the Creative Commons Attribution 4.0 License.
the Creative Commons Attribution 4.0 License.
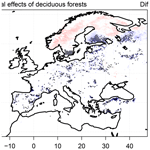
The potential of an increased deciduous forest fraction to mitigate the effects of heat extremes in Europe
Marcus Breil
Annabell Weber
Joaquim G. Pinto
Deciduous forests are characterized by a higher albedo, a reduced stomatal resistance, and a deeper root system in comparison to coniferous forests. As a consequence, less solar radiation is absorbed and evapotranspiration is potentially increased, making an increase in the deciduous forest fraction a potentially promising measure to mitigate the burdens of heat extremes for humans and nature. We analyze this potential by means of an idealized 30-year-long regional climate model (RCM) experiment, in which all coniferous forests in Europe are replaced by deciduous forests and compared to a simulation using the actual forest composition.
Results show that an increase in the deciduous forest fraction reduces the heat intensity during heat periods in most regions of Europe. During heat periods, there is a slight reduction in the mean daily maximum 2 m temperatures simulated of about 0.2 K locally and 0.1 K non-locally. Regions with a high cooling potential are southwestern France and northern Turkey, where heat period intensities are reduced by up to 1 K. Warming effects are simulated in Scandinavia and eastern Europe.
Although the cooling effect on heat period intensities is statistically significant over large parts of Europe, the magnitude of the temperature reduction is small. Consequently, an increase in the deciduous forest fraction only has a limited potential to reduce heat period intensities in Europe and can therefore only be considered as a supporting mitigation measure to complement more effective mitigation strategies.
More frequent and more intense heat periods constitute one of the most serious impacts of anthropogenic climate change in Europe (Seneviratne et al., 2021). Since the 1950s, the number of days with extreme heat has tripled and the intensity of heat extremes has increased by about 2.3 K in Europe (Lorenz et al., 2019). Although the intensities and characteristics of heat extremes depend on the applied heat extreme indices (Becker et al., 2022), the results of the latest CMIP6 projections indicate that this trend will continue within the next decades (Li et al., 2021). The resulting heat stress will entail enormous burdens for humans and nature. Therefore, in order to minimize future heat extreme impacts, effective mitigation strategies will be required.
In this context, one of the most frequently debated mitigation strategies to anthropogenic climate change is afforestation (e.g., Sonntag et al., 2016; Harper et al., 2018). Forests actively remove CO2 from the atmosphere and store the carbon long term in their biomass (Pan et al., 2011). Thus, afforestation has a beneficial biogeochemical effect on the greenhouse effect. Furthermore, the capability of forests to transpire water is higher than for other vegetation types (Bonan, 2008). A larger part of the available energy at the surface can consequently be transformed into latent heat instead of heating up the land surface (Strandberg and Kjellström, 2019).
Beyond these beneficial effects on the local surface energy balance, afforestation can also affect climate conditions non-locally (Winckler et al., 2019). For instance, Meier et al. (2021) showed that forests increase downwind precipitation in most regions of Europe, partially counteracting the projected precipitation decrease from climate change. Afforestation is therefore an indispensable component of all shared socioeconomic pathways (SSPs) for reaching the target of the Paris Agreement to keep the rise in mean global temperature well below 2 K above pre-industrial levels (Roe et al., 2019).
However, the efficiency of afforestation as a mitigation strategy strongly depends on the planted forest type (Jackson et al., 2008; Anderson et al., 2011). For instance, deciduous forests are brighter than coniferous forests (e.g., Breuer et al., 2003; Jackson et al., 2008; Otto et al., 2014). Thus, less solar radiation is absorbed and the energy input into the climate system is reduced. Moreover, deciduous forests are characterized by a deeper root system (e.g., Canadell et al., 1996) and a reduced stomatal resistance (Breuer et al., 2003; Carnicer et al., 2013). As a consequence, deciduous forests are able to extract water from deeper soil layers and the available amount of soil water for evapotranspiration is increased, reducing the water stress, particularly during heat periods (e.g., Brinkmann et al., 2019). Due to the reduced stomatal resistance of deciduous forests, the release of this additional water amount into the atmosphere via transpiration is facilitated and surface temperatures are reduced. The general effects of different forest types on the climate conditions are already documented (e.g., Duveiller et al., 2018; Luyssaert et al., 2018). For instance, using a statistical model based on remote sensing data, Schwaab et al. (2020) concluded that deciduous forests have an increased cooling effect on heat extremes in comparison to coniferous forests.
However, the current composition of European forests is dominated by coniferous trees (Bartholome and Belward, 2005) due to forestry reasons. The composition of primary European forests is different and depends on the regional conditions. In boreal and mountainous regions, cold and wet climate conditions cause a leaching and acidification of soils, favoring the dominant establishment of cold-tolerant coniferous trees (Bohn et al., 2000). Otherwise, primary European forests are mainly characterized by large deciduous tree fractions, like beech (Bohn et al., 2000). After humans started to cultivate landscapes in the course of the Holocene, European forests were extensively cleared for croplands, timber, and firewood (Kaplan et al., 2009), particularly during the medieval period (Pongratz et al., 2008). As a consequence, the forest cover on usable land for agriculture declined to under 6 % in central and western Europe in the mid-19th century (Kaplan et al., 2009). The resulting scarcity of timber and firewood made the management of forests necessary, which led to an intensive plantation of coniferous trees (McGrath et al., 2015). The persistent cold climate conditions of the so-called “little ice age” during this period, and the high yields of coniferous trees, favored their cultivation in Europe. This yield orientation is still driving forest management today (Ceccherini et al., 2020); it is also the reason why primary forests only cover 0.7 % of Europe's forest area today (Sabatini et al., 2018).
The resulting high proportion of coniferous trees in today's European forests and the associated dark vegetation surface results in the absorption of comparatively large amounts of solar radiation, and a relatively lower fraction of this available energy amount is transformed into latent heat. The energy balance of European forests is consequently not ideal, potentially increasing the intensity and duration of heat extremes. Therefore, a potential strategy to optimize this energy balance, and thus to mitigate hot temperature extremes in Europe, would be to increase the broadleaf tree fraction in European forests. The goal of this study is to investigate this mitigation potential and quantify its effects in an idealized setup.
For this purpose, we designed an idealized multidecadal modeling experiment, in which the whole coniferous forest fraction in Europe is replaced by deciduous forests. In order to simulate the complex effects of such a forest replacement on the regional climate system as accurately as possible, a regional climate model (RCM) is applied by which global reanalysis data are downscaled over Europe. The results of this RCM simulation (DECID) are compared with the results of a reference simulation (REF), in which the actual composition of European forests is used. By means of this idealized modeling experiment, we are able to quantify the general potential effect of an increase in the deciduous forest fraction on heat extreme characteristics in Europe. The design of the modeling experiment is described in Sect. 2. In Sect. 3, the general potential effect of an optimized composition of European forests on the intensity (Sect. 3.1) and duration (Sect. 3.2) of heat periods is assessed. Results are discussed in Sect. 4 and conclusions are drawn in Sect. 5.
In the course of this study, the regional climate model COSMO-CLM (CCLM; Rockel et al., 2008) is two-way coupled to the land surface model VEG3D (Breil and Schädler, 2021) and used to simulate the effects of an increased deciduous forest fraction on heat extremes in Europe. The CCLM-VEG3D runs are performed for the Coordinated Downscaling Experiment – European Domain (EURO-CORDEX; Jacob et al., 2014) on a horizontal resolution of 0.11∘ (∼ 12.5 km). All simulations were driven by ERA5 reanalyses (Hersbach et al., 2020) at the lateral boundaries and the lower boundary over sea. The simulation period is 1986–2015 and a spin-up of 7 years was performed before 1986.
In the first simulation, yearly updated maps of the actual land cover conditions in Europe are implemented in CCLM-VEG3D (Fig. 1a). This experiment constitutes the reference simulation (REF). The applied land use maps were developed in the framework of the Land Use and Climate Across Scales (LUCAS) project (Davin et al., 2020), based on the European Space Agency Climate Change Initiative Land Cover (ESA-CCI LC) dataset (ESA, 2017). Changes in the land use cover during the simulation period were derived from the Land-Use Harmonization 2 (LUH2) dataset (Hurtt et al., 2020). A detailed description of the method, by which this land use dataset was created, can be found in Hoffmann et al. (2022a).
