the Creative Commons Attribution 4.0 License.
the Creative Commons Attribution 4.0 License.
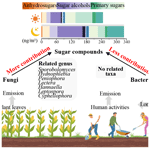
Characteristics of bacterial and fungal communities and their associations with sugar compounds in atmospheric aerosols at a rural site in northern China
Mutong Niu
Shu Huang
Yajie Wang
Wanyun Xu
Wan Wei
Qiang Zhang
Zihan Wang
Donghuan Zhang
Rui Jin
Libin Wu
Junjun Deng
Fangxia Shen
Bioaerosols play significant roles in causing health and climate effects. Sugar compounds in air have been widely used to trace the source of bioaerosols. However, knowledge about the association of sugar molecules and the microbial community at taxonomic levels in atmospheric aerosols remains limited. Here, microbial community compositions and sugar molecules in total suspended particles collected from a typical rural site, Gucheng, in the North China Plain were investigated by gas chromatography–mass spectrometry and high-throughput gene sequencing, respectively. Results show that fungal community structure exhibited distinct diurnal variation with largely enhanced contribution of Basidiomycota at night, while bacterial community structure showed no obvious difference between daytime and night. SourceTracker analysis revealed that fungi and bacteria were mainly from plant leaves and unresolved sources (presumably human-related emissions and/or long-distance transport). All the detected anhydrosugars and sugar alcohols and trehalose showed diurnal variations with lower concentrations in the daytime and higher concentrations at night, which may be affected by enhanced fungal emissions at night, while primary sugars (except trehalose) showed an opposite trend. Mantel's test showed that more sugar compounds exhibited significant associations with fungal community structure than bacterial community structure. Co-occurrence analysis revealed the strong associations between sugar compounds and a few saprophytic fungal genera with low relative abundances, e.g., Hannaella, Lectera, Peniophora, Hydnophlebia, Sporobolomyces and Cyphellophora. This study suggests that the entire fungal community, rather than specific fungal taxa, likely greatly contributes to sugar compounds in rural aerosols, while the contribution of bacteria is limited.
- Article
(2788 KB) - Full-text XML
-
Supplement
(3109 KB) - BibTeX
- EndNote
Bioaerosols mainly refer to particulate matter with biological origin, including viruses, bacteria, fungi, pollen and their biological debris (Yao, 2018; Fröhlich-Nowoisky et al., 2016; Santl-Temkiv et al., 2020; Shen and Yao, 2022). Bioaerosols have attracted progressively more attention during the past decades due to their adverse health effects. Health problems can arise in living organisms such as humans, animals and plants through respiratory or surface-contact exposure to airborne pathogenic or allergenic microbes (Taipale et al., 2021; Arzt et al., 2011; Hicks et al., 2012), which have caused serious economic losses and even human deaths. Bioaerosols also play potentially important roles in cloud and precipitation formation by acting as cloud condensation nuclei and ice-nucleating particles (Hoose et al., 2010; Ariya et al., 2009; Hu et al., 2020; Huang et al., 2021). Certain airborne microorganisms can participate in atmospheric chemical processes by transforming inorganic or organic matter (Liu et al., 2020; Ariya et al., 2002).
To accurately assess the health-related and climatic effects of bioaerosols and, more importantly, to develop effective bioaerosol pollution control approaches, investigations into the abundance, compositions and emission fluxes of bioaerosols need to be taken seriously. Previous studies have found that in terms of number and mass concentrations, bioaerosols could account for ∼ 30 % of total aerosols (size larger than ∼ 1 µm) in urban or rural sites (Després et al., 2012; Huffman et al., 2013; Schumacher et al., 2013), while in the rainforest, bioaerosols account for up to 80 % (Huffman et al., 2012; Pöschl et al., 2010). Current estimates of global bioaerosol mass emission fluxes vary widely (about 10–1000 Tg a−1) (Després et al., 2012). In recent decades, molecular biology technology (e.g., fluorescence in situ hybridization techniques, denaturing gradient gel electrophoresis, terminal restriction fragment length polymorphism and sequencing technology) and fluorescence techniques (e.g., with ultraviolet aerodynamic particle sizers and wideband integrated bioaerosol sensors) have been applied to study the compositions and abundances of bioaerosols (Prass et al., 2021; Drautz-Moses et al., 2022; Zhang et al., 2017), whereas the compositions, sources and emission fluxes of bioaerosols remain less documented and quantified (Yue et al., 2016; Fröhlich-Nowoisky et al., 2016).
Sugar compounds are the main energy storage and cellular structural materials in organisms (Wang et al., 2021), and their presence in the atmosphere is often considered to be closely related to biological sources (Bauer et al., 2008a; Schliemann et al., 2008). Hence sugar compounds are often used as biomarkers to track natural sources of bioaerosols and estimate the contribution of bioaerosols to organic aerosols (Fu et al., 2008, 2010, 2013). For instance, arabitol and mannitol have been commonly used to assess fungal spore emissions (Bauer et al., 2008a; Marynowski et al., 2019), and trehalose has been considered a fungal-specific sugar since it is mainly present in mycorrhizal plants (Kaur and Suseela, 2020). Sucrose and glucose, as end products of photosynthesis, were often used to trace the emissions from plant debris and some photosynthetic prokaryotes (Goddijn and van Dun, 1999). Fructose can be used to study the spread of plant debris or pollen in the atmosphere (Fu et al., 2013).
However, most sugar compounds are widely present in living organisms. For example, a variety of bacteria have been found to synthesize mannitol (Monedero et al., 2010). Trehalose participates in osmotic protection and maintains desiccation tolerance in bacteria, yeast, insects and some plants (Zancan and Sola-Penna, 2005). Glucose, fructose, galactose and mannose have been widely found in bacteria (Walmsley et al., 1998; Deutscher et al., 2006). That is, although the sugar compounds used as biomarkers tend to be predominant in organisms they characterize, there are many possible biological sources of these sugar compounds.
A large number of studies using sugar compounds as biomarkers have focused on bacteria and fungi at the kingdom level, which may result in inaccurate source identification and emission flux estimation of bioaerosols. To the best of our knowledge, only Samaké et al. (2020) have explored the relationship between sugar compounds and microorganisms in PM10, collected in an agricultural area in France, and there is still a large lack of understanding of the relationship between microbial communities and sugar compounds at the molecular level. Such information is important for clarifying the sources of bioaerosols and improving the accuracy of bioaerosol emission flux estimates.
In this study, to refine the marker categories of sugar compounds in atmospheric aerosols, airborne total suspended particles (TSPs) were collected from a typical rural site, Gucheng, in the North China Plain. Atmospheric microbial communities (bacteria and fungi) and common sugar compounds in the TSP samples were analyzed using high-throughput gene sequencing and gas chromatography–mass spectrometry (GC-MS) techniques, respectively. Statistical analyses were exploited to search for the association between microbial communities and sugar compounds in atmospheric aerosols.
2.1 Observation and sample collection
The observation was carried out at the rooftop of the Gucheng Ecological and Agrometeorological Experiment Station, the Chinese Academy of Meteorological Sciences (39.15∘ N, 115.73∘ E; about 5 m above the ground level), in the summer of 2020 (7–13 August). The observation site is located in Dingxing County, Hebei Province, which is about 100 km and 128 km away from the two nearby megacities of Beijing and Tianjin, respectively (Fig. S1). The site is located in a rural area in the north of the North China Plain. It is a high-yield agricultural area with maize as the main summer crop, about 8 km from the nearest river and 178 km from the closest ocean. The site is about 900 m away from the nearest railway and 2.7 km from the expressway. There was no intense agricultural activity observed around the site during the observation period.
Considering the broad size range of airborne microorganisms and their attachment to other coarse particles (Fröhlich-Nowoisky et al., 2016), TSP samples loaded on quartz fiber filters (25 × 20 cm) were collected using a high-volume air sampler (Tisch Environmental, Inc., USA) daily based on daytime (D, 08:00–19:30 UTC+8; this time zone applies throughout) and nighttime (N, 20:00–07:30) at a flow rate of 1.05 m3 min−1. All filters before sampling were combusted at 450 ∘C for 6 h to remove organic matter and microorganisms. The filters after sampling were stored in a refrigerator at −20 ∘C for later analysis.
Meteorological parameters including air temperature, relative humidity, wind speed and direction, and precipitation are recorded in real-time monitoring data obtained from a weather station in Xushui, Hebei (about 18 km away from the sampling site). Air quality parameters including the AQI (air quality index), PM2.5, PM10, NO2, O3, SO2 and CO at the Baoding environmental monitoring station (about 36 km away from the sampling site) were obtained from China National Environmental Monitoring Center (http://www.cnemc.cn/, last access: 15 November 2023).
2.2 Taxonomic identification of airborne microbial community
High-throughput gene sequencing technique was used to analyze airborne bacterial and fungal community structures. A piece of 18 cm2 filter for each sample was cut and placed in a lysis tube, added to Solution 1 provided by PowerSoil DNA Isolation Kit (MO BIO Laboratories, USA), and then placed at 65 ∘C for 20 min to reduce the adsorption of quartz to DNA. Subsequently, the solution was processed according to the manufacturer's instructions. Polymerase chain reaction (PCR) amplification was conducted in a 25 µL reaction solution, including 12.5 µL KAPA2G Robust HotStart ReadyMix, 1 µL of 5 µM of each primer, 1.5 µL genome DNA and 9 µL double-distilled H2O. The PCR primer pairs are 338F (5′-ACTCCTACGGGAGGCAGCA-3′) and 806R (5′-GGACTACHVGGGTWTCTCATAT-3′) in the bacterial V3–V4 region of 16S ribosomal RNA (rRNA) and ITS1F (5′-CTTGGTCATTTAGAGGAAGTAA-3′) and ITS2 (5′-TGCGTTCTTCATCGATGC-3′) in the fungal ITS region.
The PCR amplification was performed as follows: denaturation at 94 ∘C for 5 min; 30 cycles for bacteria and 35 cycles for fungi of denaturation, annealing and extension (94 ∘C for 30 s, 50 ∘C for bacteria and 55 ∘C for fungi for 30 s, and 72 ∘C for 60 s); and final extension at 72 ∘C for 7 min. The final PCR products were purified by AMPure XP magnetic beads and qualified with NanoDrop 2000 spectrophotometers (Thermo Fisher Scientific, Inc., USA). Then the purified DNA was used for generating a sequencing library. Finally, the library was sequenced using the Illumina MiSeq 2500 platform (2 × 250 paired ends) at Allwegene Tech. Co., Ltd., Beijing, China.
