the Creative Commons Attribution 4.0 License.
the Creative Commons Attribution 4.0 License.
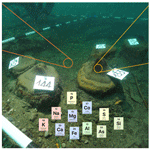
Inorganic component in oak waterlogged archaeological wood and volcanic lake compartments
Giancarlo Sidoti
Federica Antonelli
Giulia Galotta
Maria Cristina Moscatelli
Davor Kržišnik
Vittorio Vinciguerra
Swati Tamantini
Rosita Marabottini
Natalia Macro
Waterlogged archaeological wood (WAW) is a rare and precious organic material that can hold outstanding cultural values. In order to protect WAW for the next generations, this material must be accurately characterised to set its proper conservation, storage and exhibition conditions in museum environments. In this study, the mineral content found in WAW retrieved in a volcanic lake was investigated by analysing wood ash through scanning electron microscopy (SEM) coupled with energy dispersive spectroscopy (SEM-EDS). This micro-destructive approach was coupled with morphological studies carried out through optical microscopy. SEM-EDS was also performed on the WAW and surrounding sediment to study the possible relationship between the mineral composition and the wood degradation state. The analysis revealed that calcium was the most abundant element in all poles, with weight percentages ranging between 24 % and 42 %. This element was more represented in heartwood (HW) than sapwood (SW). In sapwood the second most abundant element was arsenic. Sulfur, iron and potassium were present in all the analysed samples as well. Arsenic was also detected in the sediments; it was particularly concentrated in the samples taken near archaeological wood. The presence of this element can be linked to the volcanic origin of the lake, and its high concentration points to bioaccumulation processes induced by bacteria (erosion bacteria and sulfate-reducing bacteria) and biogeochemical processes favouring precipitation of insoluble compounds. The present work is the first investigation of the mineral content in archaeological wood establishing a contingent relationship with the surrounding volcanic lake sediments.
Waterlogged archaeological wood (WAW) can be defined as wood which was used by an extinct human culture and was then preserved in a water-saturated environment (e.g. lake, sea, rivers, land waterlogged sites) (EN 16873, 2016; Rowell and Barbour, 1989). This precious material can overcome an extended time of exposure in nature and be retrieved in a good preservation state thanks to particular conditions typical of waterlogged sites. The main factor contributing to wood preservation is the low oxygen concentration that slows down the microbial degradation by selecting anaerobic or microaerophilic microorganisms (mainly erosion bacteria and soft rot fungi) (Björdal, 2012; Nilsson and Björdal, 2009). Even if waterlogged wooden objects often preserve their original shape and dimension, the material undergoes chemical and physical modification that alters its features (such as changes in the cellulose/lignin ratio, augmented porosity and reduced density).
According to EN 16873 (2016), the physico-chemical characterisation of WAW is considered essential to set proper conservation protocols and to suggest the most appropriate environmental conditions for long-term storage and/or exhibition (Blanchette et al., 1994; Florian et al., 1990). On the one hand, chemical characterisation of waterlogged wood is usually limited to qualitative and quantitative analysis of the main organic wood components (cellulose, hemicellulose, lignin), which most commonly deteriorate due to selective enzymatic hydrolysis by anaerobic microorganisms (Capretti et al., 2008; Macchioni et al., 2012; Pizzo et al., 2010; Romagnoli et al., 2018). On the other hand, physical analyses focus on properties related to wood mass and volume (e.g. maximum water content, basic density, residual density) (Babiński et al., 2014; High and Penkman, 2020; Jensen and Gregory, 2006; Macchioni et al., 2012; Pizzo et al., 2010).
Chemical and physical analyses are carried out almost routinely, while the content of inorganics inside the wood structure is an aspect often overlooked in the characterisation of WAW. It is in fact well known that archaeological wood contains higher amounts of inorganics with respect to sound wood (Hedges, 1990); nonetheless, the process that leads to this accumulation is not still completely understood. Since microbial diversity inside archaeological wood (Landy et al., 2008) has been sometimes associated with the turnover of sulfur and iron (Björdal and Fors, 2019; Fors et al., 2014, 2012, 2008; Pop Ristova et al., 2017), the presence of some inorganic compounds could be associated with degradation by biotic processes. Sediment composition and elements present in water where the wood was preserved during centuries or millennia greatly influence the content of inorganic compounds in the material (Passialis, 1997). Furthermore, changes in wood porosity and permeability due to degradation processes affect the penetration of minerals (Hedges, 1990). Usually, the concentration of metals increases as the loss of wood substances rises from cores to outwards (Broda and Frankowski, 2017). The accumulation of inorganics inside WAW can occur either by deposition on the surface of the cell walls or inside the lumens (process known as negative casting), or by replacement of the organic component (positive casting), and sometimes it can also occur in short times (Gillard et al., 1994). Accumulation of minerals in wood submerged under water or buried in soil in the absence of oxygen represents the first step of the mineralisation phenomenon that can lead to fossilisation in geological times (hundreds of thousands or millions of years) (Fengel, 1991).
The possible influence of the laying time, and its extent in degradation processes and mineral accumulation in wood remnants, remains currently mostly unquantified; however, it has been supposed that it plays a secondary role in the process (Kolář et al., 2014; Passialis, 1997) compared to the environmental conditions. In spite of the state of art where more aspects need to be clarified, among publications focused on quantifying the inorganic components in archaeological wooden objects, very few analysed the relationships between these elements and the environment (represented by water and sediments) (Broda and Frankowski, 2017), and none, to the authors knowledge, focused on volcanic lakes.
Both sediments and water are important components of lake ecosystems and serve as a sink and source of minerals and metallic ions. However, since these inorganic components can easily deposit and interact with sediments after entering water bodies, the final concentration of both minerals and metallic ions is expected to be higher in the sediments than in the water. However, when the physico-chemical or hydrological conditions change, desorption and resuspension into the water body may occur. The distribution of minerals and metallic ions in water and sediments may in fact vary in relation to several factors either linked to their specific chemical behaviour (i.e. speciation) or to environmental properties. In particular, the accumulation of minerals from overlying water to the sediments may be influenced by the presence of organic and inorganic ligands, sediment type and available surfaces for adsorption, pH, electrical conductivity, redox conditions and temperature (Shalaby et al., 2017). Adsorption may be exerted by organic matter and/or the mineral component of the sediment. Particle size distribution affects heavy metals' enrichment in sediments through physical and chemical processes (Huang et al., 2020). The geology of the basin also strongly influences the content of minerals and metallic ions in lake sediments. Their distribution and relative concentration reflect, in fact, the occurrence and abundance of specific rocks or mineralised deposits in the drainage basin (de Anda et al., 2019). Cation exchange capacity of clays is a key factor in the adsorption of metals in aqueous solutions (Stathi et al., 2010).
Interactions of heavy metals with sediments' organic component or mineral fraction have been widely described in urban lakes (Yang et al., 2010) and rivers (Lin and Chen, 1998). Ferraz and Lourençlo (2000) reported that most of the metals were trapped in the sediment by dissolved organic matter through metal complexation.
