the Creative Commons Attribution 4.0 License.
the Creative Commons Attribution 4.0 License.
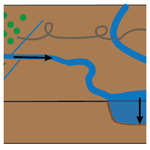
Soil-biodegradable plastic films do not decompose in a lake sediment over 9 months of incubation
Sigrid van Grinsven
Carsten Schubert
Agriculture relies heavily on the use of plastic mulch films, which increase crop yields and can lower water demands. In recent years, soil-biodegradable mulch films have been marketed to replace the non-biodegradable, conventional polyethylene-based mulch films. These biodegradable mulch films are designed to be ploughed into the soil after use to be biodegraded in situ by soil microorganisms. However, research has shown that part of the mulch film material may be transported from the fields to neighboring environments, including aquatic ecosystems. Research on potential biodegradation of soil-biodegradable plastics in freshwater habitats is lacking. Here, we investigated the mineralization of soil-biodegradable agricultural mulch films in freshwater lake sediments of Lake Lucerne, Switzerland. Two types of commercial soil-biodegradable mulch films were incubated within lake sediment cores, along with traditional polyethylene (PE) plastic, and the production of CO2 and CH4 was followed over time relative to non-plastic-containing control sediments. After the 40-week incubation period, the films were visually intact and showed no signs of mineralization. Gas analyses showed no additional production of either CO2 or CH4 in the degradable mulch film incubations, compared to the control or PE plastic incubations. We conclude that these two used soil-biodegradable mulch films have a low biodegradability in lake sediments, likely reflecting that the microbial community structure in the lake sediment lacks active microbial degraders. Our results highlight the importance of preventing transport of soil-biodegradable mulch films from agricultural soils to surrounding aquatic environments.
- Article
(917 KB) - Full-text XML
-
Supplement
(1139 KB) - BibTeX
- EndNote
Part of the solution to overcoming plastic pollution in the environment is to use biodegradable plastic instead of conventional plastics in applications in which plastics are used in the open environment and for which complete recollection of the plastic after use is impossible. A picture-case example is thin agricultural mulch films. Modern agriculture relies heavily on the use of plastic mulch films to elevate soil temperature and maintain soil moisture, to provide protection, and to prevent weed growth. Conventional mulch films are composed of polyethylene (PE). If these films are thin, they cannot readily be reused or recycled after application, which means they need to be incinerated or landfilled after a single use (Serrano-Ruiz et al., 2021). Such a short life cycle of plastic products is undesirable, and both incineration and landfilling are considered undesirable end-of-life treatment options. Another disadvantage of conventional thin (< 25 µm) mulch films is that are cannot easily be entirely recollected after their application, leaving PE plastic residues in the soil. Because PE films persist, these residues build up in soils over time, eventually harming agricultural productivity (Gao et al., 2019). Furthermore, the films may slowly decompose into microplastics. Both macroplastics and microplastics have been shown to be transported by wind and water, away from the initial application site and towards waterways, lakes, and coastal seas (Fig. 1; Egessa et al., 2020; Ren et al., 2021; Yang et al., 2022), where they have been shown to cause harm to the ecosystem (Galloway et al., 2017; Hale et al., 2020).
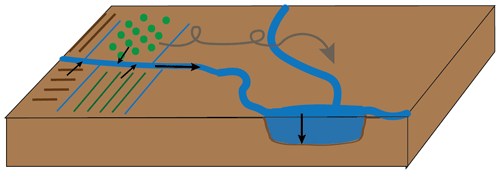
Figure 1Transport of plastics from agricultural sources to lake sediments, either via water ways or via wind transport.
In an attempt to limit soil plastic pollution, the agricultural industry has developed soil-biodegradable mulch films as substitutes for conventional films. These soil-biodegradable films can be composed of different materials, for example, biologically derived polymers, forms of polysaccharides, polypeptides, and also lipid-based products, and are designed to be degradable by soil microorganisms under the moisture and temperature conditions found in the soil environment (Yang et al., 2020). A standard has been set by the EU (2018 EU standard EN 17033) for the biodegradability of mulch films named as such. In general, the biodegradation of the soil-biodegradable mulch films (hereafter referred to as BD films) involves two stages: (i) breakdown of the large pieces into small organic molecules by abiotic or biological (enzymatic) processes and (ii) uptake and metabolic use of these organic molecules by microorganisms under conversion into CO2 and microbial biomass (Zumstein et al., 2018).
Soil-biodegradable mulch films are designed to be biodegraded in soil. It is, however, possible that fragments of the of BD films may be transported away from agricultural soils before being completely biodegraded. While we are not aware of studies specifically on the transport of BD films, the transport of conventional mulch films by wind or water has been observed (Dris et al., 2015; Ren et al., 2021; Yang et al., 2022).
