the Creative Commons Attribution 4.0 License.
the Creative Commons Attribution 4.0 License.
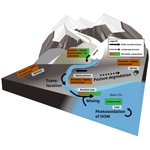
Complex dissolved organic matter (DOM) on the roof of the world – Tibetan DOM molecular characteristics indicate sources, land use effects, and processing along the fluvial–limnic continuum
Philipp Maurischat
Michael Seidel
Thorsten Dittmar
Georg Guggenberger
The Tibetan Plateau (TP) is the world's largest and highest plateau, comprising the earth's biggest alpine pasture system. It is sensitive to the impacts of climate change and anthropogenic pressure. Carbon cycling on the TP is influenced by glaciation and degradation of the pasture ecosystem. Dissolved organic matter (DOM) connects carbon reservoirs, following the hydrological continuum from glaciers and headwaters to lakes. Due to its complexity, DOM cycling along the aquatic continuum and the impact of land use and climate change on DOM characteristics are still not well understood. Here, we study solid phase extracted (SPE) DOM molecular characteristics using ultrahigh-resolution mass spectrometry (FT-ICR-MS) along the TP hydrological continuum from glaciers, groundwater springs, and wetlands, including pastures and alpine steppes, to the endorheic Lake Nam Co. Our study revealed that the SPE-DOM composition was largely influenced by local sources of glaciers, wetlands, and groundwater springs as well as pasture degradation. Glacial meltwater SPE-DOM contained more saturated compounds suggesting microbial sources together with aromatic compounds probably derived from aeolian deposition. In comparison, wetland and stream SPE-DOM were characterised by a higher percentage of highly unsaturated and aromatic molecular formulae. These were likely derived from inputs of vascular plants and soils. Groundwater spring SPE-DOM from degraded pastures differed from intact pasture samples. In degraded systems a strongly oxidised signature with the lowest counts of P heteroatoms, a lower ratio, and a higher aromaticity of SPE-DOM together with a high degradation index suggested a strong transformation of SPE-DOM. SPE-DOM of the endorheic lake was richer in unsaturated molecular formulae compared to the tributaries. This suggests algae and microbial sources and production in the lake. The SPE-DOM rich in aromatic and highly unsaturated formulae visible in the brackish zone of the lake shore contrasted sharply with that of the lake. Aromatic molecular formulae were strongly depleted in the lake deep water suggesting photooxidation of riverine SPE-DOM. This indicates that alpine SPE-DOM signatures are shaped by small-scale catchment properties, land degradation, and the influence of glaciers and wetlands. The close link of alpine SPE-DOM composition to landscape properties is indicative of a strong susceptibility of DOM characteristics to climatic and land use changes in High Asia.
- Article
(5352 KB) -
Supplement
(1385 KB) - BibTeX
- EndNote
Nutrient and energy cycles of ecosystems are connected by dissolved organic matter (DOM) fluxes (Spencer et al., 2014). DOM can connect fluvial ecosystems over hundreds of kilometres (Seidel et al., 2015) and links terrestrial and aquatic ecosystems (Goodman et al., 2011). Biogeochemical processing and DOM sources are known to shape the molecular composition of DOM (Liu et al., 2020; Roebuck et al., 2020; Seifert et al., 2016; Wilson and Xenopoulos, 2009). But how the DOM characteristics in alpine aquatic systems are influenced by different ecosystems and how DOM responds to ecosystem degradation is not well understood yet.
The Tibetan Plateau (TP) comprises the largest alpine pasture system in the world (Miehe et al., 2019) and is known as Asia's water tower (Bandyopadhyay, 2013), forming the source of large river systems. Large amounts of the water are stored in the ice masses of the TP, forming the largest frozen freshwater reservoir outside the polar regions. This third pole environment is well investigated (Qiu, 2008; Yao et al., 2012), revealing that High Asia's ecosystems are threatened (Hopping et al., 2018) by climate change (Yao et al., 2019) and by intensification of land use (Harris, 2010). Furthermore, emerging freshwater quality issues give reason for concern (Qu et al., 2019).
The Nam Co catchment, located in the southern part of the TP, lies in the transition zone of the K. pygmaea dominated alpine pasture ecosystem (Miehe et al., 2008) and the alpine steppe. The unique positioning in this transition zone is expressed between the south, with a more humid glacial-influenced high-mountain ecosystem, contrasting the hilly northern margin of the catchment, with more arid climate and vegetation dominated by alpine steppe, where clear signs of pasture degradation are visible (Maurischat et al., 2022a). This makes the Nam Co catchment a suitable natural laboratory to test for effects on organic matter (OM) characteristics and the cycling of OM in different ecosystems (Anslan et al., 2020). The effects of pasture degradation of Tibetan soils on OM stocks have been studied previously (Liu et al., 2017), but investigations on degradation-induced changes of DOM composition have fallen short. Also DOM characteristics and transformation in this complex natural interplay have only been investigated to a limited extent. Spencer et al. (2014) found complex OM sources in glaciers, streams, and Lake Nam Co. A recent study highlighted a coupled decrease of “protein-like” DOM with an increase of “humic-like” DOM along the flowpath (Li et al., 2021), whereas other works found indications for less reactive stream DOM due to the cold and fast flowing water (Maurischat et al., 2022a). This leaves important questions of (1) how DOM signatures are influenced by the diverse biotic and abiotic processes in the catchment with differing degrees of glaciation, alpine wetlands, and groundwater sources, as well as land degradation and the alpine pasture–alpine steppe ecotone and (2) how DOM is processed along the streams and in the lake.
Fourier-transform ion cyclotron resonance mass spectrometry (FT-ICR-MS) is an ultrahigh-resolution method allowing the identification of thousands of molecular formulae, offering an opportunity to better understand molecular, solid phase extracted (SPE) DOM characteristics, sources, and transformations (Hawkes et al., 2020; Leyva et al., 2020; Šantl-Temkiv et al., 2013). Here we used FT-ICR-MS to decipher the characteristics and processing of stream SPE-DOM of different subcatchments, glaciers, a groundwater spring, and an alpine wetland as well as SPE-DOM of an endorheic lake on the TP.
Our investigations aim at a better understanding of SPE-DOM processing at present conditions which will help to assess the potential consequences of changing environmental conditions in the future. It is important to link the potential vulnerability and responses of sensitive high-alpine ecosystems to ongoing anthropogenic changes. In this respect, we hypothesised the following.
- I.
SPE-DOM derived from different ecosystems (glaciers, groundwater springs, and wetlands) and streams in degraded land possess unique DOM signatures compared to the integrated DOM of subcatchment streams.
- II.
The SPE-DOM transformation along the stream path is limited; no major compositional shift is expected in-stream.
- III.
The SPE-DOM characteristics of lake water are chemically distinct from the terrestrial DOM sources and integrated stream SPE-DOM composition.
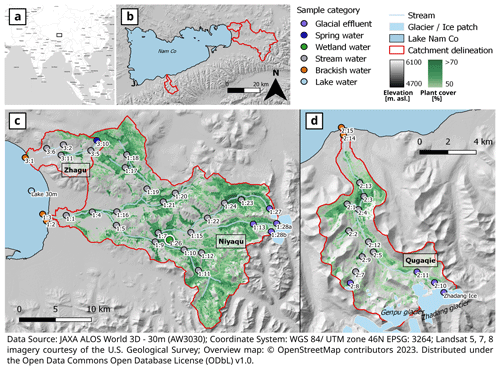
Figure 1(a) Overview map © OpenStreetMap contributors 2023. Distributed under the Open Data Commons Open Database License (ODbL) v1.0. The rectangle represents the sampling area. Panel (b) shows the outline of the Nam Co lake and the three subcatchments. (c, d) Map of the investigated subcatchments and sampling locations with sample categories. Plant cover estimations from Maurischat et al. (2022a) represent K. pygmaea pastures, the zones of most prominent yak grazing.