Understanding the complex relationships with the surrounding environments, the set-up of possible phenomena of wood mineralisation and, in general, the state and the rate of degradation of the material are key factors for the study of mineral compounds in waterlogged wood. Moreover, metals like iron, copper and lead can interfere with WAW conservation, causing depolymerisation of both wood components and preservation agents (e.g. consolidants like polyethylene glycol – PEG) (Broda and Frankowski, 2017); understanding and quantifying the inorganic components is hence of paramount importance when choosing the more suitable restoration strategy for WAW.
The aim of this paper is to investigate the inorganic components present in archaeological wood found in a volcanic lake, comparing them with the surrounding sediment and water environment, and establish a possible relationship between the inorganic composition and the wood degradation state. To the knowledge of the authors, this is the first time that this type of investigation has been carried out in a volcanic lake environment, and it is aimed to assess the best practice for conservation and understand wood modification process.
2.1 Materials
The wood samples analysed in the present work were obtained from eight oak poles belonging to pile dwellings of the Villanovan village of Gran Carro (about 8th century BC) in Lake Bolsena (Viterbo, Latium, Italy), which has been a long-time object of archaeological studies (Fioravanti, 1994; Severi and Sciancalepore, 2016; Tamburini, 1995).
Cross sections (ca. 5–10 cm in thickness) were cut from the tops of the poles and named after the codes used by archaeologists to identify the poles (125, 126, 133, 144, 146, 152, 163 and 183). They were cut in cubical subsamples, following the orthotropic wood directions, to perform laboratory analyses. Specimens were prepared from both sapwood (SW) and heartwood (HW) of the same disc when present. Poles 126 and 163 represented an exception, having only heartwood and sapwood, respectively. For pole 163, analyses were carried out on two cubes taken from the external and the internal part of the section, named sapwood outward (SW-out) and sapwood inner (SW-in).
2.2 Methods
2.2.1 Wood
The degradation of WAW was measured by parameters well assessed in the technical standard (i.e. maximum water content and ash content), while the organic fraction (cellulose, hemicellulose and lignin) was assessed by thermogravimetry. The results of physical and chemical characterisation are reported in Romagnoli et al. (2018). The inorganic deposits noted inside the wood structure during the micro-morphological characterisation carried out during that study are the object of the present study.
At first, wood cubes were observed under a stereomicroscope (Leica M205C). Then, thin sections (10–20 µm) were obtained for the three anatomical planes (cross, longitudinal radial and longitudinal tangential) by a cryo-microtome (Cryostat CM1900, Leica) and observed under an optical microscope (Zeiss Axio Imager M2) using both bright field and polarised light. A deep insight by scanning electron microscopy (SEM) was carried out on SW and HW samples, showing a massive presence of inorganic material inside the wood pores. Wood sections were attached to aluminium stubs using a carbon tape and sputter coated with gold (coating thickness 8 ± 1 nm) in a Balzers MED 010 unit. Afterwards, the observations were made by a JEOL-JSM 6010LA electron microscope using secondary electron (SE) and backscattered electron (BSE) detectors. Then, to determine the elements, energy dispersive spectroscopy (EDS) was also carried out at 20 kV using a mapping measurement.
Ashes obtained as a residue at 650 ∘C from thermogravimetric analysis (TGA) (for procedure see Romagnoli et al., 2018) were examined without gold or carbon coating by the scanning electron microscope Zeiss EVO 60 equipped with an INCA X-sight dispersive X-ray spectrometer (EDS Oxford Instruments Detector 7636 Energy) for semi-quantitative analysis (SEM-EDS). Samples were analysed in variable pressure (100 Pa), working in backscattered mode. EDS analysis was performed at 20 kV, at 12 mm of working distance and with 100 µs of dwell time, on an area of about 4 mm2. The Inca 5.05 software was used to collect and analyse data, with a cobalt quant optimisation. All the results are expressed as oxide percentage, excluding carbon and oxygen.
To further characterise the mineral components, X-ray diffraction (XRD) analysis was performed by Bruker D8 Advance diffractometer working with a Cu Kα radiation (40 kV and 30 mA) and a 1 mm beam collimator.
Regression analysis was performed between oxides' percentages present in the ashes and maximum water content, as determined in Romagnoli et al. (2018). Furthermore, a comparative observation was carried out looking at the main elements found by sediment and water chemical analysis.
2.2.2 Sediment and water
Sediment samples were collected in March 2022 in the vicinity of the eight poles, so to cover the entire study area (Wsed). In particular, the portion of sediment that surrounded each pole was carefully removed up to 10 cm depth and placed in a plastic vial for transportation. Four sub-replicates were collected from each pole. Additionally, to highlight sediment variations due to the presence of WAW, two areas outside the archaeological site, not impacted by the presence of WAW, were selected as the control, and 10 sub-replicates were sampled in each area (Sed). A total of 52 sediment samples were collected and transferred on ice to the laboratory.
Physical and chemical analyses of sediments included: texture, pH, redox potential (Eh), organic C (TOC) and total N content, and total content of Al, As, Ca, Co, Cr, Cu, Mg, Na, Ni, P, Pb, S, Si, V, Zn, W. Texture analysis was carried out in accordance with the Soil Survey Laboratory Methods Manual (Burt, 2014). Soil pH was measured potentiometrically in a 1 : 2.5 () soil-deionised water suspension with a pH meter (Hanna Instruments) (van Reeuwijk, 2002), while Eh was assessed in a 1 : 5 () soil-deionised water suspension by means of a commercially available combination oxidation–reduction potential (Redox (ORP)) electrode connected to a millivolt meter. (METTLER TOLEDO©, Italia).
Total organic C (TOC) and nitrogen content (TN) were determined by means of an elemental analyser vario MACRO cube Elementar. The total content of metals was assessed by means of ICP-OES (inductively coupled plasma optical emission spectroscopy).
Each sediment sample was heated in an oven at 105 ∘C for 48 h, the dried sediment samples to pass through a 2 mm sieve. For each sample, three replicates of approximately 0.5 g each were placed into 100 mL PFA HP 500 Plus digestion vessel, and 8 mL of ultrapure (68 % ) nitric acid and 2 mL of hydrogen peroxide (30 % ) were added to each vessel and then were loaded into a microwave oven (MARSXpress, CEM Corporation, Matthews, North Carolina). The digestion programme (EPA Method 3052) was executed, and the samples were removed to cool. After cooling, the samples were filtered and diluted to a volume of 50 mL with Milli-Q water. For each batch of sample, reference blanks were prepared.
Mineralised sediment samples were subjected to measurement of the elements by emission spectrometry with ICP-OES (PerkinElmer® OptimaTM 8000 DV) in axial configuration, and each sample was analysed in triplicate.
