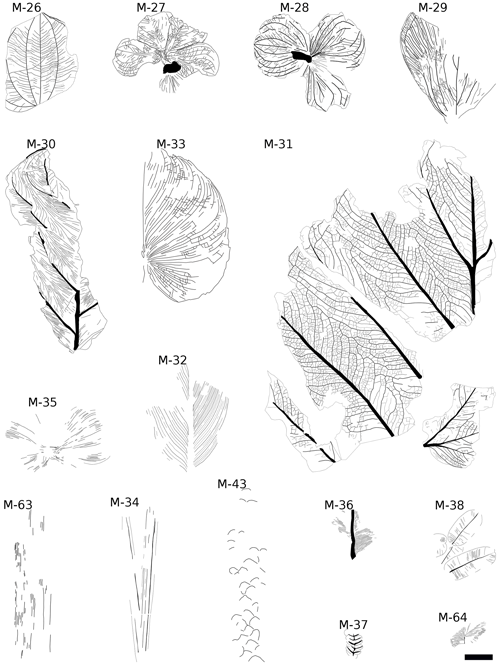
Figure 4Line drawings of monocot, conifer (only M-43), and fern (M-36, M-37, M-38, M-64) morphotypes, Baris, Egypt. The scale bar is 4 cm.
Considering its relation to the Guaduas assemblage (Carvalho et al., 2021), the Baris assemblage displays a piperalean morphotype (M-15) quite similar to Piper margaritae (Guaduas morphotype GD05), as well as a fern (M-36) similar to GD103. However, the monocots of Guaduas seem to be dominated by Zingiberales and palms, of which the latter have not been observed in NE Africa. Only two morphotypes have possible araceous affinities, but neither of them is similar to the Araceae of NE Africa. However, palynological evidence highlighted its occurrence in the Campanian in North Africa (e.g. Jardiné and Magloire, 1965; Méon, 1990; Mahmoud, 2003). The majority of palm and palm-like records in the Campanian (and Maastrichtian) are from coastal regions of western, northern, and eastern Africa (Somalia), e.g. Schrank (1994). These records also occurred in tropical South America and, to a lesser extent, in Southeast Asia, forming the core of the Late Cretaceous Palmae Province (Herngreen et al., 1996; Morley, 2000; Pan et al., 2006).
In contrast to Guaduas, the monocot assemblage of Cerrejón based on Wing et al. (2009) is to that at Baris, displaying not only Zingiberales (especially morphotype CJ49) similar to M-32 but also Montrichardia (CJ3) a colocasioid member of Araceae similar to Afrocasia (M-31) (Coiffard and Mohr, 2016), a probable Cobbania (M-29) (Coiffard and Mohr, 2018) (CJ59) and a member of Araceae similar to Lejalia (M-30) (Coiffard and Mohr, 2015) (CJ47). In addition to similarities with Cerrejón, terrestrial Araceae at Baris show affinities with modern TRF taxa. Furthermore, Afrocasia is a member of Aroideae with huge leaves that display close affinity with taxa that are usually found in tropical rainforests but also occur in warm temperate forests. More important is Lejalia, which is closely related to Homalomena and Philodendron, both genera that belong to groups restricted to tropical rainforests. Furthermore, in contrast to the above-mentioned taxa, Aroideae growing in seasonal (monsoonal) tropical vegetation have compound leaves, such as Amorphophallus. The dicot morphotypes CJ5 (Lauraceae) and CJ48 (Salicaceae) are similar to M-03 and M-04 respectively. M-03 and CJ5 share the presence of one pair of acute basal secondaries, simple brochidodromous secondaries, and simple agrophic veins. Also, M-04 and CJ-48 share the same basal actinodromous venation, well-developed simple agrophic veins, and a toothed margin. The teeth of M-04 also display spherulate apices.
The Campanian floral assemblages from NE Africa are thus more similar, in terms of physiognomy, species richness, and taxonomic composition, to younger tropical assemblages than to contemporary and younger temperate and subtropical forests.
The leaf physiognomy of the NE African Campanian leaf assemblages is consistent with a wet tropical climate comparable to that of modern African TRFs. Wet tropics during the Late Cretaceous agree with models predicting increased precipitation during the Late Cretaceous (Chaboureau et al., 2014) and suggest that a seasonal intersecting overlap of the Intertropical Convergence Zone (ITCZ) existed at least during the Campanian (Spicer et al., 2013).
