the Creative Commons Attribution 4.0 License.
the Creative Commons Attribution 4.0 License.
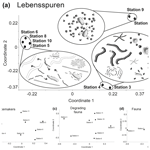
Diversity and density relationships between lebensspuren and tracemaking organisms: a study case from abyssal northwest Pacific
Olmo Miguez-Salas
Angelika Brandt
Henry Knauber
Torben Riehl
In the deep sea, interactions between benthic fauna and seafloor sediment primarily occur through bioturbation that can be preserved as traces (i.e. lebensspuren). Lebensspuren are common features of deep-sea landscapes and are more abundant than the organisms that produce them (i.e. tracemakers), rendering lebensspuren promising proxies for inferring biodiversity. The density and diversity relationships between lebensspuren and benthic fauna remain unclear, and contradicting correlations have been proposed (i.e. negative, positive, or even null correlations). To approach these variable correlations, lebensspuren and benthic fauna were characterized taxonomically at eight deep-sea stations in the Kuril-Kamchatka Trench area, together with two novel categories: tracemakers (specific epibenthic fauna that produce these traces) and degrading fauna (benthic fauna that can erase lebensspuren). No general correlation (overall study area) was observed between diversities of lebensspuren, tracemakers, degrading fauna, and fauna. However, a diversity correlation was observed at specific stations, showing both negative and positive correlations depending on: (1) the number of unknown tracemakers (especially significant for dwelling lebensspuren); (2) the lebensspuren with multiple origins; and (3) tracemakers that can produce different lebensspuren. Lebensspuren and faunal density were not correlated. However, lebensspuren density was either positively or negatively correlated with tracemaker densities, depending on the lebensspuren morphotypes. A positive correlation was observed for resting lebensspuren (e.g. ophiuroid impressions, Actiniaria circular impressions), while negative correlations were observed for locomotion-feeding lebensspuren (e.g. echinoid trails). In conclusion, lebensspuren diversity may be a good proxy for tracemaker biodiversity when the lebensspuren–tracemaker relationship can be reliable characterized. Lebensspuren–density correlations vary depending on the specific lebensspuren residence time, tracemaker density, and associated behaviour (rate of movement). Overall, we suggest that lebensspuren density and diversity correlations should be studied with tracemakers rather than with general benthic fauna. On a global scale, abiotic (e.g. hydrodynamics, substrate consistency) and other biotic factors (e.g. microbial degradation) may also play an important role.
- Article
(5610 KB) - Full-text XML
-
Supplement
(306 KB) - BibTeX
- EndNote
Neoichnology studies the interactions between animals and substrates (e.g. bioturbation) in modern environments as well as the biogenic sediment resulting from these interactions, the so-called lebensspuren (German for “life traces”; e.g. faecal casts, trails, mounds, burrows) (Ewing and Davis, 1967; Gage and Tyler, 1991). In the marine realm, lebensspuren analyses are usually image based (e.g. Bell et al., 2013; Miguez-Salas et al., 2023). Lebensspuren are portraits of the diverse linkages between environmental conditions and the animal responses to them. Thus, neoichnological analyses offer a useful tool set for deducing environmental factors both in contemporary and in past environments through comparisons between lebensspuren and trace fossils (Buatois and Mángano, 2011). However, neoichnology as a field is not yet as developed as paleoichnology (i.e. trace fossil research), and most quantitative studies are restricted to shallow marine environments and tank experiments (e.g. shoreface, foreshore, marginal marine settings) (La Croix et al., 2022, and references therein). Even though the abyssal zone (i.e. 3500–6500 m deep) represents the largest marine ecosystem and covers approx. 75 % of the seafloor (Ramirez-Llodra et al., 2010; Watling et al., 2013), neoichnological analyses are scarce and limited by the cost of observation and sampling procedures (e.g. Heezen and Hollister, 1971; Przeslawski et al., 2012; Bell et al., 2013; Miguez-Salas et al., 2022). As such, neoichnological analyses are emerging as a promising tool for furthering our understanding of deep-sea environments and faunal–sediment interactions.
Quantitative marine ecological research comprises two main components – diversity and density analyses (Halpern and Warner, 2002). Deep-sea neoichnological studies have addressed diversity and density characterizations by considering all identified lebensspuren as morphotypes (Przeslawski et al., 2012; Bell et al., 2013). However, tracemaker (i.e. the benthic organisms that produce lebensspuren) diversity and density have been approached from a generalist perspective as megafauna, epifauna, or lebensspuren-forming epifauna (Young et al., 1985; Dundas and Przeslawski, 2009; Przeslawski et al., 2012; Bell et al., 2013).
Early deep-sea neoichnological studies suggested a correlation between lebensspuren and faunal diversity (Kitchell et al., 1978; Young et al., 1985). However, more recent studies show no significant correlation between epifaunal and lebensspuren richness (Przeslawski et al., 2012). Bell et al. (2013) stated that “improvements in imaging technology allow [for] more refined classification of lebensspuren and organisms, which may affect the strength of the correlation between faunal and lebensspuren diversity, compared with the more direct proportionality of faunal and lebensspuren diversity demonstrated in earlier studies”. Thus, in deep-sea research, diversity comparisons based on more precise taxonomic tracemaker identification and differentiation are a pending task, promising a deeper understanding of the dependencies between fauna and lebensspuren variability.
Early studies found an inverse relationship between lebensspuren and faunal density (Kitchell et al., 1978; Young et al., 1985; Gerino et al., 1995). These studies suggested that this relationship is related to the fact that lebensspuren formed in low biomass regions have the capacity to persist for a long time (high residence time), ultimately leading to a steady increase of the lebensspuren density through accumulation. Nevertheless, recent data seemed to contradict this initial assumption. Przeslawski et al. (2012) observed no discernible correlation between lebensspuren and epifaunal abundance. By contrast, Bell et al. (2013) reported a strong positive correlation between lebensspuren and faunal densities (see Fig. 10 in Bell et al., 2013). The results from Bell et al. (2013) suggest that megafaunal activity might not be the only factor influencing the preservation or destruction of lebensspuren. Small-scale biotic factors (e.g. microbial degradation), as well as abiotic factors (e.g. hydrodynamic regimes, sedimentation rates, sediment composition) potentially limit lebensspuren residence time and density across different spatial scales in the deep sea (Wheatcroft et al., 1989; Smith et al., 2005; Miguez-Salas et al., 2020). In summary, earlier investigations and their contradicting results highlight that neoichnology and its fundamental concepts are still in their early stages and warrant further investigation.
