the Creative Commons Attribution 4.0 License.
the Creative Commons Attribution 4.0 License.
Revisiting chlorophyll extraction methods in biological soil crusts – methodology for determination of chlorophyll a and chlorophyll a + b as compared to previous methods
Jennifer Caesar
Alexandra Tamm
Nina Ruckteschler
Anna Lena Leifke
Chlorophyll concentrations of biological soil crust (biocrust) samples are commonly determined to quantify the relevance of photosynthetically active organisms within these surface soil communities. Whereas chlorophyll extraction methods for freshwater algae and leaf tissues of vascular plants are well established, there is still some uncertainty regarding the optimal extraction method for biocrusts, where organism composition is highly variable and samples comprise major amounts of soil. In this study we analyzed the efficiency of two different chlorophyll extraction solvents, the effect of grinding the soil samples prior to the extraction procedure, and the impact of shaking as an intermediate step during extraction. The analyses were conducted on four different types of biocrusts. Our results show that for all biocrust types chlorophyll contents obtained with ethanol were significantly lower than those obtained using dimethyl sulfoxide (DMSO) as a solvent. Grinding of biocrust samples prior to analysis caused a highly significant decrease in chlorophyll content for green algal lichen- and cyanolichen-dominated biocrusts, and a tendency towards lower values for moss- and algae-dominated biocrusts. Shaking of the samples after each extraction step had a significant positive effect on the chlorophyll content of green algal lichen- and cyanolichen-dominated biocrusts. Based on our results we confirm a DMSO-based chlorophyll extraction method without grinding pretreatment and suggest the addition of an intermediate shaking step for complete chlorophyll extraction (see Supplement S6 for detailed manual). Determination of a universal chlorophyll extraction method for biocrusts is essential for the inter-comparability of publications conducted across all continents.
- Article
(1804 KB) -
Supplement
(132 KB) - BibTeX
- EndNote
Chlorophyll (Chl) is a pigment commonly occurring in photosynthesizing organisms. It facilitates organisms to utilize sunlight as an energy source to build glucose and carbohydrates from CO2 and water. The composition of photosynthetic pigments varies between organisms: cyanobacteria possess only Chl a and the accessory antenna pigments, phycocyanin and phycoerythrin; green algae and vascular plants comprise Chl a and Chl b; brown algae contain Chl c instead of Chl b and Rhodophyta contain only Chl a whilst Chl b is replaced by Chl d (Mohr and Schopfer, 1995).
Biological soil crusts (biocrusts) are surface soil communities commonly occurring in arid and semiarid regions throughout the world, as well as in areas where the lack of water or other environmental conditions (e.g., disturbance) restrict the development of vascular plants (Garcia-Pichel et al., 2003; Zaady et al., 2016). They grow within the uppermost millimeters of the soil in close association with soil particles. It has been shown that biocrusts play significant functional roles in desert ecosystems (Eldridge and Greene, 1994; Evans and Belnap, 1999; Lan et al., 2011), as they stabilize the soil surface and reduce erosion by wind and water (Zhao et al., 2014; Belnap et al., 2014; Belnap and Büdel, 2016), they contribute to soil fertility through carbon and nitrogen fixation (Elbert et al., 2012; Sancho et al., 2016; Barger et al., 2016; Brankatschk et al., 2013), and they positively affect water retention and distribution in drylands (Rodriguez-Caballero et al., 2014; Chamizo et al., 2016). Biocrusts and their organisms have also been shown to release gaseous nitrogen compounds, as nitrous acid (Lenhart et al., 2015), nitric oxide, and nitrous oxide into the atmosphere (Weber et al., 2015; Meusel et al., 2018). Biocrusts are composed of photosynthesizing cyanobacteria, algae, lichens, and bryophytes plus decomposers, i.e., fungi, bacteria and archaea (Maier et al., 2016), and heterotrophic consumers, like protozoa, collembolans, and snails (Darby and Neher, 2016; Bamforth, 2008). Thus, they form one of the smallest ecosystems with photosynthetic carbon fixation being the main source of carbohydrates. The chlorophyll content of these communities is therefore a good indicator of the photosynthetic capacity and thus the capability of these systems to acquire energy, jointly used by the community but also exchanged with the surrounding environment. The capability to acquire energy under favorable environmental conditions is in turn a relevant proxy indicating successional stage, system stability, and its ability to recover from disturbance (Dojani et al., 2011; Gomez et al., 2012; Weber et al., 2016). As cyanobacteria and cyanolichens (photobiont: cyanobacteria) only comprise Chl a, high Chl a ∕ Chl b ratios may indicate their dominance. Unfortunately, the Chl a ∕ Chl b ratio in algae, chlorolichens (photobiont: green algae), and bryophytes (i.e., liverworts and mosses) is variable, thus not facilitating the proportional quantification of both groups; nevertheless, it allows tentative estimates on the relevance of eukaryotic Chl b-comprising partners (Thorne et al., 1977).