Earlier studies have shown successful biodegradation of BD films in soil environments (Li et al., 2014; Sintim et al., 2020), with complete biodegradation being predicted to take several years. Research on the aquatic biodegradation of BD plastics is predominantly focused on the marine realm. There, several studies have shown microbial colonization on BD plastics (Eich et al., 2015; Morohoshi et al., 2018; Nauendorf et al., 2016), and some showed a disintegration of the plastics over time (Briassoulis et al., 2020; Lott et al., 2020; Wei et al., 2021), although these studies were not performed on mulch films but on other BD plastic products, such as bags. The transferability of studies on different BD plastics is limited, but as there are currently no studies on BD mulch films in the aquatic environment available, we still introduce these studies on other BD plastic products here. All of the above presented studies are disintegration studies, which generally cannot distinguish whether BD plastics have merely physically degraded into smaller particles (i.e., fragmentation) or whether they have been microbially biodegraded, resulting in microbial biomass and CO2 and/or CH4 production, which makes an assessment of the mineralization of the BD plastics difficult. A recent study by Lott et al. (2021) measured marine biodegradation of BD plastic components (PHB, PBSe) by CO2 measurements in experiments with marine sediments. There, they found half-lives of 116 and 222 d in incubation experiments under benthic and eulittoral marine conditions.
Here, we determine the biodegradability of BD films in freshwater lake sediments by performing sediment incubation studies with two types of soil-biodegradable, and one traditional PE plastic, mulch films (MFs). Our results show that none of the tested films biodegraded over the 40-week incubation period: we detected no excess production of CO2 or methane in the incubation experiments with BD plastics as compared to PE films and plastic-free control sediments. Furthermore, there were no signs of any physical degradation of any of the films after the 40-week incubation. Our findings suggest that the conditions in the tested sediment, likely including abiotic factors (unfavorable temperature and water chemistry) and biotic factors (low abundance and activity of BD-MF degrading microorganisms), impaired biodegradation in the lake. Our findings suggest significant higher stability of soil-biodegradable mulch films in lake sediments than soils, highlighting the need for measures to control off-field transport of soil BD films.
2.1 Sediment collection
Sediment cores were taken from Lake Lucerne (Switzerland) at 46∘59′38′′ N, 8∘20′57′′ E in June 2021, at an approximate water depth of 40 m. Multiple deployments with a multicorer device were used to collect the required number of sediment cores, which were later randomly distributed over the treatments. Sediments were collected into 10 cm diameter transparent butyrate plastic core liners. All sediment cores were brought into a climate room of 10 ∘C (to mimic the temperature of Lake Lucerne bottom waters; see Fiskal et al., 2019) within 2 h of core collection.
2.2 Gas analysis incubation experiment
To determine the production of CO2 and CH4 from the sediments, 16 cores were set up for incubation studies in the 10 ∘C climate room. An overview of the experimental setup is presented in Fig. 2. Four cores did not receive any treatment (film-free control group), four cores were supplied with PE films, and eight cores were supplied with biodegradable mulch films purchased from two Swiss agricultural vendors: four cores received BD mulch films purchased from Sansonnens FG Frères SA (Rueyres-les-Prés, Switzerland), called MF-S from here on, and four had biodegradable plastics purchased from gvz-rossat AG (Otelfingen, Switzerland), called MF-R from here on. These plastics are classified as “OK biodegradable soil” by TÜV Austria and are composed mainly of poly(butylene adipate-co-terephthalate) (PBAT) and polylactic acid (PLA) (Nelson et al., 2020). The plastics (all kinds) were cut and weighed. Each core received 300 mg of plastics, folded multiple times to form a rectangular stack. To increase the surface area for degradation and to make the stacks of plastic film loose, rather than squeezed together, the plastics were cut into comb-shaped pieces (see Fig. S1 in the Supplement for plastic weights and shape), which allowed for sediment between the folded layers. Part of the water was removed from each core to allow for an air headspace above the overlying water. The oxygen concentration in the headspace was not tracked, but results from earlier experiments with the same sediments showed that oxygen was not completely consumed over 1 year's time (Sigrid van Grinsven, unpublished data).