The paired-end reads were quality-controlled using Trimmomatic v0.33 and then merged using FLASH v1.2.11 software to produce the raw tags. Subsequently, the raw tags were identified based on the barcode and primer using mothur v1.35.1 and were filtered by removing the barcode and primer. Then the clean sequences with ≥ 97 % similarity were assigned to one operational taxonomic unit (OTU). Taxonomic annotation of each OTU for bacteria and fungi was performed using the SILVA 16S and UNITE databases, respectively.
2.3 Determination of sugar components
A piece of each sampled filter was extracted by sonication for 10 min using a 10 mL dichloromethane and methanol mixture (2:1, ). The extracts were filtered through a Pasteur pipette filled with quartz wool, concentrated to approximately 100 µL using a rotary evaporator and then dried under nitrogen. The silylation derivatization was performed by reaction with 60 µL N,O-bis-(trimethylsilyl)trifluoroacetamide (BSTFA) including 1 % trimethylsilyl chloride and pyridine (5:1, ) at 70 ∘C for 3 h. After the derivatization, hexane solution containing C13 n-alkane internal standard (1.43 ng µL−1) was added to the derivatives. The samples were stored at −20 ∘C before GC-MS analysis.
Sugar compounds were quantified by a GC-MS system (7890A–5975C, Agilent Technologies, USA). The GC instrument was equipped with a split–splitless injection and a fused silica capillary column (DB-5MS, 30 m × 0.25 mm × 0.25 µm, Hewlett-Packard). The GC oven temperature procedure was as follows: initiation at 50 ∘C for 2 min, heating to 120 ∘C at a rate of 15 ∘C min−1, heating to 300 ∘C at a rate of 5 ∘C min−1, stabilization for 16 min and finally decreasing to 50 ∘C. The carrier gas was ultrapure helium (1.3 mL min−1). The GC injector temperature was 280 ∘C, the ion source temperature was 230 ∘C, and the quadrupole temperature was 150 ∘C. The MS instrument was operated in electron ionization (EI) mode at 70 eV and scanned from 50 to 650 Da. Mass spectral data were processed using the ChemStation software, and sugar compounds were identified and quantified using authentic standards. The recoveries of sugar compounds ranged from 80 % to 120 %. Reproducibility of the method was larger than 90 %.
2.4 Chemical component analyses
Organic carbon (OC) and elemental carbon (EC) were quantified by an OC EC analyzer (Sunset Laboratory Inc., USA) according to the thermal optical transmission (TOT) method of the NIOSH protocol.
A portion of the filters was cut and extracted with 20 mL ultrapure water by ultrasonication for 20 min. Then the extracts were filtered using 0.22 m PTFE filters (Millex-GV, Millipore, USA). The filtrate was used for the determination of water-soluble inorganic components including Na+, K+, Mg2+, Ca2+, NH, SO, NO and Cl− with an ion chromatography system (ICS-5000+, Thermo Fisher, USA).
2.5 Statistical analyses
SourceTracker utilizes a Bayesian approach to identifying sources and estimating source proportions for microbial surveys (Knights et al., 2011). The source samples used as source tracking reference databases in this study were soils, plant leaves and seawater. Maize leaves and soil samples were collected around the observation site, the samples were subjected to high-throughput sequencing as described in Sect. 2.2 to obtain the gene sequences, and the marine microbial sequences were obtained from the NCBI SRA database (https://www.ncbi.nlm.nih.gov/sra/, last access: 15 November 2023, Table S1). The sequences of all source and sink samples were processed together by QIIME 2 and were assigned to OTUs based on the similarity of sequences ≥ 97 %. The output data were performed for source tracking analysis based on the “SourceTracker2” package in R (https://github.com/caporaso-lab/sourcetracker2, last access: 15 November 2023).
One-way ANOVA was performed using SPSS v.22.0 to analyze the diurnal differences in the microbial community. Spearman correlation analysis was used to evaluate the relationship between microbial taxa and environmental or pollution factors. A p value < 0.05 indicates a significant correlation. Non-metric multidimensional scaling (NMDS), principal coordinate analysis (PCoA), redundancy analysis (RDA), linear discriminant analysis effect size (LEfSe) analysis (http://huttenhower.sph.harvard.edu/lefse/, last access: 15 November 2023) and Mantel's test were conducted using R scripts. The taxa with linear discriminant analysis (LDA) score > 2 were selected. The Infer Community Assembly Mechanisms by Phylogenetic bin-based null model analysis (iCAMP) is a general framework to quantitatively estimate community assembly mechanisms (Ning et al., 2020; Chen et al., 2022). The assembly mechanisms of airborne microbes at the Gucheng site were investigated using the iCAMP package in R. For network analysis, Spearman's correlation between microbial taxa with sugar compounds was calculated using the “Hmisc” package in R. All p values were adjusted using the Benjamini and Hochberg false discovery rate (FDR) controlling procedure (Benjamini et al., 2006). Then, those significant correlations between microbial taxa and sugar molecules (FDR-adjusted p value < 0.05, correlation coefficients > 0.6) were selected. Network properties were calculated using the “igraph” package in R. Network visualization was conducted using Gephi v0.9.3. Modules were identified using the Louvain method provided by Gephi v0.9.3 (Bastian et al., 2009).
3.1 Diurnal variation in microbial community
3.1.1 Microbial diversity and richness
A total of 38 565 16S rRNA gene sequences and 56 920 ITS sequences were obtained by high-throughput sequencing technology and were assigned to 404 bacterial OTUs and 391 fungal OTUs. The sequencing coverage of all the samples was > 99 %, indicating that the sequencing data could represent the microbial communities in the atmosphere.
Alpha diversity indices were used to evaluate the diurnal differences in microbial community structure (Fig. 1 and Table S2). For bacterial communities, OTU and the Chao1 index used to evaluate richness showed no significant diurnal patterns. Except for the samples collected on 13 August, the bacterial diversity (Shannon's and Simpson's indices) in the daytime samples was slightly lower than or comparable to that in the nighttime samples (p>0.05, Fig. 1a). In contrast, the diversity and richness of fungal community were significantly higher at night than during the daytime (p<0.05, Fig. 1b). Abdel Hameed et al. (2009) found that bacteria and fungi had similar diurnal variation patterns over Cairo, Egypt. However, the bacterial diversity in aerosols over Guilin, China, was slightly more abundant during the day than at night (Long et al., 2022), which was similar to the finding over tropical forest aerosols in August (Gusareva et al., 2019). These different results suggest that the diversity and biogeography of airborne microorganisms might be influenced by multiple factors, e.g., geographical locations, airflow patterns and global atmospheric circulation (Fröhlich-Nowoisky et al., 2012; Martiny et al., 2006; Womack et al., 2010).
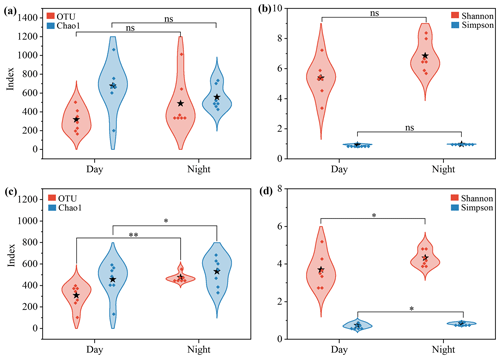
Figure 1The α diversity of the airborne microbial community at the rural Gucheng site. (a–b) Bacterial and (c–d) fungal diversity and richness in the daytime and nighttime samples were compared based on one-way ANOVA. The dots in the violin shapes represent all data points. Stars represent the average value of all data. * and represent the p value being less than 0.05 and 0.01, respectively; ns indicates that there is no significant difference.
3.1.2 Bacterial community structure
Figure 2 shows the bacterial and fungal community compositions in TSP samples collected at rural Gucheng, northern China. At the phylum level, 38 bacterial phyla were detected. Proteobacteria (42.2 %), Firmicutes (26.1 %), Actinobacteria (9.0 %), Cyanobacteria (8.7 %) and Bacteroidetes (8.4 %) were the most abundant bacterial phyla among all the TSP samples (Fig. 2a), which were also observed as the dominant phyla in the air of other regions (Acuna et al., 2022; Chen et al., 2022; Xu et al., 2017). The relative abundance of Cyanobacteria slightly increased in the daytime (p>0.05) with warmer temperatures (Singh et al., 2018). Firmicutes had a higher relative abundance in the nighttime (p<0.05, Fig. 3a). Gusareva et al. (2019) also found that high temperature had an important impact on the bacterial community structure, and the abundance of Firmicutes was higher at noon when the temperature was the highest. Drautz-Moses et al. (2022) reported significant diurnal differences in the relative abundance of the phylum Firmicutes in aerosols in the lower troposphere.
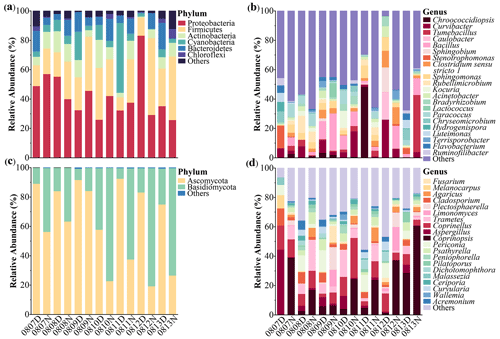
Figure 2Taxonomic compositions of microorganisms in TSP samples collected at the rural Gucheng site. Relative abundances of identified dominant bacterial (a–b) and fungal (c–d) taxa at the phylum (a, c) and genus (b, d) levels are shown. D and N in the sample IDs mean daytime and nighttime, respectively.
At the order level, Bacillales (15.1 %), Clostridiales (9.6 %), Burkholderiales (8.8 %), Sphingomonadales (5.8 %) and Myxococcales (5.4 %) were predominant (Fig. S2a) and no significant diurnal differences were found. At the genus level, Bacillus (6.9 %), Curvibacter (6.3 %), Chroococcidiopsis (4.2 %) and Tumebacillus (4.1 %) were the most abundant bacterial genera (Fig. 2b). Bacillus and Chroococcidiopsis were the dominant bacterial genera in the air worldwide (Zhao et al., 2022). Curvibacter occurring mainly in the aqueous environment (Xu et al., 2021) and the spore-forming bacterium Tumebacillus occurring in permafrost (Steven et al., 2008) and soils (Wemheuer et al., 2017) had smaller relative abundances observed in previous studies (Yan et al., 2018). Geographical locations and land use types may shape atmospheric bacterial community structure (Bowers et al., 2011). Shen et al. (2019) found that the relative abundances of Bacillus and Curvibacter in rural aerosols were about 90 times higher than those in urban aerosols.
3.1.3 Fungal community structure
Fungal community structure exhibited distinct diurnal differences based on the results of NMDS analysis (Fig. S3). The Bray–Curtis dissimilarity index of fungal communities was slightly higher at night (0.46) than in the daytime (0.41), suggesting the high β diversity of fungal community at night. Co-occurrence network analysis also revealed that the average degree of fungal communities at night (10.0) was greater than in the daytime (8.9), suggesting that fungal taxa cooperated more closely with each other at night.