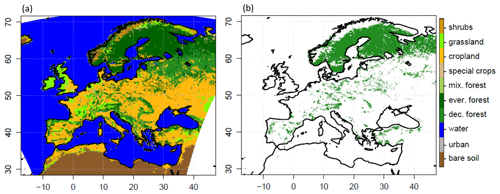
Figure 1(a) CCLM-VEG3D land use classes. (b) Grid cells in which coniferous forests were replaced by deciduous forests in the DECID simulation.
Since only the dominant land use class in a grid cell is considered in VEG3D, land use changes are only taking place in CCLM-VEG3D if the dominant land use class in a grid cell is changing. In addition, the vegetation characteristics of different deciduous tree species (beech, oak, etc.) and different coniferous tree species (pine, spruce, etc.) are all combined in one representative forest class, respectively. This means that for the different vegetation parameters, describing the characteristics of these different tree species, the mean values over the parameter space of the respective deciduous and coniferous trees are used. In CCLM-VEG3D, therefore, only one deciduous forest class and one coniferous forest class are considered (see Table 1). In this context, for the deciduous forest class, only deciduous broadleaf trees are considered, while in the coniferous forest class, only evergreen needleleaf trees are included. Evergreen broadleaf trees (e.g., Mediterranean oaks) or deciduous needleleaf trees (e.g., larch) are consequently not considered. This approach is associated with a certain degree of uncertainty since the parameter spaces of deciduous and coniferous trees have a certain overlap (e.g., Wright et al., 2005; van Bodegom et al., 2012). This means that the characteristics of several deciduous and coniferous tree species do show a high level of agreement. However, we assume in our approach that the parameter similarity is generally higher within the respective deciduous and coniferous tree classes than between the two classes (e.g., Gitay and Noble, 1997). The averaged parameter values of deciduous and coniferous trees can consequently be used to differentiate between the general characteristics of these tree species. Therefore, the use of two representative forest classes in this study is assumed to be suitable for investigating the general effects of deciduous and coniferous trees on heat period characteristics.
In the second simulation (DECID), all grid cells covered with coniferous forests in REF are replaced by deciduous forests (Fig. 1b). As a consequence, deciduous forests in this simulation are located in regions where their growth is ecologically limited to some extent (e.g., Högberg et al., 2017). For instance, broadleaf deciduous forests generally have high nitrogen needs. However, soils are generally nitrogen poor in boreal regions; this explains why the ecological conditions are not optimal for the growth of deciduous forests and why only deciduous tree species with low nitrogen demands, like birch or poplar, grow naturally. Therefore, coniferous trees have a naturally high proportion in boreal forests. However, these ecological limitations of an increased deciduous forest cover fraction are not considered in this idealized sensitivity study; the focus is only on the potential climatological effects.
By comparing the results of this idealized DECID with the results of the REF, the general potential effect of an increase in the deciduous forest fraction on the intensity and duration of heat periods in Europe is assessed. In order to quantify changes in the heat period intensities, days above the 90th percentile of the daily maximum temperatures in 2 m height during summer (JJA) are analyzed. In this context, we define the heat period intensities as the mean daily maximum 2 m temperature for the warmest 10 % of summer days and compare these mean values for DECID and REF with each other. Changes in the duration of heat periods in both simulations are quantified by counting the number of days, in which the daily maximum 2 m temperature exceeds the 90th percentile of daily maximum temperatures in REF over at least 3 consecutive days (Russo et al., 2015). The analyzed processes are separated in local effects; changes in the climate conditions in grid cells with an increase in the deciduous forest fraction, which are directly caused by changes in the surface energy balance and non-local effects; and changes in the climate conditions in grid cells with no increase in the deciduous forest fraction, which are only indirectly caused by changes in the surface energy balance.
3.1 Heat period intensity
3.1.1 Local effects
We first analyze the local effects of an increased deciduous forest fraction on heat period intensities. Figure 2a shows the local changes in the net short-wave radiation for the regions in which coniferous forests were replaced by deciduous forests. Net short-wave radiation is reduced in all these regions due to the increased albedo of deciduous forests in comparison to coniferous forests. Thus, less radiative energy at the land surface is locally available. At the same time, in central and southern Europe, the latent heat fluxes of deciduous forests are increased (Fig. 2b) and the sensible heat fluxes are reduced (Fig. 2c). This indicates that, in a deciduous forest in central and southern Europe, the radiative energy input during heat periods is reduced and a larger part of this reduced available energy at the surface is additionally used for evapotranspiration instead of heating up the land surface. During heat periods, the replacement of coniferous forests with deciduous forests consequently leads to a local reduction in the daily maximum 2 m temperatures, and thus the heat period intensities (Fig. 2d). In northern Europe, or more precisely in the northern part of Scandinavia, this is not the case. In this region, a warming of the mean daily 2 m temperatures during heat periods is simulated with an increase in the deciduous forest fraction. This warming effect is directly caused by a reduction in the evapotranspiration rates in Scandinavia (Fig. 2b).
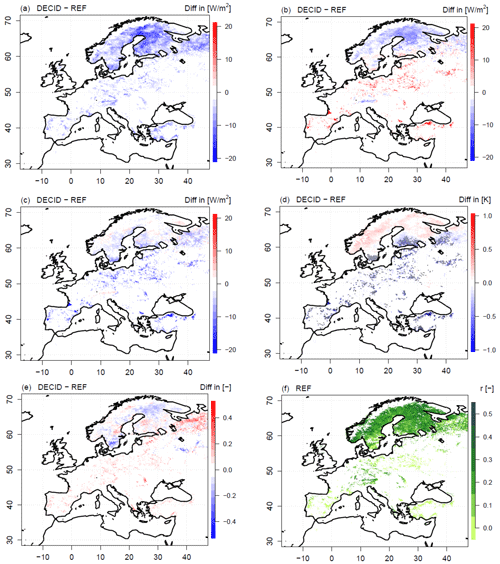
Figure 2Local differences between DECID and REF for (a) net short-wave radiation, (b) latent heat fluxes, (c) sensible heat fluxes, (d) daily maximum 2 m temperatures, and (e) temporal correlation between latent heat fluxes and daily maximum 2 m temperatures during heat periods. Panel (f) shows the temporal correlation between latent heat fluxes and daily maximum 2 m temperatures of coniferous forests in REF during heat periods. The black circles in (d) indicate significant results calculated with a Wilcoxon rank-sum test at a 95 % level.
During heat periods, different evapotranspiration responses to an increase of the deciduous forest fraction are caused by a different weighting of opposing vegetation characteristics of deciduous and coniferous forests. On the one hand, the stomatal resistance of a deciduous forest is reduced in comparison to a coniferous forest (see Table 1), and transpiration through the leaf stomata is facilitated. Furthermore, the root system of deciduous forests reaches deeper than that of coniferous forests (Table 1). Therefore, deciduous forests are able to extract water from deeper soil layers. Consequently, the available amount of soil water for evapotranspiration increases during phases of water limitation, reducing the water stress and enabling an enhanced evapotranspiration, particularly during heat periods. These two characteristics of deciduous forests have a facilitating effect on evapotranspiration during heat periods.
On the other hand, the albedo of a deciduous forest is higher than that of a coniferous forest (Table 1). Therefore, less solar radiation is absorbed and the energetic forcing of evapotranspiration is reduced (Fig. 2a). Additionally, the surface roughness of a deciduous forest is lower than that of a coniferous forest (Table 1). Thus, the turbulent transport of water from the surface to the atmosphere is not as efficient as for a coniferous forest. These two characteristics of deciduous forests consequently have an attenuating effect on evapotranspiration during heat periods.
In most parts of Europe, the weight of the reduced stomatal resistance and the reduced water stress of deciduous forests is dominating the evapotranspiration response during heat periods, and latent heat fluxes are increased (Fig. 2b). But this is not the case in Scandinavia. In general, the net short-wave radiation and the saturation deficit are lower in Scandinavia than in central and especially southern Europe. Thus, the energetic forcing of evapotranspiration and the atmospheric demand for evapotranspiration are reduced in comparison to the southern parts of Europe, generally attenuating evapotranspiration in Scandinavia (Breil et al., 2021). Now, by increasing the deciduous forest fraction in Scandinavia, the already comparatively low energetic forcing of evapotranspiration is further reduced (Fig. 2a). In addition, due to the generally low radiative energy input in Scandinavia, surface temperatures are lower than in central and southern Europe, with the consequence that buoyancy is comparatively small and wind sheer becomes more important for the turbulent exchange between the surface and the atmosphere. Therefore, the surface roughness has a stronger impact on evapotranspiration rates in Scandinavia than in the rest of Europe (Breil et al., 2021). In a deciduous forest, this surface roughness is lower than in a coniferous forest (Table 1). Wind sheer is consequently reduced and the turbulent transport of water between the surface and the atmosphere is further attenuated in Scandinavia, leading to reduced latent heat fluxes (Fig. 2b).
