the Creative Commons Attribution 4.0 License.
the Creative Commons Attribution 4.0 License.
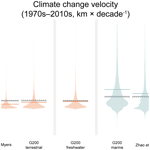
Not all biodiversity rich spots are climate refugia
Qianshuo Zhao
Mark J. Costello
Wolfgang Kiessling
Anthropogenic climate change is increasingly threatening biodiversity on a global scale. Rich spots of biodiversity, regions with exceptionally high endemism and/or number of species, are a top priority for nature conservation. Terrestrial studies have hypothesized that rich spots occur in places where long-term climate change was dampened relative to other regions. Here we tested whether biodiversity rich spots are likely to provide refugia for organisms during anthropogenic climate change. We assessed the spatial distribution of both historic (absolute temperature change and climate change velocities) and projected climate change in terrestrial, freshwater, and marine rich spots. Our analyses confirm the general consensus that global warming will impact almost all rich spots of all three realms and suggest that their characteristic biota is expected to witness similar forcing to other areas, including range shifts and elevated risk of extinction. Marine rich spots seem to be particularly sensitive to global warming: they have warmed more, have higher climate velocities, and are projected to experience higher future warming than non-rich-spot areas. However, our results also suggest that terrestrial and freshwater rich spots will be somewhat less affected than other areas. These findings emphasize the urgency of protecting a comprehensive and representative network of biodiversity-rich areas that accommodate species range shifts under climate change.
- Article
(2797 KB) - Full-text XML
-
Supplement
(5352 KB) - BibTeX
- EndNote
It has been suggested that some geographic areas are exceptionally rich in biodiversity and endemic species because they have had little climate change over geological timescales. This long-term stability has led to high numbers of species that are unique to these “climate refugia” (i.e. endemic species; Dynesius and Jansson, 2000; Jansson, 2003; Harrison and Noss, 2017; Senior et al., 2018; Brown et al., 2020). Here, following Manes et al. (2021), we call these areas biodiversity “rich spots” to emphasize the distinction from the fuzzy concept of biodiversity hotspots, which can be areas of increased temperature, invasive species, pollution, and/or habitat destruction. If the climate refugia hypothesis is true, then rich spots may continue to provide safe harbours (refugia) for species under anthropogenic climate change. Conserving these areas would thus not only protect species against current human impacts, such as hunting, fishing, and habitat loss (Halpern et al., 2015; Díaz et al., 2019; Tedesco et al., 2013), but also limit the effects of climate change on global biodiversity (García Molinos et al., 2016).
1.1 Climate change and the biota
The effects of climate change on biodiversity are detectable since the 1950s (Chaudhary et al., 2021) and are projected to accelerate in coming decades (Manes et al., 2021). However, existing human impacts are also impacting biodiversity in all environments. While most confirmed extinctions and threatened species are terrestrial, a higher proportion of freshwater species are threatened, which is reflected in the higher proportion of freshwater rich spots affected by human impacts (Collen et al., 2014; Costello, 2015; Harrison et al., 2018). Based on species ranges and conservation status, >25 % of IUCN-assessed marine species are threatened in 83 % of the oceans (O'Hara et al., 2019). The lower thermal safety margins of marine ectotherm species renders them more vulnerable to climate change than terrestrial ectotherms (Pinsky et al., 2019).
1.2 Biodiversity rich spots
Biodiversity rich spots have been proposed in many studies based on different criteria, taxa, and geographic contexts (Myers et al., 2000; Mittermeier et al., 2004, 2011; Asaad et al., 2017; Noss et al., 2015). A total of 18 different classifications of marine biodiversity rich spots alone have been proposed (Jefferson and Costello, 2020). The most comprehensive scheme of rich spots is the so-called “WWF Global 200” (Olson and Dinerstein, 2002; G200), which covers terrestrial, freshwater, and marine environments, and has been used in previous climate risk assessments (Warren et al., 2018; Manes et al., 2021). In all cases, the delineation of rich spots was based on expert opinion and limited to a few well-known taxa, such as flowering plants and vertebrates. Thus, it is possible that these biodiversity rich spots may have taxonomic and/or expert knowledge biases. An objective approach to mapping biodiversity rich spots has been applied for the world oceans, using globally standardized data-driven measures of species richness, endemism, habitat, biome, and ecosystem distributions (Zhao et al., 2020). This objective designation of representative biodiversity areas (RBAs) indicated that the 30 % most biodiversity-rich areas of the ocean would contain 68 % of all species, 94 % of coral reefs and mangrove forests, and 86 % of kelp forests and seagrass meadows.
1.3 Climate velocity and range shifts
Climate velocity (Loarie et al., 2009; Burrows et al., 2011) is a key concept to understand the origin and fate of biodiversity rich spots under climate change. The velocity of climate change is the pace and direction at which a specified climate variable moves across geographic space due to changing climate. For example, climate velocity for temperature is the speed at which points of the same temperature (isotherms) move due to changing climate (kilometres per decade). Climate velocities can be assessed over different time intervals (i.e. over decades for recent changes and over millennia for prehistoric changes) to assess the spatial patterns of global temperature change. Regions of high historic, short-term climate velocities are those with low topographic relief on land, particularly flooded grasslands and deserts (Loarie et al., 2009) and tropical and Arctic regions as well as offshore tropical and polar regions in the oceans (Burrows et al., 2011, 2014; García Molinos et al., 2016; Brito-Morales et al., 2018, 2020).
Some terrestrial areas that have experienced relatively low climate velocities since the last glacial maximum are rich in endemic species and hence more likely to be identified as rich spots (Sandel et al., 2011). The related biome constancy (i.e. similar plant-formed habitats over large areas) was also highlighted recently to be associated with higher biodiversity and the likely distribution of biodiversity rich spots (Huntley et al., 2021). Climate velocities are also able to predict the direction and pace of past and future species range shifts (Pinsky et al., 2013; Brito-Morales et al., 2018). Marine species tend to follow the physical pathway dictated by climate velocities more closely than terrestrial species probably due to fewer dispersal barriers than on land and the smaller thermal safety margins of marine species (Sunday et al., 2012; Pinsky et al., 2019). Spatial patterns of climate velocities show regions where species are expected to leave, pass through, or arrive within a certain period under a particular climate change scenario (Burrows et al., 2014). Elevated climate velocities are expected to be especially problematic for endemic species, which may have limited dispersal ability (Sandel et al., 2011; Brito-Morales et al., 2018), especially when they live on islands, mountaintops, or in enclosed seas such as the Mediterranean, from where they can be trapped under global warming.