Despite the prevalence of lebensspuren on the deep seafloor (Heezen and Hollister, 1971), only a few organisms are recognized in the process of forming these features. Thus, understanding the density–diversity relationship between lebensspuren and benthic megafauna may help decipher indirectly the variability of the former (i.e. without having seen the organisms). The research presented here aims to compare diversity indices and densities of lebensspuren, tracemakers (specific organisms that produce them), degrading fauna (benthic fauna that can erase lebensspuren), and megabenthic fauna from the northwest Pacific abyssal plain, near the Kuril-Kamchatka Trench (KKT) (Fig. 1). By conducting a detailed classification of both lebensspuren and tracemakers, this research aims to go one step further, with the main objective of testing how diversity and density relationships vary from previous results where lebensspuren was compared with fauna diversity in a much coarser taxonomic resolution. This geographic region has been studied extensively, with research dating back from 11 expeditions aboard the R/V Vityaz (1949, 1953, and 1966) to more recent research efforts aboard the R/V Sonne (KuramBio I (2012) and KuramBio II (2016)). These expeditions have significantly contributed to establishing the most comprehensive taxonomic foundation for fauna in this region (e.g. Zenkevitch et al., 1955; Zenkevitch, 1963; Belyaev, 1983; Brandt and Malyutina, 2015; Brandt et al., 2020; Saeedi and Brandt, 2020).
2.1 Study sites, data acquisition, and video analysis
The joint German–Russian expedition KuramBio 1 (Kuril-Kamchatka Biodiversity Studies) aboard the RV Sonne (cruise SO223) explored the KKT and its adjacent abyssal plain from 21 July to 7 September 2012 (Brandt and Malyutina, 2012). During the KuramBio 1 expedition, 13 Ocean Floor Observation System (OFOS) deployments were conducted (Table 1). The aim of these deployments was to use the OFOS to study 11 deep-sea stations spanning a range of depths (4868–5768 m), located between 34-48∘ N and 147-157∘ E (Fig. 1). Stations 1, 2, and 5–11 were located in the abyssal plains adjacent to the KKT, while stations 3 and 4 were located at the upper slope of the KKT (Fig. 1) (Table 1).
Table 1Station data of the OFOS deployments during KuramBio (2012). “Start” and “End” coordinates refer to the time between bottom view and beginning of heaving (survey duration). Note: The first four deployments were aborted due to technical problems.
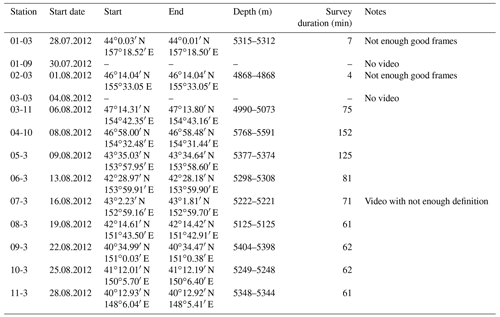
The OFOS was lowered into the water at the conductivity, temperature, and depth position. For the first 300 m, the OFOS was lowered at 0.5 m s−1. Then, the speed was increased to 0.8 m s−1 while the ship was kept in position. The speed of the OFOS was reduced to 0.5 m s−1 once it was 500 m above the ground, and further reduced to 0.3 m s−1 once it was 200 m above the ground. Once the bottom was visible, the winch lowering the OFOS was stopped and the ship resumed steaming at 0.5 kn in a direction chosen depending on the water current and wind situation. Then, the winch operator manually kept the OFOS at an appropriate distance from the seafloor to observe the seafloor benthos. Two laser pointers with 10 cm between them were used as a scale. The first four deployments were aborted due to technical problems, affecting stations 1–3 (Table 1). Thus, limited video footage was obtained. Moreover, station 7 lacked high-definition video and was therefore not considered for the current analysis. All technical aspects, including pre-deployment preparation, and post-deployment maintenance (i.e. including video download) were managed by a scientific–technical service (“WTD”, Wissenschaftlich-Technischer Dienst, Jörg Leptien, Reederei).
Still images were extracted from the OFOS videos at a frequency of one frame per 5 s (Miguez-Salas and Riehl, 2023a). These still images were then sub-sampled to delete frames that were out of focus so as to minimize frame overlap. Out-of-focus frames were defined as frames showing the OFOS moving up and down due to ocean swell. Fifty frames per station (400 still images in total), covering a seafloor area of 878 m2 (109 m2 per station approx.), were randomly selected and uploaded to the BIIGLE 2.0 software for later annotation and measurements (Langenkämper et al., 2017). Specific frames were treated with Fiji software (Schindelin et al., 2012) to enhance the visibility (CLAHE tool) of certain lebensspuren features (Miguez-Salas et al., 2019).
2.2 Lebensspuren classification and tracemaker identification
Lebensspuren morphotypes were categorized in terms of inferred tracemaker behaviour during their formation, their morphology, and the taxonomic origin of the tracemakers. The behavioural classification was adapted from Seilacher's (1954) categories for marine lebensspuren: (1) resting (imprints of stationary animals); (2) locomotion–feeding (sediment displaced by the movement of deposit feeders and surface sediment disturbances formed as organisms are foraging); (3) wasting (e.g. faecal casts, pellets); and (4) dwelling (e.g. mounds and burrows). The morphological classification followed previous morphological names (e.g. Ewing and Davis, 1967; Young et al., 1985; Dundas and Przeslawski, 2009; Przeslawski et al., 2012; Althaus et al., 2015), where such names exist. The morphological features measured included in the classification were length, width, and diameter. Lebensspuren with unclear morphology and origin (e.g. degraded faecal casts, trails with diffuse outlines) were excluded from analyses. Additionally, lebensspuren and fauna smaller than 1 cm (macrofauna and smaller) were also excluded from analyses as the resolution of the still images is below high-definition ( pixels). Hence, this study focuses only on megafauna (i.e. fauna >1 cm), implied whenever fauna is mentioned in this study henceforth.
This study aimed for the species rank, which is the fundamental, gold standard taxonomic level to which ecological studies generally aspire. However, names of described species could not be attached to these taxa in the majority of our image-based observations for reasons of limitations in the image quality and the general difficulty of observing diagnostic characteristics in the in situ photographs. As such, open nomenclature has been used instead, following the recommendations for image-based identifications laid out by Horton et al. (2021). Fauna has been grouped into different categories for comparisons with the diversity and density of lebensspuren: (1) tracemakers (fauna that has been clearly recognized as makers of a trace); (2) degrading fauna (fauna that can affect lebensspuren density negatively by eroding the seafloor); and (3) benthic fauna (all fauna identified in the still images).