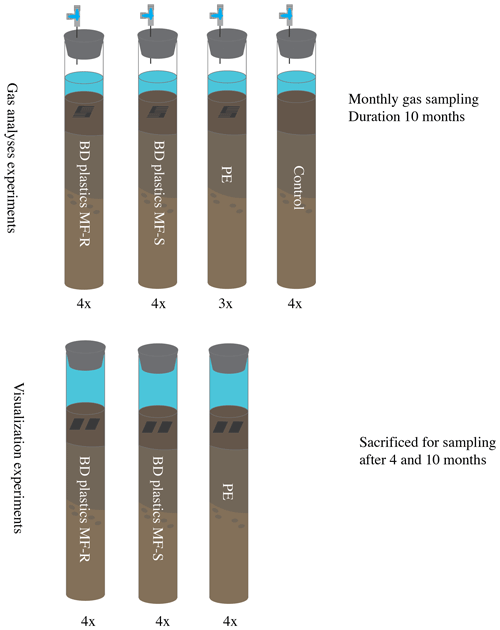
Figure 2Experimental setup. The gas analysis experiments contained a headspace to allow for gas sampling via a sampling port. The visualization experiments had no gas headspace; the entire space above the sediment was filled with in situ bottom water. No control experiment was included in the visualization experiments, as these experiments were solely used for microscopy of the retrieved plastics (see methods). All experiments were performed at in situ (Lake Lucerne) temperature, in the dark. BD – biodegradable; PE – polyethylene; MF-R and MF-S – biodegradable mulch films of two Swiss companies (see methods).
For a part of the cores, the stopper at the bottom was pushed upwards slightly (5–15 cm) to decrease the water and headspace volumes above the sediment surface, to create more equal headspace volumes in each core. This type of cores was distributed over the treatments (two per treatment). The procedure was not repeated for all used cores because not all stoppers turned out to be tight enough to be pushed up and remain in position. All cores, pushed up or not, had a 7 cm height gas headspace (±550 cm3). Water headspace volumes varied between cores. One of the PE cores leaked water over the course of the experiment and was therefore discarded from the results. Films (biodegradable/PE) were pushed into the sediment surface (1–2 cm). Each of the cores was closed off with an adapted stopper that contained two sampling ports, as specified in van Grinsven et al. (2023). These stoppers allowed for non-invasive gas sampling over the course of the experiment.
The cores were incubated in the dark at 10 ∘C for 40 weeks. Gas samples (10 mL) were taken monthly. The removed gas was resupplied with N2 gas to prevent under-pressure in the cores. The volume of gas exchanged on a monthly basis was less than 2 % of the gas headspace. Gas samples were pushed into 60 mL serum bottles containing N2 gas, which were analyzed for CO2, CH4, and N2O concentrations. Gas samples were analyzed by gas chromatography (GC; Agilent 6890N, Agilent Technologies) using a Carboxen-1010 Column (30 m × 0.53 mm; Supelco), a flame ionization detector, and an auto-sampler (Valco Instruments Co. Inc.)
The change in headspace CO2 and CH4 concentration over time was taken as a measure of biodegradation. The headspace CO2 and CH4 concentrations of all cores of each treatment were averaged. The data of each individual core are shown in the Supplement (Figs. S2 and S3).
2.3 Visualization incubation experiment
Identical cores were used for the gas analysis and visualization experiments. A total of 12 cores were used for the visualization experiment: four cores received PE plastics, four cores MF-S BD plastics, and four cores MF-R BD plastics, as shown in Fig. 2. No control cores were included, as these experiments were purely used for visualization of the extracted plastics, and control experiments would therefore not produce any results, as nothing could have been visualized. As a smaller quantity of plastics could be added to the visualization experiments, the plastics did not need to be folded, and therefore they were not cut either (Fig. S1). Two unfolded pieces of plastics were added to each core. No cores were pushed up, and no headspace was created; the cores were filled with water up to the stopper to cause minimal disturbance and to keep the conditions close to in situ conditions. The bottom water contained around 400 µM of oxygen at the start of the experiment. Earlier experiments showed limited oxygen consumption over the course of 1 year (Sigrid van Grinsven, unpublished data). Non-adapted stoppers were used, as these cores were not subsampled over the course of the experiment. Incubation occurred in parallel to the gas analysis experiment, at 10 ∘C in the dark.
After 18 weeks, two cores per treatment were sampled and sacrificed. The plastics were removed from the sediments with tweezers, pulled through the overlying water, and put into a 4 % paraformaldehyde solution overnight at 4 ∘C to fixate the samples. The samples were then rinsed twice with PBS (phosphate-buffered saline) 1X to remove paraformaldehyde and then stored at −20 ∘C until further usage. This was repeated after 40 weeks for the remaining sediment cores.
(Biodegradable) plastic samples were used for microscopy after all samples were retrieved at the end of the experiments. Samples were stained with DAPI 1 mg mL−1 and placed on microscope slides with a mounting and anti-fading medium. The samples were viewed under a Leica fluorescence microscope with 100 × and 400 × magnification to look for biofilm growth and visible degradation of the plastics.