The elements determined and their corresponding wavelengths (nm) are listed as follows: Fe (259.939), Al (308.215), Pb (220.353), As (193.696), Cu (324.752), Zn (206.200), P (213.617), Ca (317.933), Mg (279.072), Cr (267.716), Co (228.616), V (292.464), Ni (231.604), Na (588.669), S (180.669), Si (SiO2) (251.611), and W (224.876). Calibration curves for each of the elements listed were constructed with one calibration blank and nine calibration standards (standard solution, CaPurAn, CPAchem, Stara Zagora, Bulgaria). To ensure that the instrument was performing consistently and efficiently during the entire analysis, an instrument performance check solution was analysed after every 15 samples and at the end of the analysis. The instrument performance checked solutions with different concentrations of the calibration standard. Statistical analyses were performed using the JMP11.0 (SAS Inst. Inc. Cary, NC, USA) software package. One-way ANOVA and Tukey post hoc test were used to highlight differences between Sed and Wsed properties.
Water samples were collected at poles depth in 500 mL glass bottles at different sampling points covering the whole study area. Minerals' and metals' concentration was determined, by means of ICP-OES, using the same procedure as indicated for sediments. Water chemistry was comparable to the results obtained by Mosello et al. (2004), showing an alkaline pH (8.2) and the highest concentration for Na (57 737 ppb) followed by Mg (14 425 ppb), Ca (10 317 ppb) and S (586 ppb). P content was 9.0 ppb, while, referring to the category of heavy metals, As was 25 ppb.
3.1 Wood
Observations of all samples under a stereomicroscope showed a diffuse presence of inorganic compounds inside wood pores, appearing as a yellowish layer present in most parts of cells (Fig. 1a). In transversal thin sections this substance was opaque when observed in bright field (Fig. 1b), while it was characterised by orange birefringence under polarised light (Fig. 1c). The inorganic material was detected in cell lumina, often forming a layer adhering to the vessels' secondary wall or filling parenchyma cells.
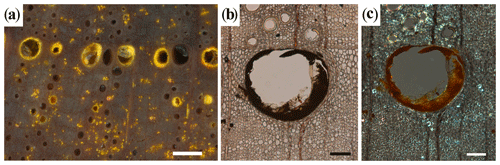
Figure 1Pole 125, microscope view in cross section and elemental analysis of inorganic deposits in wood cells. (a) Stereomicroscope, (b) bright field optical microscope, and (c) polarised light optical microscope. Scale bar: (a) 0.5 mm and (b, c) 100 µm.
Figure 2 shows sapwood BSE and EDS analyses. The yellowish layers present in the fibres, tracheids and radial parenchyma are highlighted in white (Fig. 2a, f) thanks to the BSE image, which means the yellow deposits were mainly composed of elements of high atomic weight. It seems that the internal cell wall of the vessel was covered in calcium, and the yellow deposits were mainly composed of arsenic and sulfur. In particular, the two peaks of S and As trace back to the presence of arsenic sulfide compounds. The correct attribution to a specific mineral was carried out by means of XRD. The analysis allowed the identifying of crystals of realgar (As4S4) (Fig. 3).
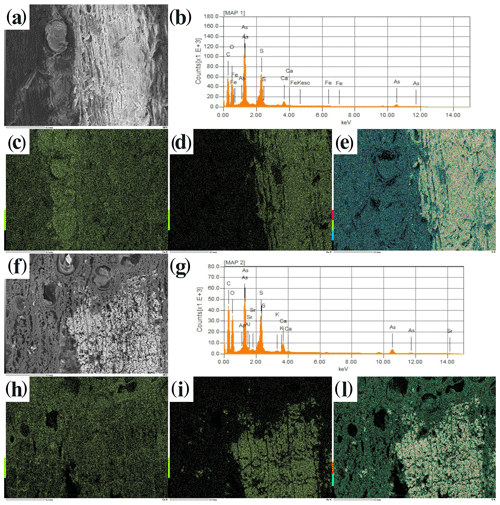
Figure 2BSE (a, f) and EDS maps and spectra (b–e, g–j) of sapwood representative samples. (a–e) Radial section. (f–j) Tangential section. (a, f) The white areas correspond to the yellowish deposit in the sample. (b) EDS spectrum of the section in panel (a). (c) Ca, (d) As, (e) S, and (g) EDS spectrum of the section in panel (f). (h) Ca, (i) As, and (j) S.
SEM-EDS performed on ashes obtained from TGA highlighted the presence of 15 chemical elements. The weight percentage of elements reported as oxides and the ash percentage in the analysed wood samples are reported in the graph of Fig. 4.
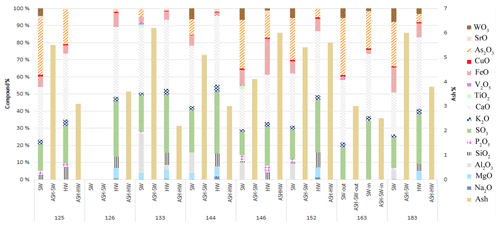
Figure 4SEM–EDS analyses of ashes obtained from TGA. Elements are given as oxides (%). Ashes reported as percent of wood dry weight.
As clearly shown, calcium was the most abundant element in all poles, with weight percentages ranging between 24 % and 42 %. It was more represented in heartwood (HW) than sapwood (SW) (mean values SW 32 %, HW 38 %).
For SW, the second most abundant element was arsenic, present in all poles with a mean weight percentage of 22 %. Its distribution was not homogeneous as shown by the variation coefficient of 47.2 %. In HW, arsenic was even more variable (CV 121.5 %). In particular, this element was absent in pole 133, present in traces (< 1 %) in poles 126 and 144, and exceeded 4 % in poles 152, 183, and 146, reaching 20 % in 125. Sulfur, iron and potassium were also present in all the analysed samples, with mean percentages of 25 %, 7 % and 2.7 %, respectively. It is worth emphasising that heartwood sulfur content was higher than sapwood for all the poles.
Other elements were detected in only a few poles. Aluminium and tungsten were always present, with higher values in sapwood; opposingly, magnesium and silicon were more represented in heartwood. Other elements like sodium, phosphorus, titanium, vanadium, copper and strontium were present with percentages lower than 4 %, without a differential distribution between SW and HW.
To evaluate the effect of the most abundant metals on the wood's state of preservation, relationships between metal percentages and the maximum water content (MWC) were investigated through least square regression (Fig. 5). The analyses showed that no relationship was present between As, S and heartwood MWC, while for Ca a negative correlation was observed (R2 = 0.76).
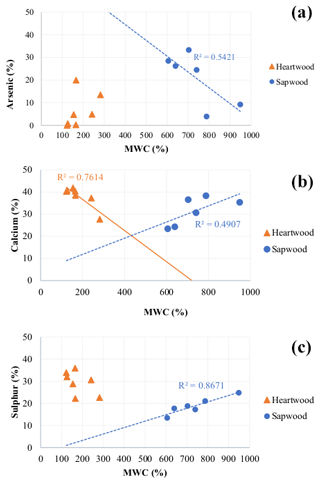
Figure 5Least square regression analyses performed between the percentages of the three most abundant metals and maximum water content (MWC). (a) Arsenic, (b) calcium, and (c) sulfur.
For sapwood, the association between the two variables was around 0.5 for Ca (R2 = 0.49) and As (R2 = 0.54) and 0.86 for S. In the case of Ca and S, the relationship was positive; this means that a greater amount of these elements were present in the most degraded wood. For As the relationship was negative; the highest As values were related to the less deteriorated material.