1.4 Anthropogenic climate change in rich spots
Current policies put the world on track for around 3 ∘C of heating by the end of the century (Hausfather and Peters, 2020). Manes et al. (2021) suggested (based on studies available for half of the rich spots) that at this degree of warming 92 % of land-based endemic species and 95 % of marine endemics face negative consequences, such as a reduction in abundance and increased extinction risk. With the doubling of global warming from 1.5 to 3 ∘C, there is at least a 10 times increase in local extinction risk in biodiversity rich spots: rising from 2 % for all species on land and sea to 20 % and 32 % at risk. Of endemic species, 34 % and 46 % in terrestrial and marine ecosystems and 100 % and 84 % of island and mountain species were projected to face high extinction risk, respectively. The fact that these species are endemic suggests that they cannot disperse to other areas, and thus a local extinction within a rich spot would mean global extinction. However, if warming rates are lower inside than outside these rich spots, then impacts of climate change should be reduced relative to other regions. In other words, biodiversity rich spots, if climate refugia, might represent a “slow lane” that increases chances of adaption and conservation in a changing climate, even if they are not excluded from the changes (Morelli et al., 2020).
The effectiveness of conservation in biodiversity rich spots cannot be estimated without accurate assessment of how much these represent climate refugia. Yet, until now, there has been no comparison of recent or projected global warming inside and outside biodiversity rich spots. Here we assessed the past and future-projected magnitude of climate change in biodiversity rich spots and compared those variables with other regions.
2.1 Environmental data
2.1.1 Observed climatic change and climate velocities
To assess past changes of temperature, we used monthly interpolated data from the CRU TS 4.05 (Harris et al., 2020) and the HadISST1.1 (Rayner et al., 2003) compilations for the near-surface air and ocean surface temperatures (hereafter air and ocean temperatures). We used measurements compiled from the last 50 years to assess the magnitude of change from averages of the 1971–1980 interval until the 2011–2020 interval. The same time span (1971–2020) was used to calculate the velocities of climate change for temperature. Climate change velocities were calculated separately for air and ocean temperatures using the “VoCC” R package (Molinos et al., 2019). Original data layers of historic air temperature had 0.5∘ × 0.5∘ and ocean temperature had 1∘ × 1∘ latitude–longitude resolution. Antarctica was not represented in the air temperature data.
2.1.2 Future climate projections
Future projections of changes of air and ocean temperatures as well as aggregated precipitation were downloaded from the IPCC Atlas of the Working Group I, AR6 report (Iturbide et al., 2021). These are the results of the Coupled Model Intercomparison Project Phase 6 (CMIP6; Eyring et al., 2016) and represent multi-model averages at different stages of warming when global warming reaches the +1.5, +2, and +3 ∘C thresholds compared to the simulated pre-industrial baseline (1850–1900). Data layers that represented the same stage of global warming were averaged across four different scenarios (SSP1-2.6, SSP2-4.5, SSP3-7.0, and SSP5-8.5, when applicable) to obtain a single expectation for the three stages of warming. The data also show comparisons to the pre-industrial baseline. Changes of precipitation were rescaled to mm yr−1. The downloaded future-projected climate data had 1∘ × 1∘ resolution.
2.2 Rich spot schemes
The impact of climate change was assessed using three rich spot schemes: (i) the “WWF Global 200” (G200) scheme of Olson and Dinerstein (2002) is the most comprehensive and was designed to represent areas prioritized for conservation on land, freshwater, and ocean; (ii) the partly overlapping group of terrestrial “hotspots” proposed by Myers et al. (2000) with the modifications of Mittermeier et al. (2011) and Noss et al. (2015; hereafter called Myers rich spots), which is based on species endemism and habitat loss; and (iii) the highest 30 % of marine biodiversity areas of Zhao et al. (2020). Results based on air temperature and precipitation were used to assess terrestrial and freshwater, and ocean temperature data were used to assess marine rich spots.
2.3 Analysis of climate change variables
Prior to the analyses, the climatic data layers were resampled to 0.25∘ × 0.25∘ resolution using the bilinear method, which was necessary to ensure that adequate (albeit smoothed) information was passed to small rich spots. All spatial data items (climate variables and rich spot schemes) were projected to Mollweide equal-area projection. This step ensured that every pixel represents an equal area, so pixel counts translate to cumulated area, and global means are not biased by the unequal spatial sampling along latitudes. For air temperature and precipitation, only land-based values were included in this assessment. The coordinates of rich spot centroids were tabulated to assess the latitudinal patterns of their distribution and those of their characteristic impacts.
We separated our impact variables (historic temperature difference, climate change velocity, projected warming, and precipitation) into values that fall inside and outside a rich spot scheme. Inside and outside rich spot scheme areas were compared with their respective mean values. We also tabulated the impacts for every individual rich spot of all schemes, except that of Zhao et al. (2020), which represents a single area covering 30 % of the ocean. Every rich spot was characterized with one mean value of the equal-area pixels that fell within its boundaries. To express the uncertainty of within-rich-spot climate change due to variability among individual rich spots in a scheme, we executed bootstrap simulations of rich spot means and tabulated their mean in every simulation trial using the areas of rich spots as weights. Errors are reported as the standard deviations of the bootstrap distributions, based on 10 000 trials.
For estimates of historic temperature change, we tabulated the proportion of pixels in a rich spot that have been warming in the past 50 years. Rich spots that had more than 95 % of their pixels above +0 ∘C were considered to have been significantly affected by climate change. We also tabulated percentiles 2.5 and 97.5 of the distributions of pixels in every rich spot and contrasted these with the global and latitudinal means of the respective variables. Rich spots where the global mean was above the rich spot's percentile 97.5 were considered global refugia; those with the global mean below percentile 2.5 were considered critically warming. Refugia and critically warming rich spots within latitudinal bands were tabulated the same way and only compared to the variable's value at the latitude of the rich spot's centroid (Fig. 4, Table 1).
