the Creative Commons Attribution 4.0 License.
the Creative Commons Attribution 4.0 License.
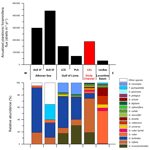
Planktonic foraminifera assemblage composition and flux dynamics inferred from an annual sediment trap record in the central Mediterranean Sea
Thibauld M. Béjard
Andrés S. Rigual-Hernández
Javier P. Tarruella
José-Abel Flores
Anna Sanchez-Vidal
Irene Llamas-Cano
Francisco J. Sierro
The Sicily Channel, located in the central Mediterranean Sea, represents a key point for the regional oceanographic circulation, as it is regarded as the sill that separates the western and eastern basins. Therefore, it is regarded as a unique zone in the well-documented west-to-east Mediterranean productivity gradient. Here we present a time series of settling planktonic foraminifera assemblages from November 2013 to October 2014. Altogether, 19 samples from the sediment trap C01 deployed at a water depth of around 400 m have been used. More than 3700 individuals and 15 different species have been identified. Globorotalia inflata, Globorotalia truncatulinoides, Globigerina bulloides, Globigerinoides ruber, and Globigerinoides ruber (pink) were the five main species identified, accounting for more than 85 % of the total foraminifera.
The total planktonic foraminifera flux mean value was 630 shells m−2 d−1, with a minimum value of 45 shells m−2 d−1 displayed during late autumn 2013 and a maximum of 1890 shells m−2 d−1 reached during spring 2014. This is likely due to the regional oceanographic configuration and the marked seasonality in the surface circulation. During spring and winter, Atlantic Water (AW) dominates the surface circulation, bringing cool and nutrient-enriched waters. This results in a planktonic foraminifera flux increase and a dominance of western basin taxa. During summer and autumn, the circulation is dominated by the eastern warm and oligotrophic Levantine water, which leads to a planktonic foraminifera flux decrease and the dominance of eastern basin species. Our comparison with satellite-derived sea surface temperatures (SSTs) and chlorophyll a data showed that G. inflata was associated with cool and nutrient-rich conditions, while both G. ruber morphotypes were associated with warm and oligotrophic conditions. However, no trends were identified for G. truncatulinoides or G. bulloides. As the latter species flux increased coincidently with that of benthic foraminifera, we considered that this species might have a resuspended origin.
The comparison of the Sicily Channel data with other Mediterranean time series indicates that the annualized planktonic foraminifera flux was lower than in the westernmost Alboran Sea but higher than in the easternmost Levantine Basin. The Sicily Channel species diversity was the highest among the compared zones, highlighting the influence of the different basins and its transitional aspect from a planktonic foraminifera population perspective.
Finally, we compared the settling planktonic foraminifera assemblage with the assemblages from seabed sediment located in the vicinity of the Sicily Channel. The differences in the seabed populations varied according to the sites studied. The deep-dwelling species dominated the settling assemblage samples, while eutrophic and oligotrophic species were more abundant in the sediment. Finally, a high-resolution chronology comparison allowed us to show that this planktonic foraminifera population shift likely developed during the late Holocene prior to the industrial period; however, its causes remain uncertain.
- Article
(6200 KB) - Full-text XML
-
Supplement
(1656 KB) - BibTeX
- EndNote
Planktonic foraminifera are a group of marine calcareous single-celled protozoans with a cosmopolitan distribution. Around 50 morphospecies of planktonic foraminifera have been described in today's oceans (Schiebel and Hemleben, 2017), and, although most of those species are surface dwellers, some species can be found in waters below 2000 m (Schiebel and Hemleben, 2005). Their abundance and distribution are affected by a wide array of factors, such as temperature, salinity, chlorophyll a, and nutrient concentrations, among others (Hemleben et al., 1989; Schiebel and Hemleben, 2005). According to Schiebel (2002), the production and export of their calcareous shells account for 23 % to 56 % of the open marine CaCO3 flux, thereby playing a key role in the marine carbon cycle. Moreover, the high preservation potential of their shells makes them one of the most used groups for multi-proxy studies. Numerous palaeoclimatic (e.g. Barker and Elderfield, 2002; Lirer et al., 2014; Margaritelli et al., 2020; Sierro et al., 2005) and palaeoceanographic (Cisneros et al., 2016; Ducassou et al., 2018; Margaritelli et al., 2022; Toucanne et al., 2007) reconstructions have used planktonic foraminifera as a proxy. In addition, their capacity to reflect the water column's chemical properties has propelled studies that have focused on the impact of recent climate and environmental variability on the water column in different parts of the ocean (e.g. Azibeiro et al., 2023; Beer et al., 2010; Bijma et al., 2002; Chapman, 2010; Cherniovsky et al., 2018; Marshall et al., 2013; Osborne et al., 2016). As marine calcifying organisms, they are regarded as particularly vulnerable to the ongoing ocean warming and acidification (Bijma et al., 2002; Fox et al., 2020). Shell calcification of several foraminifera species has been shown to decrease in response to ocean acidification; therefore, changes in the weight of their shells are regarded as an indicator of the ocean acidification impact on different timescales (Béjard et al., 2023; de Moel et al., 2009; Fox et al., 2020; Kroeker et al., 2013; Moy et al., 2009; Pallacks et al., 2023). In contrast, ocean warming has been proposed to produce an opposite effect on foraminifera calcification, as some studies have documented that an increase in water temperature results in larger shells and enhanced growth rates (Lombard et al., 2011, 2009; Schmidt et al., 2006).
Despite the wide array of studies focused on planktonic foraminifera ecology and distribution, several aspects of their ecology remain uncertain, such as their ecological tolerance limits (Mallo et al., 2017), their geographical and temporal distributions, and their contribution to marine biogeochemical cycles (Jonkers and Kučera, 2015). As major contributors to the pelagic calcite production (Schiebel, 2002), understanding their life cycle on different timescales is essential for constraining the role they play in the marine carbon cycle and the impact of environmental change on these organisms. In this regard, sediment traps represent a powerful tool to improve our knowledge of planktonic foraminifera ecology and their impact on biogeochemical cycles, as they allow the monitoring of foraminifera shell fluxes for extended periods, thereby allowing us to document their seasonal and interannual variability and estimate their contribution to annual budgets of carbonate export to the seafloor (Jonkers et al., 2019).
The Mediterranean Sea is a semi-enclosed sea often regarded as a “miniature ocean” (Bethoux et al., 1999) from an oceanographic point of view or a “laboratory basin” (Bergamasco and Malanotte-Rizzoli, 2010) for studying processes occurring on a global scale. In addition, it is supersaturated regarding calcite (Álvarez et al., 2014), a key aspect in foraminifera studies, as this parameter favours shell preservation and represents one of the main environmental controls on planktonic foraminifera abundance and calcification (Aldridge et al., 2012; Marshall et al., 2013; Osborne et al., 2016). These features make it an interesting zone of the global ocean to study the life cycle and seasonal response to changing environmental conditions of calcifying plankton. The Sicily Channel in the central Mediterranean is the sill that divides the Mediterranean into its western and eastern basins. It is a choke point for the regional surface and deep-water circulation (Malanotte-Rizzoli et al., 2014; Pinardi et al., 2015) and a transition region regarding the well-known west-to-east oligotrophy gradient, functioning as a “biological corridor” (Siokou-Frangou et al., 2010) known in the Mediterranean (Navarro et al., 2017).
Despite these characteristics, time series that focus on planktonic foraminifera in the Mediterranean Sea are scarce. So far, the best-monitored regions are the Alboran Sea (Bárcena et al., 2004; Hernández-Almeida et al., 2011), the Gulf of Lions (Rigual-Hernández et al., 2012), and, more recently, the Levantine Basin (Avnaim-Katav et al., 2020). The latter studies showed that planktonic foraminifera followed a unimodal distribution with maximum shell export occurring during the months of April–May, February–March, and February, respectively, which agreed with the local hydrographic conditions. However, the central Mediterranean remains understudied and poorly documented regarding both continuous time series and planktonic foraminifera dynamics.
Therefore, this work aims to provide new planktonic foraminifera data from a sediment trap mooring line located in the Sicily Channel to improve the current knowledge about their community composition and seasonal patterns in the central Mediterranean. For that purpose,we document the magnitude and composition of planktonic foraminifera fluxes identified in the > 150 µm fraction (i.e. the most commonly used size fraction for studying planktonic foraminifera distribution) from November 2013 to October 2014. We compare our planktonic foraminifera data with a suite of environmental parameters to assess the main environmental drivers that control the seasonal variations in the composition and abundance of the sinking planktonic foraminifera assemblages. To provide further insight on a regional and global scale of the planktonic foraminifera association and fluxes identified here, we compare our data with other time series from the Mediterranean, the Atlantic Ocean, and other regions of the world's oceans. Lastly, we compare the assemblages collected by the sediment with seabed sediment located in the vicinity of the Sicily Channel to document the potential shift in recent planktonic foraminifera populations.
The Mediterranean is an elongated, semi-enclosed sea with an anti-estuarine circulation. It is regarded as a concentration basin (Bethoux et al., 1999) in which the evaporation exceeds the freshwater inputs, forcing a negative hydrological balance (Robinson and Golnaraghi, 1994). This negative balance is compensated for by the entrance of surface oceanic water from the Atlantic Ocean through the Strait of Gibraltar. The colder and nutrient-richer Atlantic Water (AW) spreads eastward into the Mediterranean Basin (Millot, 1991; Pinardi et al., 2015), where it progressively becomes warmer, saltier, and more oligotrophic as it mixes with resident waters (Modified Atlantic Water (MAW), also known as Atlantic Water (AW)). MAW circulates following a cyclonic circuit along the Algerian coast (Algerian Current (AC)) (Malanotte-Rizzoli et al., 2014; Millot, 1999) and divides into two main branches at the entrance of the Sicily Channel (Fig. 1a). One of these branches spreads into the northwestern part of the basin, into the Tyrrhenian Sea, where it continues its path cyclonically. The second branch flows south of Sicily into the Ionian Sea (Lermusiaux and Robinson, 2001). In the Sicily Channel itself, the water masses are split again into two different streams (Béranger et al., 2004): (i) the Atlantic Tunisian Current (ATC) that flows to the southeast following the African coast and (ii) the Atlantic Ionian Stream (AIS) that flows into the deep eastern part of the basin (Fig. 1b) and contributes to MAW transport in the eastern Mediterranean (Jouini et al., 2016; Lermusiaux and Robinson, 2001).
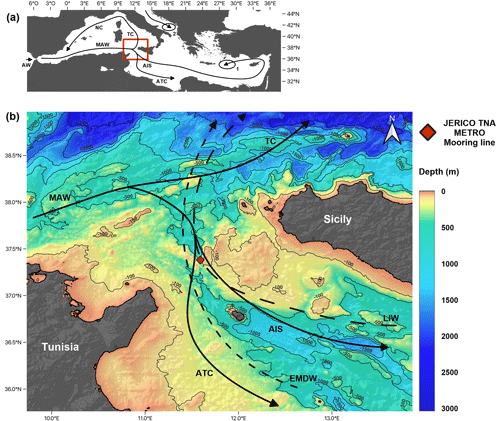
Figure 1(a) Mediterranean Sea general surface circulation (Astraldi et al., 2002; Béranger et al., 2004; Incarbona et al., 2011; Macias et al., 2019) and location of the study zone. The ellipses show the deep-water formation zones for the Levantine Intermediate Water (LIW) (1) and the Eastern Mediterranean Deep Water (EMDW) (2). (b) Regional oceanographic and geographic setting of the Sicily Channel. The red diamond represents the location of the JERICO TNA METRO C01 mooring line. Continuous black lines represent the surface circulation dominated by the Atlantic Ionian Stream (AIS) and the Atlantic Tunisian Current (ATC), while dashed lines show deep-water circulation influences by the LIW and the EMDW. The difference in spacing between the dashed lines stands for the occasional aspect of the EMDW. The topographic model was downloaded from the GEBCO database.
The Sicily Channel is located in the central Mediterranean (Fig. 1a) and acts as a sill that topographically separates the western and eastern Mediterranean Basin. The circulation through the Sicily Channel is characterized by water masses that flow in opposite directions at different depths of the water column (Béranger et al., 2004; Garcia-Solsona et al., 2020; Pinardi et al., 2015; Schroeder et al., 2010, 2017). The Levantine Intermediate Water (LIW), which enters the Channel from the Ionian Sea, occupies the deeper part of the water column along with occasional thin Eastern Mediterranean Deep Water (EMDW) layers (Gasparini et al., 2005; Lermusiaux and Robinson, 2001). The Ionian Water (IW) can be present at intermediate depths (Fig. 1), while the MAW covers the surface to subsurface part of the water column (Garcia-Solsona et al., 2020; Warn-Varnas et al., 1999). Temperature and salinity range from 15–17 °C and 37.2–37.8 psu for the MAW, 15–16.5 °C and 37.8–38.4 psu for the IW, and 13.7–13.9 °C and 38.7–38.8 psu for the LIW (Astraldi et al., 2002; Bouzinac et al., 1999; Robinson et al., 1999). Lastly, it is important to note that the surface circulation in the Sicily Channel presents a large seasonal variability concerning the water mass distribution (Béranger et al., 2004; Lermusiaux and Robinson, 2001). Surface circulation experiences a substantial seasonality in the Sicily Channel: during late autumn to late spring, the MAW dominates the surface circulation, allowing nutrient- and chlorophyll-enriched waters to enter the Channel (Astraldi et al., 2002; D'Ortenzio, 2009). In turn, summer and autumn are dominated by LIW waters. Deep-water circulation remains relatively stable on a seasonal scale (Béranger et al., 2004), with a continuous LIW presence over the year. Finally, during summer, an upwelling settles in the Sicily Channel, allowing the impoverished LIW to reach the surface (Lermusiaux and Robinson, 2001).
Regarding its nutrient distributions, the Mediterranean Sea is generally regarded as an oligotrophic (Huertas et al., 2012) to ultraoligotrophic sea (Krom et al., 1991). However, this oligotrophy is not homogenous and displays a clear west-to-east gradient which is reflected in the nutrient concentration and algal biomass accumulation derived from colour remote sensing (Navarro et al., 2017; Siokou-Frangou et al., 2010). The eastern part of the Mediterranean is regarded as more nutrient-depleted than the western part of the basin (Krom et al., 2005; Raimbault et al., 1999), with N : P ratios around 50 : 1 (Krom et al., 2005). At times of maximum annual algal concentration, primary productivity (PP) in the Levantine Basin reaches values of ca. 0.1 g C m−2 d−1 (Hazan et al., 2018). This value is substantially lower than those recorded in the high-productivity regions of the western basin such as the Gulf of Lions, ca. 0.4–0.65 g C m−2 d−1 (Gaudy et al., 2003; Rigual-Hernández et al., 2012), or the Alboran Sea, ca. 0.3–1.3 g C m−2 d−1 (Bárcena et al., 2004; Morán and Estrada, 2001), during the corresponding period.
3.1 Field experiments
The sediment trap (Fig. 1) was deployed in the C01 mooring line maintained by ISMAR-CNR in the Sicily Channel (37.38° N, 11.59° E) thanks to a TransNational Access (TNA) call in the FP7 JERICO project. The mooring line was equipped with a sequential sampling sediment trap located 413 m below the sea surface in a water column of around 450 m deep. The sediment trap was a PPS3/3 model, conical in shape with a 2.5 height / diameter ratio and equipped with 12 sampling cups. Further information about this sediment trap configuration and model can be found in Heussner et al. (2006, 1990).
Here we present data from November 2013 to mid-October 2014. The sampling period was 15 to 16 d from November 2013 to July 2014 and from September 2014 to October 2014. Between July 2014 and September 2014, the sampling was set to 31 d. Before deployment and to limit the degradation of the material caught, sediment trap sampling cups from both mooring lines were filled with a 5 % formalin solution prepared with 40 % formaldehyde mixed with 0.45 µm of filtered seawater. The solution was then buffered with sodium borate to keep the pH stable and prevent the dissolution of carbonate.