3.2 Sediments
The physico-chemical properties of Lake Bolsena sediments are reported in Table 1. The sediment texture was sandy, loamy sand; the pH was slightly alkaline in Sed with a low significant acidification in Wsed; total organic carbon ranged from 0.17 % to 21.9 %, while total nitrogen ranged from 0.02 % to 1.16 %; and TOC was significantly higher in Wsed. The redox potential indicated anaerobic reducing conditions and significantly increased in Wsed, showing enhanced availability of O2.
Table 1Main physico-chemical properties of Lake Bolsena sediments. Sed: sediment (n = 20) and Wsed (n = 32): sediment surrounding waterlogged wood. TOC: total organic carbon, TN: total nitrogen and Eh: redox potential. Mean values, standard errors and P values are reported (one-way ANOVA, Tukey post hoc test, ns = not significant).
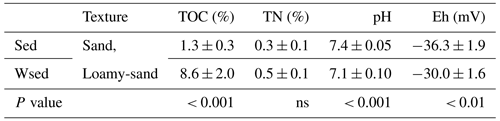
The concentrations of the elements and heavy metals in the sediments of Lake Bolsena are reported in Fig. 6a, b and c. For almost all heavy metals, P, S and Si, the content in Wsed was always significantly higher than in Sed, indicating a specific concentration of these elements in the vicinity of the archaeological wood (Fig. 6b and c). Conversely, for elements such as Fe, Al, Na, Ca and Mg, their abundance was significantly higher, except for Al, in the sediment not in contact with wood (Sed) (Fig. 6a, b).
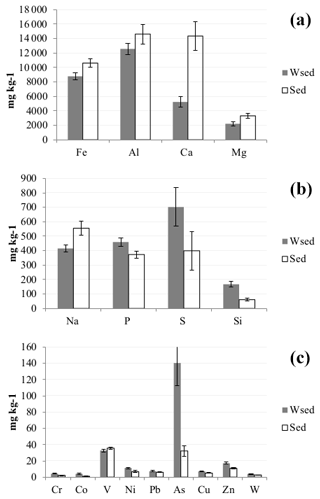
Figure 6Concentration of elements and heavy metals in the sediments of Lake Bolsena. (a) Elements originated from the lithological substrate, (b) elements of mixed origin, and (c) heavy metals (natural and/or anthropogenic sources).
Table 2Percentage variation of sediment elements and metallic ions content due to wood presence. Positive values indicate increase of metal concentration due to wood presence. P values are reported (one-way ANOVA, Tukey post hoc test).

Table 2 summarises the “wood effect” representing the percentage variation of each element/metal concentration in Wsed with respect to Sed. Ca, Fe, Al, Mg, Na and V showed a negative effect of the wood presence, while for all the other heavy metals a significant positive variation has been observed in Wsed. Since the Al, P, Pb, S and V content did not show significant variations between Sed and Wsed, they are not reported in the table.
In the discussion of the results, the interactions between different biological and environmental aspects must be considered. Particularly relevant are the following factors: the concentration of minerals in a standing tree (specifically oak wood), the specific laying conditions (i.e. type of elements and their concentration in sediments) and the level of degradation of waterlogged archaeological wood.
The chemical composition of recent oak wood is characterised by holocellulose (over 40 % on average) and lignin, ranging from 19 % to 27 % in percentage (Kolář et al., 2014; Krutul et al., 2010). Ash content, which represents the inorganic component of the material, in recent wood is in general lower than 1 % (Baar et al., 2020; Fengel and Wegener, 1984; Kolář et al., 2014). WAW degradation impacts the content of wood inorganic compounds. The study by Romagnoli et al. (2018) showed that the heartwood of the poles from the Gran Carro village is quite well preserved, with medium–low values of MWC. However, the corresponding sapwood was always more degraded reaching MWC values over 900 %. In all the analysed poles, the amount of ash was, on average, 4.3 % (maximum 6.2 %). These values exceed 1 %, which is usually found in recent wood, but are below 10 %–13 %, which is the mean value in WAW (Fengel, 1991; Hedges, 1990; Lucejko et al., 2020; Passialis, 1997; Tsoumis, 1969). The increased percentage of ash in degraded waterlogged wood is due to the loss of the organic components (cellulose, hemicellulose and lignin) and the relative enrichment in inorganics that are not removed by biodeteriogens (Hedges, 1990). Furthermore, the biodegradation of the cell wall results in increased porosity (Han et al., 2020; Svedström et al., 2012), favouring accumulation of inorganics in the tissues. In wood remnants of Lake Bolsena, the most degraded sapwood is more susceptible to the accumulations of inorganics, as testified by the percentage of ashes always being higher compared to the corresponding heartwood (Fig. 4); indeed, there are also differences in the composition of inorganic compounds present in both tissues because some of them are more represented in heartwood than in sapwood. In term of ash composition, it is interesting to note that the most abundant elements usually present in recent oak wood were also found in the WAW of Lake Bolsena. In recent oak, calcium is the most abundant, followed by magnesium and potassium. In particular, the latter is a metal fundamental for plant photosynthesis and respiration (Broda and Frankowski, 2017; Schiopu and Tiruta-Barna, 2012). Moreover, Mn, Na, P, Cl, Al, Fe, Zn, Cu, Pb, Co and Si are reported in traces in the ash of the recent wood (Baar et al., 2020; Kolář et al., 2014; Tsoumis, 1991).
Lake Bolsena lies within the Vulsini volcanic district, in which the volcanic activity occurred in alternate phases. The geological evolution of the district is complex, showing the presence of vulcanites (derived from lava and ignimbrite flows) and a clayey flyschioid sedimentary substrate (Mosello et al., 2004). The composition of inorganic compounds in sediments is strictly related to that of lake water and is strongly dependent on the geological substrate nature and weathering processes, atmospheric deposition, biological processes, and physical properties (such as pH, Eh, temperature etc.). Also, for very mobile and particularly reactive elements, a seasonal fluctuation can be observed, and it is due to the temperature changes and occurrence of overturn. The concentration of elements found in Bolsena sediments shows the following ranking: Al > Fe > Ca > Mg > S > P > Si. A large concentration of Ca, Mg and S was also found in the water chemistry at Lake Bolsena by Mosello et al. (2004). Ca was the most abundant inorganic element also in WAW, and usually it was higher in heartwood than in sapwood, with the lowest variability in both the tissues compared to the other elements. Waterlogged wood is degraded by bacteria, which causes a pH decrease, confirmed in this study in Wsed. This may suggest larger mobilisation of Ca from calcium carbonate forms and can be one of the reasons for a lower amount of Ca in sapwood compared to heartwood. Ca is very well represented in standing trees and recent wood as well (Broda and Frankowski, 2017).
