the Creative Commons Attribution 4.0 License.
the Creative Commons Attribution 4.0 License.
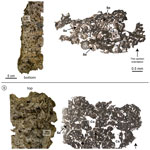
Origin and role of non-skeletal carbonate in coralligenous build-ups: new geobiological perspectives in biomineralization processes
Mara Cipriani
Carmine Apollaro
Daniela Basso
Pietro Bazzicalupo
Marco Bertolino
Valentina Alice Bracchi
Fabio Bruno
Gabriele Costa
Rocco Dominici
Alessandro Gallo
Maurizio Muzzupappa
Antonietta Rosso
Rossana Sanfilippo
Francesco Sciuto
Giovanni Vespasiano
The coralligenous build-ups located on the Mediterranean shelf in front of Marzamemi (SE Sicily, Italy) represent useful natural examples to use in studying the relationship between skeletal organisms and non-skeletal components in marine bioconstructions. Coralligenous build-ups are formed in open marine systems, and their comparison with coeval bioconstructions (biostalactites) of confined environments, like submarine caves, allows depicting the complex interactions between metazoans and microbial communities in the formations of recent bioconstructions in different Mediterranean settings. In this study, two coralligenous build-ups were characterized in terms of organisms and sediments involved in their formation. The framework mainly consists of coralline algae and subordinate bryozoans and serpulids. Sponges affect the general morphology of the bioconstructions both interacting with skeletonized organisms and through bioerosion activity. The micrite or microcrystalline calcite is present in minor amounts compared to other components that form the build-ups and consists of two types: autochthonous (in situ) and allochthonous (detrital). Fine autochthonous micrite mineralized directly inside the framework cavities and shows aphanitic or peloidal fabric, produced by organomineralization processes of soft sponge tissues and microbial metabolic activity, respectively. The detrital micrite occurring inside cavities derives from external sources or erosion processes of the bioconstructions themselves. This component has been classified as organic or inorganic based on the organic matter contents deduced by UV epifluorescence. A great quantity of sponges live in cavities of the coralligenous build-ups and compete with carbonatogenic bacteria for the same cryptic spaces, limiting the production of microbialites. The sharing of a similar relationship between sponges and microbial communities by coralligenous concretion and biotic crusts of particular submarine caves suggests that this competition is not habitat-specific. On the contrary, it may develop in a range of environmental settings, from open to cryptic systems, and could be used to clarify the role of metazoans vs. microbialites in palaeoecological reconstructions.
- Article
(23036 KB) - Full-text XML
- BibTeX
- EndNote
Bioconstructions consisting of in-place reef-building organisms in temperate waters of the Mediterranean Sea shelf are known as coralligenous (Pérès and Picard, 1964). The term coralligenous (Coralligène) is derived from the French literature (Marion, 1883; Pérès and Picard, 1964) and generally indicates mesophotic bioconstructions of the Mediterranean Sea, primarily built by crustose coralline algae. However, in recent years, the definition of coralligenous has been under review, in part due to exploration efforts on the deepest part of the shelf (Cerrano et al., 2019).
These structures are primarily made up of calcareous red algae, which are able to develop algal-dominated frameworks characteristic of this basin (e.g. Ballesteros, 2006). Pérès and Picard (1964) consider the coralligenous a climax biocoenosis of the circalittoral zone, in which crustose coralline algae (CCA) and mineralized Peyssonneliales develop on primary or secondary hard bottoms, in dim-light conditions. The crustose coralline algae have an excellent fossil record from the Early Cretaceous onwards (Aguirre et al., 2010), but the fossil record of build-ups similar to the coralligenous dates back only to the Quaternary (Sartoretto et al., 1996; Bertolino et al., 2017a, b; Basso et al., 2022).
Due to its importance as a hot spot of biodiversity, the European Community considers the coralligenous to be among the most important habitats to monitor and protect (see Ballesteros, 2006; Gennaro et al., 2020), also considering its low accretion rate of 0.06–0.27 mm yr−1 (Sartoretto et al., 1996; Di Geronimo et al., 2001; Bertolino et al., 2019; Basso et al., 2022). The coralligenous is usually considered to be an association of several communities (Ballesteros, 2006; La Rivière et al., 2021). Indeed, the external surface and the cavities of the build-ups host a rich association of calcareous red algae, sponges, bryozoans, serpulids, molluscs and corals (Pérès, 1982; Bellan-Santini et al., 1994; Di Geronimo et al., 2002; Ballesteros, 2006; Nalin et al., 2006; Rosso and Sanfilippo, 2009; Bertolino et al., 2017a, b, 2019; Costa et al., 2019; Basso et al., 2022; Bracchi et al., 2022; Cipriani et al., 2023). Coralligenous build-ups (i) modify the seafloor and the seascape (Laborel, 1961; Basso et al., 2007; Bracchi et al., 2015, 2017), (ii) promote the production of carbonate (Marchese et al., 2020), and (iii) may be recognized in the sedimentary succession (Bosence and Pedley 1982; Carannante and Simone 1996; Rasser, 2000; Basso et al., 2007, 2009; Titschack et al., 2008; Bracchi et al., 2014, 2016, 2019). The presence of the coralligenous 3D structure and the related high biodiversity and biomass also determine the increase in available resources. These attract microorganisms such as ostracods and foraminifera which, while not contributing directly to the bioconstruction, raise its biodiversity (e.g. Hong, 1982; Ballesteros, 2006; Sciuto et al., 2023).
Unlike other aspects, geobiological features of the coralligenous and the role of skeletonized and non-skeletonized (e.g. bacteria) communities in forming these build-ups have so far not been explored in detail. The lack of this information produces a gap between knowledge of the build-ups developed in the open settings of the Mediterranean Sea and those forming in confined environments, like the “biostalactites” of submarine caves. Actually, biostalactites from Apulia, the Adriatic Sea (Onorato et al., 2003; Belmonte et al., 2009; Rosso et al., 2020; Guido et al., 2022); Sicily (Guido et al., 2012, 2017a; Sanfilippo et al., 2015); the island of Lesvos, the Aegean Sea (Sanfilippo et al., 2017; Guido et al., 2019a, b); and Cyprus, the Levantine Sea (Guido et al., 2017b; Jimenez et al., 2019) have been studied in detail, and biotic and abiotic processes involved in their formation have been clarified. These studies have shown the fundamental role of bacteria in strengthening the bioconstructions, through the biomineralization processes of autochthonous micrite (Guido et al., 2013; Gischler et al., 2017a). Like these systems, it is conceivable that the highly porous framework of the coralligenous could also promote the development of non-skeletal biomineralization processes.
Biomineralization indicates a relationship between biotic activity or products and mineralization. Several biomineralization processes have been extensively investigated, mainly in carbonate rocks (Riding, 2000, 2011; Van Driessche et al., 2019). Trichet and Défarge (1995) introduced the term organomineralization for mineral precipitation mediated by non-living microbial organic substrates via acidic macromolecules of extracellular polymeric substances (EPS). These macromolecules provide a template for biofilm organomineralization. Dupraz et al. (2009) later utilized the term “organomineralization sensu lato” for processes not genetically controlled that mediate mineral precipitation on an organic matrix, including in the definition both the active (biologically induced, mediated by living organic substrates) and the passive (biologically influenced, mediated by non-living organic substrates) processes. Summarizing, crystal nucleation in biomineralization processes can be (i) controlled directly by the organisms (Blakemore, 1975; Mann, 1983, 2001; Weiner and Dove, 2003; Bazylinski and Frankel, 2004; Komeili, 2007; Altermann et al., 2009; Riding and Virgone, 2020), (ii) induced by microbial communities (Lowenstam and Weiner, 1989; Perry et al., 2007; Altermann et al., 2009; Dupraz et al., 2009; Borch et al., 2010; Phillips et al., 2013; Anbu et al., 2016; Riding and Virgone, 2020) or (iii) influenced by the presence of cell surface organic matter (Trichet and Défarge, 1995; Perry et al., 2007; Altermann et al., 2009; Dupraz et al., 2009). In all these cases, the formation of biominerals also depends on the chemical–physical conditions of the environment (Riding and Liang, 2005; Riding, 2011). Here the autochthonous micrite (“automicrite” sensu Wolf, 1965) was considered a product of induced or influenced biomineralization. To date no research has addressed this topic, and, in the frame of the project CRESCIBLUREEF, we aim to improve knowledge on the coralligenous by (1) describing the origin of the sediments filling the cavities of the skeletal framework; (2) investigating the relationships between sponges and microbial processes through biomineralization-mediated processes; and (3) comparing the coralligenous, formed in open marine systems, with the bioconstructions of submarine caves developed in confined marine settings.
In the studied area, coralligenous bioconstructions, mostly columnar-shaped and distributed in more or less dense clusters, extend widely in a belt between ca. 36 and 100 m depth. The image analysis and computed axial tomography reveal that the bioconstruction's framework is mainly formed of coralline algae and a minor number of invertebrates (mostly serpulids and bryozoans) and cavities filled with sediment (Bracchi et al., 2022; Varzi et al., 2023). The surfaces of the studied build-ups were covered with a dense, up to 8–10 cm thick canopy of fleshy algae and, locally, also by subordinate erect bryozoan colonies (Bracchi et al., 2022; Donato et al., 2022; Rosso et al., 2022, 2023; Sciuto et al., 2023). The most abundant components are CCA and mineralized Peyssonneliales with a cover of up to about 61 %. Fleshy algae are also abundant, at up to about 32 %. The faunal groups have negligible covers, except for bryozoans reaching about 12 % (Bracchi et al., 2022).
Two build-ups called CBR2_3_7c and CBR2_4_21c from this coralligenous field located in the Ionian Sea were collected (Fig. 1). The build-up CBR2_3_7c (36∘43.394′ N, 15∘09.469′ E) was sampled at 36.2 m depth in a zone covered by coralligenous hybrid banks (Bracchi et al., 2017), made of distinct, though sometimes coalescent, coralligenous columnar build-ups (Fig. 1b and d). The build-up CBR2_4_21c (36∘43.454′ N, 15∘09.657′ E) was collected at 36.7 m depth in an area characterized by sparse and distinct build-ups growing on biogenic gravel and sand substrate (Fig. 1c and e).
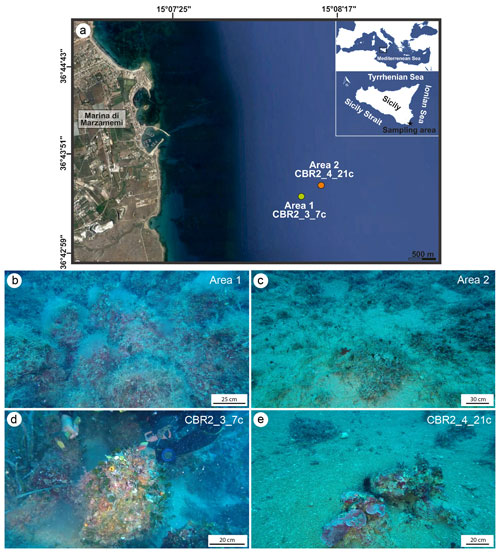
Figure 1(a) Location of the study areas off the coast of the village of Marzamemi in the Ionian Sea. (b–c) Underwater photos of the sampling sites. (d) Area 1, characterized by high coralligenous cover, from where the CBR2_3_7c build-up was collected at 37 m. (e) Area 2, showing sparse and isolated coralligenous columnar bioconstructions, from where CBR2_4_21c build-up was sampled at 36 m.
After drying, the build-ups were cut with a diamond saw following the putative grow direction of the structures (Fig. 2a, b, d and e). The cutting planes showed that the coralline algae framework forms a highly porous structure with cavities filled with sediments (Fig. 2c and h). Photos at macroscale were acquired at the University of Milano-Bicocca with a Nikon D3500 camera. Photos at mesoscale were acquired at the University of Catania through a stereomicroscope Zeiss Discovery V8A stocked with an AxioCam MRc and a system for automatic acquisition of the images (AxioVision). To investigate the role of sediment in the growth and stabilization of the skeletal components (at micro- and nano-scale), small fragments (Fig. 2g and h) and thin sections (Fig. 3) were analysed at the Laboratory of Geobiology of the Department of Biology, Ecology and Earth Science, University of Calabria.