3.2 Processing of sediment trap samples
After the recovery, the cups were stored at 2–4 °C until their processing according to the procedure of Heussner et al. (1990). In the laboratory, the largest swimmers that entered the trap were removed by wet sieving through a 1 mm nylon mesh, and samples were subsequently split into six aliquots using a peristaltic pump. One subsample was used for total mass flux measurements after having < 1 mm of swimmers and formaldehyde removed.
Another subsample of a total of 19 samples from the sediment trap was processed for micropalaeontological analyses in the micropalaeontology laboratory of the Geology Department at the University of Salamanca. The samples consisted of aliquots of one-sixth of the original mooring line cups and were preserved in seawater with a pH between 7.6 and 7.8. All samples were first wet-sieved to separate the < 63 µm fraction and then dry-sieved to separate the 63–150 and > 150 µm fractions. The washing was carried out with a potassium-phosphate-buffered solution (pH = 7.5) to prevent carbonate dissolution.
3.3 Planktonic foraminifera identification, flux calculations, and imaging
The planktonic foraminifera identification (Plate 1) and counting to the species level were carried out in the > 150 µm fraction using a microscope (Leica Wild M3B). To have a representative picture of the planktonic foraminifera population, the complete samples were analysed (i.e. no splits were applied). Identification was carried out according to Schiebel and Hemleben (2017). A total of 15 species were identified (Plate 1): Globigerinella siphonifera, G. calida, Globigerinoides sacculifer, G. ruber, G. ruber (pink), Globoturborotalita tenella, G. rubescens, Orbulina universa, Globorotalia truncatulinoides, G. inflata, G. scitula, Globigerina bulloides, G. falconensis, Neogloboquadrina incompta, and Turborotalita quinqueloba (Plate 1). In addition, benthic foraminifera shells were identified to the lowest taxonomic level possible and counted. The 150 µm size limit was used to compare our results with other time series and seabed sediment populations, as it is widely used in planktonic foraminifera studies; however, we acknowledge that some “small-sized” species such as N. incompta and G. tenella may be undersampled, as their adult size tends to be smaller (Chernihovsky et al., 2023).
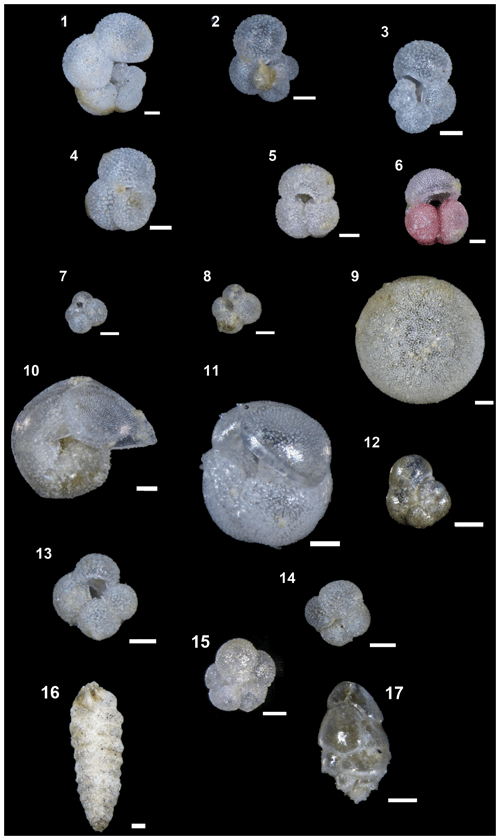
Plate 1Planktonic (1–15) and the most common benthic foraminifera (16–17) species trapped in the sediment trap in mooring line C01. The white scale bars on all figures represent 100 µm. (1) G. siphonifera, side view. (2) G. calida, umbilical view. (3) G. calida, apertural view. (4) G. sacculifer, umbilical view. (5) G. ruber, umbilical view. (6) G. ruber (pink), umbilical view. (7) G. tenella, umbilical view. (8) G. rubescens, umbilical view. (9) O. universa. (10) G. truncatulinoides, umbilical view. (11) G. inflata, apertural view. (12) G. scitula, umbilical view. (13) G. bulloides, umbilical view. (14) N. incompta, umbilical view. (15) T. quinqueloba, umbilical view. (16) Textularia spp. (17) Bulimina marginata, apertural view.
The foraminifera fluxes were calculated according to the following formula:
“PF” stands for planktonic foraminifera, “N” accounts for the number of individuals identified, “aliq.” refers to the aliquot (one-sixth of all samples), and “DS” represents the sampling interval during which the sediment trap cup stayed open. The relative abundance for each species was also calculated for all samples.
Here we refer to the planktonic foraminifera collected by the sediment trap as the settling assemblage.
Lastly, to describe the seasonal flux variations and to put our results into a regional context and be coherent with previous studies, each season was defined as spring (March–May), summer (June–August), autumn (September–November), and winter (December–February).
To showcase the species collected by the traps (Plate 1), foraminifera imaging was carried out using a Nikon SMZ18 stereomicroscope equipped with a Nikon DS-Fi3 camera and the image processing software NIS-Elements (version 5.11.03).
3.4 Satellite-derived environmental parameters
To assess the possible relationship of planktonic foraminifera fluxes to environmental variability, satellite-derived chlorophyll a and sea surface temperatures (SSTs) were retrieved from global datasets. Satellite-derived chlorophyll a concentration (mg m−3) was obtained from the MODIS L3m satellite through NASA's Giovanni web interface with an 8 d and 4 km resolution for a 0.2° × 0.2° area around the mooring location between 1 October 2013 and 1 November 2014. Additionally, SSTs (°C) were also obtained from the same site with the same resolution to use as a proxy for water temperature and water column stratification.
3.5 Planktonic foraminifera flux and surface sediment data from other Mediterranean settings
In order to put into context our observations of the regional variability in planktonic foraminifera communities in the Mediterranean Sea, modern planktonic foraminifera flux datasets were retrieved from different sites. Foraminifera fluxes were obtained for (i) the Levantine Basin (LevBas) from Avnaim-Katav et al. (2020), (ii) the Gulf of Lions (stations Planier (PLA) and Lacaze-Duthiers (LCD)) from Rigual-Hernández et al. (2012), and (iii) the Alboran Sea (stations ALB 1F and ALB 5F) from both Bárcena et al. (2004) and Hernández-Almeida et al. (2011). The foraminifera fluxes of the Gulf of Lions and the Alboran Sea concerned the > 150 µm fraction, while the ones from the Levantine Basin represented the > 125 µm fraction (Fig. 7).
Core-top data from the ForCenS database (Siccha and Kucera, 2017) were used to compare the planktonic foraminifera abundance patterns from the C01 mooring line with the seabed sediment. Only seabed sediment located on a 2.5° difference in both latitude and longitude was selected to compare our data with sites in the vicinity of the Sicily Channel. This corresponded to a total of 16 core tops, part of the MARGO database. The complete details of the latter can be found in the Béjard et al. (2023).
Additionally, the planktonic foraminifera population data from two box cores analysed by Incarbona et al. (2019) were also included: sites 342 (36.42° N, 13.55° E) and 407 (36.23° N, 14.27° E). These two sites are located in the Sicily Channel, and they provide a robust chronology (210Pb) that allowed us to document abundance changes across the recent Holocene. The dating covered the years 1558 to 1994 CE. Here we compared the sediment trap from the C01 mooring line samples with the mean relative abundance from the 23 (site 342) and 24 (site 407) samples available.
Finally, to have a more complete picture of the modern planktonic foraminifera communities currently living in the surface ocean, the annual integrated data of our sediment trap were compared with the BONGO nets data from Mallo et al. (2017), specifically with the sample retrieved in the axis of the Sicily Channel (37.08° N, 13.18° E) in spring 2013.
3.6 Statistical analysis
To have uninterrupted monthly and daily values from NASA's Giovanni environmental parameters that coincide with the mean sampling date from the sediment trap, a daily resampling was carried out using QAnalySeries software.
Pearson correlation and p-value tests between the foraminifera abundances and the environmental parameters (SST and chlorophyll a) were carried out with the Past4 program. A p < 0.05 was used to denote statistical significance.
In addition, a canonical correspondence analysis (CCA) was used to evaluate the influence of both SST and chlorophyll a on foraminifera species fluxes. A CCA is a correspondence analysis of a species matrix where each site has given values for one or more environmental variables (SST and chlorophyll a concentration in this case). The ordination axes are linear combinations of the environmental variables. A CCA is regarded as an example of direct gradient analysis, where the gradient in environmental variables is known and the species abundances/fluxes are regarded as a response to or being affected by this gradient (Nielsen, 2000).
Additionally, to evaluate the magnitude of the foraminifera fluxes across major regions of the Mediterranean, an estimation of the annual planktonic foraminifera flux (shells m−2 yr−1) was calculated using the sediment trap data from the literature review and from our study. To that purpose, the data were annualized according to the following formula:
where “PFF” stands for planktonic foraminifera flux (shells m−2 d−1), “SD” accounts for sampling days, “cPF” represents calculated planktonic foraminifera flux (shells m−2 d−1), and “mSD” stands for missing sampling days. The calculation of “cPF” depended on the site. For the datasets retrieved from the Sicily Channel and the Levantine Basin, fewer than 20 sampling days were missing, so the corresponding planktonic foraminifera fluxes were replaced by the mean of the first and last flux values recorded. The two datasets from the Alboran Sea displayed more than 70 missing days, so the corresponding flux values used were a mean of the 2 closest months to the missing data. Concerning the two time series from the Gulf of Lions, they covered more than 1 year. Therefore, a mean year was estimated: a mean monthly flux value was calculated for all 12 months based on all the available measurements and then multiplied by the corresponding mean duration of each month; then, all monthly fluxes were added together.
To compare the species richness and diversity across the previously described sites, Simpson (D) and Shannon–Weiner () indexes were calculated. Here, we reported the inverse Simpson index (1−D). None of these indexes were calculated for the Alboran Sea sites (ALB 1F and ALB 5F) because only information about the four main species was documented (Bárcena et al., 2004; Hernández-Almeida et al., 2011).
Finally, the squared-chord distance (SCD) between the C01 sediment trap and every core-top sample downloaded from the ForCenS database (Siccha and Kucera, 2017) planktonic foraminifera relative abundance was calculated. It is a widely used metric in palaeoecological and palaeontological studies, as it is the most effective index for identifying the closest analogues in planktonic foraminifera datasets (Prell, 1985). This is mainly because it shows the best balance in weighing the contribution of abundant and rare species in a given association (Jonkers et al., 2019). In this study, SCD values lower than 0.25 are regarded as reliable analogues (Ortiz and Mix, 1997).
4.1 General considerations of the planktonic foraminifera assemblages
A total of 3723 planktonic foraminifera shells and 141 benthic foraminifera were counted. Planktonic foraminifera were identified at the species level, resulting in a total of 15 different species identified (Plate 1). A mean of 196 planktonic foraminifera specimens per sample was identified, with a minimum of 14 individuals in November 2013 and a maximum of 633 individuals in mid-March 2014 (Table 1).
Table 1Counts and key statistics of the planktonic foraminifera species and the benthic foraminifera group from the > 150 µm fraction identified in the 19 sediment trap cups of the C01 mooring line. Mean, maximum (max), minimum (min), and standard deviation (SD) of the relative abundances and fluxes. Raw counts also include a total and a percentage of the total description. Note that G. falconensis was documented but not included in the table due to its scarcity (only one individual was identified).
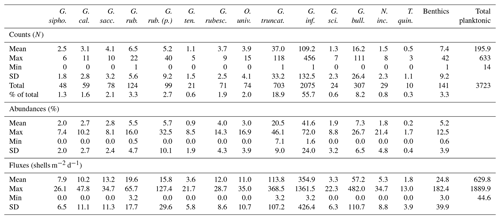
According to the raw count results, the most abundant species was G. inflata, which represented 55.7 % of the total planktonic foraminifera individuals. The second most represented species was G. truncatulinoides, with 18.9 %, followed by G. bulloides with 8.2 %. These three species alone accounted for more than 80 % of the planktonic foraminifera identified. The remaining species abundances were below 5 %. G. ruber, G. ruber (pink), O. universa, G. rubescens, and G. sacculifer represented between 2 and 3.3 % of the total individuals. Species like G. tenella, G. scitula, N. incompta, and T. quinqueloba were very scarce and accounted individually for less than 1 % of the total planktonic individuals (Table 1). Finally, only one individual of G. falconensis was identified. Note that G. inflata, G. truncatulinoides, and G. ruber were the only species present in all samples. Concerning the differentiation between lobulated and sac-type Globigerinoides, we mainly found individuals belonging to the first group, and the sac-type individuals were scarce. The latter were identified mainly during summer and autumn.
Finally, the benthic foraminifera only represented 3.3 % of the total foraminifera identified, and 80 % of the individuals were identified in the two samples retrieved during April 2014 (see Supplement).
4.2 Total mass and planktonic foraminifera fluxes
The mean total mass flux for the whole period of the study was 772.5 mg m−2 d−1, with a maximum value of 1737.7 mg m−2 d−1 and a minimum value of 179.5 mg m−2 d−1 reached in mid-May 2014 and November 2013, respectively (Fig. 2). Higher total mass flux values were reached during spring 2014, while lower values appeared during autumn of 2013 and 2014.
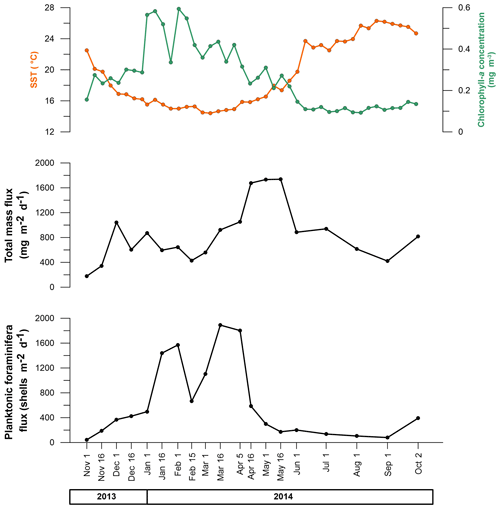
Figure 2Total mass flux (TMF) (mg m−2 d−1), total planktonic foraminifera flux (PFF) (shells m−2 d−1), SST (°C), and chlorophyll a concentration (mg m−3) changes between November 2013 and October 2014.
The planktonic foraminifera mean flux across the interval studied was 629.8 shells m−2 d−1, with a maximum value of 1889.9 shells m−2 d−1 and a minimum of 44.6 shells m−2 d−1 reached in mid-March 2014 and in November 2013, respectively. Higher values occurred during two periods, early spring and winter 2014, while the lower ones occurred from late spring to autumn 2014. Overall, the seasonal mean values were 1194.3 shells m−2 d−1 for the winter period, 612.3 shells m−2 d−1 for spring, 283.5 shells m−2 d−1 for autumn, and 107.2 shells m−2 d−1 for summer.
The SST mean value was 19.2 °C, and values ranged between a maximum of 26.1 and a minimum of 14.5 °C. The mean chlorophyll a value was 0.27 mg m−3, the maximum value displayed was 0.56 mg m−3, and the minimum one was 0.09 mg m−3 (Fig. 2).
4.3 Foraminifera species fluxes
Overall, most of the planktonic foraminifera species collected by the trap exhibited either a unimodal or bimodal flux distribution, with a few exceptions (Fig. 3).
