the Creative Commons Attribution 4.0 License.
the Creative Commons Attribution 4.0 License.
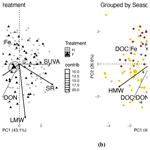
Seasonal controls override forest harvesting effects on the composition of dissolved organic matter mobilized from boreal forest soil organic horizons
Keri L. Bowering
Kate A. Edwards
Dissolved organic matter (DOM) mobilized from the organic (O) horizons of forest soils is a temporally dynamic flux of carbon (C) and nutrients, and the fate of this DOM in downstream pools is dependent on the rate and pathways of water flow as well as its chemical composition. Here, we present observations of the composition of DOM mobilized weekly to monthly from O horizons in mature forest and adjacent harvested treatment plots. The study site was experimentally harvested, without replanting, 10-years prior to this study. Thus, the treatments differ significantly in terms of forest stand and soil properties, and they interact differently with the regional hydrometeorological conditions. This presented an opportunity to investigate the role of forest structure relative to environmental variation on soil DOM mobilization. On an annual basis, fluxes of total dissolved nitrogen (TDN) and dissolved organic nitrogen (DON) were largest from the warmer and thinner O horizons of the harvested (H) treatment compared to the forest (F) treatment; however, neither phosphate nor ammonium fluxes differed by treatment type. On a short-term basis in both H and F treatments, all fluxes were positively correlated to water input, and all concentrations were positively correlated to soil temperature and negatively correlated to water input. Soil moisture was negatively correlated to the C : N of DOM. These results suggest common seasonal controls on DOM mobilization regardless of harvesting treatment. Optical characterization of seasonally representative samples additionally supported a stronger control of season over harvesting. The chemical character of DOM mobilized during winter and snowmelt: lower C : N, higher specific ultraviolet absorbance and lower molecular weight of chromophoric DOM (CDOM; higher spectral slope ratio) were representative of relatively more decomposed DOM compared to that mobilized in summer and autumn. This shows that the decomposition of soil organic matter underneath a consistently deep snowpack is a key determinant of the composition of DOM mobilized from O horizons during winter and the hydrologically significant snowmelt period regardless of harvesting impact. Despite the higher proportion of aromatic DOM in the snowmelt samples, its lower molecular weight and rapid delivery from O to mineral horizons suggests that the snowmelt period is not likely to be a significant period of DOM sequestration by mineral soil. Rather, the higher-molecular-weight, high-C : N DOM mobilized during slow and relatively infrequent delivery during summer and rapid, frequent delivery during autumn are more likely to support periods of mineral soil sequestration and increased export of fresher terrestrial DOM, respectively. These observed seasonal dynamics in O horizon DOM suggest the predicted decreases in winter and spring snowfall and increasing autumn and winter rainfall with climate warming in this region will enhance mobilization of DOM that is more reactive to mineral interactions in deeper soil, but also more biological and photoreactive in the aquatic environment. Understanding the downstream consequences of this mobilized DOM in response to these shifts in precipitation timing and form can improve our ability to predict and manage forest C balance but requires understanding the response of landscape hydrology to these changing precipitation regimes.
- Article
(2103 KB) - Companion paper
-
Supplement
(2311 KB) - BibTeX
- EndNote
Dissolved organic matter (DOM) mobilized from organic horizons of forest soils represents an ecologically significant source of carbon (C) and nutrients both within forest catchments (Qualls and Haines, 1991) and from soils to aquatic systems (Jansen et al., 2014). The fate of mobilized soil DOM is influenced by both water flow dynamics (rate and pathways) and the chemical composition of DOM (Roulet and Moore, 2006), although the interaction of these two factors is not often captured in soil studies. The composition of mobilized organic horizon DOM is the net result of production and uptake processes, as well as the relative solubility of organic matter inputs from different sources. While soil extractions provide valuable information on potential sources, bioavailability, and production mechanisms of soil DOM (i.e., Jones and Kielland, 2012; Hensgens et al., 2020), as well as transformation and fate in mineral soil (Kothawala et al., 2008), they cannot capture the interaction of these factors with local hydrometeorological conditions important to understanding the net movement of DOM in situ. Measurements that incorporate the role of soil hydrology with DOM mobilization place the knowledge gained from extraction studies into the larger-catchment-scale context. For instance, these measurements can more directly inform regional chromatography (i.e., Kaiser and Kalbitz, 2012; Shen et al., 2014) and terrestrial-to-aquatic C flux conceptual models (i.e., Tank et al., 2018), which further allow us to assess the impacts of disturbances such as harvesting and climate change on landscape C balance (Casas-Ruiz et al., 2023).
Forest C and nitrogen (N) cycles are tightly linked, and the C : N of bulk soil provides clues about ecosystem functioning and the bioavailability of soil organic matter. Similarly, the C : N of DOM is considered a measure of DOM bioavailability (McDowell and Likens, 1988; McDowell et al., 2004; McGroddy et al., 2008). However, while C : N of DOM correlates to C : N of soil in some studies (Gödde et al., 1996; Michalzik and Matzner, 1999), it does not in others (Cortina et al., 1995; Michel and Matzner, 1999). The mobilization of dissolved organic C (DOC) relative to dissolved organic N (DON) is correlated on an annual basis (Michalzik et al., 2001), but whether this correlation holds across seasons is not known and could help explain the discrepancies in the relationship between soil C : N and DOM C : N. Additionally, boreal forests accumulate particularly large amounts of C in surface layers because of temperature limitations on soil organic matter decomposition and the recalcitrance of coniferous tree litter and forest floor mosses (Philben et al., 2018; Hensgens et al., 2020). Nitrogen limitations can also affect decomposition and soil C accumulation (Averill and Waring, 2018) and may explain why the C : N of boreal soil organic horizons is higher in areas not affected by industrial N deposition (for instance, Alaskan compared to Swedish boreal forests), with likely effects on soil DOM. These dynamics are further impacted by snow cover, especially during seasonal transition periods (Groffman et al., 2018), but the type of snowpack change is regionally variable with differing effects on the underlying soil (Stark et al., 2020).
Spectroscopic characterization (absorbance and fluorescence) of chromophoric DOM (CDOM) is an efficient technique for describing broad DOM compositional differences in surface waters and identifying terrestrial DOM sources (Helms et al., 2008; McKnight et al., 2001; Jaffé et al., 2008; Berggren and Giorgio, 2015). These techniques have also been used to assess the compositional variability of terrestrial DOM. In litter incubation experiments, for instance, specific ultraviolet absorbance (SUVA) of leached spruce needles increased during decomposition because of increased solubility of lignin as it became more degraded (Hansson et al., 2010; Klotzbücher et al., 2013). Similarly, an increase in aromaticity of soil DOM, but a decrease in C : N of DOM, was observed in snowmelt simulation performed over soil columns collected from both coniferous and deciduous sites (Campbell et al., 2014), and the aromatic content of O horizon DOM from different forest types in Sweden was found not to differ (Fröberg et al., 2011). Variations in aromaticity of O horizon DOM suggests variation in mineral stabilization potential of DOM in mineral soils. For instance, an input of aromatic compounds from O horizons to the mineral soil is more likely to result in formation of organo-mineral complexes that stabilize organic matter (OM) than an input of mobile, hydrophilic compounds such as carbohydrates (Guggenberger and Zech, 1993; Kaiser and Kalbitz, 2012). However, in situ, the consequences of these transformations are dependent on the rate and pathways of water and DOM composition and flow. Clarification is needed on the controls over both the rate and composition of mobilized DOM which could then inform potential mineral stabilization versus export potential of soil DOM from upland forests under varying hydrometeorological conditions.
The large- and small-scale effects of hydrometeorological conditions on DOM dynamics can be confounded by effects of disturbances, such as fire, insects and forest harvesting. Forest harvesting is a significant anthropogenic disturbance in boreal forests with known impacts on soil moisture and temperature during the growing season and increased export of water and DOM (Kreutzweiser et al., 2008). Mid- to long-term effects on soils in naturally regenerating forest are not well known but are likely significant in the situation when clear-cut, or otherwise disturbed, boreal black spruce forests remain open for long periods of time. As seasonal- and decadal-scale harvesting effects on DOM dynamics are both potentially significant but confounding, our objective with this study was to parse out the main effects of each. Mobilized soil DOM was sampled on a weekly to monthly basis over a year using passive pan lysimeters in open harvested plots compared to adjacent mature black spruce forest plots as part of a case study. Previously, we demonstrated that the thickness of the organic horizon was reduced by almost 50 % in the open harvested plots, that the quantity of DOC mobilized in the harvested plots was larger than in the forested plots, and that the relationship between water fluxes and mobilized DOC varied seasonally (Bowering et al., 2020). Here, we describe the temporal and spatial variability of DOM composition mobilized from soil organic horizons to better understand the controls of forest stand and soil structure relative to short-term hydrometeorological variability. Focusing on the hot spots and moments of boreal forest DOM mobilization likely sensitive to climate change, these results help identify the top-down controls on C and nutrient storage in boreal forest mineral soils and the potential fate of forest soil DOM exported to aquatic systems.