Table 1The percentage of rich spots in each environment that have (a) the global and latitudinal mean above 97.5 % of the values within them (global/latitudinal refugia) and (b) those that have the mean below percentile 2.5 (globally/latitudinally warming). The number of rich spots considered is shown in parentheses. The Lord Howe and Norfolk Island rich spot of the terrestrial G200 is not included in the assessment due to its small size.
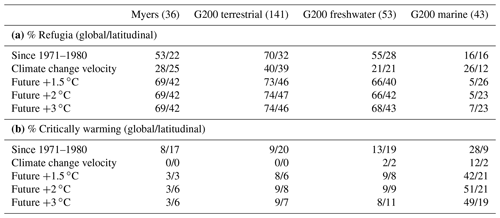
All analyses were performed in the R programming environment (R Development Core Team, 2021). Spatial calculations were executed using the “sp” (Pebesma and Bivand, 2005), “raster” (Hijmans, 2016), and “rgeos” (Bivand and Rundel, 2020) packages, with the utilities of the GDAL library (GDAL/OGR contributors, 2021) directly, and via its R interface “rgdal” (Bivand et al., 2017). Distributions of areas were plotted using the “beanplot” (Kampstra, 2008) package.
3.1 Observed changes
Global warming has increased air temperature of all Myers rich spots, as well as the terrestrial and freshwater G200 rich spots in the past 50 years (Figs. 1, 2a, Table 1). On average, warming in the Myers (+0.91 ± 0.07 ∘C) and G200 freshwater (+0.89 ± 0.07 ∘C) rich spots was less than the global average increase (+1.08 ∘C), whereas the G200 terrestrial rich spots were on par (+1.04 ± 0.1 ∘C). Climate change velocities were slower in all three of these rich spot schemes than in the areas outside them (47 %, 29 %, and 10 % less, in the Myers, G200 terrestrial, and G200 freshwater rich spots, respectively).
Although 10 of the 43 marine rich spots (23 %) did not witness significant overall increases in ocean temperature (Okhotsk Sea, Galápagos, Humboldt Current, Fiji Barrier Reef, Benguela Current, Agulhas Current, Rapa Nui, Patagonian Southwest Atlantic, New Zealand Marine, Antarctic Peninsula and Weddell Sea, Fig. 1a), marine rich spots on average have been affected more by climate change than terrestrial or freshwater rich spots. Ocean temperature in the G200 marine rich spots has increased 41 % more than outside (0.53 ± 0.06 vs. 0.38, with a global average of 0.39 ∘C), and climate velocities were 69 % higher than areas outside (11.24 ± 1.86 vs. 6.64 km per decade, Fig. 2a). This difference is less pronounced when the RBAs of Zhao et al. (2020) were considered: this area faced 4 % more warming (0.41 vs. 0.39 ∘C), and climate velocities have been 33 % larger than outside (8.86 vs. 6.65 km per decade).
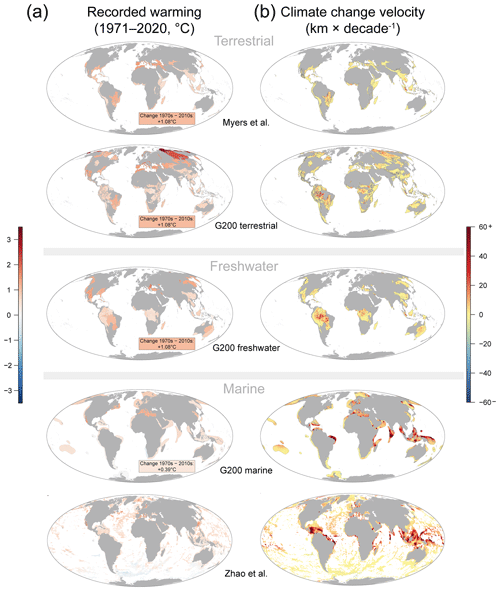
Figure 1Recorded global warming in the terrestrial, freshwater, and marine environments in the past 50 years. (a) The mean absolute changes (∘C) in rich spots between the average annual means between the 1971–1980 interval and the 2011–2020 interval. (b) Spatial distribution of climate change velocities (kilometres per decade). Terrestrial and freshwater rich spots are assessed with near-surface air temperatures, and ocean surface temperatures were used with marine rich spots. Note the high spatial variability of climate change velocities.
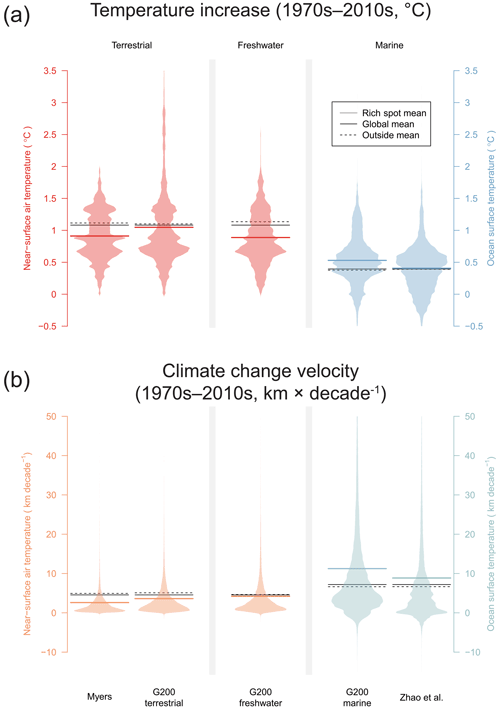
Figure 2Recorded patterns of global warming in rich spots of the terrestrial, freshwater, and marine realm. (a) The difference between 1971–1980 and 2011–2020 and (b) velocities of climate change in the same interval. Beanplots show the distribution of area (density of equal area cells) in the rich spot schemes.