Concerning the presence of heavy metals in sediments, the highest concentration was found for As followed by V, Zn, Ni, Pb, Cu, Cr, W and Co. However, except for As, all concentrations of heavy metals did not exceed the PEC (probable effect concentration) as reported in the sediment quality guideline (MacDonald et al., 2000), indicating, therefore, the lack of potential toxicity within this environmental matrix in the lake of Bolsena. Conversely, in the sediment surrounding the waterlogged archaeological wood, As content reached 140 mg kg−1 as an average value (the maximum value was about 500 mg kg−1), thus exceeding the PEC of 33 mg kg−1 (MacDonald et al., 2000). However, As concentration in the lake water was very low (25 µg L−1), with 150 µg L−1 being the threshold of chronic pollution (Ingersoll and Mac Donald, 2002; Osuna-Martínez et al., 2021).
A significant wood effect was therefore found in this study for As, Co, Cr, Cu, Ni, Pb and W, indicating a preferential concentration of these heavy metals in the sediment surrounding the WAW. This effect was also particularly evident for other elements such as P, S and Si.
The reasons for this peculiar behaviour should be found in the different environmental conditions characterising the Wsed. Shaheen et al. (2020) reported decreasing or increasing concentrations of heavy metals in lake sediments in response to a gradient of pH and Eh applied during a 22 d incubation in microcosms. In the present study, in Wsed, the different environmental conditions, such as the increase of Eh values accompanied by a lower pH, could have induced an immobilisation of heavy metals, favouring their speciation and/or ionisation that may have promoted adsorption processes on sediment and consequently into WAW structure.
In particular, this mechanism may explain the specific biogeochemistry of arsenic in this environment. The presence of As in sediments and then in WAW is obviously linked to the volcanic origin of the lake (Samadzadeh Yazdi and Khodadadi Darban, 2010). The high concentration of this element in the sediment points to its immobilisation process through a potential adsorption and/or fixation either on sediment mineral and/or organic fractions or on wood tissues. Arsenic chemistry in anaerobic environments depends on pH, Eh and Fe oxidation state. At the environmental conditions characterising Lake Bolsena sediments, such as neutral–subalkaline pH and Eh ranging from −45 to −10 mV, the dominant As species is H3AsO3 (AsIII, mobile and toxic form). Furthermore, Fe oxides, minerals that can adsorb the oxidised form (AsV) in these conditions, release the metal as AsIII when Fe is turned from FeIII to FeII, therefore further mobilising the metal. The high level of As in wood and wood-surrounding sediments can thus be explained by bioaccumulation processes induced by bacteria and biogeochemical processes favouring precipitation of insoluble compounds. In micro-aerophilic and near-anaerobic conditions, the lignocellulosic structure of wood is actively broken down by erosion bacteria (EB). The simple sugars produced by this process represent a metabolic source for sulfate-reducing bacteria (SRB), a group of anaerobic prokaryotes classified as secondary wood degraders or scavenging bacteria, able to reduce sulfate, SO, to sulfide, S2−, during the metabolisation of simple organic molecules (Fors et al., 2008). The hydrogen sulfide produced by these microorganisms in the presence of Fe and As ions is not stable and tends to transform into iron and arsenic sulfides that precipitate in wood porous structure (Fors et al., 2012; Sandström et al., 2003). The precipitation of As in the form of realgar, one of the insoluble forms of As, and its further accumulation into WAW was confirmed in this study through the XRD analysis (Fig. 3). This could explain the significant negative relationship between As and SRB found in this study (r = −0.36, p < 0.01; data not shown). In a recent review, Sun et al. (2016) showed that SRBs can use arsenic to form insoluble sulfide mineral-like phases in the form of an orpiment-like phase (As2S3), a realgar-like phase (AsS), an arsenopyrite-like phase (FeAsS) (Alam and McPhedran, 2019; Fors et al., 2012; Sandström et al., 2003). As previously hypothesised, the metabolism of SRB is strictly involved in As transformations under anaerobic environments. SRB depend on sulfate availability as a source of energy. In this study, the activity of arylsulfatase, the enzyme involved in the release of sulfate from organic matter, was positively and significantly related to SRB biomass (data not shown).
Fors et al. (2008) proved that, in waterlogged wood, EB and SRB promote the accumulation of sulfur, as thiols in the lignin, and iron sulfides, as particles, and that a positive correlation exists between these elements and bacterial wood degradation. A further study (Björdal and Fors, 2019) demonstrated that an analogous role can be played by soft rot fungi that, degrading cellulose and hemicellulose components of cell walls and creating a substrate valuable for secondary colonisers, actually promote the accumulation of both compounds, particularly in the tannin-rich oak wood.
In the present work, the least square regression analyses performed between S and MWC (Fig. 5c) confirms the relationship between this element and wood biodegradation in sapwood. In fact, the graph clearly shows that the highest S concentrations are reported in the most degraded samples. This same relationship was not observed in heartwood samples (Fig. 5c) that, on the other hand, were always less degraded and had higher S percentages with respect to sapwood. A similar pattern of degradation and S distribution was observed by Björdal and Fors (2019) in oak samples from the XVII century shipwreck called the Crown. The authors hypothesised that the accumulation of S in the deeper, more anoxic and more preserved parts of the wood can take place via chemical reactions, without the presence of microbial degradation. However, this hypothesis has still to be investigated to provide further explanations.
As for arsenic, in sapwood there seems to be a negative relationship between concentration and degradation. The highest values of As are found in the best-preserved samples. This could be explained by assuming an initial phase of wood bacterial degradation which led to the production of hydrogen sulfides and then to the precipitation of As. The presence of the latter subsequently slowed the progression of biological degradation. This effect is not perceptible in heartwood, in which the presence of As is not related to the level of degradation which is much lower than that of sapwood. The natural durability of oak heartwood must have prevailed over the preservative effect of this element.
Regarding the presence of iron in WAW, it must be taken into account that, regardless of bacterial attack, oak wood is particularly susceptible to iron accumulation due to the high concentration of tannins that form stable chemical compounds reacting with iron, so that the natural wood colour turns into dark brownish to almost black (Broda and Frankowski, 2017). This could explain the highest concentration of Fe in heartwood where tannins are mainly present.
The presence of tungsten in wood is probably related to the volcanic origin of the lake. In fact, W in the form of calcium and iron tungstate has been reported in the volcanic area of the Tyrrhenian side of the Italian peninsula (Bellatreccia et al., 1999). As already discussed for As, tungsten is a redox-sensitive element exhibiting contrasting geochemical behaviours under different redox and/or sulfidic conditions (Johannesson et al., 2013; Watanabe et al., 2017). Therefore, it is possible that in the environmental conditions characterising Lake Bolsena this metal may also be subjected to immobilisation processes in the sediment and then in the WAW. Also, silicon is ascribable to the volcanic nature of the lake bed. Its non-uniform distribution in wood samples is probably linked to the random penetration of sand grains inside wood structure (Fors et al., 2008). Furthermore, it must be considered that Si is usually found in the heartwood of recent oak (Kolář et al., 2014).