Considering their composition, the NE African Campanian floras display a set of Aroideae that belong to typical TRF clades (Nauheimer et al., 2012) and thus support the idea that the Late Cretaceous tropics already housed such lineages under conditions similar to their modern habitat. This reconciles an apparent conflict between the fossil record and phylogenetic evidence (Eiserhardt et al., 2017). Such conflict was due to the almost complete absence of published tropical floras for the Cretaceous, with the notable exception of the Crato flora (Mohr and Friis, 2000), and some preliminary reports from NE Africa (Kahlert et al., 2009), as well as the recent record from the Maastrichtian of Guaduas from the central Andes of Colombia (Carvalho et al., 2021).
The Aptian Crato flora in NE Brazil is supposed to have grown in a rather (seasonally) dry climate and is dominated by gymnosperms (Mohr et al., 2006). The angiospermous component of this vegetation differs strongly from that of younger vegetation, consisting of magnoliids and early diverging eudicots and monocots (Coiffard et al., 2019). If the Crato flora is representative of tropical vegetation during the late Early Cretaceous, this implies that modern-looking TRFs must have developed during the 120–80 Ma time interval. Unfortunately, except for the Turonian floras of the Negev desert (Krassilov, 2005), fossil floras from this time interval are undersampled, although new collections are planned in Egypt and Sudan. The Turonian flora of the Negev desert (Krassilov, 2005) apparently grew further away from the palaeoequator and seems to correspond to a drier climate, and it has rather low diversity (19 leaf morphotypes) and strong affinities to Laurasia.
To sum up, the present results show that TRFs occurred in NE Africa during the Campanian in an area extending between 19 and 26∘ N and between 26 and 33∘ E, covering at least 550 000 km2, which corresponds to 10 % of the Amazonian or 25 % of the Congolese TRF.
A detailed investigation based on leaf physiognomy, species richness, and taxonomic composition was carried out on fossil leaf collections obtained from Baris Oasis, Egypt. The obtained results imply a wet tropical climate, comparable to that of modern African TRFs. In terms of vegetation composition, the Campanian NE African floras display a set of Aroideae that belong to typical TRF clades and thus support the idea that Late Cretaceous tropics already housed such lineages under conditions similar to their modern habitat.
Data in this paper are available in the main text. Further data are available in the Supplement as follows.
The supplement related to this article is available online at: https://doi.org/10.5194/bg-20-1145-2023-supplement.
CC contributed to the design and scope of the paper. JR provided the statistical and CLANN analysis. CC, HEA, RB, and DU contributed to writing and revising the manuscript. CC and HEA prepared the paper, with contributions from all co-authors.
The contact author has declared that none of the authors has any competing interests.
Publisher’s note: Copernicus Publications remains neutral with regard to jurisdictional claims in published maps and institutional affiliations.
We would like to thank the institutions and researchers cited in the text that made this publication possible. The paper benefitted greatly from the positive criticism of James A. Doyle and Salah Y. El Beialy.
This research has been supported by the Deutsche Forschungsgemeinschaft (grant no. CO1060/3-1) and the Alexander von Humboldt-Stiftung (grant no. EGY-1190326-GF-P).
This open-access publication was funded by the University of Tübingen.
This paper was edited by Petr Kuneš and reviewed by James Doyle and Salah El Beialy.
Abu El-Kheir, G. A.: Taphonomic conditions and assessment of the Late Cretaceous vertebrates bearing sites in the Western Desert, Egyptian Journal of Geology, 64, 471–484, 2020.
Amante, C. and Eakins, B.: ETOPO1 Global Relief Model Converted to PanMap Layer Format (NOAA-National Geophysical Data Center), https://doi.org/10.1594/PANGAEA.769615, 2009.
Barazi, N.: Sedimentologie und Stratigraphie des Abyad-Beckens (NW Sudan), Berliner geowissenschaftliche Abhandlungen, Reihe A, 64, 1–86, 1985.
Belcher, C. M., Yearsley, J. M., Hadden, R. M., McElwain, J. C., and Rein, G.: Baseline intrinsic flammability of Earth's ecosystems estimated from paleoatmospheric oxygen over the past 350 million years, P. Natl. Acad. Sci. USA, 107, 22448–22453, 2010.