3.2 Projected changes
Near-surface air temperature is projected to warm considerably faster over land than over the seas (+2.03, +2.64, and +3.93 ∘C with +1.5, +2, and +3 ∘C of warming). However, terrestrial rich spots defined using the Myers scheme will be less affected by temperature changes (20 %, 20 %, and 20 % less within than outside at the +1.5, +2, and +3 ∘C warming stages, respectively), whereas areas using the terrestrial G200 are projected to be about as much affected by temperature changes as areas outside them (5 %, 5 %, and 5 % less than outside). When compared to other land areas (Antarctica included), freshwater rich spots are also projected to experience a lower increase in temperature, with 16 %, 15 %, and 14 % less warming than areas outside (Fig. 3).
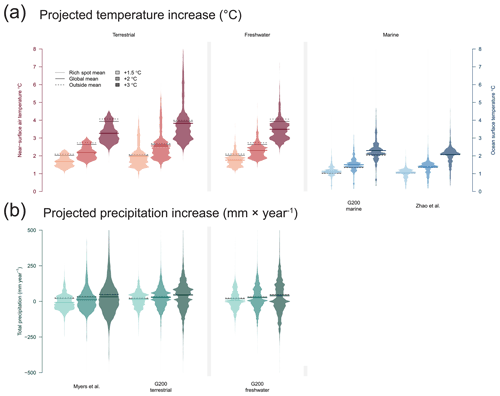
Figure 3Future-projected temperature change and precipitation using the CMIP6-based scenarios at stages of +1.5, +2, and +3 ∘C global warming. Beanplots show the distribution of area (density of equal-area cells) in the rich spot scheme. Solid black lines indicate the land- or ocean-based global means, and dashed lines indicate the mean value outside and inside the rich spots.
Marine rich spots of the G200 will continue to be more affected (12 %, 13 %, and 13 %) than outside areas, with highest and lowest projected warming in the northern and southern polar regions, respectively. The 30 % RBA of Zhao et al. (2020), on the other hand, is expected to be only 1 % more affected by global warming than other areas.
Global precipitation on land is expected to increase by 20, 31, and 46 mm yr−1 with +1.5, +2, and +3 ∘C of warming. Lower-than-outside increases are expected in precipitation in the terrestrial and freshwater rich spots with each projected warming level: Myers: 128 %, 67 %, and 35 % less increase; G200: 43 %, 12 %, and 5 % less increase; freshwater: 59 %, 19 %, and 18 %, respectively. Thus, with greater warming the difference between inside and outside terrestrial and freshwater rich spots decreases.
3.3 Variation across rich spots
Compared to the global mean temperature changes (both observed and future), most terrestrial and freshwater rich spots represent climate refugia (Table 1a, Fig. 4), and only a minority of these (<20 %) are expected to warm critically (Table 1b). In contrast to terrestrial and freshwater rich spots, most marine ones of the G200 are not climate refugia, with the notable exception of the Antarctic rich spot (Fig. 1), and a considerable number of marine rich spots are positioned in high-velocity areas. Almost half of the marine G200 rich spots are expected to face higher-than-global warming in this century.
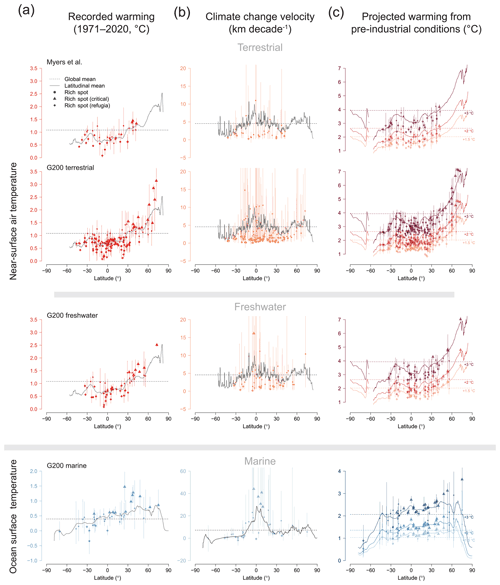
Figure 4Latitudinal patterns of global warming in individual rich spots. (a) Recorded absolute changes between the average annual means in the 1971–1980 interval and the 2011–2020 interval, (b) climate change velocities in the same interval, (c) projected warming compared to pre-industrial conditions when warming reaches the +1.5, +2, and +3 ∘C levels (averaged across multiple scenarios). Dashed lines indicate global means (only land or ocean, respectively), and solid curves indicate the latitudinal means. Vertical bars denote the interval between percentiles 2.5 and 97.5 of values within one rich spot. Triangles indicate rich spots that are critically warming compared to the global mean, and diamonds indicate global refugia. See Table 1 for the tabulation of refugia and critically warming rich spots.
These differences cannot be attributed to latitudinal bias because the latitudinal distribution of rich spots is similar in all three environments (see Supplement). Northern high-latitude rich spots will warm most, whereas the Southern Ocean and the upwelling on the Atlantic coast of southern Africa will cool (Figs. 1, 4). Following the latitudinal patterns of warming, rich spots in the Northern Hemisphere are disproportionally more affected by the magnitude of temperature increase than those in the Southern Hemisphere (Fig. 4). Terrestrial and freshwater rich spots tend to occur in places where climate velocities are comparatively lower than those suggested by the latitudinal average (Fig. 4b).
Our results show that although the impacts of climate change have been lower in terrestrial and freshwater rich spots, they have been and are projected to be affected by climate change. Marine biodiversity rich spots have and are projected to experience greater effects of climate change than other areas. This discrepancy reflects both the spatial distribution of rich spots and the latitudinal patterns of climate change. The hemisphere and latitudinal imbalance of global warming is expected to further exacerbate the already asymmetric human impact on the marine environment and biodiversity (Halpern et al., 2015; Sydeman et al., 2021).
Although overall warming is expected to affect marine rich spots only slightly more based on future projections, the velocity of climate change is extremely high in tropical rich spots. Species have already responded to these changes by shifting their latitudinal distributions poleward (Lenoir et al., 2020), which has already led to the loss of thousands of marine species from equatorial latitudes and increases in species richness in the subtropics (Chaudhary et al., 2021).
