the Creative Commons Attribution 4.0 License.
the Creative Commons Attribution 4.0 License.
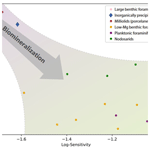
Element ∕ Ca ratios in Nodosariida (Foraminifera) and their potential application for paleoenvironmental reconstructions
Laura Pacho
Lennart de Nooijer
Gert-Jan Reichart
The chemical composition of foraminiferal shells is a well-known tool in paleoceanography to reconstruct past environments and climate. Their application is based on the relation between environmental variables and the concentration of elements incorporated or stable isotope fractionation during calcification. The vast majority of these so-called proxy relationships are based on the foraminiferal order of the Rotaliida, which, for example, encompasses all living planktonic species. However, there are more orders of foraminifera with calcifying members, some of which have fundamentally different biomineralization pathways, such as the Nodosariida, the Polymorphinida and the Vaginulinida. All these belong to the class of the Nodosariata and produce calcite shells, which may serve as carriers of paleoenvironmental and climate signals. The microstructures of these shells and overall morphology of these foraminifera strongly deviate from the Rotaliida, suggesting that their elemental and stable isotopic composition do not necessarily respond similarly to environmental parameters. A potential advantage of the Nodosariata is that they appear considerably earlier in the fossil record (Carboniferous) than the Rotaliida (Jurassic), thereby possibly extending the range of foraminifer-based paleoceanographic reconstructions considerably. To test the potential application of Nodosariata foraminifera as paleoproxies, we investigated incorporation of 5 elements in 11 species as a function of environmental parameters from a transect sampled in the Gulf of Mexico. Their element composition (B Ca, Na Ca, Mg Ca, Sr Ca and Ba Ca) shows a distinct geochemical signature for these foraminifera, different to that of members of other foraminiferal orders. Results also show an increase in Mg Ca values with increasing temperature, similar to that known for the Rotaliida, which suggest that Nodosariata shells might be useful for paleotemperature reconstructions. The difference in Mg Ca–temperature calibration in Nodosariata compared to Rotaliida, with the large differences in their morphology, shell microstructures and overall geochemical composition, suggests that the Mg Ca-to-temperature relationship is partly independent of the exact calcification mechanism. We compare Mg Ca–temperature sensitivities across foraminiferal orders and describe a relationship between the average Mg Ca and the sensitivity of the Mg Ca–temperature calibration. For other elements, the variability across orders is smaller compared to that in Mg Ca, which results in more similar El Ca–environmental calibrations.
- Article
(1154 KB) - Full-text XML
-
Supplement
(638 KB) - BibTeX
- EndNote
Reconstructing past climates is an integral part of predicting the impact of the ongoing rise in atmospheric CO2 levels on the Earth's future climate. The expected temperature increase for a doubling in pCO2 (the so-called climate sensitivity) has, for instance, been estimated by comparing past seawater temperatures and carbon dioxide levels (Rosenthal et al., 2017; D'Arrigo et al., 2006; Mann et al., 1998). Reconstructions of such parameters rely on accurate and precise tools that can be applied to past episodes in Earth's history with conditions similar to those expected in the future. In this context, foraminifera are popular tools as they are proxy signal carriers for constraining past seawater temperature and pH. Field and culturing studies have shown the dependence of the chemical composition of their shells on the seawater chemistry and physics in which they calcified. For example, the amount of incorporated Mg (expressed as the shell's Mg Ca) increases exponentially with temperature (Nürnberg et al., 1996) and can hence be used to reconstruct past changes in bottom water temperature using benthic foraminifera (Lear et al., 2002) and sea surface temperature using planktonic foraminifera (Hastings et al., 1998; Lea et al., 2000).
The incorporation of Mg into the calcite of most foraminifera is, however, much lower compared to calcite precipitated inorganically from seawater (Morse et al., 2007). This offset and the observed differences in Mg Ca values between species (Wit et al., 2012) are hypothesized to be caused by the strong biological control that foraminifera exert on the chemistry of the calcifying fluid from which they form their shells (Erez, 2003; de Nooijer et al., 2014). This biological control and the resulting interspecies variability in calcite chemistry have highlighted the need for species-specific calibrations (Wit et al., 2017; Allen et al., 2016). With this in mind, Mg Ca and other proxies based on foraminiferal shell composition, including Na Ca for salinity (Dämmer et al., 2020; Bertlich et al., 2018; Wit et al., 2013) and δ11B for seawater pH (Foster and Rae, 2016; Rae et al., 2011; Spivack et al., 1993), have been developed and successfully applied. Another complicating factor when applying foraminiferal proxy signals is the dependency of element incorporation and isotope fractionation on more than one environmental parameter. For example, shell Mg Ca values are also affected by the marine inorganic carbon system (Evans et al., 2016), salinity (Raitzsch et al., 2010; Dissard et al., 2010) and the [Mg2+] of the seawater (Evans and Müller, 2012). Ideally, proxy application would therefore include multiple elements to reconstruct a single parameter or, alternatively, use multiple proxy relationships to simultaneously reconstruct multiple environmental parameters.
Our knowledge of the controls on foraminiferal shell geochemistry is almost exclusively based on results obtained from Rotaliida. These foraminifera are characterized by multilocular shells composed of bilamellar calcite (Reiss, 1957, 1963) that can be optically radial or granular. The popularity of using members of this order is partly due to the fact that they encompass all extant planktonic foraminiferal species, while the diversity and overall high abundance of benthic Rotaliida add to their popularity for reconstructions of bottom water conditions. Few studies have investigated element incorporation in the Miliolida, which have a fundamentally different calcification mechanism (ter Kuile et al., 1989; de Nooijer et al., 2009; Debenay et al., 1998). The composition of their calcite is markedly different from that of the Rotaliida, with for example markedly high Mg Ca (Toyofuku et al., 2000; van Dijk et al., 2017b) and more depleted δ25Mg values (Dämmer et al., 2021).
Reconstructions based on Rotaliida could theoretically span the last ∼ 190 Myr since they first occur in the fossil record in the Pliensbachian (Haynes, 1981b). The order of Nodosariata evolved calcification much earlier in the Permian (Haynes, 1981a), and their application would therefore roughly double the age for which paleoceanographic reconstructions could be made using foraminiferal shell chemistry. They separated from the Rotaliida and Miliolida likely before the Cambrian (Pawlowski et al., 2003) and are currently found in many marine habitats and are easily recognizable by their uniserial chamber arrangement (Haynes, 1981a). Their walls are fibrous, composed of conical bundles of one to tens of micrometers in length, that are only found in the Nodosariata and therefore suggest that they have a unique biomineralization mechanism (Dubicka et al., 2018). The isotopic composition of Nodosariida (Reolid, 2014; Dubicka and Wierzbowski, 2021)and the isotopic difference between Rotaliida and Nodosariida have been reported before (Dubicka and Wierzbowski, 2021); variability between species in both δ13C and δ18O can be explained by differences in their microhabitats. For instance, Lenticulina sp. is often found deeper in the sediment, and such species are often δ13C depleted compared to epifaunal foraminifera. Furthermore, Lenticulina can live under dysoxic conditions, which explains the overall lower δ18O in this genus.
So far, the Nodosariata elemental composition has not been studied; therefore, we analyzed element incorporation such as Na Ca, Mg Ca, Sr Ca, B Ca and Ba Ca of different species collected along a depth transect in the Gulf of Mexico. Accompanying environmental data (temperature, salinity, etc.) allow us to detect any dependencies of the incorporation of elements on these parameters and compare them to existing calibrations for Rotaliida foraminiferal species.
2.1 Sampling location
In February 2020, sediment samples were collected from the continental margin in the northern Gulf of Mexico using the research vessel Pelagia (expedition 64PE467). Samples were collected along a transect close to the outflow of the Atchafalaya River, at depths of 105, 272 and 619 m (Fig. 1). From box cores, smaller subcores were collected on deck and subsequently sliced (with a resolution of 0.5 cm for the upper 2 cm and in 1 cm slices down to a depth of 10 cm). The sediment was stored in ethanol, with rose bengal (rB) (2 g L−1) added to stain the cytoplasm of living foraminifera.