2.1 Site description
This study was conducted in an experimental forest at the Pynn's Brook Experimental Watershed Area (PBEWA) located near Deer Lake, western Newfoundland and Labrador, Canada (48∘53′14′′ N, 63∘24′8′′ W). The forest is mesic relative to other areas of the boreal ecozone, located in the Maritime Low Boreal Ecoclimate (Lbm) region of the Ecoregions Working Group (1989), and is dominated by black spruce (Picea mariana). The site is underlain by humo-ferric podzolic soils (Soils Classification Working Group, 1998) that have developed on fresh glacial, poorly sorted till deposits of granite, porphyry, sandstone and siltstone clasts (Batterson and Catto, 2001) with quartz most abundant, followed by plagioclase, muscovite, chlorite, K feldspar and biotite (Patrick et al., 2022). The surrounding boreal landscape is characterized by a mosaic of different age classes resulting from a history of periodic disturbance, including that from forest harvesting. The climate of the study region is characterized by winters with consistent snowpack for 4–5 months of the year from December to April. The area receives on average 1095 mm of precipitation annually, with approximately 40 % as snow, with a mean annual temperature of 3.6 ∘C (Environment Canada Climate Normals, Deer Lake Airport 1981–2010). During the study year total precipitation was 1402 mm with 516 mm (37 %) of that as snowfall water equivalent, and snowpack at the start of snowmelt period during the study ranged from 83 to 110 mm snowfall water equivalent across the study plots and was higher in the harvested plots (Bowering et al., 2020) similar to the long-term average in the region (Environment Canada Climate Normals, Deer Lake Airport 1981–2010). The site consists of 2 ha divided into eight 50×50 m plots (Fig. S1). Four of the plots were left unharvested and four were randomly selected for clear-cut harvesting. The four clear-cut plots were harvested on 7–10 July 2003 using a short-wood mechanical harvester, with minimal disturbance to the underlying soil and with any deciduous trees left standing. The close proximity of the plots enabled comparison of mature and harvested forest areas over a similar slope, aspect, elevation, and soil type and thus represents a case study of the harvesting effects within the context of seasonal variation. The two treatments of this case study will be referred to as harvested (H) and mature forest (F) throughout. In this study we used three plots of each treatment as indicated in Fig. S1.
Following common forestry practices for the area, the harvested plots were not replanted following clear cutting. Moss coverage persisted in the H plots where a larger proportion was Sphagnum sp. as compared with F plots where moss cover was dominated by Hylocomium splendens. The harvested plots also consisted of naturally regenerated herbs (including Cornus canadensis, Chamerion angustifolium and Vaccinium angustifolium) and shrubs (Alnus alnobetula) and few young conifers (ages 5–8 years; below 1.5 m in height) at much lower density than the adjacent mature stands (see Fig. S1 for aerial image taken a year after lysimeter installation). Average (± SD) organic horizon thickness was 8.2 ± 0.6 and 4.3 ± 0.6 cm, and C stocks were 2.4 ± 0.2 and 1.3 ± 0.3 kg C m−2 in the F and H plots, respectively (Bowering et al., 2020). Further information on site preparation and conditions can be found in Moroni et al. (2009), while further soil features (e.g., hydrologic properties) can be found in Bowering et al. (2020).
2.2 Sampling design, lysimeter installation and sample collection
Passive pan lysimeters were installed just underneath the organic horizon. Each lysimeter has a 0.12 m2 footprint and collects water percolating through the O horizon with a maximum solution collection capacity of 25 L. The lysimeters were designed using reported recommendations for achieving accurate volumetric measurements of soil leachate (Radulovich and Sollins, 1987; Titus et al., 2000). A detailed description of the lysimeter design can be found in Bowering et al. (2020), and an illustration of the design and installation of the lysimeters is provided in Fig. S2. Installation of lysimeters began in July 2012 and was completed the following spring in May 2013. The design includes the fixed effect of stand type (mature and harvested forest; F and H, respectively) at a scale above the individual lysimeters. Four lysimeters were installed in each of the three plots of each treatment for a total of 12 F plot lysimeters and 12 H plot lysimeters (Fig. S1). Collection began in July 2013, and sampling of all lysimeters in both treatments (n=24) was carried out on a weekly to monthly basis, with the exception of the winter season when only one collection was made, for a total of 27 sampling days within the study year. Lysimeter samples were stored in a cooler immediately following collection. Once transported back to the laboratory the pH of each sample was measured, and then samples were filtered using pre-combusted GF/F (0.45 µm pore size) Whatman filter paper, preserved with mercuric chloride within 24 h of collection and stored at 4 ∘C in the dark until analysis. This approach, capturing both vertical and lateral flow, previously revealed an effect of stand type on the mobilization of DOC with the H plots exhibiting a nearly 50 % increase in DOC mobilization relative to the F plots (Bowering et al., 2022).
2.3 Environmental monitoring
Three soil temperature and moisture probes per treatment (Decagon ECH2O-TM) were installed mid-organic horizon at approximately 5 cm depth, and two were installed in the mineral layer at approximately 15 cm depth. Soil moisture was measured as percent (%) of volumetric water content (VWC). One tipping bucket rain gauge (RST Instruments model TR-525) was installed in an open area on site to monitor local rain and air temperature. Data from this tipping bucket were compared with regional rainfall and air temperature (T) reported by Environment Canada at the Deer Lake Airport (49∘13′00′′ N, 57∘24′00′′ W) approximately 50 km away, and Deer Lake Airport data were a good predictor of the PBEWA rainfall and air T on a weekly basis (R2=0.882, p<0.0001). Regional data from the Deer Lake Airport were used to fill a gap in our on-site daily rainfall and mean daily air temperature data between 7 and 24 July 2013. Snowmelt water input was estimated using changes in snow depth between each lysimeter collection day measured near each lysimeter in both H and F. The average snow depth change by treatment was multiplied by an estimated maritime snow density of 0.343 g cm−3 (Sturm et al., 2010) to provide an estimated snowmelt water input value. Snowmelt water input estimates were combined with rain (in H) or throughfall (in F) where applicable to give a total water input to the O horizon over each collection period, which is different from the soil water fluxes independently measured by the lysimeters. Observations of significant lateral flow along the O-to-mineral-horizon interface have been previously reported (Bowering et al., 2020).
2.4 Chemical analysis and flux calculations
The DOC and TDN concentration of each lysimeter sample collected was measured using a high-temperature combustion analyzer (Shimadzu TOC-V and TN analyzer, Japan). Nitrate (NO; detection limit = 0.01 mg N L−1), ammonium (NH; detection limit = 0.004 mg N L−1) and phosphate (PO; detection limit = 0.01 mg P L−1) were measured using QuickChem Methods no. 10-107-04-1-B, 10-107-06-2-A and 10-115-01-1-A, respectively, using flow injection analysis (Lachat QuickChem 8500 Series 2, USA). No NO was detected using this colorimetric method. Total dissolved phosphorus (TDP; detection limit = 2.9 µg L−1), aluminum (Al; detection limit = 1.1 µg L−1) and iron (Fe; detection limit = 0.3 µg L−1) were measured using inductively coupled plasma optical emission spectroscopy (Perkin Elmer 5300 DV, USA). These measured concentrations along with the total volume collected by lysimeters, the number of collection days and the lysimeter collection area were used to calculate a flux (g solute m−2 d−1). Measures of Fe and Al and their ratio to DOC were included to track reactive metals relevant in these organic soils because of their role in formation of organo-mineral complexes (Torn et al., 1997) as well as their translocation into O horizons from the lower mineral horizon via fungal activity (Clarholm and Skyllberg, 2013).
2.5 Seasonal designations
Lysimeter collections were grouped into four distinct hydrological periods throughout the year described by observed soil moisture, precipitation and soil temperature patterns, together with water flux dynamics measured via the lysimeters as shown in Bowering et al. (2020). Briefly, summer is characterized by fluctuations in soil drying and rewetting, as well as frequent periods without any O horizon water fluxes. The transition to autumn is described by more consistent soil moisture and frequent precipitation events that resulted in frequent soil water fluxes as temperatures dropped. Winter was characterized by a consistent snowpack that insulated the soil, where soil temperatures were maintained above 0 ∘C despite sub-zero atmospheric temperatures. A very short-term melting event resulted in only a small delivery of water to the soil and, therefore, only small cumulative water flux throughout the whole winter. The snowmelt period is characterized by rapid water input to soil, wet soils and increasing soil temperatures.
2.6 Absorbance properties
For optical property measures, a subset of lysimeter samples from F and H treatments was selected to broadly represent the four seasons (Table 5). Each sample was diluted to approximately 15 mg DOC L−1 for absorbance measurements. An absorbance scan from 200–800 nm was performed on each sample in a 1 cm cuvette using a Perkin Elmer Lambda UV–Vis spectrophotometer following a blank consisting of Nano UV water (Barnsted Inc). Specific UV absorbance was calculated using the sample absorbance at 254 nm normalized to DOC concentration (SUVA254 nm). Spectral slopes of the 275–295 nm low-molecular-weight (LMW) region and 350–400 nm high-molecular-weight (HMW) region were calculated from each absorbance spectra based on Helms et al. (2008), and a slope ratio (SR) indicative of the LMW : HMW of CDOM was used to describe changes in relative molecular weight of CDOM. The absorbance spectra were corrected for potential Fe(III) interference, using correction factors based on Poulin et al. (2014) but derived for these specific sample types. Although the specific speciation of Fe was not measured, a 100 % Fe (III) was assumed to facilitate an estimate of the highest possible interference given the oxic nature of samples when analyzed in the laboratory. A negligible effect of Fe on the absorbance measurement was observed for these samples (Fe represented 0.4 %–0.6 % of total sample absorbance per collection date). Seasonally representative absorbance properties (SUVA254 nm, SS275−295, SS350−400 and SR), the C : N of DOM, and DOC : Fe were included in a principal component analysis to explore the predominant variables contributing to the effect of treatment relative to season.
2.7 Statistical analysis
Repeated-measures linear mixed effects models (RM-LMMs) were used to assess the effects of collection day and the interaction between sampling date and treatment on the fluxes and concentrations using the “nlme” package (Pinheiro et al., 2022). Post hoc Tukey tests using the “lsmeans” package (Lenth, 2016) were used to determine significant differences between H and F treatments on individual collection days. Further RM-LMMs were used to assess the effects of season and treatment on DOM fluxes and to assess the effects of collection day and treatment on absorbance properties, metals and their ratio to DOC. Diagnostic autocorrelation plots were generated using the ACF function in R (Mangiafico, 2016) to assess autocorrelation associated with lysimeter measurements. These plots demonstrate that each lag point is smaller than the proceeding lag point signifying a lack of autocorrelation. One-way ANOVAs were used to determine differences in total annual fluxes and mean concentrations between H and F treatments over the entire study period. Annual fluxes by treatment are shown in boxplots. Correlation testing was used to examine the association between weekly to monthly lysimeter-captured fluxes and concentrations and environmental predictor variables: mean soil temperature, mean soil moisture and total water input. Multiple regressions were not used due to the multi-collinearity of the predictor variables, which affected the estimated regression parameters (Quinn and Keough, 2002). Individual Pearson correlations, however, were used here to evaluate the degree of association between variables within the dataset. A Bonferroni correction was applied in the evaluation of these correlations to reduce the type I error. A principal component analysis was performed using the ggfortify (Tang et al., 2016) and factoextra (Kassambara and Mundt, 2017). All statistical analyses were performed using RStudio version 1.0.136.