Concentrations of CO2 and methane in the gas headspace of the sediment cores were followed over the course of the experiment to assess plastic biodegradation. Given the organic composition of each of the plastic types, being composed of organic polymers, CO2 and possibly CH4 were expected to be key biodegradation products, alongside microbial biomass.
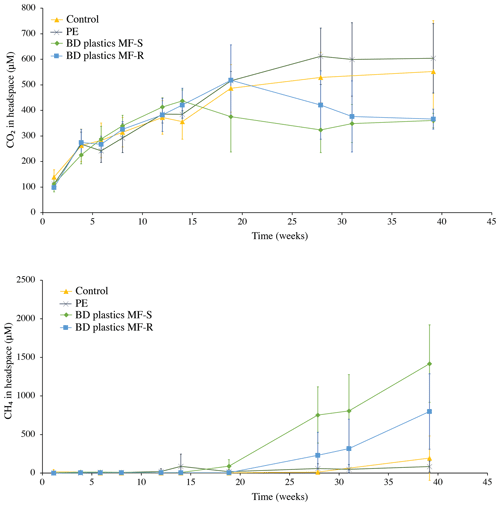
Figure 3Headspace CO2 and methane concentrations, in µM. An excerpt of the CH4 graph, with a smaller y axis, is available in Fig. S4.
Overall, the change in CO2 concentration over time did not differ significantly between any of the treatments, including the control (pairwise ANOVA with post hoc analyses and a Bonferroni adjustment revealed that all the pairwise differences between groups were not statistically significantly different; p > 0.05). During the first 20 weeks, the four treatments behave similarly, with a steady increase in headspace CO2 concentration, with the exception of MF-S, which started to differ from 15 weeks onwards (Fig. 3). From 20 weeks (15 for MF-S) to the termination at 40 weeks, the concentration change differed slightly between treatments, but variation within treatments was large and the difference between groups not statistically significant, as described above. In the incubations with PE films, the CO2 concentration increased until week 30 and then stabilized. In the control experiment, this stabilization was observed from week 20 until week 40. In both the BD plastic treatments, a decrease in the CO2 concentration relative to the preceding weeks was observed in the middle part of the incubation period, from either week 15 or 20 (MF-S and MF-R, respectively) to week 30, after which the concentrations stabilized. Individual cores, however, showed variations within the treatments, and the difference between treatments was not statistically significant, as stated above. Overall, the results show that the BD plastic treatments did not produce larger amounts of CO2 than the control (no addition) treatment.
Methane production from all sediments, including the control treatment, remained around zero from week 0 to week 15. After week 15, the methane concentration in the PE treatments increased slightly (Fig. 3; more detailed view available in the Supplement, Fig. S4), and in the BD plastics treatment MF-S it was more pronounced. In the control experiment, methane concentrations dipped slightly below the starting concentrations but then increased from week 28 onwards, albeit with large variations between individual sediment cores. Both BD plastic treatments showed large increases in relative amounts of methane from week 20 onwards, although the large variations between individual cores within the treatments lead to large, overlapping error bars. The stabilization of the CO2 concentration and the increase in CH4 concentration in the BD plastics experiments matched time-wise, both occurring from week 15–20 onwards, although the increase in CH4 continued after week 28, when the CO2 concentration in the BD plastic experiments had stabilized.
Visualization of the plastic film surfaces after retrieval from the sediments after 40 weeks did not show any clear signs of degradation or biofilm formation on any of the film types (PE, MF-S, MF-R; Fig. S5). DAPI staining showed a low number of microbes on the plastics' surfaces. Both regular and microscopic visualization, of all types of used plastics, showed no variation in appearance between the end and the start of the experiment.