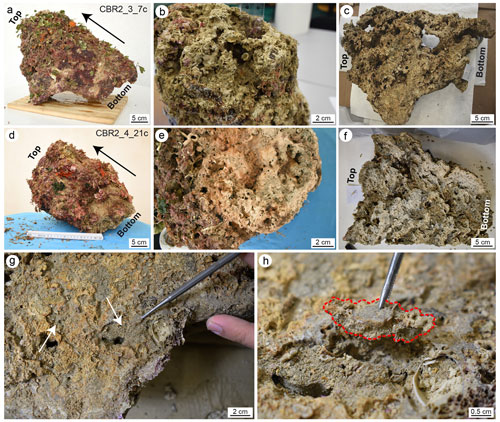
Figure 2(a, d) Sampled coralligenous build-ups; note the high biogenic cover (largely consisting of soft-bodied organisms) on the external surfaces. Black arrows indicate the main growth direction. (b, e) Surfaces of detachment from the sea bottom. (c, f) Plane slab after the longitudinal cut of the build-ups showing the internal structure; note the highly porous skeletal framework with cavities partly filled with sediment. (g, h) Selection and sampling of small micritic fragments from the cutting surfaces. (g) The white arrows point to cavities partly filled with sediment on a cutting plane of the CBR2_3_7c build-up; (h) detail of a fragment (dotted red line) sampled for the analyses. The bottom indicates the portion of build-ups detached from the substrate. Panels (a), (c) and (h) are adapted from Cipriani et al. (2023).
A total of 29 small blocks, selected following a grid with sides of 5×3 cm on the cutting plane, have been utilized for thin-section preparation (Fig. 3). The blocks have been chosen based on the mesoscopic aspect and relative number of skeletal components and micrite sediments. The fragments and thin sections have been investigated using an optical microscope (Zeiss Axioplan 2 Imaging) at different magnifications (2.5×, 5×, 10×, 20× and 40×). Thin sections were used for point-counting analyses of the main components (skeletons, micrite and cavities). A total of 300 points per thin section were counted. Fluorescence intensity has been evaluated in incident light, utilizing a Hg high-pressure vapour bulb and high-performance wide bandpass filters (436/10 nm) and long pass (470 nm), no. 488006, for the green light and bandpass filters (450–490 nm) and long pass (515 nm), no. 488009, for the yellow light. UV epifluorescence was used to discriminate the presence and distribution of organic compounds and to recognize, together with microfacies textures, biotic and abiotic components, especially in those cases showing a similar general aspect under reflected light (Neuweiler et al., 2000, 2003, 2023).
Selected fragments, used for scanning electron microscopy (SEM) observations and energy-dispersive X-ray spectroscopy (EDS) microanalysis, were carbon coated. The SEM apparatus was used is ultra-high resolution (UHR-SEM) – ZEISS Crossbeam 350. The working conditions were resolution 123 eV, high voltage 10 keV, probe current 100 pA and working distance 11 mm. Mineralogical and chemical compositions were investigated with an EDAX Octane Elite Plus – silicon drift type – Si3N4 window apparatus under high voltage 15 keV, probe current 60 mm, working distance 12 mm, take-off angle 40∘ and live time 30 s. The standardless quantitative analysis was checked on the SPI no. 02757-AB serial 4AK standard and was collected through the AMETEK APEX software suite V2.
3.1 Morphology and framework at mesoscale observation
The CBR2_3_7c build-up is 56 cm high, with circumferences of 59 cm at the base, 78 cm at the top and a maximum of 116 cm in between (Fig. 2a). The CBR2_4_21c build-up is 38 cm high, with circumferences of 71 cm at the base, 52.5 cm at the top and a maximum of 112 cm in between (Fig. 2d). Both build-ups show a prevalent upward growth and a rough surface.
The surfaces of detachment (Fig. 2b and e, for CBR2_3_7c and CBR2_4_21c, respectively) and selected longitudinal plane slabs (Fig. 2c and f, for CBR2_3_7c and CBR2_4_21c, respectively) highlight the internal framework of the two build-ups, characterized by a primary skeletal framework forming a highly porous structure, with cavities ranging from a few millimetres to tens of centimetres. Sometime, the cavities' surfaces are encrusted by skeletonized organisms, mainly serpulids and bryozoans. In general, cavities show cylindrical barrel shapes (like those produced by boring bivalves) or irregular shapes and may be empty or (partially or totally) filled with sediment. This appears either brownish-greyish or greenish-brown to grey/dark grey in colour. Brownish-greyish sediment is muddy, is usually located in larger cavities, includes a bioclastic component (planktonic and benthic foraminifer shells, small fragments of coralline algae, serpulids, bryozoans, ostracods, molluscs) and appears loose. Greenish-coloured sediment is subordinate and distributed in smaller (millimetre- to centimetre-sized) cavities; it seems cemented and consists of mud lacking skeletal fragments in mesoscale observations (Fig. 2g). This component mainly occurs along the borders of partly filled cavities possibly originally occupied by sponges, as testified by remains of their soft tissue connected with spicules (Fig. 4a and b). The two types of sediment (loose and cemented) seem distributed according to the size of the cavities, but they do not show a preferential distribution, from the bottom to the top of the structures, inside the build-up frameworks.
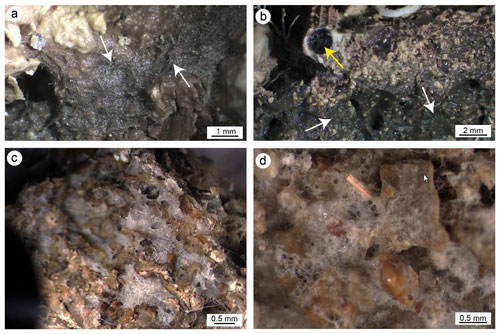
Figure 4(a, b) Fine micritic sediment associated with remains of sponge tissue (white arrows) in internal cavities of the framework; the yellow arrow points to a sponge colonizing the internal cavity of a serpulid tube. (c, d) Pervasive colonization of sponges in internal cavities and the external surface, respectively.
3.2 Analyses of the fragments
The observation of the build-up fragments at the microscale (Fig. 2h and Fig. 5) highlights the superimposition of successive generations of different taxa producing the crusts. The skeletal framework is mainly composed of CCA (Fig. 5a–c). Serpulids (Fig. 5a, d and e) and bryozoans (Fig. 5a, f and g) participate subordinately to the formation of the structure. Sponges seem to contribute to the general morphologies, regulating the direction of growth of the encrusting organisms and altering the internal body of the build-ups through bioerosive processes. The activity of sponges is evident both on the external surface and in the internal microcavities of the fragments, testifying to the pervasive colonization of these organisms at different scales (Fig. 4). Spicules are sometimes associated with fine carbonate mud (Fig. 4a and b). In other cases, their original presence in empty cavities is testified by organic remains with spicules (Fig. 4c and d) and specific micro-morphologies of the cavity boundaries which testify to the boring activity of these organisms. Molluscs and other skeletonized invertebrates make a negligible contribution to the build-up growth. Rare solitary corals are also present.
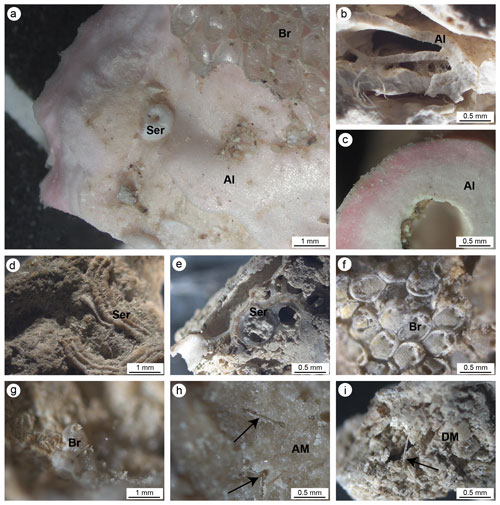
Figure 5(a, b) Main carbonate components of the small fragments collected on the cut surfaces of the build-ups. (a) Pink crustose coralline algae (Al) encrusted by serpulids (Ser) and bryozoans (Br). (b) Different generations of crustose coralline algae encrusted one on top of another. (c) Cross section of a crustose coralline alga. (d) Serpulid tube encrusting cemented micrite. (e) Section of serpulid tubes intermingled with micritic sediments. (f, g) Bryozoan colonies. (h) Dense and homogeneous autochthonous micrite (AM) engulfing sponge spicules. (i) Heterogeneous and loose detrital micrite sediment (DM) engulfing sponge spicules. In (h) and (i) black arrows point to sponge spicules engulfed in the micrite sediments.
Two types of sediments (micrite) have been observed: homogeneous or autochthonous (compact and dense; Fig. 5h) , which emits a bright fluorescence when excited by UV light, and heterogeneous or detrital (less cemented and rich in fine bioclasts; Fig. 5i), which does not emit fluorescence (Fig. 6). Both types of micrite engulf sponge spicules.
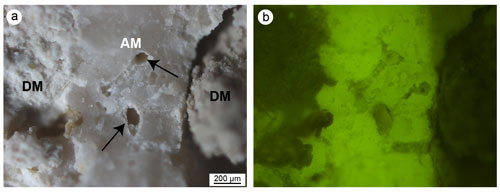
Figure 6Micrite sediments from CBR2_4_21c build-up observed in reflected light (a) and ultraviolet light (b). The bright fluorescence indicates a high content in organic matter of the autochthonous micrite, whereas the absence of fluorescence of the detrital micrite denotes an inorganic origin. Note the microcavities left by sponge spicules (black arrows). AM: autochthonous micrite; DM: detrital micrite.
3.3 Microfacies characterization
Thin-section observation confirms the main role of skeletonized organisms in forming the carbonate framework of the coralligenous (Fig. 7). CCA are the main builders through successive generations of specimens encrusted one on top of another (Fig. 7). Bryozoans and serpulids play a subordinate bioconstructional role. Sponges are abundant, and their amorphous remains are widely distributed in cavities and microcavities, often associated with loose sediment, engulfed in cemented micrite. Numerous other bioclasts, produced by organisms that do not participate directly in building the bioconstructions, were detected in the cavities together with muddy material. Non-skeletal carbonate material was also detected (see below).
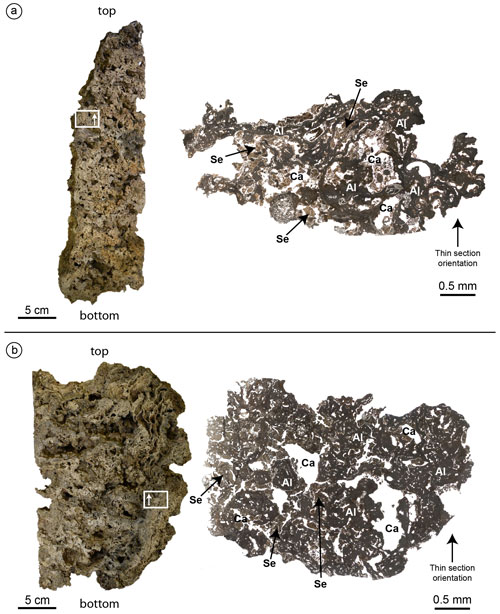
Figure 7Cutting planes (left) and optical microscope photocomposition of representative thin sections (right) of the build-ups CBR2_3_ 7c (a) and CBR2_4_21c (b). Note the primary role of the coralline algae (Al) as a major constituent of both build-ups and the presence of cavities (Ca) empty or filled with micrite sediment (Se). White rectangles and the arrows within them indicate the location and the orientation of the thin sections.
3.3.1 Skeletal components
Articulate and crustose coralline red algae are the main skeletal components detected in all thin sections (Fig. 8). The skeletons of the algae clearly form a continuous framework at the mesoscale (Fig. 7), but at the microscale, laminae are rarely continuous, often showing traces of bioerosion (Fig. 8a–c, f). Two main types of bioturbation are distinguishable: (i) irregular borings and (ii) tube-like micro-borings. Borings, showing sizes from a few micrometres to a few millimetres, are mainly formed by endolithic sponges that corrode and perforate skeletons. These cavities could be (a) empty due to the decaying of the sponge's organic tissue (Fig. 8b), (b) filled with detrital sediment (Fig. 8b), or (c) filled with spicules and remains of organic matter deriving from soft-tissue decay of the boring sponge Cliona sp. (Fig. 8f).
The skeletons often also show micritization phenomena contributing to the alteration of the original microstructures together with bioerosion.