3.1 Annual fluxes and concentrations in harvested and forest treatments
Total annual flux of TDN and DON was largest from the organic (O) horizons of the H compared to F treatment (Fig. 1d, e and Table S1), consistent with DOC, and total water (soil solution) fluxes previously reported (Bowering et al., 2020). In both treatments DON comprised approximately 85 % of the annually mobilized total dissolved nitrogen (TDN) flux. Ammonium (NH) was the predominant form of inorganic nitrogen (N), with no detectable nitrate. The annual NH flux and the annual average C : N of DOM were not different between H and F treatments. However, the C : N of DOM in the H treatment was higher than the C : N of the O horizon soil from which it was derived, while the C : N of DOM in the F treatment was similar to the C : N of the O horizon soil (Fig. 2b, c). Despite treatment differences in annual fluxes of TDN and DON (Fig. 1 and Table S1), the average annual concentrations of all solutes did not differ between treatments (Table S2).
Table 1Repeated-measures linear mixed effects model results assessing the effect of collection day and the interaction with treatment on mobilized soil concentrations of total dissolved nitrogen (TDN), ammonium (NH), dissolved organic nitrogen (DON), and orthophosphate (PO), as well as the ratios between NH and TDN and between dissolved organic carbon (DOC) and DON (DOC : DON) collected from plots located within the Pynn's Brook Experimental Forest in western Newfoundland, Canada.
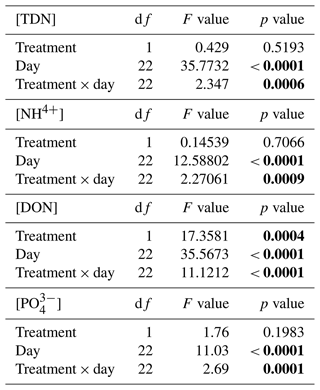
3.2 Intra-annual fluxes and concentrations in harvested and forest treatments
The intra-annual fluxes and concentrations of PO, TDN, NH, and DON as well as NH : TDN and C : N of DOM were variable on a weekly to monthly basis (Figs. 1 and 2), indicated by the significant effect of collection day (p<0.001; Tables 1, S3). There was also an interactive effect of treatment and day on all concentrations and fluxes (Table 1, Figs. 1 and 2) though the effect of day exhibited p values much lower than the interaction in the case of TDN fluxes, DON fluxes and C : N of DOM. An effect of treatment was detected for DON fluxes only (p = 0.0208) with H often exceeding F treatment fluxes. All concentrations were positively correlated to soil temperature (Table 2a), except for DON in the F treatment, and all concentrations were negatively correlated with water input (Table 2c), except for PO in both treatments and NH in H. All fluxes were positively correlated to the water input into the soil (Table 2c), except NH and PO in H. No relationship was observed between concentrations and fluxes with soil moisture, except the negative correlation with DOC concentration in the H treatment (Table 2b). Soil moisture was negatively correlated with the C : N of DOM in both treatments.
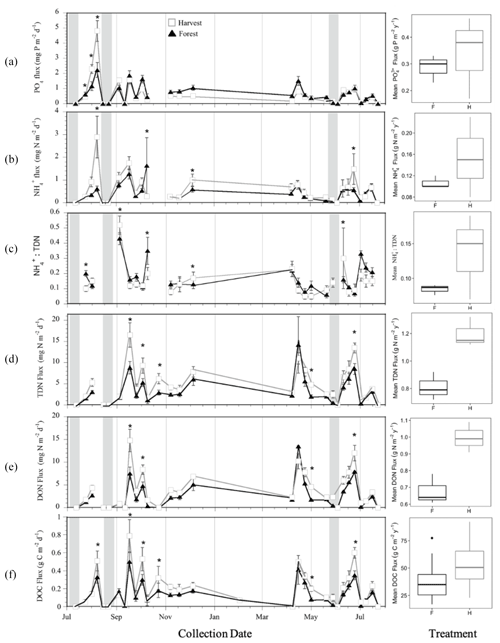
Figure 1Intra-annual variation of lysimeter-captured (a) phosphate (PO) fluxes, (b) ammonium (NH) fluxes, and (c) total dissolved nitrogen (TDN) fluxes; (d) the ammonium-to-total-dissolved-nitrogen ratio (NH TDN); and (e) dissolved organic nitrogen (DON) from soil organic horizons in mature forest (F) and harvested (H) treatment plots located within the Pynn's Brook Experimental Forest in western Newfoundland, Canada. Values are means of 12 lysimeters per treatment. Asterisks indicate significant differences between treatments determined by repeated-measures two-way ANOVA and post hoc least-square means tests, alpha = 0.05. Grey bars indicate soil drying periods characterized by 10 or more consecutive days receiving less than 10 mm of rainfall. Boxplots show the median (line inside box), upper and lower quartiles (box top and bottom, respectively), and minimum and maximum values (error bars) associated with plot-scale annual means (n=3 per plot type; a–e).
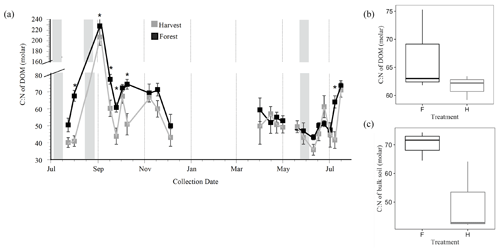
Figure 2Intra-annual variation of the dissolved organic carbon (DOC) to dissolved organic nitrogen (DON) ratio collected by lysimeters (a) located in plots within the Pynn's Brook Experimental Forest in western Newfoundland, Canada. Each point is a mean of 12 lysimeters per treatment per collection day. Grey bars designate periods of 10 consecutive days receiving less than 10 mm d−1 of precipitation. Breaks in the line graphs between points indicate periods of time when a sampling attempt was made but no water was captured by the lysimeters, indicating a zero flux. Asterisks indicate collection days where significant differences between treatments occurred as determined by a repeated-measures ANOVA and post hoc least-square means test, alpha = 0.05. Boxplot of C : N of DOM in harvest (H) and forest (F) treatments (b) compared to organic horizon C : N in H and F (c). Boxplots show the median (line inside box), upper and lower quartiles (box top and bottom, respectively), and minimum and maximum values (error bars) associated with plot-scale annual means (n=3 per plot type).
Table 2Pearson correlations between lysimeter-captured dissolved organic matter (concentrations, ratios and fluxes) and environmental variables (soil temperature, moisture and water input) within mature forest (F) and harvested (H) treatments plots located within the Pynn's Brook Experimental Forest in western Newfoundland, Canada. Dissolved organic carbon (DOC), total dissolved nitrogen (TDN), dissolved organic nitrogen (DON), ammonium (NH) and orthophosphate (PO). Bold font highlights significant correlations. A Bonferroni correction was applied to account for type I error (alpha = 0.05/3 = 0.017).
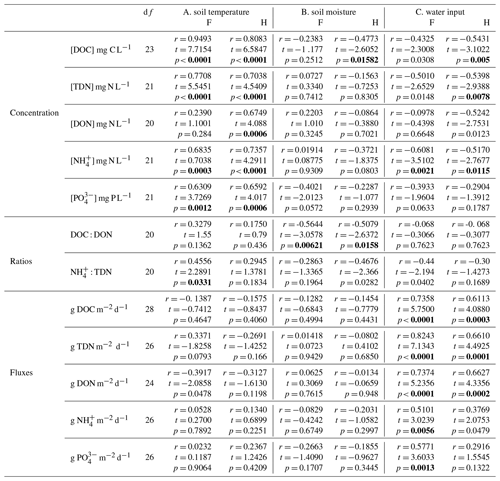
3.3 Seasonal fluxes and concentrations in harvested and forest treatments
An effect of season (p<0.0001) and treatment (p = 0.0358) was observed on total soil water fluxes with no interactive effect (Table 3). Soil water fluxes were always greater through the O horizons of H treatment compared to F, and the four seasonal periods (summer, autumn, winter and snowmelt/spring; see “Seasonal designations” in methods) exhibited four different cumulative water fluxes (Fig. 3a). The largest cumulative water fluxes in H and F treatments occurred over the snowmelt period, and the smallest water fluxes occurred during the winter when a consistent snowpack resulted in very low inputs of water to the soil. The second largest flux of water occurred during autumn, the only seasonal period when water fluxes were significantly different between treatments. A relatively small cumulative water flux occurred during the summer period, though still larger than the overwinter flux. All DOM fluxes exhibited an effect of season (Table 3; p<0.0001), but an effect of treatment was only observed for the DON flux (p = 0.0167). No interactive effect of season and treatment on DOM fluxes was observed.
The largest total flux of DOC (Fig. 3b) occurred during the autumn, and intermediate fluxes of DOC occurred in the summer and during snowmelt, which were not significantly different. The smallest total flux of DOC occurred during the winter. The largest total fluxes of DON occurred during both autumn and snowmelt periods. An intermediate flux of DON occurred during the summer, and the smallest flux occurred during the winter (Fig. 3c). The relative seasonal DOC and DON patterns described above resulted in a C : N of DOM that was highest in the summer, decreased in autumn, and was lowest during winter and snowmelt (Fig. 3d). The variation in values for the water, DOC and DON flux is likely a result of variation in litterfall and throughfall across the plots overall and observed through the individual lysimeters (Bowering et al., 2020).