Suitable chlorophyll extraction procedures are essential to obtain reliable results, and standardized methods are needed to allow comparison between studies (Schagerl and Künzl, 2007). During the last decades, several different methods have been described. The solvents most commonly used for chlorophyll extraction have been ethanol, acetone, N, N-Dimethylformamide (DMF), and dimethyl sulfoxide (DMSO) (Mackinney, 1941; Shoaf and Lium, 1976; Moran and Porath, 1980; Barnes et al., 1992; Inskeep and Bloom, 1985). In a recent publication, Castle et al. (2011) compared the efficiency of four different solvents: acetone, ethanol, DMSO, and methanol for biological soil crusts of three different successional stages. They found that ethanol and DMSO extracted the greatest amount of Chl a using a double extraction technique. At a similar time, Lan et al. (2011) also compared the Chl a extraction efficiency using ethanol, acetone, DMF, and DMSO as solvents to analyze algal-, lichen-, and moss-dominated biocrusts. They concluded ethanol extractions expressed on an area basis to be most efficient and found DMSO extractions of lichens to be unreliable. Apart from the extraction solvent, preparatory steps and handling during extraction also varied between methods. Both Castle et al. (2011) and Lan et al. (2011) ground the samples with a mortar and pestle to facilitate the following extraction steps, and Castle et al. (2011) also applied a two-step extraction and placed the samples on a shaker after each extraction cycle.
In this study we investigate these methodological techniques, which have been recently suggested for the extraction of Chl a from biocrusts. Our overall analytical technique is based on the photometric method established by Ronen and Galun (Ronen and Galun, 1984; Hiscox and Israelstam, 1978) and on methodological adaptions made in the lab of O. L. Lange, personal communication, 2000. We compare the efficiency of two extraction methods, using the two solvents DMSO and ethanol, which have been rated as most effective during the most recent studies by Lan et al. (2011) and Castle et al. (2011), for green algae-dominated, green algal lichen-dominated, moss-dominated, and cyanolichen-dominated biocrusts.
In this research we address the following three questions:
-
Which is the most potent chlorophyll extraction method for biocrusts? The ethanol method by Castle et al. (2011) or the DMSO method by Ronen and Galun (1984)?
-
Is a disruption of the cells (grinding) necessary prior to the extraction procedure and does it influence the chlorophyll yield?
-
Does shaking (20 min) after each extraction step influence the chlorophyll yield?
The overall goal of this study is to determine an extraction method best suited for chlorophyll determination of the analyzed samples, which we consider as an important step towards a universal chlorophyll determination method for different types of biocrusts.
2.1 Sampling site
The biocrust samples for this study were collected in: the Mehlinger Heide nature reserve, located about 15 km north of Kaiserslautern, in Rhineland-Palatinate; and the Ruine Homburg nature reserve at Gössenheim, in northern Bavaria.
The Mehlinger Heide is about 410 ha in size, being one of the biggest heathlands in southern Germany. Until 1912, the area was completely covered by forest. In the First and Second World War, the region was partly deforested to build a military training ground (http://mehlinger-heide.de/). After the Second World War, the area was used for military training by French and American troops until 1992 and 1994, respectively. The prolonged continuous disturbance by military use caused the formation of a distinct flora and fauna typical of nutrient-depleted sandy soils, which are rarely found in central Europe. The vegetation is characterized by dwarf shrubs (heather), lichens (fruticose, foliose and crustose) and bryophytes (mosses and liverworts). The Mehlinger Heide has been under conservation since 2001.
The Ruine Homburg nature reserve is an open anthropogenic landscape with bare rock and gravel spots covered by a thin vegetation layer dominated by cryptogams including lichens, bryophytes, and cyanobacteria. Its bedrock is Triassic shell limestone and its flora is composed of a relic flora from after the last ice age with sub-Mediterranean–continental and sub-Mediterranean–sub-Atlantic elements (Lösch, 1980). The landscape has remained open owing to the presence of a castle which was built nearby in 1080.
2.2 Sample collection
Four different biocrust types were collected:
-
Green algae-dominated biocrust with Klebsormidium sp. as the dominating organism.
-
Green algal lichen-dominated biocrust with Cladonia sp. as the dominating genus.
-
Moss-dominated biocrust with Hypnum sp. as the dominating organism.
-
Cyanolichen-dominated biocrust with Peltigera rufescens as the dominating lichen.
All four biocrusts types were collected in 2014 (January and May) and 2016 (June and September) in the Mehlinger Heide. Some samples of the cyanolichen-dominated biocrusts were collected in May 2014 in Aschfeld/Gössenheim. Within each experiment, only samples of the same sampling batch were used. During sampling, special care was taken to avoid variability between replicate samples within each type. For sampling, a metal ring of 14 mm diameter (surface size: 153.9 mm2) and 3.5 cm in height was used. The metal ring was pressed 3 cm deep into the soil, a trowel was pushed underneath, both were pulled out again, and the biocrust sample within the ring was transferred into a plastic zip lock bag. For each biocrust type five replicates were collected (total number of samples: n=20). The samples were transported back to the institute, where they were air dried and stored at room temperature at low light intensities for less than four weeks until the chlorophyll extraction experiments were conducted.
2.3 Chlorophyll extraction
2.3.1 Pretreatment of the biocrust samples
Prior to chlorophyll extraction, all biocrust samples were dried in a drying oven at 60 ∘C for at least 24 h until constant weight was reached and the dry weight was determined. On the day before the chlorophyll extraction the samples were slightly sprinkled with distilled water to activate the biocrust organisms, which is known to facilitate the subsequent chlorophyll extraction. The photosynthetic apparatus undergoes disassembly during desiccation, so rehydration is required to repair the photosynthetic apparatus (Harel et al., 2004). During the entire chlorophyll extraction procedure, the samples were kept in the dark or at minimum light to prevent the degradation of chlorophyll (Molnár et al., 2013; Hosikian et al., 2010).
2.3.2 Chlorophyll extraction with dimethyl sulfoxide (DMSO)
Chlorophyll extraction with DMSO as solvent was conducted based on the method described by Ronen and Galun (Hiscox and Israelstam, 1978; Ronen and Galun, 1984) and on methodological adaptions made in the lab of O. L. Lange, personal communication, 2000.