2.1 Site description and sampling
The Nam Co catchment has a total size of ∼ 10 800 km2. Two main landscape units are distinguished, the southern mountainous and the northern upland zone. The south of the catchment is characterised by the Nyainqentanglha mountain ridge (NMR), with glaciation at the highest elevations of more than 7000 (Bolch et al., 2010). Glacial meltwater is the dominant water source of southern streams (Adnan et al., 2019b). K. pygmaea pastures developed from 5300 downward (Anslan et al., 2020) and are associated with a felty organic-rich topsoil (Kaiser et al., 2008). Closer to the lake, alpine steppe vegetation is prevailing (Nieberding et al., 2021). The southern part of the catchment is characterised by higher precipitation compared to the north. Up to 530 mm yr−1 are measured at the NMR (Anslan et al., 2020). In the hilly uplands a less steep relief dominates (Yu et al., 2021), and an annual precipitation of around 300 mm yr−1 is reported (Anslan et al., 2020). Alpine pasture is developed in north-exposed hill flanks and valley bottoms, while alpine steppe grows on south-exposed flanks in the upland and at the lake shoreline (Maurischat et al., 2022a). Along with this aridity gradient from south to north, land degradation is increasing (Anslan et al., 2020). The endorheic Lake Nam Co with an elevation of 4726 has a total size of ∼ 2000 km2. The lake is dimictic, oligotrophic, and lightly saline (0.9 g salt L−1; Keil et al., 2010) and has a depth of 99 m. It is well supplied with oxygen and has a clear water column (Wang et al., 2020).
Three subcatchments of the Nam Co catchment were selected to represent the natural diversity (Fig. 1c and d). The Niyaqu catchment (sample IDs 1:n in Fig. 1a, low glaciation) in the east has a total area of 406 km2. Two streams drain the subcatchment; the southern stream receives meltwater from a glacier of the NMR located 700 m above lake level. This river runs through extensive alpine pastures and feeds a large alpine wetland (Fig. 1c, point 1:26). The northern stream drains a hilly upland area in the transition of the alpine steppe and the alpine pasture. The herding of yaks takes place throughout the year. The Zhagu subcatchment (sample IDs 3:n Fig. 1c, no glaciation – degraded) is located in the arid north. It has a size of 46 km2 and is mainly characterised by hilly upland relief (Keil et al., 2010). There is no glacial influence and only a small altitudinal gradient in this catchment, with the highest elevation at 5230 Two creeks drain the catchment, both fed by groundwater springs. During sampling, the streams were arheic, and clear signs of degradation of K. pygmaea pastures were visible (Maurischat et al., 2022a). Besides degraded pastures, the alpine steppe is developed and used for animal husbandry. In the south, the Qugaqie subcatchment (sample IDs 2:n Fig. 1d, high glaciation) represents the NMR zone. The catchment has a size of 58 km2 and is characterised by steep relief and a valley course in the south–north direction (Keil et al., 2010). The altitudinal difference between the lake and the summit is 2200 m. This catchment is used as a summer pasture.
Water samples were taken in September 2019 following the streams from source to terminus. Glaciers, wetlands, and groundwater springs were sampled directly. Three source groups (glacial effluents, groundwater springs, and alpine wetlands) and three sampling units (stream water, brackish water, and lake water) were distinguished, resulting in six sample categories (Fig. 1). Glacial effluents were drawn directly at or close to the glacial terminus, while groundwater was sampled directly at the springs. Alpine wetland samples were taken from the standing water column in the wetlands. Brackish water samples were taken in the mixing zone of stream and lake water offshore in Lake Nam Co.
Samples were taken from the middle of the stream profile using a telescopic sampling device. Lake water was sampled from 30 m depth with a submersible sampler. All samples were taken in seven subsamples with a volume of 1 L each, mixed, and a 0.5 L aliquot of this was taken for analysis. Samples were filtered on-site using a 0.45 µm mesh size polyethersulfone membrane (Supor, Pall, Port Washington, USA), a filtration device, and a portable electric pump. Samples were stored in pre-cleaned high-density polyethylene bottles (ROTILABO, Carl Roth, Karlsruhe, Germany) and kept at −21∘C until analysis.
2.2 Solid-phase extraction
DOM samples were acidified to pH 2 using 32 % HCl (ROTIPURAN p.a., Carl Roth, Karlsruhe, Germany). Dissolved organic carbon (DOC) concentrations were measured from 20 mL of sample by high-temperature oxidation on a total organic carbon analyser (vario TOC cube, Elementar, Langenselbold, Germany). DOM samples were diluted with ultrapure water to a concentration of 1.5 mg C L−1; 250 mL of diluted sample were used for extraction. Cartridges with 100 mg of styrene divinylbenzene polymer (PPL) resin (Bond Elut, Agilent Technologies, Santa Clara, USA) were used for extraction. SPE-DOM was prepared following Dittmar et al. (2008). The SPE elute was transferred to cauterised brown glass flasks (neochrom, neoLab Migge, Heidelberg, Germany), sealed with polytetrafluorethylene caps (neochrom, neoLab Migge, Heidelberg, Germany), and stored at −18∘C until analysis. The extraction efficiency was evaluated by drying 0.2 mL of SPE-DOM under N2 atmosphere and resolving the aliquot in ultrapure water. The samples were then analysed for their DOC concentrations by high-temperature oxidation, and the volumetric proportion of initial DOC to extracted SPE-DOC concentrations was calculated. The reference material (Suwannee River/IHSS) (Green et al., 2015) was compared with routine assays. Blank samples with ultrapure water were used to check for contamination.
2.3 Fourier-transform ion cyclotron resonance mass spectrometry
SPE-DOM samples were diluted in 1:1 methanol/ultrapure water to a final concentration of 5 mg C L−1 for analysis. Samples were analysed in duplicates on a SolariX XR 15 Tesla FT-ICR-MS (Bruker Daltonics, Bremen, Germany). Electrospray ionisation (ESI) was carried out in negative mode; samples were injected at a flow rate of 122 µL h−1. A total of 200 broadband scans (mass range of 92.14 to 2000 Da) were acquired per sample; accumulation time was 0.2 s per scan. Mass spectra were internally calibrated with a list of known CxHyOz molecular formulae over the mass range in the samples, achieving a mass error of < 0.1 ppm. Instrument variability was assessed with an in-house standard of SPE-DOM from North Equatorial Pacific Intermediate Water (NEqPIW) collected near Hawaii (Natural Energy Laboratory of Hawaii Authority, NELHA) (Green et al., 2014). Molecular formula attribution was done with ICBM-OCEAN (Merder et al., 2020). The method detection limit (MDL) was applied (Riedel and Dittmar, 2014) with a minimum signal-to-noise ratio (S/MDL) of 2.5. Minimum signal to MDL ratio as backbone for recalibration was 5 using mean recalibration mode. Molecular formulae were assigned with a tolerance of 0.5 ppm as in the mass range 95 to 1000 Da. Molecular formulae assignments were accepted if the molecular formula was present in >5 % of the samples. Contaminants were identified and excluded using the contaminant reference mass list and in additional conformity with the SPE-DOM process blanks. Detection limits for peaks were normalised to sample peak intensities. The overall peak intensities were scaled to the local sample maxima using the sum of peaks. Molecular formulae containing isotopes (13C, 18O, 15N, 34S) were removed from the data table for further processing and molecular formulae with molar ratios of oxygen to carbon (O:C) = 0, O:C ≥ 1, and hydrogen to carbon (H:C) > 2.5 were removed as well. Duplicate samples were normalised, and molecular formulae were retained only when present in both duplicates.