From community compositions, fungi were almost always composed of the phyla Ascomycota (63.1 %) and Basidiomycota (36.7 %) (Fig. 2c). Their relative abundances were similar to the results of rural aerosols collected from Backgarden, a village near Guangzhou, southern China (Fröhlich-Nowoisky et al., 2012). Due to the more abundant Basidiomycota found in natural soils (Hui et al., 2017), Basidiomycota accounted for a relatively high proportion of rural aerosols compared with urban aerosols (Liu et al., 2019). Significant diurnal differences were found in these two phyla (daytime: Ascomycota 81.9 % vs. Basidiomycota 17.8 %, nighttime: Ascomycota 44.3 % vs. Basidiomycota 55.5 %, p<0.01) (Fig. 3b). LEfSe analysis likewise shows clear diurnal differences in fungal taxa. The percentage of taxa belonging to Ascomycota increased significantly in the daytime, while taxa belonging to Basidiomycota dominated at night (Fig. S4). The release of fungal spores is strongly linked to environmental humidity (Niu et al., 2021). It has been found that Ascomycota prefers to release spores in relatively dry environments, while Basidiomycota prefers wet environments (Almaguer et al., 2014; Elbert et al., 2007). Drautz-Moses et al. (2022) also observed significant diurnal differences in certain fungal phyla; e.g., Basidiomycota had higher proportions at night. At the order level, Pleosporales (67.4 %) dominated in the daytime, while Pleosporales (33.5 %), Agaricales (34.6 %) and Polyporales (13.3 %) were the dominant orders in the nighttime (Fig. S2b). Polyporales and Agaricales, belonging to Basidiomycota, were observed to be enhanced in autumn when rainfall was higher (Abrego et al., 2018). These saprotrophic Basidiomycetes could release more spores at night due to higher air moisture (Kramer, 1982).
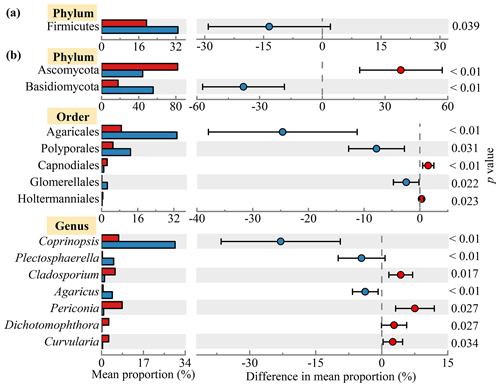
Figure 3Diurnal variations in the proportions of microbial taxa. The relative abundances of (a) bacterial and (b) fungal phyla, orders and genera with significant diurnal differences (p<0.05) based on one-way ANOVA are shown in the left part of the figure. The right part of the figure shows the differences between the average abundances of the microbial taxa in the daytime and nighttime samples.
Significant diurnal variation was also observed for the fungal genera (Fig. 2d). More fungal genera showed higher abundances at night, influenced by temperature and humidity (Table S3) (Reyes et al., 2016). Among the dominant genera, the abundances of Coprinopsis, Coprinellus and Trametes were significantly higher at night than in the daytime (Coprinopsis: 29.9 % > 6.9 %, Coprinellus: 11.2 % > 8.9 %, Trametes: 7.7 % > 5.8 %). The abundances of Aspergillus (10.1 %), Periconia (8.4 %) and Cladosporium (5.5 %) increased significantly in the daytime (Fig. 3b). Similar diurnal patterns were found in the studies of airborne fungal spores in Valladolid (Spain) (Reyes et al., 2016) and Oklahoma (USA) (Gillum and Levetin, 2008).
Air relative humidity (RH) facilitates the sporulation process, accompanied by a reduction in atmospheric turbulence (Oliveira et al., 2009; Drautz-Moses et al., 2022), so fungal community structure showed more significant diurnal variations than bacteria.
3.2 Possible factors affecting the microbial community
Previous studies have shown that airborne microbial community structure may be influenced by the synergistic effects of meteorological and pollution factors (Zhai et al., 2018; Zhao et al., 2022). To analyze the factors affecting the microbial community at our typical rural site, Gucheng, and to identify the influences of the surrounding environment on microbial communities, the SourceTracker method and RDA were performed.
Results revealed that the airborne fungi at the Gucheng site were mainly derived from terrestrial plants (44.1 ± 29.1 %), which was similar to the previous view that plant leaves make a greater contribution to local airborne fungi (Qi et al., 2020). The sources of bacteria were mostly unresolved (98.9 ± 1.0 %) (Fig. S5a). Zhao et al. (2022) found that human-related sources contributed more to the airborne bacteria than other sources in urban areas. Bacterial communities are more likely to be influenced by human body surface flora than other environments (Jiang et al., 2022). The bacterial community over the eastern Mediterranean originated likewise mainly from anthropogenic sources (Mazar et al., 2016). However, only the contribution of natural sources including terrestrial plants, soils and ocean to the airborne microbial community was considered in this study, which may partly explain the high number of unresolved sources of bacteria. It is also noticeable that in this study only local soil and vegetation samples were selected as the source samples, and the impact of long-distance transport was not considered. Therefore, unresolved sources may also come from long-range-transported air masses (Gat et al., 2017) and other local sources, e.g., water, other vegetation and human-related sources.
RDA indicated that Ca2+ and wind speed (WS) were the main environmental variables affecting the bacterial community structure (Fig. S5b). Ca2+ is an important tracer of soil dust particles (Mace et al., 2003), and high WS is conducive to the resuspension of soil microorganisms, bringing soil microorganisms into the atmosphere (Savage et al., 2012; Jones and Harrison, 2004). The results implied that the emissions from soils played an important role in shaping the bacterial community. For fungi, air temperature and RH were the vital factors affecting the fungal community (Fig. S5b). Temperature and RH could affect the growth and release of microorganisms from the sources, especially fungi. A large number of studies have proved that temperature and RH are able to interfere with airborne fungal communities (Wang et al., 2020; Núñez et al., 2021). Different temperatures can activate the formation and release of different types of fungal spores (Pickersgill et al., 2017). High RH could promote the release of many types of basidiospores and ascospores (Elbert et al., 2007), while when the RH decreases to 40 %–60 %, the release of conidia becomes easier (Jones and Harrison, 2004). Oxidation by ozone can effectively inhibit the growth of microorganisms (Zhai et al., 2018). It should be noted that environmental factors explained only 43.4 % of the variation in bacterial communities, and it is hypothesized that ecological processes other than environmental filtration had impacts on shaping bacterial communities. Here, iCAMP analysis showed that the relative contributions of deterministic processes (environmental filtration and microbial interactions) and stochastic processes (birth and/or death, speciation/extinction, and immigration) to shaping bacterial communities were 53.7 % and 46.3 %, respectively, demonstrating the importance of other ecological processes in bacterial communities.
3.3 Diurnal variation in sugar compounds
A total of 13 sugar compounds including 3 anhydrosugars, 5 primary sugars and 5 sugar alcohols were analyzed in this study. The total concentration of sugar compounds in all the TSP samples averaged 289 ± 85.3 ng m−3 (Fig. 4a), which was comparable to previously reported concentrations in rural and mountain aerosols (Yan et al., 2019; Li et al., 2012). The daytime concentration of sugar compounds was 247 ± 67.0 ng m−3, which was lower than that (331 ± 84.4 ng m−3) in the nighttime (Table S4).
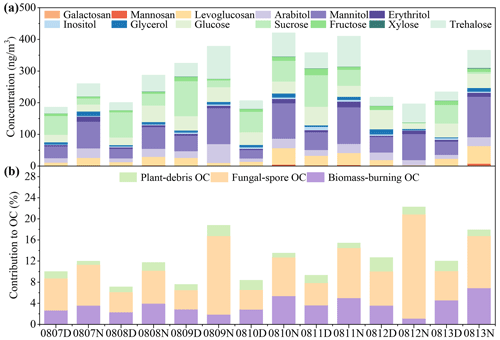
Figure 4Diurnal variations in sugar molecules in TSP samples from the rural Gucheng site. The concentrations of 13 sugar compounds including 3 anhydrosugars, 5 primary sugars and 5 sugar alcohols are shown in (a). (b) The contributions of plant-debris OC, fungal spore OC and biomass-burning OC to total OC. The concentrations of plant-debris OC, fungal spore OC and biomass-burning OC were estimated according to the previous studies (Bauer et al., 2008a; Fu et al., 2014; Puxbaum et al., 2007). D and N in the sample IDs mean daytime and nighttime, respectively.
3.3.1 Anhydrosugars
Anhydrosugars (levoglucosan and its isomers, galactosan and mannosan) are the main constituents of cellulose (Simoneit, 2002) and are specific markers for biomass burning (Simoneit et al., 1999). The concentrations of levoglucosan were in the range of 10.3–30.3 ng m−3 (average: 18.4 ± 7.43 ng m−3) in the daytime and 2.97–55.8 ng m−3 (average: 30.0 ± 20.1 ng m−3) in the nighttime, showing a diurnal variation. The abundance of levoglucosan was about 10–30 times higher than that of galactosan (daytime: 0.7 ± 0.1 ng m−3, nighttime: 1.4 ± 1.0 ng m−3) and mannosan (daytime: 1.3 ± 0.5 ng m−3, nighttime: 2.1 ± 1.6 ng m−3) (Table S4). Anhydrosugar concentrations exhibited diurnal variations (Fig. 4a), which may be influenced by cooking activities (Verma et al., 2021). Moreover, the concentrations of anhydrosugars were significantly affected by temperature (Fig. S6), which may also be responsible for the diurnal differences in anhydrosugars. Yi et al. (2021) also reported that the anhydrosugar concentrations in PM2.5 were lower in the daytime (Tai'an: 52.3 ± 36.3 ng m−3, Mt. Tai: 25.4 ± 16.2 ng m−3) than at night (Tai'an: 55.8 ± 44.3 ng m−3, Mt. Tai: 30.0 ± 19.5 ng m−3) in Shandong Province, China.
3.3.2 Primary sugars
Primary sugars including fructose, glucose, sucrose, trehalose and xylose were detected in all the TSP samples. The primary sugars in the daytime and nighttime samples were 152 ± 49.0 and 141 ± 47.2 ng m−3, respectively. These primary sugars dominated the total sugar compounds in the daytime samples (61.3 ± 0.1 %), and relatively low proportions were found at night (42.1 ± 0.1 %, Table S4), which was consistent with the results in previous studies (Zhao et al., 2020; Ren et al., 2018). Sucrose was the primary saccharide with the highest concentration in the daytime (67.6 ± 35.8 ng m−3), followed by glucose (42.4 ± 14.7 ng m−3). Sucrose has the potential origins of pollen (Fu et al., 2012) and soil resuspension (Rogge et al., 2007) and can be produced by photosynthesis in plant leaves (Medeiros et al., 2006b). However, no significant correlation was found between sucrose and soil-resuspension-related indicators (Ca2+ and PM10). Sucrose had a significant positive correlation with xylose (Table S5), which mainly originates from wood and rice straw (Chen et al., 2013; Sullivan et al., 2011), indicating that sucrose and xylose may have a similar source. It is speculated that the elevated concentrations of sucrose in the daytime may be caused by the more active metabolic activity of plants.