From the overlying water of the box cores, vials were filled for analysis of DIC (dissolved inorganic carbon) and TA (total alkalinity) after filtering over 0.4 µm filters. For both analyses, 5 mL vials were filled with seawater and stored at 4 ∘C after addition of 15 µL of HgCl2 to prevent biological alteration of the inorganic carbon system. The samples were analyzed after returning to the laboratory using a QuAAtro continuous flow analyzer. DIC samples were acidified, and the carbon dioxide that was formed was dialyzed over a membrane that reduces the phenolphthalein indicator and was spectrophotometrically recorded at 550 nm (Stoll et al., 2001). For TA, a slightly acid buffered solution of potassium hydrogen phthalate was added to the sample, after which the intensity at 590 nm was recorded (Sarazin et al., 1999). Values obtained for DIC and TA were consistent with the ones obtained from earlier expeditions (Sirois, 2017). Bottom water temperature and salinity were taken from a device that measures conductivity, temperature and depth along the seawater column (CTD) casts at the same station where the sediment samples were taken approximately 4 m above the sea floor. Values for all inorganic carbon system parameters can be estimated using two measured parameters, since any combination of two such parameters will allow calculating all others, including dissolved CO2 (Zeebe et al., 1999), performed with PyCO2SYS (Lewis et al., 1998), using the recently published Python script (Humphreys et al., 2022) (Table 1).
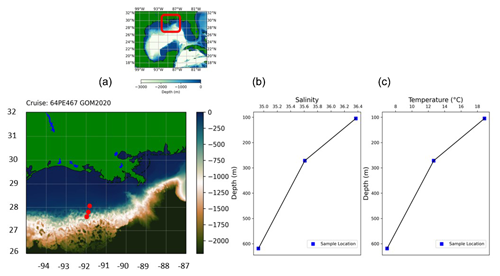
Figure 1(a) Sampling location in the Gulf of Mexico (GEBCO, 2022). (b) Salinity and (c) temperature data from CTD at the bottom sample.
Table 1Chemical and physical seawater parameters at the stations where foraminifera were collected. Temperature, salinity, DIC and TA were measured; the other parameters (pCO2 and all parameters to the right of pCO2) were calculated using the software PyCO2SYS (Lewis et al., 1998), using the recently published Python script (Humphreys et al., 2022).
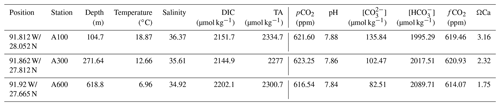
2.2 Sample preparation
Samples were washed using sieves with mesh sizes of 63 and 150 µm and dried in an oven at 60 ∘C. When selecting the specimens, rB-stained foraminifera were separated from non-stained specimens to allow detection of possible postmortem alteration of the primary geochemical signal. The foraminifera were cleaned after isolation from the sediment by immersion in a solution of 1 % H2O2 and 0.1 M NH4OH and three consecutive rinses with double-deionized water. During the latter step, the Eppendorf tubes were placed in an ultrasonic bath to remove any particles adhering to the shells. In this way, 188 individuals were prepared for single-chamber geochemical analysis using laser ablation inductively coupled plasma mass spectrometry (LA-ICP-MS).
2.3 Analytical procedure
Specimens were ablated for 50 s in an NWR193UC TV2 dual-volume chamber using circular spots of 80 µm set at a repetition rate of 6 Hz, using an energy density of 1.00 ± 0.05 J cm−2. The 193 nm wavelength of the LA-ICP-MS used is deep ultraviolet (193 nm), which is excellently suited for ablating carbonates (Reichart et al., 2003) (Table 2). The aerosol produced during the ablation was transported to a quadrupole ICP-MS (Thermo Fisher Scientific iCAP-Q) on a helium flow with a flow rate of 0.6 L min−1, with 0.4 L min−1 argon make-up gas being added before entering the ICP torch. The background signal was measured for 60 s prior to ablation and 10 s after stopping the ablation. Calibration was performed against USGS MACS-3 (synthetic calcium carbonate) pressed powder standard with 43Ca as an internal standard. Scanned masses include 11B, 23Na, 25Mg, 27Al, 43Ca, 88Sr and 138Ba. Standard reference material for quality control was NFHS-2-NP (Boer et al., 2022). Data reduction was performed using an adapted version of the data reduction software SILLS (Signal Integration for Laboratory Laser Systems, Boer et al., 2022; Guillong et al., 2008) in MATLAB. Repeatability based on related standard deviations of measurements of NFHS-2-NP in this study (n=8) is 4 % for Na Ca, 1 % for Mg Ca, 2 % for Sr Ca, 5 % for B Ca, 6 % for Al Ca and 2 % for Ba Ca. For all specimens, two to three spot analyses were performed on the final chamber (Fig. 2). The average element calcium ratio was calculated from the entire profile of the foraminifera chamber wall with a delay of 3 s after firing the laser. The end point of the profile, where the laser penetrates the chamber wall, was calculated in the adapted MATLAB application based on a drop of 30 % of the 43Ca intensity. This end point of the profile was visually checked using laser ablation screen shots continuously made and stored every 2 s.
Since they were not correlated and the occasional high Mg Ca was not accompanied by high Al Ca, the elevated Mg Ca could not be attributed to contamination (e.g., by clay particles or a recrystallized phase at the surface of the shells; Figs. S1, S2, S3, S4), and we did not remove any of the original data points. Instead, we statistically tested for outliers to identify El Ca ratios that are outside the expected distribution given the data. These outliers (n=25) are highlighted in the figures; including or excluding them was found to have an insignificant effect on the reported regressions.
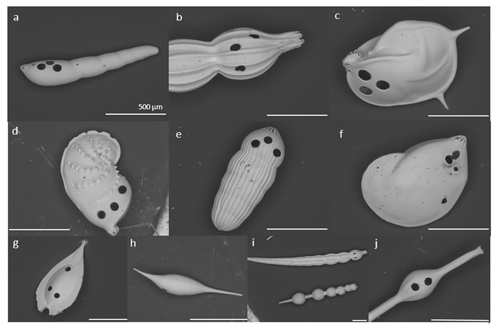
Figure 2Nodosariata species studied. (a) Dentalina spp.; (b) Nodosaria flintii; (c) Lenticulina calcar; (d) Vaginulinopsis baggi; (e) Pseudoglandulina comatula; (f) Lenticulina calcar; (g) Fissurina radiata; (h) Procerolagena sp.; (i) Nodosaria flintii top and Amphicoryna sp. bottom; (j) Grigelis semirugosus. (The SEM images were taken after LA-ICP-MS; holes in the pictures are a consequence of the analyses, and they were placed in the most recent chamber.) All scale bars are 500 µm. The sizes of the ablation craters are all 80 µm (closest picture of the aperture in Fig. S5).
2.4 Statistical analyses
For the three species present at all depths sampled, we performed an ordinary least sum of squares regression analysis to test dependency of the elements incorporated on environmental parameters. For Mg Ca and temperature, an exponential response model was assumed, and for Sr Ca and temperature a linear model was assumed while assuming a linear response model for Na Ca and salinity and for Ba Ca and salinity. For B Ca and the inorganic carbon parameters, a linear response model was assumed. Prior to regression analysis, outliers were identified based on studentized residuals using the package “statsmodels” for Python and applying the method “sidak” from the Holm–Šídák method with a one-step correction (Seabold and Perktold, 2010). Identified outliers are highlighted in the figures: their presence or absence had only a marginal effect on the regression analysis.
When plotting the results, the analytical error was plotted for individual analyses (which mostly falls within the size of the sample marker), the standard deviation for the sample to identify the variability within the sample as well as the standard error (SE), to show the confidence interval for the estimate of the average. Standard deviation (SD) and SE are related according to SE = SD .
Since all data are derived from three locations, in addition to the regression analysis, a two-tailed t test was performed to test whether the variances of the El Ca between locations significantly differed.