The soil crust samples of the four types (n=5) were placed in 15 mL screw-cap vials without prior grinding. A spatula tip of MgCO3 or CaCO3 was added to avoid acidification and the associated chlorophyll degradation (Weber et al., 2013; Rapsch and Ascaso, 1985). Following this, 6 mL of DMSO (ROTIDRY® ≥ 99.5 %, ≤ 200 ppm H2O, Carl Roth GmbH + Co. KG, Karlsruhe, Germany) were added to the samples. A vial with only DMSO and MgCO3 ∕ CaCO3 (no sample added) was used as a blank. All vials were placed on a water bath at 65 ∘C for 90 min. The caps of the vials were half tightened to allow extension of the liquid during heating but avoid evaporation loss. After the first extraction cycle the supernatant was poured into a separate vial, and another 6 mL of DMSO were added to the samples for a second extraction cycle. After a second extraction in the water bath for 90 min, the supernatants of both extractions were pooled and centrifuged (Megafuge 16R, Thermo Fisher Scientific, Dreieich, Germany) for 10 min at 3000 × g and 15 ∘C before photometric determination.
The absorption was measured with a spectrophotometer (Lambda 25 UV/VIS, PerkinElmer, Rodgau, Germany) at 648, 665, and 700 nm. If absorption values at 665 nm were above 0.8, the sample was diluted 1:1 with DMSO and the equation was adjusted accordingly, changing the dilution factor from 1 to 2. The Chl a+b concentrations were calculated according to O. L. Lange, personal communication, 2000.
Total Chl a+b amount in sample:
Chl a+b amount based on surface area:
Chl a+b amount based on dry weight:
where
The Chl a concentration of biocrusts dominated by cyanobacterial lichens was calculated according to Arnon et al. (1949).
Total Chla amount in sample:
Chl a amount based on surface area:
Chl a amount based on dry weight:
2.3.3 Reproducibility of chlorophyll extraction with dimethyl sulfoxide (DMSO)
In order to analyze the reproducibility of chlorophyll extraction with DMSO, ∼ 90 g of green algae-dominated biocrust were oven-dried (see Sect. 2.3.1), homogenized with a mortar and pestle and evenly distributed into eight screw-cap vials. The subsequent chlorophyll extraction followed the described DMSO extraction procedure (see Sect. 2.3.2). The analyzed chlorophyll content refers to the initial sample weight.
2.3.4 Chlorophyll extraction with ethanol
The Chl a double extraction with ethanol was carried out according to the protocol of Castle et al. (2011). The soil crust samples (four types, n=5) were ground with mortar and pestle until they were homogenous and placed in screw-cap vials, a spatula tip of MgCO3 and 6 mL of ethanol (≥ 99.8 %, Sigma-Aldrich, Steinheim, Germany) were added and the caps were half-tightened. A vial with ethanol and a spatula tip of MgCO3 was used as blank. All samples were heated in a water bath at 80 ∘C until they started to boil. Once they had started to boil they were kept in the water bath for 5 min. After boiling, the vials were cooled down for 10 min and then placed on a horizontal shaker for 20 min. Subsequently, the samples were centrifuged at 3000× g and 15 ∘C for 10 min. The supernatant was poured into separate vials and another 6 mL of ethanol were added to the samples for a second extraction cycle conducted in the same way as the first.
After the second cycle, the supernatants of both extractions were combined and the absorbance was measured spectrophotometrically at 665, 649, and 750 nm. The absorption at 750 nm was measured in addition to eliminate a potential effect of the intrinsic color of the samples. The Chl a+b content was determined according to the formula of Ritchie (2006).
Total Chl amount in sample:
The total Chl a+b content is the sum of the results obtained from formula (7) and (8).
Analogous to the DMSO method, the total chlorophyll content can be calculated per area by dividing the total amount of Chl a+b by the area (AR) (see also Sect. 2.3.2).
Chl amount based on surface area:
The Chl a concentration of biocrusts dominated by cyanobacterial lichens was calculated according to the formula by Ritchie (2006).
Total Chl a amount in sample:
Chl a amount based on surface area:
2.4 Effect of prior grinding and additional shaking on chlorophyll extraction efficiency
Additional experiments were performed to evaluate the effect of two methodological steps, i.e., grinding prior to extraction and shaking after each extraction cycle of the samples, as described in the protocol by Castle et al. (2011). These experiments were only carried out with the DMSO method described above. To analyze the effect of grinding, the dry samples were ground to homogeneity with a mortar and pestle prior to the extraction procedure. Based on a reviewer suggestion, a second set of samples was ground in a wet state with some of the solvent (DMSO) added prior to grinding. In a separate approach, the effect of shaking the samples after extraction was analyzed. To achieve this, the samples were placed on a horizontal shaker for 20 min after each extraction cycle. Both treatments were applied to the four biocrust types (n=5, except in the shaker experiment: green algal- and green algal lichen-dominated biocrust: n=4).
2.5 Statistical evaluation
All data were analyzed with the statistical and analytical software OriginPro (Version 8.6; OriginLab Corporation, Northampton, MA, USA). Before statistical analyses all data were tested for normality and variance homogeneity. To determine statistical differences between normally distributed samples, where homogeneity of variance was given, unpaired t tests were performed. For samples, where normal distribution could not be reached, a Mann–Whitney U test was performed. Normally distributed samples without homogeneity of variance were analyzed using the Welch test.
For some samples the relative standard deviation (RSD) was calculated to reveal the extent of variability in relation to the average value of the population, using the following formula:
where is σ= standard deviation and μ = average value.
The RSD indicates the precision of the data and shows if the data is tightly clustered around the mean.