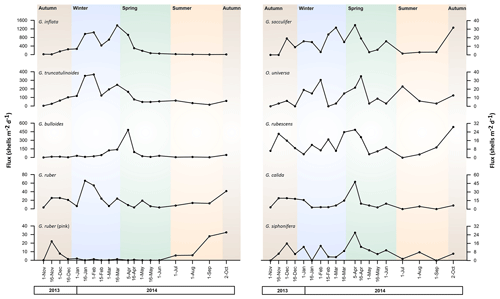
Figure 3Planktonic foraminifera fluxes (shells m−2 d−1) from November 2013 to October 2014 of the 10 most abundant species identified. Note that the scale of the fluxes depends on the species. Background colours represent the different seasons: brown for autumn, blue for winter, green for spring, and orange for summer.
Globorotalia inflata exhibited the highest fluxes of all species, with a mean flux of 368 shells m−2 d−1 throughout the record, with peak values in mid-March 2014 (1361 shells m−2 d−1) and minimum values in November 2013 (3 shells m−2 d−1). G. truncatulinoides was the second most important contributor (mean of 114 shells m−2 d−1), with a maximum in mid-February and a minimum in November 2013 (368 and 3 shells m−2 d−1, respectively). G. bulloides was the third most important contributor to the total planktonic foraminifera fluxes, with a mean flux of 57.2 shells m−2 d−1 and with maximum values registered in April 2014 and minimum values in November 2013 (482 and 0 shells m−2 d−1, respectively).
The remaining species displayed mean fluxes lower than 50 shells m−2 d−1. G. calida, G. ruber, G. ruber (pink), G. rubescens, and O. universa mean fluxes comprised between 10 and 20. Among these species, G. ruber and G. ruber (pink) stood out and showed maximum fluxes of 66 shells m−2 d−1 in February 2014 and 127 shells m−2 d−1 in October 2014, respectively. For the remaining species, G. siphonifera, G. scitula, N. incompta, and T. quinqueloba, mean and maximum fluxes were lower than 10 and 35 shells m−2 d−1, respectively, thereby representing a low contribution to the total foraminifera fluxes.
Finally, it is worth noting that benthic foraminifera were also collected by the trap, displaying a mean flux of 25 shells m−2 d−1. The peak contribution of these taxa was recorded in April 2014 (182 shells m−2 d−1), and a minimum value was recorded in January 2014 (3 shells m−2 d−1). In terms of annualized foraminifera flux, their contribution was only 1.1 % of the total foraminifera identified, of which 75 % was recorded during April 2014 (Fig. 6).
The variations in relative abundance differed according to the species. Most of the species displayed a unimodal distribution across the studied interval (Fig. S3 in the Supplement), with some exceptions, such as G. siphonifera, G. calida, and G. ruber. Overall,cG. inflata dominated the association from late autumn until mid-spring. The relative abundance pattern of G. truncatulinoides was similar to that of G. inflata, with maximum values in autumn and late summer. In turn, G. bulloides displayed a pronounced seasonal change in its relative abundance, reaching values up to 27 % in early spring (April 2014) and dropping to about 5 %–8 % in November 2014.
Overall, G. inflata is the only species that displayed its maximum mean relative abundance during winter: 64 %. For G. siphonifera, G. sacculifer, and G. bulloides, maximum mean relative abundances were reached during spring: 3 %, 3.5 %, and 14 %, respectively. For G. calida, G. tenella, G. rubescens, and N. incompta, maximum mean abundances appeared to be in autumn: 5.7 %, 2.2 %, 8 %, and 4.8 %, respectively. Finally, for G. ruber, G. ruber (pink), O. universa, G. truncatulinoides, and G. scitula, maximum mean relative abundances were displayed in summer: 11.6 %, 13.2 %, 8.9 %, 32.8 %, and 6.4 %, respectively (Fig. S3).
4.4 Chlorophyll-a and SST impact on foraminifera fluxes
A CCA (see Sect. 3.4) was carried out to characterize the impact of both SST and chlorophyll a on the planktonic foraminifera fluxes (Fig. 4).
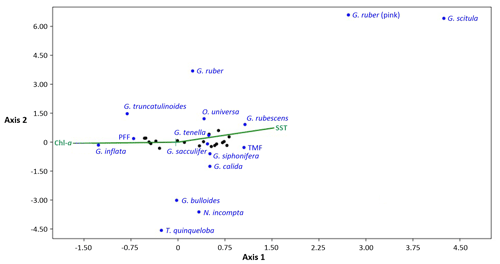
Figure 4CCA of all planktonic foraminifera species fluxes with SST (°C) and chlorophyll a (“chl a” in the CCA, in mg m−3) as the explanatory variables. The total mass flux (“TMF”) and planktonic foraminifera flux (“PFF”) are also included. Black dots represent the 19 sediment trap samples studied.
Axis 1 shows, overall, the differences between deep dwellers and surface dwellers. The total planktonic foraminifera flux (PFF) and the fluxes of G. inflata and G. truncatulinoides are positively affected by the chlorophyll a concentration and negatively affected by the SST. On the other hand, G. ruber, G. ruber, (pink) and G. scitula fluxes show an opposite pattern, being positively related to the SST and negatively related to the chlorophyll a concentration. O. universa, G. rubescens, G. tenella, G. sacculifer, G. siphonifera, and G. calida fluxes are positively correlated with the SST and negatively correlated with chlorophyll a concentration; nonetheless, the impact of these parameters is weaker compared with the previous species. Finally, G. bulloides, N. incompta, and T. quinqueloba fluxes are slightly positively influenced by the chlorophyll a concentration. Axis 2 tends to separate the species between the different trophic regimes. Overall, it confirms that, on the one hand, G. ruber, G. ruber (pink), and G. scitula display a strong negative correlation with chlorophyll a and therefore an affinity for oligotrophic and warm conditions; on the other hand, it shows that G. bulloides, N. incompta, and T. quinqueloba display a positive correlation with chlorophyll a and eutrophic conditions. Furthermore, G. bulloides flux shows a strong correlation with the latter two species: 0.89 and 0.83 (p < 0.05).
5.1 Seasonal variations in the magnitude of planktonic foraminifera fluxes in the Sicily Channel
The strong seasonality in the planktonic foraminifera fluxes registered by the trap is generally similar in amplitude to previous studies in the Mediterranean (Bárcena et al., 2004; Rigual-Hernández et al., 2012) and other temperate settings (Kuroyanagi and Kawahata, 2004; Wilke et al., 2009), thereby suggesting the C01 record mainly reflects the temporal variations in planktonic foraminifera abundance in the upper water column. Therefore, next, we discuss the influence of oceanographic controls on the planktonic foraminifera fluxes.
Our data show that, despite differences in the magnitude of their fluxes, most of the species identified display their maximum flux during winter, the winter–spring transition, or spring (Fig. 3), thereby coinciding with the period of maximum algal biomass accumulation and coldest SSTs (Fig. 2). The enhanced primary productivity during winter and spring is mostly related to an intensification of the chlorophyll a and nutrient-richer MAW flow into the eastern Mediterranean Basin (D'Ortenzio, 2009; Pinardi et al., 2015; Siokou-Frangou et al., 2010). Our CCA results (Fig. 4) show that, although the flux patterns increase during winter and spring, only fluxes of G. inflata, G. truncatulinoides, and, arguably, G. bulloides (further discussed below) are negatively related to SSTs and positively related to the chlorophyll a concentration. The dominance of the planktonic foraminifera fluxes by these three species and their affinity for mesotrophic waters is not surprising, as G. inflata and G. truncatulinoides are typically associated with the MAW, winter water-mixing events, and hydrologic fronts in the western Mediterranean, while G. bulloides is generally associated with eutrophic environments linked to upwelling conditions (Azibeiro et al., 2023). Overall, these three taxa have been described as dominant during winter in various western regions of the Mediterranean, such as the Alboran Sea (Bárcena et al., 2004; Hernández-Almeida et al., 2011), the Provençal Basin, and the Gulf of Lions (Pujol and Grazzini, 1995; Rigual-Hernández et al., 2012). Interestingly, G. inflata, G. truncatulinoides, and G. bulloides are almost absent in the eastern part of the basin, most likely due to the low algal biomass accumulation (Avnaim-Katav et al., 2020; Thunell, 1978).
Conversely, species such as G. ruber, G. ruber (pink), G. scitula, G. rubescens, and G. sacculifer display their maximum fluxes in summer or autumn (Fig. 3). During the warm periods, summer and autumn, the eastward advection of Atlantic Water in the Sicily Channel is weakened due to an increased meandering of the ATC (Fig. 1) and the local hydrography patterns (Béranger et al., 2004), leading to a local water column stratification period which is also well documented in the whole Mediterranean Basin during summer (Siokou-Frangou et al., 2010). This translates into a reduced MAW influence and a larger influence of the LIW at intermediate depths (Astraldi et al., 2002, 2001; Jouini et al., 2016). Therefore, the water column becomes warmer, saltier, and more nutrient-depleted than the general conditions of the western basin (Gasparini et al., 2005; Navarro et al., 2017; Siokou-Frangou et al., 2010) and provides the necessary environmental and oceanographical configuration for eastern basin taxa to develop or to be transported from the easternmost part of the Mediterranean. Indeed, our CCA results (Fig. 4) support these observations (Fig. 3). The latter species have been described to reach their maximum abundances in the eastern part of the Mediterranean, specifically in the Ionian and Levantine basins during both summer and autumn (Avnaim-Katav et al., 2020; Pujol and Grazzini, 1995).
Some species, such as O. universa or G. calida, do not display a clear flux pattern over the period studied. CCA results suggest that these species have an affinity for warm and less productive conditions. These taxa are regarded as widespread in the Mediterranean Basin, although their relative contributions are generally higher in the eastern part of the basin (Avnaim-Katav et al., 2020; Pujol and Grazzini, 1995; Thunell, 1978). Lastly, it is important to note that the low number of specimens of G. falconensis, N. incompta, T. quinqueloba, and G. tenella found in our samples makes the estimation of shell fluxes for these species unreliable. These results are not surprising, since N. incompta is mainly found in the northwestern part of the basin owing to cold and eutrophic conditions (Azibeiro et al., 2023; Millot and Taupier-Letage, 2005), while T. quinqueloba has generally been associated with cool Atlantic Water or cool marginal seas (Azibeiro et al., 2023).
In summary, planktonic foraminifera flux was at its maximum during winter and spring, coinciding with the maximum seasonal eastward advection that brings MAW further east into the Sicily Channel. These waters are less saline and nutrient-enriched compared to the easternmost waters from the Levantine Basin. G. inflata, G. truncatulinoides, and G. bulloides (the three most abundant species that dominate the PFF), which are species described to come from the western basins, are probably brought by the MAW and then dominate the planktonic foraminifera population. On the other hand, during summer and autumn, the eastward advection weakens, allowing the LIW and AIS to dominate the surface circulation due to the water column stratification and set favourable conditions for eastern-basin-dominant taxa, such as both morphotypes of G. ruber, G. rubescens, and G. sacculifer. This results in a significantly decreased planktonic foraminifera flux due to the absence of western-basin-dominant species.
5.2 Species succession, ecology, and the impact of SST and chlorophyll a
The time series of settling planktonic foraminifera reflects a diverse assemblage with species with contrastingly different ecological preferences, encompassing a wide range of depth habitats and diverse feeding strategies. Overall, the annual assemblage composition agrees well with previous shipboard observations (Pujol and Grazzini, 1995) in the Sicily Channel during the 1988 VICOMED cruise, where G. inflata, G. truncatulinoides, and G. bulloides were documented as the most abundant taxa.
Next, we discuss the ecology of the most abundant species and the impact of chlorophyll a and SST on their distribution. We also discuss the foraminifera groups suggested by Jonkers and Kučera (2015) to explore their correlation with the previous parameters on an interannual scale. The latter work proposed three groups: group 1 is formed by tropical and subtropical species, group 2 consists of temperate to subpolar taxa, and group 3 represents the deep-dwelling species. These groups were described as the result of the seasonal maximum flux timing of each species and its relationship with both temperatures and nutrients (amongst other parameters) in different time series across the world ocean. Therefore, here, we also use this grouping to compare and complete this classification from a new time series dataset.
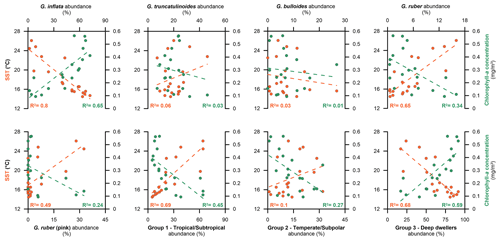
Figure 5SST and chlorophyll a concentration against the relative abundance of the five most abundant species and the three ecological groups proposed by Jonkers and Kučera (2015). Orange dots stand for SST, while the green ones correspond to chlorophyll a.
Globorotalia inflata is the most abundant taxon in our samples. Our data show that maximum fluxes and relative abundances of this species are reached during winter and during the winter–spring transition (Fig. 3). The relative abundances showed strong positive and significant negative (p < 0.05) correlations with the chlorophyll a concentration and the SST: 0.808 and −0.896, respectively (Fig. 5). G. inflata is a non-spinose species and is regarded as a deep dweller (Hemleben et al., 1989; Schiebel and Hemleben, 2017). It is generally regarded as showing limited opportunistic behaviour, and it has often been associated with eddies and hydrological fronts (Chapman, 2010; Retailleau et al., 2011). Concerning the Mediterranean, its maximum stocks and abundances have been recorded along the southern margin of the western Mediterranean Basin (Azibeiro et al., 2023), especially during winter (Bárcena et al., 2004; Pujol and Grazzini, 1995; Rigual-Hernández et al., 2012), while it is poorly represented in the eastern part, even absent in the Levantine Basin (Avnaim-Katav et al., 2020). As a consequence, G. inflata can be regarded as a mesotrophic species which is dominant in regions with some degree of stratification of the water column and an intermediate amount of nutrients, and it has been used as a tracer of the Atlantic inflow into the Mediterranean Basin (Azibeiro et al., 2023), which agrees with the local hydrography in the Sicily Channel during winter and spring. As G. inflata appeared in periods of cool and nutrient-enriched waters (Fig. 3), which coincided with the periods of higher MAW influence in the Sicily Channel (Béranger et al., 2004), we regard our results as further confirmation that G. inflata is a tracer of the MAW in the Sicily Channel.
Globorotalia truncatulinoides is the second most abundant species in our record. However, our CCA results suggest that the seasonal variations in G. truncatulinoides are not directly correlated with either chlorophyll a concentration or SSTs (r = −0.162 and 0.256, respectively; p > 0.05) (Fig. 5). This highlights the fact that environmental controls other than the ones considered here may be affecting its distribution. This taxon is a cosmopolitan species found in all major oceans (Schiebel and Hemleben, 2017) and is regarded as a deep dweller with an affinity for water-mixing conditions (Margaritelli et al., 2020; Schiebel and Hemleben, 2005). It is a non-spinose species with a complex life cycle. In the Mediterranean, peak abundances of this species are found in the northwestern part of the basin, where it represents a major component of the assemblages (Pujol and Grazzini, 1995; Rigual-Hernández et al., 2012), while it is absent in the easternmost part of the basin (Avnaim-Katav et al., 2020). This species has been documented to have a complex life cycle and reproductive strategy. G. truncatulinoides has been described as reproducing once a year in the upper layers of the water column, generally when the water mixing allows the migration of juvenile individuals to the surface (Lohmann and Schweitzer, 1990; Schiebel et al., 2002). Then, adult individuals migrate down the water column, where they spend the rest of their life cycle (Rebotim et al., 2017; Schiebel and Hemleben, 2005). Hence, we speculate that these complex migratory patterns may play a role here. As its reproduction cycle is mainly controlled by the gametogenesis process, and, as described previously, it reproduces once a year (a slower rate than the majority of the planktonic foraminifera species) (Schiebel and Hemleben, 2017), although different stages of its life cycle could be affected by SST and chlorophyll a, this is not necessarily registered by the sediment traps in every stage of its growth.