The biodegradability of BD plastic films depends largely on the match between their composition and the environment. In soils, BD mulch films have been shown to biodegrade successfully (Li et al., 2014; Sintim et al., 2020). However, due to this required match between the properties of the material and the in situ conditions for biodegradation, transport of BD mulch films to aquatic environments could result in a buildup of material, as is suggested by our results. The biodegradability of the mulch films investigated in this research is in strong contrast to the biodegradability of these films under previously investigated soil conditions. Whereas within several soils substantial degradation of the incorporated BD plastic films was observed (11 %–98 % after 12 months, determined by “percentage of area remaining”; Li et al., 2014; 12 %–23 % degradation of powdered BD plastics after 28 d, determined by weight loss; Adhikari et al., 2016), no biodegradation could be observed in our sediment experiments, either based on produced CO2 or from visual analysis by microscopy. Similar conclusions were drawn based on a 100 d marine coastal zone sediment experiment (Nauendorf et al., 2016), even though biofilm growth on biodegradable plastic bags (composed of biodegradable polyester and corn starch) was observed in that study. Freshwater BD plastic degradation studies are more rare and are focused on biofilm formation rather than on biodegradation rates (Morohoshi et al., 2018). As observed by Nauendorf et al. (2016), biofilm formation and degradation are not necessarily related. Most marine sediment studies combine a biofilm assessment with visual assessments of degradation but do not quantify the degradation as either respiration or fragmentation. Quantification of degradation percentages based on assessment of the remaining plastics is difficult, as microparticles that form as a result of physical breakdown are difficult to recover and quantify (Wei et al., 2021). Furthermore, weight assessments are often unreliable, as microbial biomass attached to the plastics is difficult to remove completely without disturbing the plastic mass itself (Nauendorf et al., 2016). Our results show a large variation between individual incubations within treatments (Fig. S2). Sediment cores were all taken from the same location but by several multicorer casts. Potentially, variations between the cores existed from the start, for example, in the amount of carbon present in each sediment core. By randomly distributing the cores over the treatments, we have guaranteed that this did not cause a biased result in the treatment comparisons. It may, however, have resulted in larger variations within treatments. Furthermore, it is possible that certain cores were more disturbed than others while being transported to the lab, resulting in slightly changed oxygen conditions within the upper centimeters of the cores. Overall, the effect of the treatments did not exceed the variation between incubations within each treatment (Fig. 3), indicating that there was no strong treatment effect.
The BD mulch films used in this study were designed to degrade in agricultural soils. A major difference between aquatic sediment conditions and soils may be the oxygen availability: due to the low diffusion speed of oxygen through water, oxygen is limiting in lake sediments, despite oxygen-rich overlying water. Lake Lucerne, where the sediments used in this study were collected, is an oligotrophic lake, with a high oxygen availability in the bottom waters (which has also remained during the experiment duration). In Lake Lucerne sediments, oxygen has been shown to be depleted from 1.0 cm downwards (Fiskal et al., 2019). The plastics were buried shallowly in the sediments and will, at least partly, have been surrounded by sediments containing dissolved O2 (DO) in our experiments. Although exact DO penetration depths can only be determined with micro-sensors, we can assume initial oxic conditions here based on the natural oxygen penetration depth of these sediments (Fiskal et al., 2019), the oxygen concentration in the overlying water (±400 µM; Fiskal et al., 2019), and the disturbance caused by the pushing of the plastics into the sediments, bringing oxygen-rich water into contact with the sediment surrounding the plastic film. After the initial oxic conditions, DO may have become depleted within the originally anoxic sediment depths (> 1 cm) or shallower. Although under oxic conditions microbial growth and substrate turnover is expected to occur more rapidly, earlier research showed similar BD plastic biodegradation rates under oxic and anoxic conditions, despite higher microbial biomass formation on BD plastics under oxic incubation conditions (Nauendorf et al., 2016). The onset of methane production in our incubations, from week 15 onwards, paired with the stabilization of the CO2 concentration suggests that anoxic conditions established after 15 weeks into the experiments. Although the increase in methane concentration in our MF-S and MF-R experiments could suggest enhanced breakdown of the BD plastics, it's timely match to the decrease in CO2 production rather suggests that the respiration of sedimentary carbon, rather than the BD plastics carbon, is being degraded, similarly to the control and PE experiment. It is, however, interesting to note that the BD plastic experiments experienced this oxic–anoxic conversion, whereas the control and PE plastics did not.