3.1 Average El Ca in Nodosariata species
Combining the data from all stations shows that the average El Ca in the individual Nodosariata species varies between 6.65 and 13.2 mmol mol−1 for Na Ca, between 5.94 and 20.1 mmol mol−1 for Mg Ca, and between 1.09 and 1.81 mmol mol−1 for Sr Ca (Table 3) (for more information about variability for two species within the same genus, see Table S2). For all measured Mg Ca of a single species, the SD is on average 3.01 mmol mol−1, where it is 1.07 mmol mol−1 for Na Ca, and 0.14 mmol mol−1 for Sr Ca. This translates to a relative variability in El Ca within a species of 19.6 % for Sr Ca, 17 % for Na Ca and 56 % for Mg Ca.
For the Nodosariata Ba Ca varies considerably, between 2.5 and 4.6 µmol mol−1, with an average SD of 1.2 µmol mol−1, which corresponds to a 71 % variability. The B Ca data vary between 51 and 83 µmol mol−1, with a modest variability in the Nodosariata, with an average SD of 9.8 (or 31.2 %) per species (Table 3).
3.2 Impact of temperature on Mg Ca and salinity on Na Ca
Mg Ca correlates positively with temperature in Nodosaria flintii, Dentalina spp. and Lenticulina calcar over the 12 ∘C range studied here (for more information about the variability between living and non-living species, see Table S1). The lowest and highest values for Mg Ca were found in Dentalina spp., ranging from 3.09 mmol mol−1 at 6.96 ∘C to 29.2 mmol mol−1 at 18.87 ∘C (Fig. 3b and Table 3). For Nodosaria flintii, Mg Ca ranges from 3.93 to 14.9 mmol mol−1 (Fig. 3c and Table 3), and for Lenticulina calcar Mg Ca increases from 6.6 to 18 mmol mol−1 (Fig. 3 and Table 3). Despite the differences in absolute values, the sensitivity of changes in Mg Ca as a function of temperature is similar for the three species, with Dentalina spp. having a slightly higher Mg Ca–temperature sensitivity than the other two species (Table 3).
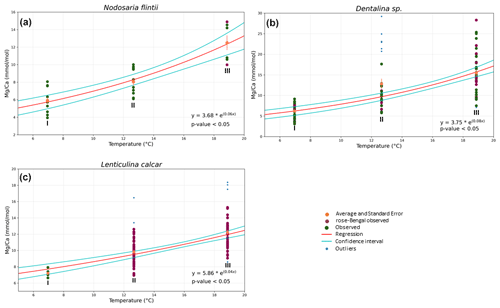
Figure 3Correlations between Mg Ca and temperature for three species of Nodosariata. Dots in blue show the outliers that were omitted before regression analysis based on studentized residuals. Red points are individuals living when sampled (i.e., stained with rose bengal), and green points are non-living individuals when sampled. Red line indicates the result of the exponential ordinary least squares (OLS) regression using both stained and non-stained specimens; cyan lines indicate the 95 % confidence interval of the regression.
Results also show a significant positive increase in Na Ca with salinity for two species, Dentalina spp. and Nodosaria flintii, despite the relatively small range in salinities between sites (1.45 units). For N. flintii, average Na Ca varied between 6.7 and 10 mmol mol−1, which is similar to the increase of 5.6 to 13 mmol mol−1 by Nodosaria flintii (Fig. 4).
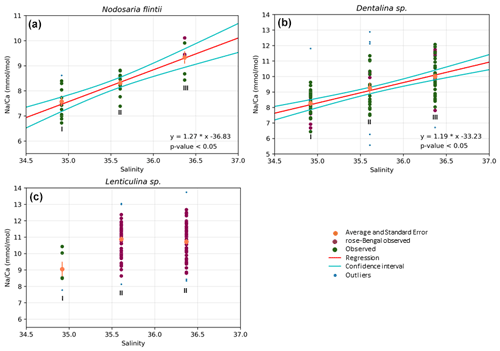
Figure 4Correlations between Na Ca and salinity for three species of Nodosariata. Outliers are shown in blue that were omitted before regression analysis (panels a, b and c) based on studentized residuals. Red points are individuals living when sampled (stained with rose bengal), and data in green are non-stained individuals (panels a, b and c). Red lines in (a) and (b) indicate the result of the linear OLS regression using both the stained and non-stained specimens, and the two cyan lines indicate the 95 % confidence interval of the regression (panels a and b).
For Lenticulina sp., no regression was found between salinity and Na Ca (panel c); “I” and “II” indicate significant differences in the averages between the three groups of data points (i.e., the average Na Ca at lowest salinity is significantly different from the average Na Ca in the other two sampled stations).
4.1 Element incorporation in Nodosariata shells
The average values of Na Ca, Sr Ca and Ba Ca of the Nodosariata are similar to those observed in planktonic (Barker et al., 2005) and many benthic Rotaliida (Lear et al., 2002; Elderfield et al., 2006), whereas Miliolida have considerably lower Na Ca and higher Sr Ca and Ba Ca (van Dijk et al., 2017b). The coral's aragonite Sr Ca has been suggested as a proxy for temperature (Cohen et al., 2001; Reynaud et al., 2007), and this ratio has also been suggested to reflect temperature in the calcite of planktonic foraminifera (Cléroux et al., 2008). Here, we found no correlation between Sr Ca and bottom water temperature (results not shown here). Nor did we find a correlation between Ba Ca and salinity, which has been reported for benthic foraminifera from continental margins with noticeable freshwater input (Bahr et al., 2013). In addition, the Nodosariata's Mg Ca, is higher (5–30 mmol mol−1; Fig. 3) than those in planktonic and many low Mg Ca benthic Rotaliida (Allen et al., 2016; Barker et al., 2005; Lowenstein and Hönisch, 2012). Many larger benthic, tropical Rotaliida and most Miliolida have Mg Ca values an order of magnitude higher (Evans et al., 2018; Toyofuku et al., 2000) compared to those observed for the Nodosariata. The Nodosariata's B Ca values are considerably lower compared to those reported for other foraminiferal species (Rae et al., 2011). A similar low B Ca value was found for the rotaliid Operculina umbonatus (Rae et al., 2011), but this species has much higher Mg Ca compared to the species measured here (Fig. 3). B Ca has been demonstrated to correlate with different parameters of the inorganic carbon system such as DIC, HCO and CO (Yu and Elderfield, 2007; Allen and Hönisch, 2012), but here we found no correlation (results not shown here). Together, our measurements imply that the Nodosariata have a unique El Ca signature that can easily be distinguished from that of the other calcifying foraminiferal orders (Rotaliida, Miliolida, etc.), despite overlap of some ratios with those of some other species.
This order- or class-specific signature of the shell's composition support a fundamental difference in their calcification mechanisms (Dubicka et al., 2018; Dubicka and Gorzelak, 2017). Such a difference is also suggested with a fundamentally distinct morphology (i.e., chamber arrangement), as well as the micrometer-scale structures observed within the chamber walls. The chamber walls of the Nodosariata show a lamellar and fibrous texture, while Rotaliida show a granular texture (Dubicka et al., 2018) (Fig. S6). Such differences in the shell's microstructure coincide with the contrasting Mg Ca values observed here, similar to what was already reported for comparisons between other pairs of foraminiferal orders (van Dijk et al., 2016; Bentov and Erez, 2006). Deep evolutionary branching between Nodosariata and Rotaliida and the large difference in time of first fossil occurrence further support the hypothesis that they evolved their biomineralization mechanism independently. With different seawater chemical conditions (van Dijk et al., 2016; Tanner et al., 2020) at the time when Nodosariata and Rotaliida evolved, calcification biomineralization mechanisms may well reflect contrasting selective pressures, which in turn is reflected by the shells' Mg Ca ratios.