Globigerina bulloides is the third most abundant planktonic foraminifera species identified here. It is a surface to subsurface dweller and one of the most common species across the world ocean (Schiebel and Hemleben, 2017). Interestingly, our analysis showed no significant correlation between changes in the relative abundance of G. bulloides and in the chlorophyll a concentration or SST (r = −0.145 and −0.111, respectively; p > 0.05). However, across the time span studied, this taxon showed its maximum abundance and fluxes during relatively high chlorophyll a and cool SST conditions (Fig. 3). This highlights that other environmental parameters than the ones considered here might play a role in its distribution. It is a spinose species known for its opportunistic feeding strategy (Schiebel et al., 2001) and affinity for upwelling and eutrophic environments (Azibeiro et al., 2023; Bé et al., 1977). Within the Mediterranean Sea, it displays peak export fluxes to the deep sea in areas of high productivity, such as the Gulf of Lions and the Alboran Sea, during the high productivity period in late winter to spring (Azibeiro et al., 2023; Bárcena et al., 2004; Hernández-Almeida et al., 2011; Rigual-Hernández et al., 2012), while few individuals are found in the eastern part of the Mediterranean (Avnaim-Katav et al., 2020). We surmise that, owing to its multiple trophic strategies and its multi-diet characteristics, it could adapt and feed on varying chlorophyll a concentrations. Also, the lack of correlation with both parameters could be explained by the fact that this taxon is associated with eutrophic conditions. In the Sicily Channel, the high productivity period ranges from winter to spring, and the conditions allow deep mesotrophic dwellers (i.e. G. inflata) to dominate the assemblage, while, in summer and autumn, the upwelling setting brings oligotrophic conditions that are not favourable for this species.
Generally, fluxes and abundances of both G. bulloides and G. truncatulinoides are positively linked to favourable food conditions and high-productivity environments. The first species tends to exhibit a “bloom” strategy on short timescales, while the second species tends to be related to nutrient advection zones in the Mediterranean Sea (Margaritelli et al., 2022). Furthermore, in the northwestern Mediterranean, a previous study showed that the fluxes of these two species are almost in phase (Rigual-Hernández et al., 2012). Interestingly, in the Sicily Channel, this relation is not straightforward. In the Gulf of Lions, G. bulloides is the main species and shows the classical “bloom” behaviour, while the G. truncatulinoides pattern is more constant and its variations are more gradual (Rigual-Hernández et al., 2012). Although the timing of the two species is different in our record, the response of G. truncatulinoides is similar across the record. Furthermore, from a productivity standpoint, the Sicily Channel is less productive than the Gulf of Lions (Siokou-Frangou et al., 2010), which, in turn, does not benefit G. bulloides abundances, and, as the upwelling in our study zone is less pronounced than in other parts of the Mediterranean, the timing between the two species is different. Additionally, the intensity of the upwelling in the central Mediterranean is controlled by variations in the intensity of the LIW flowing to the western part of the basin (Astraldi et al., 2001; Lermusiaux and Robinson, 2001; Pinardi et al., 2015), with higher intensity leading to reduced upwelling and therefore reduced productivity. This could explain the lack of high abundance of G. bulloides in our study region, as the upwelling in the Sicily Channel is reduced compared to other places in the Mediterranean (D'Ortenzio, 2009; Siokou-Frangou et al., 2010); therefore, the increase in productivity is diminished compared to other regions in which the productivity and the abundance of G. bulloides are higher, such as the Alboran Sea (Bárcena et al., 2004). Therefore, we conclude that a combination of ecological preferences and oceanographic processes could explain the lack of synchronicity between these two species' fluxes and abundances.
Globigerinoides ruber and G. ruber (pink) are the fourth and fifth most abundant species in our samples (Table 1). Our correlation analyses showed a significant positive effect of SST (r = 0.803 and 0.678, p < 0.05) and a significant negative effect of chlorophyll a (r = −0.567 and −0.464, respectively; p < 0.05) on both G. ruber and G. ruber (pink), respectively (Fig. 5). These species have been described as tropical to subtropical taxa, with an affinity for oligotrophic and stratified waters (Bé et al., 1977). Both of these species are among the shallowest dwellers of the extant planktonic foraminifera species and are regarded as some of the most adaptable to varying surface water conditions (Kemle-von Mücke and Oberhänsli, 1999; Schiebel and Hemleben, 2017). Due to its temperature and salinity limits for food acceptance, the white variety is one of the most studied foraminifera species in culture experiments, highlighting its euryhaline and eurythermal life cycle (Bijma et al., 1990; Lombard et al., 2009). In today's ocean, the white variety is substantially more abundant than the pink one (Schiebel and Hemleben, 2017). In the case of the Mediterranean Basin, G. ruber is generally associated with warm and oligotrophic waters (Pujol and Grazzini, 1995) and is abundant in the eastern oligotrophic basin, where it dominates the assemblages in the Levantine Basin during spring and autumn (Avnaim-Katav et al., 2020). However, although present in the western basin, its abundance is much lower in the Gulf of Lions (Rigual-Hernández et al., 2012) and in the Alboran Sea (Bárcena et al., 2004). Overall, the correlation data agree with the previous work that linked G. ruber (both varieties) to warm and oligotrophic conditions generally displayed during a higher stratification of the water column (Schiebel et al., 2004). As this species is mostly abundant in the eastern part of the Mediterranean, it should be expected that the LIW, when it dominates the circulation during summer and autumn, brings this species along with other oligotrophic taxa. However, fluxes (Fig. 3) and relative abundance data (Fig. S3) showed that the maximum appearances of this species were recorded during winter, coincidently with G. inflata and G. truncatulinoides. Therefore, the winter recorded in our dataset showed favourable conditions for both deep mesotrophic dwellers and oligotrophic species such as G. ruber. We interpret this pattern as a reduced influence of the MAW during winter in the Sicily Channel that could lead to slightly warmer than usual surface conditions that favour the stratification and, hence, the G. ruber abundances. Concerning G. ruber (pink), as its fluxes and abundances were higher during summer and it is mainly identified in the eastern part of the Mediterranean, as well, we conclude that the LIW influence brings this species into the Sicily Channel.
According to Jonkers and Kučera (2015), the foraminifera fluxes can be predicted on a seasonal scale for three different groups of planktonic foraminifera. Following this approach, we explore the relative abundance of these three aggrupations to document if these correlate with both SST and chlorophyll a concentration (see Table S1) in the period covered by the sediment trap (Fig. 5). The first group (group 1) consists of both G. ruber varieties, G. sacculifer, O. universa, G. siphonifera, G. rubescens, and G. tenella. The second group (group 2) is formed by G. bulloides, T. quinqueloba, N. incompta, G. scitula, and G. calida. In our record, however, neither G. bulloides nor G. calida displayed a similar trend, and the abundance of the remaining three species was < 1.5 %, making any significant assumption difficult (Table 1). The third (group 3) is composed of the deep dwellers G. inflata and G. truncatulinoides. Group 1 showed a strong and significant positive correlation with the SST (Fig. 5) and a negative correlation with the chlorophyll a (r = 0.828 and −0.668, respectively; p < 0.05; see Table S1). This is not surprising, as the majority of the group is formed by species that are not only regarded as tropical but are also well adapted to oligotrophic and nutrient-impoverished environments (Chapman, 2010; Hemleben et al., 1989; Schiebel and Hemleben, 2017). In addition, most components of this group are symbiont-bearing species (Takagi et al., 2019), which have been described as being more adapted to nutrient-depleted and oligotrophic conditions. Group 2, on the other hand, did not show any strong correlation to either SST and chlorophyll a concentration, although a significant negative correlation was displayed between the group abundances and the latter parameter (r = −0.525; see Table S1). This result is not surprising, as the main component of this group is G. bulloides, which previously showed a lack of correlation with both SST and chlorophyll a, while the remaining species of this group were taxa that tend to be outnumbered by more opportunistic species (i.e. N. incompta and T. quinqueloba) (Kuroyanagi and Kawahata, 2004; Schiebel, 2002). Also, the overall abundance of these taxa was very low in our samples compared to the other two groups, which in turn could affect the correlation results. Here we propose that the mesotrophic conditions of the Sicily Channel developed during the relatively high productivity period are not favourable enough for the development of the taxa comprising group 2. Finally, group 3 displayed a strong and significant positive correlation with chlorophyll a concentration (r = 0.771, p < 0.05), which is an expected trend according to the affinity shown to mesotrophic conditions by the two species that constitute this group; however, as compared to Jonkers and Kučera (2015), we show a strong and significant negative correlation of these two species' abundances with the SST (Fig. 5). The latter work stated that the cycles of these species were independent of the temperature changes; however, these two species tend to be used as tracers of cool and deep mesotrophic waters in the Mediterranean, generally associated with the MAW (Azibeiro et al., 2023).
In summary, our data show that, in the Sicily Channel, the three major ecological groups proposed by Jonkers and Kučera (2015) exhibited a different response to environmental variability. Overall, groups 1 and 3 showed significant correlation with the latter parameters and were in accordance with their corresponding species ecologies. However, group 2 did not show any significant correlation, which we interpreted as the result of very low abundances of the taxa comprising this group. This translates into the dominance of group 1 during summer and autumn, when oligotrophic and warm eastern waters dominate the water column, while the mesotrophic taxa from group 3 dominate during winter and spring, coincidently with higher primary productivity, yet the waters are not eutrophic enough for the opportunistic taxa comprising group 2, which are less well represented in the Sicily Channel.
5.3 Influence of the hydrodynamic conditions on the planktonic foraminifera assemblage
A possible source of variability between the living foraminifera assemblages and those collected by the trap could be the preferential transport of certain species by the currents and differences in the sinking rates between species. Typically, deep-dwelling species produce heavier shells that the surface-dwelling ones (Zarkogiannis et al., 2022). Theoretically, lighter species are easier to remobilize than the heavier ones; however, if the current is strong enough, lighter species could travel far away, while heavier species could be reworked in the vicinity of their deposition zone. G. truncatulinoides is among the heaviest planktonic foraminifera species (Beer et al., 2010; Béjard et al., 2023). Therefore, if the current is strong enough, it could be resuspended and be recorded by the sediment trap. The record in the seabed sediment (see Sect. 5.5) showed that G. truncatulinoides was more abundant in the settling particles from the C01 mooring line (Fig. 8), and, according to the winnowing theory, G. inflata should follow a similar pattern, as it also a heavy species (Zarkogiannis et al., 2022). However, surface data (Mallo et al., 2017) show that the latter is also the dominant species in the BONGO nets (see Sect. 5.5). Furthermore, Takahashi and Be (1984) presented data about the sinking speeds of different planktonic foraminifera species. As an example, G. inflata showed a sinking speed of 500 m d−1 compared to 330 m d−1 for G. bulloides. These different sinking rates applied in a water column of around 450 m suggest that the likely origins of the planktonic foraminifera collected by the traps must be similar and are insufficient to generate discrepancies between the foraminifera assemblages living in the upper water column and those collected by the trap.
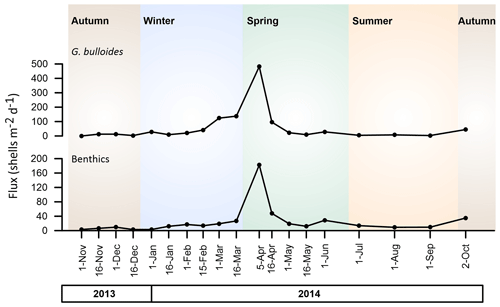
Figure 6G. bulloides and benthic foraminifera fluxes (shells m−2 d−1) between November 2013 and October 2014.
The identification of benthic foraminifera individuals suggests an impact of the hydrodynamic conditions on the settling particle populations. The main species identified were T. saggitula spp. and B. marginata (Plate 1), along with a small number of Uvigerina mediterranea and Lagena striata. These taxa are regarded as infaunal species; i.e. they live buried in the sediment (Balestra et al., 2017; Milker and Schmiedl, 2012) and are commonly found in continental shelves and slopes. Overall, benthic foraminifera accounted for a mean of only 3.4 % of the total foraminifera identified in the C01 settling particles (Table 1), and the percentage of planktonic oscillated between 89 % and 99.4 %. Most of the annual benthic fluxes occurred during April, when a total of 80 % of the annual benthic foraminifera fluxes were recorded (Fig. 6). As described previously, the Sicily Channel hydrography is complex from both a vertical and a seasonal point of view (Astraldi et al., 2001; Garcia-Solsona et al., 2020; Incarbona et al., 2011; Pinardi et al., 2015; Schroeder et al., 2017). In the Sicily Channel, the tidal and subtidal current speed is known to reach maximum annual values during spring (Gasparini et al., 2004), which could be invoked as a possible source of sediment resuspension including benthic species. This has also been observed in different parts of the Mediterranean (Grifoll et al., 2019). Indeed, in our record, the highest benthic foraminifera fluxes were collected during spring (Fig. 6), i.e. the period of peak current intensity in the Channel. Coincidently, it also showed the highest fluxes of G. bulloides (Fig. 3), which is the third most abundant species in our record (Table 1). Interestingly, this species annual flux distribution showed no correlation with either the SST or the chlorophyll a (Fig. 5). These observations, coupled with the fact that the fluxes of G. bulloides and the benthic foraminifera were positively and significantly correlated (r = 0.89, p < 0.05), suggest that benthic species were resuspended, being caught at a water depth of 40 m by our sediment trap. Furthermore, a low amount of detritus and debris, such as mica flakes, was identified in the samples that contained the highest number of benthic foraminifera (April 2014), which again suggests a secondary influence of resuspended sediments in the sediment trap record in specific intervals of the annual cycle. However, no such relationship has been identified with the other species that did not show any correlation with the previous environmental parameters: G. truncatulinoides. Consequently, we hereby propose that G. bulloides distribution and abundances are blurred in specific intervals by the resuspension of seafloor sediments. Finally, the increase in G. bulloides abundance and fluxes, which has been identified coincidently with a higher number of benthic foraminifera during early April, could lead to the interpretation that the benthic foraminifera are the result of the intensification of the MAW. However, as the presence of the benthic foraminifera is patchy and not constant, we do not believe their presence is ruled out as a reliable proxy for the MAW/LIW intensity. Therefore, it can be concluded that the C01 sediment trap mainly records a pelagic signal with a secondary influence of resuspended sediments.
5.4 Geographical variability in the magnitude and composition of planktonic foraminifera fluxes across the Mediterranean
The comparison of the settling planktonic foraminifera assemblage from the Sicily Channel with the ones retrieved from different parts of the Mediterranean offers a unique opportunity to provide further insight into the central Mediterranean dynamics and ecology of this group.
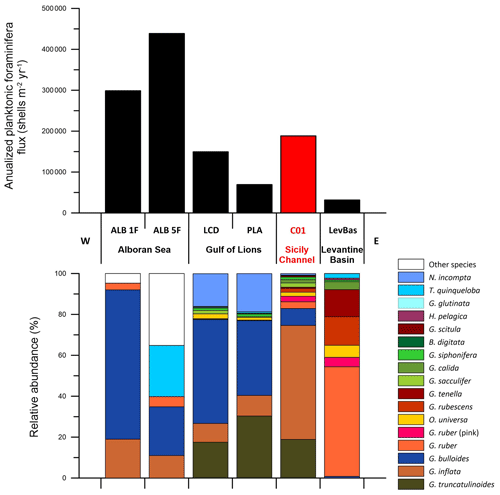
Figure 7Comparison of the annualized (see Sect. 3.4) planktonic foraminifera flux and the relative abundance of each species identified in different time series across the Mediterranean Sea (see Sect. 3.5). The data from the Sicily Channel (C01) are depicted in red. Note that the Levantine Basin (LevBas) dataset covers the > 125 µm fraction. The “other species” (white bar) category in the Alboran Sea corresponds to any species different from the main four taxa identified in Bárcena et al. (2004) and Hernández-Almeida et al. (2011).