2.3 Statistical analysis
Diversity indices (Shannon–Wiener H′ (log e) and Simpson's D) and evenness (J′) were calculated for four groups: lebensspuren, tracemaker fauna, degrading fauna, and fauna. As the data from all groups show non-parametric distribution for all stations, diversity variability among stations was tested using the Wilcoxon signed-rank test (considering all groups and all indices). The Spearman rank correlation was used to test the relationships between the diversity indices of all groups. As the number of frames was the same (i.e. same observation area), density correlation analyses were performed by separately considering the total density for each group at every station. Additionally, lebensspuren and tracemaker densities were further subdivided into three groups: wasting, resting, and locomotion-feeding. Dwelling was not considered as the tracemakers of most dwelling lebensspuren are unknown.
Multivariate analysis was conducted to investigate potential differences within the groups (lebensspuren, tracemakers, degrading fauna, and benthic fauna). First, a square root transformation was carried out to give less weight to the more abundant taxa of organisms and lebensspuren morphotypes. Then, differences in the composition of the four groups between stations were assessed using hierarchical cluster analysis and displayed as non-metric multidimensional scaling plots (n-MDS). Both plots were constructed using the Bray–Curtis similarity index. All statistical procedures were conducted using PAST v.4.12 (Hammer et al., 2001).
A total of 9426 lebensspuren were identified and classified from 400 still images (see Supplement), corresponding to 23 morphotypes associated with dwelling, wasting, resting, and locomotion–feeding behaviours (Fig. 2 and Table 2) (for raw dataset report at each station, see Miguez-Salas and Riehl, 2023b). The fauna comprised a total of 4009 individual animals that were classified into 93 different taxa, of which 66 were classified as degrading fauna and 43 as tracemakers (with 790 and 676 individuals, respectively) (Table 3; Miguez-Salas and Riehl, 2023b). Linking dwelling lebensspuren with tracemakers proved to be challenging, with the exception of rare and ambiguous cases where vermiform organisms, most likely polychaetes, partially emerged from paired burrows (Fig. 2P). Tracemaker identification was possible in most of the cases for wasting lebensspuren. It is common for different tracemakers to produce the same lebensspuren morphotypes and it is also common for one tracemaker (taxon) to produce several lebensspuren morphotypes (see Table 2). However, in the case of cf. Elpidia – the most abundant tracemaker of station 4 (see Miguez-Salas and Riehl, 2023b) – the complete characterization of its associated rounded faecal cast (smaller than 1 cm) was impossible due to image resolution limitations. Tracemaker identification of locomotion–feeding lebensspuren was mostly possible except for mounded trails which have been produced by endobenthic organisms. However, as for wasting lebensspuren (see Supplement), also in this case different tracemakers can be responsible for similar trails (see Table 2). Tracemaker identification of resting lebensspuren was possible for most of the cases.
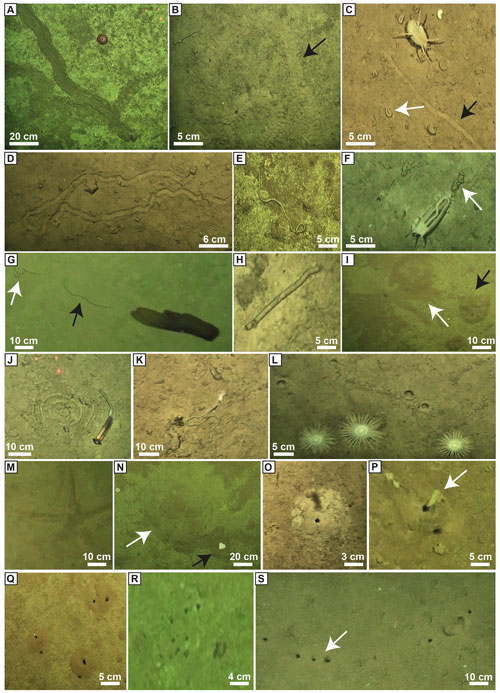
Figure 2Examples of lebensspuren morphotypes observed and quantified in this study. (A) M-ridged trail produced by Asteroidea fam. indet. gen. indet. sp. 1. (B) Mounded trail (unknown tracemaker). (C) Flat trail (black arrow; unknown tracemaker) and rounded faecal casts (white arrow) produced by Scotoplanes sp. 1. (D) M-ridged trail produced by Echinoidea fam. indet. gen. indet. sp.5; (E) Wavy faecal cast produced by Peniagone sp. 1 to Peniagone sp. 3. (F) Knotted faecal cast produced by Peniagone sp.1 to Peniagone sp. 3. (G) Coiled-curly (white arrow) and smooth (black arrow) faecal cast produced by Psychropotes sp. 2. (H) Smooth (black arrow) faecal cast produced by various tracemakers (see Table 2). (I) Rosette-shape trace (white arrow) produced by an echiuran worm and mound shape nearby (black arrow). (J) Spiral faecal cast produced by Enteropneusta fam. indet. gen. indet. sp. 1. (K) Switchbacks faecal cast produced by Torquaratoridae. gen. sp. 1. (L) Circular impression produced by Actiniaria fam. indet. gen. indet. sp. 1. (M) Asteroid impression produced by an Asteroidea (Asteroidea fam. indet. gen. indet. spp. 3, 4, 7, 8, 9). (N) Mound (white arrow) with a semi-buried asteroidean nearby (black arrow). (O) Single burrow located in the apex of a cone-shaped mound. (P) Paired burrow with an unidentified organism coming out. (Q) Three paired burrows. (R) Cluster burrows. (S) Lined burrows (black arrow).
Table 2Lebensspuren and associated tracemakers identified in the present study. Note that several lebensspuren can be produced by different tracemakers.
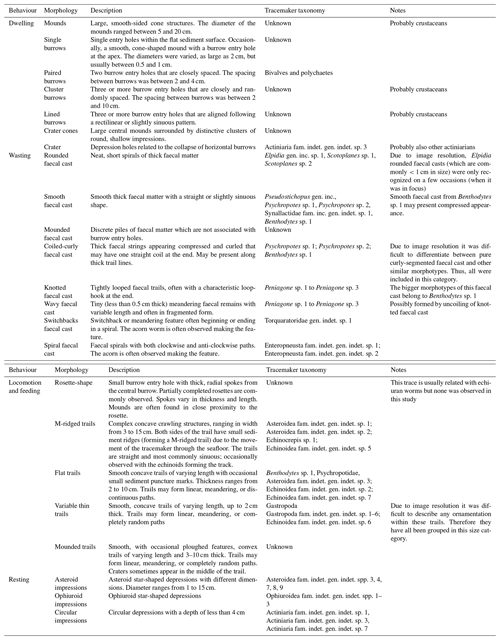
Table 3Total number of lebensspuren, tracemakers, degrading fauna, and fauna identified through the eight deep-sea stations at the Kuril Kamchatka area.