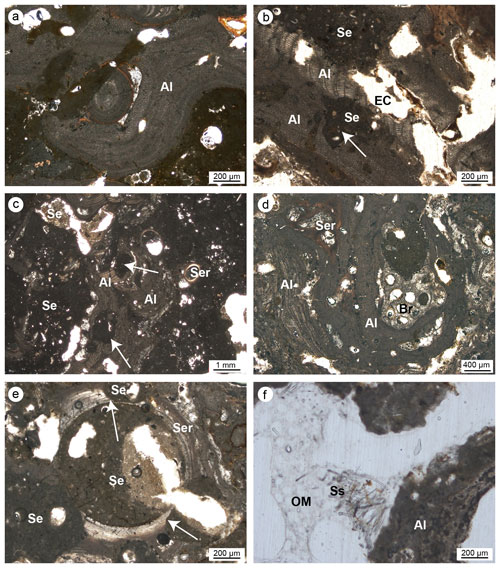
Figure 8(a–c) Skeletal tissues of algae (Al) showing microcavities due to bioturbations (white arrows); the cavities are empty (EC) or filled with sediment (Se). (d) Strict interconnection among the main builders of the studied coralligenous build-ups (Al: algae; Ser: serpulids; Br: ? bryozoans). (e) Bioeroded serpulid skeleton (Ser); note the borings (white arrows) filled with sediment (Se). (f) Bioerosion cavity in algae (Al) with remains of organic matter (OM) and spicules (Ss) derived from the sponge's decay (Cliona sp.) (a–b, e–f: CBR_2_3_7c; c–d: CBR_2_4_21c).
The biological activity involved in these bioerosional processes was not investigated but could represent a further step in the reconstruction of the complex biological relationships which develop in these coralligenous ecosystems. The erosive action of sponges is clearly visible where remains of amorphous material and spicules are associated with corroded substrates (Fig. 9).
The sponge spicules, mainly belonging to species of the genus Jaspis, also fill the algae's conceptacles, and often, small spherical corpuscles are recognizable among spicules in UV epifluorescence.
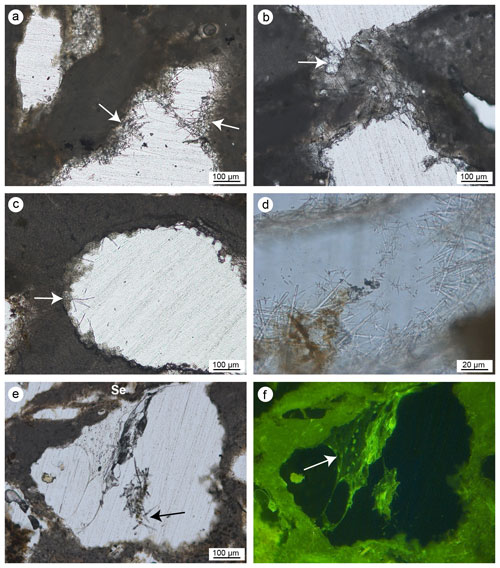
Figure 9(a–c) Microcavities inside the skeletal framework of the coralligenous build-ups showing sponge spicules (plurispicular tracts) associated with the corroded substrates (white arrows). (d–f) Details of sponge spicules of Jaspis sp. (d) and Cliona sp. observed in transmitted light (e) and UV epifluorescence (f); the non-fluorescent siliceous spicules are engulfed in remains of organic matter (f) deriving from sponge soft-tissue decay (a–c: CBR_2_4_21c; d–f: CBR_2_3_7c).
Serpulids are common in the microfacies of both build-ups. They occur as isolated or grouped tubes with outer diameters ranging from 200 µm to 1 mm. The number of clustered tubes usually increases with a decreasing in their size. They are empty, filled with sediment or sometimes occupied by endolytic sponges (Fig. 4b). Serpulids are often encrusted or encrust bryozoans and/or algae (Fig. 8d). Serpulids show different degrees of preservation, and their original microstructure is observable in the bigger tubes, while dissolution, recrystallization and/or micritization often alter the smaller ones. Serpulids also suffer bioerosion by endolytic organisms (Fig. 8e).
Remains of amorphous material and spicules inside the skeletons of bryozoans may derive from insinuating and/or bioeroding sponges, as observed for other skeletonized taxa.
3.3.2 Non-skeletal carbonate components: autochthonous and allochthonous (detrital) micrite
The cavities of the skeletal framework are filled with different micrite types distinguished under light microscope examination and UV epifluorescence. The texture and organic matter content allowed us to distinguish an autochthonous and an allochthonous (detrital) micrite.
The autochthonous micrite consists of very fine-grained calcite and shows aphanitic (Fig. 10a–f) or peloidal to clotted peloidal textures (Fig. 10g–h). The autochthonous aphanitic micrite displays a light-brown colour and shows a structureless mud-supported texture with rare bioclasts. Autochthonous peloidal micrite displays a darker colour, does not exhibit grain-supported textures and shows interclot areas indicating a non-gravitational genesis. Peloidal micrite fills microcavities or coats serpulid tubes or other bioclasts. Peloids aggregate often in clots separated by calcite microspar (euhedral Mg-calcite crystals), forming a clotted texture or, less commonly, a compact texture through the coalescence of several clots. Peloidal and aphanitic microfabric derives from mineralization mediated indirectly by organic processes and represents in situ precipitation of the micrite, whose syndepositional cementation contributes to stabilizing the skeletal structures of the build-ups.
Aphanitic and peloidal micrites show a bright autofluorescence under UV light, indicating a high content of organic matter relicts, most likely related to the bioinduced crystal precipitation. Aphanitic micrite is widely associated with the presence of sponge spicules (Fig. 10a–f) and generally fills bioeroded cavities inside the skeletal framework. On the contrary, peloidal micrite is not associated with sponge spicules and often occludes serpulid tubes and spaces within adjacent individuals, contributing to cementing the skeletons together. Small terebellid tubes are often associated with this micrite type (Fig. 10g–h).
The amount of the autochthonous micrite is variable in the thin sections but always represents a minor component in comparison to the skeletal framework and shows a different distribution along the bottom-top direction of each build-up, with the major content in the column CBR_2_ 4_21c.
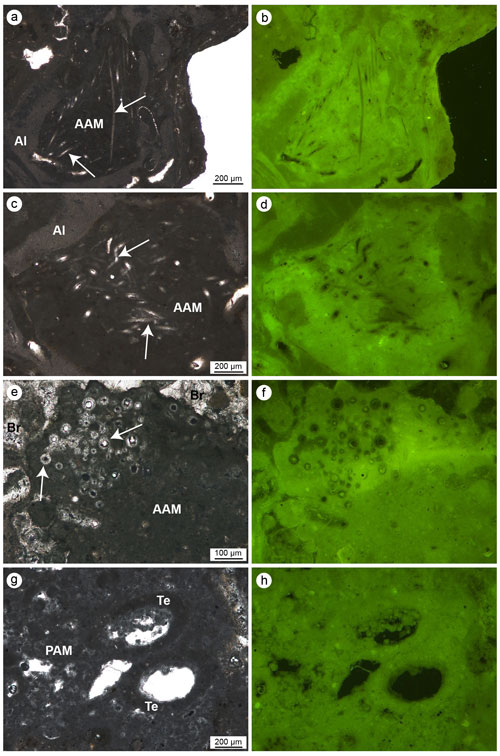
Figure 10(a–f) Aphanitic autochthonous micrite (AAM) engulfing sponge spicules (white arrows) observed in transmitted light (left) and UV epifluorescence (right); the bright epifluorescence of the AAM indicates the presence of organic matter relics closely related to the bioinduced crystals. (g–h) Peloidal autochthonous micrite (PAM) engulfing some agglutinated skeletons of terebellids (Te) observed in transmitted light (left) and UV epifluorescence (right); even in this case the bright epifluorescence of the PAM indicates the presence of organic matter relics closely related to the bioinduced crystals (a–b, e–h: CBR_2_ 4_21c; c–d: CBR_2_3_7c).
The detrital micrite shows a light-brownish colour and is characterized by a texture with variable density (Fig. 11). Two types of detrital micrite with different textures have been tentatively recognized: organic and inorganic. Organic detrital micrite shows a denser muddy texture, and it is enriched in bioclasts, intraclasts and sponge spicules (Fig. 11a, d, e). It shows very faint to scarce epifluorescence (Fig. 11f). The inorganic detrital micrite is made up of particles with larger sizes (in the silty range) and includes a minor number of bioclasts. Due to the absence of epifluorescence under UV light, an inorganic nature of these components is assumed. Detrital micrites represent the main non-biomineralized component of both build-ups and fill primary inter- and intra-skeletal cavities and secondary microcavities generated by boring organisms.
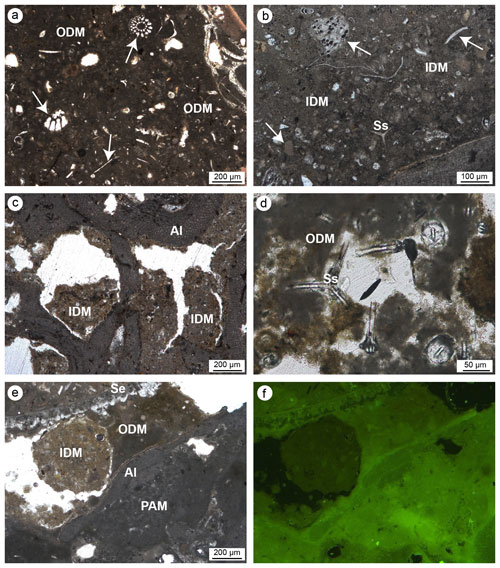
Figure 11Detrital micrite textures. (a) Organic detrital micrite (ODM) with a dense muddy texture enriched in bioclasts (white arrows), intraclasts and sponge spicules. (b) Inorganic detrital micrite (IDM) consisting of particles with larger sizes incorporating a minor number of bioclasts (white arrows). (c) Microcavities in bioeroded algae (Al) filled with IDM. (d) ODM engulfing sponge spicules (Ss). (e–f) Relationship between IDM (light brown), ODM (dark brown), algae (Al) and peloidal autochthonous micrite (PAM) observed in transmitted light (left) and UV epifluorescence (right); faint and scarce fluorescence of the detrital micrite denotes an inorganic nature of this component (a–b, d–f: CBR_2_4_21c; c: CBR_2_ 3_7c).
3.3.3 Cements
Cement only sporadically fills cavities, representing a subordinate component of the build-ups. Two types of cement have been recognized: primary (syndepositional) and secondary (diagenetic) cement. Syndepositional cement shows isopachous, botryoidal and peloidal microcrystalline fabric. Isopachous cements develop with homogeneous fringes on the surface of intra- and inter-skeletal microcavities. Botryoids consist of dome-shaped hemispheres built by radiating fibrous calcite crystals and crystal fans filling the primary cavities and voids created by bioerosion processes. Peloidal microcrystalline cement is composed of tiny peloids within a microcrystalline calcite matrix; it was mainly detected in small intra-skeletal cavities. Secondary cement is rare and fills residual microcavities with drusy micro-textures.
3.3.4 Point-counting analyses
Seven structural and non-structural components have been counted: carbonate framework builders, bioclasts, autochthonous micrite, organic detrital micrite, inorganic detrital micrite, boring sponges and empty cavities. Bioclasts include all skeletal remains of organisms that did not participate in the formation of the skeletal framework but were trapped inside the cavities either first living in association with the build-up or transported by neighbouring habitats. Among these, bivalves, gastropods, foraminifers, ostracods, echinoid plates and spines, and algal fragments have been recognized. The boring-sponge counting class includes perforations interpreted as originally occupied by sponges because they are infilled with amorphous organic remains rich in spicules. It is worth noting that this component could be underestimated due to the cutting procedures and the preparation of the thin sections, which could have washed away the residues of the sponge tissue that originally occupied the cavities. The analysis shows the following average percentages for the CBR_2_3_7c and CBR_2_4_21c build-up, respectively: 46.2 % and 47.1 % carbonate framework builders (algae, serpulids, bryozoans), 4.4 % and 3.6 % other bioclasts (planktonic and benthic foraminifer shells, ostracods, molluscs, echinoid plates and spines, and small fragments of coralline algae), 3.6 % and 9.2 % autochthonous micrite, 16.4 % and 14.3 % organic detrital micrite, 13.6 % and 9.3 % inorganic detrital micrite, 14.8 % and 15.4 % empty cavities, and 1 % and 1.1 % boring sponges (Tables 1–2).
Table 1Quantitative percentage of the main components recognized in the thin-section samples from the CBR_2_3_7c build-up.
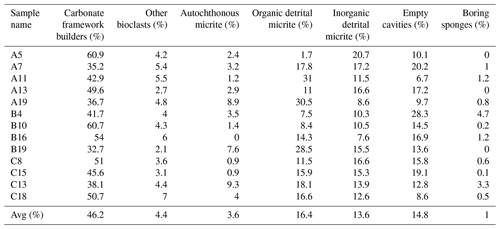
Table 2Quantitative percentage of the main components recognized in the thin-section samples from the CBR_2_4_21c build-up.