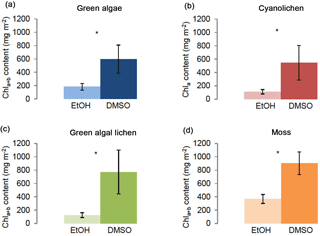
Figure 1Chlorophyll a+b/chlorophyll a content (mg m−2) of green algae- (a), cyanolichen- (b), green algal lichen- (c), and moss-dominated biocrusts (d) using the DMSO and ethanol extraction method. To prove statistical differences between both extraction methods, the Mann–Whitney U test was performed for moss-dominated biocrusts (data not normally distributed), for the other three biocrust types a Welch test was performed (data without homogeneity of variance) (* p≤0.05; p≤0.01; p≤0.001).
3.1 Comparison of chlorophyll extraction methods: DMSO versus ethanol
The two different chlorophyll extraction methods for DMSO and ethanol showed significant differences in chlorophyll yield for all four biocrust types (Fig. 1). Using the DMSO method, significantly higher chlorophyll contents were obtained compared to the ethanol method. Whereas Chl a+b ∕ Chl a contents determined with the DMSO extraction method ranged between mean values of ∼ 550 and 900 mg m−2 (i.e., 598 mg m−2 for green algae-dominated, 547 mg m−2 for cyanolichen-dominated, 772 mg m−2 for green algal lichen-dominated, and 903 mg m−2 for moss-dominated biocrusts), values obtained by the ethanol extraction method were between ∼ 110 and 370 mg m−2 (i.e., 182 mg m−2 for green algae-dominated, 111 mg m−2 for cyanolichen-dominated, 125 mg m−2 for green algal lichen-dominated, and 367 mg mg−2 for moss-dominated biocrusts; Supplement Table S1). Thus, the mean values obtained by the ethanol extraction procedure were ∼ 70, 80, 84, and 59 % lower for green algae-, cyanolichen-, green algal lichen-, and moss-dominated biocrusts than those obtained by the DMSO method. However, for all biocrust types, the results showed higher standard deviations for the DMSO as compared to the ethanol extraction method, and all types had higher relative standard deviations (Table S2).
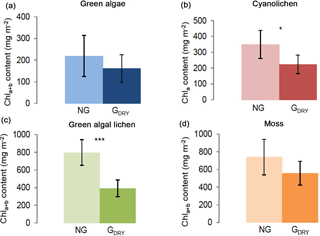
Figure 2Chlorophyll a+b/chlorophyll a content (mg m−2) depending on preparatory grinding in a dry state (GDRY) as compared to control extractions without grinding (NG). Investigation of green algae- (a), cyanolichen- (b), green algal lichen- (c), and moss-dominated biocrusts (d) using the DMSO extraction method. To prove statistical differences, an unpaired t test was performed for all four biocrust types (* p≤0.05; p≤0.01; p≤0.001).
To evaluate reproducibility of the chlorophyll extraction procedure by means of the DMSO method, eight replicate samples of homogeneous biocrust material were analyzed. Here, a RSD of 12.1 % was obtained, certifying a good reproducibility of the DMSO extraction method (Table S3).
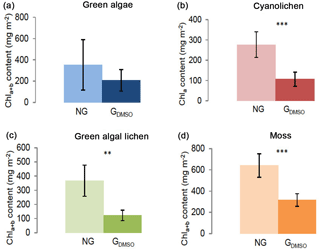
Figure 3Chlorophyll a+b/chlorophyll a content (mg m−2) depending on preparatory grinding in a wet state with DMSO added (GDMSO) as compared to control extractions without grinding (NG). Investigation of green algae- (a), cyanolichen- (b), green algal lichen- (c), and moss-dominated biocrusts (d) using the DMSO extraction method. To prove statistical differences, an unpaired t test was performed for all four biocrust types (* p≤0.05; p≤0.01; p≤0.001).
3.2 Pretreatment of the samples: grinding versus non-grinding
As the ethanol extraction method contains an additional grinding step during sample pretreatment, the impact of this step was tested for the DMSO extraction method, which had gained higher chlorophyll extraction yields (Fig. 1). The results reveal that preparatory grinding caused a decrease in Chl a+b ∕ Chl a contents, resulting in significantly lower values for cyanolichen- and green algal lichen- and a similar tendency in green algae- and moss-dominated biocrusts (Fig. 2). In fact, this preparatory step caused mean Chl a+b ∕ Chl a yields to be ∼ 36 and 51 % lower in cyanolichen-, and green algal lichen-dominated biocrusts (Table S4).
In an additional experiment the samples were ground in a wet state with solvent (DMSO) added prior to grinding (Fig. 3). Also under these conditions grinding had a negative effect and caused Chl a+b ∕ Chl a contents of ground samples to be significantly lower for cyanolichen-, green algal lichen-, and moss-dominated biocrusts. Solely for green algae-dominated biocrusts no significant reduction was observed.
3.3 Intermediate step during chlorophyll extraction: shaking versus non-shaking
The effect of shaking after each extraction cycle was evaluated for all four crust types. As shown in Fig. 4, shaking caused increased chlorophyll yields in green algal lichen- and cyanolichen-dominated biocrusts and a trend in the same direction was observed for green algae-dominated crusts. Chl a+b ∕ Chl a values were ∼ 73 and 42 % higher in cyanolichen- and green algal lichen-dominated biocrusts, respectively (Table S5).