Trehalose was the dominant primary saccharide at night with an average of 67.6 ± 23.4 ng m−3, followed by glucose (36.6 ± 12.8 ng m−3) (Fig. 4a). Trehalose exhibited a diurnal variation with higher concentrations at night and lower concentrations in the daytime, which was in contrast to the variations in the other four detected primary sugars (Table S3). Trehalose, as a reserve carbohydrate and pressure protector (Medeiros et al., 2006a), is widely found in various organisms, such as bacteria, fungi, insects, invertebrates, algae and higher plants (Medeiros et al., 2006b). As the most abundant sugar compound in soils, trehalose was used as the tracer for soil dust resuspension (Jia and Fraser, 2011; Rogge et al., 2007), whereas, in this study, there was no significant correlation between trehalose and soil-resuspension-related indicators (Ca2+ and PM10). Trehalose was significantly negatively and positively correlated with air temperature and relative humidity, respectively (Table S5). Trehalose exhibited an apparent diurnal variation (Fig. 4a), which was consistent with the diurnal variation trend of fungal OTUs (Fig. 1b). These results indicate that trehalose was possibly affected by fungal emissions.
3.3.3 Sugar alcohols
Arabitol, mannitol, erythritol, glycerol and inositol were the main sugar alcohols detected in the TSP samples. The total concentration of sugar alcohols was averaged 115.4 ± 50.3 ng m−3, and their relative abundance was lower in the daytime (30.6 ± 0.07 %) than in the nighttime (48.4 ± 0.08 %) (Fig. 4a). Mannitol was the predominant sugar alcohol in all the samples (71.1 ± 34.8 ng m−3), followed by arabitol (23.6 ± 12.5 ng m−3). Mannitol and arabitol were the most predominant organic compounds used to indicate fungal spores and showed significant correlations with fungal community diversity in terms of OTU abundance (Table S5). Meanwhile, the temperature significantly affected the sugar alcohols (Fig. S6). Oduber et al. (2021) also reported high correlations between sugar alcohols and air temperature. This phenomenon may be corroborated by the finding that the activities of fungal spores are regulated by temperatures (Zhu et al., 2015).
The concentrations of mannitol and arabitol are commonly used to evaluate the contribution of fungal spores to ambient OC (Bauer et al., 2008a). The contributions of other primary emission sources such as biomass burning and plant debris to OC could be estimated by levoglucosan and glucose, respectively (Fu et al., 2014; Puxbaum et al., 2007; Puxbaum and Tenze-Kunit, 2003; Andreae and Merlet, 2001). The results estimated based on these tracers show that fungal-spore-derived OC dominated (204.3–977.8 ngC m−3), accounting for 3.6 %–19.7 % of OC. Plant debris and biomass burning contributed 0.4 %–1.6 % and 1.1 %–6.9 % to OC, respectively (Fig. 4b). Biological sources contributed more to primary OC in rural aerosols, while biomass-burning OC in urban aerosols was dominant in total OC (Wu et al., 2020; Yan et al., 2019). The nighttime fungal-spore-derived OC (525.5–977.8 ngC m−3) was significantly higher than that in the daytime (204.3–427.9 ngC m−3) (p<0.01), which was consistent with observations in other studies (Kang et al., 2018; Zhu et al., 2016). Many fungal spores are wet spores, preferring to release spores at night when the RH is higher.
3.4 Relationship between microbial community and sugar compounds
3.4.1 Correlation between microbial community structure and sugar compounds
To analyze the relationship between different microbial communities and sugar compounds, this study adopted Mantel's test (Fig. 5a). The bacterial community structure was closely related to sucrose, while fungal communities were associated with more frequently detected sugar molecules, including all the detectable anhydrosugars, the two sugar alcohols mannitol and erythritol, and one primary sugar sucrose. These results indicate that the fungal community may make a larger contribution to more sugar molecules than the bacterial community in the rural atmosphere. Compared to bacteria in the air, fungal emissions and concentrations are lower in number but much (an order of magnitude) higher in mass (Fröhlich-Nowoisky et al., 2016; Hoose et al., 2010).
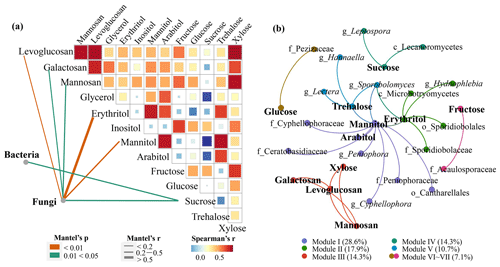
Figure 5Association between the microbial community and sugar compounds. (a) The correlations of the microbial community structures with sugar compounds based on the Bray–Curtis distance. The edge width corresponds to Mantel's r value, and the edge color denotes statistical significance. Pairwise correlations of sugar compounds with a heatmap are calculated by Spearman's correlation analysis. (b) Co-occurrence networks between microbial taxa and sugar components constructed based on Spearman's correlation analysis. The nodes are colored according to different types of modularity class.
Anhydrosugars are important tracers of biomass burning (Simoneit et al., 1999), and sucrose is considered to come mainly from wood and rice straw. Fungi are more common than bacteria on wood or plant surfaces (Lladó et al., 2017; Andrews and Harris, 2000). In this study, three genera – Coprinopsis, Coprinellus and Trametes – that are dominant in the fungal community are typical wood saprophytic fungi (Kamada et al., 2010; Nagy et al., 2012). Minor fungal genera, such as Periconia and Cladosporium, belong to endophytes (Gunasekaran et al., 2021). As mentioned above, SourceTracker analysis revealed that fungi were mainly derived from plants (Fig. S5a). Meanwhile, fungi can use levoglucosan or sucrose as the carbon source to promote growth (Prosen et al., 1993; Van der Nest et al., 2015). It was also found that bacterial community structure may be closely related to sucrose-traced plant origins, although the SourceTracker analysis results did not show the plant origin of bacteria. This inconsistency may be caused by single-plant (maize) source samples and/or the negligence of long-distance transport in this study.
The correlations between the fungal community and two sugar alcohols (mannitol and erythritol) were the most significant (correlation coefficients: 0.49 and 0.55) (Fig. 5a). Mannitol exists in fungal spores, fruiting bodies and mycelia of fungi (Solomon et al., 2007) and is considered to be the most abundant dissoluble starch inside mycelia and fruiting bodies (Dulermo et al., 2009). In fungi, mannitol can be used to store energy and extinguish reactive oxygen species (Upadhyay et al., 2015). The natural sources of erythritol are mainly yeasts and yeast-like fungi, such as Moniliella pollinis, Trichosporonoides megachiliensis, Aureobasidium sp., Trigonopsis variabilis, Trichosporon sp., Torula sp. and Candida magnoliae (Regnat et al., 2018). They mainly produce erythritol when encountering salt or osmotic pressure (Yang et al., 2015). Mannitol has been widely used to trace the origin of fungal spores in aerosols (Bauer et al., 2008a, b), and erythritol was considered to have similar sources to mannitol (Chen et al., 2013).
3.4.2 Interaction between sugar molecules and microorganisms at taxonomic levels
To elucidate the interaction between sugar molecules and microorganisms at taxonomic levels, a co-occurrence network was constructed based on Spearman's correlation between different microbial taxa and sugar molecules. Based on correlation analysis, 27 edges, which depict significant correlations between sugar molecules and taxa (FDR-adjusted p value < 0.05, correlation coefficients > 0.6), were captured between 28 nodes (Fig. 5b). All microbial taxa related to the sugar molecules belonged to fungi, suggesting the interaction between sugar molecules and fungal taxa. Modularity analysis revealed that the entire network was divided into seven modules, only three of which were comprised of sugar molecule nodes and more than two OTU nodes (Modules I, II and IV). In general, nodes in one module interconnected more frequently among themselves than with nodes in other modules. However, the nodes of these three modules were mixed, suggesting that certain taxa (e.g., Sporobolomyces) may contribute to multiple sugar molecules at the same time. A previous study has shown that there is a significant positive correlation between Sporobolomyces and sugar compounds (Samaké et al., 2020).
Meanwhile, we found that only three sugar alcohols (mannitol, arabitol and erythritol) used to trace fungal spores were associated with a few fungal genera (Peniophora, Sporobolomyces, Hydnophlebia, Hannaella, Lectera and Cyphellophora) (Fig. 5b), and other sugar molecules had limited links with microbial taxa. The three sugar alcohols had significant positive correlations (p<0.05) with fungal OTU abundances (Table S5), possibly implying the important roles that sugar alcohols play in tracing fungal spores. In contrast, no bacterial taxon was significantly related to sugar molecules. Combined with the results of Mantel's test and network analysis, it is considered that sugar compounds in rural aerosols have a strong association with overall fungal community structure, while bacteria have a weak effect on sugar compounds, possibly due to their limited mass.
In addition, it should be noted that the taxa associated with sugar compounds found in this study were not the same as those found in a survey conducted by Samaké et al. (2020) at a rural site in France, the only study to date investigating the association between microorganisms and sugar compounds. The types and quantities of sugar species produced by microorganisms are affected by microbial species and external environmental conditions such as carbon sources, temperature and humidity (Hrynkiewicz et al., 2010). Here, we performed network construction separately for daytime and nighttime samples. Results showed that the sugar molecules had more associations with microbial taxa at night, particularly fungal taxa (Fig. S7), demonstrating the importance of environmental factors in the relationship between sugar compounds and microbial taxa. More fungal spores could be released at night due to high temperature and high relative humidity (Elbert et al., 2007; Hummel et al., 2015), and the proportions of some fungal genera (e.g., Agaricus) were enhanced at night (Table S3), which was a possible explanation for the greater associations between sugar molecules and microbial taxa at night.