Table 3Repeated-measures linear mixed effects model results assessing the effect of treatment, season, and their interaction on the total O horizon flux of water, dissolved organic carbon (DOC), dissolved organic nitrogen (DON), and the ratio between DOC and DON determined from plots located within the Pynn's Brook Experimental Forest in western Newfoundland, Canada. Seasonal variations of water flux, DOC flux, DON flux and DOC : DON are shown in Fig. 3.
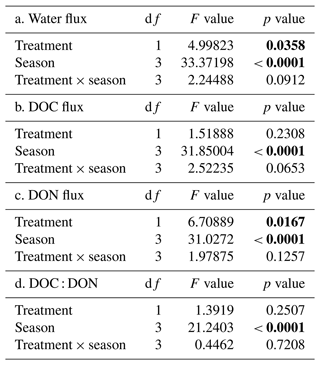
There was an effect of collection date on all absorbance properties (Table 4a–d; p<0.0001) and an effect of treatment on SUVA254 nm only where values were often higher in the H treatment (p = 0.0033). An interactive effect was observed for SS275−295 nm (p = 0.001) and SS350−395 nm (p = 0.0045). An effect of collection date on both Fe and Al (Table 4e, f; p<0.0001) was also observed, but only Fe exhibited a treatment effect (p = 0.0332) with higher concentrations in the H treatment. There was an effect of collection day on DOC : Fe (Table 4g; p<0.0001) and no effect of harvesting, while only a harvesting effect was observed with DOC : Al, with higher values observed in the F relative to H treatment (Table 4h; p=0.0247). The elevated values and variability in DOC : Fe and DOC : Al as well as the lack of a collection date effect for DOC : Al indicate little evidence for relevant seasonal controls on proportions of these reactive metals that might impact the fate of organic horizon DOC. The optical properties of CDOM in snow, collected as a bulk snow core of the entire profile just prior to snowmelt, contrasted with that of the lysimeter samples (Table 5). The snow SUVA254 nm values were lower than all lysimeter samples, and the LMW spectral slope (SS275−295 nm) was higher than lysimeter samples collected during snowmelt. The HMW spectral slope (SS350−395 nm) of snow was higher in F than H treatments and was higher in F snow samples than F lysimeter samples. The large differences in HMW spectral slope between treatments, compared to the LMW spectral slope, resulted in an elevated SR value for the H (2.60) in comparison to the F (0.69) treatment snow. This is likely attributable to greater litterfall sources of DOM in the F treatment plots given the greater needle litterfall on the snow surface and higher input of DOC from snow in the F relative to H plots (2.1 versus 1.3 g DOC m−2 yr−1 for F and H plots, respectively; Bowering et al., 2020).
Table 4Repeated-measures linear mixed effects model results assessing the effect of treatment, collection day, and their interaction on specific UV absorbance 254 nm (SUVA), low-molecular-weight spectral slope (LMW), high-molecular-weight spectral slope (HMW), iron concentrations ([Fe]), aluminum concentrations ([Al]), the ratio of dissolved organic carbon to iron (DOC : Fe) and the ratio of dissolved organic carbon to aluminum (DOC : Al) of mobilized soil dissolved organic matter collected from lysimeters across plots located within the Pynn's Brook Experimental Forest in western Newfoundland, Canada.
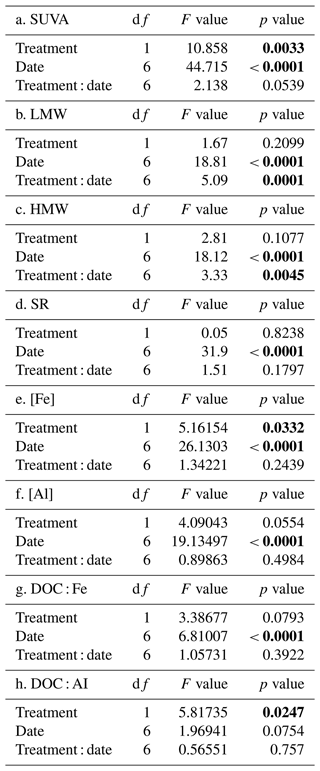
Table 5Optical properties and dissolved organic matter–metal associations within the Pynn's Brook Experimental Forest in western Newfoundland, Canada. Specific UV absorbance measured at 254 nm (SUVA). The slope ratio (SR) is the spectral slope at 275–295 nm divided by the spectral slope at 350–400 nm, pH, and Fe and Al concentration in mature forest (F) and harvested (H) treatments. Bolded values show significant treatment differences on certain sampling dates. Values with the same letter within each analysis type column are not significantly different. Standard deviations of the mean of 12 lysimeters per treatment shown in brackets. ND: no data.
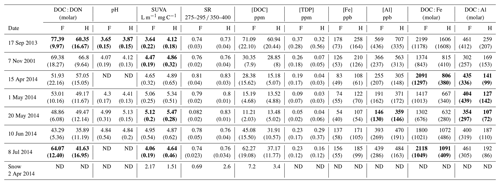
A principal component analysis (PCA) including absorbance properties (SUVA254 nm, SS275−295 nm, SS350−395 nm, and SR) as well as the C : N of DOM and DOC : Fe grouped by treatment (Fig. 4a) and season (Fig. 4b) demonstrated the overriding effect of season compared to harvesting. PC1 and PC2 describe 41 % and 26.6 % of the dataset variability, respectively. Seasonally, autumn soil DOM is characterized by HMW CDOM, signified by higher SS350−395 nm and lower SR, as well as higher C : N of DOM, while winter and snowmelt samples are characterized by LMW CDOM signified by higher SR and to a lesser extent higher SUVA254 nm and lower C : N. The samples from the H and F plots were weakly separated by SUVA254 nm and DOC : Fe, with higher values of both in the H treatment.
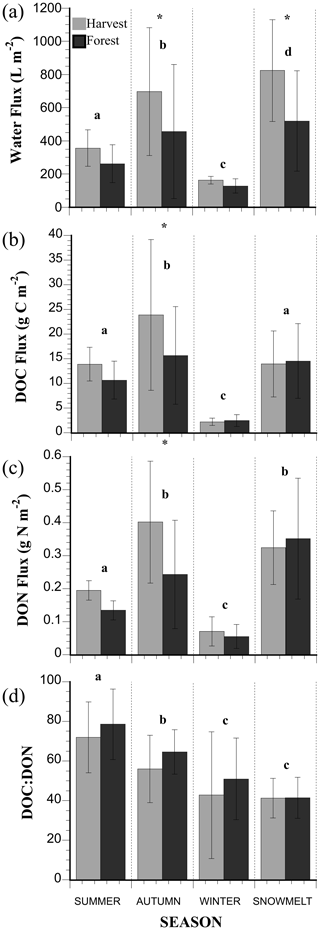
Figure 3Total seasonal fluxes of water, dissolved organic carbon (DOC), dissolved organic nitrogen (DON), and the C : N of DOM in the mature forest and harvested treatment plots located within the Pynn's Brook Experimental Forest in western Newfoundland, Canada. Seasonal designations are described in the methods section. Seasonal periods sharing the same letter are not significantly different. Asterisks indicate significant differences between treatments. Error bars show the standard deviation of 12 lysimeters per treatment per season.
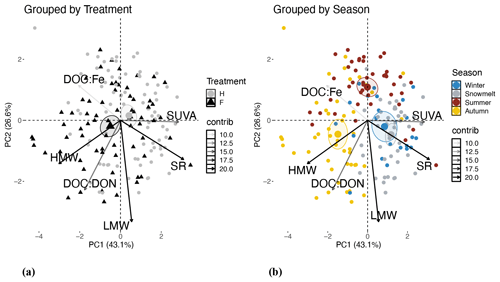
Figure 4Principal component analysis biplots used to explore the predominant variables describing the harvesting effect compared to the seasonal effect on the composition of mobilized dissolved organic matter (DOM) within the Pynn's Brook Experimental Forest in western Newfoundland, Canada. Variables included are the dissolved organic carbon (DOC)-to-iron ratio (DOC : Fe), the spectral slope for 350 to 395 nm (SS350−395 nm) indicative of high-molecular-weight (HMW) DOM, the spectral slope for 275 to 295 nm (SS275−295 nm) indicative of low-molecular-weight (LMW) DOM, the spectral slope ratio (SR), specific ultraviolet absorbance at 254 nm (SUVA) and the C : N of DOM (DOC : DON). Treatments shown in panel (a) include samples taken from the forest (F) and harvest (H) plots. Seasons shown in panel (b) include samples taken from winter, snowmelt, summer and autumn. Vectors are shaded according to their combined percent contribution (contrib) to PCA1 and PCA2. Ellipses represent the 95 % confidence interval around each group mean.
This study provides evidence for a strong control of season on the chemical composition of dissolved organic matter (DOM) mobilized from boreal forest organic (O) horizons that supersedes controls attributed to the decadal timescale effect of forest harvesting. Clear-cut harvesting immediately reduces the interception of water through the removal of trees but also through the longer-term reduction of the O horizon thickness and associated moss layer. Similar to DOC fluxes (Bowering et al., 2020), clear cutting increased the mobilization of DON on weekly to annual timescales. The relative temporal patterns of DOM composition, however, were similar in the F (mature forest) and H (harvested) treatments, supporting other works which describe a dominating control of environmental factors compared to soil composition on DOM composition (Cronan and Aiken, 1985; Kaiser et al., 2001; Fröberg et al., 2011). The compositional patterns observed in this study are indicative of DOM from fresh plant and microbial origin in the summer through autumn, as well as a shift to DOM from microbial biomass and microbially processed materials underneath a consistent snowpack in winter and during snowmelt. These seasonal shifts highlight a potential sensitivity of DOM composition to the changing climate, particularly in northern forests experiencing snowpack reductions and an increased autumn–winter wet period. The more dynamic snowpacks (those experiencing melt) associated with warmer winters can increase winter soil water fluxes and may consequently drive the mobilization of less processed soil DOM (i.e., higher HMW and C : N) during winter. This hypothesized soil response to increasingly warmer winters in this region is consistent with observed increases in river DOM export attributed to enhanced wintertime exports from a large catchment in a similar landscape further south (Huntington et al., 2016). The temporal and compositional changes observed here have implications for the ultimate lability, physiochemical and biological, and fate of soil DOM within northern forests and once exported to aquatic systems.