As stated previously, the planktonic foraminifera flux in the Sicily Channel was higher from mid-January to mid-March, which coincided with the highest chlorophyll concentrations and the coolest SST recorded (Fig. 2). This seasonality is similar to the one observed in the Gulf of Lions, where the planktonic foraminifera flux reached its highest values from mid-February to mid-March during different years (Rigual-Hernández et al., 2012). Although slightly different, the planktonic foraminifera flux patterns from both the Levantine Basin and the Alboran Sea also displayed maximum values from mid-February to mid-March and from mid-January to mid-February, respectively (Avnaim-Katav et al., 2020; Hernández-Almeida et al., 2011). However, the magnitude of the planktonic foraminifera flux values displayed some differences between the sites (see Fig. S2). Overall, for the Sicily Channel, values ranged between 0–1889 shells m−2 d−1 with a mean value of 629 shells m−2 d−1. These values were comparable to the ones from the Gulf of Lions: 0–2114 and 4268 shells m−2 d−1 with a mean value of 225.4 in the Planier sediment trap to 419 shells m−2 d−1 in the Lacaze-Duthiers sediment trap (Fig. 7). On the other hand, the Levantine Basin values were lower: 0–429 shells m−2 d−1, with a mean value of 93 shells m−2 d−1. Finally, the highest values belonged to the Alboran Sea: 0–6000 shells m−2 d−1 with a mean value of 783 to 970 shells m−2 d−1 depending on the gyres. Note that the planktonic foraminifera flux values from the Levantine Basin used here represent the foraminifera shells from the > 125 µm fraction, which highlights the fact that, compared to the > 150 µm, the flux values should be even lower. The corresponding chlorophyll a values registered in the latter sites were 0.2–0.65 mg m−3 for the Sicily Channel (Fig. 5), 0.25–0.85 mg m−3 for the Gulf of Lions (0–0.65 mg m−3 in the Planier site and 0.25–0.85 mg m−3 in the Lacaze-Duthiers site) (Rigual-Hernández et al., 2012), 0.02–0.4 mg m−3 for the Levantine Basin (Avnaim-Katav et al., 2020), and 0.1–1.2 mg m−3 in the Alboran Sea (Hernández-Almeida et al., 2011), indicating a similar productivity in terms of chlorophyll a between the Gulf of Lions and the Sicily Channel. In addition, we calculated an annualized planktonic foraminifera flux (Sect. 3.4) for each of the six sites compared here (Fig. 7). Overall, the highest annualized fluxes were displayed in the Alboran Sea (Fig. 7), around 3 × 105 and 4.4 × 105 shells m−2 yr−1, while the lowest one was displayed in the Levantine Basin, a little over 30 000 shells m−2 yr−1 (Fig. 7). The Gulf of Lions and the Sicily Channel displayed comparable annualized fluxes (although higher for the latter): around 1.5 × 105 and 1.85 × 105 shells m−2 yr−1, respectively. Note that PLA site values were significantly lower: around 7 × 104 shells m−2 yr−1 (Fig. 7). Previous work showed that these planktonic foraminifera patterns were mainly linked to specific regional oceanographic processes. First of all, the Levantine Basin is well known for being an ultraoligotrophic region and for being the warmest and saltiest of the Mediterranean basins (Ozer et al., 2017), mainly due to the W–E anti-estuarine circulation. On the other hand, the Gulf of Lions is regarded as an exception to the general oligotrophy of the Mediterranean. The seasonal vertical-mixing phenomenon occurs in winter, generated by cold winds. This winter mixing recharges the surface waters with nutrients, allowing a winter/spring productivity bloom (Durrieu de Madron et al., 2013; Houpert et al., 2016). Finally, the Alboran Sea is a transitional region between the Atlantic Ocean and the Mediterranean Sea (Hernández-Almeida et al., 2011), and, unlike the latter, it is not an oligotrophic region, due to the two systems of high productivity related to the gyres generated by intense westerlies, which allow nutrient-enriched (compared to the resident waters) Atlantic Water to spread into the Mediterranean. This results in an enhanced primary productivity period from November to March. According to the PFF patterns displayed in this study, the Sicily Channel presents similar values and flux distributions to the Gulf of Lions; however, its oceanographic circulation is significantly different from the latter. These observations agree with the work of Mallo et al. (2017) carried out with plankton tows in the whole Mediterranean Basin. The latter work found that the Alboran Sea displayed the highest-standing stocks of planktonic foraminifera, while the easternmost part of the Mediterranean showed the minimum values. Also, the Gulf of Lions and the Sicily Channel displayed similar stocks, although slightly superior in the case of the Sicily Channel.
Concerning the species composition, we identified 15 planktonic foraminifera species in the Sicily Channel, which is a similar species number to the one from the Gulf of Lions (14 species) and higher than in the Levantine Basin (10 different species). The Sicily Channel site displayed the highest planktonic foraminifera assemblage diversity among the three sites compared: a mean 1−D and index of 0.68 and 1.57, respectively (Table 2). Interestingly, despite showing a similar number of different species, the Gulf of Lions displayed the lowest diversity values, especially for the PLA site (mean 1−D of 0.55 and mean of 1.08), while the 1−D and of the LCD site were 0.58 and 1.15, respectively. These observations highlight that, although the annualized planktonic foraminifera flux was similar between the Gulf of Lions (for the LCD site) and the Sicily Channel (Fig. 7), the assemblage in the latter site was significantly more diverse regarding species composition. The composition of the annual planktonic foraminifera population of the different species showed some differences between the sites compared here. In the Levantine Basin, the majority of the planktonic foraminifera population consisted of surface symbiont-bearing species, such as G. ruber, G. ruber (pink), G. rubescens, G. tenella, and O. universa, which are well adapted to the ultraoligotrophic conditions (Lombard et al., 2011; Schiebel and Hemleben, 2017). The latter species represented 96 % of the total planktonic foraminifera in the Levantine Basin, while the same species in the Sicily Channel accounted for around 10 % of the total individuals (Fig. 7). Note that both G. rubescens and G. tenella are regarded as small-sized species (Chernihovsky et al., 2023), and their adult size is often smaller than 150 µm, so it is possible that some individuals of those species may not be recorded in our data. On the other hand, in the Gulf of Lions, the four main species were G. bulloides, N. incompta, G. inflata, and G. truncatulinoides, which represented 88 % to 95 % of the total planktonic foraminifera (Rigual-Hernández et al., 2012). These species tend to be associated with eutrophic to mesotrophic environments, which coincides with the locally enhanced primary productivity conditions of the Gulf of Lions. In the Sicily Channel, the same species accounted for 83 % of the total individuals, and, except for N. incompta, the remaining three species were also the most abundant in our samples.
Table 2Inverse Simpson (1−H) and Shannon–Weiner indexes for the mean, standard deviation (“SD”), and maximum values of the two Gulf of Lions sites (PLA and LCD), the Sicily Channel (C01, this study), and the Levantine Basin (LevBas).
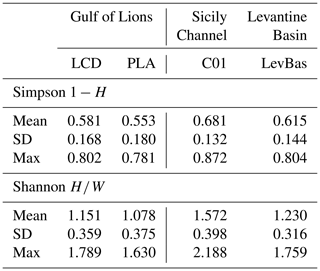
Considering the planktonic foraminifera flux patterns, the species diversity, and the most abundant planktonic foraminifera species from each of the three Mediterranean time series with which we compared our data, we interpret that, from a planktonic foraminifera population point of view, the Sicily Channel could be regarded as a transition zone and a biological corridor between the western and eastern basins.
Finally, to put our data into a global context, we compare our dataset with planktonic foraminifera data from the same-sized fraction retrieved in the Gulf of Mexico, in high latitudes of the North Atlantic Ocean, and in the gyres region of the North Atlantic Ocean. In the northern Gulf of Mexico, from 2008 to 2010, the > 150 µm PFF comprised between 0 and slightly over 800 shells m−2 d−1, with a mean value of around 250 shells m−2 d−1 (Poore et al., 2013). A total of 12 species were identified, with G. truncatulinoides, G. ruber (pink), and N. dutertrei as the most abundant species recorded. On the other hand, in the high latitudes of the Atlantic Ocean, Wolfteich (1994) showed that the PFF oscillated between 0 and around 5000 shells m−2 d−1 for a mean value of 800 shells m−2 d−1, while G. bulloides and N. incompta were the most abundant species. Although the latter work only focused on the most abundant species, additional work has documented more than 20 species in the vicinity of the North Atlantic gyres (Salmon et al., 2015) but only around 3 to 4 in the high latitudes. This highlights that, from a planktonic foraminifera population point of view on a wider scale, the Sicily Channel displayed a higher planktonic foraminifera flux and species richness compared to the tropical to subtropical Gulf of Mexico and to the high latitudes of the North Atlantic but lower values compared to the North Atlantic gyres region.
5.5 Recent planktonic foraminifera assemblage comparison with seabed sediment
The Mediterranean Sea is often referred to as a climate change hotspot and a “laboratory basin”, where many global environmental trends are amplified (Bethoux et al., 1999). In particular, ocean warming is expected to exceed the global average (Hassoun et al., 2022, 2015; Lazzari et al., 2014), and it is regarded as a specially sensitive zone of the ocean to acidification due to the fast turnover of its waters and the penetration of anthropogenic CO2 (Bethoux et al., 1999; Schneider et al., 2007). One of the main questions about planktonic foraminifera concerns the way they are going to react to the ongoing climate change in the global ocean (Jonkers and Kučera, 2015; Schiebel and Hemleben, 2017). Previous work suggests that global communities of planktonic foraminifera have already been affected by environmental change since the onset of industrialization (Jonkers et al., 2019). Moreover, recent work has shown that the calcification of several planktonic foraminifera species has decreased during the industrial era in the northwestern Mediterranean (Béjard et al., 2023). Therefore, we aim to assess if modern planktonic foraminifera communities dwelling in the Sicily Channel differ from their pre-industrial counterparts. To do so, next, we compare the annual integrated assemblages collected by the sediment trap in the C01 mooring line with the ones from a set of core tops, two box cores, and BONGO nets retrieved in the vicinity of the studied zone (see Sect. 3.5).
As planktonic foraminifera are a group of calcifying plankton, when comparing sediment trap and seabed sediment data, the possible role of calcite dissolution must be discussed. Firstly, the Mediterranean Sea is supersaturated with respect to calcite (Álvarez et al., 2014; Millero et al., 1979), and the depth of the studied material is substantially shallower than the calcite saturation horizon (Álvarez et al., 2014). Secondly, recent work suggests that calcite experiences little to negligible changes in the water column and burial in recent sediments (Béjard et al., 2023; Pallacks et al., 2023). All this evidence suggests that dissolution played a negligible role in the preservation of planktonic foraminifera preserved in the sediment record in the study region.
The core tops used for comparison were part of the MARGO database (see Sect. 3.5 for more details). Note that seabed sediment was taken from MARGO sites 3735 to 3739 using a trigger weight corer (Thunell, 1978). However, samples 3658, 3672, and 3673 were retrieved using a piston corer (Hayes et al., 2005). Generally, sampling with the trigger weight method is regarded as retrieving less mixed and disturbed sediment than the piston or box corer sampling methods (Skinner and McCave, 2003; Wu et al., 2020). Therefore, the foraminifera assemblages from the core tops may likely represent a mix of Holocene populations rather than exclusively modern assemblages, although the lack of dating control makes it impossible to determine the exact date of the core-top assemblages.
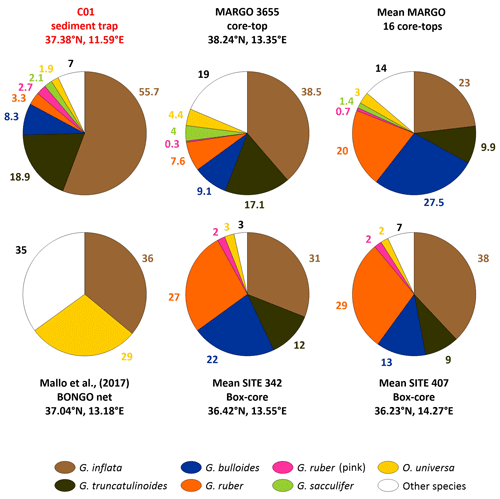
Figure 8Comparison of the relative abundance of the planktonic foraminifera from the sediment trap and seabed sediment. From top left to bottom right: the settling assemblage from the sediment, depicted in red; MARGO site 3655, which corresponds to the lowest squared-chord distance; the mean relative abundance of all MARGO sites included in this study (see Supplement); the results from the BONGO net retrieved in the Sicily Channel from Mallo et al. (2017); and the mean abundances (see Sect. 3.5) from the two sites presented in Incarbona et al. (2019), sites 342 and 407.
Samples from sites 342 and 407, studied by Incarbona et al. (2019), were retrieved with a box corer. A total of 23 and 24 samples were analysed in the latter work, respectively. The advantage of comparing the C01 assemblages with those of Incarbona et al. (2019) is the availability of high-resolution 210Pb chronology. The ages ranged from 1718 to 1962 CE for site 342 and from 1558 to 1994 CE for site 407. Therefore, we present a comparison with the mean relative abundance of the main planktonic foraminifera species from all the samples available (Fig. 8).
Finally, to provide a more complete snapshot of the surface assemblages, we also include the abundances from Mallo et al. (2017) that were collected with a BONGO net during spring 2013 in the axis of the Sicily Channel (Fig. 8).
Table 3MARGO core tops analysed, their latitude and longitude, and the squared-chord distance (SCD) between the sediment trap in the C01 mooring line and the core tops in the MARGO database. The complete SCD for all sites can be found in the Supplement.

In terms of planktonic foraminifera assemblage composition, major differences were observed between the different seabed sediment datasets (Fig. 8). Overall, the settling population from the C01 mooring line appeared to be closer to the assemblages from sites 342 and 407 (Fig. 8) than to the mean from the MARGO database (see Supplement). The most evident observation relies on the shift in the dominant species when comparing the settling population with sites 342 and 407, the BONGO net, and the core-top assemblages (Fig. 8). As described previously, G. inflata dominated the assemblages collected by the sediment trap (Table 1). This is also the case for sites 342 and 407 and for the BONGO net (Fig. 8). However, G. bulloides was the best-represented species in the core tops from the MARGO database. Also, the second most abundant species varied across the datasets: G. ruber in sites 342 and 407, O. universa in the BONGO nets, and G. inflata in the MARGO core tops, with abundances around 27 %–29 %, 29 %, and 27.5 %, respectively. Interestingly, the abundance of G. truncatulinoides was significantly lower in the seabed datasets and absent in the BONGO nets, highlighting the deep aspect of its ecology (Fig. 8). On the other hand, the “other species” category, which consists of minor taxa such as G. rubescens, G. siphonifera, and G. calida (amongst others), played a more significant role in the MARGO core-top and BONGO net assemblages, reaching abundances up to 26 % (Fig. 8), while, in sites 342 and 407, these species abundances are similar to those of the sediment trap.