Total P content in sediments was on average about 400 mg kg−1. Zhang et al. (2021) indicate values of total phosphorus below 450 mg kg−1 as an index of nominal P pollution. Low contents of P were also found in the water lake in accordance with the results reported in Mosello et al. (2004) and in the availability of sediment labile P forms (data not shown), pointing, therefore, to the absence of an eutrophication process. Studying chemical modification of wood induced by bacteria, Gelbrich et al. (2008) proved that in WAW the content of phosphorus increases for higher degrees of bacterial attack. In the present work no significant relationship was observed between state of preservation and P content. In fact, the highest P percentage was recorded for heartwood of pole 146 (4.4 %) that had a low–medium level of degradation (mean MWC 280 %), while this element was absent in the worst-preserved poles (e.g. 144 SW with MWC > 900 %).
The presence of elements found in low concentration in the archaeological wood (e.g. K, Al, Mg, Na, Cu, V) is more difficult to explain, taking into account external influences, as they are usually reported also in recent wood as components of organic macromolecules or involved in cellular metabolic reactions (Baar et al., 2020; Broda and Frankowski, 2017; Tsoumis, 1969). Aluminium, present mainly in sapwood, sometimes has been explained as the presence of aluminium-containing minerals (e.g. clay minerals) in the soil.
The present study represents a first attempt to explore the relationship between the mineral composition of archaeological wood and the surrounding natural environment in a volcanic lake in order to understand wood contamination and inorganic accumulation.
The results showed that Ca, As and S were the most-represented elements in wood, and an uneven distribution was observed between heartwood and sapwood. While Ca is very well represented also in standing trees and recent wood, the presence of As and S in WAW is linked to biogeochemical and microbial bioaccumulation processes. Furthermore, As is strictly related to the volcanic nature of the basin.
The concentration of elements found in sediments showed the following ranking: Al > Fe > Ca > Mg > S > P > Si. Concerning the presence of heavy metals, the highest concentration was found for As followed by V, Zn, Ni, Pb, Cu, Cr, W and Co. Interestingly, for some of these elements (e.g. As, Co, Cr, Cu, Ni, Pb and W) a wood effect was observed: their concentration was significantly higher in the surroundings of waterlogged poles.
The study proved that an integrated methodological approach, including different analytical/diagnostic techniques and the inclusion of additional natural matrices other than wood, helps in better understanding degradation processes of archaeological wood in submerged environments. This may provide a reliable tool that could help in drawing future scenarios on the conservation of waterlogged organic materials.
The data presented in this study are available on request from the corresponding author.
Conceptualisation: GS, GG, MCM, FA, MR. Methodology: GS, MR, GG, MCM, DK. Investigation: GS, RM, DK, ST. Writing – original draft: MR, GG, FA, MCM. Writing – review and editing: MR, GG, FA, MCM, ST, VV, NM. Project administration: MR. Funding acquisition: MR. All authors have read and agreed to the published version of the paper.
The contact author has declared that none of the authors has any competing interests.
The funding source had no involvement in the study
design; in the collection, analysis and interpretation of data; in the
writing of the report; and in the decision to submit the article for
publication.
Publisher's note: Copernicus Publications remains neutral with regard to jurisdictional claims in published maps and institutional affiliations.
The authors would like to thank Barbara Barbaro, archaeologist of the Superintendence of Archaeology, Fine Arts and Landscape for the metropolitan area of Rome the Province of Viterbo and Southern Etruria, together with the technician Egidio Saveri, the staff of the Underwater Archaeology Research Centre (CRAS) of Bolsena and Marco Ciabattoni, and the conservation scientists of ICR, for their support in underwater sampling and monitoring of lake environmental parameters. Moreover, the authors would like to thank Anna Rita Taddei, a technician at the Interdepartmental Centre of Electron Microscopy (CIME), University of Tuscia, for the support during SEM observations.
This research has been supported by JPICH-19 – Italian Ministry of University project “Archaeological Wooden Pile-Dwelling in Mediterranean European lakes: strategies for their exploitation, monitoring and conservation (WOODPDLAKE)”.
This paper was edited by Jack Middelburg and reviewed by two anonymous referees.
Alam, R. and McPhedran, K.: Applications of biological sulfate reduction for remediation of arsenic – A review, Chemosphere, 222, 932–944, https://doi.org/10.1016/j.chemosphere.2019.01.194, 2019.
Baar, J., Paschová, Z., Hofmann, T., Kolár, T., Koch, G., Saake, B., and Rademacher, P.: Natural durability of subfossil oak: Wood chemical composition changes through the ages, Holzforschung, 74, 47–59, https://doi.org/10.1515/hf-2018-0309, 2020.
Babiński, L., Izdebska-Mucha, D., and Waliszewska, B.: Evaluation of the state of preservation of waterlogged archaeological wood based on its physical properties: Basic density vs. wood substance density, J. Archaeol. Sci., 46, 372–383, https://doi.org/10.1016/j.jas.2014.03.038, 2014.
Bellatreccia, F., Caprilli, E., Della Ventura, G., Rossi, P., and Fiori, S.: Scheelite (CaWO4) e ferberite (FeWO4) associate a minerali di Th, U e REE negli inclusi sienitici del Lazio ed ipotesi genetiche, Rend. Lincei, 10, 9–19, https://doi.org/10.1007/bf02904449, 1999.
Björdal, C. G.: Microbial degradation of waterlogged archaeological wood, J. Cult. Herit., 13, S118–S122, https://doi.org/10.1016/J.CULHER.2012.02.003, 2012.
Björdal, C. G. and Fors, Y.: Correlation between sulfur accumulation and microbial wood degradation on shipwreck timbers, Int. Biodeterior. Biodegrad., 140, 37–42, https://doi.org/10.1016/j.ibiod.2019.03.010, 2019.
Blanchette, R. A., Haight, J. E., Koestler, R. J., Hatchfield, P. B., and Arnold, D.: Assessment of Deterioration in Archaeological Wood from Ancient Egypt, J. Am. Inst. Conserv., 33, 55–77, https://doi.org/10.2307/3179670, 1994.
Broda, M. and Frankowski, M.: Determination of the content of selected elements in medieval waterlogged oak wood from the Lednica Lake – a case study, Environ. Sci. Pollut. Res., 24, 23401–23410, https://doi.org/10.1007/s11356-017-9972-7, 2017.
Burt, R. (Ed.): Kellogg soil survey laboratory methods manual, Soil Survey Investigations Report No. 42, Version 6.0, U.S. Department of Agriculture, Natural Resources Conservation Service, 2014.
Capretti, C., Macchioni, N., Pizzo, B., Galotta, G., Giachi, G., and Giampaola, D.: The characterization of waterlogged archaeological wood: The three roman ships found in Naples (Italy), Archaeometry, 50, 855–876, https://doi.org/10.1111/j.1475-4754.2007.00376.x, 2008.
de Anda, J., Gradilla-Hernández, M. S., Díaz-Torres, O., de Jesús Díaz-Torres, J., and de la Torre-Castro, L. M.: Assessment of heavy metals in the surface sediments and sediment-water interface of Lake Cajititlán, Mexico, Environ. Monit. Assess., 191, 1–13, https://doi.org/10.1007/s10661-019-7524-y , 2019.
EN 16873: Conservation of cultural heritage. Guidelines for the management of waterlogged wood on archaeological terrestrial sites, European Committee for Standardization (CEN), Brussels, Belgium, 2016.