4.1 Summer soil DOM reflects decomposition of plant products and N mineralization
The high C : N and HMW DOM mobilized in summer is explained by the dominance of the decomposition of fresh plant litter releasing water-soluble organic C relative to organic N. Summer in this forested landscape is a period of relatively lower precipitation, high soil temperature and multi-day periods of soil drying followed by rewetting. Decomposition of litter resulting in the release of soluble materials at high soil temperatures (when moisture is not limiting) results in the release of soil C and uptake or immobilization of N (Kirschbaum, 1995; Conant et al., 2011; Hilli et al., 2008). While greater proportions of soil C are mineralized and released as CO2 during this period, a byproduct of greater microbial activity is greater production of soluble C, resulting in the high DOC concentrations and low pH often observed at high soil temperatures in laboratory extractions (Moore et al., 2008; Lee et al., 2018) and in situ (Kalbitz et al., 2007; Bowering et al., 2020). Additional concurrent processes known to affect DOM production at high seasonal temperatures are soil drying and rewetting cycles (Fierer and Schimel, 2002) and rhizodeposition (Weintraub et al., 2006; Heijden et al., 2008). These processes could contribute to mobilized DOC in summer, although the later would contribute LMW DOM instead of the HMW DOM observed (Giesler et al., 2007).
While the above processes result in an increase in DOC in summer, a number of other concurrent processes result in the transformation and uptake of dissolved ON. Higher rates of N mineralization likely contributed to the larger ratio of dissolved inorganic N (DIN) relative to total dissolved N (TDN) observed (Fig. 1), highlighting the likelihood of greater ON processing during summer. No detectable nitrate in soil leachates along with low pH of soil solution suggests that nitrification in this system is limited (Ste-Marie and Paré, 1999). In addition, direct uptake of DON by vegetation during the growing season is possible in northern latitudes that are N deficient (Neff et al., 2003; Schimel and Bennett, 2008; Näsholm et al., 1998), with plants and microbes competing for LMW DON, such as amino acids and peptides (Farrell et al., 2014). Combined, these processes limit the amount of ON available for mobilization during summer and contribute to the elevated C : N of the DOM observed during the summer period.
4.2 Autumn soil DOM indicates a progressive reduction in soluble C but maintenance of organic N
Following the relatively warm, dry summer period, the reduced temperature combined with increased plant inputs and decreased plant N demands in autumn lead to shifts in composition of mobilized DOM. Autumn, defined here as the period of continuous leaching of soil, constant soil moisture and decreasing soil temperatures, resulted in initially high C : N of DOM that decreased over the season. The sudden decrease in C : N of DOM observed at late autumn (Fig. 2) suggests that the O horizon had been leached of much of the soluble organic C, while the available soluble organic N was maintained. Decomposition of litter and soil during summer in boreal coniferous forests is dominated by fungi, whose activity relies on seasonally dependent rhizodeposition (Žifčáková et al., 2017). Two important C inputs associated with photosynthesis are therefore likely reduced in this system in late autumn: that from rhizodeposition and that from rhizo-dependent fungal decomposition of litter. In contrast to organic C trends, continued rapid cycling of organic N has been observed in northern black spruce forests of Alaska, even at low soil temperatures (Kielland et al., 2007), suggesting that continued breakdown of proteins replenishes the soluble ON pool during autumn. Furthermore, if DON uptake by plants is a relevant mechanism in this system, as is true in other northern systems (Schimel and Bennett, 2008), the demand for ON would decrease as plant activity slows in late autumn, reducing competition between the plant and microbial community for ON. This, in addition to decreasing rates of N mineralization with decreasing soil temperature contributes to the maintenance of the soluble ON pool compared to a decreasing soluble organic carbon (OC) pool during the wet fall-to-winter transition.
4.3 Winter and snowmelt soil DOM reflect soil microbial contributions underneath the snowpack
Fluxes of low C : N, LMW DOM occurring during winter and the following snowmelt period were likely the result of reduced plant inputs and maintenance of soil microbial activity underneath the snowpack. The winter period in this study year was characterized by a thick, consistent snowpack that maintained constant soil temperatures at 2 ∘C in both treatments. The snowpack developed before decreasing ambient temperatures could freeze the soil, allowing conditions for significant microbial activity underneath a consistently deep snowpack (> 40 cm; Brooks et al., 2011). Soils under shallower snowpacks are more vulnerable to freeze–thaw events, resulting in fluctuations in microbial biomass through winter and periodic release of labile C (Schimel and Clein, 1996; Patel et al., 2018), such as carbohydrates and amino sugars (Kaiser et al., 2001). This can have significant impacts on growing season soil and stream DOC (Haei et al., 2010) and DON (Groffman et al., 2018) concentrations. In the absence of freeze–thaw cycles, cell lysis events may not be a significant mechanism of DOM release. Instead, microbial activity and decomposition of soil organic matter underneath the snowpack is likely the dominant source of DOM production over the duration of the winter. The low C : N is indicative of a greater microbial contribution to the DOM during this period. Interestingly, the winter and snowmelt samples exhibited high SUVA254 nm coupled with elevated SR values, suggesting the mobilization of relatively more aromatic-rich DOM but with a lower molecular weight in comparison to soil DOM from summer and autumn. SUVA254 nm and SR are typically negatively correlated in surface water indicative of HMW aromatic DOM (Helms et al., 2008). These results support the occurrence of microbial degradation of soil organic matter in the absence of fresh litter inputs underneath the snowpack, increasing the solubility of aromatic compounds such as lignin in soil (Malcolm, 1990; Hansson et al., 2010; Klotzbücher et al., 2013), enhancing aromaticity and reducing the relative molecular weight of the CDOM. Congruent with this, the lower DOC : DON is attributable to microbial metabolites or proteinaceous products released following this long period of degradation in absence of fresh plant inputs as observed in soil incubations (Kalbitz, 2003). These observations are also consistent with the initial steps in the synthesis of CDOM or humic substances; the fungal breakdown of lignin–cellulose as part of the polyphenol theory of humic substance formation processes (Stevenson, 1994) is also observed in organic-rich peat (Prijac et al., 2022).
4.4 The seasonal variability of soil DOM composition has implications for its fate in a changing climate
This study demonstrates that the composition of mobilized soil DOM is similarly variable between sites of contrasting forest stand and soil properties. Clear-cut harvesting causes changes to forest water balance, through immediate removal of the canopy and longer-term reduction of the O horizon thickness, and results in larger quantities of mobilized DOM on decadal timescales. Despite this, the response of soil DOM composition to season suggests that the mobilization of soluble materials in the two treatments is controlled by similar responses to environmental conditions. Optical properties and the C : N of DOM during summer and autumn, compared to winter and snowmelt, were reflective of shifts from fresh-plant-derived to microbial-derived DOM. This compositional shift is especially noteworthy because temperature change at high latitudes is expected to be more pronounced in the winter, with repercussions on snowpack formation and duration (Mellander et al., 2007; Laudon et al., 2013). Winter temperatures in this northern maritime climate are often near 0 ∘C, and the projected 7 ∘C increase in mean winter temperature (Finnis and Daraio, 2018) is likely to cause increases in winter rain and melt events, soil water fluxes, and, consequently, mobilization of less processed soil DOM. The delivery of less processed DOM, exhibiting a higher-MW CDOM fraction, could result in increased mineral soil adsorption (Guggenberger and Zech, 2002; Kaiser and Guggenberger, 2000; Lilienfein et al., 2004; Kothawala et al., 2008) dependent on the texture and mineralogy of the underlying soil and including the pathway and resident time of water (e.g., Oades, 1988; Marschner and Kalbitz, 2003; Patrick et al., 2022; Slessarev et al., 2022).
Research conducted in these same and similar forest sites underlain by podzolic soils indicates adsorption of DOM within shallow mineral soils is highly dynamic and dependent upon seasonal conditions (e.g., solution pH), as well as reactive Al availability and its saturation with C. The siliciclastic sedimentary till in the study area provides an ample source of reactive Fe and Al to support the generation of organo-mineral complexes via co-precipitation of DOM and these metals as well as adsorption onto existing complexes (Patrick et al., 2022). In some hillslope locations of this study site, deeper mineral horizons have a large potential for DOM adsorption dependent on infiltration depths that may occur with the enhanced fall and winter rainfall expected in this region (Patrick et al., unpublished data). Therefore, the differences in DOM composition between fall and spring snowmelt further support the potential for enhanced formation of these complexes with the expected decreases in spring snowmelt and increasing autumn and winter rainfall and infiltration.
Warmer winters are linked to enhanced catchment export of DOM in an area just south of our study region (Huntington et al., 2016), consistent with regional evidence for enhanced soil DOM mobilization with warming winters (Bowering et al., 2022), and this suggests a shift to increased export of fresher terrestrial DOM. In the freshwater environment fresher terrestrial DOM, characterized by an increased high-MW fraction, degrades more readily relative to low-MW, N-rich DOM (Kellerman et al., 2015; Köhler et al., 2013; Kothawala et al., 2014). However, fresher terrestrial DOM also contributes more to sediment burial in high-latitude lakes through light- and microbial-mediated flocculation (Wachenfeldt et al., 2008, 2009) and can have a greater potential to remain buried longer relative to more microbially derived OM (Gudasz et al., 2012). Enhanced export of more labile, higher-MW DOM coupled with enhanced water input, and thus lower water residence times in the aquatic environment such as in this study region, suggests increases in a high-MW DOM fraction throughout the aquatic environment (Weyhenmeyer et al., 2014) and potential for lake burial or export to the marine environment opposite of those regions experiencing decreases in precipitation (Catalán et al., 2016). Therefore, the ultimate impact of these shifts in forest soil DOM composition on the C balance of these landscapes depends on both the connectivity with and properties of deep mineral soils as well as the net effect on downstream aquatic processes of burial and metabolism.