These results lead to several observations. Firstly, concerning the seabed sediment comparison, the sediment trap assemblage is closer to sites 342 and 407 than to the MARGO database core tops. The comparison with the surface BONGO nets shows that, although the dominant species are the same (i.e. G. inflata), the influence of O. universa and of the overall diversity is less important in surface waters. This highlights the complexity of the Sicily Channel configuration and the differences between the surface (BONGO nets), the water column (sediment trap), and the seabed sediment (MARGO database and sites 342 and 407) regarding the planktonic foraminifera populations. Secondly, the seabed sediment planktonic foraminifera populations showed a reduced influence of deep-dwelling species (except G. inflata in sites 342 and 407) and a more pronounced influence of both eutrophic and oligotrophic species. These eutrophic species (such as G. bulloides and N. incompta) are associated with MAW and western basins in the modern Mediterranean Sea, while the more oligotrophic taxa (G. ruber, G. rubescens, G. calida, etc.) are regarded as being abundant in the easternmost part of the basin (Azibeiro et al., 2023). As noted previously, although the settling assemblage differs to the ones from the seabed sediment, it is more similar to sites 342 and 407 than to the MARGO database core tops. Also, the 210Pb chronology available for sites 342 and 407 covers the years 1558 to 1994 CE (Incarbona et al., 2019). A possible interpretation of these results is that the MAW influence in the basin may have shifted. Instead of bringing rich and eutrophic waters that would allow the development of opportunistic species, nowadays, it brings more mesotrophic water masses that favour the development of deep dwellers in the Sicily Channel. On the other hand, this could also lead to the assumption of a reduced eastward and LIW influence in the present day, as seen by the significantly lower abundance of oligotrophic species in the settling assemblages. Also, a change in the environmental conditions could lead to the increase in deep dwellers in substitution of eutrophic species such as G. bulloides. As described previously, the Mediterranean Sea has already been described as a climate change “hotspot”; therefore the already documented ocean warming and the consequent stratification (Malanotte-Rizzoli et al., 2014; Siokou-Frangou et al., 2010) could have led to unfavourable conditions for several taxa. A decrease in the primary production might have caused a shift in the dominance of the opportunistic G. bulloides by G. inflata. As described previously, G. bulloides shows a high affinity for high-productivity environments, while deep dwellers, such as G. inflata and G. truncatulinoides, tend to prefer mesotrophic and stratified waters. Finally, note that the high abundance of G. bulloides in the seabed sediment could also be the result of punctual high-productivity events. In the Alboran Sea, during upwelling events, large numbers of G. bulloides are deposited in the seabed and dominate the assemblages, which reduces the relative abundance of other mesotrophic taxa (Bárcena et al., 2004; Hernández-Almeida et al., 2011). Then, multiple recurring high-productivity events occurring over time in the Sicily Channel could explain the amount of G. bulloides in both the MARGO core tops and sites 342 and 407. In that sense, the recent warming and stratification of the Mediterranean could explain the recent trend in the planktonic foraminifera population registered by the sediment trap. However, in that case, species such as G. ruber and other oligotrophic species should at least be as well represented as they are in the seabed sediment. Alternatively, this could imply a change in the intensity of the water mass flow, such as an increased mesotrophic MAW influence and a reduced oligotrophic LIW influence.
Additionally, from a chronological point of view, we propose that the main assemblage change between the settling and the seabed sediment assemblages (i.e. the dominance of G. inflata) took place during the late Holocene but preceded the industrial period. The dates of Incarbona et al. (2019) show that, overall, since 1558 CE, G. inflata has dominated the samples. Also, the chronology in the work of Margaritelli et al. (2020), coupled with the abundances presented therein, shows that, since the Little Ice Age, the most dominant species in the western Sicily Channel has been G. inflata, followed by G. ruber and G. bulloides. This further confirms that G. inflata dominated the seabed sediment in the late Holocene but also that the shift in the secondary species (i.e. G. truncatulinoides instead of G. ruber and G. bulloides) is rather recent. Also, we assume that the discrepancy in the MARGO core-top sample is the result of the low temporal resolution.
To document the differences between the assemblage in the C01 mooring line and the MARGO database core tops, we hereby analyse the SCD between the annual integrated settling foraminifera assemblage of the C01 mooring line and all core tops located in the Sicily Channel (see Fig. S2). Overall, the SCD ranged between 0.27 and 1.1 (Table 3). By using a dissimilarity coefficient value of < 0.25 as a cutoff criterion (see Sect. 3.6 for more details), it can be concluded that none of the core-top assemblages can be regarded as close analogues to the C01 mooring line. The only exception might be MARGO site 3655, located around 180 km northeast of the mooring line, which displayed an SCD value of 0.27, very close to our cutoff threshold. Interestingly, from a geographical point of view, the closest site analysed (MARGO 3680) displayed a high SCD (0.89) despite being retrieved virtually in the underlying sediments beneath the C01 mooring line (Table 3). Overall, the four most similar sites (SCD < 0.6) to the settling assemblage are all located eastward, while the four most different sites (SCD > 1) are all located northward to the location of the mooring line. This highlights the geographical variability in the Sicily Channel regarding the planktonic foraminifera population and the complex oceanographic conditions. Note that, as mentioned previously, the lack of dating in these samples does not allow us to make further interpretations about the timing of planktonic foraminifera populations shifts. In addition to the lack of chronology control in these samples, no data are available for the sedimentation rate, which makes any assumption about the intensity of the hydrodynamics impossible. Finally, as mentioned earlier, the retrieval method applied for the different core tops could also be cited as a source of the differences between the MARGO core tops and the sediment trap in the C01 mooring line. While a box corer was used for sampling in sites 342 and 407 (Incarbona et al., 2019), various devices were used for the MARGO core tops, including piston and gravity cores, which are known to often experience stretching or loss of material during sediment recovery. Therefore, it is likely that the different MARGO surface sediment datasets represent different time intervals.
Taking into consideration all the uncertainties presented above, our data suggest that a change in the composition of the planktonic foraminifera assemblages took place at some stage of the late Holocene but before the onset of the industrial period. However, the available data preclude the determination of the main environmental drivers causing this change.
The C01 mooring line, located on the axis of the Sicily Channel, provided the opportunity to document the planktonic foraminifera population on an interannual scale. We analysed 19 samples that covered the time span between November 2013 and October 2014. A total of 3723 individuals and 15 different species were identified. G. inflata, G. truncatulinoides, G. bulloides, G. ruber, and G. ruber (pink) were the five most abundant species, representing 56 %, 19 %, 8 %, 3.5 %, and 3 % of the total foraminifera. The remaining species represented less than 5 % of the total individuals. Total planktonic foraminifera flux ranged between 44 and 1890 shells m−2 d−1; higher values were reached during spring, while values were lower during summer. Our data indicate that the planktonic foraminifera fluxes mainly reflect the oceanographic configuration of the Sicily Channel and its seasonal surface circulation variability. During winter and spring, a stronger eastward advection favours the MAW entrance in the Sicily Channel, allowing cool and nutrient-enriched waters to enter the Channel. This results in an increased planktonic foraminifera flux and a higher presence of G. inflata, G. truncatulinoides, and G. bulloides, which are taxa associated with the western basin. On the other hand, during summer, the eastward advection is reduced and the LIW dominates the water column, favouring the increase in species associated with the eastern basin, such as G. ruber and G. ruber (pink). Our correlation data with both SST and chlorophyll a showed that G. inflata was associated with cool and nutrient-rich waters. In contrast, both G. ruber species were associated with warm and oligotrophic waters, which agrees with their ecology. Surprisingly, no significant trends were identified for either G. truncatulinoides or G. bulloides. As the G. bulloides flux increased coincidently with the benthic foraminifera one, we considered that this species might have a resuspended origin. In comparison with integrated annual data from other sediment trap experiments conducted in different regions of the Mediterranean Basin, our flux and diversity data indicated that the Sicily Channel can be regarded as a transitional zone with regard to planktonic foraminifera populations: annualized fluxes were lower compared to the westernmost Alboran Sea but higher than in the easternmost Levantine Basin. However, the Sicily Channel exhibited the highest diversity values across all the sites analysed, highlighting the influence of both the western and eastern basins. Finally, the planktonic foraminifera assemblages from the sediment trap were also compared with seabed sediment assemblages. Overall, both eutrophic and oligotrophic taxa were more abundant in the seabed sediment; however, G. inflata dominated the assemblages in the closest samples to the sediment trap location. Our dataset was similar to the assemblages from sites 342 and 407 (Incarbona et al., 2019) but different to the ones from the MARGO core tops. This is likely due to the fact that they represented different time periods. Finally, the high-resolution chronology from sites 342 and 407 allowed us to show that the planktonic foraminifera population shift likely developed during the late Holocene prior to the industrial period. However, the causes of this shift remain uncertain, and our results call for increasing the monitoring of planktonic foraminifera populations and accentuating the comparisons between recent and seabed sediment assemblages in the Mediterranean to determine if the trends suggested by our data are the result of the recent environmental change.
All data used in this study are presented in the Supplement and are available online at https://doi.org/10.17632/tp4v6hm7dc.1 (Bejard, 2023; Béjard et al., 2023).
The supplement related to this article is available online at: https://doi.org/10.5194/bg-21-4051-2024-supplement.
ASRH, FJS, and TMB designed the study. JPT designed Fig. 1 and contributed to planktonic foraminifera identification and imaging. ASV and ILC provided the JERICO C01 sediment trap samples and led the sample processing. TMB led the microscopy and image analysis, the foraminifera study, and the statistical analysis and wrote the paper with feedback from all authors.
The contact author has declared that none of the authors has any competing interests.
Publisher's note: Copernicus Publications remains neutral with regard to jurisdictional claims made in the text, published maps, institutional affiliations, or any other geographical representation in this paper. While Copernicus Publications makes every effort to include appropriate place names, the final responsibility lies with the authors.
The authors would like to thank Aidan Hunter from the BAS (Cambridge) for the statistical analysis inputs and Francesca Bulian (University of Groningen) for benthic foraminifera identification support.
This research has been supported by the Ministerio de Ciencia e Innovación (grant nos. RTI2018-099489-B-100 and PID2021-128322NB-100); the project BASELINE (Andrés Rigual-Hernández) through the Ministerio de Ciencia, Innovación y Universidades (grant no. PID2021-126495NB-741 C33); the Direcció General de Recerca, Generalitat de Catalunya (grant no. 2021 SGR 01195); and the FP7 Capacities (grant no. 262584) supported by ISMAR CNR.
This paper was edited by Julia Uitz and reviewed by two anonymous referees.
Aldridge, D., Beer, C. J., and Purdie, D. A.: Calcification in the planktonic foraminifera Globigerina bulloides linked to phosphate concentrations in surface waters of the North Atlantic Ocean, Biogeosciences, 9, 1725–1739, https://doi.org/10.5194/bg-9-1725-2012, 2012.
Álvarez, M., Sanleón-Bartolomé, H., Tanhua, T., Mintrop, L., Luchetta, A., Cantoni, C., Schroeder, K., and Civitarese, G.: The CO2 system in the Mediterranean Sea: a basin wide perspective, Ocean Sci., 10, 69–92, https://doi.org/10.5194/os-10-69-2014, 2014.
Astraldi, M., Gasparini, G. P., Gervasio, L., and Salusti, E.: Dense Water Dynamics along the Channel of Sicily (Mediterranean Sea), J. Phys. Oceanogr., 31, 3457–3475, https://doi.org/10.1175/1520-0485(2001)031<3457:DWDATS>2.0.CO;2, 2001.
Astraldi, M., Gasparini, G. P., Vetrano, A., and Vignudelli, S.: Hydrographic characteristics and interannual variability of water masses in the central Mediterranean: a sensitivity test for long-term changes in the Mediterranean Sea, Deep-Sea Res. Pt. I, 49, 661–680, https://doi.org/10.1016/S0967-0637(01)00059-0, 2002.
Avnaim-Katav, S., Herut, B., Rahav, E., Katz, T., Weinstein, Y., Alkalay, R., Berman-Frank, I., Zlatkin, O., and Almogi-Labin, A.: Sediment trap and deep sea coretop sediments as tracers of recent changes in planktonic foraminifera assemblages in the southeastern ultra-oligotrophic Levantine Basin, Deep-Sea Res. Pt. II, 171, 104669, https://doi.org/10.1016/j.dsr2.2019.104669, 2020.
Azibeiro, L. A., Kučera, M., Jonkers, L., Cloke-Hayes, A., and Sierro, F. J.: Nutrients and hydrography explain the composition of recent Mediterranean planktonic foraminiferal assemblages, Mar. Micropaleontol., 179, 102201, https://doi.org/10.1016/j.marmicro.2022.102201, 2023.
Balestra, B., Grunert, P., Ausin, B., Hodell, D., Flores, J.-A., Alvarez-Zarikian, C. A., Hernandez-Molina, F. J., Stow, D., Piller, W. E., and Paytan, A.: Coccolithophore and benthic foraminifera distribution patterns in the Gulf of Cadiz and Western Iberian Margin during Integrated Ocean Drilling Program (IODP) Expedition 339, J. Marine Syst., 170, 50–67, https://doi.org/10.1016/j.jmarsys.2017.01.005, 2017.
Bárcena, M. A., Flores, J. A., Sierro, F. J., Pérez-Folgado, M., Fabres, J., Calafat, A., and Canals, M.: Planktonic response to main oceanographic changes in the Alboran Sea (Western Mediterranean) as documented in sediment traps and surface sediments, Mar. Micropaleontol., 53, 423–445, https://doi.org/10.1016/j.marmicro.2004.09.009, 2004.
Barker, S. and Elderfield, H.: Foraminiferal Calcification Response to Glacial-Interglacial Changes in Atmospheric CO 2, Science, 297, 833–836, https://doi.org/10.1126/science.1072815, 2002.
Bé, A. W. H., Hutson, W. H., and Be, A. W. H.: Ecology of Planktonic Foraminifera and Biogeographic Patterns of Life and Fossil Assemblages in the Indian Ocean, Micropaleontology, 23, 369–414, https://doi.org/10.2307/1485406, 1977.
Beer, C. J., Schiebel, R., and Wilson, P. A.: Testing planktonic foraminiferal shell weight as a surface water [CO] proxy using plankton net samples, Geology, 38, 103–106, https://doi.org/10.1130/G30150.1, 2010.
Bejard, T.: Béjard et al., 2023. Supplementary data. Planktic foraminifera population in a modern Central Mediterranean Sea sediment trap compared to basin-wide and seafloor assemblages, Mendeley Data, V1 [data set], https://doi.org/10.17632/tp4v6hm7dc.1, 2023.
Béjard, T. M., Rigual-Hernández, A. S., Flores, J. A., Tarruella, J. P., Durrieu de Madron, X., Cacho, I., Haghipour, N., Hunter, A., and Sierro, F. J.: Calcification response of planktic foraminifera to environmental change in the western Mediterranean Sea during the industrial era, Biogeosciences, 20, 1505–1528, https://doi.org/10.5194/bg-20-1505-2023, 2023.
Béranger, K., Mortier, L., Gasparini, G.-P., Gervasio, L., Astraldi, M., and Crépon, M.: The dynamics of the Sicily Channel: a comprehensive study from observations and models, Deep-Sea Res. Pt. II, 51, 411–440, https://doi.org/10.1016/j.dsr2.2003.08.004, 2004.
Bergamasco, A. and Malanotte-Rizzoli, P.: The circulation of the Mediterranean Sea: a historical review of experimental investigations, Advances in Oceanography and Limnology, 1, 11–28, https://doi.org/10.1080/19475721.2010.491656, 2010.
Bethoux, J. P., Gentili, B., Morin, P., Nicolas, E., Pierre, C., and Ruiz-Pino, D.: The Mediterranean Sea: a miniature ocean for climatic and environmental studies and a key for the climatic functioning of the North Atlantic, Progr. Oceanogr., 44, 131–146, https://doi.org/10.1016/S0079-6611(99)00023-3, 1999.
Bijma, J., Faber, W. W., and Hemleben, C.: Temperature and salinity limits for growth and survival of some planktonic foraminifers in laboratory cultures, J. Foramin. Res., 20, 95–116, https://doi.org/10.2113/gsjfr.20.2.95, 1990.