In this study, the airborne microbial taxonomy and the compositions of sugar molecules in atmospheric aerosols collected in rural Gucheng, North China Plain, were obtained. Results confirm that fungal communities have more pronounced diurnal differences than bacteria. Airborne fungi mainly originated from plant leaves, while the sources of airborne bacteria were mostly unresolved and may have been human-related and/or had long-distance transport sources. Among sugar compounds, sucrose dominated in the daytime samples (27.2 ± 12.7 %), while mannitol dominated at night (31.1 ± 5.9 %). From the perspective of the overall microbial community, most sugar molecules were strongly associated with the fungal community structure, while the bacteria community structure was weakly related to sucrose only. At the microbial taxon level, only a few saprophytic fungal taxa were closely associated with sugar compounds. The results suggest that the entire fungal community may largely contribute to sugar compounds, while the bacterial community contributed little to them. The results presented in this study will help to understand the contribution of bioaerosols to organic carbon in atmospheric aerosols and build links between airborne microorganisms and aerosol chemistry. Importantly, it should be noted that the uncertainties in estimating the concentrations and emission fluxes of bioaerosols using certain sugar compounds need to be seriously considered. For example, arabitol, usually used as a fungal spore tracer, showed no significant correlation with fungi in this study. More likely, different seasons, climates and locations may lead to differences in the microbial community structure as well as corresponding sugar composition and metabolites.
Moreover, the airborne microbiota is highly complex, and biomolecules such as carbohydrates, proteins and lipids are major chemical compositions and metabolites of airborne microorganisms. In this study, the measured carbohydrate species are limited to small molecule compounds. In the future, it will be necessary to detect more carbohydrates and other compounds in aerosols from different typical regions using high-resolution mass spectrometry to further study the relationship between microorganisms and atmospheric aerosol chemistry.
The sequence data are available in the NCBI under BioProject numbers PRJNA936567 for bacteria (https://www.ncbi.nlm.nih.gov/bioproject/PRJNA936567/, Tianjin University, last access: 21 November 2023) and PRJNA936562 for fungi (https://www.ncbi.nlm.nih.gov/bioproject/PRJNA936562/, Tianjin University, last access: 21 November 2023).
The supplement related to this article is available online at: https://doi.org/10.5194/bg-20-4915-2023-supplement.
MN analyzed data and prepared all figures. SH and WW conducted the observation. SH, YW, RJ, QZ, ZW and DZ performed experiments. MN and WH wrote the manuscript. WX, LW, JD, FS and PF revised the original manuscript draft. WH and PF supervised the project. All authors were involved in helpful discussions and contributed to the manuscript.
The contact author has declared that none of the authors has any competing interests.
Publisher's note: Copernicus Publications remains neutral with regard to jurisdictional claims made in the text, published maps, institutional affiliations, or any other geographical representation in this paper. While Copernicus Publications makes every effort to include appropriate place names, the final responsibility lies with the authors.
Thanks are given for the help of the staff at the Gucheng Ecological and Agrometeorological Experiment Station, Chinese Academy of Meteorological Sciences, during the observation.
This research has been supported by the National Natural Science Foundation of China (grant nos. 41977183 and 42130513).
This paper was edited by Luo Yu and reviewed by three anonymous referees.
Abdel Hameed, A. A., Khoder, M. I., Yuosra, S., Osman, A. M., and Ghanem, S.: Diurnal distribution of airborne bacteria and fungi in the atmosphere of Helwan area, Egypt, Sci. Total Environ., 407, 6217–6222, https://doi.org/10.1016/j.scitotenv.2009.08.028, 2009.
Abrego, N., Norros, V., Halme, P., Somervuo, P., Ali-Kovero, H., and Ovaskainen, O.: Give me a sample of air and I will tell which species are found from your region: Molecular identification of fungi from airborne spore samples, Mol. Ecol. Resour., 18, 511–524, https://doi.org/10.1111/1755-0998.12755, 2018.
Acuna, J. J., Ruiz-Gil, T., Marileo, L., Carrazana, E., Rilling, J., Campos, M., Correa-Araneda, F., Fujiyoshi, S., and Jorquera, M. A.: Airborne bacterial community associated with fine particulate matter (PM2.5) under different air quality indices in Temuco city, southern Chile, Arch. Microbiol., 204, 148, https://doi.org/10.1007/s00203-021-02740-6, 2022.
Almaguer, M., Aira, M. J., Rodríguez-Rajo, F. J., and Rojas, T. I.: Temporal dynamics of airborne fungi in Havana (Cuba) during dry and rainy seasons: Influence of meteorological parameters, Int. J. Biometeorol., 58, 1459–1470, 2014.
Andreae, M. O. and Merlet, P.: Emission of trace gases and aerosols from biomass burning, Global Biogeochem. Cy., 15, 955–966, https://doi.org/10.1029/2000gb001382, 2001.
Andrews, J. H. and Harris, R. F.: The ecology and biogeography of microorganisms on plant surfaces, Annu. Rev. Phytopathol., 38, 145, https://doi.org/10.1146/annurev.phyto.38.1.145, 2000.
Ariya, P. A., Nepotchatykh, O., Ignatova, O., and Amyot, M.: Microbiological degradation of atmospheric organic compounds, Geophys. Res. Lett., 29, 341–344, https://doi.org/10.1029/2002GL015637, 2002.
Ariya, P. A., Sun, J., Eltouny, N. A., Hudson, E. D., Hayes, C. T., and Kos, G.: Physical and chemical characterization of bioaerosols – Implications for nucleation processes, Int. Rev. Phys. Chem., 28, 1–32, https://doi.org/10.1080/01442350802597438, 2009.
Arzt, J., Juleff, N., Zhang, Z., and Rodriguez, L. L.: The pathogenesis of foot-and-mouth disease I: Viral pathways in cattle, Transbound. Emerg. Dis., 58, 291–304, https://doi.org/10.1111/j.1865-1682.2011.01204.x, 2011.
Bauer, H., Claeys, M., Vermeylen, R., Schueller, E., Weinke, G., Berger, A., and Puxbaum, H.: Arabitol and mannitol as tracers for the quantification of airborne fungal spores, Atmos. Environ., 42, 588–593, https://doi.org/10.1016/j.atmosenv.2007.10.013, 2008a.
Bastian, M., Heymann, S., and Jacomy, M.: Gephi: An Open Source Software for Exploring and Manipulating Networks, Proceedings of the International AAAI Conference on Web and Social Media, 17–20 May 2009, San Jose California USA, 3, 361–362, https://doi.org/10.1609/icwsm.v3i1.13937, 2009.
Bauer, H., Schueller, E., Weinke, G., Berger, A., Hitzenberger, R., Marr, I. L., and Puxbaum, H.: Significant contributions of fungal spores to the organic carbon and to the aerosol mass balance of the urban atmospheric aerosol, Atmos. Environ., 42, 5542–5549, https://doi.org/10.1016/j.atmosenv.2008.03.019, 2008b.
Benjamini, Y., Krieger, A. M., and Yekutieli, D.: Adaptive linear step-up procedures that control the false discovery rate, Biometrika, 93, 491–507, https://doi.org/10.1093/biomet/93.3.491, 2006.
Bowers, R. M., McLetchie, S., Knight, R., and Fierer, N.: Spatial variability in airborne bacterial communities across land-use types and their relationship to the bacterial communities of potential source environments, ISME J., 5, 601–612, https://doi.org/10.1038/ismej.2010.167, 2011.
Chen, D., Hou, H., Zhou, S., Zhang, S., Liu, D., Pang, Z., Hu, J., Xue, K., Du, J., Cui, X., Wang, Y., and Che, R.: Soil diazotrophic abundance, diversity, and community assembly mechanisms significantly differ between glacier riparian wetlands and their adjacent alpine meadows, Front. Microbiol., 13, 1063027, https://doi.org/10.3389/fmicb.2022.1063027, 2022.
Chen, J., Kawamura, K., Liu, C. Q., and Fu, P. Q.: Long-term observations of saccharides in remote marine aerosols from the western North Pacific: A comparison between 1990–1993 and 2006–2009 periods, Atmos. Environ., 67, 448–458, https://doi.org/10.1016/j.atmosenv.2012.11.014, 2013.
Chen, N. T., Tam, L. M., Wu, J. H., Cheong, N. S., Lin, C. Y., Tseng, C. C., and Su, H. J.: Changes in ambient bacterial community in northern Taiwan during long-range transport: Asian dust storm and frontal pollution, Atmosphere, 13, 841, https://doi.org/10.3390/atmos13050841, 2022.
Després, V. R., Alex Huffman, J., Burrows, S. M., Hoose, C., Safatov, A. S., Buryak, G., FröHlich-Nowoisky, J., Elbert, W., Andreae, M. O., and PöSchl, U.: Primary biological aerosol particles in the atmosphere: A review, Tellus B, 64, 15598, https://doi.org/10.3402/tellusb.v64i0.15598, 2012.
Deutscher, J., Francke, C., and Postma, P. W.: How Phosphotransferase System-Related Protein Phosphorylation Regulates Carbohydrate Metabolism in Bacteria, Microbiol. Mol. Biol. Rev., 70, 939–1031, https://doi.org/10.1128/MMBR.00024-06, 2006.
Drautz-Moses, D. I., Luhung, I., Gusareva, E. S., Kee, C., Gaultier, N. E., Premkrishnan, B. N. V., Lee, C. F., Leong, S. T., Park, C., Yap, Z. H., Heinle, C. E., Lau, K. J. X., Purbojati, R. W., Lim, S. B. Y., Lim, Y. H., Kutmutia, S. K., Aung, N. W., Oliveira, E. L., Ng, S. G., Dacanay, J., Ang, P. N., Spence, S. D., Phung, W. J., Wong, A., Kennedy, R. J., Kalsi, N., Sasi, S. P., Chandrasekaran, L., Uchida, A., Junqueira, A. C. M., Kim, H. L., Hankers, R., Feuerle, T., Corsmeier, U., and Schuster, S. C.: Vertical stratification of the air microbiome in the lower troposphere, P. Natl. Acad. Sci. USA, 119, e2117293119, https://doi.org/10.1073/pnas.2117293119, 2022.
Dulermo, T., Rascle, C., Chinnici, G., Gout, E., Bligny, R., and Cotton, P.: Dynamic carbon transfer during pathogenesis of sunflower by the necrotrophic fungus Botrytis cinerea: From plant hexoses to mannitol, New Phytol., 183, 1149–1162, https://doi.org/10.1111/j.1469-8137.2009.02890.x, 2009.
Elbert, W., Taylor, P. E., Andreae, M. O., and Pöschl, U.: Contribution of fungi to primary biogenic aerosols in the atmosphere: wet and dry discharged spores, carbohydrates, and inorganic ions, Atmos. Chem. Phys., 7, 4569–4588, https://doi.org/10.5194/acp-7-4569-2007, 2007.
Fröhlich-Nowoisky, J., Burrows, S. M., Xie, Z., Engling, G., Solomon, P. A., Fraser, M. P., Mayol-Bracero, O. L., Artaxo, P., Begerow, D., Conrad, R., Andreae, M. O., Després, V. R., and Pöschl, U.: Biogeography in the air: fungal diversity over land and oceans, Biogeosciences, 9, 1125–1136, https://doi.org/10.5194/bg-9-1125-2012, 2012.