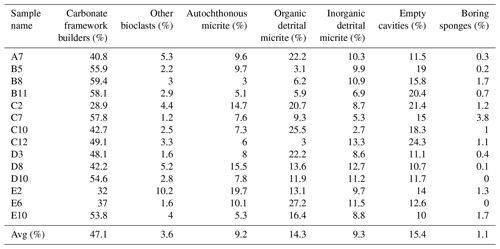
Among the non-skeletal carbonate components, the allochthonous (detrital) micrites are definitively more abundant in comparison to the component directly mineralized (autochthonous micrite) in the cavities of the build-ups. Noteworthily, the percentage of autochthonous micrite, whose early cementation contributes to reinforcing the coralline algae framework, is higher in the CBR_2_4_21c build-up than in the CBR_2_3_7c build-up. The average percentages of total cavities, i.e. the sum of empty cavities and cavities filled with detrital sediment, are similar in the two build-ups.
3.4 Characterization of micro-morphology and geochemistry with electron microscopy
SEM observations and EDS microanalyses allowed us to detect the micro-/nano-morphologies and the composition of the main skeletal and non-skeletal components. The presence of pristine micro- and nano-morphology and the original mineralogy of skeletons (high Mg-calcite or aragonite of algae and serpulids) testify to the fact that the carbonate components of the build-ups have not undergone neomorphic processes, like recrystallization, polymorphic transformation or aggrading neomorphism. In some cases, the skeletal components display evidence of the dissolution process.
Microcavities are filled with sponge spicules and remains of carbonaceous amorphous substances (Fig. 12). Spicules are mainly oxeas and (sub-)tylostyles, which may be associated with species of the Cliona genus, but there are also triactines and rare tetractines. Spicules are closely intermingled with organic matter and are often well-visible cleats with sterrasters and oxyasters, typical of species of the genus Erylus (Fig. 12e and f). Areas close to the corroded boundaries of some microcavities containing spicules (Fig. 12g–h) also include small carbonate chips (Fig. 12i) seemingly detached from the encasing skeleton due to mechanical boring activity of the sponges. The substrate of the bioeroded cavities shows the typical erosion scars (pits) left by the perforating activity of sponges of the Clionaidae family (Fig. 12d). Spicules often show circular erosion marks and an enlarged axial canal (Fig. 13a–e) due to silica dissolution caused by high pH values of the porewater inside the crevices of the coralligenous build-up.
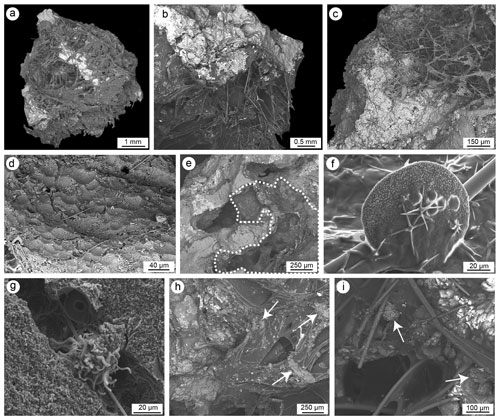
Figure 12SEM images of sponge spicules and remains of carbonaceous amorphous substances. (a–c) Coralligenous fragments with pervasive colonization of sponges both on the external surface and in internal microcavities. (d) Sponge erosion scars (pits) on a skeletal substrate. (e) Endolithic sponge (dotted line) inside a microcavity of the skeletal framework. (f) Detail of sterraster and oxyaster of Erylus sp. (g) Cliona vermifera spiraster associated with a bioeroded cavity. (h) Spicules and amorphous organic matter in an internal microcavity. (i) Detail of spicules and amorphous organic matter englobing small carbonate chips (white arrows) detached through sponge bioerosion activity (a, d–f, g–i: CBR_2_3_7c; b–c: CBR_ 2_ 4_21c).
A high quantity of siliceous and rare calcareous sponge spicules (still under study: Bertolino et al., 2023) has been recognized in both autochthonous (Fig. 13) and detrital (Fig. 14) micrite, pointing to a considerable diversity.
Autochthonous micrite shows micro- to nano-metre anhedral to sub-euhedral crystals engulfed in amorphous organic material (Fig. 13f) and has a high magnesium calcite (Ca ∼ 91 wt %; Mg ∼ 6.5 wt %) composition with minor terrigenous components (< 2 wt %). Micrite engulfing spicules is well cemented (Fig. 13a, b and e). Peloidal micrite passes to aphanitic textures when cavities become filled. In comparison to the detrital micrite, the autochthonous micrite that engulfs sponge spicules lacks skeleton fragments or allochthonous components like planktonic foraminifers or coccoliths.
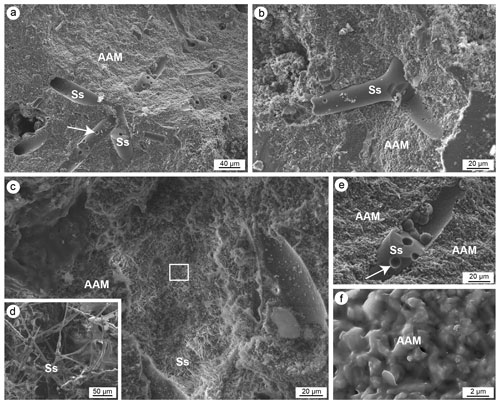
Figure 13(a–f) SEM images of aphanitic autochthonous micrite (AAM) engulfing sponge spicules (Ss). In (a) and (e) note the well-defined circular boreholes (white arrows) and enlarged axial canal of the spicules. (d) Magnification of the spicules in (c). (f) Detail of the aphanitic autochthonous micrite showing the micrometre sub-euhedral crystals engulfed in amorphous organic material (a, e–f: CBR_2_3_7c; b–d: CBR_2_4_21c).
Detrital micrite shows a heterogeneous composition (Fig. 14), in terms of the type and size of the grains; has a magnesium calcite composition; and has a high percentage of terrigenous components. It is composed of Ca (∼ 46 wt %), Mg (∼ 3 wt %), Fe (∼ 6 wt %), K (∼ 3 wt %), a discrete quantity of Si (∼ 26 wt %) and Al (∼ 12 wt %), and a lower quantity of other elements (S, Na, Cl < 2 wt % each). Detrital micrite constantly incorporates sponge spicules (Fig. 14) but occasionally also small intraclasts and several bioclasts of benthic and planktonic organisms. At the microscale, detrital micrites denote a high quantity of nannoplankton plates (e.g. Emiliania huxleyi) (Fig. 14e).
The analysed coralligenous build-ups from the Ionian Sea are mainly composed of skeletonized organisms with dominant coralline algae and subordinate bryozoans and serpulids. CCA form a porous self-sustaining framework whose stabilization is reinforced by bryozoans and serpulids. The role of foraminifers and corals is negligible. Morphological growth is influenced by sponges. These organisms are highly diversified and play a triple role: they locally affect the direction of encrustations of the skeletonized builders, weaken the framework through bioerosion processes and induce precipitation of autochthonous sediments (autochthonous micrite). An important role is also played by the micrite, which could be subdivided into two types: autochthonous, directly produced in the build-ups by organic-induced biomineralization processes, and allochthonous (or detrital), derived by the accumulation of loose fine particles in the cavities of the build-ups.
4.1 Skeletal builders and framework density
The two build-ups are characterized by a highly porous skeletal framework, showing primary and secondary cavities, from a few millimetres to tens of centimetres large. Primary cavities represent the interspaces generated during the superimposition of skeletonized encrusting organisms, whereas secondary cavities could derive from the necrolysis of soft-body organisms sandwiched between the skeletonized ones or from the bioerosion produced by endolytic organisms, mainly sponges and subordinately bivalves.
Quantitative counting of the microfacies components demonstrates the role of algae, serpulids and bryozoans as builders of the two build-ups (see Tables 1 and 2). These data agree with the indirect quantification obtained through image analysis and computerized axial tomography by Bracchi et al. (2022) applied to the same build-ups analysed in this paper. Bracchi et al. (2022) correlated four density classes with the framework cementation degree and distinguished the different components, identifying the CCA as dominant. This is because computerized axial tomography does not provide a direct association between skeletal and non-skeletal components and the variation in density. In contrast, and despite referring to only one surface for each build-up, the microfacies characterization at the microscale confirmed that the density is not directly correlated to specific components but is linked to the degree of packing of the skeletons (mainly CCA) and to the degree of cementation of non-skeletal carbonate components. Furthermore, it is worth noting that the presence of autochthonous micrite, which cement syndepositionally, contributes to increasing the build-up density, regardless of the nature of the components with which it is associated.
4.2 Role of the sponges in the coralligenous growth
Coralligenous growth is the result of the interplay between building activity and the physical and biological demolition (erosion and dissolution) processes (Garrabou and Ballesteros, 2000; Bressan et al., 2001; Cerrano et al., 2001; Ingrosso et al., 2018; Turicchia et al., 2022). Like for bioconstructions in the Mediterranean Sea and worldwide (Rosell and Uriz, 2002; Evcen and Çınar, 2015; Glynn and Manzello, 2015; Achlatis et al., 2017; Turicchia et al., 2022), boring sponges represent the main cause of bioerosion for the coralligenous build-ups of Marzamemi. The colonization, number and bioerosion processes of sponges are influenced by temperature, nutrients, turbidity, depth, light and pH (Schönberg, 2008; Calcinai et al., 2011; Nava and Carballo, 2013; Marlow et al., 2018). Bioerosion has a direct influence on the coralligenous build-ups due to the erosion of the substrate and the skeletal framework, which reduces the mechanical stability of the build-ups (Scott et al., 1988) but at the same time creates new space and shelter for other organisms (Cerrano et al., 2001; Calcinai et al., 2015).
Boring and insinuating, cavity-dwelling, endolithic sponges constitute a considerable proportion of the total biomass, even higher than that of the epibenthic layer, in both coralligenous build-ups (Calcinai et al., 2015) and marine caves (Corriero et al., 2000). The main boring taxa belong to the family Clionaidae, and especially Cliona celata, C. schmidtii and C. viridis. The contribution of cryptic fauna should be taken into consideration in coralligenous studies, considering that the number of sponges occurring outside these build-ups is lower than the number of taxa identified inside them (Calcinai et al., 2015).
The high quantity of sponges and their expected diversity fit well with results by Bertolino et al. (2013) that reveal the occurrence of 53 insinuating and 10 boring species inside the coralligenous build-ups. Among them, non-perforating encrusting or massive species occupy cavities of the bioconstructions previously formed by boring sponges (Bertolino et al., 2013).
When sponges die, their spicules remain trapped in the cavities of the coralligenous framework, offering the opportunity to recognize the spongofauna over a very long time (Bertolino et al., 2014, 2017a, b; 2019). Spicules of non-eroding sponges from the build-up surfaces may be mixed with spicules of boring and insinuating species in the cavities of the build-ups (Calcinai et al., 2019). In the studied coralligenous build-ups, the pervasive colonization of sponges is testified by the high quantity of spicules occurring on the surface and within cavities. Oxea and tylostyle sponge spicules were observed to be mixed or grouped by type. It is unclear if the oxeas and other spicules derive from insinuating sponges or from species (e.g. haplosclerids) which encrust the external hard surfaces of the build-ups. Spicules associated with amorphous organic matter (spongin remains) in empty cavities testify to the presence of recently dead endolithic sponges, possibly even after the collection of the samples. Among these, boring sponges are recorded by spicules associated with small chips detached from the substrate in cavities showing irregular edges and corroded surfaces. Most of the spicules are mixed with fine detrital sediment and other bioclasts filling intra- and inter-skeletal holes. These spicules may originate from sponges thriving on the build-up surfaces or their internal niches, and together with those preserved in the autochthonous micrite, they represent the record of past coralligenous sponges. The characterization and dating of these components may reveal the ecological evolution and functional role of the sponge associations during the growth of the build-ups. Sponges also seem to influence the morphological development of build-ups. They can often be bioimmurated by encrusting organisms (mainly crustose algae), thus affecting the local growth direction of the carbonate crusts that follow the morphologies of the sponges.
4.3 Origin and role of the micrite sediments in the coralligenous framework
The micro-morphological observations allowed us to recognize and investigate the role of the sediment in the coralligenous. Of the two main types of sediments detected, i.e. autochthonous and allochthonous (detrital) micrite, the former is produced in situ through biomineralization processes. Biomineralization involves organisms, processes and products and includes controlled, induced and influenced mineral precipitation (Lowenstam and Weiner, 1989; Riding, 2000, 2011; Benzerara et al., 2011; Phillips et al., 2013; Anbu et al., 2016; Riding and Virgone, 2020). These different pathways of biomineral precipitation also depend on the chemical and physical conditions of the environment (Riding, 2011; Riding and Liang, 2005; Deias et al., 2023). The recognition of biominerals, especially those not biologically controlled (like skeletons) but precipitated via organic mediation in equilibrium with the water medium, can be considered a remarkable archive, documenting the presence of non-fossilizable associations as well as their relations with environmental conditions. In the coralligenous, the presence of autochthonous micrite (“automicrite” sensu Wolf, 1965), whose precipitation could be linked to microbial metabolic activity (biologically induced mineralization, mediated by living organic substrates, sensu Dupraz et al., 2009) or decaying organic matter mediation (biologically influenced, mediated by non-living organic substrates, sensu Trichet and Défarge, 1995), documents communities with low preservation potential but which heavily affect the development of the build-ups.