On top of this, even during heat periods in Scandinavia, evapotranspiration is not water limited due to the generally high water supply and the low energetic forcing. This is shown by the higher correlation between latent heat fluxes and daily maximum 2 m temperatures during heat periods in Scandinavia in comparison to central and southern Europe (Fig. 2f). This means that also for a coniferous forest in Scandinavia with its comparatively shallow root system, enough soil water is available to exploit its higher evaporative potential, which is caused by an increased energetic forcing and surface roughness. The increased radiative energy input of a coniferous forest can consequently be transformed into higher evapotranspiration rates (Fig. 2b), although its stomatal resistance is higher (Table 1). This increase in evapotranspiration is even so strong that lower daily maximum 2 m temperatures are simulated with coniferous forests, although more energy is available to heat up the surface in Scandinavia (Fig. 2a). Therefore, an increase in the deciduous forest fraction leads to more intense heat periods in Scandinavia (Fig. 2d).
The same weighting of processes also leads to increased latent heat fluxes for coniferous forests in the Alpine region and the low mountain ranges of central Europe (Fig. 2b). However, in these regions, the effects of the increased evapotranspiration is just balancing and not exceeding the effects of the increased radiative energy input of coniferous forests. As a result, the local intensities of heat periods are of the same magnitude for coniferous and deciduous forests (Fig. 2d).
In central and southern Europe, the radiative energy input is generally higher than in Scandinavia and thus also more buoyant. The effect of wind sheer and the surface roughness on the turbulent water transport is consequently less pronounced (Breil et al., 2021), and the weighting of the stomatal resistance on evapotranspiration is increased. In addition, the water stress during heat periods is lower for deciduous forests than for coniferous forests, due to the deeper root system of deciduous forests (Table 1), which is shown in Fig. 2e by the higher temporal correlation between latent heat fluxes and daily maximum 2 m temperatures in DECID than in REF. As a consequence, evapotranspiration of deciduous forests is increased, although the energetic forcing is lower than for coniferous forests. An increase in the deciduous forest fractions therefore leads to a local reduction in heat period intensities in central and southern Europe (Fig. 2d).
However, the absolute local effects of an increased deciduous forest fraction in Europe on the daily maximum 2 m temperatures are quite small during heat periods (Fig. 2d). The mean local reduction in the heat period intensity in Europe (except Scandinavia) is 0.2 K. Regions with a pronounced temperature reduction are located in southwestern France (0.6–0.9 K) and northern Turkey (up to 1 K). The highest simulated temperature reduction is 3.7 K. But such strong local effects are absolutely exceptional. At 95 % of the grid cells, in which an increase in the deciduous forest fraction leads to a local cooling, the reduction in the daily maximum 2 m temperatures is below 0.5 K.
Although this local cooling effect is only slightly pronounced, it is statistically significant for 45 % of all grid cells, in which an increase in the deciduous forest fraction resulted in reduced daily maximum 2 m temperatures, uniformly distributed all over Europe (except Scandinavia, Fig. 2d). This means that for nearly half of these grid cells, the process chain of reduced absorbed solar radiation and increased evapotranspiration results in a significant reduction in local heat period intensities for deciduous forests. However, this also means that for slightly more than half of these grid cells, the simulated reduction in the daily maximum temperatures is not significant. Thus, for these grid cells, random causes for the temperature reduction cannot be excluded.
The mean local warming in Scandinavia is 0.1 K, with a maximum warming effect of 0.4 K. Local warmings with an increase in the deciduous forest fraction are statistically not significant.
3.1.2 Non-local effects
An increase in the deciduous forest fraction has a non-local cooling effect on the heat period intensities over large parts of Europe in non-forested areas (Fig. 3a). Over central, western, and southern Europe, a non-local cooling of the daily maximum 2 m temperatures is simulated in general. The local cooling of air masses over deciduous forests consequently also induces a cooling of air masses in the surrounding areas. This non-local cooling effect is further intensified by generally higher precipitation sums in summer in regions showing a non-local cooling (Fig. 3b). Thus, the available amount of water for evapotranspiration in these regions is increased during heat periods and the water stress is reduced, resulting in higher evapotranspiration rates (Fig. 3c) and lower heat period intensities (Fig. 3a).
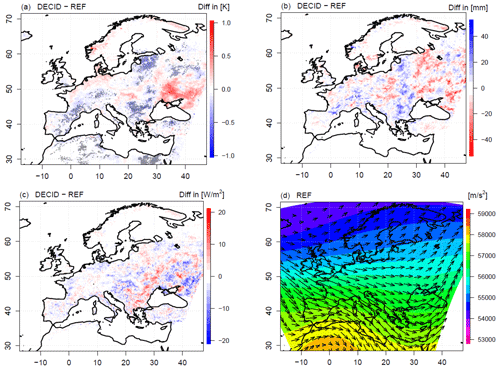
Figure 3Non-local differences between DECID and REF for (a) daily maximum 2 m temperatures during heat periods, (b) mean precipitation sums in summer, and (c) latent heat fluxes during heat periods. Panel (d) shows the geopotential height in 500 hPa and the mean wind direction (arrows) in REF during summer. The black circles in (a) indicate significant results calculated with a Wilcoxon rank-sum test a 95 % level.
However, in eastern Europe, at the North Sea coast of central Europe and the Balkan Mountains, higher daily maximum 2 m temperatures are simulated in non-forested areas (Fig. 3a). These warmer non-local temperatures arising with an increase in the deciduous forest fraction are also caused by non-local differences in the summer precipitation sums between DECID and REF. In all these regions, mean precipitation sums in summer are reduced (Fig. 3b), and thus, the available amount of water for evapotranspiration during heat periods is also reduced. Lower evapotranspiration rates are the consequence (Fig. 3c), which means that more energy is available at the surface to heat up the surface, finally leading to non-locally intensified heat periods.
These changes in the spatial distribution of precipitation sums in summer are most likely caused by local changes in the vegetation characteristics, associated with an increase in the deciduous forest fraction in Europe. Deciduous forests are increasing evapotranspiration rates locally (Fig. 2b), and thus the release of water into the atmosphere. Downwind precipitation is therefore potentially increased. However, deciduous forests are also reducing local temperatures (Fig. 2d) and are characterized by a lower surface roughness (Table 1), potentially inhibiting the development of convective precipitation events in summer in comparison to coniferous forests. Because of these opposing vegetation characteristics, an increase in the deciduous forest fraction can therefore lead to changes in the spatial and temporal precipitation distribution over Europe (Fig. 3c).
For instance, the locally increased evapotranspiration rates in central Europe (Fig. 2b) are increasing the water vapor content in the atmosphere. Thus, moister air masses are generally transported eastward with the typical westerly flow in Europe (Fig. 3d). We hypothesize that due to the increased water vapor content of this air mass, downwind rain is falling earlier and more extensively, leading to increased precipitation sums in a region from Greece to the Baltic countries (Fig. 3b). Further east, air masses are consequently drier and precipitation sums in summer are reduced (Fig. 3b), resulting in intensified heat periods (Fig. 3a).
A slight non-local warming during heat periods is additionally simulated in the coastal regions of Scandinavia (Fig. 3a). This warming is not caused by reduced precipitation sums but is a direct consequence of the warmer air masses above forested regions in Scandinavia. This is shown by the fact that evapotranspiration rates are slightly increased instead of reduced (Fig. 3c). Thus, changes in the evapotranspiration rates here are a result of warmer temperatures and not their cause, as it is the case in eastern Europe.