Future reductions in snowpack depth and duration as a result of increasing air temperature has the capacity to disrupt an important period of soil organic matter processing by microbes with repercussions on the composition of mobilized DOM; however, these effects are dependent on the type of snowpack change (Stark et al., 2020). In this wet eastern boreal region, snowpacks are deep (> 80 cm maximum snow depth) and are likely to change with warmer winters, increases in rain-on-snow events, and ice incasement, with soil freezing being a less significant concern in comparison to forests with shallower snowpacks. Future work capturing variable snowpack years (either within or across sites) and impacts on landscape and small-catchment hydrology is needed. This would help clarify the relative importance of these changes for the chemical character of soil organic matter and mobilized soil DOM and inform their implications for the fate of DOM within deeper mineral soils and the aquatic environment. Such efforts would improve our understanding of the response of soil organic matter to a rapidly changing climate and our ability to manage forest C balance in boreal landscapes.
All data associated with this study can be found at Zenodo (https://doi.org/10.5281/zenodo.8019215, Bowering et al., 2023).
The supplement related to this article is available online at: https://doi.org/10.5194/bg-20-2189-2023-supplement.
KAE and SEZ designed the study with input from KLB. KLB and KAE designed the lysimeters and planned their installation as well as the installation of all environmental monitoring equipment. KLB collected and analyzed the lysimeter, environmental monitoring and soil properties data. KLB prepared the paper with significant input from SEZ and editing from KAE and SEZ.
The contact author has declared that none of the authors has any competing interests.
Publisher’s note: Copernicus Publications remains neutral with regard to jurisdictional claims in published maps and institutional affiliations.
We extend special thanks for field assistance provided by individuals at the Atlantic Forestry Centre (Corner Brook) of Natural Resources Canada – Andrea Skinner, Darrell Harris and Gordon Butt – and Memorial University, Grenfell campus – Sarah Thompson and Danny Pink – as well for laboratory assistance provided by Jamie Warren at Memorial University, St. John's campus.
This research has been supported by the Natural Sciences and Engineering Research Council of Canada (grant nos. SPG479224-15 and DG20181867), the Canada Research Chairs Program (grant no. 950-231764), and the Forest Service Branch, Department of Natural Resources, NL Government.
This paper was edited by Erika Buscardo and reviewed by two anonymous referees.
Averill, C. and Waring, B.: Nitrogen limitation of decomposition and decay: How can it occur?, Glob. Change Biol., 24, 1417–1427, https://doi.org/10.1111/gcb.13980, 2018.
Batterson, M. J. and Catto, N. R.: Topographically-controlled deglacial history of the Humber River basin, western Newfoundland, Geogr. Phys. Quatern., 55, 213–228, https://doi.org/10.7202/006851ar, 2001.
Berggren, M. and Giorgio, P. A.: Distinct patterns of microbial metabolism associated to riverine dissolved organic carbon of different source and quality, J. Geophys. Res.-Biogeo., 120, 989–999, https://doi.org/10.1002/2015jg002963, 2015.
Bowering, K. L., Edwards, K. A., Prestegaard, K., Zhu, X., and Ziegler, S. E.: Dissolved organic carbon mobilized from organic horizons of mature and harvested black spruce plots in a mesic boreal region, Biogeosciences, 17, 581–595, https://doi.org/10.5194/bg-17-581-2020, 2020.
Bowering, K. L., Edwards, K. A., and Ziegler, S. E.: Seasonal controls override forest harvesting effects on the composition of dissolved organic matter mobilized from boreal forest soil organic horizons (1.0), Zenodo [data set], https://doi.org/10.5281/zenodo.8019215, 2023.
Brooks, P. D., Grogan, P., Templer, P. H., Groffman, P., Öquist, M. G., and Schimel, J.: Carbon and Nitrogen Cycling in Snow-Covered Environments: Carbon and nitrogen cycling in snow-covered environments, Geogr. Compass, 5, 682–699, https://doi.org/10.1111/j.1749-8198.2011.00420.x, 2011.
Campbell, J. L., Reinmann, A. B., and Templer, P. H.: Soil Freezing Effects on Sources of Nitrogen and Carbon Leached During Snowmelt, Soil Sci. Soc. Am. J., 78, 297–308, https://doi.org/10.2136/sssaj2013.06.0218, 2014.
Casas-Ruiz, J. P., Bodmer, P., Bona, K. A., Butman, D., Couturier, M., Emilson, E. J. S., Finlay, K., Genet, H., Hayes, D., Karlsson, J., Paré, D., Peng, C., Striegl, R., Webb, J., Wei, X., Ziegler, S. E., and del Giorgio, P. A.: Integrating terrestrial and aquatic ecosystems to constrain estimates of land-atmosphere carbon exchange, Nat. Commun., 14, 1571, https://doi.org/10.1038/s41467-023-37232-2, 2023.
Catalán, N., Marcé, R., Kothawala, D. N., and Tranvik, Lars. J.: Organic carbon decomposition rates controlled by water retention time across inland waters, Nat. Geosci., 9, 501–504, https://doi.org/10.1038/ngeo2720, 2016.
Clarholm, M. and Skyllberg, U.: Translocation of metals by trees and fungi regulates pH, soil organic matter turnover and nitrogen availability in acidic forest soils, Soil Biol. Biochem., 63, 142–153, https://doi.org/10.1016/j.soilbio.2013.03.019, 2013.
Conant, R. T., Ryan, M. G., Ågren, G. I., Birge, H. E., Davidson, E. A., Eliasson, P. E., Evans, S. E., Frey, S. D., Giardina, C. P., Hopkins, F. M., Hyvönen, R., Kirschbaum, M. U. F., Lavallee, J. M., Leifeld, J., Parton, W. J., Steinweg, J. M., Wallenstein, M. D., Wetterstedt, J. Å. M., and Bradford, M. A.: Temperature and soil organic matter decomposition rates – synthesis of current knowledge and a way forward, Glob. Change Biol., 17, 3392–3404, https://doi.org/10.1111/j.1365-2486.2011.02496.x, 2011.
Cortina, J., Romanyà, J., and Vallejo, V. R.: Nitrogen and phosphorus leaching from the forest floor of a mature Pinus radiata stand, Geoderma, 66, 321–330, https://doi.org/10.1016/0016-7061(95)00006-a, 1995.
Cronan, C. S. and Aiken, G. R.: Chemistry and transport of soluble humic substances in forested watersheds of the Adirondack Park, New York, Geochim. Cosmochim. Ac., 49, 1697–1705, https://doi.org/10.1016/0016-7037(85)90140-1, 1985.
Ecoregions Working Group: Ecoclimate regions of Canada, Ecol. Land Class, Series No. 23, Environment Canada, Canadian Wildlife Service, Ottawa, ON, 118 pp., https://d1ied5g1xfgpx8.cloudfront.net/pdfs/23092.pdf (last access: 7 November 2020), 1989.
Farrell, M., Prendergast-Miller, M., Jones, D. L., Hill, P. W., and Condron, L. M.: Soil microbial organic nitrogen uptake is regulated by carbon availability, Soil Biol. Biochem., 77, 261–267, https://doi.org/10.1016/j.soilbio.2014.07.003, 2014.
Fierer, N. and Schimel, J. P.: Effects of drying–rewetting frequency on soil carbon and nitrogen transformations, Soil Biol. Biochem., 34, 777–787, https://doi.org/10.1016/s0038-0717(02)00007-x, 2002.
Fröberg, M., Hansson, K., Kleja, D. B., and Alavi, G.: Dissolved organic carbon and nitrogen leaching from Scots pine, Norway spruce and silver birch stands in southern Sweden, Forest Ecol. Manag., 262, 1742–1747, https://doi.org/10.1016/j.foreco.2011.07.033, 2011.
Giesler, R., Högberg, M. N., Strobel, B. W., Richter, A., Nordgren, A., and Högberg, P.: Production of dissolved organic carbon and low-molecular weight organic acids in soil solution driven by recent tree photosynthate, Biogeochemistry, 84, 1–12, https://doi.org/10.1007/s10533-007-9069-3, 2007.
Gödde, M., David, M. B., Christ, M. J., Kaupenjohann, M., and Vance, G. F.: Carbon mobilization from the forest floor under red spruce in the northeastern USA, Soil Biol. Biochem., 28, 1181–1189, https://doi.org/10.1016/0038-0717(96)00130-7, 1996.
Groffman, P. M., Driscoll, C. T., Durán, J., Campbell, J. L., Christenson, L. M., Fahey, T. J., Fisk, M. C., Fuss, C., Likens, G. E., Lovett, G., Rustad, L., and Templer, P. H.: Nitrogen oligotrophication in northern hardwood forests, Biogeochemistry, 141, 523–539, https://doi.org/10.1007/s10533-018-0445-y, 2018.
Gudasz, C., Bastviken, D., Premke, K., Steger, K., and Tranvik, L. J.: Constrained microbial processing of allochthonous organic carbon in boreal lake sediments, Limnol. Oceanogr., 57, 163–175, https://doi.org/10.4319/lo.2012.57.1.0163, 2012.
Guggenberger, G. and Zech, W.: Dissolved organic carbon control in acid forest soils of the Fichtelgebirge (Germany) as revealed by distribution patterns and structural composition analyses, Geoderma, 59, 109–129, https://doi.org/10.1016/0016-7061(93)90065-s, 1993.
Guggenberger, G. and Zech, W.: Composition and dynamics of dissolved carbohydrates and lignin-degradation products in two coniferous forests, N.E. Bavaria, Germany, Soil Biol. Biochem., 26, 19–27, https://doi.org/10.1016/0038-0717(94)90191-0, 2002.