2.4 SPE-DOM molecular descriptive classes, counts, and indices
Molecular formulae were assigned to SPE-DOM molecular compound classes (Leyva et al., 2020). The original compound classification was taken from Šantl-Temkiv et al. (2013), and SPE-DOM compound class labels were modified according to Merder et al. (2020). The modified aromaticity index (AImod) representing DOM aromaticity, was calculated for each formula as proposed by Koch and Dittmar (2006, 2016). AImod > 0.5 was assigned as aromatic, while AImod ≥ 0.67 was considered as condensed aromatic compounds. The degradation index (IDeg) was calculated as a measure of the degradation state of SPE-DOM (Flerus et al., 2012), and the terrestrial index (ITerr) was calculated as a measure of terrestrial SPE-DOM sources (Medeiros et al., 2016). Molecular formulae that were part of the island of stability (IOS) were evaluated to gain insight into the relative abundance of recalcitrant SPE-DOM (Lechtenfeld et al., 2014). This is based on the assumption that the IOS contains SPE-DOM molecular formulae representing recalcitrant oceanic SPE-DOM that is empirically stable on millennial timescales (Lechtenfeld et al., 2014). The CHO index was calculated as a measure of organic carbon oxidation (Mann et al., 2015). Low CHO values indicate highly reduced molecular formulae, and high CHO values indicate highly oxidised molecular formulae. Molecular diversity was interpreted as α-diversity by depicting intra-community molecular diversity (Thukral, 2017). Here we used the total number of molecular formulae on the group scale of subcatchments and sample categories.
2.5 Statistical analyses
Molecular formula intensities were rescaled between 0 and 1. Grouping was conducted with two independent factors: (1) the three subcatchments of Niyaqu (low glaciation), Qugaqie (high glaciation), and Zhagu (degraded) and Lake Nam Co and (2) the sample categories glacial effluents, spring, and wetland as well as stream water, brackish water, and lake water. Samples from spring and wetland, as well as Lake Nam Co, were excluded from statistical group comparisons due to the small sample sizes. Intensity-weighted arithmetic means and standard deviations were calculated for AImod, number of formulae containing the heteroatoms nitrogen (N), phosphorous (P), and sulfur (S), as well as the total number of assigned formulae and for compound classes.
Due to violations of normal distribution and homoscedasticity in combination with unbalanced sampling design, parametrical tests were considered unreliable (Bortz and Schuster, 2010). Multiple pairwise comparisons were conducted using Kruskal–Wallis tests in combination with Bonferroni post hoc corrected Dunn tests (Birnbaum, 1956). Significance levels of α = 0.05 were applied (Tables S1 and S2 in the Supplement).
Non-metric multidimensional scaling (NMDS) was used for dimensionality reduction and ordination (Anderson, 2006; Faith et al., 1987) based on a Bray–Curtis dissimilarity index matrix (k = 3). Data for NMDS were scaled and mean-centred (Jolliffe, 2002). NMDS was performed for independent factors (sites and sample categories), while those with low statistical power (n < 3) were not incorporated. Loadings, scores, and R2 coefficients of determination are provided in Tables S3–S7. Co-correlation was checked visually and by Pearson's correlation coefficient, defined as |r| > 0.75. The following compound classes were combined because of co-correlation: aromatic O-rich and aromatic O-poor are ARO; highly unsaturated O-rich and highly unsaturated O-poor are HUSAT; and unsaturated O-rich, unsaturated O-poor, and unsaturated with N are USAT (Fig. 4). Collinear variables (Sect. 2.6) were removed.
R software (The R project for statistical computing, v3.6.3, GNU free software) was used for statistics. The R base packages (R Core Team, 2013) and tidyverse (Wickham et al., 2019) were used for data organisation, pre-processing, and statistics. The packages ggplot2 (Wickham et al., 2019) and vegan (Dixon, 2003) were used for graphical illustration and for NMDS analysis, respectively.
2.6 Environmental variables for statistical analysis
Several parameters were used as predictor variables in the NMDS. Internal predictor variables were generated from the SPE-DOM dataset obtained by FT-ICR-MS (SPE-DOM indices, compound class percentages, and heteroatom counts; described in Sect. 2.4). External predictor variables were taken from a previous study of the same site and the same sampling campaign (Maurischat et al., 2022a) and tested for statistical correlations with this SPE-DOM dataset. The selected external variables showed evidence as functional predictors in the prior study and are acknowledged as key parameters for DOM characterisation in numerous applications. The variables included fluorescent DOM (FDOM) components (here used as the product of co-correlated microbial and terrestrial-like FDOM components) (Fellman et al., 2010), DOC concentrations (Eklöf et al., 2021), δ13C of DOC (Guo and Macdonald, 2006), dissolved inorganic carbon (DIC) concentrations as a catchment geology parameter (Wang et al., 2016), nitrate concentrations () (Harms et al., 2016), and the mean plant cover at the sampling point as a land use indicator (Sankar et al., 2020).
3.1 Sample treatment and quality assessment
SPE-DOM extraction efficiencies were 61.4 % ± 18.6 % (Table S8). DOM extraction efficiencies and the number of SPE-DOM molecular formulae (detected by FT-ICR-MS) were only weakly correlated (R2 = 0.08, F (1, 43) = 5.144, β = −0.007, p = 0.02), indicating that extraction efficiency was not an important driver of molecular variability in our samples. Duplicate SPE-DOM process blanks had below 1000 molecular formulae assigned, and the Suwannee River/IHSS reference material had ∼ 3500. The minimum molecular formulae count to accept OM samples was set to 2000, roughly in the range of the number of molecular formulae expected from terrestrial aquatic samples (Seidel et al., 2017; Spencer et al., 2014). The in-house control standard (NEqPIW SPE-DOM) was run repeatedly during analysis (n = 38) to account for instrument variability. On average, 3558 (± 218) molecular formulae were assigned: no significant instrument drift was detected (Fig. S1 in the Supplement).
Table 1Overview on the mean and standard deviation of indices and elemental composition ratios and the mean and standard deviation of molecular formulae counts for sites.
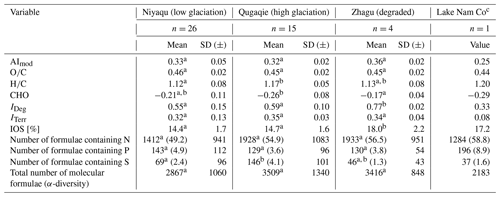
a, b Significant differences (α = 0.05) are indicated by superscript letters. c Single sample, standard deviations were not calculated, and statistical tests were not performed. For heteroatoms (N, P, S), percentages of the total number of molecular formulae are given in parentheses. Boxplots of data are presented in Fig. S2.
Table 2Overview of the mean and standard deviation of indices and elemental composition ratios and the mean and standard deviation of formulae counts for sample categories.
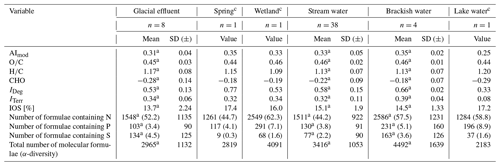
a, b Significant differences (α = 0.05) are indicated by superscript letters. c Single sample, standard deviations were not calculated, and statistical tests were not performed. For heteroatoms (N, P, S), percentages of the total number of molecular formulae are given in parentheses. Boxplots of data are presented in Fig. S3.