Within soil experiments, several biotic and abiotic factors affect the extent of biodegradation. As the used BD mulch films are designed to biodegrade in soils, we also consider these factors to compare the biodegradation in the aquatic realm to that of the soils. A first factor would be abiotic fragmentation by to photodegradation, which requires sunlight to reach the plastics. In soils, photodegradation affects the plastics, decreasing their quality and molecular weight (Zhang et al., 2021). In shallow surface waters, photodegradation may also affect plastic degradation. Our experiments, with sediment cores of 40 m water depth, were performed in the dark, as photodegradation is not relevant at such water depths. For future studies, pre-degradation of plastics by photodegradation, prior to incorporation into the sediment, would be interesting, as further discussed below. Besides photodegradation, Li et al. (2014) identified soil pH, soil temperature, and fungi abundance as potential factors for differences between degradation efficiency between sites. Sander (2019) suggested that nitrogen limitations in soils may affect and limit BD plastic biodegradation, as the N-poor BD plastics solely supply the colonizing microorganisms with carbon, hydrogen, and oxygen-rich compounds and not with nitrogen. Organisms will therefore have to acquire nitrogen from different sources, which, if not sufficiently available, may limit growth and activity of the BD-plastic-colonizing and plastic-degrading organisms. Lopardo et al. (2019) showed that the addition of BD plastics to aquaculture eluent filters can improve nitrogen removal efficiency by providing an N-limited carbon source. Lake Lucerne sediments have been shown to contain relatively high amounts of nitrate up to around 10 cm depth (2 µM at 2.5 cm, 33 µM at 0.5 cm depth; Fiskal et al., 2019). Nitrogen limitation is therefore not expected to have limited microbial colonization or growth in the experiments, although more research would be needed to determine the limiting factors for microbial growth. The here-used mulch films were new and not deployed on agricultural fields prior to incubation. Therefore, their degradation status might differ from a part of the mulch films that enters aquatic environments from agricultural fields. It is, however, unknown what the general state of plastics are when they are transported towards the aquatic ecosystem. Newer BD mulch foils are more likely to still contain the most labile, easily degradable compounds, which may be degraded after deployments in fields, either by chemical or biological degradation. On the other hand, used mulch films are more likely to contain a larger surface area and are more likely to already contain a biofilm. Further research on a potential priming effect of soil pre-incubation is necessary. Furthermore, it would be interesting to test other lake environments, such as eutrophic lakes, which differ in microbial and chemical composition from the currently studied Lake Lucerne (van Grinsven et al., 2022).
The limited mobility of soil bacteria has earlier been suggested to potentially limit colonization of BD plastics in soils, as direct contact is required between the soil and plastics to allow for colonization (Sander, 2019). Such problems may, however, be easier overcome within aquatic environment, as the water saturation allows for a higher mobility of microbes and an easier exchange of compounds via diffusion and flow. However, the community of microorganisms may differ strongly between soil and sediment environments, mostly due to different redox and nutrient conditions between the two environments. As the genera of microbes that are capable of BD plastic degradation are still being investigated and discovered, especially in freshwater environments, it is not possible to simply scan the microbial community for BD plastic degraders and make an assessment on the potential for biodegradation based on the microbial community composition. We did not determine the microbial community composition in these experiments, as the results would most likely not have been able to provide us information on the plastic degradation: the sedimentary microbial community is highly diverse, differs strongly with depth, and contains many organisms with unknown functions (Han et al., 2020). The lack of suitable BD plastic degrading microorganisms is, however, the most likely explanation for the large difference in BD mulch film decomposition between our sediment experiment and previously performed soil experiments. The sediments likely lack microorganisms that produce hydrolytic enzymes (esterases) that can cleave PBAT and PLA, the main polyesters in the BD films used. When specific genes known to be associated to BD plastic degradation are identified in the future, it would be interesting to screen our incubation experiment sediments for those genes to confirm such a hypothesis.
Two types of biodegradable plastic mulch films showed no signs of degradation over a 10-month period within lake surface sediments, suggesting very limited biodegradability in freshwater sediment environments. These findings stress the importance of limiting the transport of plastics from agricultural soils to waterways – not only for traditional plastics but also for soil-biodegradable plastics. Given that these plastics are designed to remain in the soil rather than to be removed after use, they remain present in the natural environment until complete degradation. The extent of the transport of BD mulch films from soils to waterways and lakes is up to this point unknown. Our results show that it is of high importance to investigate how much of the applied biodegradable mulch films are transported to aquatic environments to be able to assess the potential pollution of lakes and coastal areas by the current and future applications of biodegradable plastic mulch films.
The data used in this paper are available at https://doi.org/10.5281/zenodo.8426091 (van Grinsven and Schubert, 2023).
The supplement related to this article is available online at: https://doi.org/10.5194/bg-20-4213-2023-supplement.
SvG designed the experiments with help from CS. SvG conducted the experiments and laboratory analyses. SvG wrote the manuscript, with assistance from CS.
The contact author has declared that neither of the authors has any competing interests.
Publisher's note: Copernicus Publications remains neutral with regard to jurisdictional claims made in the text, published maps, institutional affiliations, or any other geographical representation in this paper. While Copernicus Publications makes every effort to include appropriate place names, the final responsibility lies with the authors.
We want to thank Michael Sander for his valuable contributions to the paper and for providing the biodegradable plastic mulch films. We thank Patrick Kathriner and Kathrin Baumann for the help in the lab and field, as well as Natsumi Maeda for the help with the incubation experiments.
This paper was edited by Tina Treude and reviewed by two anonymous referees.
Adhikari, D., Mukai, M., Kubota, K., Kai, T., Kaneko, N., Araki, K. S., and Kubo, M.: Degradation of Bioplastics in Soil and Their Degradation Effects on Environmental Microorganisms, J. Agric. Chem. Environ., 5, 23–34, https://doi.org/10.4236/jacen.2016.51003, 2016.