Quantitatively, the non-local effects of an increased deciduous forest fraction in Europe are of the same magnitude as the local effects, and thus quite small (Fig. 3a). The non-local warming effect in eastern Europe, at the North Sea coast of central Europe and the Balkan Mountains, has a mean value of 0.1 K and statistically not significant. The warming at 95 % of the respective grid cells is again below 0.4 K. The non-local cooling effect over central, western, and southern Europe also has a mean value of 0.1 K with a 95th percentile of 0.3 K. As already identified for the local effects, non-local cooling effects are statistically significant for 23 % of all grid cells with reduced daily maximum 2 m temperatures (Fig. 3a). However, this also means that the simulated non-local reduction in the daily maximum temperatures is statistically not significant for the other 77 % of these grid cells. Although the same process chain is simulated for these non-significant grid cells as for the significant grid cells, random causes for the temperature reduction during heat periods cannot be excluded.
3.2 Heat period duration
We finally analyze potential impacts of an increase in the deciduous forest fraction in Europe on changes in the heat period durations (Fig. 4). Therefore, in both simulations a heat event is defined as a period in which the daily maximum 2 m temperature exceeds the 90th percentile of daily maximum temperatures in the reference run over at least 3 consecutive days.
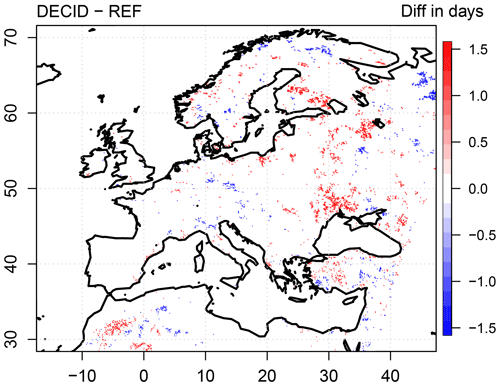
Figure 4Differences between DECID and REF for the mean heat period durations over the whole simulation period from 1986–2015.
Results show that the duration of heat periods is generally not affected by changes in the European forest composition. In eastern Europe, isolated grid cells with a heat period extension of up to 1 d are simulated. In central Europe and northern Scandinavia, a few grid cells with a shortening of heat periods are simulated. However, these grid cells are not systematically connected to local or non-local processes. Thus, no significant impact of an increase in the deciduous forest fraction on heat period durations is detected in Europe.
The results of this study show that the benefit of an increased deciduous forest fraction on the heat period intensities in Europe strongly depends on the water availability for evapotranspiration. In northern Europe, evapotranspiration of (deciduous as well as coniferous) forests is not water limited under current climate conditions, even during heat periods (Fig. 2e, f). By means of a higher energetic forcing (Fig. 2a) and a higher surface roughness, coniferous forests are consequently able to transpire more water than deciduous forests (Fig. 2b). This simulated property of coniferous forests in northern Europe depends of course on the considered vegetation parameters. As mentioned in Sect. 2, the used vegetation parameters in Table 1 are averaged values over the parameter space of deciduous and coniferous forests and consequently vary for specific deciduous and coniferous tree species. This variability in the vegetation characteristics is also reflected in observations. For instance, higher transpiration rates of deciduous forests are reported in Baldocchi et al. (2000), who reviewed several field studies in Canada, Siberia, and Scandinavia; in Eugster et al. (2000) based on the analysis of several eddy-covariance stations in the boreal regions of Europe and northern America; and in Grossiord et al. (2013) for measurements in a boreal plantation in southwestern Finland. On the other hand, contradictory results have been reported in Augusto et al. (2015), who reviewed studies on the water use efficiencies of deciduous and coniferous forests in boreal regions, implying higher transpiration rates of coniferous forests; in Ewers et al. (2005) for a comparison between pines and poplar in the BOREAS Northern Study Area in Canada; and in Baumgarten et al. (2019), where higher transpiration rates of coniferous forests are measured in hemiboreal regions, even during the warm summer months.
The increased transpiration rates of coniferous forest identified in our model study are consequently within the range of the observed transpiration variability in boreal regions and lead to slightly increased heat period intensities for northern Europe in our simulations (Fig. 2d). The low potential of an increased deciduous forest fraction to reduce heat period intensities in northern Europe was already suggested in Schwaab et al. (2020), who applied a statistical model. The application of a regional climate model with its capability to account for all associated atmospheric feedbacks indicates that this potential might not only be low but also negative. However, considering the missing significance of the warming effect of an increased deciduous forest fraction in Scandinavia in the model and the observed variability in the sign of the transpiration response, a final assessment of the mitigation potential for heat extremes in northern Europe is not possible.
Conversely, evapotranspiration is moisture limited during heat periods in central and southern Europe (Fig. 2e, f). For the heat intensities, an increase in the deciduous forest fraction is beneficial in these regions (Fig. 2d) because of the deeper root system of deciduous forests and the associated increased evapotranspiration rates (Fig. 2b). However, in some regions of southern Europe, current climate conditions are already so dry that the root system of a deciduous forest does not reach deep enough to meet the atmospheric water demand during heat periods. Therefore, the benefit of an increased deciduous forest fraction arises in these areas only from the reduced radiative energy input (Fig. 2a). These effects of an increased deciduous forest fraction were already hypothesized by Schwaab et al. (2020) and are now underpinned by this study.
However, the results of recent climate projections indicate that the water availability for evapotranspiration will change in Europe in the future. For any climate change scenario, regional as well as global climate models (GCMs) simulate a dipole in the projected precipitation changes in Europe (Douville et al., 2021; Coppola et al., 2021). In northern Europe, precipitation will slightly increase, while in southern Europe, the opposite is the case, particularly during summer. Simultaneously, the atmospheric water demand will increase in both regions, due to the generally increased atmospheric temperatures. In northern Europe, this will likely lead to a slight reduction in the available soil water amount, although precipitation sums are slightly increased (Cook et al., 2020). This might have the consequence that the evapotranspiration of shallow-rooted coniferous forests in northern Europe will become water limited, and the cooling effect of coniferous forests on heat period intensities might get smaller in comparison to deciduous forests.
In southern Europe, reduced precipitation sums and increased atmospheric water demand will result in more frequent drought conditions (e.g., Mömken et al., 2022). It can therefore be concluded that in southern Europe, the cooling effect of an increased deciduous forest fraction on the heat period intensities will even decrease since progressing water limitation will further constrain evapotranspiration for deciduous forests in future as well, as it is already the case in the driest regions of southern Europe (e.g., Forner et al., 2018). Thus, the climate benefit of deciduous forests will then be restricted only on the reduced incoming solar radiation.
Central Europe is in the transition zone of this precipitation dipole (Giorgi and Coppola, 2007). Thus, in this region, the lowest changes in water availability are expected with climate change in annual mean (Douville et al., 2021; Coppola et al., 2021). This means that in the future, an increase in the deciduous forest fraction will also have a slight cooling effect on the heat period intensities in central Europe. However, the location of this transition zone is considerably varying between models (GCMs and RCMs) and climate change scenarios (Coppola et al., 2021). Therefore, uncertainties about changes in the spatial water availability in central Europe, and thus about the projected changes in evapotranspiration rates, are quite large (Douville et al., 2021), whereby a small decrease in water availability is projected in the ensemble mean (Samaniego et al., 2018; Cook et al., 2020). This indicates that particularly during very extreme heat events, the likelihood of water stress will also increase for deciduous forests in central Europe and the cooling effect of an increased deciduous forest fraction on heat period intensities will likely decline.
Non-local changes in heat period intensities are also caused by changes in the available water amounts for evapotranspiration. In non-forested areas, these changes are obviously not caused by changes in the vegetation characteristics but are a result of changes in the mean summer precipitation sums. The interrelation between evapotranspiration rates of forests and downwind precipitation sums was already investigated by Belušić et al. (2019), Strandberg and Kjellström (2019), and Meier et al. (2021). While Strandberg and Kjellström (2019) could find almost no connection, Belušić et al. (2019) and Meier et al. (2021) provided evidence that increased evapotranspiration rates of forests can lead to increased downwind precipitation sums. It is therefore evident that a change in the forest composition and associated evapotranspiration rates also affects downwind precipitation sums in Europe. However, besides the local increase in evapotranspiration rates, deciduous forests are also characterized by lower local temperatures and surface roughness, inhibiting the formation of convective precipitation. Therefore, an increase in the deciduous forest fraction changes the spatial and temporal distribution of precipitation sums over Europe (Fig. 3c) and leads, in this way, either to an increase or a decrease of the non-local heat period intensities (Fig. 3a).