Haei, M., Öquist, M. G., Ilstedt, U., and Laudon, H.: The influence of soil frost on the quality of dissolved organic carbon in a boreal forest soil: combining field and laboratory experiments, Biogeochemistry, 107, 95–106, https://doi.org/10.1007/s10533-010-9534-2, 2010.
Hansson, K., Kleja, D. B., Kalbitz, K., and Larsson, H.: Amounts of carbon mineralised and leached as DOC during decomposition of Norway spruce needles and fine roots, Soil Biol. Biochem., 42, 178–185, https://doi.org/10.1016/j.soilbio.2009.10.013, 2010.
Heijden, M. G. A. van der, Bardgett, R. D., and Straalen, N. M. van: The unseen majority: soil microbes as drivers of plant diversity and productivity in terrestrial ecosystems, Ecol. Lett., 11, 296–310, https://doi.org/10.1111/j.1461-0248.2007.01139.x, 2008.
Helms, J. R., Stubbins, A., Ritchie, J. D., Minor, E. C., Kieber, D. J., and Mopper, K.: Absorption spectral slopes and slope ratios as indicators of molecular weight, source, and photobleaching of chromophoric dissolved organic matter, Limnol. Oceanogr., 53, 955–969, 2008.
Hensgens, G., Laudon, H., Peichl, M., Gil, I. A., Zhou, Q., and Berggren, M.: The role of the understory in litter DOC and nutrient leaching in boreal forests, Biogeochemistry, 149, 87–103, https://doi.org/10.1007/s10533-020-00668-5, 2020.
Hilli, S., Stark, S., and Derome, J.: Carbon Quality and Stocks in Organic Horizons in Boreal Forest Soils, Ecosystems, 11, 270–282, https://doi.org/10.1007/s10021-007-9121-0, 2008.
Huntington, T. G., Balch, W. M., Aiken, G. R., Sheffield, J., Luo, L., Roesler, C. S., and Camill, P.: Climate change and dissolved organic carbon export to the Gulf of Maine, J. Geophys. Res.-Biogeo., 121, 2700–2716, https://doi.org/10.1002/2015jg003314, 2016.
Jaffé, R., McKnight, D., Maie, N., Cory, R., McDowell, W. H., and Campbell, J. L.: Spatial and temporal variations in DOM composition in ecosystems: The importance of long-term monitoring of optical properties, J. Geophys. Res., 113, JG000683, https://doi.org/10.1029/2008jg000683, 2008.
Jansen, B., Kalbitz, K., and McDOWELL, W. H.: Dissolved Organic Matter: Linking Soils and Aquatic Systems, Vadose Zone J., 13, 1–4, https://doi.org/10.2136/vzj2014.05.0051, 2014.
Jones, D. L. and Kielland, K.: Amino acid, peptide and protein mineralization dynamics in a taiga forest soil, Soil Biol. Biochem., 55, 60–69, https://doi.org/10.1016/j.soilbio.2012.06.005, 2012.
Kaiser, K. and Guggenberger, G.: The role of DOM sorption to mineral surfaces in the preservation of organic matter in soils, Org. Geochem., 31, 711–725, https://doi.org/10.1016/s0146-6380(00)00046-2, 2000.
Kaiser, K. and Kalbitz, K.: Cycling downwards – dissolved organic matter in soils, Soil Biol. Biochem., 52, 29–32, https://doi.org/10.1016/j.soilbio.2012.04.002, 2012.
Kaiser, K., Guggenberger, G., Haumaier, L., and Zech, W.: Seasonal variations in the chemical composition of dissolved organic matter in organic forest floor layer leachates of old-growth Scots pine (Pinus sylvestris L.) and European beech (Fagus sylvatica L.) stands in northeastern Bavaria, Germany, Biogeochemistry, 55, 103–143, https://doi.org/10.1023/a:1010694032121, 2001.
Kalbitz, K.: Changes in properties of soil-derived dissolved organic matter induced by biodegradation, Soil Biol. Biochem., 35, 1129–1142, https://doi.org/10.1016/s0038-0717(03)00165-2, 2003.
Kalbitz, K., Meyer, A., Yang, R., and Gerstberger, P.: Response of dissolved organic matter in the forest floor to long-term manipulation of litter and throughfall inputs, Biogeochemistry, 86, 301–318, https://doi.org/10.1007/s10533-007-9161-8, 2007.
Kassambara, A. and Mundt, F.: Package “factoextra”: Extract and Visualize the Results of Multivariate Data Analyses, https://cran.r-project.org/web/packages/factoextra/ (last access: 17 May 2022), 2017.
Kellerman, A. M., Kothawala, D. N., Dittmar, T., and Tranvik, L. J.: Persistence of dissolved organic matter in lakes related to its molecular characteristics, Nat. Geosci., 8, 454–457, https://doi.org/10.1038/ngeo2440, 2015.
Kielland, K., McFarland, J. W., Ruess, R. W., and Olson, K.: Rapid Cycling of Organic Nitrogen in Taiga Forest Ecosystems, Ecosystems, 10, 360–368, https://doi.org/10.1007/s10021-007-9037-8, 2007.
Kirschbaum, M.: The Temperature-Dependence of Soil Organic-Matter Decomposition, and the Effect of Global Warming on Soil Organic-C Storage, Soil Biol. Biochem., 27, 753–760, https://doi.org/10.1016/0038-0717(94)00242-s, 1995.
Klotzbücher, T., Kaiser, K., Filley, T. R., and Kalbitz, K.: Processes controlling the production of aromatic water-soluble organic matter during litter decomposition, Soil Biol. Biochem., 67, 133–139, https://doi.org/10.1016/j.soilbio.2013.08.003, 2013.
Köhler, S. J., Kothawala, D., Futter, M. N., Liungman, O., and Tranvik, L.: In-lake processes offset increased terrestrial inputs of dissolved organic carbon and color to lakes, PLoS ONE, 8, e70598, https://doi.org/10.1371/journal.pone.0070598, 2013.
Kothawala, D. N., Moore, T. R., and Hendershot, W. H.: Adsorption of dissolved organic carbon to mineral soils: A comparison of four isotherm approaches, Geoderma, 148, 43–50, https://doi.org/10.1016/j.geoderma.2008.09.004, 2008.
Kothawala, D. N., Stedmon, C. A., Müller, R. A., Weyhenmeyer, G. A., Köhler, S. J., and Tranvik, L. J.: Controls of dissolved organic matter quality: evidence from a large-scale boreal lake survey, Glob. Change Biol., 20, 1101–1114, https://doi.org/10.1111/gcb.12488, 2014.
Kreutzweiser, D. P., Hazlett, P. W., and Gunn, J. M.: Logging impacts on the biogeochemistry of boreal forest soils and nutrient export to aquatic systems: A review, Environ. Rev., 16, 157–179, https://doi.org/10.1139/a08-006, 2008.
Laudon, H., Tetzlaff, D., Soulsby, C., Carey, S., Seibert, J., Buttle, J., Shanley, J., McDonnell, J. J., and McGuire, K.: Change in winter climate will affect dissolved organic carbon and water fluxes in mid-to-high latitude catchments, Hydrol. Process., 27, 700–709, https://doi.org/10.1002/hyp.9686, 2013.
Lee, M.-H., Park, J.-H., and Matzner, E.: Sustained production of dissolved organic carbon and nitrogen in forest floors during continuous leaching, Geoderma, 310, 163–169, https://doi.org/10.1016/j.geoderma.2017.07.027, 2018.
Lenth, R. V.: Package “lsmeans”: Least-squares means, J. Stat. Softw., 69, 1–33, https://doi.org/10.18637/jss.v069.i01, 2016.
Lilienfein, J., Qualls, R. G., Uselman, S. M., and Bridgham, S. D.: Adsorption of dissolved organic carbon and nitrogen in soils of a weathering chronosequence, Soil Sci. Soc. Am. J., 68, 292–305, 2004.
Malcolm, R. L.: The uniqueness of humic substances in each of soil, stream and marine environments, Anal. Chim. Acta, 232, 19–30, https://doi.org/10.1016/s0003-2670(00)81222-2, 1990.
Mangiafico, S. S.: Summary and Analysis of Extension Program Evaluation in R, version 1.20.01, https://rcompanion.org/handbook/I_09.html (last access: 17 May 2022), 2016.
Marschner, B. and Kalbitz, K.: Controls of bioavailability and biodegradability of dissolved organic matter in soils, Geoderma, 113, 211–235, https://doi.org/10.1016/s0016-7061(02)00362-2, 2003.
McDowell, N. G., Bowling, D. R., Schauer, A., Irvine, J., Bond, B. J., Law, B. E., and Ehleringer, J. R.: Associations between carbon isotope ratios of ecosystem respiration, water availability and canopy conductance: carbon isotope ratio of ecosystem respiration, Glob. Change Biol., 10, 1767–1784, https://doi.org/10.1111/j.1365-2486.2004.00837.x, 2004.
McDowell, W. H. and Likens, G. E.: Origin, Composition, and Flux of Dissolved Organic Carbon in the Hubbard Brook Valley, Ecol. Monogr., 58, 177–195, https://doi.org/10.2307/2937024, 1988.
McGroddy, M. E., Baisden, W. T., and Hedin, L. O.: Stoichiometry of hydrological C, N, and P losses across climate and geology: An environmental matrix approach across New Zealand primary forests, Global Biogeochem. Cy., 22, GB003005, https://doi.org/10.1029/2007gb003005, 2008.
McKnight, D. M., Boyer, E. W., Westerhoff, P. K., Doran, P. T., Kulbe, T., and Andersen, D. T.: Spectrofluorometric characterization of dissolved organic matter for indication of precursor organic material and aromaticity, Limnol. Oceanogr., 46, 38–48, https://doi.org/10.4319/lo.2001.46.1.0038, 2001.