Fröhlich-Nowoisky, J., Kampf, C. J., Weber, B., Huffman, J. A., Pöhlker, C., Andreae, M. O., Lang-Yona, N., Burrows, S. M., Gunthe, S. S., and Elbert, W.: Bioaerosols in the Earth system: Climate, health, and ecosystem interactions, Atmos. Res., 182, 346–376, 2016.
Fu, P., Kawamura, K., Okuzawa, K., Aggarwal, S. G., Wang, G., Kanaya, Y., and Wang, Z.: Organic molecular compositions and temporal variations of summertime mountain aerosols over Mt. Tai, North China Plain, J. Geophys. Res., 113, D19107, https://doi.org/10.1029/2008jd009900, 2008.
Fu, P., Kawamura, K., Pavuluri, C. M., Swaminathan, T., and Chen, J.: Molecular characterization of urban organic aerosol in tropical India: Contributions of primary emissions and secondary photooxidation, Atmos. Chem. Phys., 10, 2663–2689, https://doi.org/10.5194/acp-10-2663-2010, 2010.
Fu, P., Kawamura, K., Kobayashi, M., and Simoneit, B. R. T.: Seasonal variations of sugars in atmospheric particulate matter from Gosan, Jeju Island: Significant contributions of airborne pollen and Asian dust in spring, Atmos. Environ., 55, 234–239, https://doi.org/10.1016/j.atmosenv.2012.02.061, 2012.
Fu, P., Kawamura, K., Chen, J., Charrière, B., and Sempéré, R.: Organic molecular composition of marine aerosols over the Arctic Ocean in summer: Contributions of primary emission and secondary aerosol formation, Biogeosciences, 10, 653–667, https://doi.org/10.5194/bg-10-653-2013, 2013.
Fu, P., Kawamura, K., Chen, J., and Miyazaki, Y.: Secondary production of organic aerosols from biogenic VOCs over Mt. Fuji, Japan, Environ. Sci. Technol., 48, 8491–8497, https://doi.org/10.1021/es500794d, 2014.
Gat, D., Mazar, Y., Cytryn, E., and Rudich, Y.: Origin-dependent variations in the atmospheric microbiome community in Eastern Mediterranean dust storms, Environ. Sci. Technol., 51, 6709–6718, https://doi.org/10.1021/acs.est.7b00362, 2017.
Gillum, S. J. and Levetin, E.: The air spora close to a compost facility in Northeast Oklahoma: Part I – spore trap sampling, Aerobiologia, 24, 3–12, https://doi.org/10.1007/s10453-007-9074-y, 2008.
Goddijn, O. J. M. and van Dun, K.: Trehalose metabolism in plants, Trends Plant Sci., 4, 315–319, https://doi.org/10.1016/S1360-1385(99)01446-6, 1999.
Gunasekaran, R., Janakiraman, D., Rajapandian, S. G. K., Appavu, S. P., Namperumalsamy Venkatesh, P., and Prajna, L.: Periconia species – An unusual fungal pathogen causing mycotic keratitis, Indian J. Med. Microbiol., 39, 36–40, https://doi.org/10.1016/j.ijmmb.2020.10.006, 2021.
Gusareva, E. S., Acerbi, E., Lau, K. J., Luhung, I., Premkrishnan, B. N., Kolundžija, S., Purbojati, R. W., Wong, A., Houghton, J. N., and Miller, D.: Microbial communities in the tropical air ecosystem follow a precise diel cycle, P. Natl. Acad. Sci. USA, 116, 23299–23308, 2019.
Hicks, C. W., Sweeney, D. A., Cui, X., Li, Y., and Eichacker, P. Q.: An overview of anthrax infection including the recently identified form of disease in injection drug users, Intensive Care Med., 38, 1092–1104, https://doi.org/10.1007/s00134-012-2541-0, 2012.
Hoose, C., Kristjánsson, J. E., and Burrows, S. M.: How important is biological ice nucleation in clouds on a global scale?, Environ. Res. Lett., 5, 024009, https://doi.org/10.1088/1748-9326/5/2/024009, 2010.
Hrynkiewicz, K., Baum, C., and Leinweber, P.: Density, metabolic activity, and identity of cultivable rhizosphere bacteria on Salix viminalis in disturbed arable and landfill soils, J. Plant Nutr. Soil Sci., 173, 747–756, https://doi.org/10.1002/jpln.200900286, 2010.
Hu, W., Wang, Z., Huang, S., Ren, L., Yue, S., Li, P., Xie, Q., Zhao, W., Wei, L., Ren, H., Wu, L., Deng, J., and Fu, P.: Biological aerosol particles in polluted regions, Curr. Pollut. Rep., 6, 65–89, https://doi.org/10.1007/s40726-020-00138-4, 2020.
Huang, S., Hu, W., Chen, J., Wu, Z., Zhang, D., and Fu, P.: Overview of biological ice nucleating particles in the atmosphere, Environ. Int., 146, 106197, https://doi.org/10.1016/j.envint.2020.106197, 2021.
Huffman, J. A., Sinha, B., Garland, R. M., Snee-Pollmann, A., Gunthe, S. S., Artaxo, P., Martin, S. T., Andreae, M. O., and Pöschl, U.: Size distributions and temporal variations of biological aerosol particles in the Amazon rainforest characterized by microscopy and real-time UV-APS fluorescence techniques during AMAZE-08, Atmos. Chem. Phys., 12, 11997–12019, https://doi.org/10.5194/acp-12-11997-2012, 2012.
Huffman, J. A., Prenni, A. J., DeMott, P. J., Pohlker, C., Mason, R. H., Robinson, N. H., Frohlich-Nowoisky, J., Tobo, Y., Despres, V. R., Garcia, E., Gochis, D. J., Harris, E., Mueller-Germann, I., Ruzene, C., Schmer, B., Sinha, B., Day, D. A., Andreae, M. O., Jimenez, J. L., Gallagher, M., Kreidenweis, S. M., Bertram, A. K., and Poschl, U.: High concentrations of biological aerosol particles and ice nuclei during and after rain, Atmos. Chem. Phys., 13, 6151–6164, https://doi.org/10.5194/acp-13-6151-2013, 2013.
Hui, N., Jumpponen, A., Francini, G., Kotze, D. J., Liu, X., Romantschuk, M., Strömmer, R., and Setälä, H.: Soil microbial communities are shaped by vegetation type and park age in cities under cold climate, Environ. Microbiol., 19, 1281–1295, https://doi.org/10.1111/1462-2920.13660, 2017.
Hummel, M., Hoose, C., Gallagher, M., Healy, D. A., Huffman, J. A., O'Connor, D., Pöschl, U., Pöhlker, C., Robinson, N. H., Schnaiter, M., Sodeau, J. R., Stengel, M., Toprak, E., and Vogel, H.: Regional-scale simulations of fungal spore aerosols using an emission parameterization adapted to local measurements of fluorescent biological aerosol particles, Atmos. Chem. Phys., 15, 6127–6146, https://doi.org/10.5194/acp-15-6127-2015, 2015.
Jia, Y. and Fraser, M.: Characterization of saccharides in size-fractionated ambient particulate matter and aerosol sources: The contribution of primary biological aerosol particles (PBAPs) and soil to ambient particulate matter, Environ. Sci. Technol., 45, 930–936, https://doi.org/10.1021/es103104e, 2011.
Jiang, X. Q., Wang, C. H., Guo, J. Y., Hou, J. H., Guo, X., Zhang, H. Y., Tan, J., Li, M., Li, X., and Zhu, H. Q.: Global meta-analysis of airborne bacterial communities and associations with anthropogenic activities, Environ. Sci. Technol., 56, 9891–9902, https://doi.org/10.1021/acs.est.1c07923, 2022.
Jones, A. M. and Harrison, R. M.: The effects of meteorological factors on atmospheric bioaerosol concentrations – A review, Sci. Total Environ., 326, 151–180, 2004.
Kamada, T., Sano, H., Nakazawa, T., and Nakahori, K.: Regulation of fruiting body photomorphogenesis in Coprinopsis cinerea, Fungal Genet. Biol., 47, 917–921, https://doi.org/10.1016/j.fgb.2010.05.003, 2010.
Kang, M., Fu, P., Kawamura, K., Yang, F., Zhang, H., Zang, Z., Ren, H., Ren, L., Zhao, Y., Sun, Y., and Wang, Z.: Characterization of biogenic primary and secondary organic aerosols in the marine atmosphere over the East China Sea, Atmos. Chem. Phys., 18, 13947–13967, https://doi.org/10.5194/acp-18-13947-2018, 2018.
Kaur, S. and Suseela, V.: Unraveling Arbuscular Mycorrhiza-Induced Changes in Plant Primary and Secondary Metabolome, Metabolites, 10, 335, https://doi.org/10.3390/metabo10080335, 2020.
Knights, D., Kuczynski, J., Charlson, E. S., Zaneveld, J., Mozer, M. C., Collman, R. G., Bushman, F. D., Knight, R., and Kelley, S. T.: Bayesian community-wide culture-independent microbial source tracking, Nat. Method., 8, 761–763, https://doi.org/10.1038/nmeth.1650, 2011.
Kramer, C. L.: Production, release and dispersal of basidiospores, in: Decomposer basidiomycetes: Their biology and ecology, edited by: Frankland, J. C., et al., Cambridge University Press, 33–49, 1982.
Li, J., Wang, G., Zhou, B., Cheng, C., Cao, J., Shen, Z., and An, Z.: Airborne particulate organics at the summit (2060 m, a.s.l.) of Mt. Hua in central China during winter: Implications for biofuel and coal combustion, Atmos. Res., 106, 108–119, https://doi.org/10.1016/j.atmosres.2011.11.012, 2012.
Liu, H., Hu, Z., Zhou, M., Hu, J., Yao, X., Zhang, H., Li, Z., Lou, L., Xi, C., Qian, H., Li, C., Xu, X., Zheng, P., and Hu, B.: The distribution variance of airborne microorganisms in urban and rural environments, Environ. Pollut., 247, 898–906, https://doi.org/10.1016/j.envpol.2019.01.090, 2019.
Liu, H., Hu, Z., Zhou, M., Zhang, H., Li, Z., Zhang, H., Hu, J., Yao, X., Lou, L., and Xi, C.: Airborne microorganisms exacerbate the formation of atmospheric ammonium and sulfate, Environ. Pollut., 263, 114293, https://doi.org/10.1016/j.envpol.2020.114293, 2020.
Lladó, S., López-Mondéjar, R., and Baldrian, P.: Forest soil bacteria: Diversity, involvement in ecosystem processes, and response to global change, Microbiol. Mol. Biol. Rev., 81, 1–27, https://doi.org/10.1128/MMBR.00063-16, 2017.