The autochthonous micrites detected in the studied coralligenous build-ups show two fabrics: (1) structureless (aphanitic) and (2) peloidal to clotted peloidal. Both types consist of Mg-calcite and show an intense fluorescence when excited by UV light, suggesting a high content in organic matter. Despite these similarities, the two fabrics may have different origins. The massive presence of spicules engulfed in the aphanitic micrite could indicate a carbonate precipitation in association with decaying organic substrates of sponges as repeatedly suggested in the literature (Leinfelder and Keupp, 1995; Reitner and Neuweiler, 1995; Reitner et al., 1995; Trichet and Défarge, 1995; Pickard, 1996; Pratt, 2000; Neuweiler et al., 2000, 2003, 2023; Reolid, 2007, 2010). This organic mineralization (organomineralization, sensu Trichet and Défarge, 1995) is supposed to form via the Ca2+-binding ability of humic and fulvic amino acids, derived from organic-matter-degraded metazoan during early diagenesis (Braga et al., 1995; Neuweiler et al., 1999, 2007; Wood, 2001; Dupraz et al., 2009). This micrite type is usually darker than the allochthonous micrite due to the organic matter content (Warnkle, 1995; Delecat et al., 2001; Delecat and Reitner, 2005). Shen and Neuweiler (2018) suggested an organomineralization (produced by induced or supported processes), rather than microbial mediation, for the deposition of the autochthonous micrite in the Ordovician carbonate mounds, formed mainly of calathid–demosponge (north-west China). A similar process was proposed for the autochthonous micrite mineralized in the biotic crust of submarine caves of Lesvos (Guido et al., 2019a, b).
The peloidal and clotted peloidal micrites have commonly been linked to anaerobic bacteria activity and represent the main component of the microfacies recognized in modern and ancient microbialites (Monty, 1976; Chafetz, 1986; Kennard and James, 1986; Buczynski and Chafetz, 1991; Reitner 1993; Kazmierczak et al., 1996; Dupraz and Strasser, 1999; Folk and Chafetz, 2000; Riding, 2002; Riding and Tomás 2006; Dupraz et al., 2009; Guido et al., 2013, 2016; Riding et al., 2014). The microorganisms and metabolic pathways responsible for the formation of clotted and peloidal micrites still remain unknown in most instances. In the studied build-ups, the scarce peloidal fabric is mainly confined to framework microcavities, particularly the interior of serpulid tubes and spaces between skeletons. In these suboxic/anoxic confined microenvironments, anaerobic heterotrophic bacterial communities can flourish, as observed in several submarine caves by Guido et al. (2017a, b, 2019a, 2022). In the coralligenous, the clotted peloidal micrite is very subordinate in comparison to aphanitic micrite, and generally it is not associated with sponge spicules but includes terebellid polychaetes often intermingled with the autochthonous peloidal micrite. The presence of terebellids associated with peloidal micrite suggests a close association between these polychaetes and microbial communities. A symbiotic relationship between terebellids and sulfate-reducing bacteria has been described in confined environments of submarine caves (Guido et al., 2014, 2022). These authors proposed that in pendant bioconstructions, terebellids use the peloids produced by microbial activity to form their skeletons and the bacteria flourish on the biomass produced by the terebellids and other metazoans. The occurrence of a similar association in the coralligenous build-ups seems to suggest that this symbiosis is not habitat-specific but develops in different marine settings, from open to confined habitats, where conditions of cryptic microenvironments in the framework of bioconstructions may promote the development of carbonatogenic microbial communities.
An early lithification of autochthonous micrite has been suggested by many authors (e.g. Grotzinger and Knoll, 1999; Reid et al., 2000) as an explanation for the modes of accretion and textures of various types of bioconstructions. The accretion of the Sicilian coralligenous was clearly produced by the superposition of different generations of skeletonized organisms, but early lithification of autochthonous micrite, inside primary and secondary cavities, further contributes to cementation and stabilization of the skeletal framework.
The detrital micrite generally derives from the degradation and transport of organism's skeletons, transported from neighbouring areas, or from the erosion of pre-existing bioclastic rocks (Stockman et al., 1967; Tucker and Wright 1990). The two different types of detrital micrite observed in the studied coralligenous (i.e. organic and inorganic) differ by the occurrence or absence of organic material trapped in the muddy sediment, the different degree of fluorescence under UV light, and the bioclast content. The common loose state of these sediments in the studied build-ups seems to point to their lithification rate being generally lower in comparison to the autochthonous micrite, and cementation may take place some time after the formation of the primary framework. The different amount of organic matter, however, may have a role in the lithification processes. Further knowledge is needed to help with comprehension of the diagenetic dynamic of the detrital micrite that would, in turn, help to clarify the general growth and morphological development of the coralligenous build-ups.
4.4 Coralligenous build-ups vs. biostalactites: comparison between recent bioconstructions of different marine settings
Knowledge of the compositional and morphological characterization of the coralligenous build-ups forming along the open marine sectors of the Mediterranean Sea shelf allows their comparison with bioconstructions forming in confined marine settings, such as blind submarine dark and semi-dark caves. These confined environments have been recently utilized as natural laboratories to study the role of metazoan and microbial communities in forming unusual bioconstructions (Guido et al., 2013; Gischler et al., 2017a). Due to the peculiar conditions of cave environments, notably low water circulation, reduced or null light intensity, oxygen depletion, and remarkable oligotrophy, caves are colonized mainly by cryptic organisms like serpulids, bryozoans, sponges and corals (Harmelin, 1985; Fichez, 1990, 1991). These organisms may be involved in the formation of small biogenic crusts or larger bioconstructions named biostalactites that develop under suitable conditions (Onorato et al., 2003; Belmonte et al., 2009, 2020; Guido et al., 2013, 2017b, 2019a, 2022; Sanfilippo et al., 2015; Gischler et al., 2017a, b; Onorato and Belmonte, 2017; Kazanidis et al., 2022). Serpulids and bryozoans are the main skeletal builders of the biostalactites that are further stabilized by the early cementation induced by the precipitation of autochthonous peloidal and aphanitic micrites mediated by microbial activity (Guido et al., 2013; Gischler et al., 2017a, b). Despite the difference in size (from a few centimetres up to 1–2 m), biostalactites forming in submarine caves of Sicily, Cyprus and Apulia show a uniform style of growth (Guido et al., 2013, 2017b, 2022). In contrast, the biotic crusts forming in the caves from the island of Lesvos of the Aegean Sea (Fara and Agios Vasilios caves) show a rich sponge association, widely present both on the surface and in the framework microcavities. There, the pervasive presence of sponges in almost all the micro-niches of the bioconstructions plays a limiting role in the development of heterotrophic bacteria (like sulfate-reducing bacteria) involved in carbonate precipitation (Guido et al., 2013, 2019a, b, 2022). The competition for space between sponges and carbonatogenic bacteria has been used to explain the morphological differences between large biostalactites and biogenic crusts common in the Mediterranean caves (Guido et al., 2019a, b). In the biotic crusts from Lesvos, sponge spicules are mainly concentrated in detrital micrite that fills primary cavities. They are only sporadically associated with autochthonous micrite, suggesting that organomineralization linked to soft-sponge-tissue decay is a relatively minor process in the in situ production of micrite (Guido et al., 2019a).
Very similar competition could be suggested for the coralligenous build-ups. Despite the different environmental conditions, size and morphologies characterizing the centimetre-sized biotic crust of Lesvos caves versus the some decimetre- to metre-sized coralligenous build-ups of Marzameni, sponges pervasively cover the surfaces and the internal cavities of both types of bioconstruction. The coralligenous framework is produced by encrusting red algae, but the primary inter-skeletal porosity derived from the superposition of different generations of skeletons is enhanced by the bioerosive activity of endolithic organisms. Though cavities could be a site for the settlement of anaerobic bacterial communities (Guido et al., 2013), they are occupied by insinuating and/or perforating endolithic sponges that reduce the availability of micro-niches favourable for the development of sulfate-reducing bacteria, hampering the precipitation of autochthonous micrite through their metabolic activity.
The decaying of the soft sponge tissue produces a huge number of spicules that are trapped in cavities together with fine detrital material. Occasionally, the spicules are engulfed in aphanitic micrite rich in organic matter that does not enclose detrital fragments. This material presumably results from induced and/or supported organomineralization of the soft tissue, like that observed in the biotic crusts of the Lesvos caves (Guido et al., 2019a). The same process has also been suggested for the Ordovician calathid–demosponge carbonate mounds of north-west China (Shen and Neuweiler, 2018).
The study of the build-ups from the Ionian Sea (Marzamemi area) offered the opportunity to investigate the relationship between skeletal builders and associated sediments. These components influence the general morphology and internal framework of the coralligenous and allow the development of a unique ecosystem where peculiar geobiological processes occur, making these build-ups natural laboratories that are useful for the palaeoecological reconstruction of the fossil record.
The studied build-ups are formed mainly of crustose coralline algae, which constitute a self-sustaining skeletal framework further stabilized by bryozoans and serpulids. The superposition of different generations of builders forms a highly porous structure. The porosity is further enhanced by bioerosive activity of perforating organisms. These discontinuities in the skeletal framework represent ideal niches for the colonization of cryptic organisms like sponges, bryozoans, serpulids and microbial communities. Among these, sponges are especially common with both insinuating and perforating taxa. After decaying of soft tissue, the spicules of these organisms accumulate in the cavities together with allochthonous micrite and other bioclasts.
Muddy to silty sediments represent the main non-skeletal carbonate component. Sediments consist mainly of allochthonous (detrital) components derived from external sources or from (bio)erosive processes of the build-up components. The autochthonous micrite, mineralized directly inside the build-up through organomineralization processes, represents a minor component. It shows mainly structureless textures, and it is associated with sponge spicules. The microbially derived micrite, showing peloidal to clotted peloidal texture, is rare and fills small intra- or inter-skeletal microcavities. Actually, sponges colonize the cryptic micro-niches which are ideal microenvironments for the growth of carbonatogenic bacteria, and the small quantities of autochthonous micrite engulfing the spicules probably results from induced and supported organomineralization of the soft tissue of sponges, rather than from microbial mediation. Despite the subordinate abundance in comparison to the skeletonized organisms, the occurrence of autochthonous micrite suggests a possible contribution of this component in cementing and strengthening the skeletal framework due to the early cementation of this type of micrite.
The formation of microbialites seems to be prevented by the competition between sponges and microbial communities colonizing the same cryptic spaces. The similar competition among these organisms in the biotic crusts of confined submarine caves suggests that this relationship is not habitat-specific. It may develop in similar microhabitats of different open to cryptic environments and could be used for palaeoecological reconstructions and for interpreting the role of metazoans and microbialite in the fossil build-ups.
All raw data can be provided by the corresponding authors upon request.
DB, AR and MM designed the project and allowed the collection of the samples; AdG and MC conducted the study and prepared the first draft. All authors contributed with ideas and in reviewing the manuscript.
The contact author has declared that none of the authors has any competing interests.
Publisher’s note: Copernicus Publications remains neutral with regard to jurisdictional claims made in the text, published maps, institutional affiliations, or any other geographical representation in this paper. While Copernicus Publications makes every effort to include appropriate place names, the final responsibility lies with the authors.
The authors wish to express their gratitude to the associate editor Chiara Borrelli and the reviewers, Fritz Neuweiler and the anonymous reviewer, for their constructive comments and suggestions. We thank Nunzio Pietralito and SUTTAKKUA diving school (Pachino, SR) and Riccardo Leonardi (University of Catania) for sampling and Alessandra Savini, Luca Fallati and Andrea Giulia Varzi (University of Milan-Bicocca) for providing underwater images. The authors sincerely thank Mariano Davoli and Chiara Benedetta Cannata of the Microscopy and Microanalysis (CM2) Laboratory Center, University of Calabria (SILA infrastructure), for SEM analysis. This is the Catania Palaeoecological Research Group contribution no. 507.