Fengel, D.: Aging and fossilization of wood and its components, Wood Sci. Technol., 25, 153–177, https://doi.org/10.1007/BF00223468, 1991.
Fengel, D. and Wegener, G.: Wood: chemistry, ultrastructure, reactions, Walter de Gruyter, Berlin – New York, https://doi.org/10.1002/pol.1985.130231112, 1984.
Ferraz, M. C. M. A. and Lourençlo, J. C. N.: The influence of organic matter content of contaminated soils on the leaching rate of heavy metals, Environ. Prog., 19, 53–58, https://doi.org/10.1002/ep.670190118, 2000.
Fioravanti, A.: Pali e palafitte di ieri e di oggi, Forma lacus Antiq, Second. semi, Atti II Seminario di Geoarcheologia, BollSR, anno IX: 1–46, 1994.
Florian, M.-L. E., Kronkright, D. P., and Norton, R. E.: The Conservation of artifacts made from plant materials, The conservation of artifacts made from plant materials, edited by: Florian, M.-L. E., The J. Paul Getty Trust, https://doi.org/10.1017/CBO9781107415324.004, 1990.
Fors, Y., Nilsson, T., Risberg, E. D., Sandström, M., and Torssander, P.: Sulfur accumulation in pinewood (Pinus sylvestris) induced by bacteria in a simulated seabed environment: Implications for marine archaeological wood and fossil fuels, Int. Biodeter. Biodegr., 62, 336–347, https://doi.org/10.1016/J.IBIOD.2007.11.008, 2008.
Fors, Y., Jalilehvand, F., Damian Risberg, E., Björdal, C., Phillips, E., and Sandström, M.: Sulfur and iron analyses of marine archaeological wood in shipwrecks from the Baltic Sea and Scandinavian waters, J. Archaeol. Sci., 39, 2521–2532, https://doi.org/10.1016/J.JAS.2012.03.006, 2012.
Fors, Y., Grudd, H., Rindby, A., Jalilehvand, F., Sandström, M., Cato, I., and Bornmalm, L.: Sulfur and iron accumulation in three marine-archaeological shipwrecks in the Baltic Sea: The Ghost, the Crown and the Sword, Sci. Rep., 4, 1–6, https://doi.org/10.1038/srep04222, 2014.
Gelbrich, J., Mai, C., and Militz, H.: Chemical changes in wood degraded by bacteria, Int. Biodeter. Biodegr., 61, 24–32, https://doi.org/10.1016/j.ibiod.2007.06.007, 2008.
Gillard, R. D., Hardman, S. M., Thomas, R. G., and Watkinson, D. E.: The mineralization of fibres in burial environments, Stud. Conserv., 39, 132–140, https://doi.org/10.1179/sic.1994.39.2.132, 1994.
Han, L., Tian, X., Keplinger, T., Zhou, H., Li, R., Svedström, K., Burgert, I., Yin, Y., and Guo, J.: Even Visually Intact Cell Walls in Waterlogged Archaeological Wood Are Chemically Deteriorated and Mechanically Fragile: A Case of a 170 Year-Old Shipwreck, Molecules, 25, 1113, https://doi.org/10.3390/molecules25051113, 2020.
Hedges, J. I.: The chemistry of archaeological wood, in: Archaeological Wood. Properties, Chemistry and Preservation, edited by: Rowell, R. M. and Barbour, R. J., Advances in Chemistry Series 225, Washington D.C., American Chemical Society, 111–139, https://doi.org/10.1021/ba-1990-0225, 1990.
High, K. E. and Penkman, K. E. H.: A review of analytical methods for assessing preservation in waterlogged archaeological wood and their application in practice, Herit. Sci., 8, 1–33, https://doi.org/10.1186/s40494-020-00422-y, 2020.
Huang, Z., Liu, C., Zhao, X., Dong, J., and Zheng, B.: Risk assessment of heavy metals in the surface sediment at the drinking water source of the Xiangjiang River in South China, Environ. Sci. Eur., 32, 1–9, https://doi.org/10.1186/s12302-020-00305-w, 2020.
Ingersoll, C. G. and Mac Donald, D. D.: A Guidance Manual to Support the Assessment of Contaminated Sediments in Freshwater Ecosystems. Volume III – Interpretation of the Results of Sediment Quality Investigations, US Environmental Protection Agency, Great Lakes National Program Office, Chicago, EPA-905-B02-001-C, 2002.
Jensen, P. and Gregory, D. J.: Selected physical parameters to characterize the state of preservation of waterlogged archaeological wood: A practical guide for their determination, J. Archaeol. Sci., 33, 551–559, https://doi.org/10.1016/j.jas.2005.09.007, 2006.
Johannesson, K. H., Dave, H. B., Mohajerin, T. J., and Datta, S.: Controls on tungsten concentrations in groundwater flow systems: The role of adsorption, aquifer sediment Fe(III) oxide/oxyhydroxide content, and thiotungstate formation, Chem. Geol., 351, 76–94, https://doi.org/10.1016/j.chemgeo.2013.05.002, 2013.
Kolář, T., Rybníček, M., Střelcová, M., Hedbávný, J., and Vít, J.: The changes in chemical composition and properties of subfossil oak deposited in holocene sediments, Wood Res., 59, 149–166, 2014.
Krutul, D., Radomski, A., Zawadzki, J., Zielenkiewicz, T., and Antczak, A.: Comparison of the chemical composition of the fossil and recent oak wood, Wood Res., 55, 113–120, 2010.
Landy, E. T., Mitchell, J. I., Hotchkiss, S., and Eaton, R. A.: Bacterial diversity associated with archaeological waterlogged wood: Ribosomal RNA clone libraries and denaturing gradient gel electrophoresis (DGGE), Int. Biodeter. Biodegr., 61, 106–116, https://doi.org/10.1016/J.IBIOD.2007.07.007, 2008.
Lin, J. G. and Chen, S. Y.: The relationship between adsorption of heavy metal and organic matter in river sediments, Environ. Int., 24, 345–352, https://doi.org/10.1016/S0160-4120(98)00012-9, 1998.
Lucejko, J. J., Tamburini, D., Zborowska, M., Babiński, L., Modugno, F., and Colombini, M. P.: Oak wood degradation processes induced by the burial environment in the archaeological site of Biskupin (Poland), Herit. Sci., 8, 44, https://doi.org/10.1186/s40494-020-00390-3, 2020.
Macchioni, N., Pizzo, B., Capretti, C., and Giachi, G.: How an integrated diagnostic approach can help in a correct evaluation of the state of preservation of waterlogged archaeological wooden artefacts, J. Archaeol. Sci., 39, 3255–3263, https://doi.org/10.1016/j.jas.2012.05.008, 2012.
MacDonald, D. D., Ingersoll, C. G., and Berger, T. A.: Development and evaluation of consensus-based sediment quality guidelines for freshwater ecosystems, Arch. Environ. Contam. Toxicol., 39, 20–31, https://doi.org/10.1007/s002440010075, 2000.
Mosello, R., Arisci, S., and Bruni, P.: Lake Bolsena (Central Italy): an updating study on its water chemistry, J. Limnol., 63, 1–12, 2004.