Bijma, J., Hönisch, B., and Zeebe, R. E.: Impact of the ocean carbonate chemistry on living foraminiferal shell weight: Comment on “Carbonate ion concentration in glacial-age deep waters of the Caribbean Sea” by W. S. Broecker and E. Clark: COMMENT, Geochem. Geophy. Geosy., 3, 1–7, https://doi.org/10.1029/2002GC000388, 2002.
Bouzinac, C., Font, J., and Millot, C.: Hydrology and currents observed in the channel of Sardinia during the PRIMO-1 experiment from November 1993 to October 1994, J. Marine Syst., 20, 333–355, https://doi.org/10.1016/S0924-7963(98)00074-8, 1999.
Chapman, M. R.: Seasonal production patterns of planktonic foraminifera in the NE Atlantic Ocean: Implications for paleotemperature and hydrographic reconstructions: CURRENTS, Paleoceanography, 25, PA1101, https://doi.org/10.1029/2008PA001708, 2010.
Chernihovsky, N., Torfstein, A., and Almogi-Labin, A.: Seasonal flux patterns of planktonic foraminifera in a deep, oligotrophic, marginal sea: Sediment trap time series from the Gulf of Aqaba, northern Red Sea, Deep-Sea Res. Pt. I, 140, 78–94, https://doi.org/10.1016/j.dsr.2018.08.003, 2018.
Chernihovsky, N., Torfstein, A., and Almogi-Labin, A.: Daily timescale dynamics of planktonic foraminifera shell-size distributions, Front. Mar. Sci., 10, 1126398, https://doi.org/10.3389/fmars.2023.1126398, 2023.
Cisneros, M., Cacho, I., Frigola, J., Canals, M., Masqué, P., Martrat, B., Casado, M., Grimalt, J. O., Pena, L. D., Margaritelli, G., and Lirer, F.: Sea surface temperature variability in the central-western Mediterranean Sea during the last 2700 years: a multi-proxy and multi-record approach, Clim. Past, 12, 849–869, https://doi.org/10.5194/cp-12-849-2016, 2016.
de Moel, H., Ganssen, G. M., Peeters, F. J. C., Jung, S. J. A., Kroon, D., Brummer, G. J. A., and Zeebe, R. E.: Planktic foraminiferal shell thinning in the Arabian Sea due to anthropogenic ocean acidification?, Biogeosciences, 6, 1917–1925, https://doi.org/10.5194/bg-6-1917-2009, 2009.
D'Ortenzio, F.: On the trophic regimes of the Mediterranean Sea: a satellite analysis, Biogeosciences, 6, 139–148, https://doi.org/10.5194/bg-6-139-2009, 2009.
Ducassou, E., Hassan, R., Gonthier, E., Duprat, J., Hanquiez, V., and Mulder, T.: Biostratigraphy of the last 50 kyr in the contourite depositional system of the Gulf of Cádiz, Mar. Geol., 395, 285–300, https://doi.org/10.1016/j.margeo.2017.09.014, 2018.
Durrieu de Madron, X., Houpert, L., Puig, P., Sanchez-Vidal, A., Testor, P., Bosse, A., Estournel, C., Somot, S., Bourrin, F., Bouin, M. N., Beauverger, M., Beguery, L., Calafat, A., Canals, M., Cassou, C., Coppola, L., Dausse, D., D'Ortenzio, F., Font, J., Heussner, S., Kunesch, S., Lefevre, D., Le Goff, H., Martín, J., Mortier, L., Palanques, A., and Raimbault, P.: Interaction of dense shelf water cascading and open-sea convection in the northwestern Mediterranean during winter 2012: shelf cascading and open-sea convection, Geophys. Res. Lett., 40, 1379–1385, https://doi.org/10.1002/grl.50331, 2013.
Fox, L., Stukins, S., Hill, T., and Miller, C. G.: Quantifying the Effect of Anthropogenic Climate Change on Calcifying Plankton, Sci. Rep., 10, 1620, https://doi.org/10.1038/s41598-020-58501-w, 2020.
Garcia-Solsona, E., Pena, L. D., Paredes, E., Pérez-Asensio, J. N., Quirós-Collazos, L., Lirer, F., and Cacho, I.: Rare earth elements and Nd isotopes as tracers of modern ocean circulation in the central Mediterranean Sea, Progr. Oceanogr., 185, 102340, https://doi.org/10.1016/j.pocean.2020.102340, 2020.
Gasparini, G. P., Smeed, D. A., Alderson, S., Sparnocchia, S., Vetrano, A., and Mazzola, S.: Tidal and subtidal currents in the Strait of Sicily, J. Geophys. Res., 109, 2003JC002011, https://doi.org/10.1029/2003JC002011, 2004.
Gasparini, G. P., Ortona, A., Budillon, G., Astraldi, M., and Sansone, E.: The effect of the Eastern Mediterranean Transient on the hydrographic characteristics in the Channel of Sicily and in the Tyrrhenian Sea, Deep-Sea Res. Pt. I, 52, 915–935, https://doi.org/10.1016/j.dsr.2005.01.001, 2005.
Gaudy, R., Youssara, F., Diaz, F., and Raimbault, P.: Biomass, metabolism and nutrition of zooplankton in the Gulf of Lions (NW Mediterranean), Oceanol. Acta, 26, 357–372, https://doi.org/10.1016/S0399-1784(03)00016-1, 2003.
Grifoll, M., Cerralbo, P., Guillén, J., Espino, M., Hansen, L. B., and Sánchez-Arcilla, A.: Characterization of bottom sediment resuspension events observed in a micro-tidal bay, Ocean Sci., 15, 307–319, https://doi.org/10.5194/os-15-307-2019, 2019.
Hassoun, A. E. R., Gemayel, E., Krasakopoulou, E., Goyet, C., Abboud-Abi Saab, M., Guglielmi, V., Touratier, F., and Falco, C.: Acidification of the Mediterranean Sea from anthropogenic carbon penetration, Deep-Sea Res. Pt. I, 102, 1–15, https://doi.org/10.1016/j.dsr.2015.04.005, 2015.
Hassoun, A. E. R., Bantelman, A., Canu, D., Comeau, S., Galdies, C., Gattuso, J.-P., Giani, M., Grelaud, M., Hendriks, I. E., Ibello, V., Idrissi, M., Krasakopoulou, E., Shaltout, N., Solidoro, C., Swarzenski, P. W., and Ziveri, P.: Ocean acidification research in the Mediterranean Sea: Status, trends and next steps, Front. Mar. Sci., 9, 892670, https://doi.org/10.3389/fmars.2022.892670, 2022.
Hayes, A., Kucera, M., Kallel, N., Sbaffi, L., and Rohling, E. J.: Compilation of planktonic foraminifera modern data from the Mediterranean Sea, PANGAEA, https://doi.org/10.1594/PANGAEA.227305, 2005.
Hazan, O., Silverman, J., Sisma-Ventura, G., Ozer, T., Gertman, I., Shoham-Frider, E., Kress, N., and Rahav, E.: Mesopelagic Prokaryotes Alter Surface Phytoplankton Production during Simulated Deep Mixing Experiments in Eastern Mediterranean Sea Waters, Front. Mar. Sci., 5, 1, https://doi.org/10.3389/fmars.2018.00001, 2018.
Hemleben, C.: Modern Planktonic Foraminifera, Springer New York, New York, NY, https://doi.org/10.1007/978-1-4612-3544-6, 1989.
Hernández-Almeida, I., Bárcena, M. A., Flores, J. A., Sierro, F. J., Sanchez-Vidal, A., and Calafat, A.: Microplankton response to environmental conditions in the Alboran Sea (Western Mediterranean): One year sediment trap record, Mar. Micropaleontol., 78, 14–24, https://doi.org/10.1016/j.marmicro.2010.09.005, 2011.
Heussner, S., Ratti, C., and Carbonne, J.: The PPS 3 time-series sediment trap and the trap sample processing techniques used during the ECOMARGE experiment, Cont. Shelf Res., 10, 943–958, https://doi.org/10.1016/0278-4343(90)90069-X, 1990.
Heussner, S., Durrieu de Madron, X., Calafat, A., Canals, M., Carbonne, J., Delsaut, N., and Saragoni, G.: Spatial and temporal variability of downward particle fluxes on a continental slope: Lessons from an 8-yr experiment in the Gulf of Lions (NW Mediterranean), Mar. Geol., 234, 63–92, https://doi.org/10.1016/j.margeo.2006.09.003, 2006.
Houpert, L., Durrieu de Madron, X., Testor, P., Bosse, A., D'Ortenzio, F., Bouin, M. N., Dausse, D., Le Goff, H., Kunesch, S., Labaste, M., Coppola, L., Mortier, L., and Raimbault, P.: Observations of open-ocean deep convection in the northwestern Mediterranean Sea: Seasonal and interannual variability of mixing and deep water masses for the 2007–2013 Period, J. Geophys. Res.-Oceans, 121, 8139–8171, https://doi.org/10.1002/2016JC011857, 2016.
Huertas, I. E., Ríos, A. F., García-Lafuente, J., Navarro, G., Makaoui, A., Sánchez-Román, A., Rodriguez-Galvez, S., Orbi, A., Ruíz, J., and Pérez, F. F.: Atlantic forcing of the Mediterranean oligotrophy, Global Biogeochem. Cy., 11, GB004167, https://doi.org/10.1029/2011GB004167, 2012.
Incarbona, A., Sprovieri, M., Lirer, F., and Sprovieri, R.: Surface and deep water conditions in the Sicily channel (central Mediterranean) at the time of sapropel S5 deposition, Palaeogeogr. Palaeocl., 306, 243–248, https://doi.org/10.1016/j.palaeo.2011.04.030, 2011.
Incarbona, A., Jonkers, L., Ferraro, S., Sprovieri, R., and Tranchida, G.: Sea Surface Temperatures and Paleoenvironmental Variability in the Central Mediterranean During Historical Times Reconstructed Using Planktonic Foraminifera, Paleoceanog. Paleoclimatol., 34, 394–408, https://doi.org/10.1029/2018PA003529, 2019.
Jonkers, L. and Kučera, M.: Global analysis of seasonality in the shell flux of extant planktonic Foraminifera, Biogeosciences, 12, 2207–2226, https://doi.org/10.5194/bg-12-2207-2015, 2015.
Jonkers, L., Hillebrand, H., and Kucera, M.: Global change drives modern plankton communities away from the pre-industrial state, Nature, 570, 372–375, https://doi.org/10.1038/s41586-019-1230-3, 2019.
Jouini, M., Béranger, K., Arsouze, T., Beuvier, J., Thiria, S., Crépon, M., and Taupier-Letage, I.: The Sicily Channel surface circulation revisited using a neural clustering analysis of a high-resolution simulation, J. Geophys. Res.-Oceans, 121, 4545–4567, https://doi.org/10.1002/2015JC011472, 2016.
Kemle-von Mücke, S. and Oberhänsli, H.: The Distribution of Living Planktonic Foraminifera in Relation to Southeast Atlantic Oceanography, in: Use of Proxies in Paleoceanography, edited by: Fischer, G. and Wefer, G., Springer Berlin Heidelberg, Berlin, Heidelberg, 91–115, https://doi.org/10.1007/978-3-642-58646-0_3, 1999.
Kroeker, K. J., Kordas, R. L., Crim, R., Hendriks, I. E., Ramajo, L., Singh, G. S., Duarte, C. M., and Gattuso, J.: Impacts of ocean acidification on marine organisms: quantifying sensitivities and interaction with warming, Glob. Change Biol., 19, 1884–1896, https://doi.org/10.1111/gcb.12179, 2013.
Krom, M. D., Kress, N., Brenner, S., and Gordon, L. I.: Phosphorus limitation of primary productivity in the eastern Mediterranean Sea, Limnol. Oceanogr., 36, 424–432, https://doi.org/10.4319/lo.1991.36.3.0424, 1991.
Krom, M. D., Woodward, E. M. S., Herut, B., Kress, N., Carbo, P., Mantoura, R. F. C., Spyres, G., Thingstad, T. F., Wassmann, P., Wexels-Riser, C., Kitidis, V., Law, C. S., and Zodiatis, G.: Nutrient cycling in the south east Levantine basin of the eastern Mediterranean: Results from a phosphorus starved system, Deep-Sea Res. Pt. II, 52, 2879–2896, https://doi.org/10.1016/j.dsr2.2005.08.009, 2005.
Kuroyanagi, A. and Kawahata, H.: Vertical distribution of living planktonic foraminifera in the seas around Japan, Mar. Micropaleontol., 53, 173–196, https://doi.org/10.1016/j.marmicro.2004.06.001, 2004.
Lazzari, P., Mattia, G., Solidoro, C., Salon, S., Crise, A., Zavatarelli, M., Oddo, P., and Vichi, M.: The impacts of climate change and environmental management policies on the trophic regimes in the Mediterranean Sea: Scenario analyses, J. Marine Syst., 135, 137–149, https://doi.org/10.1016/j.jmarsys.2013.06.005, 2014.
Lermusiaux, P. F. J. and Robinson, A. R.: Features of dominant mesoscale variability, circulation patterns and dynamics in the Channel of Sicily, Deep-Sea Res. Pt. I, 48, 1953–1997, https://doi.org/10.1016/S0967-0637(00)00114-X, 2001.
Lirer, F., Sprovieri, M., Vallefuoco, M., Ferraro, L., Pelosi, N., Giordano, L., and Capotondi, L.: Planktonic foraminifera as bio-indicators for monitoring the climatic changes that have occurred over the past 2000 years in the southeastern Tyrrhenian Sea, Integr. Zool., 9, 542–554, https://doi.org/10.1111/1749-4877.12083, 2014.
Lohmann, G. P. and Schweitzer, P. N.: Globorotalia truncatulinoides' Growth and chemistry as probes of the past thermocline: 1. Shell size, Paleoceanography, 5, 55–75, https://doi.org/10.1029/PA005i001p00055, 1990.
Lombard, F., Erez, J., Michel, E., and Labeyrie, L.: Temperature effect on respiration and photosynthesis of the symbiont-bearing planktonic foraminifera Globigerinoides ruber, Orbulina universa, and Globigerinella siphonifera, Limnol. Oceanogr., 54, 210–218, https://doi.org/10.4319/lo.2009.54.1.0210, 2009.
Lombard, F., Labeyrie, L., Michel, E., Bopp, L., Cortijo, E., Retailleau, S., Howa, H., and Jorissen, F.: Modelling planktic foraminifer growth and distribution using an ecophysiological multi-species approach, Biogeosciences, 8, 853–873, https://doi.org/10.5194/bg-8-853-2011, 2011.
Macias, D., Cózar, A., Garcia-Gorriz, E., González-Fernández, D., and Stips, A.: Surface water circulation develops seasonally changing patterns of floating litter accumulation in the Mediterranean Sea. A modelling approach, Mar. Pollut. Bull., 149, 110619, https://doi.org/10.1016/j.marpolbul.2019.110619, 2019.
Malanotte-Rizzoli, P., Artale, V., Borzelli-Eusebi, G. L., Brenner, S., Crise, A., Gacic, M., Kress, N., Marullo, S., Ribera d'Alcalà, M., Sofianos, S., Tanhua, T., Theocharis, A., Alvarez, M., Ashkenazy, Y., Bergamasco, A., Cardin, V., Carniel, S., Civitarese, G., D'Ortenzio, F., Font, J., Garcia-Ladona, E., Garcia-Lafuente, J. M., Gogou, A., Gregoire, M., Hainbucher, D., Kontoyannis, H., Kovacevic, V., Kraskapoulou, E., Kroskos, G., Incarbona, A., Mazzocchi, M. G., Orlic, M., Ozsoy, E., Pascual, A., Poulain, P.-M., Roether, W., Rubino, A., Schroeder, K., Siokou-Frangou, J., Souvermezoglou, E., Sprovieri, M., Tintoré, J., and Triantafyllou, G.: Physical forcing and physical/biochemical variability of the Mediterranean Sea: a review of unresolved issues and directions for future research, Ocean Sci., 10, 281–322, https://doi.org/10.5194/os-10-281-2014, 2014.