3.2 Molecular group counts and statistics
The number of assigned molecular formulae, here termed α-diversity, decreased in the order Qugaqie (high glaciation), Zhagu (degraded), Niyaqu (low glaciation), and Lake Nam Co. Lake Nam Co SPE-DOM had 50 % less assigned molecular formulae compared to the subcatchments (Table 1). SPE-DOM samples from wetland and brackish environments had the highest numbers of assigned molecular formulae (Table 2); compositional shifts in the and ratio of brackish and lake SPE-DOM are displayed in Fig. 6a, with a zoom on one example mass range (371–371.3 , Fig. 6b).
Lake Nam Co SPE-DOM had relatively more N-containing molecular formulae compared to SPE-DOM samples from the subcatchments (Table 2). The relative abundance of S-containing molecular formulae was significantly lower in low glaciation Niyaqu compared to high glaciation Qugaqie SPE-DOM (p = 0.005). P-containing molecular formulae were enriched in the lake compared to SPE-DOM of the subcatchments (Tables 1 and S1). N-containing molecular formulae were more abundant in wetland water and brackish SPE-DOM samples, while groundwater spring SPE-DOM samples had the lowest count. Glacial SPE-DOM had the highest relative abundances of S-containing molecular formulae.
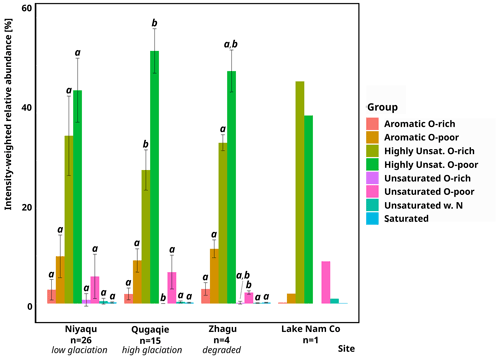
Figure 2Relative intensity-weighted averages of SPE-DOM compound classes for stream water of the three subcatchments and Lake Nam Co (sites). For sample sizes n < 3, no standard deviations are given. Error bars indicate standard deviations; significant differences (α = 0.05) are indicated by superscript letters (a, b).
SPE-DOM of Lake Nam Co had lower AImod and ITerr values compared to the subcatchments (Table 1). For the sample categories, brackish SPE-DOM had higher ITerr values, but no significant differences were observed (Table 2). The ratios were highest in Lake SPE-DOM (Table 1), with significantly higher relative numbers of hydrogen compared to Niyaqu (p = 0.005). Additionally, the CHO index showed that Lake SPE-DOM and SPE-DOM of the high glaciation Qugaqie catchment were less oxidised compared to SPE-DOM of the degraded Zhagu catchment (p = 0.042, statistically tested only for subcatchments). Correspondingly, IDeg values, were significantly higher for degraded Zhagu compared to the glaciated Niyaqu and Qugaqie subcatchments SPE-DOM (p = 0.0002). IOS values, indicative of recalcitrant SPE-DOM with relatively long residence times, showed a significantly higher contribution in degraded Zhagu and Lake Nam Co compared to low glaciation Niyaqu and high glaciation Qugaqie (p = 0.04, 0.05, respectively, statistically tested only for subcatchments). For sample categories (Table 2), ratios were higher in glacial and lake SPE-DOM. The CHO index suggested less oxidised SPE-DOM in glacial effluents and the lake compared to terrestrial sources (Fig. 5). Correspondingly, the highest IDeg values were observed in SPE-DOM of the brackish zone and in groundwater springs. The percentage of IOS values increased in lake and groundwater springs compared to glacial effluents, indicating differences in the contribution of recalcitrant SPE-DOM.
Compound classes (Fig. 2) give an overview of the composition of SPE-DOM. The largest differences among the sites were found for aromatic classes. Lake Nam Co had 80 % less aromatic (O)-rich molecular classes compared to the subcatchments. The lake had only 5 % of the abundance of aromatic O-poor compounds compared to subcatchment SPE-DOM. For highly unsaturated O-rich molecular formulae, Lake Nam Co had higher values compared to subcatchment SPE-DOM, while highly unsaturated O-poor molecular formulae decreased in the lake. Furthermore, the unsaturated O-poor and unsaturated N-containing SPE-DOM classes were higher in lake SPE-DOM compared to the terrestrial systems. Highly unsaturated O-rich formulae were more abundant in SPE-DOM of high glaciation Qugaqie compared to low glaciation Niyaqu (Fig. 2).
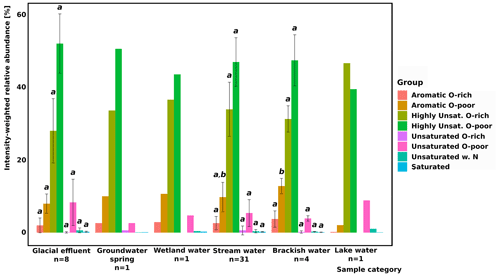
Figure 3Relative intensity-weighted averages of DOM compound classes according to environmental sample categories. For sample sizes n < 3, no standard deviations are given. Error bars indicate standard deviations; significant differences (α = 0.05) are indicated by superscript letters (a, b).
The relative abundances of aromatic compounds were different between SPE-DOM of the lake and SPE-DOM assigned to other sample categories, with samples from Nam Co having the lowest relative abundances (Fig. 3). Brackish SPE-DOM had significantly more aromatic O-poor compounds compared to glacial effluents (p = 0.05). SPE-DOM from the Nam Co Lake had the highest relative abundances of highly unsaturated O-rich compounds compared to all other groups; glacial SPE-DOM, especially, had on average 40 % less O-rich compounds.
Unsaturated O-poor compounds were relatively more abundant in lake SPE-DOM and glacial effluents compared to the other environmental sample categories; this class was especially depleted in spring SPE-DOM. Alongside lake and glacial, SPE-DOM were relatively rich in unsaturated N-containing formulae. Spring water had the fewest of this compound class (Fig. 3).
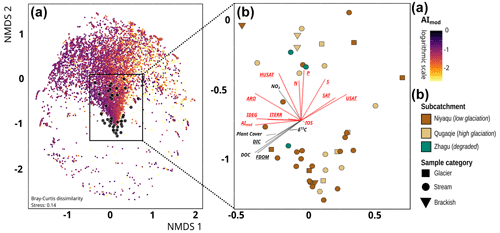
Figure 4Non-metric multidimensional (NMDS) scaling analysis based on the relative abundances of SPE-DOM molecular formulae (calculated on a Bray–Curtis dissimilarity matrix) (a) with the colour-coded modified aromaticity index (AImod, logarithmic scale) (Koch and Dittmar 2006, 2016). (b) Zoom of NMDS analysis based on the relative abundances of SPE-DOM molecular formulae with post hoc fitted SPE-DOM parameters (internal predictor variables, red) and environmental parameters (external predictor variables, black) shown as vectors. Underlined parameters were significantly (p ≤ 0.05) related to NMDS ordination (stress = 0.14, k = 3). ARO = aromatic O-rich and aromatic O-poor; HUSAT = highly unsaturated O-rich and highly unsaturated O-poor; USAT = unsaturated O-rich, unsaturated O-poor, and unsaturated with N; and SAT = saturated. Subcatchments are represented by the colour of sample points, while sample categories are represented by shapes.