Brown, S. A., Scott, A. C., Glasspool, I. J., and Collinson, M. E.: Cretaceous wildfires and their impact on the Earth system, Cretaceous Res., 36, 162–190, 2012.
Carvalho, M. R., Jaramillo, C., de la Parra, F., Caballero-Rodríguez, D., Herrera, F., Wing, S., Turner, B. L., D'Apolito, C., Romero-Báez, M., Narváez, P., Martínez, C., Gutierrez, M., Labandeira, C., Bayona, G., Rueda, M., Paez-Reyes, M., Cárdenas, D., Duque, A., Crowley, L. J., Santos, S., and Silvestro, D.: Extinction at the end-Cretaceous and the origin of modern Neotropical rainforests, Science, 372, 63–68, 2021.
Chaboureau, A.-C., Sepulchre, P., Donnadieu, Y., and Franc, A.: Tectonic-driven climate change and the diversification of angiosperms, P. Natl. Acad. Sci. USA, 111, 14066–14070, 2014.
Chamley, H.: Clay Minerals, in: Clay Sedimentology, Springer, Berlin, Heidelberg, https://doi.org/10.1007/978-3-642-85916-8_1, ISBN 9783642859168, 1989.
Coiffard, C. and Mohr, B. A. R.: Lejalia sagenopteroides gen. nov. et comb. nov.: A new tropical member of Araceae from Late Cretaceous strata of northern Gondwana (Jebel Abyad, Sudan), Taxon, 64, 987–997, 2015.
Coiffard, C. and Mohr, B. A. R.: Afrocasia kahlertiana gen. et sp. nov., a new tropical member of Araceae from Late Cretaceous strata of northern Gondwana (Baris, Egypt), Taxon, 65, 1374–1384, 2016.
Coiffard, C. and Mohr, B. A. R.: Cretaceous tropical Alismatales in Africa: diversity, climate and evolution, Bot. J. Linn. Soc., 188, 117–131, 2018.
Coiffard, C., Kardjilov, N., Manke, I., and Bernardes-de Oliveira, M. E.: Fossil evidence of core monocots in the Early Cretaceous, Nat. Plants, 5, 691–696, 2019.
Davis, C. C., Webb, C. O., Wurdack, K. J., Jaramillo, C. A., and Donoghue, M. J.: Explosive radiation of Malpighiales supports a Mid-Cretaceous origin of modern tropical rain forests, Am. Natural., 165, 36–65, 2005.
Dominik, W. and Schaal, S.: Anmerkungen zur Stratigraphie der Phosphate der Ober-Kreide (Campan) der westlichen Wüste, Ägypten, Berliner geowissenschaftliche Abhandlungen, Reihe A, 50, 153–175, 1984.
Eiserhardt, W. L. Couvreur, T. L. P., and Baker, W. J.: Plant phylogeny as a window on the evolution of hyperdiversity in the tropical rainforest biome, New Phytol., 214, 1408–1422, 2017.
El Atfy, H., Sallam, H., Jasper, A., and Uhl, D.: The first evidence of paleo-wildfire from the Campanian (Late Cretaceous) of North Africa, Cretaceous Res., 57, 306–310, 2016.
El Beialy, S.: Campanian-Maastrichtian palynomorphs from the Duwi (Phosphate) Formation of the Hamrawein and Umm El Hueitat mines, Red Sea Coast, Egypt. Rev. Palaeobot. Palynol., 85, 303–317, 1995.
Ellis, B., Daly, D., Hickey, L., Johnson, K., Mitchell, J., Wilf, P., and Wing, S.: Manual of Leaf Architecture. Cornell University Press, ISBN 9780801475184, 2009.
Estrada-Ruiz, E., Upchurch, G. R., and Cevallos-Ferriz, S. R.: Flora and climate of the Olmos Formation (upper Campanian–lower Maastrichtian), Coahuila, Mexico: a preliminary report, Gulf Coast Assoc. Geol. Soc. Trans., 58, 273–283, 2008.
Hammer, Ø. Harper, D. A., and Ryan P. D.: PAST, Paleontological statistics software package for education and data analysis, Palaeontol. Elec., 4, 1–9, 2001.