The high climate velocities in marine rich spots seem to contradict the previously suggested relationship between climate and endemism based on long-term climate change velocity (Sandel et al., 2011). In comparison to terrestrial and freshwater areas, the distribution of biodiversity in the ocean is more influenced by environmental conditions than geographic isolation, reflecting the higher habitat connectivity in the ocean. The rate of species endemism also reflects differences among the environments and is exceptionally high in freshwater biogeographic realms, at 89 %–96 % for fish in all but one realm, compared to 11 %–98 % for terrestrial vertebrate groups and 17 %–84 % for marine realms (Costello and Chaudhary, 2017; Costello et al., 2017; Leroy et al., 2019). The effects of the assessed variables likely have varying importance among the different realms, and marine species also tend to utilize more of their fundamental abiotic niches (Sunday et al., 2012), which might manifest in a different distribution of biodiversity. Also, climate change today is happening on much shorter timescales than what may have influenced the evolutionary origin of rich spots and the distribution of endemics.
It is also possible that the definition of older rich spot schemes is not representative of true biodiversity. The G200 rich spots were partly driven by political priorities (“make every nation a stakeholder”; Olson and Dinerstein, 2002), and the Myers et al. (2000) rich spots were also prioritized based on threat from other human impacts in addition to their rich biodiversity. The systematically lower difference between warming inside and outside the RBA of Zhao et al. (2020) compared to the marine G200 might suggest that the former grasps patterns of richness and endemism better than the latter (Fig. 1).
The present study did not consider annual variation and additional climatic variables that might influence the distribution of species (Fick and Hijmans, 2017). Small-scale climate refugia might exist within the individual rich spots, which are not detected due to the spatial scale of our analyses. Where there is high heterogeneity of climate change velocities (e.g. due to topographic variation) at a spatial resolution finer than that used in our analysis, species may find thermal refugia within terrestrial and freshwater rich spots. Projections as used here need to be validated by in situ monitoring of changes in species distribution, which might also inform species-based approaches to refugia (Michalak et al., 2020).
The result that terrestrial and freshwater rich spots represent relative climate refugia suggests that focusing efforts on terrestrial and freshwater rich spots may offer a potential increase in the effectiveness of conservation. As these areas represent most of the world's biodiversity it may therefore be speculated that biodiversity may not be as badly affected by climate change. That being said, our prediction is that climate change will impact rich spot areas as well, which agrees with simulations that suggest biome changes by the end of the century in a considerable area of the terrestrial environment – including rich spots (Huntley et al., 2021). Accordingly, conservation of species will benefit from expanding protection to areas adjacent to rich spots (Huntley et al., 2021) and/or a network of protected areas so species can adjust their ranges in a changing world.
Our findings support the hypothesis that most terrestrial rich spots have been climate refugia in a relative sense, but they do not relax concerns regarding the effects of global warming on endemic species. While thousands of species are shifting their geographic ranges rapidly in response to a warming climate, there is a high risk that endemic species will not be able to disperse to more suitable climates and go extinct (Manes et al., 2021). Climate change mitigation is thus essential to keeping climate warming to less than 2 ∘C to reduce extinction risk in all rich spots (Manes et al., 2021).
Assessment of the impact of climate change on biodiversity rich spots is compounded by human-induced losses of species and habitats across all environments. As stated repeatedly in the scientific literature for decades, strict protection of biodiversity from local human impacts within rich spots is the most area-effective way to minimize species extinctions and increase resilience to biodiversity loss (Mittermeier et al., 2011; Darwall et al., 2018; Zhao et al., 2020). In addition, environmentally sustainable practices inside and outside rich spots must facilitate species dispersal between habitats as climate change occurs.
Past climate data are openly available from the website of the MetOffice Hadley Centre (https://www.metoffice.gov.uk/hadobs/hadisst/, last access: 13 April 2021, Rayner et al., 2003) and Climatic Research Unit (University of East Anglia, https://crudata.uea.ac.uk/cru/data/hrg/, last access: 12 April 2021, Harris et al., 2020). Results of the CMIP6 climate data are publicly available from the IPCC Atlas of the AR6 report (https://interactive-atlas.ipcc.ch/, last access: 27 May 2021, Iturbide et al., 2021). Rich spot definition schemes are available from the WWF (https://www.worldwildlife.org/publications/global-200, last access: 15 May 2020, Olson and Dinerstein, 2002), Zenodo (https://doi.org/10.5281/zenodo.3261807, Hoffman et al., 2016, and https://www.sciencedirect.com/science/article/pii/S0006320719312182#ec-research-data Zhao, 2020). Coastlines were plotted using free vector data from Natural Earth (https://www.naturalearthdata.com/about/terms-of-use/, last access: 8 June 2020). Used data and the analytical code are archived on Zenodo along with supplementary display items and the results used to plot figures (https://doi.org/10.5281/zenodo.5669968, Kocsis et al., 2021).
The supplement related to this article is available online at: https://doi.org/10.5194/bg-18-6567-2021-supplement.
This paper arose from discussions within IPCC WGII Cross Cutting Chapter on Biodiversity Hotspots. The study was designed by MJC and WK. ÁTK and MJC drafted the first versions of the paper. ÁTK carried out all the analyses and wrote the final version of the paper. All authors contributed to the discussions and revisions of the study.
The contact author has declared that neither they nor their co-authors have any competing interests.
Publisher’s note: Copernicus Publications remains neutral with regard to jurisdictional claims in published maps and institutional affiliations.
Comments from the two anonymous referees helped improve the study.
The research has been supported by the Deutsche Forschungsgemeinschaft (Ko 5382/2-1 and Ki 806/15-2) and is part of the DFG Research Unit TERSANE 2 (FOR 2332).
This paper was edited by Sara Vicca and reviewed by two anonymous referees.
Asaad, I., Lundquist, C. J., Erdmann, M. V., and Costello, M. J.: Ecological criteria to identify areas for biodiversity conservation, Biol. Conserv., 213, 309–316, https://doi.org/10.1016/j.biocon.2016.10.007, 2017.
Bivand, R. and Rundel, C.: rgeos: Interface to Geometry Engine – Open Source (“GEOS”), version 0.5-5, available at: https://cran.r-project.org/package=rgeos, 2020.
Bivand, R., Keitt, T., and Rowlingson, B.: rgdal: Bindings for the Geospatial Data Abstraction Library, version 1.5-23, available at: https://cran.r-project.org/package=rgdal, 2017.