This research has been supported by the Ministero dell'Istruzione, dell'Università e della Ricerca – Fondo Integrativo Speciale per la Ricerca (FIRS), project: FISR2019_04543 “CRESCIBLUREEF – Grown: in the blue: new technologies for knowledge and conservation of Mediterranean reefs”.
This paper was edited by Chiara Borrelli and reviewed by Fritz Neuweiler and one anonymous referee.
Achlatis, M., Van der Zande R. M., Schönberg, C. H. L., Fang, J. K. H., Hoegh-Guldberg, O., and Sophie Dove, S.: Sponge bioerosion on changing reefs: ocean warming poses physiological constraints to the success of a photosymbiotic excavating sponge, Sci. Rep., 7, 10705, https://doi.org/10.1038/s41598-017-10947-1, 2017.
Aguirre, J., Perfectti, F., and Braga, J. C.: Integrating phylogeny, molecular clocks, and the fossil record in the evolution of coralline algae (Corallinales and Sporolithales, Rhodophyta), Paleobiology, 34, 519–533, 2010.
Altermann, W., Böhmer, C., Gitter, F., Heimann, F., Heller, I., Läuchli, B., and Putz, C.: “Defining biominerals and organominerals: direct and indirect indicators of life, Perry et al., Sediment. Geol., 201, 157–179”, Sediment. Geol., Response, 213, 150–151, 2009.
Anbu, P., Kang, C. H., Shin, Y. J., and So, J. S.: Formations of calcium carbonate minerals by bacteria and its multiple applications, Springer Plus, 5, 250, https://doi.org/10.1186/s40064-016-1869-2, 2016.
Ballesteros, E.: Mediterranean Coralligenous Assemblages, in Oceanography and Marine Biology, Ann. Rev., 44, 123–195, 2006.
Basso, D., Nalin, R., and Massari, F.: Genesis and composition of the Pleistocene Coralligene de plateau of the Cutro Terrace (Calabria, southern Italy), Jb. Geol. Paläont. Abh., 244, 173–182, 2007.
Basso, D., Nalin, R., and Nelson, C. S.: Shallow-water Sporolithon Rhodoliths from North Island (New Zealand), Palaios, 24, 92–103, 2009.
Basso, D., Bracchi, V. A., Bazzicalupo, P., Martini, M., Maspero, F., and Bavestrello, G.: Living coralligenous as geo-historical structure built by coralline algae, Front. Earth Sci., 10, 961632, https://doi.org/10.3389/feart.2022.961632, 2022.
Bazylinski, D. A. and Frankel, R. B.: Magnetosome formation in prokaryotes, Nat. Rev. Microbiol., 2, 217–230, 2004.
Blakemore, R.: Magnetotactic Bacteria, Science, 190, 377–379, 1975.
Bellan-Santini, D., Lacaze, J. C., and Poizat, C.: Les biocénoses marines et littorales de Méditerranée, Synthèse, menaces et perspectives, Muséum National d'Histoire Naturelle, Sécretariat de la Flore et de la Faune, Paris, 246 pp., 1994.
Belmonte, G., Ingrosso, G., Poto, M., Quarta, G., D'elia, M., Onorato, O. and Calcagnile, L.: Biogenic stalactites in submarine caves at the Cape of Otranto (SE Italy): dating and hypothesis on their formation, Mar. Ecol., 30, 376–382, 2009.
Belmonte, G., Guido, A., Mastandrea, A., Onorato, R., Rosso, A., and Sanfilippo, R.: Animal Forests in Submarine Caves, in: Perspectives on the Marine Animal Forests of the World, edited by: Rossi, S. and Bramanti, L., 129–145, Springer Nature Switzerland AG, 2020.
Benzerara, K., Miot, J., Morin, G., Ona-Nguema, G., Skouri-Panet, F., and Ferard, C.: Significance, mechanisms and environmental implications of microbial biomineralization, Cr. Geosci., 343, 160–167, 2011.
Bertolino, M., Calcinai, B., Cattaneo-Vietti, R., Cerrano, C., Lafratta, A., Pansini, M., Pica, D., and Bavestrello, G.: Stability of the sponge assemblage of Mediterranean coralligenous concretions along a millennial time span, Mar. Ecol., 35, 149–158, 2013.
Bertolino, M., Calcinai, B., Cattaneo-Vietti, R., Cerrano, C., Lafratta, A., Pansini, M., Pica, D., and Bavestrello, G.: Stability of the sponge assemblage of Mediterranean coralligenous concretions along a millennial time span, Ecology, 35, https://doi.org/10.1111/maec.12063, 2014.
Bertolino, M., Costa, G., Carella, M., Cattaneo-Vietti, R., Cerrano, C., Pansini, M., Quarta, G., Calcagnile, L., and Bavestrello, G.: The dynamics of a Mediterranean coralligenous sponge assemblage at decennial and millennial temporal scales, PLoS ONE, 12, e0177945, https://doi.org/10.1371/journal.pone.0177945, 2017a.
Bertolino, M., Cattaneo-Vietti, R., Costa, G., Pansini, M., Fraschetti, S., and Bavestrello, G.: Have Climate Changes Driven the Diversity of a Mediterranean Coralligenous Sponge Assemblage on a Millennial Time scale?, Palaeogeogr. Palaeocl., 487, 355–363, 2017b.
Bertolino, M., Costa, G., Cattaneo-Vietti, R., Pansini, M., Quarta, G., Calcagnile, L., and Bavestrello, G.: Ancient and recent sponge assemblages from the Tyrrhenian coralligenous over millennia (Mediterranean Sea), Facies, 65, 1–12, 2019.
Borch, T., Kretzschmar, R., Kappler, A., Van Cappellen, P., Ginder-Vogel, M., Voegelin, A., and Campbell, K.: Biogeochemical redox processes and their impact on contaminant dynamics, Environ. Sci. Technol., 44, 15–23, 2010.
Bosence, D. W. J. and Pedley, H. M.: Sedimentology and Palaeoecology of a Miocene Coralline Algal Biostrome from the Maltese Islands, Palaeogeogr. Palaeocl., 38, 9–43, 1982.
Bracchi, V. A., Nalin, R., and Basso, D.: Paleoecology and Dynamics of Coralline Dominated Facies during a Pleistocene Transgressive-Regressive Cycle (Capo Colonna Marine Terrace, Southern Italy), Palaeogeogr. Palaeocl., 414, 296–309, 2014.
Bracchi, V., Savini, A., Marchese, F., Palamara, S., Basso, D., and Corselli, C.: Coralligenous Habitat in the Mediterranean Sea: a Geomorphological Description from Remote Data, Ital. J. Geosci., 134, 32–40, 2015.
Bracchi, V. A., Nalin, R., and Basso, D.: Morpho-structural Heterogeneity of Shallow-Water Coralligenous in a Pleistocene Marine Terrace (Le Castella, Italy), Palaeogeogr. Palaeocl., 454, 101–112, 2016.
Bracchi, V. A., Basso, D., Marchese, F., Corselli C., and Savini A.: Coralligenous morphotypes on subhorizontal substrate: A new categorization, Cont. Shelf Res., 144, 10–20, 2017.
Bracchi, V. A., Basso, D., Savini, A. E., and Corselli, C.: Algal Reefs (Coralligenous) from Glacial Stages: Origin and Nature of a Submerged Tabular Relief (Hyblean Plateau, Italy), Mar. Geol., 411, 119–132, 2019.
Bracchi V. A., Bazzicalupo P., Fallati L., Varzi A. G., Savini A., Negri M. P., Rosso A., Sanfilippo R., Guido A., Bertolino M., Costa, G., De Ponti, E., Leonardi, R., Muzzupappa, M., and Basso, D.: The main builders of Mediterranean coralligenous: 2D and 3D quantitative approaches for its identification, Front. Earth Sci., 10, 910522, https://doi.org/10.3389/feart.2022.910522, 2022.
Braga, J. C., Martìn, J. M., and Riding, R.: Controls on microbial dome development along a carbonate-siliciclastic shelf-basin transect, Miocene, S.E. Spain, Palaios, 10, 347–361, 1995.
Bressan, G., Babbini, L., Ghirardelli, L., and Basso, D.: Bio-costruzione e bio-distruzione di corallinales nel mar mediterraneo, Biol. Mar. Mediterr., 8, 131–174, 2001.
Buczynski, C. and Chafetz, H. S.: Habit of bacterially induced precipitates of calcium carbonate and the influence of medium viscosity on mineralogy, J. Sediment. Petrol., 61, 226–233, 1991.
Calcinai, B., Bavestrello, G., Cuttone, G., and Cerrano, C.: Excavating sponges from the Adriatic Sea: description of Cliona adriatica sp. nov. (Demospongiae: Clionaidae) and estimation of its boring activity, J. Mar. Biol. Assoc. UK, 91, 339–346, 2011.
Calcinai, B., Bertolino, M., Bavestrello, G., Montori, S., Mori, M., Pica, D., Valisano, L., and Cerrano, C.: Comparison between the sponge fauna living outside and inside the coralligenous bioconstruction: a quantitative approach, Mediterr. Mar. Sci., 16, 413–418, 2015.
Calcinai, B., Sacco Perasso, C., Davide Petriaggi, B., and Ricci, S.: Endolithic and epilithic sponges of archaeological marble statues recovered in the Blue Grotto, Capri (Italy) and in the Antikythera shipwreck (Greece), Facies, 65, 21, https://doi.org/10.1007/s10347-019-0562-7, 2019.
Carannante, G. and Simone, L.: Rhodolith Facies in the Central-southern Apennines Mountains, Italy, in: Models for Carbonate Stratigraphy from Miocene Reef Complexes of Mediterranean Regions, Italy, edited by: Franseen, E. K., Esteban, M., Ward, W. C., and Rouchy, J. M., 261–275, SEPM Concepts Sedimentol. Paleontol., 1996.
Cerrano, C., Bastari, A., Calcinai, B., Di Camillo, C., Pica, D., Puce, S., Valisano, L., and Torsani, F.: Temperate mesophotic ecosystems: gaps and perspectives of an emerging conservation challenge for the Mediterranean Sea, The Eur. Zool. J., 8, 370–388, 2019.
Cerrano, C., Bavestrello, G., Bianchi, C. N., Calcinai, B., Cattaneo-Vietti, R., Morri, C., and Sarà, M.: The Role of Sponge Bioerosion in Mediterranean Coralligenous Accretion, in: Mediterranean Ecosystems: Structures and Processes, edited by: Faranda, F. M., Guglielmo, L., and Spezie, G., 235–240, Springer Milan, 2001.
Chafetz, H. S.: Marine peloids: a product of bacterially induced precipitation of calcite, J. Sediment. Petrol., 56, 812–817, 1986.
Cipriani, M., Basso, D., Bazzicalupo, P., Bertolino, M., Bracchi, V. A., Bruno, F., Costa, G., Dominici, R., Gallo, A., Muzzupappa, M., Rosso, A., Sanfilippo, R., Sciuto, F., and Guido, A.: The role of non-skeletal carbonate component in Mediterranean Coralligenous: new insight from the CRESCIBLUREEF project, Rend, Online Soc. Geol. It., 59, 75–79, 2023.
Corriero, G., Scalera Liaci, L., Ruggiero, D., and Pansini, M.: The sponge community of a semi-submerged Mediterranean cave, Mar. Ecol., 21, 85–96, 2000.
Costa, G., Bavestrello, G., Micaroni, V., Pansini, M., Strano, F., and Bertolino, M.: Sponge Community Variation along the Apulian Coasts (Otranto Strait) over a Pluri-Decennial Time Span. Does Water Warming Drive a Sponge Diversity Increasing in the Mediterranean Sea?, J. Mar. Biol. Ass., 99, 1519–1534, 2019.
Deias, C., Guido, A., Sanfilippo, R., Apollaro, C., Dominici, R., Cipriani, M., Barca, D., and Vespasiano, G.: Elemental Fractionation in Sabellariidae (Polychaeta) Biocement and Comparison with Seawater Pattern: A New Environmental Proxy in a High-Biodiversity Ecosystem?, Water, 15, 1549, https://doi.org/10.3390/w15081549, 2023.
Delecat, S. and Reitner, J.: Sponge communities from the Lower Liassic of Adnet (Northern Calcareous Alps, Austria), Facies, 51, 385–404, 2005.
Delecat, S., Peckman, J., and Reitner, J.: Non-rigid cryptic sponges in oyster patch reefs (Lower Kimmeridgian, Langenberg/Oker, Germany), Facies, 45, 231–254, 2001.
Di Geronimo, I., Di Geronimo, R., Improta, S., Rosso, A., andSanfilippo, R.: Preliminary Observations on a Columnar Coralline Build-Up from off SE Sicily, Biol. Mar. Mediterr., 8, 1–10, 2001.