Herman, A. B. and Kvaček, J.: Late Cretaceous Grünbach Flora of Austria, Naturhistorisches Museum, 1–222, 2010.
Herngreen, G. F. W., Kedves, M., Rovnina, L. V., and Smirnova, S. B.: Cretaceous palynofloral provinces: a review, in: Palynology: Principles and Applications Vol. 3, edited by: Jansonius, J. and McGregor D. C. (Eds.), Dallas (TX): AASP Foundation, 1157–1188, 1996.
Jardiné, S. and Magloire L.: Palynologie et stratigraphie du Cretace des basins du Sénégal et de Côte D'Ivoire, Mémoires du Bureau de Recherches Géologiques Ministrie, 32, 187–245, 1965.
Kahlert, E.,Rüffle, L., and Gregor, H.: Die Oberkreide-Flora (Campanian) von Baris (Ägypten) und ihre ökologisch-geographischen Beziehungen unter plattentektonischen Aspekten, Documenta naturae, 71 pp., 2009.
Klitzsch, E. and Hermina, M.: The Mesozoic, in: Stratigraphic lexicon and explanatory notes to the geologic map of Egypt 1:500,000, edited by: Hermina, M., Klitzsch, E., and List, F., Conoco Incorporation, 77–139, 1989.
Klitzsch, E. and Lejal-Nicol, A.: Flora and fauna from strata in southern Egypt and northern Sudan. Berliner geowissenschaftliche Abhandlungen, Reihe A, 50, 47–79, 1984.
Klitzsch, E. and Wycisk, P.: Geology of the sedimentary basins of northern Sudan and bordering areas, Berliner geowissenschaftliche Abhandlungen, Reihe A, 75, 97–136, 1987.
Kowalski, E. A. and Dilcher, D. L.: Warmer paleotemperatures for terrestrial ecosystems, P. Natl. Acad. Sci. USA, 100, 167–170, 2003.
Krassilov, V.: Late Cretaceous (Turonian) Flora of Southern Negev, Israel, Pensoft Publishers, Moscow, Russia, ISBN 9546422290, 2005.
Krebs, C. J.: Ecological methodology, New York, NY: Harper and Row Publishers Inc., 654 pp., ISBN 9780321021731, 1989.
Lejal-Nicol, A.: Flores nouvelles du Paleozoique et du Mesozoique d'Egypte et du Soudan septentrional, Berliner geowissenschaftliche Abhandlungen, Reihe A, 75, 151–248, 1987.
Li, S.-F., Jacques, F. M. B., Spicer, R. A., Su, T., Spicer, T. E. V., Yang, J., and Zhou, Z.-K.: Artificial neural networks reveal a high-resolution climatic signal in leaf physiognomy, Palaeogeogr. Palaeoclim. Palaeoeco., 442, 1–11, 2016.
Mahmoud, M. S.: Palynology and palaeoenvironment of the Quseir Formation (Campanian) from central Egypt, J. Afr. Earth Sci., 36, 135–148, 2003.
Mayo, S. Bogner, J., and Boyce, P.: The Genera of Araceae (Continental Printing, Belgium), The Trustees, Royal Botanic Gardens, Kew, 1–374, 1997.
Méon, H.: Palynologic studies of the Cretaceous–Tertiary boundary interval at El Kef outcrop, northwestern Tunisia: paleogeographic implications, Rev. Palaeobot. Palynol., 65, 85–94, 1990.
Miller, I. M., Brandon, M. T., and Hickey, L. J.: Using leaf margin analysis to estimate the mid-Cretaceous (Albian) paleolatitude of the Baja BC block, Earth Planet. Sc. Lett., 245, 95–114, 2006.
Mohr, B. A. R. and Friis, E. M.: Early angiosperms from the Lower Cretaceous Crato Formation (Brazil), a preliminary report, Int. J. Plant Sci., 161, 155–167, 2000.
Mohr, B. A. R., Bernardes-de Oliveira, M. E., Barale, G., and Ouaja, M.: Palaeogeographic distribution and ecology of Klitzschophyllites, an early Cretaceous angiosperm in southern Laurasia and northern Gondwana, Cretaceous Res., 27, 464–472, 2006.
Morley, R. J.: Origin and evolution of tropical rain forests, Chichester: John Wiley and Sons, Ltd., 384 pp., 2000.