Mallo, M., Ziveri, P., Mortyn, P. G., Schiebel, R., and Grelaud, M.: Low planktic foraminiferal diversity and abundance observed in a spring 2013 west–east Mediterranean Sea plankton tow transect, Biogeosciences, 14, 2245–2266, https://doi.org/10.5194/bg-14-2245-2017, 2017.
Margaritelli, G., Lirer, F., Schroeder, K., Alberico, I., Dentici, M. P., and Caruso, A.: Globorotalia truncatulinoides in Central – Western Mediterranean Sea during the Little Ice Age, Mar. Micropaleontol., 161, 101921, https://doi.org/10.1016/j.marmicro.2020.101921, 2020.
Margaritelli, G., Lirer, F., Schroeder, K., Cloke-Hayes, A., Caruso, A., Capotondi, L., Broggy, T., Cacho, I., and Sierro, F. J.: Globorotalia truncatulinoides in the Mediterranean Basin during the Middle–Late Holocene: Bio-Chronological and Oceanographic Indicator, Geosciences, 12, 244, https://doi.org/10.3390/geosciences12060244, 2022.
Marshall, B. J., Thunell, R. C., Henehan, M. J., Astor, Y., and Wejnert, K. E.: Planktonic foraminiferal area density as a proxy for carbonate ion concentration: A calibration study using the Cariaco Basin ocean time series, Paleoceanography, 28, 363–376, https://doi.org/10.1002/palo.20034, 2013.
Milker, Y. and Schmiedl, G.: A taxonomic guide to modern benthic shelf foraminifera of the western Mediterranean Sea, Palaeontol. Electron., 15, 1–134, https://doi.org/10.26879/271, 2012.
Millero, F. J., Morse, J., and Chen, C.-T.: The carbonate system in the western Mediterranean sea, Deep-Sea Res. Pt. A., 26, 1395–1404, https://doi.org/10.1016/0198-0149(79)90007-4, 1979.
Millot, C.: Mesoscale and seasonal variabilities of the circulation in the western Mediterranean, Dynam. Atmos. Oceans, 15, 179–214, https://doi.org/10.1016/0377-0265(91)90020-G, 1991.
Millot, C.: Circulation in the Western Mediterranean Sea, J. Marine Syst., 20, 423–442, https://doi.org/10.1016/S0924-7963(98)00078-5, 1999.
Millot, C. and Taupier-Letage, I.: Circulation in the Mediterranean Sea, in: The Mediterranean Sea, edited by: Saliot, A., Springer Berlin Heidelberg, Berlin, Heidelberg, vol. 5K, 29–66, https://doi.org/10.1007/b107143, 2005.
Morán, X. and Estrada, M.: Short-term variability of photosynthetic parameters and particulate and dissolved primary production in the Alboran Sea (SW Mediterranean), Mar. Ecol. Prog. Ser., 212, 53–67, https://doi.org/10.3354/meps212053, 2001.
Moy, A. D., Howard, W. R., Bray, S. G., and Trull, T. W.: Reduced calcification in modern Southern Ocean planktonic foraminifera, Nat. Geosci., 2, 276–280, https://doi.org/10.1038/ngeo460, 2009.
Navarro, G., Almaraz, P., Caballero, I., Vázquez, Á., and Huertas, I. E.: Reproduction of Spatio-Temporal Patterns of Major Mediterranean Phytoplankton Groups from Remote Sensing OC-CCI Data, Front. Mar. Sci., 4, 246, https://doi.org/10.3389/fmars.2017.00246, 2017.
Nielsen, S. N.: Numerical Ecology, Legendre P. and Legendre L., second ed., Elsevier, Amsterdam, p. 853, 1998., Ecol. Model., 132, 303–304, https://doi.org/10.1016/S0304-3800(00)00291-X, 2000.
Ortiz, J. D. and Mix, A. C.: Comparison of Imbrie-Kipp Transfer Function and modern analog temperature estimates using sediment trap and core top foraminiferal faunas, Paleoceanography, 12, 175–190, https://doi.org/10.1029/96PA02878, 1997.
Osborne, E. B., Thunell, R. C., Marshall, B. J., Holm, J. A., Tappa, E. J., Benitez-Nelson, C., Cai, W., and Chen, B.: Calcification of the planktonic foraminifera Globigerina bulloides and carbonate ion concentration: Results from the Santa Barbara Basin, Paleoceanography, 31, 1083–1102, https://doi.org/10.1002/2016PA002933, 2016.
Ozer, T., Gertman, I., Kress, N., Silverman, J., and Herut, B.: Interannual thermohaline (1979–2014) and nutrient (2002–2014) dynamics in the Levantine surface and intermediate water masses, SE Mediterranean Sea, Global Planet. Change, 151, 60–67, https://doi.org/10.1016/j.gloplacha.2016.04.001, 2017.
Pallacks, S., Ziveri, P., Schiebel, R., Vonhof, H., Rae, J. W. B., Littley, E., Garcia-Orellana, J., Langer, G., Grelaud, M., and Martrat, B.: Anthropogenic acidification of surface waters drives decreased biogenic calcification in the Mediterranean Sea, Commun. Earth Environ., 4, 301, https://doi.org/10.1038/s43247-023-00947-7, 2023.
Pinardi, N., Zavatarelli, M., Adani, M., Coppini, G., Fratianni, C., Oddo, P., Simoncelli, S., Tonani, M., Lyubartsev, V., Dobricic, S., and Bonaduce, A.: Mediterranean Sea large-scale low-frequency ocean variability and water mass formation rates from 1987 to 2007: A retrospective analysis, Progr. Oceanogr., 132, 318–332, https://doi.org/10.1016/j.pocean.2013.11.003, 2015.
Poore, R. Z., Tedesco, K. A., and Spear, J. W.: Seasonal Flux and Assemblage Composition of Planktonic Foraminifers from a Sediment-Trap Study in the Northern Gulf of Mexico, J. Coast. Res., 63, 6–19, https://doi.org/10.2112/SI63-002.1, 2013.
Prell, W.: The Stability of Low-Latitude Sea-Surface Temperatures, an Evaluation of the CLIMAP Reconstruction with Emphasis on the Positive SST Anomalies, Report No. TR025, US Department of Energy, 1985.
Pujol, C. and Grazzini, C. V.: Distribution patterns of live planktonic foraminifers as related to regional hydrography and productive systems of the Mediterranean Sea, Mar. Micropaleontol., 25, 187–217, https://doi.org/10.1016/0377-8398(95)00002-I, 1995.
Raimbault, P., Pouvesle, W., Diaz, F., Garcia, N., and Sempéré, R.: Wet-oxidation and automated colorimetry for simultaneous determination of organic carbon, nitrogen and phosphorus dissolved in seawater, Mar. Chem., 66, 161–169, https://doi.org/10.1016/S0304-4203(99)00038-9, 1999.
Rebotim, A., Voelker, A. H. L., Jonkers, L., Waniek, J. J., Meggers, H., Schiebel, R., Fraile, I., Schulz, M., and Kucera, M.: Factors controlling the depth habitat of planktonic foraminifera in the subtropical eastern North Atlantic, Biogeosciences, 14, 827–859, https://doi.org/10.5194/bg-14-827-2017, 2017.
Retailleau, S., Schiebel, R., and Howa, H.: Population dynamics of living planktonic foraminifers in the hemipelagic southeastern Bay of Biscay, Mar. Micropaleontol., 80, 89–100, https://doi.org/10.1016/j.marmicro.2011.06.003, 2011.
Rigual-Hernández, A. S., Sierro, F. J., Bárcena, M. A., Flores, J. A., and Heussner, S.: Seasonal and interannual changes of planktonic foraminiferal fluxes in the Gulf of Lions (NW Mediterranean) and their implications for paleoceanographic studies: Two 12-year sediment trap records, Deep-Sea Res. Pt. I, 66, 26–40, https://doi.org/10.1016/j.dsr.2012.03.011, 2012.
Robinson, A. R. and Golnaraghi, M.: The Physical and Dynamical Oceanography of the Mediterranean Sea, in: Ocean Processes in Climate Dynamics: Global and Mediterranean Examples, edited by: Malanotte-Rizzoli, P. and Robinson, A. R., Springer Netherlands, Dordrecht, 255–306, https://doi.org/10.1007/978-94-011-0870-6_12, 1994.
Robinson, A. R., Sellschopp, J., Warn-Varnas, A., Leslie, W. G., Lozano, C. J., Haley Jr., P. J., Anderson, L. A., and Lermusiaux, P. F. J.: The Atlantic Ionian Stream, J. Marine Syst., 20, 129–156, https://doi.org/10.1016/S0924-7963(98)00079-7, 11999.
Salmon, K. H., Anand, P., Sexton, P. F., and Conte, M.: Upper ocean mixing controls the seasonality of planktonic foraminifer fluxes and associated strength of the carbonate pump in the oligotrophic North Atlantic, Biogeosciences, 12, 223–235, https://doi.org/10.5194/bg-12-223-2015, 2015.
Schiebel, R.: Planktonic foraminiferal sedimentation and the marine calcite budget, Global Biogeochem. Cy., 16, 3-1–3-21, https://doi.org/10.1029/2001GB001459, 2002.
Schiebel, R. and Hemleben, C.: Modern planktonic foraminifera, Paläontol. Z., 79, 135–148, https://doi.org/10.1007/BF03021758, 2005.
Schiebel, R. and Hemleben, C.: Planktonic Foraminifers in the Modern Ocean, Springer Berlin Heidelberg, Berlin, Heidelberg, https://doi.org/10.1007/978-3-662-50297-6, 2017.
Schiebel, R., Waniek, J., Bork, M., and Hemleben, C.: Planktonic foraminiferal production stimulated by chlorophyll redistribution and entrainment of nutrients, Deep-Sea Res. Pt. I, 48, 721–740, https://doi.org/10.1016/S0967-0637(00)00065-0, 2001.
Schiebel, R., Waniek, J., Zeltner, A., and Alves, M.: Impact of the Azores Front on the distribution of planktic foraminifers, shelled gastropods, and coccolithophorids, Deep-Sea Res. Pt. II, 49, 4035–4050, https://doi.org/10.1016/S0967-0645(02)00141-8, 2002.
Schiebel, R., Zeltner, A., Treppke, U. F., Waniek, J. J., Bollmann, J., Rixen, T., and Hemleben, C.: Distribution of diatoms, coccolithophores and planktonic foraminifers along a trophic gradient during SW monsoon in the Arabian Sea, Mar. Micropaleontol., 51, 345–371, https://doi.org/10.1016/j.marmicro.2004.02.001, 2004.
Schmidt, D. N., Lazarus, D., Young, J. R., and Kucera, M.: Biogeography and evolution of body size in marine plankton, Earth-Sci. Rev., 78, 239–266, https://doi.org/10.1016/j.earscirev.2006.05.004, 2006.
Schneider, A., Wallace, D. W. R., and Körtzinger, A.: Alkalinity of the Mediterranean Sea, Geophys. Res. Lett., 34, 2006GL028842, https://doi.org/10.1029/2006GL028842, 2007.
Schroeder, K., Gasparini, G. P., Borghini, M., Cerrati, G., and Delfanti, R.: Biogeochemical tracers and fluxes in the Western Mediterranean Sea, spring 2005, J. Marine Syst., 80, 8–24, https://doi.org/10.1016/j.jmarsys.2009.08.002, 2010.
Schroeder, K., Chiggiato, J., Josey, S. A., Borghini, M., Aracri, S., and Sparnocchia, S.: Rapid response to climate change in a marginal sea, Sci. Rep., 7, 4065, https://doi.org/10.1038/s41598-017-04455-5, 2017.
Siccha, M. and Kucera, M.: ForCenS, a curated database of planktonic foraminifera census counts in marine surface sediment samples, Sci. Data, 4, 170109, https://doi.org/10.1038/sdata.2017.109, 2017.
Sierro, F. J., Hodell, D. A., Curtis, J. H., Flores, J. A., Reguera, I., Colmenero‐Hidalgo, E., Bárcena, M. A., Grimalt, J. O., Cacho, I., Frigola, J., and Canals, M.: Impact of iceberg melting on Mediterranean thermohaline circulation during Heinrich events, Paleoceanography, 20, 2004PA001051, https://doi.org/10.1029/2004PA001051, 2005.
Siokou-Frangou, I., Christaki, U., Mazzocchi, M. G., Montresor, M., Ribera d'Alcalá, M., Vaqué, D., and Zingone, A.: Plankton in the open Mediterranean Sea: a review, Biogeosciences, 7, 1543–1586, https://doi.org/10.5194/bg-7-1543-2010, 2010.
Skinner, L. C. and McCave, I. N.: Analysis and modelling of gravity- and piston coring based on soil mechanics, Mar. Geol., 199, 181–204, https://doi.org/10.1016/S0025-3227(03)00127-0, 2003.
Takagi, H., Kimoto, K., Fujiki, T., Saito, H., Schmidt, C., Kucera, M., and Moriya, K.: Characterizing photosymbiosis in modern planktonic foraminifera, Biogeosciences, 16, 3377–3396, https://doi.org/10.5194/bg-16-3377-2019, 2019.
Takahashi, K. and Be, A. W. H.: Planktonic foraminifera: factors controlling sinking speeds, Deep-Sea Res. Pt. A, 31, 1477–1500, https://doi.org/10.1016/0198-0149(84)90083-9, 1984.
Thunell, R. C.: Distribution of recent planktonic foraminifera in surface sediments of the Mediterranean Sea, Mar. Micropaleontol., 3, 147–173, https://doi.org/10.1016/0377-8398(78)90003-8, 1978.
Toucanne, S., Mulder, T., Schönfeld, J., Hanquiez, V., Gonthier, E., Duprat, J., Cremer, M., and Zaragosi, S.: Contourites of the Gulf of Cadiz: A high-resolution record of the paleocirculation of the Mediterranean outflow water during the last 50 000 years, Palaeogeogr. Palaeocl., 246, 354–366, https://doi.org/10.1016/j.palaeo.2006.10.007, 2007.
Warn-Varnas, A., Sellschopp, J., Haley, P. J., Leslie, W. G., and Lozano, C. J.: Channel of Sicily water masses, Dynam. Atmos. Oceans, 29, 437–469, https://doi.org/10.1016/S0377-0265(99)00014-7, 1999.
Wilke, I., Meggers, H., and Bickert, T.: Depth habitats and seasonal distributions of recent planktonic foraminifers in the Canary Islands region (29° N) based on oxygen isotopes, Deep-Sea Res. Pt. I, 56, 89–106, https://doi.org/10.1016/j.dsr.2008.08.001, 2009.
Wolfteich, C. M.: Sattelite-derived sea surface temperature, mesoscale variability, and foraminiferal production in the North Atlantic, MSc, MIT and WHOI, Cambridge, MS, 91 pp., http://hdl.handle.net/1721.1/17355, 1994.
Wu, H., Liu, N., Peng, J., Ge, Y., and Kong, B.: Analysis and modelling on coring process of deep-sea gravity piston corer, J. Eng., 2020, 900–905, https://doi.org/10.1049/joe.2020.0077, 2020.
Zarkogiannis, S. D., Iwasaki, S., Rae, J. W. B., Schmidt, M. W., Mortyn, P. G., Kontakiotis, G., Hertzberg, J. E., and Rickaby, R. E. M.: Calcification, Dissolution and Test Properties of Modern Planktonic Foraminifera From the Central Atlantic Ocean, Front. Mar. Sci., 9, 864801, https://doi.org/10.3389/fmars.2022.864801, 2022.