3.3 Multivariate statistical analysis
NMDS was conducted with a graphical overlay for sites and sample categories. The stress value of 0.14 is within tolerance (< 0.2; Dexter et al., 2018). In NMDS ordination (Fig. 4a), molecular formulae expanded in the ordination plane above the coordinate origin. Samples were distributed in two groups depending on aromaticity and related indicators (AImod, IDeg, and ITerr). AImod values increased from the positive to the negative direction of the first dimension. In the positive direction of dimension 1, samples containing relatively more unsaturated and saturated SPE-DOM compound classes as well as S-containing compounds were distributed (Fig. 4b); these samples also had lower AImod, IDeg, and ITerr. In the negative direction of dimension 1, samples with a higher abundance of aromatic compound classes were distributed. The external predictors of DIC and plant cover were loading in this direction along with DOC concentration and values of FDOM and δ13C of DOM (black vectors in Fig. 4b), suggesting that these samples were influenced by DOM inputs of plants and soils. The second dimension of the NMDS ordination separated samples according to the abundance of highly unsaturated and saturated SPE-DOM compounds, together with the N, P, and S heteroatoms. These samples were also characterised by elevated NO3 concentrations. Samples located closer to the origin of predictor variable vectors were related to higher IOS percentages and suggested a higher abundance of recalcitrant SPE-DOM.
Samples of the degraded Zhagu subcatchment were located close to the centre of predictor variable vectors (Fig. 4b), while samples of high glaciation Qugaqie were scattered on the dimension plane. SPE-DOM from the low glaciation Niyaqu subcatchment was more uniformly placed in the lower part. Stream water SPE-DOM was scattered, indicating large chemical diversity. Most brackish SPE-DOM samples were placed in the upper left of the plane, associated with higher terrestrial indicators (AImod, IDeg, and ITerr) and more abundance of N and P heteroatoms. Glacial SPE-DOM was associated with heteroatoms, saturated and unsaturated molecular compounds, and less depleted δ 13C of DOM, visible by its positioning in the bottom left to top right of the ordination plane.
4.1 Catchment properties shape SPE-DOM composition at Lake Nam Co
The subcatchments (high glaciation, low glaciation, and degraded) of Lake Nam Co differed significantly in their molecular SPE-DOM composition. The high glaciation subcatchment had the largest molecular α-diversity and a larger abundance of S heteroatoms (Table 1). This can be influenced by the productivity of the glacial ecosystem (Hodson et al., 2008). S heteroatoms in DOM are likely related to high sulfide contents in the runoff of Zhadang glacier (Yu et al., 2021). Sulfate reduction takes place in glacial sediments and ice (Wu et al., 2012). Under sulfidic conditions, sulfide can be incorporated into DOM (“sulfurisation”) (Pohlabeln et al., 2017). The higher abundance of O-poor compounds, namely with depleted unsaturated O-rich and highly unsaturated O-rich molecular formulae and increased highly unsaturated O-poor and unsaturated O-poor formulae alongside negative CHO values, indicates less intensively microbial transformed SPE-DOM compounds (Anesio et al., 2009; Hood et al., 2009; Spencer et al., 2014) compared to the degraded and low glaciation subcatchments. Accordingly, D'Andrilli et al. (2019) observed the relative increase of O-enriched molecular formulae after bio-incubations of DOM. Likely, low water temperatures of the glacial meltwater in Qugaqie hamper the microbial decomposition of DOM (Adams et al., 2010).
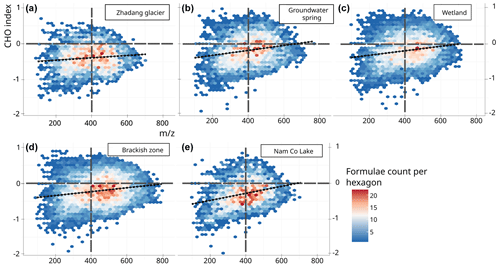
Figure 5Hexagon scatters plot the count of the chemical formula in the space of the CHO index (Mann et al., 2015) and . The black dotted lines represent the linear model of the CHO index and of the respective sample, and the dashed grey lines split the plot into quadrants for orientation. The regression and ledger lines are printed to guide the eyes only. (a) Ice from a glacier in high glaciation Qugaqie, (b) groundwater spring from the upland of degraded Zhagu (3:10), (c) water from the standing water column of a wetland (1:26), (d) water from the brackish zone of Lake Nam Co and a tributary stream (2:15), and (e) water sample from 30 m depth of Lake Nam Co. Axis scales are fixed.
Compared to the Qugaqie subcatchment, the contribution of glacial meltwater was smaller in the Niyaqu subcatchment and absent in Zhagu. The main water sources for Zhagu are precipitation and groundwater (Adnan et al., 2019a; Anslan et al., 2020; Tran et al., 2021). In the degraded Zhagu subcatchment, a stronger indication of aromaticity with higher AImod and higher relative abundance of aromatic compounds was found. Likewise, the higher oxidation state indicated by the CHO index (Fig. 5b, Table 2) and higher degradation index (IDeg, Table 2) suggests a larger share of soil-borne, aromatic DOM compounds. This was also corroborated by the higher percentage of the IOS (+ ∼ 3 %), indicating a larger input of degradation products. The low glaciation Niyaqu catchment in comparison had a higher contribution of aromatic and unsaturated compounds together with a lower ratio, suggesting less oxidised DOM. In combination with the AImod and ITerr indices, this suggests inputs of soils and plants, with, for example, lignin and hemicellulose and their degradation products (Roebuck et al., 2018; Seifert et al., 2016).
The high glaciation Qugaqie subcatchment comprises of a signature rich in highly unsaturated O-poor and unsaturated O-poor formulae, likely derived from a glacial-borne microbial source. Furthermore, the high aromatic indices (AImod, ITerr) and high percentages of aromatic compound classes are indicative of SPE-DOM derived from soil and plant material. Likely, there is a steady input of soil-derived material into the streams from pastoral land as demonstrated for other grassland systems (Seifert et al., 2016; Lu et al., 2015). Notably, this influence became smaller when glacial-borne more unsaturated DOM was more dominant on the subcatchment level (Fig. 3b).
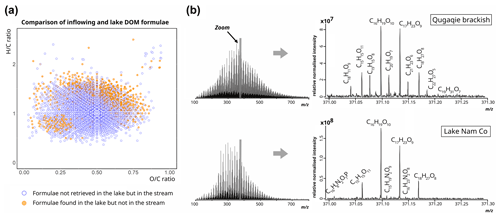
Figure 6(a) A van Krevelen diagram of molecular formulae retrieved in lake DOM but not in brackish DOM and vice versa. (b) Left: intensity normalised overview mass spectra (: 100–800) of Qugaqie brackish DOM (Id 2:15) and a water sample from 30 m depth of Lake Nam Co. (b) Right: exemplary zoom into one mass range (371.0–371.3 ) of the respective samples. Note the axis scales shift.