Mellander, P.-E., Löfvenius, M. O., and Laudon, H.: Climate change impact on snow and soil temperature in boreal Scots pine stands, Climatic Change, 85, 179–193, https://doi.org/10.1007/s10584-007-9254-3, 2007.
Michalzik, B. and Matzner, E.: Dynamics of dissolved organic nitrogen and carbon in a Central European Norway spruce ecosystem: Dynamics of dissolved organic nitrogen and carbon in soil, Eur. J. Soil Sci., 50, 579–590, https://doi.org/10.1046/j.1365-2389.1999.00267.x, 1999.
Michalzik, B., Kalbitz, K., Park, J. H., Solinger, S., and Matzner, E.: Fluxes and concentrations of dissolved organic carbon and nitrogen – a synthesis for temperate forests, Biogeochemistry, 52, 173–205, https://doi.org/10.1023/a:1006441620810, 2001.
Michel, K. and Matzner, E.: Release of dissolved organic carbon and nitrogen from forest floors in relation to solid phase properties, respiration and N-mineralization, J. Plant Nutr. Soil Sc., 162, 645–652, https://doi.org/10.1002/(sici)1522-2624(199912)162:6<645::aid-jpln645>3.0.co;2-t, 1999.
Moore, T. R., Paré, D., and Boutin, R.: Production of Dissolved Organic Carbon in Canadian Forest Soils, Ecosystems, 11, 740–751, https://doi.org/10.1007/s10021-008-9156-x, 2008.
Moroni, M. T., Carter, P. Q., and Ryan, D. A. J.: Harvesting and slash piling affect soil respiration, soil temperature, and soil moisture regimes in Newfoundland boreal forests, Can. J. Soil Sci., 89, 345–355, 2009.
Näsholm, T., Ekblad, A., NORDIN, A., Giesler, R., Högberg, M., and Högberg, P.: Boreal forest plants take up organic nitrogen, Nature, 392, 914–916, https://doi.org/10.1038/31921, 1998.
Neff, J. C., Chapin, F. S., and Vitousek, P. M.: Breaks in the cycle: dissolved organic nitrogen in terrestrial ecosystems, Front Ecol. Environ., 1, 205–211, https://doi.org/10.1890/1540-9295(2003)001[0205:bitcdo]2.0.co;2, 2003.
Oades, J. M.: The retention of organic matter in soils, Biogeochemistry, 5, 35–70, https://doi.org/10.1007/bf02180317, 1988.
Patel, K. F., Tatariw, C., MacRae, J. D., Ohno, T., Nelson, S. J., and Fernandez, I. J.: Soil carbon and nitrogen responses to snow removal and concrete frost in a northern coniferous forest, Can. J. Soil Sci., 98, 436–447, https://doi.org/10.1139/cjss-2017-0132, 2018.
Patrick, M. E., Young, C. T., Zimmerman, A. R., and Ziegler, S. E.: Mineralogic controls are harbingers of hydrological controls on soil organic matter content in warmer boreal forests, Geoderma, 425, 116059, https://doi.org/10.1016/j.geoderma.2022.116059, 2022.
Philben, M., Butler, S., Billings, S. A., Benner, R., Edwards, K. A., and Ziegler, S. E.: Biochemical and structural controls on the decomposition dynamics of boreal upland forest moss tissues, Biogeosciences, 15, 6731–6746, https://doi.org/10.5194/bg-15-6731-2018, 2018.
Pinheiro, J., Bates, D., and Team, R. C.: Package “nlme”: Linear and Nonlinear Mixed Effects Models, https://svn.r-project.org/R-packages/trunk/nlme/ (last access: May 17 2022), 2022.
Poulin, B. A., Ryan, J. N., and Aiken, G. R.: Effects of Iron on Optical Properties of Dissolved Organic Matter, Environ. Sci. Technol., 48, 10098–10106, https://doi.org/10.1021/es502670r, 2014.
Prijac, A., Gandois, L., Jeanneau, L., Taillardat, P., and Garneau, M.: Dissolved organic matter concentration and composition discontinuity at the peat–pool interface in a boreal peatland, Biogeosciences, 19, 4571–4588, https://doi.org/10.5194/bg-19-4571-2022, 2022.
Qualls, R. G. and Haines, B. L.: Geochemistry of Dissolved Organic Nutrients in Water Percolating through a Forest Ecosystem, Soil Sci. Soc. Am. J., 55, 1112–1123, https://doi.org/10.2136/sssaj1991.03615995005500040036x, 1991.
Quinn, G. P. and Keough, M. J.: Experimental Design and Data Analysis for Biologists, Cambridge University Press, Cambridge, UK, 553 pp., ISBN 9780521009768, https://doi.org/10.1017/cbo9780511806384, 2002.
Radulovich, R. and Sollins, P.: Improved Performance of Zero-Tension Lysimeters, Soil Sci. Soc. Am. J., 51, 1386–1388, https://doi.org/10.2136/sssaj1987.03615995005100050054x, 1987.
Roulet, N. and Moore, T. R.: Environmental chemistry: browning the waters, Nature, 444, 283–284, https://doi.org/10.1038/444283a, 2006.
Schimel, J. P. and Bennett, J.: Nitrogen mineralization: challenges of a changing paradigm, Ecology, 85, 591–602, https://doi.org/10.1890/03-8002, 2008.
Schimel, J. P. and Clein, J. S.: Microbial response to freeze-thaw cycles in tundra and taiga soils, Soil Biol. Biochem., 28, 1061–1066, https://doi.org/10.1016/0038-0717(96)00083-1, 1996.
Shen, Y., Chapelle, F. H., Strom, E. W., and Benner, R.: Origins and bioavailability of dissolved organic matter in groundwater, Biogeochemistry, 122, 61–78, https://doi.org/10.1007/s10533-014-0029-4, 2014.
Slessarev, E. W., Chadwick, O. A., Sokol, N. W., Nuccio, E. E., and Pett-Ridge, J.: Rock weathering controls the potential for soil carbon storage at a continental scale, Biogeochemistry, 157, 1–13, https://doi.org/10.1007/s10533-021-00859-8, 2022.
Soil Classification Working Group: The Canadian System of Soil Classification, 3rd Edn., Agriculture and Agri-Food Canada Publication 1646, 187 pp., ISBN 0-660-17404-9, 1998.
Stark, S., Martz, F., Ovaskainen, A., Vuosku, J., Männistö, M. K., and Rautio, P.: Ice-on-snow and compacted and absent snowpack exert contrasting effects on soil carbon cycling in a northern boreal forest, Soil Biol. Biochem., 150, 107983, https://doi.org/10.1016/j.soilbio.2020.107983, 2020.
Ste-Marie, C. and Paré, D.: Soil, pH and N availability effects on net nitrification in the forest floors of a range of boreal forest stands, Soil Biol. Biochem., 31, 1579–1589, https://doi.org/10.1016/s0038-0717(99)00086-3, 1999.
Stevenson, F. J.: Humus Chemistry: Genesis, Composition, Reactions, Second Edition, J. Chem. Educ., 72, A93, https://doi.org/10.1021/ed072pa93.6, 1995.
Sturm, M., Taras, B., Liston, G. E., Derksen, C., Jonas, T., and Lea, J.: Estimating Snow Water Equivalent Using Snow Depth Data and Climate Classes, J. Hydrometeorol., 11, 1380–1394, https://doi.org/10.1175/2010jhm1202.1, 2010.
Tang, Y., Horikoshi, M., and Li, W.: Package “ggfortify”: Unified interface to visualize statistical results of popular r packages, R Journal, 8, 478–489, https://doi.org/10.32614/rj-2016-060, 2016.
Tank, S. E., Fellman, J. B., Hood, E., and Kritzberg, E. S.: Beyond respiration: Controls on lateral carbon fluxes across the terrestrial-aquatic interface, Limnol. Oceanogr. Lett., 3, 76–88, https://doi.org/10.1002/lol2.10065, 2018.
Titus, B. D., Kingston, D. G. O., Pitt, C. M., and Mahendrappa, M. K.: A lysimeter system for monitoring soil solution chemistry, Can. J. Soil Sci., 80, 219–226, https://doi.org/10.4141/s99-018, 2000.
Torn, M. S., Trumbore, S. E., Chadwick, O. A., Vitousek, P. M., and Hendricks, D. M.: Mineral control of soil organic carbon storage and turnover, Nature, 389, 170–173, https://doi.org/10.1038/38260, 1997.
Wachenfeldt, E. von, Sobek, S., Bastviken, D., and Tranvik, L. J.: Linking allochthonous dissolved organic matter and boreal lake sediment carbon sequestration: The role of light-mediated flocculation, Limnol. Oceanogr., 53, 2416–2426, https://doi.org/10.4319/lo.2008.53.6.2416, 2008.
Wachenfeldt, E. von, Bastviken, D., and Tranvika, L. J.: Microbially induced flocculation of allochthonous dissolved organic carbon in lakes, Limnol. Oceanogr., 54, 1811–1818, https://doi.org/10.4319/lo.2009.54.5.1811, 2009.
Weintraub, M. N., Scott-Denton, L. E., Schmidt, S. K., and Monson, R. K.: The effects of tree rhizodeposition on soil exoenzyme activity, dissolved organic carbon, and nutrient availability in a subalpine forest ecosystem, Oecologia, 154, 327–38, https://doi.org/10.1007/s00442-007-0804-1, 2006.
Weyhenmeyer, G. A., Prairie, Y. T., and Tranvik, L. J.: Browning of boreal freshwaters coupled to carbon-iron interactions along the aquatic continuum, PLoS ONE, 9, e88104, https://doi.org/10.1371/journal.pone.0088104, 2014.
Žifčáková, L., Větrovský, T., Lombard, V., Henrissat, B., Howe, A., and Baldrian, P.: Feed in summer, rest in winter: microbial carbon utilization in forest topsoil, Microbiome, 5, 122, https://doi.org/10.1186/s40168-017-0340-0, 2017.