However, in our study, the effects of an increased deciduous forest fraction on heat period intensities are not as clearly pronounced as one could expect from the results of other studies like Schwaab et al. (2020). On the one hand, this might be due to the different methodological designs of the studies. In contrast to statistically based approaches as used in Schwaab et al. (2020), relevant atmospheric feedback processes are explicitly simulated in our regional climate model approach, potentially attenuating the impact of different vegetation characteristics on heat period intensities.
On the other hand, this might be related to the general representation of these vegetation characteristics in the regional climate model itself. In CCLM-VEG3D, different species of deciduous and coniferous trees are all aggregated in one representative forest class, respectively (see Table 1). However, the same species of deciduous and coniferous trees do not grow everywhere in Europe (Bohn and Gollub, 2006), and these different tree types do not all have the same vegetation characteristics. An example of such differences are the different vegetation characteristics of beech trees and oaks. The stomatal resistance of beech trees is lower than that of oaks (Jonard et al., 2011), while the root system of oaks reaches deeper than that of beech trees (Leuschner et al., 2001). It is therefore possible that the vegetation characteristics of deciduous and coniferous forests in CCLM-VEG3D are slightly overestimated at some locations or slightly underestimated at others. Thus, an increase in the deciduous forest fraction can have slightly deviating effects on the heat period intensities locally in a regional climate model.
Another model constraint of CCLM-VEG3D is that only the dominant land use class is considered in a grid cell. This means that grid cells in which forest is the dominant land use class are completely assigned to forest in the model and the forest fraction is overestimated in these areas. In return, forested areas with a lower percentage in a grid cell are consequently not considered in the model and the forest fraction is underestimated. The spatial distribution of forests in Europe is therefore not as extensive in CCLM-VEG3D as in reality (Hoffmann et al., 2022b), leading to a potential underestimation of the spatial extension of local effects. However, the goal of this study is to disentangle the general feedback processes of an increased deciduous forest and its general effects on local and non-local heat period intensities and not to analyze the effects of realistic transformations in the forest composition in Europe. Against this background, from our point of view, the use of the dominant land use class is reasonable and suitable to investigate general deciduous forest effects on heat periods. Of course, the simulated responses of other modeling systems to changes in the forest cover composition might be different.
Beyond these model constraints, the advantage of our modeling approach is that both local and non-local effects of an increased deciduous forest fraction can be analyzed in detail, under the consideration of all relevant feedback processes represented in the regional climate model. This is not possible with studies focusing on plant physiological differences of trees and their effects on the local energy budget of forests. Thus, our study contributes to complementing our knowledge on the general effects of deciduous forests on heat period intensities, by deriving a comprehensive understanding of the associated local and non-local process chains. With this in mind, we could show that an increase in the deciduous forest fraction has significant as well as non-significant effects on local and non-local heat wave intensities. While consistent physical process chains are the reason for the local and non-local temperature reductions during heat periods in the grid cells with significant effects, random causes for the temperature reductions in non-significant grid cells cannot generally be excluded. However, a missing significance does not necessarily mean that there is no connection (Wasserstein and Lazar, 2016) between an increase in the deciduous forest fraction and reduced heat period intensities in these grid cells. On the contrary, also for the non-significant grid cells with reduced daily maximum temperatures, the same process chains were identified as for the significant ones. From our point of view, this high physical consistency of the simulated processes is a strong indicator that the reduced heat period intensities are also not random in the non-significant grid cells. In particular, downwind processes are spatially and temporally highly variable. Thus, locally induced changes in the atmospheric moisture conditions do not always lead to precipitation at the same downwind locations (Perugini et al., 2017). This high spatial and temporal variability, therefore, has the consequence that the physical processes are difficult to detect and the temperature reductions are statistically not significant. However, the potential of an increased deciduous forest fraction to reduce heat period intensities is small in comparison to the substantial intensification of heat extremes of about 2.3 K in Europe since the 1950s (Lorenz et al., 2019).
In the course of idealized regional climate simulations, the general potential effects of an increased deciduous forest fraction on heat period characteristics in Europe are quantified. Results show that an increase in the deciduous forest fraction has significant as well as non-significant effects on the local and non-local scale. Locally, mean heat period intensities are slightly reduced about 0.2 K, except for Scandinavia, where a mean warming of 0.1 K is simulated. The simulated temperature reductions in grid cells with replaced coniferous forests are statistically significant at 45 % of the grid cells and not significant at 55 % of the grid cells. The simulated local warming in Scandinavia is not statistically significant.
Non-locally, mean heat period intensities are slightly reduced by about 0.1 K in central, western, and southern Europe, but they slightly increased in eastern Europe, the North Sea coast of central Europe, and the Balkan Mountains, by about 0.1 K as well. Significant results are only simulated for 23 % of the grid cells in which an increase of the deciduous forest fraction leads to a cooling. The duration of heat periods is not affected by a change in the forest composition in Europe.
These results indicate that an increase in the deciduous forest fraction has no potential to reduce the intensity of heat periods in Scandinavia. This might change in future to a certain extent since a slight decrease in water availability is projected in this region by regional as well as global climate models. This might limit evapotranspiration rates of shallow-rooted coniferous forest during heat periods, and the cooling effect of coniferous forests on heat period intensities might get smaller in comparison to deciduous forests. Furthermore, an increase in the deciduous forest fraction leads to reduced precipitation sums and non-locally intensified heat periods in several regions of Europe.
In central and southern Europe, deciduous forests have a cooling effect on heat period intensities. Although this effect is significant in part, its magnitude is rather small in comparison to the intensification of heat periods since the 1950s. In addition, the cooling effect is likely to decrease with climate change due to a projected reduction in water availability during heat periods. However, not all deciduous forest types necessarily have such small effects. For instance, species which have an optimal balance between a reduced absorption of solar radiation and an increased transformation of the solar radiation in turbulent heat fluxes might reduce heat period intensities stronger at certain locations. But such species are not considered in the general forest classes of CCLM-VEG3D, and their impact on heat period intensities is consequently not simulated in the model. Therefore, a next step will be to implement more forest classes in the regional climate model, enabling a more detailed differentiation of the respective vegetation characteristics. However, the results of our study indicate that a replacement of coniferous forests with common deciduous forest types only has a limited cooling effect on heat periods in Europe. Thus, the method can only be considered as a supporting mitigation measure to complement other, more effective mitigation strategies for reducing heat extreme intensities.
The applied land use dataset is accessible at the World Data Center for Climate (WDCC) at DKRZ (https://doi.org/10.26050/WDCC/LUC_hist_EU_v1.1, Hoffmann et al., 2022c). The ERA5 reanalysis data are obtained from the ECMWF (https://doi.org/10.24381/cds.adbb2d47, Hersbach et al., 2018). The CCLM-VEG3D data are available upon request from the corresponding author.
MB designed the study, performed the CCLM-VEG3D simulations and wrote the paper. MB and AW analyzed the data and MB prepared the figures. MB, AW, and JGP contributed with discussion, interpretation of results, and text revisions.
The contact author has declared that none of the authors has any competing interests.
Publisher’s note: Copernicus Publications remains neutral with regard to jurisdictional claims in published maps and institutional affiliations.
Joaquim G. Pinto was supported by the AXA Research Fund.
This paper was edited by Sebastiaan Luyssaert and reviewed by two anonymous referees.
Anderson, R. G., Canadell, J. G., Randerson, J. T., Jackson, R. B., Hungate, B. A., Baldocchi, D. D., Ban-Weiss, G. A., Bonan, G. B., Caldeira, K., Cao, L., Diffenbaugh, N. S., Gurney, K. R., Kueppers, L. M., Law, B. E., Luyssaert, S., and O'Halloran, T. L.: Biophysical considerations in forestry for climate protection, Front. Ecol. Environ., 9, 174–182, https://doi.org/10.1890/090179, 2011.
Augusto, L., De Schrijver, A., Vesterdal, L., Smolander, A., Prescott, C., and Ranger, J.: Influences of evergreen gymnosperm and deciduous angiosperm tree species on the functioning of temperate and boreal forests, Biol. Rev., 90, 444–466, https://doi.org/10.1111/brv.12119, 2015.
Baldocchi, D., Kelliher, F. M., Black, T. A., and Jarvis, P.: Climate and vegetation controls on boreal zone energy exchange, Glob. Change Biol., 6, 69–83, https://doi.org/10.1046/j.1365-2486.2000.06014.x, 2000.