Although they have a long geological and evolutionary history, El Ca variability in the elemental-to-calcium ratio within the Nodosariata is remarkably small compared to that observed in, for example, the Rotaliida. The Mg Ca values do not vary significantly between the different families: the Lagenidae, Nodosariidae, Ellipsolagenidae and the Vaginulinidae (one-way ANOVA, p value > 0.05 and F=1.082). Also, for the other elements no significant difference in the average elemental ratios is observed between species and, thus, between families. This does not exclude the existence of species or families within the Nodosariata that may have a different elemental signature than those reported here as we only investigated three species. Still, the analyzed species span three different orders within the Nodosariata. The relative uniformity in shell carbonate composition across the orders may indicate that the calcification mechanism invented by the Nodosariata is very well suited for a wide range for seawater chemical conditions. Alternatively, the relatively low species diversity of the present-day Nodosariata compared to that during the Jurassic (Haynes, 1981a) may reflect a selective loss of calcification mechanisms due to past changes in ocean chemistry and/or physics, possibly related to past climate variability. Such a hypothesis on the potential interplay between calcification and climate can be tested by comparing the El Ca of extinct species from a suite of eras to that of species living today (Evans et al., 2013; Maeda et al., 2017; Toyofuku et al., 2011; Barrientos et al., 2018).
4.2 Effect of environment in the element incorporation
4.2.1 Na Ca versus salinity
Na Ca correlates with salinity in two of the Nodosariata species investigated: Dentalina spp. and Nodosaria flintii (Fig. 4). Sensitivities of Na Ca to salinity relationships appear somewhat higher than those reported for Rotaliida species (Wit et al., 2013; Geerken et al., 2019; Allen et al., 2016; Mezger et al., 2016; Hauzer et al., 2021) (Table 4). Parallel to the increasing number of reports on the correlation between Na incorporation and salinity, there is discussion of what precisely controls foraminiferal Na Ca, which could be [Ca2+] (Hauzer et al., 2018) as well as the [Na+]sw and/or salinity (Wit et al., 2013). In addition, it may be that Na incorporation is affected by precipitation rates as well, such as indicated by inorganic experiments showing that Na incorporation is affected by saturation state (Devriendt et al., 2021). Although poorly constrained in foraminifera (Geerken et al., 2022), environmental factors may affect the rate at which foraminifera precipitate their calcite, making the relationship between Na Ca and an environmental parameter indirect. Still, the consistent increase in Na Ca with salinity in many Rotaliida foraminifera and the correlations reported here for two Nodosariata species (Fig 4; Table 4) suggest a more direct coupling between seawater [Na+] and [Ca2+] and underscore the robustness of this proxy.
4.2.2 Mg Ca versus temperature
The Mg Ca–temperature relationships found for the Nodosariata species reported here (Fig. 3) are likely also affected by different bottom water [CO] at the sampled stations (Sadekov et al., 2014). The effect of saturation state on Mg incorporation was found to be approximately 40 mmol mol−1 for a difference between the lowest and the highest of ∼ 1000 µmol [CO] kg−1 seawater in culturing experiments (Dissard et al., 2010; Yu et al., 2019; van Dijk et al., 2016). This would amount to a change of approximately 2 mmol mol−1 Mg Ca over the total change at the three locations studied here, assuming that the sensitivity of Mg incorporation as a function of [CO] in the Nodosariata is similar to that in Rotaliida. This would reduce the change in Mg Ca as a function of temperature by less than 10 %, and hence this would only have a very modest impact on the Mg Ca–temperature sensitivities reported here (Fig. 3).
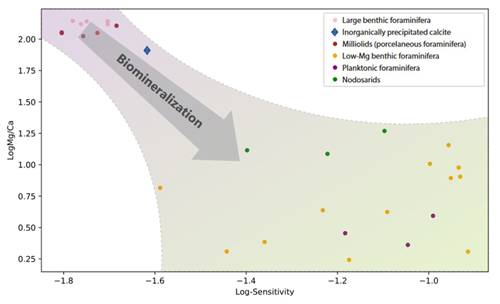
Figure 5Comparison between the sensitivity and the average Mg Ca for different groups of foraminifera. Results for the Nodosariata are from this study; all other Mg Ca–temperature calibrations are from previous studies (Toyofuku et al., 2011; Douglas and Staines-Urias, 2007; Barrientos et al., 2018; Rosenthal et al., 1997; Quillmann et al., 2012; Raitzsch et al., 2008; Lea et al., 1999; Anand et al., 2003; Barker et al., 2005; Rosenthal et al., 2011; Kristjánsdóttir et al., 2007; Lear et al., 2002; Evans et al., 2013; Maeda et al., 2017; Wit et al., 2012; Morse et al., 2007; Toyofuku et al., 2000; de Nooijer et al., 2017; Knorr et al., 2015). Species towards the lower right corner are increasingly affected by biomineralization. These calibrations were used to calculate and plot the Mg Ca at 20 ∘C.
For each of the three species that were found at all three stations, Mg Ca increased exponentially with temperature (Fig. 3) (Rosenthal et al., 1997). The application of these calibrations for Nodosariata for reconstructing past temperature can be challenging since using the chemical composition of fossil shells this far back in time requires careful assessment of the calcite diagenetic overprints (Stainbank et al., 2020), although the case of Lenticulina is reported to have a thick calcite and high fossilization potential (Dubicka and Wierzbowski, 2021). Furthermore, proxies for the chemical composition of the seawater such as fluid inclusion in evaporite minerals indicate that the concentration of major ions such as [Mg2+]sw and [Ca2+]sw changed significantly during the Phanerozoic (Fantle and DePaolo, 2005), and these changes can affect the incorporation of Mg into the calcite. Nevertheless, few studies have developed new proxies to better understand these changes for both [Mg2+]sw (Pogge Von Strandmann et al., 2014; van Dijk et al., 2016) and [Ca2+]sw (Nambiar et al., 2023).
The Mg Ca–temperature sensitivities are slightly lower than those reported for most Rotaliida species. On average, Mg Ca increases by 6 % per degrees Celsius in the Nodosariata species analyzed here (Fig. 3), while in many planktonic species Mg Ca increases by 10 % per degrees Celsius (Barker et al., 2005). Low-Mg Ca benthic rotaliids display an increase of approximately 8 % in Mg Ca per degrees Celsius (Lear et al., 2002; Raitzsch et al., 2010; Russell et al., 2004). High-Mg Ca benthic Rotaliida species, on the other hand, increase by only 2 % (Maeda et al., 2017), which is similar to the slopes of those reported for Miliolida (de Nooijer et al., 2017; Toyofuku et al., 2000).
Combining sensitivities for the different groups of foraminifera and their average Mg Ca and comparing them to those of inorganically precipitated calcites (Morse et al., 2007; Wit et al., 2012) suggest a negative relation between Mg incorporation and sensitivity to temperature (Fig. 5). The relative increase in Mg Ca with temperature is smaller for species incorporating a relatively large amount of Mg in their calcite and vice versa. Highest Mg Ca ratios are found in inorganically precipitated calcites (Morse et al., 2007) in which the increase of Mg Ca is approximately 2 % for a 1 ∘C temperature increase. For species incorporating equally as much Mg (e.g., Operculina ammonoides; Evans et al., 2013), the slope of the Mg Ca–temperature calibration is similar, while for species like Ammonia tepida (incorporating 50–100 times less Mg in their shell), the increase is approximately 7 % per degrees Celsius temperature increase (Fig. 5).
This suggests that the observed high sensitivity of Mg Ca to temperature in the low Mg Ca species actually consists of two factors: an inorganic temperature-dependent fractionation and a biomineralization-related partitioning, which is also temperature dependent. The large difference in Mg Ca between foraminifera (Wit et al., 2012) has been suggested to reflect the efficiency to lower the Mg Ca in the calcifying fluid, either achieved by active Mg2+ removal (Elderfield et al., 1996; Spero et al., 2015) or by selective inward Ca2+ transport (Toyofuku et al., 2017). Foraminiferal species with calcite Mg Ca ratios close to those found in inorganic precipitation experiments may well lack such a mechanism, and the increase in Mg Ca with temperature hence matches that found in inorganic precipitation experiments. The species that are capable of lowering the Mg Ca in the fluid from which they calcify incorporate consistently more Mg at increased temperatures (Fig. 5). This suggests that foraminiferal Mg Ca–temperature relationships are determined by two components (Dämmer et al., 2021). The first component is the biological control on Mg partitioning, and the second component is the thermodynamic effect of temperature on Mg Ca. In foraminiferal species where the first component is absent (i.e., when they precipitate from a seawater like fluid), the second component determines the Mg Ca–temperature sensitivity. For species that lower the Mg2+ in the fluid from which they precipitate their calcite, the biological component dominates the Mg Ca–temperature calibration. The relatively large variability in the low-Mg Ca species may be explained by small environmental factors (e.g., salinity or water depth) or by processes that are part of the calcification mechanism (e.g., Rayleigh fractionation, organic templates) that may vary slightly between species. The Nodosariata studied here have similar Mg Ca but differ in their sensitivities (Fig. 5), which may well reflect the environmental and/ or calcification-related differences between species. (Elderfield et al., 1996; Branson et al., 2018).