Brito-Morales, I., Molinos, J. G., Schoeman, D. S., Burrows, M. T., Poloczanska, E. S., Brown, C. J., Ferrier, S., Harwood, T. D., Klein, C. J., McDonald-Madden, E., Moore, P. J., Pandolfi, J. M., Watson, J. E. M., Wenger, A. S., and Richardson, A. J.: Climate Velocity Can Inform Conservation in a Warming World, Trend. Ecol. Evol., 33, 441–457, https://doi.org/10.1016/j.tree.2018.03.009, 2018.
Brito-Morales, I., Schoeman, D. S., Molinos, J. G., Burrows, M. T., Klein, C. J., Arafeh-Dalmau, N., Kaschner, K., Garilao, C., Kesner-Reyes, K., and Richardson, A. J.: Climate velocity reveals increasing exposure of deep-ocean biodiversity to future warming, Nat. Clim. Change, 10, 576–581, https://doi.org/10.1038/s41558-020-0773-5, 2020.
Brown, S. C., Wigley, T. M. L., Otto-Bliesner, B. L., Rahbek, C., and Fordham, D. A.: Persistent Quaternary climate refugia are hospices for biodiversity in the Anthropocene, Nat. Clim. Change, 10, 244–248, https://doi.org/10.1038/s41558-019-0682-7, 2020.
Burrows, M. T., Schoeman, D. S., Buckley, L. B., Moore, P., Poloczanska, E. S., Brander, K. M., Brown, C., Bruno, J. F., Duarte, C. M., Halpern, B. S., Holding, J., Kappel, C. V., Kiessling, W., O'Connor, M. I., Pandolfi, J. M., Parmesan, C., Schwing, F. B., Sydeman, W. J., and Richardson, A. J.: The Pace of Shifting Climate in Marine and Terrestrial Ecosystems, Science, 334, 652–655, https://doi.org/10.1126/science.1210288, 2011.
Burrows, M. T., Schoeman, D. S., Richardson, A. J., Molinos, J. G., Hoffmann, A., Buckley, L. B., Moore, P. J., Brown, C. J., Bruno, J. F., Duarte, C. M., Halpern, B. S., Hoegh-Guldberg, O., Kappel, C. V., Kiessling, W., O'Connor, M. I., Pandolfi, J. M., Parmesan, C., Sydeman, W. J., Ferrier, S., Williams, K. J., and Poloczanska, E. S.: Geographical limits to species-range shifts are suggested by climate velocity, Nature, 507, 492–495, https://doi.org/10.1038/nature12976, 2014.
Chaudhary, C., Richardson, A. J., Schoeman, D. S., and Costello, M. J.: Global warming is causing a more pronounced dip in marine species richness around the equator, P. Natl. Acad. Sci., 118, e2015094118, https://doi.org/10.1073/pnas.2015094118, 2021.
Collen, B., Whitton, F., Dyer, E. E., Baillie, J. E. M., Cumberlidge, N., Darwall, W. R. T., Pollock, C., Richman, N. I., Soulsby, A.-M., and Böhm, M.: Global patterns of freshwater species diversity, threat and endemism, Global Ecol. Biogeogr., 23, 40–51, https://doi.org/10.1111/geb.12096, 2014.
Costello, M. J.: Biodiversity: The Known, Unknown, and Rates of Extinction, Curr. Biol., 25, R368–R371, https://doi.org/10.1016/j.cub.2015.03.051, 2015.
Costello, M. J. and Chaudhary, C.: Marine Biodiversity, Biogeography, Deep-Sea Gradients, and Conservation, Curr. Biol., 27, R511–R527, https://doi.org/10.1016/j.cub.2017.04.060, 2017.
Costello, M. J., Tsai, P., Wong, P. S., Cheung, A. K. L., Basher, Z., and Chaudhary, C.: Marine biogeographic realms and species endemicity, Nat. Commun., 8, 1057, https://doi.org/10.1038/s41467-017-01121-2, 2017.
Darwall, W., Bremerich, V., Wever, A. D., Dell, A. I., Freyhof, J., Gessner, M. O., Grossart, H.-P., Harrison, I., Irvine, K., Jähnig, S. C., Jeschke, J. M., Lee, J. J., Lu, C., Lewandowska, A. M., Monaghan, M. T., Nejstgaard, J. C., Patricio, H., Schmidt-Kloiber, A., Stuart, S. N., Thieme, M., Tockner, K., Turak, E., and Weyl, O.: The Alliance for Freshwater Life: A global call to unite efforts for freshwater biodiversity science and conservation, Aquat. Conserv., 28, 1015–1022, https://doi.org/10.1002/aqc.2958, 2018.
Díaz, S., Settele, J., Brondízio, E. S., Ngo, H. T., Agard, J., Arneth, A., Balvanera, P., Brauman, K. A., Butchart, S. H. M., Chan, K. M. A., Garibaldi, L. A., Ichii, K., Liu, J., Subramanian, S. M., Midgley, G. F., Miloslavich, P., Molnár, Z., Obura, D., Pfaff, A., Polasky, S., Purvis, A., Razzaque, J., Reyers, B., Chowdhury, R. R., Shin, Y.-J., Visseren-Hamakers, I., Willis, K. J., and Zayas, C. N.: Pervasive human-driven decline of life on Earth points to the need for transformative change, Science, 366, eaax3100, https://doi.org/10.1126/science.aax3100, 2019.
Dynesius, M. and Jansson, R.: Evolutionary consequences of changes in species' geographical distributions driven by Milankovitch climate oscillations, P. Natl. Acad. Sci. USA, 97, 9115–9120, https://doi.org/10.1073/pnas.97.16.9115, 2000.
Eyring, V., Bony, S., Meehl, G. A., Senior, C. A., Stevens, B., Stouffer, R. J., and Taylor, K. E.: Overview of the Coupled Model Intercomparison Project Phase 6 (CMIP6) experimental design and organization, Geosci. Model Dev., 9, 1937–1958, https://doi.org/10.5194/gmd-9-1937-2016, 2016.
Fick, S. E. and Hijmans, R. J.: WorldClim 2: new 1-km spatial resolution climate surfaces for global land areas, Int. J. Climatol., 37, 4302-4315, https://doi.org/10.1002/joc.5086, 2017.