Bartholome, E. and Belward, A. S.: GLC2000: a new approach to global land cover mapping from Earth observation data, Int. J. Remote Sens., 26, 1959–1977, https://doi.org/10.1080/01431160412331291297, 2005.
Baumgarten, M., Hesse, B. D., Augustaitienė, I., Marozas, V., Mozgeris, G., Byčenkienė, S., Mordas, G., Pivoras, A., Pivoras, G., Juonyte, D., Ulevicius V., Augustaitis, A., and Matyssek, R.: Responses of species-specific sap flux, transpiration and water use efficiency of pine, spruce and birch trees to temporarily moderate dry periods in mixed forests at a dry and wet forest site in the hemi-boreal zone, J. Agr. Meteorol., 75, 13–29, https://doi.org/10.2480/agrmet.D-18-00008, 2019.
Becker, F. N., Fink, A. H., Bissolli, P., and Pinto, J. G.: Towards a more comprehensive assessment of the intensity of historical European heat waves (1979–2019), Atmos. Sci. Lett., 23, e1120, https://doi.org/10.1002/asl.1120, 2022.
Belušić, D., Fuentes-Franco, R., Strandberg, G., and Jukimenko, A.: Afforestation reduces cyclone intensity and precipitation extremes over Europe, Environ. Res. Lett., 14, 074009, https://doi.org/10.1088/1748-9326/ab23b2, 2019.
Bohn, U. and Gollub, G.: The use and application of the map of the natural vegetation of Europe with particular reference to Germany, Biol. Environ., 106B, 199–213, https://doi.org/10.3318/BIOE.2006.106.3.199, 2006.
Bohn, U., Gollub, G., Hettwer, C., Neuhäuslová, Z., Raus, T., Schlüter, H., and Weber, H.: Karte der natürlichen Vegetation Europas, Maßstab 1 : 2 500 000 [Map of the Natural vegetation of Europe, Scale 1 : 2 500 000], Landwirtschaftsverlag, Münster, 2000.
Bonan, G. B.: Forests and climate change: forcings, feedbacks, and the climate benefits of forests, Science, 320, 1444–1449, https://doi.org/10.1126/science.1155121, 2008.
Breil, M. and Schädler, G.: The reduction of systematic temperature biases in soil moisture-limited regimes by stochastic root depth variations, J. Hydrometeorol., 22, 1897–1911, https://doi.org/10.1175/JHM-D-20-0265.1, 2021.
Breil, M., Davin, E. L., and Rechid, D.: What determines the sign of the evapotranspiration response to afforestation in European summer?, Biogeosciences, 18, 1499–1510, https://doi.org/10.5194/bg-18-1499-2021, 2021.
Breuer, L., Eckhardt, K., and Frede, H. G.: Plant parameter values for models in temperate climates, Ecol. Model., 169, 237–293, https://doi.org/10.1016/S0304-3800(03)00274-6, 2003.
Brinkmann, N., Eugster, W., Buchmann, N., and Kahmen, A.: Species-specific differences in water uptake depth of mature temperate trees vary with water availability in the soil, Plant Biol., 21, 71–81, https://doi.org/10.1111/plb.12907, 2019.
Canadell, J., Jackson, R. B., Ehleringer, J. B., Mooney, H. A., Sala, O. E., and Schulze, E. D.: Maximum rooting depth of vegetation types at the global scale, Oecologia, 108, 583–595, https://doi.org/10.1007/BF00329030, 1996.
Carnicer, J., Barbeta, A., Sperlich, D., Coll, M., and Peñuelas, J.: Contrasting trait syndromes in angiosperms and conifers are associated with different responses of tree growth to temperature on a large scale, Front. Plant Sci., 4, 409, https://doi.org/10.3389/fpls.2013.00409, 2013.
Ceccherini, G., Duveiller, G., Grassi, G., Lemoine, G., Avitabile, V., Pilli, R., and Cescatti, A.: Abrupt increase in harvested forest area over Europe after 2015, Nature, 583, 72–77, https://doi.org/10.1038/s41586-020-2438-y, 2020.
Cook, B. I., Mankin, J. S., Marvel, K., Williams, A. P., Smerdon, J. E., and Anchukaitis, K. J.: Twenty-first century drought projections in the CMIP6 forcing scenarios, Earth's Future, 8, e2019EF001461, https://doi.org/10.1029/2019EF001461, 2020.
Coppola, E., Nogherotto, R., Ciarlo', J. M., Giorgi, F., van Meijgaard, E., Kadygrov, N., Iles, C., Corre, L., Sandstad, M., Somot, S., Nabat, P., Vautard, R., Levavasseur, G., Schwingshackl, C., Sillmann, J., Kjellström, E., Nikulin, G., Aalbers, E., Lenderink, G., Christensen, O. B., Boberg, F., Sorland, S. L., Demory, M.-E., Bülow, K., Teichmann, C., Warrach-Sagi, K., and Wulfmeyer, V.: Assessment of the European Climate Projections as Simulated by the Large EURO-CORDEX Regional and Global Climate Model Ensemble, J. Geophys. Res.-Atmos., 126, e2019JD032356, https://doi.org/10.1029/2019JD032356, 2021.
Davin, E. L., Rechid, D., Breil, M., Cardoso, R. M., Coppola, E., Hoffmann, P., Jach, L. L., Katragkou, E., de Noblet-Ducoudré, N., Radtke, K., Raffa, M., Soares, P. M. M., Sofiadis, G., Strada, S., Strandberg, G., Tölle, M. H., Warrach-Sagi, K., and Wulfmeyer, V.: Biogeophysical impacts of forestation in Europe: first results from the LUCAS (Land Use and Climate Across Scales) regional climate model intercomparison, Earth Syst. Dynam., 11, 183–200, https://doi.org/10.5194/esd-11-183-2020, 2020.
Douville, H., Raghavan, K., Renwick, J., Allan, R. P., Arias, P. A., Barlow, M., Cerezo-Mota, R., Cherchi, A., Gan, T. Y., Gergis, J., Jiang, D., Khan, A., Pokam Mba, W., Rosenfeld, D., Tierney, J., and Zolina, O.: Water Cycle Changes, in: Climate Change 2021: The Physical Science Basis. Contribution of Working Group I to the Sixth Assessment Report of the Intergovernmental Panel on Climate Change, edited by: Masson-Delmotte, V., Zhai, P., Pirani, A., Connors, S. L., Péan, C., Berger, S., Caud, N., Chen, Y., Goldfarb, L., Gomis, M. I., Huang, M., Leitzell, K., Lonnoy, E., Matthews, J. B. R., Maycock, T. K., Waterfield, T., Yelekçi, O., Yu, R., and Zhou, B., Cambridge University Press, Cambridge, United Kingdom and New York, NY, USA, 1055–1210, https://doi.org/10.1017/9781009157896.010, 2021.
Duveiller, G., Hooker, J., and Cescatti, A.: The mark of vegetation change on Earth's surface energy balance, Nat. Commun., 9, 1–12, https://doi.org/10.1038/s41467-017-02810-8, 2018.
ESA: Land Cover CCI Product User Guide Version 2, Tech. rep., European Space Agency, https://www.esa-landcover-cci.org/?q=webfm_send/84 (last access: 16 June 2023), 2017.
Eugster, W., Rouse, W. R., Pielke Sr., R. A., Mcfadden, J. P., Baldocchi, D. D., Kittel, T. G. F., Chapin, F. S., Liston, G. E., Vidale, P. L., Vaganov, E., and Chambers, S.: Land–atmosphere energy exchange in Arctic tundra and boreal forest: available data and feedbacks to climate, Glob. Change Biol., 6, 84–115, https://doi.org/10.1046/j.1365-2486.2000.06015.x, 2000.
Ewers, B. E., Gower, S. T., Bond‐Lamberty, B., and Wang, C. K.: Effects of stand age and tree species on canopy transpiration and average stomatal conductance of boreal forests, Plant Cell Environ., 28, 660–678, https://doi.org/10.1111/j.1365-3040.2005.01312.x, 2005.
Forner, A., Valladares, F., Bonal, D., Granier, A., Grossiord, C., and Aranda, I.: Extreme droughts affecting Mediterranean tree species' growth and water-use efficiency: the importance of timing, Tree Physiol., 38, 1127–1137, https://doi.org/10.1093/treephys/tpy022, 2018.