Long, T., Ye, Z., Tang, Y., Shi, J., Wen, J., Chen, C., and Huo, Q.: Comparison of bacterial community structure in PM2.5 during hazy and non-hazy periods in Guilin, South China, Aerobiologia, 39, 87–103, https://doi.org/10.1007/s10453-022-09777-0, 2022.
Mace, K. A., Kubilay, N., and Duce, R. A.: Organic nitrogen in rain and aerosol in the eastern Mediterranean atmosphere: An association with atmospheric dust, J. Geophys. Res.-Atmos., 108, 4320, https://doi.org/10.1029/2002JD002997, 2003.
Martiny, J. B. H., Bohannan, B. J. M., Brown, J. H., Colwell, R. K., Fuhrman, J. A., Green, J. L., Horner-Devine, M. C., Kane, M., Krumins, J. A., Kuske, C. R., Morin, P. J., Naeem, S., Øvreås, L., Reysenbach, A.-L., Smith, V. H., and Staley, J. T.: Microbial biogeography: putting microorganisms on the map, Nat. Rev. Microbiol., 4, 102–112, https://doi.org/10.1038/nrmicro1341, 2006.
Marynowski, L., Goryl, M., Bucha, M., Smolarek-Lach, J., Detman, A., Sikora, A., Chojnacka, A., and Simoneit, B. R. T.: Trehalose, mannitol and arabitol as indicators of fungal metabolism in Late Cretaceous and Miocene deposits, Int. J. Coal Geol., 201, 51–61, https://doi.org/10.1016/j.coal.2018.11.003, 2019.
Medeiros, P. M., Conte, M. H., Weber, J. C., and Simoneit, B. R. T.: Sugars as source indicators of biogenic organic carbon in aerosols collected above the Howland Experimental Forest, Maine, Atmos. Environ., 40, 1694–1705, https://doi.org/10.1016/j.atmosenv.2005.11.001, 2006a.
Mazar, Y., Cytryn, E., Erel, Y., and Rudich, Y.: Effect of dust storms on the atmospheric microbiome in the Eastern Mediterranean, Environ. Sci. Technol., 50, 4194–4202, https://doi.org/10.1021/acs.est.5b06348, 2016.
Medeiros, P. M., Fernandes, M. F., Dick, R. P., and Simoneit, B. R. T.: Seasonal variations in sugar contents and microbial community in a ryegrass soil, Chemosphere, 65, 832–839, https://doi.org/10.1016/j.chemosphere.2006.03.025, 2006b.
Monedero, V., Pérez-Martínez, G., and Yebra, M. J.: Perspectives of engineering lactic acid bacteria for biotechnological polyol production, Appl. Microbiol. Biotechnol., 86, 1003–1015, https://doi.org/10.1007/s00253-010-2494-6, 2010.
Nagy, L. G., Hazi, J., Szappanos, B., Kocsubé, S., Bálint, B., Rakhely, G., Vágvölgyi, C., and Papp, T.: The evolution of defense mechanisms correlate with the explosive diversification of autodigesting Coprinellus mushrooms (Agaricales, Fungi), Syst. Biol., 61, 595–607, 2012.
Ning, D., Yuan, M., Wu, L., Zhang, Y., Guo, X., Zhou, X., Yang, Y., Arkin, A. P., Firestone, M. K., and Zhou, J.: A quantitative framework reveals ecological drivers of grassland microbial community assembly in response to warming, Nat. Commun., 11, 4717, https://doi.org/10.1038/s41467-020-18560-z, 2020.
Niu, M. T., Hu, W., Cheng, B. R., Wu, L. B., Ren, L. J., Deng, J. J., Shen, F. X., and Fu, P. Q.: Influence of rainfall on fungal aerobiota in the urban atmosphere over Tianjin, China: A case study, Atmos. Environ., 12, 100137, https://doi.org/10.1016/j.aeaoa.2021.100137, 2021.
Núñez, A., García, A. M., Moreno, D. A., and Guantes, R.: Seasonal changes dominate long-term variability of the urban air microbiome across space and time, Environ. Int., 150, 106423, https://doi.org/10.1016/j.envint.2021.106423, 2021.
Oduber, F., Calvo, A. I., Castro, A., Alves, C., Blanco-Alegre, C., Fernández-González, D., Barata, J., Calzolai, G., Nava, S., Lucarelli, F., Nunes, T., Rodríguez, A., Vega-Maray, A. M., Valencia-Barrera, R. M., and Fraile, R.: One-year study of airborne sugar compounds: Cross-interpretation with other chemical species and meteorological conditions, Atmos. Res., 251, 105417, https://doi.org/10.1016/j.atmosres.2020.105417, 2021.
Oliveira, M., Ribeiro, H., Delgado, J. L., and Abreu, I.: Seasonal and intradiurnal variation of allergenic fungal spores in urban and rural areas of the North of Portugal, Aerobiologia, 25, 85–98, https://doi.org/10.1007/s10453-009-9112-z, 2009.
Pickersgill, D. A., Wehking, J., Paulsen, H., Thines, E., Pöschl, U., Fröhlich-Nowoisky, J., and Després, V. R.: Lifestyle dependent occurrence of airborne fungi, Biogeosciences Discuss. [preprint], https://doi.org/10.5194/bg-2017-452, 2017.
Pöschl, U., Martin, S. T., Sinha, B., Chen, Q., Gunthe, S. S., Huffman, J. A., Borrmann, S., Farmer, D. K., Garland, R. M., Helas, G., Jimenez, J. L., King, S. M., Manzi, A., Mikhailov, E., Pauliquevis, T., Petters, M. D., Prenni, A. J., Roldin, P., Rose, D., Schneider, J., Su, H., Zorn, S. R., Artaxo, P., and Andreae, M. O.: Rainforest Aerosols as Biogenic Nuclei of Clouds and Precipitation in the Amazon, Science, 329, 1513–1516, https://doi.org/10.1126/science.1191056, 2010.
Prass, M., Andreae, M. O., de Araùjo, A. C., Artaxo, P., Ditas, F., Elbert, W., Förster, J. D., Franco, M. A., Hrabe de Angelis, I., Kesselmeier, J., Klimach, T., Kremper, L. A., Thines, E., Walter, D., Weber, J., Weber, B., Fuchs, B. M., Pöschl, U., and Pöhlker, C.: Bioaerosols in the Amazon rain forest: Temporal variations and vertical profiles of eukarya, bacteria, and archaea, Biogeosciences, 18, 4873–4887, https://doi.org/10.5194/bg-18-4873-2021, 2021.
Prosen, E. M., Radlein, D., Piskorz, J., Scott, D. S., and Legge, R. L.: Microbial utilization of levoglucosan in wood pyrolysate as a carbon and energy source, Biotechnol. Bioeng., 42, 538–541, 1993.
Puxbaum, H. and Tenze-Kunit, M.: Size distribution and seasonal variation of atmospheric cellulose, Atmos. Environ., 37, 3693–3699, https://doi.org/10.1016/S1352-2310(03)00451-5, 2003.
Puxbaum, H., Caseiro, A., Sánchez-Ochoa, A., Kasper-Giebl, A., Claeys, M., Gelencsér, A., Legrand, M., Preunkert, S., and Pio, C.: Levoglucosan levels at background sites in Europe for assessing the impact of biomass combustion on the European aerosol background, J. Geophys. Res.-Atmos., 112, D23S05, https://doi.org/10.1029/2006JD008114, 2007.
Qi, Y., Li, Y., Xie, W., Lu, R., Mu, F., Bai, W., and Du, S.: Temporal-spatial variations of fungal composition in PM2.5 and source tracking of airborne fungi in mountainous and urban regions, Sci. Total Environ., 708, 135027, https://doi.org/10.1016/j.scitotenv.2019.135027, 2020.
Regnat, K., Mach, R., and Mach-Aigner, A.: Erythritol as sweetener – Wherefrom and whereto?, Appl. Microbiol. Biotechnol., 102, 587–595, 2018.
Ren, H., Kang, M., Ren, L., Zhao, Y., Pan, X., Yue, S., Li, L., Zhao, W., Wei, L., Xie, Q., Li, J., Wang, Z., Sun, Y., Kawamura, K., and Fu, P.: The organic molecular composition, diurnal variation, and stable carbon isotope ratios of PM2.5 in Beijing during the 2014 APEC summit, Environ. Pollut., 243, 919–928, https://doi.org/10.1016/j.envpol.2018.08.094, 2018.
Reyes, E. S., de la Cruz, D. R., and Sánchez, J. S.: First fungal spore calendar of the middle-west of the Iberian Peninsula, Aerobiologia, 32, 529–539, https://doi.org/10.1007/s10453-016-9430-x, 2016.
Rogge, W. F., Medeiros, P. M., and Simoneit, B. R. T.: Organic marker compounds in surface soils of crop fields from the San Joaquin Valley fugitive dust characterization study, Atmos. Environ., 41, 8183–8204, https://doi.org/10.1016/j.atmosenv.2007.06.030, 2007.
Samaké, A., Bonin, A., Jaffrezo, J. L., Taberlet, P., Weber, S., Uzu, G., Jacob, V., Conil, S., and Martins, J. M. F.: High levels of primary biogenic organic aerosols are driven by only a few plant-associated microbial taxa, Atmos. Chem. Phys., 20, 5609–5628, https://doi.org/10.5194/acp-20-5609-2020, 2020.
Santl-Temkiv, T., Sikoparija, B., Maki, T., Carotenuto, F., Amato, P., Yao, M. S., Morris, C. E., Schnell, R., Jaenicke, R., Pohlker, C., DeMott, P. J., Hill, T. C. J., and Huffman, J. A.: Bioaerosol field measurements: Challenges and perspectives in outdoor studies, Aerosol Sci. Technol., 54, 520–546, https://doi.org/10.1080/02786826.2019.1676395, 2020.
Savage, D., Barbetti, M. J., Macleod, W. J., Salam, M. U., and Renton, M.: Mobile traps are better than stationary traps for surveillance of airborne fungal spores, Crop Protect., 36, 23–30, https://doi.org/10.1016/j.cropro.2012.01.015, 2012.
Schliemann, W., Ammer, C., and Strack, D.: Metabolite profiling of mycorrhizal roots of Medicago truncatula, Phytochemistry, 69, 112–146, https://doi.org/10.1016/j.phytochem.2007.06.032, 2008.
Schumacher, C. J., Pöhlker, C., Aalto, P., Hiltunen, V., Petäjä, T., Kulmala, M., Pöschl, U., and Huffman, J. A.: Seasonal cycles of fluorescent biological aerosol particles in boreal and semi-arid forests of Finland and Colorado, Atmos. Chem. Phys., 13, 11987–12001, https://doi.org/10.5194/acp-13-11987-2013, 2013.