Di Geronimo, I., Di Geronimo, R., Rosso, A., and Sanfilippo, R.: Structural and Taphonomic Analysis of a Columnar Coralline Algal Build-Up from SE Sicily, Geobios, 35, 86–95, 2002.
Donato, G., Sanfilippo, R., Sciuto, F., D’Alpa, F., Serio, D., Bracchi,V. A., Bazzicalupo, P., Negri, P., Guido, A., Bertolino, M., Costa, G., and Basso, D.: Biodiversity of a Coralligenous Build-up off Marzamemi (SE Sicily, Ionian Sea), in UNEP SPA/RAC, Proceedings of the 4th Mediterranean Symposium on the Conservation of the Coralligenous and Other Calcareous Bio-Concretions, Genova, Italy, 151–152, 2022.
Dupraz, C. and Strasser, A.: Microbialites and micro-encrusters in shallow coral bioherms (Middle-Late Oxfordian, Swiss Jura Mountains), Facies, 40,101–130, 1999.
Dupraz, C., Reid, P. R., Braissant, O., Decho, A. W., Norman, R. S., and Visscher, P. T.: Processes of carbonate precipitation in modern microbial mats, Earth Sci. Rev., 96, 141–162, 2009.
Evcen, A. and Çinar, M. E.: Bioeroding sponge species (Porifera) in the Aegean Sea (Eastern Mediterranean), J. Black Sea/Medit. Environ., 21, 285–306, 2015.
Fichez, R.: Absence of redox potential discontinuity in dark submarine cave sediments as evidence of oligotrophic conditions, Estuar. Coast. Shelf Sci., 31, 875–881, 1990.
Fichez, R.: Suspended particulate organic matter in a Mediterranean submarine cave, Mar. Biol., 108, 167–174, 1991.
Folk, R. L. and Chafetz, H. S.: Bacterially induced microscale and nanoscale carbonate precipitates, in: Microbial Sediments, edited by: Riding, R. E. and Awramik, S. M., 40–49, Springer-Verlag, Berlin, Germany, 2000.
Garrabou, J. and Ballesteros, E.: Growth of Mesophyllum alternans and Lithophyllum frondosum (Corallinales, Rhodophyta) in the northwestern Mediterranean, Eur. J. Phycol., 35, 1–10, 2000.
Gennaro, P., Piazzi, L., Cecchi, E., Montefalcone, M., Morri, C., and Bianchi, C. N.: Monitoring and assessment of the ecological status of coralligenous habitat, The coralligenous cliff, ISPRA, Manuali e Linee Guida, 191bis, 2020.
Gischler, E., Heindel, K., Birgel, D., Brunner, B., Reitner, J., and Peckmann, J.: Cryptic biostalactites in a submerged karst cave of the Belize Barrier Reef revisited: Pendant bioconstructions cemented by microbial micrite, Palaeogeogr. Palaeocl., 278, 34–51, 2017a.
Gischler, E., Birgel, D., Brunner, B., Eisenhauer, A., Meyer, G., Buhre, S., and Peckmann, J.: A giant underwater stalactite from the Blue Hole, Belize, revisited: a complex history of massive carbonate accretion under changing meteoric and marine conditions, J. Sediment. Res., 87, 1260–1284, 2017b.
Glynn, P. W. and Manzello, D. P.: Bioerosion and coral reef growth: a dynamic balance, in: Coral Reefs in the Anthropocene, edited by: Birkeland, C., 67–97, Dordrecht, Springer, 2015.
Grotzinger, J. P. and Knoll, A. H.: Stromatolites in Precambrian carbonates: evolutionary mileposts or environmental dipsticks?, Annu. Rev. Earth Planet. Sci., 27, 313–358, 1999.
Guido, A., Mastandrea, A., Rosso, A., Sanfilippo, R., and Russo, F.: Micrite precipitation induced by sulphate reducing bacteria in serpulid bioconstructions from submarine caves (Syracuse, Sicily), Rend. Online Soc. Geol. Ital., 21, 933–934, 2012.
Guido, A., Heindel, K., Birgel, D., Rosso, A., Mastandrea, A., Sanfilippo, R., Russo, F., and Peckmann, J.: Pendant bioconstructions cemented by microbial carbonate in submerged marine caves (Holocene, SE Sicily), Palaeogeogr. Palaeocl., 388, 166–180, 2013.
Guido, A., Mastandrea, A., Rosso, A., Sanfilippo, R., Tosti, F., Riding, R., and Russo, F.: Commensal symbiosis between agglutinated polychaetes and sulfate reducing bacteria, Geobiology, 12, 265–275, 2014.
Guido, A., Mastandrea, A., Stefani, M., and Russo, F.: Role of autochthonous versus detrital micrite in depositional geometries of Middle Triassic carbonate platform systems, Geol. Soc. Am. Bull., 128, 989–999, 2016.
Guido, A., Rosso, A., Sanfilippo, R., Russo, F., and Mastandrea, A.: Microbial biomineralization in biotic crusts from a Pleistocene Marine Cave (NW Sicily, Italy), Geomicrobiol J., 34, 864–872, 2017a.
Guido, A., Jimenez, C., Achilleos, K., Rosso, A., Sanfilippo, R., Hadjioannou, L., Petrou, A., Russo, F., and Mastandrea, A.: Cryptic serpulid microbialite bioconstructions in the Kakoskali submarine cave (Cyprus, Eastern Mediterranean), Facies, 63, 21, https://doi.org/10.1007/s10347-017-0502-3, 2017b.
Guido, A., Gerovasileiou, V., Russo, F. Rosso, A., Sanfilippo, R., Voultsiadou, E., and Mastandrea, A.: Composition and biostratinomy of sponge-rich biogenic crusts in submarine caves (Aegean Sea, Eastern Mediterrenean), Palaeogeogr. Palaeocl., 534, 109338, https://doi.org/10.1016/j.palaeo.2019.109338, 2019a.
Guido, A., Gerovasileios, V., Russo, F., Rosso, A., Sanfilippo, R., Voultsiadou, E., and Mastandrea, A.: Dataset of biogenic crusts from submarine caves of the Aegean Sea: An example of sponges vs microbialites competition in cryptic environments, Data in Brief, 27, https://doi.org/10.1016/j.dib.2019.104745, 2019b.
Guido, A., Rosso, A., Sanfilippo, R., Miriello, D., and Belmonte, G.: Skeletal vs microbialite geobiological role in bioconstructions of confined marine environments, Palaeogeogr. Palaeocl., 593, 110920, https://doi.org/10.1016/j.palaeo.2022.110920, 2022.
Harmelin, J. G.: Organisation spatiale des communautés sessiles des grottes sousmarines de Méditerranée, in Rapports et Procés-Verbaux de la Commission International pour l'exploitation de la Mer Méditerranée, Monaco, 5, 149–153, 1985.
Hong, J. S.: Contribution a l'étude des Peuplements d'un Fond de Concrétionnement Corllaigène dans la Région Marsaillaise en Méditerranéee Nord-Occidentale, Bull. Korea Oc. Res. Develop. Inst., 4, 27–51, 1982.
Ingrosso, G., Abbiati, M., Badalamenti, F., Bavestrello, G., Belmonte, G., Cannas, R., Benedetti Cecchi, L., Bertolino, M., Bevilacqua, S., Bianchi, C. N., Bo, M., Boscari, E., Cardone, F., Cattaneo-Vietti, R., Cau, A., Cerrano, C., Chemello, R., Chimienti, G., Congiu, L., Corriero, G., Costantini, F., De Leo, F., Donnarumma, L., Falace, A., Fraschetti, S., Giangrande, A., Gravina, M. F., Guarnieri, G., Mastrototaro, F., Milazzo, M., Morri, C., Musco, L., Pezzolesi, L., Piraino, S., Prada, F., Ponti, M., Rindi, F., Russo, G. F., Sandulli, R., Villamor, A., Zane, L., and Boero, F.: Mediterranean Bioconstructions along the Italian Coast, Adv. Mar. Biol., 79, 61–136, 2018.
Jimenez, C., Achilleos, K., Petrou, A., Hadjioannou, L., Guido, A., Rosso, A., Gerovasileiou, V., Albano, P. G., Di Franco, D., Andreou, V., and Abu Alhaija, R.: A dream within a dream: Kakoskali Cave, a unique marine ecosystem in Cyprus (Levantine Sea), in: Marine Caves of the Eastern Mediterranean Sea, Biodiversity, Threats and Conservation, edited by: Oztürk, B., Turkish Marine Research Foundation (TUDAV), Istanbul, Turkey, Publication, 53, 91–110, 2019.
Kazanidis, G., Guido, A., Rosso, A., Sanfilippo, R., Roberts, J. M., and Gerovasileiou, V.: One on Top of the Other: Exploring the Habitat Cascades Phenomenon in Iconic Biogenic Marine Habitats, Diversity, 14, 290, https://doi.org/10.3390/d14040290, 2022.
Kazmierczak, J., Coleman, M. L., Gruszczynski, M., and Kempe, S.: Cyanobacterial key to the genesis of micritic and peloidal limestones in ancient seas, Acta Palaeontol. Pol., 41, 319–338, 1996.
Kennard, J. M. and James, N. P.: Thrombolites and stromatolites: two distinct types of microbial structures, Palaios, 1, 492–503, 1986.
Komeili, A.: Molecular mechanisms of magnetosome formation, Annu. Rev. Biochem., 76, 351–366, 2007.
La Rivière, M., Michez, N., Delavenne, J., Andres, S., Fréjefond, C., Janson, A.-L., Abadie, A., Amouroux, J.-M., Bellan, G., Bellan-Santini, D., Chevaldonné, P., Cimiterra, N., Derolez, V., Fernez, T., Fourt, M., Frisoni, F., Grillas, P., Harmelin, J. G., Jordana, E., Klesczewski, M., Labrune, C., Mouronval, J. B., Ouisse, V., Palomba, L., Pasqualini, V, Pelaprat, C., Pérez, T., Pergent, G., Pergent-Martini, C., Sartoretto, S., Thibaut, T., Vacelet, J., and Verlaque, M.: Fiches descriptives des biocénoses benthiques de Méditerranée, Paris: UMS PatriNat (OFB-CNRS-MNHN), 660, https://doi.org/10.1016/j.cgh.2020.03.064, 2021.
Laborel, J.: Le concrétionnement algal “Coralligene” et son importance géomorphologique en Méditerranée, Rec. Trav. Stn. Mar. d'Endoume, 37, 37–60, 1961.
Leinfelder, R. and Keupp, H.: Upper Jurassic mud mounds: Allochthonous sedimentation versus autochthonous carbonate production, in: Mud Mounds: A Polygenetic Spectrum of Fine-grained Carbonate, edited by: Reitner, J. and Neuweiler, F., 17–26, Facies, 1995.
Lowenstam, H. A. and Weiner, S.: On biomineralization, in: Biomineralization: Principles and Concepts in Bioinorganic Materials Chemistry, edited by: Mann, S., 216 pp., Oxford University Press, New York, 1989.
Mann, S.: Mineralization in biological systems, Struct. Bonding, 54, 125–174, 1983.
Mann, S.: Biomineralization: principles and concepts in bioinorganic materials chemistry, Oxford University Press, Oxford, 2001.
Marchese, F., Bracchi, V. A., Lisi, G., Basso, D., Corselli, C., and Savini, A.: Assessing Fine-Scale Distribution and Volume of Mediterranean Algal Reefs through Terrain Analysis of Multibeam Bathymetric Data, A Case Study in the Southern Adriatic Continental Shelf, Water, 12, 157, https://doi.org/10.3390/w12010157, 2020.
Marion, A. F.: Esquisse d'une topographie zoologique du Golfe de Marseille, Ann. Mus. Hist. Natur. Marseille, 1, 1–108, 1883.
Marlow, J., Schönberg, C. H. L., Davy, S. K., Haris, A., Jompa, J., and Bell, J. J.: Bioeroding sponge assemblages: the importance of substrate availability and sediment, J. Mar. Biol. Assoc. UK, 99, 343–358, 2018.
Monty, C. L. V.: The origin and development of cryptalgal fabrics, in: Stromatolites, Development in Sedimentology, edited by: Walter, M. R., 198–249, Elsevier, New York, 1976.
Nalin, R., Basso, D., and Massari, F.: Pleistocene coralline algal build-ups (coralligène de plateau) and associated bioclastic deposits in the sedimentary cover of Cutro marine terrace (Calabria, southern Italy), Geol. Soc. Lond. Spec. Publ., 255, 11–22, 2006.