Nauheimer, L., Metzler, D., and Renner, S. S.: Global history of the ancient monocot family Araceae inferred with models accounting for past continental positions and previous ranges based on fossils, New Phytol., 195, 938–950, 2012.
Pan, A. D., Jacobs, B., Dransfield, J., and Baker, W. J.: The fossil history of palms (Arecaceae) in Africa and new records from the late Oligocene (28–27 Mya) of North-Western Ethiopia, Bot. J. Linn. Soc., 151, 69–81, 2006.
Schrank, E.: Nonmarine Cretaceous correlations in Egypt and northern Sudan: palynological and palaeobotanical evidence, Cretaceous Res., 13, 351–368, 1992.
Schrank, E.: Palynology of the Yesomma Formation in northern Somalia: a study of pollen, spores and associated phytoplankton from the Late Cretaceous Palmae Province, Palaeontographica B, 231, 63–112, 1994.
Schwarz, T. and Germann, K.: Weathering surfaces, laterite‐derived sediments and associated mineral deposits in north-east Africa, in: Palaeoweathering, Palaeosurfaces and Related Continental Deposits, edited by: Thiry, M., Coincon, R. M., Wiley-Blackwell, 367–390, https://doi.org/10.1002/9781444304190.ch15, 1995.
Scott, A. C., Bowman, D. M., Bond, W. J., Pyne, S. J., and Alexander, M. E.: Fire on earth: an introduction, Wiley-Blackwell, 434 pp., ISBN 978-1-119-95357-9, 2013.
Spicer, R. A., Herman, A. B., Yang, J., and Spicer, T. E.: Why future climate change is likely to be underestimated: evidence from palaeobotany, J. Bot. Soc. Ben., 67, 75–88, 2013.
Spicer, R. A., Valdes, P., Spicer, T. E. V., Craggs, H. J., Srivastava, G., Mehrotra, R. C., and Yang, J.: New developments in CLAMP: Calibration using global gridded meteorological data, Palaeogeogr. Palaeoclimatol. Palaeoeco., 283, 91–98, 2009.
Thiry, M.: Palaeoclimatic interpretation of clay minerals in marine deposits: an outlook from the continental origin, Earth-Sci. Rev., 49, 201–221, 2000.
Upchurch, G. R. and Wolfe, J.: Mid-Cretaceous to Early Tertiary vegetation and climate: evidence from fossil leaves and woods, in: The origins of angiosperms and their biological consequences, edited by: Friis, E. M., Chaloner, W. G. and Crane, P. H., Cambridge University Press, Cambridge, UK), 75–105, 1987.
Wessel, P. and Luis, J. F.: The GMT/MATLAB Toolbox. Geochemistry, Geophysics, Geosystems 18, 811–823, 2017.
White, F.: The Vegetation of Africa, Natural Resources Research UNESCO, 356 pp., ISBN 92-3-101955-4, 1983.
Widdowson, M.: Laterite and Ferricrete, in: Geochemical sediments and landscapes, edited by: Nash, D. J. and McLaren, S. J., Blackwell Publishing, Oxford, UK, 45–94, 2007.
Wilf, P., Johnson, K. R., Cuneo, N. R., Smith, M. E., Singer, B. S., and Gandolfo, M. A.: Eocene plant diversity at Laguna del Hunco and Río Pichileufú, Patagonia, Argentina, Am. Natural., 165, 634–650, 2005.
Wilf, P., Wing, S. L., Greenwood, D. R., and Greenwood, C. L.: Using fossil leaves as paleoprecipitation indicators: An Eocene example, Geology, 26, 203–206, 1998.
Wing, S. L., Herrera, F., Jaramillo, C. A., Gomez-Navarro, C., Wilf, P., and Labandeira, C. C.: Late Paleocene fossils from the Cerrejon Formation, Colombia, are the earliest record of Neotropical rainforest, P. Natl. Acad. Sci. USA, 106, 18627–18632, 2009.
Wolfe, J.: Temperature parameters of humid to mesic forests of eastern Asia and relation to forests of other regions of the northern hemisphere and Australasia, U.S. Geological Survey Professional, Paper 1106, 1979.
Wycisk, P.: Correlation of the major late Jurassic-early Tertiary low- and highstand cycles of southwest Egypt and north-west Sudan, Geol. Rundsch., 83, 759–772, 1994.