4.2 The effect of pasture degradation on SPE-DOM composition
Molecular α-diversity defined by the number of molecular formulae and SPE-DOM characteristics can be pinpointed to landscape units/environmental sample categories (summarised in Fig. 7). Glacial SPE-DOM from Qugaqie and Niyaqu subcatchments contained two different signatures from different sources. First, with high abundances of unsaturated compounds with and without nitrogen as well as a low oxidation state of carbon (Fig. 5a), high ratios of and low percentages of recalcitrant SPE-DOM are visible by the IOS. These parameters indicate a relatively fresh, reduced (oxygen-poor) SPE-DOM of low molecular mass probably derived from microbial activity at the partly anoxic ice shield. This is in line with findings from other glacial environments worldwide (Hood et al., 2009; Telling et al., 2011; Anesio et al., 2009). Second, aromatic and highly unsaturated compound classes and aromatic and terrestrial indices (ITerr, AImod) were suggesting plant- and soil-borne SPE-DOM sources, despite the absence of plant cover in the glacial zones. Glaciers receive compounds with higher molecular mass from aeolian deposition, either condensed (poly)aromatics, e.g. from the burning of fossil fuels (Yasunari et al., 2013), or compounds uncondensed but rich in phenolics, usually associated with dust from degraded vascular plant material and soils (Singer et al., 2012; Takeuchi, 2002). Local dust formation on the TP is projected to increase with ongoing pasture degradation (Wang et al., 2008), likely affecting the DOM composition of glaciers. The coexistence of microbial produced, autochthonous DOM and airborne aromatic, allochthonous DOM renders the understanding of the downstream fate of glacial DOM difficult.
Groundwater spring SPE-DOM from the degraded Zhagu subcatchment mainly contained aromatic and highly unsaturated compounds. Molecular α-diversity and the number of N, P, and S heteroatoms were low. Together with this, high IDeg and CHO indices suggested a strong degradation of spring SPE-DOM (Fig. 5b); a further 17 % of DOM being IOS DOM indicates a large contribution of recalcitrant DOM. Spring water is generally expected to inherit aquifer and catchment characteristics in its DOM signature, also partly preserving its terrigenous source (Osterholz et al., 2022; Yoo et al., 2020). The shallow groundwater table of Zhagu (Tran et al., 2021) is in contact with soil OM and yak faeces (Maurischat et al., 2022a), which can leach soil-borne OM to the groundwater (Connolly et al., 2020) which re-emerges at groundwater springs. Hence, the highly degraded SPE-DOM compounds likely originated from the degraded pedosphere and have been transported with the groundwater. The connection of degraded pastures of Zhagu (Figs. 1 and 4b) with the molecular composition of groundwater spring SPE-DOM indicates that highly modified SPE-DOM signatures are transported with the spring water and retrieved in streams.
SPE-DOM of an alpine wetland had a high α-diversity (> 4000 molecular formulae) and was rich in N and P heteroatoms as well as in highly unsaturated O-rich and unsaturated O-poor compounds. The wetland was also enriched in ammonium and DOC compared to the streams (Maurischat et al., 2022a). Alpine wetlands are highly productive and contain large amounts of nutrients in the biomass and soil OM (Bai et al., 2010; Zhang et al., 2020). Wetlands on the TP have been massively degrading (Zhang et al., 2011), enhancing microbial decomposition in wetland mire soils (Ma et al., 2018) and driving a subsequent release of nutrients into adjacent streams (Gao, 2016). For Lake Nam Co, this would pose a severe biodiversity threat to oligotrophic streams and the sensitive lake shore (Hu et al., 2016).
Stream samples are concentrated in the lower centre of the NMDS (Fig. 4b). These samples had a dominance of aromatic compounds either associated with highly unsaturated O-poor or highly unsaturated O-rich formulae, suggesting mostly inputs of terrestrial compounds, such as lignin and tannin and their degradation products (Mann et al., 2015), further corroborated by depleted δ13C DOC signatures (Fig. 4b; Maurischat et al., 2022a). The K. pygmaea ecosystem spreads as an azonal pasture along the streams (Fig. 1). Lu et al. (2015) pointed out that grassland sites provide terrestrial inputs of aromatic and highly unsaturated compounds to surface waters. The K. pygmaea pasture browsed by yak has the potential influence of their faeces (Du et al., 2021), suggesting lower molecular mass and negative CHO inputs due to the reductive conditions in the yak gastrointestinal tract and decomposition of plant-borne material therein (Fahey et al., 1983). Stream samples can therefore also be under the influence of changing inputs from the pasture ecosystem. Faeces inputs and products of their microbial utilisation are likely associated with increases of N-containing unsaturated formulae and saturated formulae (Vega et al., 2020). Pastoral land use and pasture degradation both had a considerable impact on stream SPE-DOM composition. While intact pastures released a highly unsaturated and aromatic signature related to the pasture soils and plants, streams close to degraded pastures were characterised by highly oxidised aromatic signatures and low molecular α-diversity.
4.3 The lake reactor: photooxidation changes the SPE-DOM molecular composition
Brackish SPE-DOM samples had the highest molecular α-diversity, highest number of N and S heteroatoms, and highest number of aromatic O-poor compounds together with high AImod and ITerr (Table 2). Thus, brackish SPE-DOM retained and accumulated the terrestrial signal of streams. Brackish regions are not only zones of the gradual mixing of different water masses (Van Dongen et al. 2008), where terrestrial-derived DOM of streams is exported to lakes and the ocean (Benner et al., 2004; Dittmar and Kattner, 2003; Ruediger, 2003), but also zones of chemical transformation and uptake of riverine DOM, e.g. by flocculation and osmotrophy (Hoikkala et al. 2015). The relative increase of aromatic compounds in brackish SPE-DOM compared to stream SPE-DOM (Fig. 3) suggests a relative enrichment, for example, by selective degradation and oxidation of lower-molecular-mass compounds in the wash of the waves as indicated by the increased CHO index and IDeg values (Fig. 5d) as shown for estuaries (Asmala et al., 2014). We conclude that the high molecular α-diversity in brackish samples represents both the terrestrial input from streams mixing with the SPE-DOM signature of lake water and the selective degradation of SPE-DOM in the high-energy wave zone.
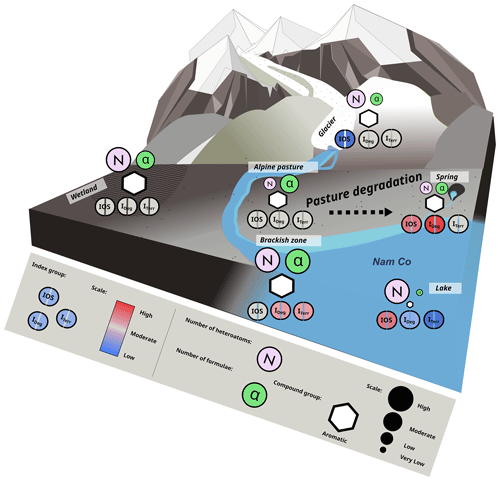
Figure 7Overview of the molecular information from SPE-DOM along the water continuum of the TP. The number of N heteroatoms is indicated relative to the total number of molecular formulae. The island of stability (IOS), degradation index (IDeg), and terrestrial index (ITerr) are ranging in a red–blue colour. Alpine steppe and groundwater spring samples are spatially correlated and thus subject to a common evaluation. The local pasture degradation gradient is indicated by a dashed arrow.
Lake water differed in its SPE-DOM composition most strongly as compared with all other sample categories (Fig. 7). Lake SPE-DOM was relatively enriched in unsaturated and saturated compounds, which can include lipids and carbohydrates, but it was relatively depleted in aromatic and highly unsaturated O-rich molecular formulae. Correspondingly, AImod and ITerr decreased. Photooxidation and microbial degradation can both remove aromatic DOM, such as lignin-derived phenolics (Spencer et al. 2009; Helms et al. 2014; Vähätalo and Wetzel, 2004). Given the clear water column and high irradiation at the TP (Wang et al., 2020), photooxidation can take place down to greater depths in the lake, making it a probable important mechanism. Photooxidation in combination with microbial degradation can explain the depletion of aromatic constituents when comparing brackish and lake SPE-DOM (blue points in Fig. 6a and mass spectra in Fig. 6b).