Giorgi, F. and Coppola, E.: European climate-change oscillation (ECO), Geophys. Res. Lett., 34, L21703, https://doi.org/10.1029/2007GL031223, 2007.
Gitay, H. and Noble, I. R.: What are functional types and how should we seek them?, in: Plant Functional Types: Their Relevance To Ecosystem Properties And Global Change, edited by: Smith, T. M., Shugart, H. H., and Woodward, F. I., 3–19, Cambridge University Press, Cambridge, 1997.
Grossiord, C., Granier, A., Gessler, A., Pollastrini, M., and Bonal, D.: The influence of tree species mixture on ecosystem-level carbon accumulation and water use in a mixed boreal plantation, Forest Ecol. Manage., 298, 82–92, https://doi.org/10.1016/j.foreco.2013.03.001, 2013.
Harper, A. B., Powell, T., Cox, P. M., House, J., Huntingford, C., Lenton, T. M., Sitch, S., Burke, E., Chadburn, S. E., Collins, W. J., Comyn-Platt, E., Daioglou, V., Doelman, J. C., Hayman, G., Robertson, E., van Vuuren, D., Wiltshire, A., Webber, C. P., Bastos, A., Boysen, L., Ciais, P., Devaraju, N., Jain, A. K., Krause, A., Poulter, B., and Shu, S.: Land-use emissions play a critical role in land-based mitigation for Paris climate targets. Nat. Commun., 9, 1–13, https://doi.org/10.1038/s41467-018-05340-z, 2018.
Hersbach, H., Bell, B., Berrisford, P., Biavati, G., Horányi, A., Muñoz Sabater, J., Nicolas, J., Peubey, C., Radu, R., Rozum, I., Schepers, D., Simmons, A., Soci, C., Dee, D., and Thépaut, J.-N.: ERA5 hourly data on single levels from 1940 to present, Copernicus Climate Change Service (C3S) Climate Data Store (CDS) [data set], https://doi.org/10.24381/cds.adbb2d47, 2018.
Hersbach, H., Bell, B., Berrisford, P., Hirahara, S., Horanyi, A., Munoz-Sabater, J., Nicolas, J., Peubey, C., Radu, R., Schepers, D., Simmons, A., Soci, C., Abdalla, S., Abellan, X., Balsamo, G., Bechtold, P., Biavati, G., Bidlot, J., Bonavita, M., de Chiara, G., Dahlgren, P., Dee, D., Diamantakis, M., Dragani, R., Flemming, J., Forbes, R., Fuentes, M., Geer, A., Haimberger, L., Healy, S., Hogan, R. J., Holm, E., Janiskova, M., Keeley, S., Laloyaux, P., Lopez, P., Lupu, C., Radnoti, G., de Rosnay, P., Rozum, I., Vamborg, F., Villaume, S., and Thepaut, J.-N.: The ERA5 global reanalysis, Q. J. Roy. Meteor. Soc., 146, 1999–2049, https://doi.org/10.1002/qj.3803, 2020.
Hoffmann, P., Reinhart, V., Rechid, D., de Noblet-Ducoudré, N., Davin, E. L., Asmus, C., Bechtel, B., Böhner, J., Katragkou, E., and Luyssaert, S.: High-resolution land use and land cover dataset for regional climate modelling: Historical and future changes in Europe, Earth Syst. Sci. Data Discuss. [preprint], https://doi.org/10.5194/essd-2022-431, in review, 2022a.
Hoffmann, P., Rechid, D., Reinhart, V., Asmus, C., Böhner, J., Breil, M., Cardoso, R. M., Davin, E. L., Katragkou, E., Lima, D. C. A., Sofiadis, G., Strada, S., and Tölle, M. H.: Implementierung von Landnutzungsänderungsdaten in ein Ensemble von regionalen Klimamodellen, DACH2022, Leipzig, Deutschland, 21–25 Mar 2022, DACH2022-204, https://doi.org/10.5194/dach2022-204, 2022b (in German).
Hoffmann, P., Reinhart, V., and Rechid, D.: LUCAS LUC historical land use and land cover change dataset for Europe (Version 1.1), World Data Center for Climate (WDCC) at DKRZ [data set], https://doi.org/10.26050/WDCC/LUC_hist_EU_v1.1, 2022c.
Högberg, P., Näsholm, T., Franklin, O., and Högberg, M. N.: Tamm Review: On the nature of the nitrogen limitation to plant growth in Fennoscandian boreal forests, Forest Ecol. Manage., 403, 161–185, https://doi.org/10.1016/j.foreco.2017.04.045, 2017.
Hurtt, G. C., Chini, L., Sahajpal, R., Frolking, S., Bodirsky, B. L., Calvin, K., Doelman, J. C., Fisk, J., Fujimori, S., Klein Goldewijk, K., Hasegawa, T., Havlik, P., Heinimann, A., Humpenöder, F., Jungclaus, J., Kaplan, J. O., Kennedy, J., Krisztin, T., Lawrence, D., Lawrence, P., Ma, L., Mertz, O., Pongratz, J., Popp, A., Poulter, B., Riahi, K., Shevliakova, E., Stehfest, E., Thornton, P., Tubiello, F. N., van Vuuren, D. P., and Zhang, X.: Harmonization of global land use change and management for the period 850–2100 (LUH2) for CMIP6, Geosci. Model Dev., 13, 5425–5464, https://doi.org/10.5194/gmd-13-5425-2020, 2020.
Jackson, R. B., Randerson, J. T., Canadell, J. G., Anderson, R. G., Avissar, R., Baldocchi, D. D., Bonan, G. B., Caldeira, K., Diffenbaugh, N. S., Field, C. B., Hungate, B. A., Jobbagy, E. G., Kueppers, L. M., Nosetto, M. D., and Pataki, D. E.: Protecting climate with forests, Environ. Res. Lett., 3, 044006, 10.1088/1748-9326/3/4/044006, 2008.
Jacob, D., Petersen, J., Eggert, B., Alias, A., Christensen, O. B., Bouwer, L. M., Braun, A., Colette, A., Deque, M., Georgievski, G., Georgopoulou, E., Gobiet, A., Menut, L., Nikulin, G., Haensler, A., Hempelmann, N., Jones, C., Keuler, K., Kovats, S., Kröner, N., Kotlarski, S., Kriegsmann, A., Martin, E., van Meijgaard, E., Moseley, C., Pfeifer, S., Preuschmann, S., Radermacher, C., Radtke, K., Rechid, D., Rounsevell, M., Samuelsson, P., Somot, S., Soussana J.-F., Teichmann, C., Valentini, R., Vautard, R., Weber, B., and Yiou, P.: EURO-CORDEX: new high-resolution climate change projections for European impact research, Reg. Environ. Change, 14, 563–578, https://doi.org/10.1007/s10113-013-0499-2, 2014.
Jonard, F., André, F., Ponette, Q., Vincke, C., and Jonard, M.: Sap flux density and stomatal conductance of European beech and common oak trees in pure and mixed stands during the summer drought of 2003, J. Hydrol., 409, 371–381, https://doi.org/10.1016/j.jhydrol.2011.08.032, 2011.
Kaplan, J. O., Krumhardt, K. M., and Zimmermann, N.: The prehistoric and preindustrial deforestation of Europe, Quaternary Sci. Rev., 28, 3016–3034, https://doi.org/10.1016/j.quascirev.2009.09.028, 2009.
Leuschner, C., Hertel, D., Coners, H., and Büttner, V.: Root competition between beech and oak: a hypothesis, Oecologia, 126, 276–284, https://doi.org/10.1007/s004420000507, 2001.
Li, C., Zwiers, F., Zhang, X., Li, G., Sun, Y., and Wehner, M.: Changes in annual extremes of daily temperature and precipitation in CMIP6 models, J. Climate, 34, 3441–3460, https://doi.org/10.1175/JCLI-D-19-1013.1, 2021.
Lorenz, R., Stalhandske, Z., and Fischer, E. M.: Detection of a climate change signal in extreme heat, heat stress, and cold in Europe from observations, Geophys. Res. Lett., 46, 8363–8374, https://doi.org/10.1029/2019GL082062, 2019.