García Molinos, J., Halpern, B. S., Schoeman, D. S., Brown, C. J., Kiessling, W., Moore, P. J., Pandolfi, J. M., Poloczanska, E. S., Richardson, A. J., and Burrows, M. T.: Climate velocity and the future global redistribution of marine biodiversity, Nat. Clim. Change, 6, 83–88, https://doi.org/10.1038/nclimate2769, 2016.
GDAL/OGR contributors: GDAL/OGR geospatial data abstraction software library, version 2.2.3, available at: https://launchpad.net/ubuntu/bionic/amd64/libgdal-dev/2.2.3+dfsg-2, 2021.
Halpern, B. S., Frazier, M., Potapenko, J., Casey, K. S., Koenig, K., Longo, C., Lowndes, J. S., Rockwood, R. C., Selig, E. R., Selkoe, K. A., and Walbridge, S.: Spatial and temporal changes in cumulative human impacts on the world's ocean, Nat. Commun., 6, 7615, https://doi.org/10.1038/ncomms8615, 2015.
Harris, I., Osborn, T. J., Jones, P., and Lister, D.: Version 4 of the CRU TS monthly high-resolution gridded multivariate climate dataset, Sci. Data, 7, 109, https://doi.org/10.1038/s41597-020-0453-3, 2020.
Harrison, I., Abell, R., Darwall, W., Thieme, M. L., Tickner, D., and Timboe, I.: The freshwater biodiversity crisis, Science, 362, 1369–1369, https://doi.org/10.1126/science.aav9242, 2018.
Harrison, S. and Noss, R.: Endemism hotspots are linked to stable climatic refugia, Ann. Bot., 119, 207–214, https://doi.org/10.1093/aob/mcw248, 2017.
Hausfather, Z. and Peters, G. P.: Emissions – the “business as usual” story is misleading, Nature, 577, 618–620, https://doi.org/10.1038/d41586-020-00177-3, 2020.
Hijmans, R. J.: raster: Geographic Data Analysis and Modeling, version 3.4-13, available at: https://cran.r-project.org/package=raster, 2016.
Hoffman, M., Koenig, K., Bunting, G., Costanza, J., and Williams, K. J.: Biodiversity Hotspots (version 2016.1) (2016.1), Zenodo [data set], https://doi.org/10.5281/zenodo.3261807, 2016.
Huntley, B., Allen, J. R. M., Forrest, M., Hickler, T., Ohlemüller, R., Singarayer, J. S., Valdes, P. J., and Williams, J.: Projected climatic changes lead to biome changes in areas of previously constant biome, J. Biogeogr., 48, 2418–2428, https://doi.org/10.1111/jbi.14213, 2021.
Iturbide, M., Fernández, J., Gutiérrez, J. M., Bedia, J., Cimadevilla, E., Díez-Sierra, J., Manzanas, R., Casanueva, A., Baño-Medina, J., Milovac, J., Herrera, S., Cofiño, A. S., San Martín, D., García-Díez, M., Hauser, M., Huard, D., and Yelekci, Ö.: Repository supporting the implementation of FAIR principles in the IPCC-WGI Atlas (v2.0), Zenodo [data set], https://doi.org/10.5281/zenodo.5176260, 2021.
Jansson, R.: Global patterns in endemism explained by past climatic change, P. Roy. Soc. Lond. B, 270, 583–590, https://doi.org/10.1098/rspb.2002.2283, 2003.
Jefferson, T. and Costello, M. J.: Hotspots of Marine Biodiversity, in: Encyclopedia of the World's Biomes, edited by: Goldstein, M. I. and DellaSala, D. A., Elsevier, 586–596, https://doi.org/10.1016/B978-0-12-409548-9.11952-9, 2020.
Kampstra, P.: Beanplot: A boxplot alternative for visual comparison of distributions, version 1.2, J. Stat. Softw., 28, 1–9, available at: https://cran.r-project.org/package=beanplot, 2008.
Kocsis, Á. T., Zhao, Q., Costello, M. J., and Kiessling, W.: Supplementary Information for “Not all biodiversity richspots are climate refugia” (v0.4.1), Zenodo [code and data set], https://doi.org/10.5281/zenodo.5669968, 2021.
Lenoir, J., Bertrand, R., Comte, L., Bourgeaud, L., Hattab, T., Murienne, J., and Grenouillet, G.: Species better track climate warming in the oceans than on land, Nat. Ecol. Evol., 4, 1044–1059, https://doi.org/10.1038/s41559-020-1198-2, 2020.
Leroy, B., Dias, M. S., Giraud, E., Hugueny, B., Jézéquel, C., Leprieur, F., Oberdorff, T., and Tedesco, P. A.: Global biogeographical regions of freshwater fish species, J. Biogeogr., 46, 2407–2419, https://doi.org/10.1111/jbi.13674, 2019.
Loarie, S. R., Duffy, P. B., Hamilton, H., Asner, G. P., Field, C. B., and Ackerly, D. D.: The velocity of climate change, Nature, 462, 1052–1055, https://doi.org/10.1038/nature08649, 2009.
Manes, S., Costello, M. J., Beckett, H., Debnath, A., Devenish-Nelson, E., Grey, K.-A., Jenkins, R., Khan, T. M., Kiessling, W., Krause, C., Maharaj, S. S., Midgley, G. F., Price, J., Talukdar, G., and Vale, M. M.: Endemism increases species' climate change risk in areas of global biodiversity importance, Biol. Conserv., 257, 109070, https://doi.org/10.1016/j.biocon.2021.109070, 2021.
Michalak, J. L., Stralberg, D., Cartwright, J. M., and Lawler, J. J.: Combining physical and species-based approaches improves refugia identification, Front. Ecol. Environ., 18, 254–260, https://doi.org/10.1002/fee.2207, 2020.
Mittermeier, R., Patricio, R., Hoffman, M., Pilgrim, J., Brooks, T., Mittermeier, C., Lamoreux, J., and da Fonseca, G.: Hotspots revisited CEMEX, Cemex, Mexico City, 392 pp., 2004.
Mittermeier, R. A., Turner, W. R., Larsen, F. W., Brooks, T. M., and Gascon, C.: Global biodiversity conservation: the critical role of hotspots, in: Biodiversity hotspots, Springer, Berlin, 3–22, 2011.