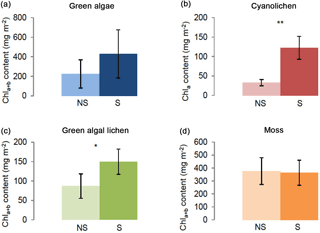
Figure 4Chlorophyll a+b/chlorophyll a content (mg m−2) depending on intermediate shaking (S) after each extraction cycle as compared to control extractions without shaking (NS). Investigation of green algae- (a), cyanolichen- (b), green algal lichen- (c), and moss-dominated biocrusts (d) using the DMSO extraction method. To prove statistical differences between shaking (S) and non-shaking (NS) a Welch test was performed for cyanolichen-dominated biocrusts (data without homogeneity of variance). For all other biocrust types an unpaired t test was carried out. (* p≤0.05; p≤0.01; p≤0.001).
Whilst biocrusts represent an important component of the landscape in arid and semi-arid environments and are a natural and most effective force in land stabilization and recovery (Campbell, 1979; Belnap et al., 2003; Weber et al., 2016), no universal method has yet been established to determine the chlorophyll content of the photosynthesizers within these microbial communities. In this study we evaluated the usefulness of different preparatory steps and methods proposed in recent publications to determine the most suitable chlorophyll extraction technique for biocrust samples. A perfect extraction procedure should deliver rapid and reproducible results and must be simple to execute. Furthermore, the solvent utilized should bring all pigments into solution, resolve pigments to extremely low levels of detection, be hazard-free, and cause no chemical changes to the pigments (Jeffrey, 1981).
Our investigations illustrate that DMSO extracted Chl a and Chl a+b pigments to a significantly larger extent than ethanol. Significant differences were observed for all types of biocrusts (Fig. 1). Grinding of samples prior to the extraction procedure, which was analyzed for both dry samples (Fig. 2) and samples that had solvent added (Fig. 3), had a significant negative effect on the extracted amounts of chlorophyll, whereas shaking of samples after each extraction cycle caused significantly increased chlorophyll contents for two of four biocrust types (Fig. 4).
Hiscox and Israelstam (1978) previously stated that DMSO is applicable for a wide range of plant types with variable leaf tissues, Lan et al. (2011) furthermore measured maximum extraction efficiency when using DMSO as the solvent for biocrust analysis. When employing the DMSO method, the duration of the chlorophyll extraction procedure is less critical; after extraction the samples can even be stored in the fridge overnight and spectrometrically analyzed the next day (Barnes et al., 1992). During chlorophyll extraction with DMSO, CaCO3 is added to prevent acidification and minimize the phaeophytinization of Chl a+b (Barnes et al., 1992), which otherwise happens easily, as chlorophyll is sensitive to extreme light exposure, pH, and temperature (Molnár et al., 2013; Hosikian et al., 2010). In studies, where acidification has not been prevented, unnaturally high absorption values were observed for lichen samples around 665 nm (Lan et al., 2011), where pheophytin also absorbs (Ritchie, 2008), therefore suggesting the phaeophytinization of Chl a by lichen acids. Solvents containing methanol, ethanol or 1-propanol are also known to easily degrade Chl a and Chl a+b, as isomerization and allomerization of chlorophyll molecules occurs very easily under acidic conditions (Hynninen, 1977), which need to be avoided by the addition of magnesium carbonate (Ritchie, 2008). The extraction efficiency of DMSO was higher, but also showed larger variability between replicates compared to the ethanol extraction method (Table S1). As thorough chlorophyll extraction has been reported for DMSO as solvent in previous studies (Barnes et al., 1992; Castle et al., 2011) and a good reproducibility of the results has been shown for biocrusts in this study (Table S3) and for leaf fragments by Tait and Hik (2003), we believe that this variability reflects the actual variability in chlorophyll contents between samples. In contrast to the extraction efficiency, ethanol has the advantage of being non-toxic (Lan et al., 2011), whereas DMSO has a potential to carry dissolved substances into the body through the skin (Horita and Weber, 1964; Sulzberger et al., 1967).
Grinding of dry biocrust samples before extraction had a particularly negative effect on green algal lichen- and cyanolichen-dominated biocrusts, which seem to be particularly sensitive to cell damage, leading to chlorophyll degradation. This again, may be caused by lichen acids; however, this negative effect also persisted when grinding was conducted in a wet state with DMSO added prior to grinding. In addition, also moss-dominated biocrusts seemed to suffer from this treatment. The biocrust samples in the current experiments were ground by hand with a mortar and pestle and no high temperatures were reached. In other experiments, cooling with ice may be necessary to avoid overheating, as chlorophyll is easily degraded by heat (Braumann and Grimme, 1979). If the cells are disrupted within less than 20 s, very rapidly and efficiently by a motor driven pestle, cooling with ice may also not be necessary (Schagerl and Künzl, 2007). Loss of extraction efficiency due to the increasing interference of humus, polysaccharides, and clay also cannot be excluded (Lan et al., 2011). In contrast to our results, in other laboratories grinding was observed to improve chlorophyll extraction efficiency (J. Belnap, personal communication, 2017). This could be caused by minor differences in extraction procedures or different sample properties, which will be analyzed in future experiments.
Shaking of biocrust samples after each extraction cycle improved extraction efficiency, as mean extraction quantities were significantly higher for cyanolichen- and chlorolichen-dominated biocrusts, and showed the same tendency for green algal-dominated biocrusts. Solely for moss-dominated biocrusts shaking of samples had no effect. The effectiveness of shaking has also been shown for other substances, such as in the extraction of polyphenols from basil leaves and of toxic elements from artificial saliva (Zlotek et al., 2016; Arain et al., 2013).
Error sources, which may cause an increased variability of the chlorophyll contents, are differences in sample size and composition. Whilst variation in sample size seems negligible, as defined sampling rings of fixed area and height were used, variation in sample growth could not be completely excluded, although during sampling special care was taken to minimize this effect.