The chemical composition of the shells of various Nodosariata species collected in the Gulf of Mexico was found to be clearly different from those of other foraminiferal orders. Their Mg Ca was between 6 and 20 mmol mol−1, and their Na Ca was relatively high compared to ratios for most planktonic Rotaliida species. Sr Ca and B Ca were comparable to those found in other foraminiferal species. In two of the species studies, the Na Ca increased linearly with salinity. Between families of the Nodosariata analyzed, the El Ca was relatively similar. The Nodosariata's Mg Ca is correlated to temperature and could thus serve as a seawater temperature proxy. Compared to Rotaliida and Miliolida orders, our analysis shows a relation between the Mg Ca of the species and its sensitivity to changes in temperature. When more Mg is incorporated, it is less sensitive to changes in temperature and vice versa. This suggests the interaction between two components that together determine the Mg Ca: the capacity of a species to control the Mg Ca of the calcite and the thermodynamic effect of temperature on Mg incorporation.
All data used in this paper are available at https://doi.org/10.25850/nioz/7b.b.lf (Pacho, 2023).
The supplement related to this article is available online at: https://doi.org/10.5194/bg-20-4043-2023-supplement.
Conceptualization: GJR and LdN; generated geochemical proxy analyses: LP; interpretation of the data: LP with the support of the co-authors; writing: LP with the support of the co-authors. GJR secured funding for the project.
The contact author has declared that none of the authors has any competing interests.
Publisher's note: Copernicus Publications remains neutral with regard to jurisdictional claims in published maps and institutional affiliations.
We are grateful to the captain and crew on board RV Pelagia expedition 64PE467 to the Gulf of Mexico, to Frans Jorissen for providing help with the identification of foraminifera, to Wim Boer for the LA-ICP-MS analyses, and to Karel Baker for the DIC and TA analyses.
This research has been supported by the Netherlands Earth System Science Centre (NESSC) (funding no. 024.002.001) and the Ministry of Education, Culture and Science (OCW).
This paper was edited by Chiara Borrelli and reviewed by Ellen Thomas and Reinhard Kozdon.
Allen, K. A. and Hönisch, B.: The planktic foraminiferal B Ca proxy for seawater carbonate chemistry: A critical evaluation, Earth Planet. Sc. Lett., 345–348, 203–211, https://doi.org/10.1016/j.epsl.2012.06.012, 2012.
Allen, K. A., Hönisch, B., Eggins, S. M., Haynes, L. L., Rosenthal, Y., and Yu, J.: Trace element proxies for surface ocean conditions: A synthesis of culture calibrations with planktic foraminifera, Geochim. Cosmochim. Ac., 193, 197–221, https://doi.org/10.1016/j.gca.2016.08.015, 2016.
Anand, P., Elderfield, H., and Conte, M. H.: Calibration of Mg Ca thermometry in planktonic foraminifera from a sediment trap time series, Paleoceanography, 18, 1050, https://doi.org/10.1029/2002pa000846, 2003.
Bahr, A., Schönfeld, J., Hoffmann, J., Voigt, S., Aurahs, R., Kucera, M., Flögel, S., Jentzen, A., and Gerdes, A.: Comparison of Ba Ca and δ18OWATER as freshwater proxies: A multi-species core-top study on planktonic foraminifera from the vicinity of the Orinoco River mouth, Earth Planet. Sc. Lett., 383, 45–57, https://doi.org/10.1016/j.epsl.2013.09.036, 2013.
Barker, S., Cacho, I., Benway, H., and Tachikawa, K.: Planktonic foraminiferal Mg Ca as a proxy for past oceanic temperatures: A methodological overview and data compilation for the Last Glacial Maximum, Quaternary Sci. Rev., 24, 821–834, https://doi.org/10.1016/j.quascirev.2004.07.016, 2005.
Barrientos, N., Lear, C. H., Jakobsson, M., Stranne, C., O'Regan, M., Cronin, T. M., Gukov, A. Y., and Coxall, H. K.: Arctic Ocean benthic foraminifera Mg Ca ratios and global Mg Ca-temperature calibrations: New constraints at low temperatures, Geochim. Cosmochim. Ac., 236, 240–259, https://doi.org/10.1016/j.gca.2018.02.036, 2018.
Bentov, S. and Erez, J.: Impact of biomineralization processes on the Mg content of foraminiferal shells: A biological perspective, Geochem. Geophy. Geosy., 7, Q01P08, https://doi.org/10.1029/2005GC001015, 2006.
Bertlich, J., Nürnberg, D., Hathorne, E. C., de Nooijer, L. J., Mezger, E. M., Kienast, M., Nordhausen, S., Reichart, G. J., Schönfeld, J., and Bijma, J.: Salinity control on Na incorporation into calcite tests of the planktonic foraminifera Trilobatus sacculifer – Evidence from culture experiments and surface sediments, Biogeosciences, 15, 5991–6018, https://doi.org/10.5194/bg-15-5991-2018, 2018.
Boer, W., Nordstad, S., Weber, M., Mertz-Kraus, R., Hönisch, B., Bijma, J., Raitzsch, M., Wilhelms-Dick, D., Foster, G. L., Goring-Harford, H., Nürnberg, D., Hauff, F., Kuhnert, H., Lugli, F., Spero, H., Rosner, M., van Gaever, P., de Nooijer, L. J., and Reichart, G.-J.: New Calcium Carbonate Nano-particulate Pressed Powder Pellet (NFHS-2-NP) for LA-ICP-OES, LA-(MC)-ICP-MS and μXRF, Geostand. Geoanal. Res., 46, 411–432, https://doi.org/10.1111/ggr.12425, 2022.
Branson, O., Redfern, S. A. T., Elmore, A. C., Read, E., Valencia, S., and Elderfield, H.: The distribution and coordination of trace elements in Krithe ostracods and their implications for paleothermometry, Geochim. Cosmochim. Ac., 236, 230–239, https://doi.org/10.1016/j.gca.2017.12.005, 2018.
Cléroux, C., Cortijo, E., Anand, P., Labeyrie, L., Bassinot, F., Caillon, N., and Duplessy, J. C.: Mg Ca and Sr Ca ratios in planktonic foraminifera: Proxies for upper water column temperature reconstruction, Paleoceanography, 23, 1–16, https://doi.org/10.1029/2007PA001505, 2008.
Cohen, A. L., Layne, G. D., Hart, S. R., and Lobel, P. S.: Implications for the paleotemperature proxy a, Paleoceanography, 16, 20–26, 2001.
D'Arrigo, R., Wilson, R., and Jacoby, G.: On the long-term context for late twentieth century warming, J. Geophys. Res.-Atmos., 111, 1–12, https://doi.org/10.1029/2005JD006352, 2006.
Dämmer, L. K., de Nooijer, L., van Sebille, E., Haak, J. G., and Reichart, G. J.: Evaluation of oxygen isotopes and trace elements in planktonic foraminifera from the Mediterranean Sea as recorders of seawater oxygen isotopes and salinity, Clim. Past, 16, 2401–2414, https://doi.org/10.5194/cp-16-2401-2020, 2020.