Shen, F., Zheng, Y., Niu, M., Zhou, F., Wu, Y., Wang, J., Zhu, T., Wu, Y., Wu, Z., and Hu, M.: Characteristics of biological particulate matters at urban and rural sites in the North China Plain, Environ. Pollut., 253, 569–577, 2019.
Shen, F. and Yao, M.: Bioaerosol nexus of air quality, climate system and human health, National Science Open, 2, 20220050, https://doi.org/10.1360/nso/20220050, 2022.
Simoneit, B. R. T., Schauer, J. J., Nolte, C. G., Oros, D. R., Elias, V. O., Fraser, M. P., Rogge, W. F., and Cass, G. R.: Levoglucosan, a tracer for cellulose in biomass burning and atmospheric particles, Atmos. Environ., 33, 173–182, https://doi.org/10.1016/S1352-2310(98)00145-9, 1999.
Simoneit, B. R. T.: Biomass burning – A review of organic tracers for smoke from incomplete combustion, Appl. Geochem., 17, 129–162, https://doi.org/10.1016/S0883-2927(01)00061-0, 2002.
Singh, H. W., Wade, R. M., and Sherwood, A. R.: Diurnal patterns of airborne algae in the Hawaiian Islands: A preliminary study, Aerobiologia, 34, 363–373, https://doi.org/10.1007/s10453-018-9519-5, 2018.
Solomon, P. S., Waters, O. D., and Oliver, R. P.: Decoding the mannitol enigma in filamentous fungi, Trends Microbiol., 15, 257–262, https://doi.org/10.1016/j.tim.2007.04.002, 2007.
Steven, B., Chen, M. Q., Greer, C. W., Whyte, L. G., and Niederberger, T. D.: Tumebacillus permanentifrigoris gen. nov., sp. nov., an aerobic, spore-forming bacterium isolated from Canadian high Arctic permafrost, Int. J. Syst. Evol. Microbiol., 58, 1497–1501, https://doi.org/10.1099/ijs.0.65101-0, 2008.
Sullivan, A. P., Frank, N., Kenski, D. M., and Collett Jr., J. L.: Application of high-performance anion-exchange chromatography–pulsed amperometric detection for measuring carbohydrates in routine daily filter samples collected by a national network: 2. Examination of sugar alcohols/polyols, sugars, and anhydrosugars in the upper Midwest, J. Geophys. Res.-Atmos., 116, D08303, https://doi.org/10.1029/2010JD014169, 2011.
Taipale, D., Kerminen, V. M., Ehn, M., Kulmala, M., and Niinemets, Ü.: Modelling the influence of biotic plant stress on atmospheric aerosol particle processes throughout a growing season, Atmos. Chem. Phys., 21, 17389–17431, https://doi.org/10.5194/acp-21-17389-2021, 2021.
Upadhyay, R., Meena, M., Prasad, V., Zehra, A., and Gupta, V.: Mannitol metabolism during pathogenic fungal–host interactions under stressed conditions, Front. Microbiol., 6, 1019, https://doi.org/10.3389/fmicb.2015.01019, 2015.
Van der Nest, M. A., Steenkamp, E. T., McTaggart, A. R., Trollip, C., Godlonton, T., Sauerman, E., Roodt, D., Naidoo, K., Coetzee, M. P. A., and Wilken, P. M.: Saprophytic and pathogenic fungi in the Ceratocystidaceae differ in their ability to metabolize plant-derived sucrose, BMC Evol. Biol., 15, 1–20, 2015.
Verma, S. K., Kawamura, K., Yang, F., Fu, P., Kanaya, Y., and Wang, Z.: Measurement report: Diurnal and temporal variations of sugar compounds in suburban aerosols from the northern vicinity of Beijing, China-an influence of biogenic and anthropogenic sources, Atmos. Chem. Phys., 21, 4959–4978, https://doi.org/10.5194/acp-21-4959-2021, 2021.
Walmsley, A. R., Barrett, M. P., Bringaud, F., and Gould, G. W.: Sugar transporters from bacteria, parasites and mammals: Structure–activity relationships, Trends Biochem. Sci., 23, 476–481, https://doi.org/10.1016/S0968-0004(98)01326-7, 1998.
Wang, F., Ouyang, D., Zhou, Z., Page, S. J., Liu, D., and Zhao, X.: Lignocellulosic biomass as sustainable feedstock and materials for power generation and energy storage, J. Energy Chem., 57, 247–280, https://doi.org/10.1016/j.jechem.2020.08.060, 2021.
Wang, Y., Qi, J., Han, C., Zhang, T., and Zhang, D.: Microbial characteristics of culturable fungi and bacteria in aerosol particles of a coastal region, Aerobiologia, 36, 507–525, https://doi.org/10.1007/s10453-020-09648-6, 2020.
Wemheuer, F., Kaiser, K., Karlovsky, P., Daniel, R., Vidal, S., and Wemheuer, B.: Bacterial endophyte communities of three agricultural important grass species differ in their response towards management regimes, Sci. Rep., 7, 40914, https://doi.org/10.1038/srep40914, 2017.
Womack, A. M., Bohannan, B. J., and Green, J. L.: Biodiversity and biogeography of the atmosphere, Philos. T. R. Soc. Lond. B, 365, 3645–3653, https://doi.org/10.1098/rstb.2010.0283, 2010.
Wu, X., Cao, F., Haque, M., Fan, M.-Y., Zhang, S.-C., and Zhang, Y.-L.: Molecular composition and source apportionment of fine organic aerosols in Northeast China, Atmos. Environ., 239, 117722, https://doi.org/10.1016/j.atmosenv.2020.117722, 2020.
Xu, A. L., Song, Z. W., Lang, X. L., Chen, X., and Xia, Y.: Seasonal variability in bacterial and fungal diversity and community composition of the near-surface atmosphere in coastal megacity, Aerobiologia, 33, 555–575, https://doi.org/10.1007/s10453-017-9489-z, 2017.
Xu, C., Chen, J., Wang, Z., Chen, H., Feng, H., Wang, L., Xie, Y., Wang, Z., Ye, X., Kan, H., Zhao, Z., and Mellouki, A.: Diverse bacterial populations of PM2.5 in urban and suburb Shanghai, China, Front. Env. Sci. Eng., 15, 37, https://doi.org/10.1007/s11783-020-1329-7, 2021.
Yan, C., Sullivan, A. P., Cheng, Y., Zheng, M., Zhang, Y., Zhu, T., and Collett, J. L.: Characterization of saccharides and associated usage in determining biogenic and biomass burning aerosols in atmospheric fine particulate matter in the North China Plain, Sci. Total Environ., 650, 2939–2950, https://doi.org/10.1016/j.scitotenv.2018.09.325, 2019.
Yan, D., Zhang, T., Su, J., Zhao, L., Wang, H., Fang, X., Zhang, Y., Liu, H., and Yu, L.: Structural variation in the bacterial community associated with airborne particulate matter in Beijing, China, during hazy and nonhazy days, Appl. Environ. Microbiol., 84, e00004–18, https://doi.org/10.1128/AEM.00004-18, 2018.
Yang, L. B., Dai, X. M., Zheng, Z. Y., Zhu, L., Zhan, X. B., and Lin, C. C.: Proteomic analysis of erythritol-producing Yarrowia lipolytica from glycerol in response to osmotic pressure, J. Microbiol. Biotechnol., 25, 1056–1069, https://doi.org/10.4014/jmb.1412.12026, 2015.
Yao, M.: Bioaerosol: A bridge and opportunity for many scientific research fields, J. Aerosol Sci., 119, 91–96, https://doi.org/10.1016/j.jaerosci.2018.01.009, 2018.
Yi, Y., Meng, J., Hou, Z., Wang, G., Zhou, R., Li, Z., Li, Y., Chen, M., Liu, X., Li, H., and Yan, L.: Contrasting compositions and sources of organic aerosol markers in summertime PM2.5 from urban and mountainous regions in the North China Plain, Sci. Total Environ., 766, 144187, https://doi.org/10.1016/j.scitotenv.2020.144187, 2021.
Yue, S., Ren, H., Fan, S., Sun, Y., Wang, Z., and Fu, P.: Springtime precipitation effects on the abundance of fluorescent biological aerosol particles and HULIS in Beijing, Sci. Rep., 6, 29618, https://doi.org/10.1038/srep29618, 2016.
Zancan, P. and Sola-Penna, M.: Trehalose and glycerol stabilize and renature yeast inorganic pyrophosphatase inactivated by very high temperatures, Arch. Biochem. Biophys., 444, 52–60, https://doi.org/10.1016/j.abb.2005.09.014, 2005.
Zhai, Y., Li, X., Wang, T., Wang, B., Li, C., and Zeng, G.: A review on airborne microorganisms in particulate matters: Composition, characteristics and influence factors, Environ. Int., 113, 74–90, https://doi.org/10.1016/j.envint.2018.01.007, 2018.
Zhang, D., Murata, K., Hu, W., Yuan, H., Li, W., Matsusaki, H., and Kakikawa, M.: Concentration and Viability of Bacterial Aerosols Associated with Weather in Asian Continental Outflow: Current Understanding, Aerosol. Sci. Eng., 1, 66–77, https://doi.org/10.1007/s41810-017-0008-y, 2017.
Zhao, J., Jin, L., Wu, D., Xie, J., Li, J., Fu, X., Cong, Z., Fu, P., Zhang, Y., Luo, X., Feng, X., Zhang, G., Tiedje, J. M., and Li, X.: Global airborne bacterial community – Interactions with Earth's microbiomes and anthropogenic activities, P. Natl. Acad. Sci. USA, 119, e2204465119, https://doi.org/10.1073/pnas.2204465119, 2022.
Zhao, Y., Ren, H., Deng, J., Li, L., Hu, W., Ren, L., Yue, S., Fan, Y., Wu, L., Li, J., Sun, Y., Wang, Z., Akimoto, H., Zeng, X., Cheng, Y., Kong, S., Su, H., Cheng, Y., Kawamura, K., and Fu, P.: High daytime abundance of primary organic aerosols over Mt. Emei, Southwest China in summer, Sci. Total Environ., 703, 134475, https://doi.org/10.1016/j.scitotenv.2019.134475, 2020.
Zhu, C., Kawamura, K., and Kunwar, B.: Organic tracers of primary biological aerosol particles at subtropical Okinawa Island in the western North Pacific Rim, J. Geophys. Res.-Atmos., 120, 5504–5523, https://doi.org/10.1002/2015JD023611, 2015.
Zhu, C., Kawamura, K., Fukuda, Y., Mochida, M., and Iwamoto, Y.: Fungal spores overwhelm biogenic organic aerosols in a midlatitudinal forest, Atmos. Chem. Phys., 16, 7497–7506, https://doi.org/10.5194/acp-16-7497-2016, 2016.