Luyssaert, S., Marie, G., Valade, A., Chen, Y. Y., Njakou Djomo, S., Ryder, J., Otto, J., Naudts, K., Lanso, A. S., Ghattas, J., and McGrath, M. J.: Trade-offs in using European forests to meet climate objectives, Nature, 562, 259–262, https://doi.org/10.1038/s41586-018-0577-1, 2018.
McGrath, M. J., Luyssaert, S., Meyfroidt, P., Kaplan, J. O., Bürgi, M., Chen, Y., Erb, K., Gimmi, U., McInerney, D., Naudts, K., Otto, J., Pasztor, F., Ryder, J., Schelhaas, M.-J., and Valade, A.: Reconstructing European forest management from 1600 to 2010, Biogeosciences, 12, 4291–4316, https://doi.org/10.5194/bg-12-4291-2015, 2015.
Meier, R., Schwaab, J., Seneviratne, S. I., Sprenger, M., Lewis, E., and Davin, E. L.: Empirical estimate of forestation-induced precipitation changes in Europe, Nat. Geosci., 14, 473–478, https://doi.org/10.1038/s41561-021-00773-6, 2021.
Moemken, J., Koerner, B., Ehmele, F., Feldmann, H., and Pinto, J. G.: Recurrence of drought events over Iberia. Part II: Future changes using regional climate projections, Tellus A, 74, 262–279, https://doi.org/10.16993/tellusa.52, 2022.
Otto, J., Berveiller, D., Bréon, F.-M., Delpierre, N., Geppert, G., Granier, A., Jans, W., Knohl, A., Kuusk, A., Longdoz, B., Moors, E., Mund, M., Pinty, B., Schelhaas, M.-J., and Luyssaert, S.: Forest summer albedo is sensitive to species and thinning: how should we account for this in Earth system models?, Biogeosciences, 11, 2411–2427, https://doi.org/10.5194/bg-11-2411-2014, 2014.
Pan, Y., Birdsey, R. A., Fang, J., Houghton, R., Kauppi, P. E., Kurz, W. A., Phillips, O. L., Shvidenko, A., Lewis, S. L., Canadell, J. G., Ciais, P., Jackson, R. B., Pacala, S. W., McGuire, A. D., Paio, S., Rautiainen, A., Sitch, S., and Hayes, D.: A large and persistent carbon sink in the world's forests, Science, 333, 988–993, https://doi.org/10.1126/science.1201609, 2011.
Perugini, L., Caporaso, L., Marconi, S., Cescatti, A., Quesada, B., de Noblet-Ducoudré, N., House, J. I., and Arneth, A.: Biophysical effects on temperature and precipitation due to land cover change, Environ. Res. Lett., 12, 053002, https://doi.org/10.1088/1748-9326/aa6b3f, 2017.
Pongratz, J., Reick, C., Raddatz, T., and Claussen, M.: A reconstruction of global agricultural areas and land cover for the last millennium, Global Biogeochem. Cy., 22, GB3018, https://doi.org/10.1029/2007GB003153, 2008.
Rockel, B., Will, A., and Hense, A.: The Regional Climate Model COSMO-CLM (CCLM), Meteorol. Z., 17, 347–348, https://doi.org/10.1127/0941-2948/2008/0309, 2008.
Roe, S., Streck, C., Obersteiner, M., Frank, S., Griscom, B., Drouet, L., Fricko, O., Gusti, M., Harris, N., Hasegawa, T., Hausfather, Z., Havlik, P., House, J., Nabuurs, G.-J., Popp, A., Sanz Sanchez, M. J., Sanderman, J., Smit, P., Stehfest, E., and Lawrence, D.: Contribution of the land sector to a 1.5 ∘C world, Nat. Clim. Change, 9, 817–828, https://doi.org/10.1038/s41558-019-0591-9, 2019.
Russo, S., Sillmann, J., and Fischer, E. M.: Top ten European heatwaves since 1950 and their occurrence in the coming decades, Environ. Res. Lett., 10, 124003, https://doi.org/10.1088/1748-9326/10/12/124003, 2015.
Sabatini, F. M., Burrascano, S., Keeton, W. S., Levers, C., Lindner, M., Pötzschner, F., Verkerk, P. H., Bauhus, J., Buchwald, E., Chaskovsky, O., Debaive, N., Horváth, F., Garbarino, M., Grigoriadis, N., Lombardi, F., Marques Duarte, , I., Meyer, P., Midteng, R., Mikac, S., Mikoláš, M., Motta, R., Mozgeris, G., Nunes, L., Panayotov, M., Ódor, P., Ruete, A., Simovski, B., Stillhard, J., Svoboda, M., Szwagrzyk, J., Tikkanen, O.-P., Volosyanchuk, R., Vrska, T., Zlatanov, T., and Kuemmerle, T.: Where are Europe's last primary forests?, Divers. Distrib., 24, 1426–1439, https://doi.org/10.1111/ddi.12778, 2018.
Samaniego, L., Thober, S., Kumar, R., Wanders, N., Rakovec, O., Pan, M., Zink, M., Sheffield, J., Wood, E. F., and Marx, A.: Anthropogenic warming exacerbates European soil moisture droughts, Nat. Clim. Change, 8, 421–426, https://doi.org/10.1038/s41558-018-0138-5, 2018.
Schwaab, J., Davin, E. L., Bebi, P., Duguay-Tetzlaff, A., Waser, L. T., Haeni, M., and Meier, R.: Increasing the broad-leaved tree fraction in European forests mitigates hot temperature extremes, Sci. Rep., 10, 1–9, https://doi.org/10.1038/s41598-020-71055-1, 2020.
Seneviratne, S. I., Zhang, X., Adnan, M., Badi, W., Dereczynski, C., Di Luca, A., Ghosh, S., Iskandar, I., Kossin, J., Lewis, S., Otto, F., Pinto, I., Satoh, M., Vicente-Serrano, S. M., Wehner, M., and Zhou, B.: Weather and Climate Extreme Events in a Changing Climate, in: Climate Change 2021: The Physical Science Basis. Contribution of Working Group I to the Sixth Assessment Report of the Intergovernmental Panel on Climate Change, edited by: Masson-Delmotte, V., Zhai, P., Pirani, A., Connors, S. L., Péan, C., Berger, S., Caud, N., Chen, Y., Goldfarb, L., Gomis, M. I., Huang, M., Leitzell, K., Lonnoy, E., Matthews, J. B. R., Maycock, T. K., Waterfield, T., Yelekçi, O., Yu, R., and Zhou, B., Cambridge University Press, Cambridge, United Kingdom and New York, NY, USA, 1513–1766, https://doi.org/10.1017/9781009157896.013, 2021.
Sonntag, S., Pongratz, J., Reick, C. H., and Schmidt, H.: Reforestation in a high-CO2 world – Higher mitigation potential than expected, lower adaptation potential than hoped for, Geophys. Res. Lett., 43, 6546–6553, https://doi.org/10.1002/2016GL068824, 2016.
Strandberg, G. and Kjellström, E.: Climate impacts from afforestation and deforestation in Europe, Earth Interact., 23, 1–27, https://doi.org/10.1175/EI-D-17-0033.1, 2019.
Van Bodegom, P. M., Douma, J. C., Witte, J. P. M., Ordoñez, J. C., Bartholomeus, R. P., and Aerts, R.: Going beyond limitations of plant functional types when predicting global ecosystem–atmosphere fluxes: exploring the merits of traits-based approaches, Global Ecol. Biogeogr., 21, 625–636, https://doi.org/10.1111/j.1466-8238.2011.00717.x, 2012.
Wasserstein, R. L. and Lazar, N. A.: The ASA statement on p-values: context, process, and purpose, Am. Stat., 70, 129–133, https://doi.org/10.1080/00031305.2016.1154108, 2016.
Winckler, J., Lejeune, Q., Reick, C. H., and Pongratz, J.: Nonlocal effects dominate the global mean surface temperature response to the biogeophysical effects of deforestation, Geophys. Res. Lett., 46, 745–755, https://doi.org/10.1029/2018GL080211, 2019.
Wright, I. J., Reich, P. B., Cornelissen, J. H., Falster, D. S., Garnier, E., Hikosaka, K., Lamont, B. B., Lee, W., Oleksyn, J., Osada, N., Poorter, H., Villar, R., Warton, D. I., and Westoby, M.: Assessing the generality of global leaf trait relationships, New Phytol., 166, 485–496, https://doi.org/10.1111/j.1469-8137.2005.01349.x, 2005.