Dämmer, L. K., van Dijk, I., de Nooijer, L., van der Wagt, B., Wilckens, F. K., Zoetemelk, B., and Reichart, G. J.: Temperature Impact on Magnesium Isotope Fractionation in Cultured Foraminifera, Front. Earth Sci., 9, 1–13, https://doi.org/10.3389/feart.2021.642256, 2021.
Debenay, J.-P., Guillou, J.-J., Geslin, E., Lesourd, M., and Redois, F.: De plaquettes rhomboedriques/k la surface d'un test porcelane de foraminifere actuel, Geobios, 31, 295302, https://doi.org/10.1016/S0016-6995(98)80013-2, 1998.
de Nooijer, L. J., Toyofuku, T., and Kitazato, H.: Foraminifera promote calcification by elevating their intracellular pH, P. Natl. Acad. Sci. USA, 106, 15374–15378, https://doi.org/10.1073/pnas.0904306106, 2009.
de Nooijer, L. J. De, Spero, H. J., Erez, J., Bijma, J., and Reichart, G. J.: Earth-Science Reviews Biomineralization in perforate foraminifera, Earth Sci. Rev., 135, 48–58, https://doi.org/10.1016/j.earscirev.2014.03.013, 2014.
de Nooijer, L. J., van Dijk, I., Toyofuku, T., and Reichart, G. J.: The Impacts of Seawater Mg Ca and Temperature on Element Incorporation in Benthic Foraminiferal Calcite, Geochem. Geophy. Geosy., 18, 3617–3630, https://doi.org/10.1002/2017GC007183, 2017.
Devriendt, L. S., Mezger, E. M., Olsen, E. K., Watkins, J. M., Kaczmarek, K., Nehrke, G., Nooijer, L. J. De, and Reichart, G.: ScienceDirect Sodium incorporation into inorganic CaCO3 and implications for biogenic carbonates, Geochim. Cosmochim. Ac., 314, 294–312, https://doi.org/10.1016/j.gca.2021.07.024, 2021.
Dissard, D., Nehrke, G., Reichart, G. J., and Bijma, J.: The impact of salinity on the Mg Ca and Sr Ca ratio in the benthic foraminifera Ammonia tepida: Results from culture experiments, Geochim. Cosmochim. Ac., 74, 928–940, https://doi.org/10.1016/j.gca.2009.10.040, 2010.
Douglas, R. and Staines-Urias, F.: Dimorphism, shell Mg Ca ratios and stable isotope content in species of bolivina (Benthic Foraminifera) in the Gulf of California, Mexico, J. Foraminifer. Res., 37, 189–203, https://doi.org/10.2113/gsjfr.37.3.189, 2007.
Dubicka, Z. and Gorzelak, P.: Unlocking the biomineralization style and affinity of Paleozoic fusulinid foraminifera, Sci. Rep., 7, 1–6, https://doi.org/10.1038/s41598-017-15666-1, 2017.
Dubicka, Z. and Wierzbowski, H.: Can oxygen and carbon isotope ratios of Jurassic foraminifera be used in palaeoenvironmental reconstructions?, Palaeogeogr. Pelaeocl., 577, 110554, ú https://doi.org/10.1016/j.palaeo.2021.110554, 2021.
Dubicka, Z., Owocki, K., and Gloc, M.: Micro- and nanostructures of calcareous foraminiferal tests: Insight from representatives of Miliolida, Rotaliida and Lagenida, J. Foraminifer. Res., 48, 142–155, https://doi.org/10.2113/gsjfr.48.2.142, 2018.
Elderfield, H., Bertram, C. J., and Erez, J.: A biomineralization model for the incorporation of trace elements into foraminiferal calcium carbonate, Earth Planet. Sc. Lett., 142, 409–423, https://doi.org/10.1016/0012-821x(96)00105-7, 1996.
Elderfield, H., Yu, J., Anand, P., Kiefer, T., and Nyland, B.: Calibrations for benthic foraminiferal Mg Ca paleothermometry and the carbonate ion hypothesis, Earth Planet. Sc. Lett., 250, 633–649, https://doi.org/10.1016/j.epsl.2006.07.041, 2006.
Erez, J.: The Source of Ions for Biomineralization in Foraminifera and Their Implications for Paleoceanographic Proxies, Rev. Mineral. Geochem. Search Dropdown Menu, 54, 115–149, 2003.
Evans, D. and Müller, W.: Deep time foraminifera Mg Ca paleothermometry: Nonlinear correction for secular change in seawater Mg Ca, Paleoceanography, 27, 1–11, https://doi.org/10.1029/2012PA002315, 2012.
Evans, D., Müller, W., Oron, S., and Renema, W.: Eocene seasonality and seawater alkaline earth reconstruction using shallow-dwelling large benthic foraminifera, Earth Planet. Sc. Lett., 381, 104–115, https://doi.org/10.1016/j.epsl.2013.08.035, 2013.
Evans, D., Wade, B. S., Henehan, M., Erez, J., and Müller, W.: Revisiting carbonate chemistry controls on planktic foraminifera Mg Ca: Implications for sea surface temperature and hydrology shifts over the Paleocene-Eocene Thermal Maximum and Eocene-Oligocene transition, Clim. Past, 12, 819–835, https://doi.org/10.5194/cp-12-819-2016, 2016.
Evans, D., Müller, W., and Erez, J.: Assessing foraminifera biomineralisation models through trace element data of cultures under variable seawater chemistry, Geochim. Cosmochim. Ac., 236, 198–217, https://doi.org/10.1016/j.gca.2018.02.048, 2018.
Fantle, M. S. and DePaolo, D. J.: Variations in the marine Ca cycle over the past 20 million years, Earth Planet. Sc. Lett., 237, 102–117, https://doi.org/10.1016/j.epsl.2005.06.024, 2005.
Foster, G. L. and Rae, J. W. B.: Reconstructing Ocean pH with Boron Isotopes in Foraminifera, Annu. Rev. Earth Pl. Sci., 44, 207–237, https://doi.org/10.1146/annurev-earth-060115-012226, 2016.
GEBCO_2022 Grid: A continuous terrain model of the global oceans and land,NERC EDS British Oceanographic Data Centre NOC, https://doi.org/10.5285/e0f0bb80-ab44-2739-e053-6c86abc0289c, 2022.
Geerken, E., de Nooijer, L. J., Roepert, A., Polerecky, L., King, H. E., and Reichart, G. J.: Element banding and organic linings within chamber walls of two benthic foraminifera, Sci. Rep., 9, 3598, https://doi.org/10.1038/s41598-019-40298-y, 2019.
Geerken, E., de Nooijer, L., Toyofuku, T., Roepert, A., Middelburg, J. J., Kienhuis, M. V. M., Nagai, Y., Polerecky, L., and Reichart, G. J.: High precipitation rates characterize biomineralization in the benthic foraminifer Ammonia beccarii, Geochim. Cosmochim. Ac., 318, 70–82, https://doi.org/10.1016/j.gca.2021.11.026, 2022.
Guillong, M., Latkoczy, C., Seo, J. H., Güntherb, D., and Heinricha, C. A.: Determination of sulfur in fluid inclusions by laser ablation ICP-MS, J. Anal. At. Spectrom., 23, 1581–1589, https://doi.org/10.1039/B807383J, 2008.
Hastings, D. W., Russell, A. D., and Emerson, S. R.: Foraminiferal magnesium in globeriginoides sacculifer as a paleotemperature proxy, Paleoceanography, 13, 161–169, https://doi.org/10.1029/97PA03147, 1998.
Hauzer, H., Evans, D., Müller, W., Rosenthal, Y., and Erez, J.: Calibration of Na partitioning in the calcitic foraminifer Operculina ammonoides under variable Ca concentration: Toward reconstructing past seawater composition, Earth Planet. Sc. Lett., 497, 80–91, https://doi.org/10.1016/j.epsl.2018.06.004, 2018.
Hauzer, H., Evans, D., Müller, W., Rosenthal, Y., and Erez, J.: Salinity Effect on Trace Element Incorporation in Cultured Shells of the Large Benthic Foraminifer Operculina ammonoides, Paleoceanogr. Paleocl., 36, e2021PA004218, https://doi.org/10.1029/2021PA004218, 2021.