In the estuary–lake gradient, riverine terrigenous SPE-DOM likely underwent a transformation, leaving more recalcitrant SPE-DOM behind (Goldberg et al., 2015) as corroborated by the 2.1 % and 2.7 % increase of IOS compared to stream and brackish SPE-DOM, respectively (Table 2). Along with transformation of imported riverine SPE-DOM, primary production in the lake plays a key role for the SPE-DOM characteristics of Lake Nam Co. The increase in unsaturated and unsaturated N-containing compound classes and the more negative CHO with lower molecular mass (Fig. 5e) in lake SPE-DOM (Fig. 3, yellow points in Fig. 6a) act as indicators for bacterial and algal primary production in the lake. Microbial DOM sources have been suggested for Lake Nam Co (Spencer et al., 2014; Maurischat et al., 2022a) and other large lakes of the TP (Liu et al., 2020). Performing a food web study in Lake Nam Co, Hu et al. (2016) reported that mainly lake-borne organic carbon sources are utilised by zooplankton, showing the importance of this type of DOM as a substrate. The comparably low CHO index (Fig. 5e) underlines the existence of low molecular mass reduced DOM produced in the lake, while higher molecular masses of aromatic and highly unsaturated riverine origin are more oxidised in the lake environment compared to other systems (indicated by the greatest steepness of the regression line in Fig. 5e), indicative of the strong processing described above. Lake SPE-DOM exhibited sources derived from algal and microbial production. Also, it is low in aromatic compounds and terrigenous indices and had a much larger percentage of IOS compared to subcatchment streams SPE-DOM, suggesting more recalcitrant compounds. The shift in SPE-DOM molecular composition hence shows that the terrigenous riverine aromatic DOM is processed in the lake, while microbiota derived DOM is produced.
We elucidated the molecular composition and the processing of SPE-DOM in the High Asian endorheic Nam Co catchment. We investigated three subcatchments: high glaciation, low glaciation, and no glaciation/degraded, including SPE-DOM samples of glaciers, groundwater springs, alpine wetlands, streams, the brackish mixing zone, and the weakly saline lake. The subcatchments differed in the molecular composition of SPE-DOM.
In the high glaciation catchment, we identified a unique dual source of a microbial, low molecular mass SPE-DOM relatively enriched in S heteroatoms and unsaturated compounds with and without nitrogen, suggesting autotrophic sources in the glacial ice shield. Meanwhile, aromatic SPE-DOM with high abundance of highly unsaturated compounds, such as plant-derived lignin degradation products or polycondensed aromatics derived from the combustion of fossil fuels or household burning of yak faeces, hint at a depositional source by aeolian transport. The influence of glacial meltwater greatly modified SPE-DOM signatures along the high glaciation Qugaqie stream, probably delivering more bio-available compounds to the southern lake shoreline. The low glaciation Niyaqu catchment comprised a lower molecular α-diversity and had a mainly terrestrial-borne SPE-DOM source of highly unsaturated and aromatic compounds, attributed to the input of the surrounding K. pygmaea pastures to the streams. In comparison, spring SPE-DOM of the degraded Zhagu subcatchment was influenced by degradation of K. pygmaea pastures visible by highly degraded and oxidised signatures with a higher percentage of recalcitrant compounds. DOM signatures can thus be used to trace pasture degradation. Wetland SPE-DOM exhibited high molecular α-diversity and was relatively enriched with N heteroatoms. Wetland SPE-DOM reflected the comparably high primary productivity of these systems. The reported degradation of alpine wetlands in High Asia can drive a large release of DOM to the streams; at Nam Co this can be considered a threat to the oligotrophic lake. The stream samples were mostly associated with the input of terrestrial-borne materials, originating from vascular plants and soils. These are attributed to the K. pygmaea ecosystem stretching along the paths of streams. From the pastures, aromatic and highly unsaturated SPE-DOM was constantly refuelled into the streams. Brackish SPE-DOM represented the mixing zone of stream and lake water. Here, riverine terrestrial DOM entered the lake, indicating that DOM transformation along the stream was limited. However, lake SPE-DOM was different compared to the tributaries. Its molecular composition suggested photooxidation and microbial degradation as transformation of imported stream DOM, together with a source of microbial and algal production in the oligotrophic lake.
Our study shows that DOM cycling in the Nam Co catchment needs a thorough assessment, as it can be diverse on the subcatchment level and between landscape units. In order to safeguard water resources and related ecosystem services, knowledge about the different sources and the fate of DOM is indispensable. SPE-DOM molecular properties have proven to be selective for the source systems and hence allow one to decipher landscape processes. In the case of the sensitive TP, they might be a way forward to better understand the local effects of global change.
The code used to evaluate data and generate the findings of this study is available on request from the corresponding author.
Data generated for this paper (ultrahigh-resolution Fourier-transform ion cyclotron resonance mass spectrometry data) are available at https://doi.org/10.5281/Zenodo.7573508 (Maurischat et al., 2022b) upon request.
The supplement related to this article is available online at: https://doi.org/10.5194/bg-20-3011-2023-supplement.
PM: conceptualization, data curation, formal analysis, investigation, validation, visualization, design of experiment, methodology, fieldwork and analyses, writing – original draft, writing – review and editing. MS: additional analysis, methodology, writing – review and editing. TD: writing – review and editing. GG: design of experiment, writing – review and editing.
The contact author has declared that none of the authors has any competing interests.
Publisher's note: Copernicus Publications remains neutral with regard to jurisdictional claims in published maps and institutional affiliations.
The authors thank the staff of ITP-CAS and the NAMORS research station for their hospitality and assistance during the sampling campaign. We further thank the associated members of the TransTiP team for organising the field work and for the helping hands during sampling.
This research is a contribution to the International Research Training Group “Geo-ecosystems in transition on the Tibetan Plateau (TransTiP)”, funded by the Deutsche Forschungsgemeinschaft (grant no. 317513741/GRK 2309). The funding for Michael Seidel is by the Cluster of Excellence EXC 2077 “The Ocean Floor – Earth's Uncharted Interface” (project no. 390741603). Open access publication funding for this article was received from the Carl von Ossietzky Universitát Oldenburg.
The publication of this article was funded by the open-access fund of Leibniz Universität Hannover.
This paper was edited by Ji-Hyung Park and reviewed by two anonymous referees.
Adams, H. E., Crump, B. C., and Kling, G. W.: Temperature controls on aquatic bacterial production and community dynamics in arctic lakes and streams, Environ. Microbiol., 12, 1319–1333, https://doi.org/10.1111/j.1462-2920.2010.02176.x, 2010.
Adnan, M., Kang, S., Zhang, G., Saifullah, M., Anjum, M. N., and Ali, A. F.: Simulation and Analysis of the Water Balance of the Nam Co Lake Using SWAT Model, Water, 11, 1383, https://doi.org/10.3390/w11071383, 2019a.
Adnan, M., Kang, S. C., Zhang, G. S., Anjum, M. N., Zaman, M., and Zhang, Y. Q.: Evaluation of SWAT Model performance on glaciated and non-glaciated subbasins of Nam Co Lake, Southern Tibetan Plateau, China, J. Mt. Sci., 16, 1075–1097, https://doi.org/10.1007/s11629-018-5070-7, 2019b.