Briassoulis, D., Pikasi, A., Papardaki, N. G., and Mistriotis, A.: Aerobic biodegradation of bio-based plastics in the seawater/sediment interface (sublittoral) marine environment of the coastal zone – Test method under controlled laboratory conditions, Sci. Total Environ., 722, 137748, https://doi.org/10.1016/j.scitotenv.2020.137748, 2020.
Dris, R., Imhof, H., Sanchez, W., Gasperi, J., Galgani, F., Tassin, B., Laforsch, C., Dris, R., Imhof, H., Sanchez, W., Gasperi, J., Galgani, F., Tassin, B., and Laforsch, C.: Beyond the ocean: contamination of freshwater ecosystems with (micro-)plastic particles, Environ. Chem., 12, 539–550, https://doi.org/10.1071/EN14172, 2015.
Egessa, R., Nankabirwa, A., Basooma, R., and Nabwire, R.: Occurrence, distribution and size relationships of plastic debris along shores and sediment of northern Lake Victoria, Environ. Pollut., 257, 113442, https://doi.org/10.1016/j.envpol.2019.113442, 2020.
Eich, A., Mildenberger, T., Laforsch, C., and Weber, M.: Biofilm and diatom succession on polyethylene (PE) and biodegradable plastic bags in two marine habitats: Early signs of degradation in the pelagic and benthic zone?, PLoS One, 10, 1–16, https://doi.org/10.1371/journal.pone.0137201, 2015.
Fiskal, A., Deng, L., Michel, A., Eickenbusch, P., Han, X., Lagostina, L., Zhu, R., Sander, M., Schroth, M. H., Bernasconi, S. M., Dubois, N., and Lever, M. A.: Effects of eutrophication on sedimentary organic carbon cycling in five temperate lakes, Biogeosciences, 16, 3725–3746, https://doi.org/10.5194/bg-16-3725-2019, 2019.
Galloway, T. S., Cole, M., and Lewis, C.: Interactions of microplastic debris throughout the marine ecosystem, Nat. Ecol. Evol., 1, 1–8, https://doi.org/10.1038/s41559-017-0116, 2017.
Gao, H., Yan, C., Liu, Q., Ding, W., Chen, B., and Li, Z.: Effects of plastic mulching and plastic residue on agricultural production: A meta-analysis, Sci. Total Environ., 651, 484–492, https://doi.org/10.1016/J.SCITOTENV.2018.09.105, 2019.
Hale, R. C., Seeley, M. E., La Guardia, M. J., Mai, L., and Zeng, E. Y.: A Global Perspective on Microplastics, J. Geophys. Res.-Oceans, 125, 1–40, https://doi.org/10.1029/2018JC014719, 2020.
Han, X., Schubert, C. J., Fiskal, A., Dubois, N., and Lever, M. A.: Eutrophication as a driver of microbial community structure in lake sediments, Environ. Microbiol., 22, 3446–3462, https://doi.org/10.1111/1462-2920.15115, 2020.
Li, C., Moore-Kucera, J., Miles, C., Leonas, K., Lee, J., Corbin, A., and Inglis, D.: Degradation of Potentially Biodegradable Plastic Mulch Films at Three Diverse U.S. Locations, Agroecol. Sustain. Food Syst., 38, 861–889, https://doi.org/10.1080/21683565.2014.884515, 2014.
Lopardo, C. R., Zhang, L., Mitsch, W. J., and Urakawa, H.: Comparison of nutrient retention efficiency between vertical-flow and floating treatment wetland mesocosms with and without biodegradable plastic, Ecol. Eng., 131, 120–130, https://doi.org/10.1016/j.ecoleng.2019.01.024, 2019.
Lott, C., Eich, A., Unger, B., Makarow, D., Battagliarin, G., Schlegel, K., Lasut, M. T., and Weber, M.: Field and mesocosm methods to test biodegradable plastic film under marine conditions, PLoS One, 15, 1–26, https://doi.org/10.1371/journal.pone.0236579, 2020.
Lott, C., Eich, A., Makarow, D., Unger, B., van Eekert, M., Schuman, E., Reinach, M. S., Lasut, M. T., and Weber, M.: Half-Life of Biodegradable Plastics in the Marine Environment Depends on Material, Habitat, and Climate Zone, Front. Mar. Sci., 8, 1–19, https://doi.org/10.3389/fmars.2021.662074, 2021.
Morohoshi, T., Oi, T., Aiso, H., Suzuki, T., Okura, T., and Sato, S.: Biofilm formation and degradation of commercially available biodegradable plastic films by bacterial consortiums in freshwater environments, Microbes Environ., 33, 332–335, https://doi.org/10.1264/jsme2.ME18033, 2018.