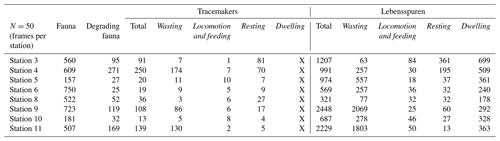
The Wilcoxon signed-rank test results revealed that the median diversity was significantly different between stations for all groups and was lowest at stations 9 and 11 (Fig. 3). Moreover, faunal diversity showed a standard deviation 3 orders smaller than the values reported for lebensspuren, tracemakers, and degrading fauna; showing that faunal diversity was more consistent among sites than the other diversity indices. Lebensspuren diversity indices (Shannon–Wiener, Simpson's, and evenness) of the overall KKT area (considering all eight stations together) showed no correlation with comparable diversity indices from the other three groups (tracemakers, degrading fauna, and benthic fauna). The only strong diversity correlation resulting from the Spearman rank analysis was between tracemakers and degrading fauna (R2>0.88, p<0.01).
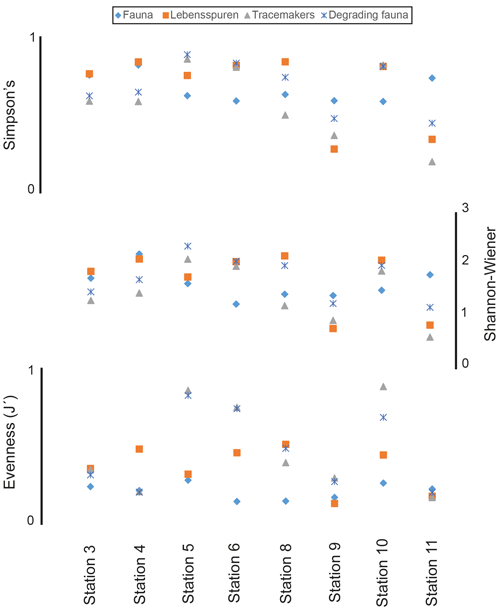
Figure 3Comparison of median diversity indices (Simpson's, Shannon–Wiener, and evenness) of lebensspuren, tracemakers, degrading fauna, and fauna at each station. Each lebensspuren morphotype was considered a different species for the calculations.
The density correlation matrix revealed no significant correlation between the fauna and the other groups (see Fig. 4). The degrading fauna showed a positive correlation with tracemakers and waste tracemaker densities. Also, tracemakers and waste tracemaker densities are positively correlated (Fig. 4). A positive density correlation was obtained between lebensspuren and wasting lebensspuren as well as resting lebensspuren and resting tracemakers. A negative correlation was observed for locomotion–feeding lebensspuren and their tracemakers (Fig. 4).
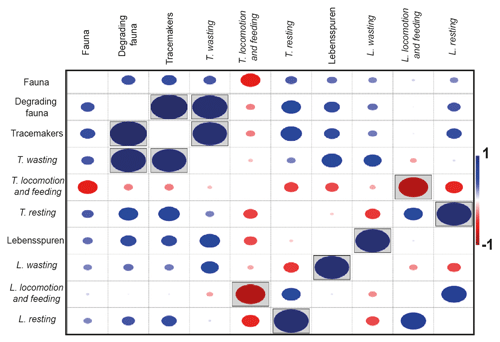
Figure 4Density correlation matrix for lebensspuren, tracemakers, degrading fauna, and fauna. Lebensspuren and tracemaker densities were subdivided into wasting, resting, and locomotion–feeding (dwelling was not considered since the tracemakers of most dwelling lebensspuren are unknown). Boxed dots indicate correlations where p<0.05.
Lebensspuren assemblages were generally similar among stations (Fig. 5a), ranging from 75 %–82 % similarity in the cluster analysis. The n-MDS showed that lebensspuren assemblages from stations 5, 6, 8, and 10 are different from the trench (stations 3 and 4) and the southern stations (stations 9 and 11) (Fig. 6a). The southern stations were less diverse, similar (82 % similarity; Fig. 5a) and dominated by rounded faecal casts produced by Scotoplanes spp. The trench stations were characterized by diverse and slightly less similar assemblages (75 % of similarity) dominated by dwelling lebensspuren (e.g. paired, lined or cluster burrows), knotted faecal casts (Peniagone spp.), ophiuroid impressions (Ophiuroidea), circular impressions (Actiniaria) and M-ridged trails (Asteroidea and Echinocrepsis spp.). Stations 5, 6, 8, and 10 showed diverse lebensspuren assemblages dominated by smooth (cf. Benthodytes, Psychropotidae) and coiled-curly faecal casts (Psychropotidae), rosette-shaped traces and flat trails (Asteroidea, cf. Benthodytes, Psychropotidae) (Fig. 6a).
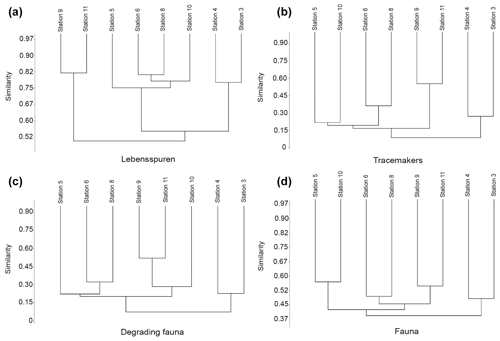
Figure 5Hierarchical cluster diagram (constructed with Bray–Curtis similarity matrix) of the abundances of lebensspuren (a), tracemakers (b), degrading fauna (c), and fauna (d) at each station.
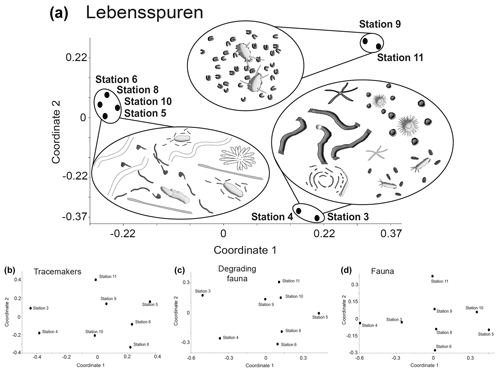
Figure 6Multivariate similarity represented with non-metric multidimensional scaling (n-MDS) plots of lebensspuren (a), tracemakers (b), degrading fauna (c), and fauna (d) at each station. Note that the only plot where stations are together is for lebensspuren abundance.