Molinos, J. G., Schoeman, D. S., Brown, C. J., and Burrows, M. T.: VoCC: An r package for calculating the velocity of climate change and related climatic metrics, Meth. Ecol. Evol., 10, 2195–2202, https://doi.org/10.1111/2041-210X.13295, 2019.
Morelli, T. L., Barrows, C. W., Ramirez, A. R., Cartwright, J. M., Ackerly, D. D., Eaves, T. D., Ebersole, J. L., Krawchuk, M. A., Letcher, B. H., Mahalovich, M. F., Meigs, G. W., Michalak, J. L., Millar, C. I., Quiñones, R. M., Stralberg, D., and Thorne, J. H.: Climate-change refugia: biodiversity in the slow lane, Front. Ecol. Environ., 18, 228–234, https://doi.org/10.1002/fee.2189, 2020.
Myers, N., Mittermeier, R. A., Mittermeier, C. G., da Fonseca, G. A. B., and Kent, J.: Biodiversity hotspots for conservation priorities, Nature, 403, 853–858, https://doi.org/10.1038/35002501, 2000.
Noss, R. F., Platt, W. J., Sorrie, B. A., Weakley, A. S., Means, D. B., Costanza, J., and Peet, R. K.: How global biodiversity hotspots may go unrecognized: lessons from the North American Coastal Plain, Divers. Distrib., 21, 236–244, https://doi.org/10.1111/ddi.12278, 2015.
O'Hara, C. C., Villaseñor-Derbez, J. C., Ralph, G. M., and Halpern, B. S.: Mapping status and conservation of global at-risk marine biodiversity, Conserv. Lett., 12, e12651, https://doi.org/10.1111/conl.12651, 2019.
Olson, D. M. and Dinerstein, E.: The Global 200: Priority Ecoregions for Global Conservation, Ann. MO Bot. Gard., 89, 199–224, https://doi.org/10.2307/3298564, 2002.
Pebesma, E. J. and Bivand, R. S.: Classes and methods for spatial data in R, R News, 5, 9–13, 2005.
Pinsky, M. L., Worm, B., Fogarty, M. J., Sarmiento, J. L., and Levin, S. A.: Marine Taxa Track Local Climate Velocities, Science, 341, 1239–1242, https://doi.org/10.1126/science.1239352, 2013.
Pinsky, M. L., Eikeset, A. M., McCauley, D. J., Payne, J. L., and Sunday, J. M.: Greater vulnerability to warming of marine versus terrestrial ectotherms, Nature, 569, 108–111, https://doi.org/10.1038/s41586-019-1132-4, 2019.
Rayner, N. A., Parker, D. E., Horton, E. B., Folland, C. K., Alexander, L. V., Rowell, D. P., Kent, E. C., and Kaplan, A.: Global analyses of sea surface temperature, sea ice, and night marine air temperature since the late nineteenth century, J. Geophys. Res.-Atmos., 108, 4407, https://doi.org/10.1029/2002JD002670, 2003.
R Development Core Team: R: A language and environment for statistical computing, R Foundation for Statistical Computing, Vienna, version 4.1.0, available at: https://www.r-project.org/, 2021.
Sandel, B., Arge, L., Dalsgaard, B., Davies, R. G., Gaston, K. J., Sutherland, W. J., and Svenning, J.-C.: The Influence of Late Quaternary Climate-Change Velocity on Species Endemism, Science, 334, 660–664, https://doi.org/10.1126/science.1210173, 2011.
Senior, R. A., Hill, J. K., Benedick, S., and Edwards, D. P.: Tropical forests are thermally buffered despite intensive selective logging, Glob. Change Biol., 24, 1267–1278, https://doi.org/10.1111/gcb.13914, 2018.
Sunday, J. M., Bates, A. E., and Dulvy, N. K.: Thermal tolerance and the global redistribution of animals, Nat. Clim. Change, 2, 686–690, https://doi.org/10.1038/nclimate1539, 2012.
Sydeman, W. J., Schoeman, D. S., Thompson, S. A., Hoover, B. A., García-Reyes, M., Daunt, F., Agnew, P., Anker-Nilssen, T., Barbraud, C., Barrett, R., Becker, P. H., Bell, E., Boersma, P. D., Bouwhuis, S., Cannell, B., Crawford, R. J. M., Dann, P., Delord, K., Elliott, G., Erikstad, K. E., Flint, E., Furness, R. W., Harris, M. P., Hatch, S., Hilwig, K., Hinke, J. T., Jahncke, J., Mills, J. A., Reiertsen, T. K., Renner, H., Sherley, R. B., Surman, C., Taylor, G., Thayer, J. A., Trathan, P. N., Velarde, E., Walker, K., Wanless, S., Warzybok, P., and Watanuki, Y.: Hemispheric asymmetry in ocean change and the productivity of ecosystem sentinels, Science, 372, 980–983, https://doi.org/10.1126/science.abf1772, 2021.
Tedesco, P. A., Oberdorff, T., Cornu, J. F., Beauchard, O., Brosse, S., Dürr, H. H., Grenouillet, G., Leprieur, F., Tisseuil, C., Zaiss, R., and Hugueny, B.: A scenario for impacts of water availability loss due to climate change on riverine fish extinction rates, J. Appl. Ecol., 50, 1105–1115, https://doi.org/10.1111/1365-2664.12125, 2013.
Warren, R., Price, J., VanDerWal, J., Cornelius, S., and Sohl, H.: The implications of the United Nations Paris Agreement on climate change for globally significant biodiversity areas, Climatic Change, 147, 395–409, https://doi.org/10.1007/s10584-018-2158-6, 2018.
Zhao, Q.: Where Marine Protected Areas would best represent 30 % of ocean biodiversity, Mendeley Data, V1, https://doi.org/10.17632/wk6s7kh48m.1, 2020.
Zhao, Q., Stephenson, F., Lundquist, C., Kaschner, K., Jayathilake, D., and Costello, M. J.: Where Marine Protected Areas would best represent 30 % of ocean biodiversity, Biol. Conserv., 244, 108536, https://doi.org/10.1016/j.biocon.2020.108536, 2020.