In conclusion, the determination of chlorophyll content using DMSO as a solvent ensures a simple, rapid, and stable extraction. A cell-disrupting pretreatment, like sample grinding or homogenization, is not required for a complete chlorophyll extraction and can even cause chlorophyll loss. Moreover, this turned out to be a time consuming step, especially when large sample numbers were processed. In contrast, shaking between two extraction cycles turned out to improve extraction efficiency. An advantage of chlorophyll extraction with DMSO is that the samples are stable for 6–10 days after incubation and can be stored at 4 to 8 ∘C without degradation of the pigments (Ronen and Galun, 1984; Barnes et al., 1992), while in other solvents significant amounts of chlorophyll are lost during storage (Hiscox and Israelstam, 1978). Thus, based on our experiments, we developed a DMSO-based chlorophyll extraction method optimized for green algae-, lichen-, and moss-dominated biocrusts (Supplement S6).
Mean values and standard deviations of all data are listed in the supplement. Raw data can be obtained from the corresponding authors upon request.
The supplement related to this article is available online at: https://doi.org/10.5194/bg-15-1415-2018-supplement.
BW, JC, AT, and NR designed the experiments. AT, ALL, and NR collected soil samples and carried out the laboratory work. JC and BW prepared the manuscript with contributions from all co-authors.
The authors declare that they have no conflict of interest.
This article is part of the special issue “Biological soil crusts and their role in biogeochemical processes and cycling”. It is a result of the BIOCRUST3 conference, Moab, USA, 26 to 30 September 2016.
This work was supported by the Max Planck Society. Bettina Weber would like
to thank Paul Crutzen for the award of a Nobel Laureate Fellowship
(2013–2015), and was financed by the German Research Foundation (DFG-FOR
1525: INUIT; WE2393/2). Alexandra Tamm and Nina Ruckteschler were supported
by the Max Planck Graduate Center (MPGC). We would like to thank Ulrich Pöschl for his overall support and the provision of lab space, as well as Jens Weber
and Heike Pfaff for their help during lab work. For field work at the study
sites, permissions were obtained from the Untere Naturschutzbehörde
Kaiserslautern and the Regierung Unterfranken (in the framework of the SCIN
project).
The article processing charges for
this open-access
publication were covered by the Max Planck
Society.
Edited by: Kees Jan van Groenigen
Reviewed by: Jayne Belnap and Kees Jan van Groenigen
Arain, S. S., Kazi, T. G., Arain, J. B., Afridi, H. I., Brahman, K. D., Shah, F., Naeemullah, Arain, S., and Panhwar, A. H.: Simultaneous preconcentration of toxic elements in artificial saliva extract of smokeless tobacco product, mainpuri by cloud point extraction method, Ecotoxicol. Environ. Saf., 92, 289–296, https://doi.org/10.1016/j.ecoenv.2013.03.001, 2013.
Arnon, D. I.: Copper Enzymes in Isolated Chloroplasts, Polyphenoloxidase in Beta Vulgaris, Plant Physiol., 24, 1–15, 1949.
Bamforth, S. S.: Protozoa of biological soil crusts of a cool desert in Utah, J. Arid Environ., 72, 722–729, https://doi.org/10.1016/j.jaridenv.2007.08.007, 2008.
Barger, N. N., Weber, B., Garcia-Pichel, F., Zaady, E., and Belnap, J.: Patterns and Controls on Nitrogen Cycling of Biological Soil Crusts, Ecol. Stud., 226, 257–285, https://doi.org/10.1007/978-3-319-30214-0_14, 2016.
Barnes, J. D., Balaguer, L., Manrique, E., Elvira, S., and Davison, A. W.: A reappraisal of the use of DMSO for the extraction and determination of chlorophyll a and b in lichens and higher plants, Environ. Exp. Bot., 32, 85–100, 1992.
Belnap, J. and Büdel, B.: Biological Soil Crusts as Soil Stabilizers, in: Biological Soil Crusts: An Organizing Principle in Drylands, edited by: Weber, B., Büdel, B., and Belnap, J., Ecological Studies Springer, Chem, 305–320, 2016.
Belnap, J., Büdel, B., and Lange, O. L.: Biological soil crusts: characteristics and distribution, in: Biological Soil Crusts: Structure, Function, and Management, edited by: Belnap, J. and Lange, O. L., Ecological Studies, Springer, Chem, 3–30, 2003.
Belnap, J., Walker, B. J., Munson, S. M., and Gill, R. A.: Controls on sediment production in two US deserts, Aeolian Res., 14, 15–24, https://doi.org/10.1016/j.aeolia.2014.03.007, 2014.
Brankatschk, R., Fischer, T., Veste, M., and Zeyer, J.: Succession of N cycling processes in biological soil crusts on a Central European inland dune, FEMS Microbiol. Ecol., 83, 149–160, https://doi.org/10.1111/j.1574-6941.2012.01459.x, 2013.
Braumann, T. and Grimme, L. H.: Single-step separation and identification of photosynthetic pigments by high-performance liquid chromatography, J. Chromatogr., 170, 264–268, 1979.
Campbell, S. E.: Soil stabilization by a prokaryotic desert crust: implications for Precambrian land biota, Orig Life, 9, 335–348, 1979.
Castle, S. C., Morrison, C. D., and Barger, N. N.: Extraction of chlorophyll a from biological soil crusts: A comparison of solvents for spectrophotometric determination, Soil Biol. Biochem., 43, 853–856, https://doi.org/10.1016/j.soilbio.2010.11.025, 2011.