The hierarchical cluster diagram for tracemakers, degrading fauna, and fauna showed less similarity between stations than was the case for lebensspuren, especially for tracemakers and degrading fauna (values ranging from 20 % to 55 % similarity in the cluster analysis) (Fig. 5b–d). However, the trench stations (stations 3 and 4) and the southern stations (stations 9 and 11) seemed to have similar compositions. The low inter-station similarity of tracemakers, degrading fauna, and fauna assemblages was also reflected in the n-MDS plots where the spacing between stations was considerably higher than in the lebensspuren plot (Fig. 6b–d).
The results from the KKT area reveal that the relationship between lebensspuren, tracemakers, and fauna is more complicated than initially hypothesized (Kitchell et al., 1978; Young et al., 1985) and may follow the complex puzzle exposed in recent research (Przeslawski et al., 2012). While a general null diversity correlation has been observed between lebensspuren, tracemakers, and fauna, density correlations seem to be morphospecific (e.g. depending on the lebensspuren-associated behaviour). But to what extent do the results obtained contradict or corroborate previous results and what are the limitations when addressing the diversity and density of lebensspuren?
4.1 Fauna, tracemakers, and lebensspuren diversity: a complex relationship
Previous comparisons between lebensspuren and faunal diversity have given rise to different contrasting hypotheses. Though pioneering research showed positive correlations (e.g. Kitchell et al., 1978; Young et al., 1985), later studies showed no correlation at all (e.g. Tilot, 1995; Turnewitsch et al., 2000; Przeslawski et al., 2012). These studies share a common approach in which the diversity comparisons were conducted from a broad perspective, especially for tracemaker organisms. Comparisons were conducted using megafaunal taxa (Young et al., 1985), epifaunal taxa (Przeslawski et al., 2012), or certain taxonomic groups of organisms (e.g. fish, holothurians, crinoids; Kitchell et al., 1978). Only Bell et al. (2013) approached the comparison between lebensspuren and fauna in greater detail by considering groups of lebensspuren-forming epifauna, and using indices to quantify lebensspuren diversity (e.g. Simpson, Shannon-Wiener). Their analyses revealed that “lebensspuren diversity was generally high and not similar to that of lebensspuren-forming faunal diversity” (Bell et al., 2013). However, the links between specific tracemakers and their lebensspuren and the subsequent tracemaker diversity indexes are missing in the study by Bell et al. (2013). In the present study we attempt to close this knowledge gap by conducting a comprehensive comparison of lebensspuren diversity. We examine not only the faunal diversity but also encompass tracemaker and degrading fauna (i.e. fauna that may alter the lebensspuren assemblage by erosion/degradation). Our results show that lebensspuren diversity (Simpson, Shannon–Wiener, and evenness) is not related to fauna, tracemaker, or degrading fauna diversity. These findings seem to corroborate results of a non-existent correlation (Przeslawski et al., 2012; Bell et al., 2013). However, can this lack of correlation be expected in all deep-sea settings? This question cannot be answered without considering the limitations of quantifying deep-sea lebensspuren diversity, which is riddled with problems. These problems include image resolution, camera systems, unknown lebensspuren, observation scale, and trace degradation, but the most important is linked to their genesis. In other words, the same lebensspuren morphotype (or indistinguishable lebensspuren) can be produced by different tracemakers and one tracemaker can produce different lebensspuren (see Table 2). For example, in this study, several different holothurians (e.g. cf. Pseudostichopus, Psychropotes, Synallactidae, and Benthodytes) could have produced the smooth faecal casts. By contrast, Psychropotes can be linked to the production of coiled-curly and smooth faecal casts as well as flat trails (Fig. 2G). Thus, when comparing their diversity, it should be taken into account that lebensspuren morphotypes may not be related to one specific taxon, and vice versa. However, while general lebensspuren diversity in the present study did not correlate with tracemaker diversity, this does not mean that the same applies to all deep-sea settings. It is possible that a more precise characterization of the tracemaker–lebensspuren relationship, or of whether tracemakers produce specific lebensspuren morphotypes, may reveal a different correlation in other settings.
We observed different correlations between tracemakers and lebensspuren when comparing diversity among specific stations. For example, comparison of the southern stations (stations 9 and 11) using Simpson and Shannon–Wiener indexes showed a correlation between tracemakers and lebensspuren diversities (Fig. 3). This can be attributed to the traces dominating the assemblage for which we have successfully identified tracemakers (e.g. rounded faecal casts of Scotoplanes). On the contrary, comparison of the trench stations 3 and 4 using Simpson and Shannon–Wiener indexes revealed a negative correlation between tracemaker and lebensspuren diversities (Fig. 3). This can be attributed to the relatively large gap in our data regarding the origin of most traces of the lebensspuren assemblage. Stations 3 and 4 have a high abundance of dwelling lebensspuren (see Table 3), single burrows, mounds, cluster burrows for which tracemakers are unknown, and dominant tracemakers (Elpidia) whose traces cannot be correctly quantified due to image resolution limitations (small rounded faecal casts). Also, the existence of unknown lebensspuren and tracemakers contributes to the correlation variability.
The enhancement of image resolution and the increase in deep-seafloor area covered by still image surveys may enable the improvement of lebensspuren classification and their tracemaker identification. There is ample room for improvement, especially with regard to locomotion and feeding lebensspuren. High-definition still images will allow researchers to characterize small morphological features of trails (e.g. podia marks from asteroids, echinoid spine impressions), allowing for a much more detailed classification than could be achieved in this study. The use of artificial intelligence, a seemingly promising tool in the assistance of benthic fauna recognition in imagery analyses, appears to have restricted applicability in the characterization of lebensspuren. This is because lebensspuren are constructed with sediment that has the same texture as the seafloor (i.e. background colour). In the case of dwelling lebensspuren diversity, making a comparison is significantly more complicated. Trace morphology is largely hidden below the seafloor surface, reducing the ability to differentiate between various burrow morphologies. Additionally, tracemakers are largely unidentified due to their predominantly endobenthic lifestyle (e.g. Brandt et al., 2023). Finally, it is worth noting that burrows and other dwelling lebensspuren may have multiple potential origins.
Our results show that in specific stations where the assemblage is dominated by traces with identifiable tracemakers, lebensspuren analysis appears to be a promising tool for predicting tracemaker diversity. Although these results are promising, it is evident that much more research is needed, especially with high-definition surveys (e.g. videos, images) to close existing knowledge gaps in the relationship between lebensspuren and tracemakers. Moreover, we emphasize that when using lebensspuren as a proxy for biodiversity, the diversity correlation should be made between lebensspuren and tracemakers, rather than with overall benthic fauna, as no correlation has been observed in the case of comparisons with the latter.