Haynes, J. R.: The Nodosariida BT – Foraminifera, edited by: Haynes, J. R., Palgrave Macmillan UK, London, 180–203, https://doi.org/10.1007/978-1-349-05397-1_9, 1981a.
Haynes, J. R.: The Rotaliida (Smaller) BT – Foraminifera, edited by: Haynes, J. R., Palgrave Macmillan UK, London, 236–273, https://doi.org/10.1007/978-1-349-05397-1_12, 1981b.
Humphreys, M. P., Lewis, E. R., Sharp, J. D., and Pierrot, D.: PyCO2SYS v1.8: Marine carbonate system calculations in Python, Geosci. Model Dev., 15, 15–43, https://doi.org/10.5194/gmd-15-15-2022, 2022.
Knorr, P. O., Robbins, L. L., Harries, P. J., Hallock, P., and Wynn, J.: Response of the miliolid Archaias angulatus to simulated ocean acidification, J. Foraminifer. Res., 45, 109–127, https://doi.org/10.2113/gsjfr.45.2.109, 2015.
Kristjánsdóttir, G. B., Lea, D. W., Jennings, A. E., Pak, D. K., and Belanger, C.: New spatial Mg Ca-temperature calibrations for three Arctic, benthic foraminifera and reconstruction of north Iceland shelf temperature for the past 4000 years, Geochem. Geophy. Geosy., 8, Q03P21, https://doi.org/10.1029/2006GC001425, 2007.
Lea, D. W., Mashiotta, T. A., and Spero, H. J.: Controls on magnesium and strontium uptake in planktonic foraminifera determined by live culturing, Geochim. Cosmochim. Ac., 63, 2369–2379, https://doi.org/10.1016/S0016-7037(99)00197-0, 1999.
Lea, D. W., Pak, D. K., and Spero, H. J.: Climate impact of late quaternary equatorial Pacific sea surface temperature variations, Science, 289, 1719–1724, https://doi.org/10.1126/science.289.5485.1719, 2000.
Lear, C. H., Rosenthal, Y., and Slowey, N.: Benthic foraminiferal Mg Ca-paleothermometry: A revised core-top calibration, Geochim. Cosmochim. Ac., 66, 3375–3387, https://doi.org/10.1016/S0016-7037(02)00941-9, 2002.
Lewis, E., Wallace, D., and Allison, L. J.: Program developed for CO2 system calculations. United States, doi10.2172/639712, 1998.
Lowenstein, T. K. and Hönisch, B.: The Use of Mg Ca as a Seawater Temperature Proxy, Paleontol. Soc. Pap., 18, 85–100, https://doi.org/10.1017/S1089332600002564, 2012.
Maeda, A., Fujita, K., Horikawa, K., Suzuki, A., Yoshimura, T., Tamenori, Y., and Kawahata, H.: Evaluation of oxygen isotope and Mg Ca ratios in high-magnesium calcite from benthic foraminifera as a proxy for water temperature, J. Geophys. Res.-Biogeo., 122, 185–199, https://doi.org/10.1002/2016JG003587, 2017.
Mann, M. E., Bradley, R. S., and Hughes, M. K.: Global-scale temperature patterns and climate forcing over the past six centuries, Nature, 392, 779–787, https://doi.org/10.1038/33859, 1998.
Mezger, E. M., de Nooijer, L. J., Boer, W., Brummer, G. J. A., and Reichart, G. J.: Salinity controls on Na incorporation in Red Sea planktonic foraminifera, Paleoceanography, 31, 1562–1582, https://doi.org/10.1002/2016PA003052, 2016.
Morse, J. W., Arvidson, R. S., and Lüttge, A.: Calcium carbonate formation and dissolution, Chem. Rev., 107, 342–381, https://doi.org/10.1021/cr050358j, 2007.
Nambiar, R., Hauzer, H., Gray, W. R., Henehan, M. J., Cotton, L., Erez, J., Rosenthal, Y., Renema, W., Müller, W., and Evans, D.: Controls on potassium incorporation in foraminifera and other marine calcifying organisms, Geochim. Cosmochim. Ac., 351, 125–138, https://doi.org/10.1016/j.gca.2023.04.020, 2023.
Nürnberg, D., Bijma, J., and Hemleben, C.: Assessing the reliability of magnesium in foraminiferal calcite as a proxy for water mass temperatures, Geochim. Cosmochim. Ac., 60, 803–814, https://doi.org/10.1016/0016-7037(95)00446-7, 1996.
Pacho, L.: Nodosarids_dataset2023, NIOZ [data set], https://doi.org/10.25850/nioz/7b.b.lf, 2023.
Pawlowski, J., Holzmann, M., Berney, C., Fahrni, J., Gooday, A. J., Cedhagen, T., Habura, A., and Bowser, S. S.: The evolution of early Foraminifera, P. Natl. Acad. Sci. USA, 100, 11494–11498, https://doi.org/10.1073/pnas.2035132100, 2003.
Pogge Von Strandmann, P. A. E., Forshaw, J., and Schmidt, D. N.: Modern and Cenozoic records of seawater magnesium from foraminiferal Mg isotopes, Biogeosciences, 11, 5155–5168, https://doi.org/10.5194/bg-11-5155-2014, 2014.
Quillmann, U., Marchitto, T. M., Jennings, A. E., Andrews, J. T., and Friestad, B. F.: Cooling and freshening at 8.2 ka on the NW Iceland Shelf recorded in paired δ18O and Mg Ca measurements of the benthic foraminifer Cibicides lobatulus, Quaternary Res., 78, 528–539, https://doi.org/10.1016/j.yqres.2012.08.003, 2012.
Rae, J. W. B., Foster, G. L., Schmidt, D. N., and Elliott, T.: Boron isotopes and B Ca in benthic foraminifera: Proxies for the deep ocean carbonate system, Earth Planet. Sc. Lett., 302, 403–413, https://doi.org/10.1016/j.epsl.2010.12.034, 2011.
Raitzsch, M., Kuhnert, H., Groeneveld, J., and Bickert, T.: Benthic foraminifer Mg Ca anomalies in South Atlantic core top sediments and their implications for paleothermometry, Geochem. Geophy. Geosy., 9, Q05010, https://doi.org/10.1029/2007GC001788, 2008.
Raitzsch, M., Duenas-Bohórquez, A., Reichart, G. J., De Nooijer, L. J., and Bickert, T. T.: Incorporation of Mg and Sr in calcite of cultured benthic foraminifera: Impact of calcium concentration and associated calcite saturation state, Biogeosciences, 7, 869–881, https://doi.org/10.5194/bg-7-869-2010, 2010.
Reichart, G. J., Jorissen, F., Anschutz, P., and Mason, P. R. D.:: Single foraminiferal test chemistry records the marine environment, Geology, 31, 355–358, 2003.
Reiss, Z.: Occurrence of Nezzazata in Israel, Micropaleontology, 3, 259–262, 1957.
Reiss, Z.: Comments on Wall Structure of Foraminifera, Micropaleontology, 9, 50–52, https://doi.org/10.2307/1484605, 1963.
Reolid, M.: Stable isotopes on foraminifera and ostracods for interpreting incidence of the Toarcian Oceanic Anoxic Event in Westernmost Tethys: Role of water stagnation and productivity, Palaeogeogr. Palaeocl., 395, 77–91, https://doi.org/10.1016/j.palaeo.2013.12.012, 2014.
Reynaud, S., Ferrier-Pagès, C., Meibom, A., Mostefaoui, S., Mortlock, R., Fairbanks, R., and Allemand, D.: Light and temperature effects on Sr Ca and Mg Ca ratios in the scleractinian coral Acropora sp., Geochim. Cosmochim. Ac., 71, 354–362, https://doi.org/10.1016/j.gca.2006.09.009, 2007.