Nauendorf, A., Krause, S., Bigalke, N. K., Gorb, E. V., Gorb, S. N., Haeckel, M., Wahl, M., and Treude, T.: Microbial colonization and degradation of polyethylene and biodegradable plastic bags in temperate fine-grained organic-rich marine sediments, Mar. Pollut. Bull., 103, 168–178, https://doi.org/10.1016/j.marpolbul.2015.12.024, 2016.
Nelson, T. F., Remke, S. C., Kohler, H. P. E., McNeill, K., and Sander, M.: Quantification of Synthetic Polyesters from Biodegradable Mulch Films in Soils, Environ. Sci. Technol., 54, 266–275, https://doi.org/10.1021/acs.est.9b05863, 2020.
Ren, S. Y., Kong, S. F., and Ni, H. G.: Contribution of mulch film to microplastics in agricultural soil and surface water in China, Environ. Pollut., 291, 118227, https://doi.org/10.1016/J.ENVPOL.2021.118227, 2021.
Sander, M.: Biodegradation of Polymeric Mulch Films in Agricultural Soils: Concepts, Knowledge Gaps, and Future Research Directions, Environ. Sci. Technol., 53, 2304–2315, https://doi.org/10.1021/acs.est.8b05208, 2019.
Serrano-Ruiz, H., Martin-Closas, L., and Pelacho, A. M.: Biodegradable plastic mulches: Impact on the agricultural biotic environment, Sci. Total Environ., 750, 141228, https://doi.org/10.1016/j.scitotenv.2020.141228, 2021.
Sintim, H. Y., Bary, A. I., Hayes, D. G., Wadsworth, L. C., Anunciado, M. B., English, M. E., Bandopadhyay, S., Schaeffer, S. M., DeBruyn, J. M., Miles, C. A., Reganold, J. P., and Flury, M.: In situ degradation of biodegradable plastic mulch films in compost and agricultural soils, Sci. Total Environ., 727, 138668, https://doi.org/10.1016/J.SCITOTENV.2020.138668, 2020.
van Grinsven, S. and Schubert, C.: Soil-biodegradable plastic films do not decompose in a lake sediment over 9 months of incubation, Zenodo [data set], https://doi.org/10.5281/zenodo.8426091, 2023.
van Grinsven, S., Meier, D. V, Michel, A., Han, X., Schubert, C. J., and Lever, M. A.: Redox Zone and Trophic State as Drivers of Methane-Oxidizing Bacterial Abundance and Community Structure in Lake Sediments, Front. Environ. Sci., 10, 1–22, https://doi.org/10.3389/fenvs.2022.857358, 2022.
van Grinsven, S., Maeda, N., Glombitza, C., Lever, M. A., and Schubert, C. J.: The effect of a short oxygen exposure period on algal biomass degradation and methane formation in eutrophic and oligotrophic lake sediments, in preparation, 2023.
Wei, X. F., Bohlén, M., Lindblad, C., Hedenqvist, M., and Hakonen, A.: Microplastics generated from a biodegradable plastic in freshwater and seawater, Water Res., 198, 117123, https://doi.org/10.1016/j.watres.2021.117123, 2021.
Yang, Y., Li, P., Jiao, J., Yang, Z., Lv, M., Li, Y., Zhou, C., Wang, C., He, Z., Liu, Y., and Song, S.: Renewable sourced biodegradable mulches and their environment impact, Sci. Hortic.-Amsterdam, 268, 109375, https://doi.org/10.1016/j.scienta.2020.109375, 2020.
Yang, Z., Lü, F., Zhang, H., Wang, W., Xu, X., Shao, L., Che, Z., Lu, B., Ye, J., and He, P.: A neglected transport of plastic debris to cities from farmland in remote arid regions, Sci. Total Environ., 807, 150982, https://doi.org/10.1016/J.SCITOTENV.2021.150982, 2022.
Zhang, K., Hamidian, A. H., Tubić, A., Zhang, Y., Fang, J. K. H., Wu, C., and Lam, P. K. S.: Understanding plastic degradation and microplastic formation in the environment: A review, Environ. Pollut., 274, 116554, https://doi.org/10.1016/j.envpol.2021.116554, 2021.
Zumstein, M. T., Schintlmeister, A., Nelson, T. F., Baumgartner, R., Woebken, D., Wagner, M., Kohler, H. P. E., McNeill, K., and Sander, M.: Biodegradation of synthetic polymers in soils: Tracking carbon into CO2 and microbial biomass, Sci. Adv., 4, eaas9024, https://doi.org/10.1126/sciadv.aas9024, 2018.