4.2 Tracemaker and lebensspuren density: morphospecific relationship
Similar to previous research, the density comparisons between lebensspuren, degrading fauna, and fauna revealed no correlation (Przeslawski et al., 2012). However, the density comparisons between lebensspuren and tracemakers revealed a positive and negative correlation (Fig. 4). The density of locomotion–feeding lebensspuren is inversely correlated with their tracemaker density, while resting lebensspuren are positively correlated with their tracemaker density. These group-specific correlations contradict previous research that showed generally positive (e.g. Bell et al., 2013) or generally negative density correlations (e.g. Kitchell et al., 1978; Young et al., 1985). The difference with these previous studies may be because their density comparisons considered the total fauna instead of separate functional groups (see Fig. 10 in Bell et al., 2013), not considering their specific impact on the sediment.
Trace residence time is the period during which a trace is recognizable on the seafloor before it is destroyed (Wheatcroft et al., 1989). Lebensspuren density values reflect the balance between lebensspuren formation and lebensspuren destruction or degradation either by biotic (e.g. microbial degradation, degrading fauna, epifaunal rate of movement) or abiotic factors (e.g. hydrodynamics, burial) (Wheatcroft et al., 1989). However, not all lebensspuren have the same residence time. Thus, traces not actively maintained by animals are short lived, lasting only days to weeks (e.g. faecal casts can be degraded within 1–2 weeks; Smith et al., 2005). By contrast, locomotion–feeding and resting lebensspuren have longer residence times as they are impressions on the seafloor (see Fig. 8 in Wheatcroft et al., 1989, or Fig. 5 in Miguez-Salas et al., 2020). Very little is known about the residence time of dwelling lebensspuren. Some tracemakers inhabit them throughout their life, while others change residence multiple times and their burrows get passively filled (Gage and Tyler, 1991). Thus, a wide range of residence times may be expected. However, irrespective of scenario, the sedimentation rate is usually low in the deep sea and dwelling lebensspuren should have higher residence time than wasting lebensspuren, and similar or higher residence time than locomotion–feeding and resting lebensspuren.
The density of locomotion–feeding lebensspuren (e.g. M-ridged trails) was inversely correlated with tracemaker density. This could be attributed to two reasons: (1) a high residence time of these lebensspuren even if the respective tracemakers are no longer in the study area; and (2) these lebensspuren represent a foraging behaviour in which the tracemakers tend to continuously search the seabed for food, often over a wide area (i.e. high rate of movement). Thus, a large quantity of lebensspuren may be produced by a single individual tracemaker in continuous movement. The density of resting lebensspuren (e.g. circular impressions, asteroid impressions) was directly correlated with tracemaker density. This is not surprising because while these lebensspuren have a high residence time, their tracemakers (e.g. asteroids, actiniarians) have low rates of movement (Durden et al., 2015, 2019). In such cases, a high density of resting lebensspuren should always be linked to a high density of their tracemakers.
The density correlation between wasting lebensspuren and their tracemakers was slightly positive but not significant (Fig. 4). This may be attributable to the fact that we were unable to quantify the exact number of faecal casts for some morphotypes. For example, in station 4, the lebensspuren of the dominant tracemakers (Elpidia; more than 150 specimens) were incorrectly quantified due to image resolution limitations (small rounded faecal casts). Thus, a positive density correlation between wasting lebensspuren and their tracemakers should be expected. However, this assumption may be influenced by the behaviour of their tracemakers , as their feeding activity can be expected to depend on the grain size, availability, and quality of the nutrients among other environmental factors (e.g. Jumars and Wheatcroft, 1989; Ginger et al., 2001).
The variability observed in the lebensspuren density correlations shows a complex scenario even without considering biotic and abiotic factors that cannot be characterized through still images. For instance, it has been demonstrated that meiofauna and microfauna have the ability to smoothen and eventually fully erase surficial biogenic structures through small-scale grain-by-grain jostling of particles (e.g. Cullen, 1973). These “small” biotic processes are impossible to quantify through images; however, they most likely influenced the lebensspuren density that we quantified for this study. Moreover, while previous studies assumed that abiotic lebensspuren degradation rates are constant over the lebensspuren residence period (Bell et al., 2013), recent studies show that this may not be always true since hourly spontaneous events (e.g. benthic storms) may erase the full lebensspuren assemblage (Miguez-Salas et al., 2020). The effects of abiotic factors (e.g. bottom currents, substrate consistency) on the density of the assemblages studied as well as those of some biotic factors (e.g. microbial degradation which cannot be characterized in a still image) are out of the scope of this research but should be considered in future studies.
The neoichnological analysis of the KKT area reveals a general null diversity correlation between lebensspuren, tracemakers, and fauna while density correlations vary depending on the lebensspuren morphotypes. Further conclusions of this study are:
-
The ability of various tracemakers to produce the same lebensspuren morphotypes, and for a single tracemaker to produce various lebensspuren morphotypes, will impact the establishment of a positive or negative diversity correlation.
-
The existence of unknown tracemakers will contribute to the diversity correlation variability. However, lebensspuren diversity may be a good proxy for tracemaker biodiversity when the lebensspuren–tracemaker relationship can be reliably characterized.
-
Lebensspuren density can be positively or negatively correlated with tracemaker densities depending on the specific lebensspuren residence time and tracemaker behaviour (e.g. locomotion, resting).
-
Lebensspuren–density correlations may be controlled on a wider spatial scale by abiotic (e.g. hydrodynamics, grain size, organic matter, substrate consistency) and biotic factors (e.g. microbial degradation).
Above all, we suggest that lebensspuren density and diversity correlations should be studied with tracemakers rather than with general benthic fauna.
All data generated or analysed during this study are included in this article. The raw data used for this study are available at: https://doi.org/10.5281/zenodo.10057636 (Miguez-Salas and Riehl, 2023b).
The supplement related to this article is available online at: https://doi.org/10.5194/bg-21-641-2024-supplement.
OMS and TR performed the data acquisition and treatment. OMS, TR, and AB, wrote and designed the main manuscript text. OMS and HK prepared all figures and tables. All authors reviewed and edited the manuscript at multiple stages and approved it for submission.
The contact author has declared that none of the authors has any competing interests.
Publisher's note: Copernicus Publications remains neutral with regard to jurisdictional claims made in the text, published maps, institutional affiliations, or any other geographical representation in this paper. While Copernicus Publications makes every effort to include appropriate place names, the final responsibility lies with the authors.