Chamizo, S., Canton, Y., Rodriguez-Caballero, E., and Domingo, F.: Biocrusts positively affect the soil water balance in semiarid ecosystems, Ecohydrology, 9, 1208–1221, https://doi.org/10.1002/eco.1719, 2016.
Darby, B. J. and Neher, D. A.: Microfauna Within Biological Soil Crusts, in: Biological Soil Crusts: An Organizing Principle in Drylands, edited by: Weber, B., Büdel, B., and Belnap, J., Ecological Studies, Springer, Chem, 139–157, 2016.
Dojani, S., Büdel, B., Deutschewitz, K., and Weber, B.: Rapid succession of Biological Soil Crusts after experimental disturbance in the Succulent Karoo, South Africa, Appl. Soil Ecol., 48, 263–269, https://doi.org/10.1016/j.apsoil.2011.04.013, 2011.
Elbert, W., Weber, B., Burrows, S., Steinkamp, J., Büdel, B., Andreae, M. O., and Pöschl, U.: Contribution of cryptogamic covers to the global cycles of carbon and nitrogen, Nat. Geosci., 5, 459–462, https://doi.org/10.1038/ngeo1486, 2012.
Eldridge, D. J. and Greene, R. S. B.: Microbiotic Soil Crusts: A Review of their Roles in Soil and Ecological Processes in the Rangelands of Australia, Aust. J. Soil Res., 32, 389–415, 1994.
Evans, R. D. and Belnap, J.: Long-term consequences of disturbance on nitrogen dynamics in an arid ecosystem, Ecology, 80, 150–160, 1999.
Garcia-Pichel, F., Johnson, S. L., Youngkin, D., and Belnap, J.: Small-scale vertical distribution of bacterial biomass and diversity in biological soil crusts from arid lands in the Colorado plateau, Microb. Ecol., 46, 312–321, https://doi.org/10.1007/s00248-003-1004-0, 2003.
Gomez, D. A., Aranibar, J. N., Tabeni, S., Villagra, P. E., Garibotti, I. A., and Atencio, A.: Biological soil crust recovery after long-term grazing exclusion in the Monte Desert (Argentina). Changes in coverage, spatial distribution, and soil nitrogen, Acta Oecol., 38, 33–40, https://doi.org/10.1016/j.actao.2011.09.001, 2012.
Harel, Y., Ohad, I., and Kaplan, A.: Activation of photosynthesis and resistance to photoinhibition in cyanobacteria within biological desert crust, Plant Physiol., 136, 3070–3079, https://doi.org/10.1104/pp.104.047712, 2004.
Hiscox, J. D. and Israelstam, G. F.: A method for the extraction of chlorophyll from leaf tissue without maceration, Can. J. Bot., 57, 1332–1334, 1978.
Horita, A. and Weber, L. J.: Skin penetrating property of drugs dissolved in dimethylsulfoxide (DMSO) and other vehicles, Life Sci., 3, 1389–1395, 1964.
Hosikian, A., Lim, S., Halim, R., and Danquah, M. K.: Chlorophyll Extraction from Microalgae: A Review on the Process Engineering Aspects, International Journal of Chemical Engineering, 1–11, https://doi.org/10.1155/2010/391632, 2010.
Hynninen, P. H.: Chlorophylls, V. Isolation of chlorophylls a and b using an improved two-phase extraction method followed by a precipitation an seperation on a sucrose column, Acta Chem. Scand., 31, 829–835, 1977.
Inskeep, W. P. and Bloom, P. R.: Extinction coefficients of chlorophyll a and b in N,N-Dimethylformamide and 80 % acetone, Plant Physiol., 77, 483–485, 1985.
Jeffrey, S. W.: An improved thin-layer chromatographic technique for marine phytoplankton pigments, Limnol. Oceanogr., 26, 191–197, https://doi.org/10.4319/lo.1981.26.1.0191, 1981.
Lan, S. B., Wu, L., Zhang, D. L., Hu, C. X., and Liu, Y. D.: Ethanol outperforms multiple solvents in the extraction of chlorophyll-a from biological soil crusts, Soil Biol. Biochem., 43, 857–861, https://doi.org/10.1016/j.soilbio.2010.12.007, 2011.
Lenhart, K., Weber, B., Elbert, W., Steinkamp, J., Clough, T., Crutzen, P., Poschl, U., and Keppler, F.: Nitrous oxide and methane emissions from cryptogamic covers, Glob. Chang Biol., 21, 3889–3900, https://doi.org/10.1111/gcb.12995, 2015.
Lösch, R.: Die Ökologie der mainfränkischen Kalktrockenrasen, Beiträge zur naturkundlichen Forschung in Unterfranken, Abh. Verein Würzburg, 21/22, 72–85, 1980.
Mackinney, G.: Absorption of light by chlorophyll solutions, J. Biol. Chem., 140, 315–322, 1941.
Maier, S., Muggia, L., Kuske, C. R., and Grube, M.: Bacteria and Non-lichenized Fungi Within Biological Soil Crusts in: Biological Soil Crusts: An Organizing Principle in Drylands, edited by: Weber, B., Büdel, B., and Belnap, J., Ecological Studies, Springer, Chem, 81–100, 2016.
Meusel, H., Tamm, A., Kuhn, U., Wu, D., Leifke, A. L., Fiedler, S., Ruckteschler, N., Yordanova, P., Lang-Yona, N., Pöhlker, M., Lelieveld, J., Hoffmann, T., Pöschl, U., Su, H., Weber, B., and Cheng, Y.: Emission of nitrous acid from soil and biological soil crusts represents an important source of HONO in the remote atmosphere in Cyprus, Atmos. Chem. Phys., 18, 799–813, https://doi.org/10.5194/acp-18-799-2018, 2018.