Rosenthal, Y., Boyle, E. A., and Slowey, N.: Temperature control on the incorporation of magnesium, strontium, fluorine, and cadmium into benthic foraminiferal shells from Little Bahama Bank: Prospects for thermocline paleoceanography, Geochim. Cosmochim. Ac., 61, 3633–3643, https://doi.org/10.1016/S0016-7037(97)00181-6, 1997.
Rosenthal, Y., Morley, A., Barras, C., Katz, M. E., Jorissen, F., Reichart, G. J., Oppo, D. W., and Linsley, B. K.: Temperature calibration of Mg Ca ratios in the intermediate water benthic foraminifer Hyalinea balthica, Geochem. Geophy. Geosy., 12, Q04003, https://doi.org/10.1029/2010GC003333, 2011.
Rosenthal, Y., Kalansky, J., Morley, A., and Linsley, B.: A paleo-perspective on ocean heat content: Lessons from the Holocene and Common Era, Quaternary Sci. Rev., 155, 1–12, https://doi.org/10.1016/j.quascirev.2016.10.017, 2017.
Russell, A. D., Hönisch, B., Spero, H. J., and Lea, D. W.: Effects of seawater carbonate ion concentration and temperature on shell U, Mg, and Sr in cultured planktonic foraminifera, Geochim. Cosmochim. Ac., 68, 4347–4361, https://doi.org/10.1016/j.gca.2004.03.013, 2004.
Sadekov, A. Y., Bush, F., Kerr, J., Ganeshram, R., and Elderfield, H.: Mg Ca composition of benthic foraminifera Miliolacea as a new tool of paleoceanography, Paleoceanography, 29, 990–1001, https://doi.org/10.1002/2014PA002654, 2014.
Sarazin, G., Michard, G., and Prevot, F.: A rapid and accurate spectroscopic method for alkalinity measurements in sea water samples, Water Res., 33, 290–294, https://doi.org/10.1016/S0043-1354(98)00168-7, 1999.
Seabold, S. and Perktold, J.: Statsmodels: Econometric and Statistical Modeling with Python, Proc. 9th Python Sci. Conf., 92–96, https://doi.org/10.25080/majora-92bf1922-011, 2010.
Sirois, S.: RB 17-04 GOMECC-3: Gulf of Mexico Ecosystems and Carbon Cycle Cruise, https://repository.library.noaa.gov/view/noaa/16758 (last access: 2020), 2017.
Spero, H. J., Eggins, S. M., Russell, A. D., Vetter, L., Kilburn, M. R., and Hönisch, B.: Timing and mechanism for intratest Mg Ca variability in a living planktic foraminifer, Earth Planet. Sc. Lett., 409, 32–42, https://doi.org/10.1016/j.epsl.2014.10.030, 2015.
Spivack, A. J., You, C.-F., and Smith, H. J.: Foraminiferal boron isotope ratios as a proxy for surface ocean pH over the past 21 Myr, Nature, 363, 149–151, https://doi.org/10.1038/363149a0, 1993.
Stainbank, S., Spezzaferri, S., De Boever, E., Bouvier, A. S., Chilcott, C., de Leau, E. S., Foubert, A., Kunkelova, T., Pichevin, L., Raddatz, J., Rüggeberg, A., Wright, J. D., Yu, S. M., Zhang, M., and Kroon, D.: Assessing the impact of diagenesis on foraminiferal geochemistry from a low latitude, shallow-water drift deposit, Earth Planet. Sc. Lett., 545, 116390, https://doi.org/10.1016/j.epsl.2020.116390, 2020.
Stoll, M. H. C., Bakker, K., Nobbe, G. H., and Haese, R. R.: Continuous-flow analysis of dissolved inorganic carbon content in seawater, Anal. Chem., 73, 4111–4116, https://doi.org/10.1021/ac010303r, 2001.
Tanner, T., Hernández-Almeida, I., Drury, A. J., Guitián, J., and Stoll, H.: Decreasing Atmospheric CO2 During the Late Miocene Cooling, Paleoceanogr. Paleocl., 35, e2020PA003925, https://doi.org/10.1029/2020PA003925, 2020.
ter Kuile, B., Erez, J., and Padan, E.: Mechanisms for the uptake of inorganic carbon by two species of symbiont-bearing foraminifera, Mar. Biol., 103, 241–251, https://doi.org/10.1007/BF00543354, 1989.
Toyofuku, T., Kitazato, H., Kawahata, H., Tsuchiya, M., and Nohara, M.: Evaluation of Mg / Ca thermometry in foraminifera: Comparison of experimental results and measurements in nature, Paleoceanography, 15, 456–464, https://doi.org/10.1029/1999PA000460, 2000.
Toyofuku, T., Suzuki, M., Suga, H., Sakai, S., Suzuki, A., Ishikawa, T., de Nooijer, L. J., Schiebel, R., Kawahata, H., and Kitazato, H.: Mg Ca and δ18O in the brackish shallow-water benthic foraminifer Ammonia “beccarii”, Mar. Micropaleontol., 78, 113–120, https://doi.org/10.1016/j.marmicro.2010.11.003, 2011.
Toyofuku, T., Matsuo, M. Y., De Nooijer, L. J., Nagai, Y., Kawada, S., Fujita, K., Reichart, G. J., Nomaki, H., Tsuchiya, M., Sakaguchi, H., and Kitazato, H.: Proton pumping accompanies calcification in foraminifera, Nat. Commun., 8, 1–6, https://doi.org/10.1038/ncomms14145, 2017.
van Dijk, I., de Nooijer, L. J., Hart, M. B., and Reichart, G.-J.: The long-term impact of magnesium in seawater on foraminiferal mineralogy: Mechanism and consequences, Global Biogeochem. Cy., 30, 438–446, https://doi.org/10.1002/2015GB005241, 2016.
van Dijk, I., de Nooijer, L. J., Wolthers, M., and Reichart, G. J.: Impacts of pH and [CO] on the incorporation of Zn in foraminiferal calcite, Geochim. Cosmochim. Ac., 197, 263–277, https://doi.org/10.1016/j.gca.2016.10.031, 2017a.
van Dijk, I., Nooijer De, L. J., and Reichart, G. J.: Trends in element incorporation in hyaline and porcelaneous foraminifera as a function of pCO2, Biogeosciences, 14, 497–510, https://doi.org/10.5194/bg-14-497-2017, 2017b.
Wit, J. C., de Nooijer, L. J., Barras, C., Jorissen, F. J., and Reichart, G. J.: A reappraisal of the vital effect in cultured benthic foraminifer Bulimina marginata on Mg/Ca values: assessing temperature uncertainty relationships, Biogeosciences, 9, 3693–3704, https://doi.org/10.5194/bg-9-3693-2012, 2012.
Wit, J. C., De Nooijer, L. J., Wolthers, M., and Reichart, G. J.: A novel salinity proxy based on na incorporation into foraminiferal calcite, Biogeosciences, 10, 6375–6387, https://doi.org/10.5194/bg-10-6375-2013, 2013.
Wit, J. C., de Nooijer, L. J., Haig, J., Jorissen, F. J., Thomas, E., and Reichart, G. J.: Towards reconstructing ancient seawater Mg Ca by combining porcelaneous and hyaline foraminiferal Mg Ca-temperature calibrations, Geochim. Cosmochim. Ac., 211, 341–354, https://doi.org/10.1016/j.gca.2017.05.036, 2017.
Yu, J. and Elderfield, H.: Benthic foraminiferal B Ca ratios reflect deep water carbonate saturation state, Earth Planet. Sc. Lett., 258, 73–86, https://doi.org/10.1016/j.epsl.2007.03.025, 2007.
Yu, Z., Lei, Y., Li, T., Zhang, S., and Xiong, Z.: Mg and Sr uptake in benthic foraminifera Ammonia aomoriensis based on culture and field studies, Palaeogeogr. Palaeocl., 520, 229–239, https://doi.org/10.1016/j.palaeo.2019.02.001, 2019.
Zeebe, R. E., Bijma, J., and Wolf-Gladrow, D. A.: A diffusion-reaction model of carbon isotope fractionation in foraminifera, Mar. Chem., 64, 199–227, https://doi.org/10.1016/S0304-4203(98)00075-9, 1999.