Special thanks to the German Federal Ministry of Education and Research (BMBF) for funding this project (PTJ, Grant 03G0223A to Angelika Brandt). We thank the crew of R/V Sonne and Marina Malyutina, the coordinator of the Russian expedition. The research of Olmo Miguez-Salas was funded by a Humboldt Postdoctoral Fellowship from the Alexander von Humboldt-Stiftung. This is contribution #18 of the Senckenberg Ocean Species Alliance (SOSA). We extend our gratitude to Autun Purser and Rachel Przeslawski for their constructive comments, which significantly contributed to enhancing the clarity of the manuscript. Special thanks to Anchita Casaubon for the English editing.
This research has been supported by the Alexander von Humboldt-Stiftung (grant no. Olmo Miguez-Salas Postdoctoral Fellow).
This paper was edited by Andrew Thurber and reviewed by Rachel Przeslawski and Autun Purser.
Althaus, F., Hill, N., Ferrari, R., Edwards, L., Przeslawski, R., Schönberg, C. H., Stuart-Smith, R., Barrett, N., Edgar, G., Colquhoun, J., and Tran, M.: A standardised vocabulary for identifying benthic biota and substrata from underwater imagery: the CATAMI classification scheme, PloS one, 10, e0141039, https://doi.org/10.1371/journal.pone.0141039, 2015.
Bell, J. B., Jones, D. O., and Alt, C. H.: Lebensspuren of the Bathyal Mid-Atlantic Ridge, Deep-Sea Res. Pt. II, 98, 341–351, https://doi.org/10.1016/j.dsr2.2012.09.004, 2013.
Belyaev, G. M.: Investigation of ultra abyssal fauna. Research Vessel “Vitjaz” and her expeditions 1949–1979, Nauka, M., 258–267, 1983 (in Russian).
Brandt, A. and Malyutina, M. V.: The German-Russian deep-sea expedition KuramBio (Kurile Kamchatka Biodiversity Study): to the Kurile Kamchatka Trench and abyssal plain on board of the R/V Sonne, 223rd Expedition, July 21th–September 7th 2012. R/V Sonne Cruise Reports, University of Hamburg, Biozentrum Grindel & Zoologisches Museum Hamburg, Hamburg, https://doi.org/10.13140/RG.2.1.4832.9362, 2012.
Brandt, A. and Malyutina, M. V.: The German–Russian deep-sea expedition KuramBio (Kurile Kamchatka biodiversity studies) on board of the RV Sonne in 2012 following the footsteps of the legendary expeditions with RV Vityaz, Deep-Sea Res., 111, 1–9, https://doi.org/10.1016/j.dsr2.2014.11.001, 2015.
Brandt, A., Brix, S., Riehl, T., and Malyutina, M.: Biodiversity and biogeography of the abyssal and hadal Kuril-Kamchatka trench and adjacent NW Pacific deep-sea regions, Prog. Oceanogr., 181, 102232, https://doi.org/10.1016/j.pocean.2019.102232, 2020.
Brandt, A., Chen, C., Tandberg, A. H. S., Miguez-Salas O., and Sigwart, J.: Complex sublinear burrows in the deep sea may be constructed by amphipods, Ecol. Evol., 13, e9867, https://doi.org/10.1002/ece3.9867, 2023.
Buatois, L. A. and Mángano, M. G.: Ichnology: Organism-substrate interactions in space and time, Cambridge University Press, ISBN 9780521855556, 2011.
Cullen, D. J.: Bioturbation of superficial marine sediments by interstitial meiobenthos, Nature, 242, 323–324, 1973.
Dundas, K. and Przeslawski, R.: Deep-sea Lebensspuren: biological traces on the sea floor of the Eastern and Western Australian margin, Geosci. Aust. Canberra, 76, eCat Id: 69358, 2009.
Durden, J. M., Bett, B. J., and Ruhl, H. A.: The hemisessile lifestyle and feeding strategies of Iosactis vagabunda (Actiniaria, Iosactiidae), a dominant megafaunal species of the Porcupine Abyssal Plain, Deep-Sea Res. Pt. I, 102, 72–77, https://doi.org/10.1016/j.dsr.2015.04.010, 2015.
Durden, J. M., Bett, B. J., Huffard, C. L., Ruhl, H. A., and Smith, K. L.: Abyssal deposit-feeding rates consistent with the metabolic theory of ecology, Ecology, e02564, https://doi.org/10.1002/ecy.2564, 2019.
Ewing, M. and Davis, R. A.: Lebensspuren photographed on the ocean floor, in: Deep-Sea Photography: The John Hopkins Oceanographic Studies, edited by: Hersey, J. B., John Hopkins Press, Baltimore, MD, ISSN 0271-2229, 1967.
Gage, J. D. and Tyler, P. A.: Deep-Sea Biology: A Natural History of Organisms at the Deep-Sea Floor, Cambridge University Press, Cambridge, UK, ISBN 9781139163637, 1991.
Gerino, M., Stora, G., Poydenot, F., and Bourcier, M.: Benthic fauna and bioturbation on the Mediterranean continental slope: Toulon Canyon, Cont. Shelf Res., 15, 1483–1496, https://doi.org/10.1016/0278-4343(94)00082-X, 1995.
Ginger, M. L., Billett, D. S., Mackenzie, K. L., Kiriakoulakis, K., Neto, R. R., Boardman, D. K., Santos, V. L., Horsfall, I. M., and Wolff, G. A.: Organic matter assimilation and selective feeding by holothurians in the deep sea: some observations and comments, Prog. Oceanogr., 50, 407–421, https://doi.org/10.1016/S0079-6611(01)00063-5, 2001.
Halpern, B. S. and Warner, R. R.: Marine reserves have rapid and lasting effects, Ecol. Lett., 5, 361–366, https://doi.org/10.1046/j.1461-0248.2002.00326.x, 2002.
Hammer, Ø., Harper, D. A., and Ryan, P. D.: PAST: Paleontological statistics software package for education and data analysis, Palaeontol. Electron., 4, 9, 2001.
Heezen, B. C. and Hollister C. D.: The face of the deep, Oxford University Press, London, 659 pp., ISBN 0195012771, 1971.
Horton, T., Marsh, L., Bett, B. J., Gates, A. R., Jones, D. O., Benoist, N., Pfeifer, S., Simon-Lledó, E., Durden, J. M., Vandepitte, L., and Appeltans, W.: Recommendations for the standardisation of open taxonomic nomenclature for image-based identifications, Front. Mar. Sci., 8, 620702, https://doi.org/10.3389/fmars.2021.620702, 2021.