Nava, H. and Carballo, J. L.: Environmental factors shaping boring sponge assemblages at Mexican Pacific coral reefs, Mar. Ecol. Evol. Persp., 34, 269–279, 2013.
Neuweiler, F., Gautret, P., Thiel, V., Lange, R., Michaelis, W., and Reitner, J.: Petrology of Lower Cretaceous carbonate mud mounds (Albian, N. Spain): insights into organomineralic deposits of the geological record, Sedimentology, 46, 837–859, 1999.
Neuweiler, F., Rutsch, M., Geipel, G., Reimer, A., and Heise, K. H.: Soluble humic substances from in situ precipitated microcrystalline calcium carbonate, internal sediment, and spar cement in a Cretaceous carbonate mud-mound, Geology, 28, 851–854, 2000.
Neuweiler, F., D'orazio, V., Immenhauser, A., Geipel, G., Heise, K.-H., Cocozza, C., and Miano, T. M.: Fulvic-acid-like organic compounds control nucleation of marine calcite under suboxic conditions, Geology, 31, 681–684, 2003.
Neuweiler, F., Daoust, I., Bourque, P. A., and Burdige, D.: Degradative Calcification of a Modern Siliceous Sponge from the Great Bahama Bank, The Bahamas: A Guide for Interpretation of Ancient Sponge-Bearing Limestones, J. Sediment. Res., 77, 552–563, 2007.
Neuweiler, F., Kershaw, S., Boulvain, F., Matysik, M., Sendino, C., Mcmenamin, M., and Munnecke, A.: Keratose sponges in ancient carbonates – A problem of interpretation, Sedimentology, 70, 927–969, 2023.
Onorato, R., Forti, P., Belmonte, G., Costantini, A., and Poto, M.: La grotta sottomarina lu Lampiùne: novità esplorative e prime indagini ecologiche, Thalass, Salentina, 26, 55–64, 2003.
Onorato, R. and Belmonte, G.: Submarine caves of the Salento Peninsula: Faunal aspects, Thalass, Salentina, 39, 47–72, 2017.
Pérès, J. M.: Major Benthic Assemblages, in Marine Ecology, edited by: Kinne O., John-Wiley Publ. Lond., 5, 373–522, 1982.
Pérès, J. M. and Picard, J.: Nouveau manuel de bionomie benthique de la Méditerranée, Rec. Trav. Stat. Mar. Endoume, 31, 137 pp., 1964.
Perry, R. S., Mcloughlin, N., Lynne, B. Y., Septhon, M. A., Oliver, J. D., Perry, C. C., Campbell, K., Engel, M. H., Farmer, J. D., Brasier, M. D., and Staley, J. T.: Defining biominerals and organominerals: direct and indirect indicators of life, Sediment. Geol., 201, 157–179, 2007.
Phillips, A. J., Gerlach, R., Lauchnor, E., Mitchell, A. C., Cunningham, A. B., and Spangler, L.: Engineered applications of ureolytic biomineralization: a review, Biofouling, 29, 715–733, 2013.
Pickard, N. A. H.: Evidence for microbial influence on the development of Lower Carboniferous buildups, in: Recent Advances in Lower Carboniferous Geology, edited by: Strogen, P., Somerville, I. D., and Jones, G. L., Geol. Soc. Lond. Spec. Publ., 107, 65–82, 1996.
Pratt, B. R.: Microbial contribution to reefal mud-mounds in ancient deep-water settings: Evidence from the Cambrian, in: Microbial Sediments, edited by: Riding, R. E. and Awramik, M., 282–288, Springer, Berlin, 2000.
Rasser, M. W.: Coralline Red Algal Limestones of the Late Eocene Alpine Foreland Basin in Upper Austria: Component Analysis, Facies and Palecology, Facies, 42, 59–92, 2000.
Reid, R. P., Visscher, P. T., Decho, A. W., Stolz, J. K., Bebout, B. M., Dupraz, C., Mactintyre, I. G., Paerl, H. W., Pinckney, J. L., Prufert-Bebout, L., Steppe, T. F., and DesMarais, D. J.: The role of microbes in accretion, lamination and early lithification of modern marine stromatolites, Nature, 406, 989–992, https://doi.org/10.1038/35023158, 2000.
Reitner, J.: Modern cryptic microbialite/metazoan facies from Lizard Island (Great Barrier Reef, Australia): formation and concepts, Facies, 29, 3–40, 1993.
Reitner, J. and Neuweiler, F.: Mud Mounds: A polygenetic spectrum of fine-grained carbonate buildups, Facies, 32, 1–70, 1995.
Reitner, J., Gautret, P., Marin, F., and Neuweiler, F.: Automicrites in modern marine microbialite. Formation model via organic matrices (Lizard Island, Great Barrier Reef, Australia), Bull. Inst. Océanogr. (Monaco), 14, 237–264, 1995.
Reolid, M.: Taphonomy of the Oxfordian-Lowermost Kimmeridgian Siliceous Sponges of the Prebetic Zone (Southern Iberia), J. Taphonomy, 5, 71–90, 2007.
Reolid, M.: Interactions between microbes and siliceous sponges from Upper Jurassic buildups of External Prebetic (SE Spain), Lect. Notes Earth Sci., 131, 319–330, 2010.
Riding, R.: Microbial carbonates: the geological record of calcified bacterial-algal mats and biofilms, Sedimentology, 47, 179–214, 2000.
Riding, R.: Structure and Composition of Organic Reefs and Carbonate Mud Mounds: Concepts and Categories, Earth-Sci. Rev., 58, 163–231, 2002.
Riding, R.: Microbialites, stromatolites, and thrombolites, in: Encyclopedia of Geobiology, Encyclopedia of Earth Science Series, edited by: Reitner, J. and Thiel, V., Springer, Heidelberg, 635–654, 2011.
Riding, R. and Liang, L.: Geobiology of microbial carbonates: metazoan and seawater saturation state influences on secular trends during the Phanerozoic, Palaeogeogr. Palaeocl., 219, 101–115, 2005.
Riding, R. and Tomás, S.: Stromatolite reef crusts, Early Cretaceous, Spain: bacterial origin of in situ precipitated peloid microspar?, Sedimentology, 53, 23–34, 2006.
Riding, R. and Virgone, A.: Hybrid Carbonates: in situ abiotic, microbial and skeletal coprecipitates, Earth Sci. Rev., 208, 103300, https://doi.org/10.1016/j.earscirev.2020.103300, 2020.
Riding, R., Liang, L., and Braga, J. C.: Millennial-scale ocean acidification and late Quaternary decline of cryptic bacterial crusts in tropical reefs, Geobiology, 12, 387–405, 2014.
Rosell, D. and Uriz, M. J.: Do associated zooxanthellae and the nature of the substratum affect survival, attachment and growth of Cliona viridis (Porifera: Hadromerida)? An experimental approach, Mar. Biol., 114, 503–507, 1992.
Rosso A. and Sanfilippo R.: The contribution of bryozoans and serpuloideans to coralligenous concretions from SE Sicily, in: UNEP-MAP-RAC/SPA, Proc. First Symposium on the Coralligenous and other calcareous bio-concretions of the Mediterranean Sea, Tabarka, 123–128, 2009.
Rosso, A., Sanfilippo, R., Guido, A., Gerovasileiou, V., Taddei Ruggiero, E., and Belmonte, G.: Colonisers of the dark: biostalactite-associated metazoans from “lu Lampiùne” submarine cave (Apulia, Mediterranean Sea), Mar. Ecol. 42, e12634, https://doi.org/10.1111/maec.12634, 2020.
Rosso, A., Altieri, C., Bazzicalupo, P., Bertolino, M., Bracchi, V.A., Bruno, F., Cipriani, M., Costa, G., D’alpa, F., Donato, G., Fallati, L., Gallo, A., Guido, A., Leonardi, R., Muzzupappa, M., Negri, M. P., Sanfilippo, R., Savini, A., Sciuto, F., Serio, D., Taddei Ruggiero, E., Varzi, A. G., Viola, A., and Basso D.: Bridging together research and technological innovation: first results and expected bearings of the project cresciblureef on mediterranean coralligenous. 4th Mediterranean Symposium on the conservation of Coralligenous & other Calcareous Bio-Concretions, Genoa, 108–113, 2023.
Sanfilippo, R., Rosso, A., Guido, A, Mastandrea, A., Russo, F., Riding, R., and Taddei Ruggiero, E.: Metazoan/microbial biostalactites from present-day submarine caves in the Mediterranean Sea, Mar. Ecol., 36, 1277–1293, 2015.
Sanfilippo, R., Rosso, A., Guido A., and Gerovasileiou, V.: Serpulid communities from two marine caves in the Aegean Sea, eastern Mediterranean, J. Mar. Biol. Assoc. UK, 97, 1059–1068, 2017.
Sartoretto, S., Verlaque, M., and Labore, J.: Age of settlement and accumulation rate of submarine “coralligène” (−10 to −60 m) of the northwestern Mediterranean Sea; relation to Holocene rise in sea level, Mar. Geol., 130, 317–331, 1996.
Schönberg, C. H. L.: A history of sponge erosion: from past myths and hypotheses to recent approaches, in: Current Developments in Bioerosion, edited by: Wisshak, M. and Tapanila, L., 165–202, Springer-Verlag Berlin, 2008.
Sciuto, F., Altieri, C., Basso, D., D’Alpa, F., Donato, G., Bracchi,V. A., Cipriani, M., Guido, A., Rosso, A., Sanfilippo, R., Serio, D., and Viola, A.: Preliminary data on ostracods and foraminifers living on coralligenous bioconstructions Offshore Marzamemi (Ionian Sea, Se Sicily), Rev. Micropaléontol., 18, 100711, https://doi.org/10.1016/j.revmic.2023.100711, 2023.
Scott, P. J. B., Moser, K. A., and Risk, M. J.: Bioerosion of concrete and limestone by marine organisms: a 13 year experiment from Jamaica, Mar. Pollut. Bull., 19, 219–222, 1988.
Shen, Y. and Neuweiler, F.: Questioning the microbial origin of automicrite in Ordovician, Calathid–Demosponge carbonate mounds, Sedimentology, 65, 303–333, 2018.
Stockman, K. W., Ginsburg, R. N., and Shinn, E. A.: The production of lime mud by algae in South Florida, J. Sediment. Petrol., 37, 633–648, 1967.
Titschack, J., Nelson, C. S., Beck, T., Freiwald, A., and Radtke, U.: Sedimentary Evolution of a Late Pleistocene Temperate Red Algal Reef (Coralligène) on Rhodes, Greece: Correlation with Global Sea-Level Fluctuations, Sedimentology, 55, 1747–1776, 2008.
Trichet, J. and Défarge, C.: Non biologically supported organomineralization, in: Proc. 7th Int. Symp. Biomineralization, edited by: Allemand, D. and Cuif, J. P., Bulletinde l'Institut Océanographique de Monaco, 14, 203–236, 1995.
Tucker, M. E. and Wright, V. P.: Carbonate Sedimentology, London, Blackwell Scientific Publications, 488 pp., 1990.
Turicchia, E., Abbiati, M., Bettuzzi, M., Calcinai, B., Morigi, M. P., Summers, A. P., and Ponti, M.: Bioconstruction and Bioerosion in the Northern Adriatic Coralligenous Reefs Quantified by X-Ray Computed Tomography, Front. Mar. Sci., 8, 790869, https://doi.org/10.3389/fmars.2021.790869, 2022.
Van Driessche, A. E. S., Stawski, T. M., and Kellermeier, M.: Calcium sulfate precipitation pathways in natural and engineered environments, Chem. Geol., 530, 119274, https://doi.org/10.1016/j.chemgeo.2019.119274, 2019.
Varzi, A. G., Fallati, L., Savini, A., Bracchi, V. A., Bazzicalupo, P., Rosso, A., Sanfilippo, R., Bertolino, M., Muzzupappa, M., and Basso, D.: Geomorphological mapping of Coralligenous reefs offshore southeastern Sicily (Ionian Sea), J. Maps, 19, 2161963, https://doi.org/10.1080/17445647.2022.2161963, 2023.
Warnkle, K.: Calcification processes of siliceous sponges in Visean Limestones (Counties Sligo and Leitrim, northwestern Ireland), Facies, 33, 215–228, 1995.
Weiner, S. and Dove, P. M.: An overview of biomineralization processes and the problem of the vital effect, Biomineralization, 54, 1–29, 2003.
Wolf, K. H.: Gradational sedimentary products of calcareous algae, Sedimentology, 5, 1–37, https://doi.org/10.1111/j.1365-3091.1965.tb01556.x, 1965.
Wood, R.: Are reefs and mounds really so different?, Sediment. Geol., 145, 161–171, 2001.