Mohr, H. and Schopfer, P.: Plant Physiology, 4th Edn., Springer-Verlag Berlin, Heidelberg, New York, 1995.
Molnár, E., Rippel-Pethö, D., and Bocsi, R.: Solid-liquid extraction of chlorophyll from microalgae from photoautotroph open-air cultivation, Hung. J. Ind. Chem., 41, 119–122, 2013.
Moran, R. and Porath, D.: Chlorophyll Determination in Intact Tissues Using N,N-Dimethylformamide, Plant Physiol., 65, 478–479, 1980.
Rapsch, S. and Ascaso, C.: Effect of evernic acid on structure of spinach chloroplasts, Ann. Bot., 56, 467–473, 1985.
Ritchie, R. J.: Consistent sets of spectrophotometric chlorophyll equations for acetone, methanol and ethanol solvents, Photosynth. Res., 89, 27–41, https://doi.org/10.1007/s11120-006-9065-9, 2006.
Ritchie, R. J.: Universal chlorophyll equations for estimating chlorophylls a, b, c, and d and total chlorophylls in natural assemblages of photosynthetic organisms using acetone, methanol, or ethanol solvents, Photosynthetica, 46, 115–126, https://doi.org/10.1007/s11099-008-0019-7, 2008.
Rodriguez-Caballero, E., Canton, Y., Lazaro, R., and Sole-Benet, A.: Cross-scale interactions between surface components and rainfall properties. Non-linearities in the hydrological and erosive behavior of semiarid catchments, J. Hydrol., 517, 815–825, https://doi.org/10.1016/j.jhydrol.2014.06.018, 2014.
Ronen, R. and Galun, M.: Pigment extraction from lichens with dimethyl sulfoxide (DMSO) and estimation of chlorophyll degradation, Environ. Exp. Bot., 24, 239–245, 1984.
Sancho, L. G., Belnap, J., Colesie, C., Raggio, J., and Weber, B.: Carbon Budgets of Biological Soil Crusts at Micro-, Meso-, and Global Scales, in: Biological Soil Crusts: An Organizing Principle in Drylands, edited by: Weber, B., Büdel, B., and Belnap, J., Ecological Studies, Springer, Chem, 287–304, 2016.
Schagerl, M. and Künzl, G.: Chlorophyll a extraction from freshwater algae – a reevaluation, Biologia, 62, 270–275, https://doi.org/10.2478/s11756-007-0048-x, 2007.
Shoaf, W. T. and Lium, B. W.: Improved extraction of chlorophyll a and b from algae using dimethyl sulfoxide, Limnol. Oceanogr., 6, 926–928, 1976.
Sulzberger, M. B., Cortese, T. A. J., Fishman, L., and Wiley, H. S.: Some effects of DMSO on human skin in vivo, Annals of the New York Academy of Sciences, 141, 437–450, 1967.
Tait, M. A. and Hik, D. S.: Is dimethylsulfoxide a reliable solvent for extracting chlorophyll under field conditions?, Photosynth. Res., 78, 87–91, 2003.
Thorne, S. W., Newcomb, E. H., and Osmond, C. B.: Identification of chlorophyll b in extracts of prokaryotic algae by fluorescence spectroscopy, P. Natl. Acad. Sci. USA, 74, 575–578, 1977.
Weber, B., Wessels, D. C. J., Deutschewitz, K., Dojani, S., Reichenberger, H., and Büdel, B.: Ecological characterization of soil-inhabiting and hypolithic soil crusts within the Knersvlakte, South Africa, Ecol. Process., 2, 13 pp., https://doi.org/10.1186/2192-1709-2-8, 2013.
Weber, B., Wu, D. M., Tamm, A., Ruckteschler, N., Rodriguez-Caballero, E., Steinkamp, J., Meusel, H., Elbert, W., Behrendt, T., Sorgel, M., Cheng, Y. F., Crutzen, P. J., Su, H., and Pöschl, U.: Biological soil crusts accelerate the nitrogen cycle through large NO and HONO emissions in drylands, P. Natl. Acad. Sci. USA, 112, 15384–15389, https://doi.org/10.1073/pnas.1515818112, 2015.
Weber, B., Bowker, M., Zhang, Y., and Belnap, J.: Natural Recovery of Biological Soil Crusts After Disturbance, in: Biological Soil Crusts: An Organizing Principle in Drylands, edited by: Weber, B., Büdel, B., and Belnap, J., Ecological Studies, Springer, Chem, 479–498, 2016.
Zaady, E., Eldridge, D. J., and Bowker, M. A.: Effects of Local-Scale Disturbance on Biocrusts, in: Biological Soil Crusts: An Organizing Principle in Drylands, edited by: Weber, B., Büdel, B., and Belnap, J., Ecological Studies, Springer, Chem, 429–449, 2016.
Zhao, Y. G., Qin, N. Q., Weber, B., and Xu, M. X.: Response of biological soil crusts to raindrop erosivity and underlying influences in the hilly Loess Plateau region, China, Biodivers. Conserv., 23, 1669–1686, https://doi.org/10.1007/s10531-014-0680-z, 2014.
Zlotek, U., Mikulska, S., Nagajek, M., and Swieca, M.: The effect of different solvents and number of extraction steps on the polyphenol content and antioxidant capacity of basil leaves (Ocimum basilicum L.) extracts, Saudi J. Biol. Sci., 23, 628–633, https://doi.org/10.1016/j.sjbs.2015.08.002, 2016.