Jumars, P. A. and Wheatcroft, R. A.: Responses of benthos to changing food quality and quantity, with a focus on deposit feeding and bioturbation, Life Sci. R., 235–253, 1989.
Kitchell, J. A., Kitchell, J. F., Johnson, G. L., and Hunkins, K. L.: Abyssal lebensspuren and megafauna: comparison of productivity, diversity and density in the Arctic and Antarctic, Paleobiology, 4, 171–180, https://doi.org/10.1017/S0094837300005844, 1978.
La Croix, A. D., Ayranci, K., and Dashtgard, S. E.: Neoichnology of siliciclastic shallow-marine environments: Invertebrates, traces, and environmental conditions, Earth-Sci. Rev., 233, 104170, https://doi.org/10.1016/j.earscirev.2022.104170, 2022.
Langenkämper, D., Zurowietz, M., Schoening, T., and Nattkemper, T. W.: Biigle 2.0-browsing and annotating large marine image collections, Front. Mar. Sci., 4, 83, https://doi.org/10.3389/fmars.2017.00083, 2017.
Miguez Salas, O. and Riehl, T.: Still images from the KuramBio expedition 2012 (Stations 3-6, 8-11) obtained with the Ocean Floor Observation System, Zenodo, https://doi.org/10.5281/zenodo.10057539, 2023a.
Miguez Salas, O. and Riehl, T.: Lebensspuren and benthic fauna diversity and density data obtained from KuramBio 2012 expedition still images (50 still images per 8 deep-sea stations), Zenodo [data set], https://doi.org/10.5281/zenodo.10057636, 2023b.
Miguez-Salas, O., Dorador, J., and Rodríguez-Tovar, F. J.: Introducing Fiji and ICY image processing techniques in ichnological research as a tool for sedimentary basin analysis, Mar. Geol., 413, 1–9, https://doi.org/10.1016/j.margeo.2019.03.013, 2019.
Miguez-Salas, O., Huffard, C. L., Smith Jr., K. L., McGill, P. R., and Rodríguez-Tovar, F. J.: Faunal assemblage changes, bioturbation and benthic storms at an abyssal station in the northeastern Pacific, Deep-Sea Res. Pt. I, 160, 103277, https://doi.org/10.1016/j.dsr.2020.103277, 2020.
Miguez-Salas, O., Vardaro, M. F., Rodríguez-Tovar, F. J., Pérez-Claros, J. A., and Huffard, C. L.: Deep-sea echinoid trails and seafloor nutrient distribution: present and past implications, Front. Mar. Sci., 9, 903864, https://doi.org/10.3389/fmars.2022.903864, 2022.
Miguez-Salas, O., Rodríguez-Tovar, F. J., Ekdale, A. A., Kaiser, S., Brandt, A., and Gooday, A. J.: Northernmost (Subarctic) and deepest record of Paleodictyon: paleoecological and biological implications, Sci. Rep.-UK, 13, 7181, https://doi.org/10.1038/s41598-023-34050-w, 2023.
Ramirez-Llodra, E., Brandt, A., Danovaro, R., De Mol, B., Escobar, E., German, C. R., Levin, L. A., Martinez Arbizu, P., Menot, L., Buhl-Mortensen, P., Narayanaswamy, B. E., Smith, C. R., Tittensor, D. P., Tyler, P. A., Vanreusel, A., and Vecchione, M.: Deep, diverse and definitely different: unique attributes of the world's largest ecosystem, Biogeosciences, 7, 2851–2899, https://doi.org/10.5194/bg-7-2851-2010, 2010.
Przeslawski, R., Dundas, K., Radke, L., and Anderson, T. J.: Deep-sea Lebensspuren of the Australian continental margins, Deep-Sea Res. Pt. I, 65, 26–35, https://doi.org/10.1016/j.dsr.2012.03.006, 2012.
Saeedi, H. and Brandt, A.: Biogeographic Atlas of the Deep NW Pacific Fauna, Advanced Books, 1, e51315, https://doi.org/10.3897/ab.e51315, 2020.
Schindelin, J., Arganda-Carreras, I., Frise, E., Kaynig, V., Longair, M., Pietzsch, T., Preibisch, S., Rueden, C., Saalfeld, S., Schmid, B., and Tinevez, J. Y.: Fiji: an open-source platform for biological-image analysis, Nat. Methods, 9, 676, https://doi.org/10.1038/nmeth.2019, 2012.
Seilacher, A.: Die geologische Bedeutung fossiler Lebensspuren, Zeitschrift der deutschen geologischen Gesellschaft, 105, 214–227, 1954.
Smith, K. L., Holland, N. D., and Ruhl, H. A.: Enteropneust production of spiral fecal trails on the deep-sea floor observed with time-lapse photography, Deep-Sea Res. Pt. II, 52, 1228–1240, https://doi.org/10.1016/j.dsr.2005.02.004, 2005.
Tilot, V.: An ecologically important type of biological activity in a deep-sea ferro-manganese nodule environment of the tropical north east Pacific, Mesogee, 54, 101–120, 1995.
Turnewitsch, R., Witte, U., and Graf, G.: Bioturbation in the abyssal Arabian Sea: influence of fauna and food supply, Deep-Sea Res. Pt. II, 47, 2877–2875, https://doi.org/10.1016/S0967-0645(00)00052-7, 2000.
Watling, L., Guinotte, J., Clark, M. R., and Smith, C. R.: A proposed biogeography of the deep ocean floor, Prog. Oceanogr., 111, 91–112, https://doi.org/10.1016/j.pocean.2012.11.003, 2013.
Wheatcroft, R. A., Smith, C. R., and Jumars, P. A.: Dynamics of surficial trace assemblages in the deep sea, Deep-Sea Res., 36, 71–91, https://doi.org/10.1016/0198-0149(89)90019-8, 1989.
Young, D. K., Jahn, W. H., Richardson, M. D., and Lohanick, A. W.: Photographs of deep-sea Lebensspuren – a comparison of sedimentary provinces in the Venezuela Basin. Caribbean Sea, Mar. Geol., 68, 269–301, https://doi.org/10.1016/0025-3227(85)90016-7, 1985.
Zenkevitch, L.: Biology of the Seas of the USSR, Interscience, New York, 955, https://doi.org/10.5962/bhl.title.6447, 1963.
Zenkevitch, L. A., Birstein, Y. A., and Belyaev, G. M.: Stadies of the Bottom Fauna of the Kurile-Kamchatka Trench, Trudy Inst. Okeanol., ANSSSR12, 345–381, 1955.