Nilsson, T. and Björdal, C.: Understanding Microbial Degradation of Waterlogged Archaeological Wood, in: Wood Science for Conservation of Cultural Heritage, Florence 2007: Proceedings of the International Conference Hld by Cost Action IE0601, 8–10 November 2007, Florence, Italy, Firenze University Press, Florence, 1000–1004, https://doi.org/10.1400/141817, 2009.
Osuna-Martínez, C. C., Armienta, M. A., Bergés-Tiznado, M. E., and Páez-Osuna, F.: Arsenic in waters, soils, sediments, and biota from Mexico: An environmental review, Sci. Total Environ., 752, 142062, https://doi.org/10.1016/j.scitotenv.2020.142062, 2021.
Passialis, C. N.: Physico-chemical characteristics of waterlogged archaeological wood, Holzforschung, 51, 111–113, https://doi.org/10.1515/hfsg.1997.51.2.111, 1997.
Pizzo, B., Giachi, G., and Fiorentino, L.: Evaluation of the applicability of conventional methods for the chemical characterization of waterlogged archaeological wood, Archaeometry, 52, 656–667, https://doi.org/10.1111/j.1475-4754.2009.00490.x, 2010.
Pop Ristova, P., Bienhold, C., Wenzhöfer, F., Rossel, P. E., and Boetius, A.: Temporal and Spatial Variations of Bacterial and Faunal Communities Associated with Deep-Sea Wood Falls, PLoS One, 12, e0169906, https://doi.org/10.1371/journal.pone.0169906, 2017.
Romagnoli, M., Galotta, G., Antonelli, F., Sidoti, G., Humar, M., Kržišnik, D., Čufar, K., and Davidde Petriaggi, B.: Micro-morphological, physical and thermogravimetric analyses of waterlogged archaeological wood from the prehistoric village of Gran Carro (Lake Bolsena-Italy), J. Cult. Herit, 33, 30–38, https://doi.org/10.1016/j.culher.2018.03.012, 2018.
Rowell, R. M. and Barbour, R. J.: Archaeological Wood, Advances in Chemistry, American Chemical Society, https://doi.org/10.1021/ba-1990-0225, 1989.
Samadzadeh Yazdi, M. R. and Khodadadi Darban, A.: Effect of Arsenic Speciation on Remediation of Arsenic-Contaminated Soils and Waters. Heavy metals in sediments and remediation technologies, in: Proceedings of 15th International Conference on Heavy Metals in the Environment ICHMET, 19–23 September 2010, Gdansk, Poland, Department of Analytical Chemistry, Chemical Faculty, Gdansk University of Technology, INIS-PL–2010-0005, p. 1071, 2010.
Sandström, M., Fors, Y., and Persson, I.: The Vasa's new battle: Sulphur, acid and iron, Swedish National Maritime Museums, Stockholm, ISBN 91-85268-94-1, 2003.
Schiopu, N. and Tiruta-Barna, L.: Wood preservatives, in: Toxicity of Building Materials, Elsevier, 138–165, https://doi.org/10.1533/9780857096357.138, 2012.
Severi, E. and Sciancalepore, A.: New computer technologies applied to the underwater archaeology of Lake Bolsena (Lazio – Italy), in: Proceedings of Underwater Archaeology – Interdisciplinary Approaches and Innovative Techologies, in: Poseidon Reich XXI, International congress on underwater archeology, 21–24 April 2016, Dresden, Germany, Deutsche Gesellschaft zur Förderung der Unterwasserarchäologie e.V., http://www.deguwa.org/?id=291 (last access: 17 July 2023), 2016.
Shaheen, S. M., El-Naggar, A., Antoniadis, V., Moghanm, F. S., Zhang, Z., Tsang, D. C. W., Ok, Y. S., and Rinklebe, J.: Release of toxic elements in fishpond sediments under dynamic redox conditions: Assessing the potential environmental risk for a safe management of fisheries systems and degraded waterlogged sediments, J. Environ. Manage., 255, 109778, https://doi.org/10.1016/j.jenvman.2019.109778, 2020.
Shalaby, B. N., Samy, Y. M., Mashaly, A. O., and El Hefnawi, M. A.: Comparative geochemical assessment of heavy metal pollutants among the Mediterranean Deltaic Lakes sediments (Edku, Burullus and Manzala), Egypt, Egypt. J. Chem., 60, 361–377, https://doi.org/10.21608/EJCHEM.2017.916.1047, 2017.
Stathi, P., Papadas, I. T., Tselepidou, A., and Deligiannakis, Y.: Heavy-metal uptake by a high cation-exchange-capacity montmorillonite: the role of permanent charge sites, Glob. NEST J., 12, 248–255, 2010.
Sun, J., Quicksall, A. N., Chillrud, S. N., Mailloux, B. J., and Bostick, B. C.: Arsenic mobilization from sediments in microcosms under sulfate reduction, Chemosphere, 153, 254–261, https://doi.org/10.1016/j.chemosphere.2016.02.117, 2016.
Svedström, K., Bjurhager, I., Kallonen, A., Peura, M., and Serimaa, R.: Structure of oak wood from the Swedish warship Vasa revealed by X-ray scattering and microtomography, Holzforschung, 66, 355–363, https://doi.org/10.1515/hf.2011.157, 2012.
Tamburini, P.: Un abitato villanoviano perilacustre. Il “Gran Carro” sul Lago di Bolsena (1959–1985), Giorgio Bretschneider Editore, Rome, ISBN 8876891145, 1995.
Tsoumis, G.: Wood as raw material: source, structure, chemical composition, growth, degradation and identification, Pergamon Press, Exeter, ISBN 9781483159522, 1969.
Tsoumis, G.: Science and technology of wood: structure, properties, utilization, Van Nostrand Reinhold, New York, ISBN 978-3-941300-22-4, 1991.
van Reeuwijk, L. P.: Procedures for Soil Analysis, Technical Paper No. 9, 6th edn., FAO/ISRIC, Wageningen, the Netherlands, 120 pp., 2002.
Watanabe, Y., Kashiwabara, T., Ishibashi, J. ichiro, Sekizawa, O., Nitta, K., Uruga, T., and Takahashi, Y.: Different partitioning behaviors of molybdenum and tungsten in a sediment–water system under various redox conditions, Chem. Geol., 471, 38–51, https://doi.org/10.1016/j.chemgeo.2017.09.009, 2017.
Yang, X., Xiong, B., and Yang, M.: Relationships among heavy metals and organic matter in sediment cores from lake nanhu, an urban lake in wuhan, China, J. Freshw. Ecol., 25, 243–249, https://doi.org/10.1080/02705060.2010.9665074, 2010.
Zhang, X., Chen, Q., Wang, C., Zhang, H., Zhao, Y., Zhang, L., Liu, B., Wu, Z., and Zhou, Q.: Characteristic analysis of phospholipid fatty acids (PLFAs) in typical nutrient polluted lake sediment in Wuhan, Int. J. Sediment Res., 36, 221–228, https://doi.org/10.1016/j.ijsrc.2020.09.002, 2021.