Anderson, M. J.: Distance-based tests for homogeneity of multivariate dispersions, Biometrics, 62, 245–253, https://doi.org/10.1111/j.1541-0420.2005.00440.x, 2006.
Anesio, A. M., Hodson, A. J., Fritz, A., Psenner, R., and Sattler, B.: High microbial activity on glaciers: importance to the global carbon cycle, Glob. Change Biol., 15, 955–960, https://doi.org/10.1111/j.1365-2486.2008.01758.x, 2009.
Anslan, S., Azizi Rad, M., Buckel, J., Echeverria Galindo, P., Kai, J., Kang, W., Keys, L., Maurischat, P., Nieberding, F., Reinosch, E., Tang, H., Tran, T. V., Wang, Y., and Schwalb, A.: Reviews and syntheses: How do abiotic and biotic processes respond to climatic variations in the Nam Co catchment (Tibetan Plateau)?, Biogeosciences, 17, 1261–1279, https://doi.org/10.5194/bg-17-1261-2020, 2020.
Asmala, E., Autio, R., Kaartokallio, H., Stedmon, C. A., and Thomas, D. N.: Processing of humic-rich riverine dissolved organic matter by estuarine bacteria: effects of predegradation and inorganic nutrients, Aquat. Sci., 76, 451–63, 2014.
Bandyopadhyay, J.: Securing the Himalayas as the Water Tower of Asia: An Environmental Perspective, Asia Policy, 16, 45–50, https://doi.org/10.1353/asp.2013.0042, 2013.
Bai, J., Ouyang, H., Xiao, R., Gao, J., Gao, H., Cui, B., and Huang L.: Spatial variability of soil carbon, nitrogen, and phosphorus content and storage in an alpine wetland in the Qinghai – Tibet Plateau, China, Soil Res., 48, 730–736, https://doi.org/10.1071/SR09171, 2010.
Benner, R., Benitez-Nelson, B., Kaiser, K., and Amon, R. M. W.: Export of young terrigenous dissolved organic carbon from rivers to the Arctic Ocean, Geophys. Res. Lett., 31, https://doi.org/10.1029/2003GL019251, 2004.
Birnbaum, Z. W.: On a use of the Mann-Whitney Statistic, in: Proceedings of the Third Berkeley Symposium on Mathematical Statistics and Probability: Held at the Statistical Laboratory, Berkeley, CA, 26–31 December 1954, edited by: Neyman J., University of California Press, Berkeley, CA, 13–18, 1956.
Bolch, T., Yao, T., Kang, S., Buchroithner, M. F., Scherer, D., Maussion, F., Huintjes, E., and Schneider, C.: A glacier inventory for the western Nyainqentanglha Range and the Nam Co Basin, Tibet, and glacier changes 1976–2009, The Cryosphere, 4, 419–433, https://doi.org/10.5194/tc-4-419-2010, 2010.
Bortz, J. and Schuster, C.: Statistik für Human- und Sozialwissenschaftler: Limitierte Sonderausgabe, 7th edn., Springer, Berlin, Heidelberg, ISBN 978-3-642-12769-4, 2010.
Connolly, C. T., Cardenas, M. B., Burkart, G. A., Spencer, R. G. M., and McClelland, J. W.: Groundwater as a major source of dissolved organic matter to Arctic coastal waters, Nat. Commun., 11, 1479, https://doi.org/10.1038/s41467-020-15250-8, 2020.
D'Andrilli, J., Junker, J. R., Smith, H. J., Scholl, E. A., and Foreman, C. M.: DOM composition alters ecosystem function during microbial processing of isolated sources, Biogeochemistry, 142, 281–298, https://doi.org/10.1007/s10533-018-00534-5, 2019.
Dexter, E., Rollwagen-Bollens, G., and Bollens, S. M.: The trouble with stress: A flexible method for the evaluation of nonmetric multidimensional scaling, Limnol. Oceanogr.-Meth., 16, 434–443, https://doi.org/10.1002/lom3.10257, 2018.
Dittmar, T. and Kattner, G.: The biogeochemistry of the river and shelf ecosystem of the Arctic Ocean: a review, Mar. Chem., 83, 3–4, 2003.
Dittmar, T., Koch, B., Hertkorn, N., and Kattner, G.: A simple and efficient method for the solid-phase extraction of dissolved organic matter (SPE-DOM) from seawater, Limnol. Oceanogr.-Meth., 6, 203–235, https://doi.org/10.4319/lom.2008.6.230, 2008.
Dixon, P.: VEGAN, a package of R functions for community ecology, J. Veget. Sci., 14, 927–930, https://doi.org/10.1111/j.1654-1103.2003.tb02228.x, 2003.
Du, Z., Wang, X., Xiang, J., Wu, Y., Zhang, B., Yan, Y., Xiaoke, Z., and Yanjiang, C.: Yak dung pat fragmentation affects its carbon and nitrogen leaching in Northern Tibet, China, Agr. Ecosyst. Environ., 310, 107301, https://doi.org/10.1016/j.agee.2021.107301, 2021.
Eklöf, K., Brömssen, C. von, Amvrosiadi, N., Fölster, J., Wallin, M. B., and Bishop, K.: Brownification on hold: What traditional analyses miss in extended surface water records, Water Res., 203, 117544, https://doi.org/10.1016/j.watres.2021.117544, 2021.
Faith, D. P., Minchin, P. R., and Belbin, L.: Compositional dissimilarity as a robust measure of ecological distance, Vegetatio, 69, 57–68, https://doi.org/10.1007/BF00038687, 1987.
Fellman, J. B., Hood, E., and Spencer, R. G. M.: Fluorescence spectroscopy opens new windows into dissolved organic matter dynamics in freshwater ecosystems: A review, Limnol. Oceanogr., 55, 2452–2462, https://doi.org/10.4319/lo.2010.55.6.2452, 2010.
Flerus, R., Lechtenfeld, O. J., Koch, B. P., McCallister, S. L., Schmitt-Kopplin, P., Benner, R., Kaiser, K., and Kattner, G.: A molecular perspective on the ageing of marine dissolved organic matter, Biogeosciences, 9, 1935–1955, https://doi.org/10.5194/bg-9-1935-2012, 2012.
Gao, J.: Wetland and Its Degradation in the Yellow River Source Zone, in: Landscape and Ecosystem Diversity, Dynamics and Management in the Yellow River Source Zone, edited by: Brierley, G., Li, X., Cullum, C., and Gao, J., Springer Geography, Springer, Cham, https://doi.org/10.1007/978-3-319-30475-5_10, 2016.
Goldberg, S. J., Ball, G. I., Allen, B. C., Schladow, S. G., Simpson, A. J., Masoom, H., Soong, R., Graven, H. D., and Aluwihare, L. I.: Refractory dissolved organic nitrogen accumulation in high-elevation lakes, Nat. Commun., 6, 6347, https://doi.org/10.1038/ncomms7347, 2015.
Goodman, K. J., Baker, M. A., and Wurtsbaugh, W. A.: Lakes as buffers of stream dissolved organic matter (DOM) variability: Temporal patterns of DOM characteristics in mountain stream-lake systems, J. Geophys. Res., 116, G00N02, https://doi.org/10.1029/2011JG001709, 2011.
Green, N. W., Perdue, E. M., Aiken, G. R., Butler, K. D., Chen, H., Dittmar, T., Niggemann, J., and Stubbins, A.: An intercomparison of three methods for the large-scale isolation of oceanic dissolved organic matter, Mar. Chem., 161, 14–19, https://doi.org/10.1016/j.marchem.2014.01.012, 2014.
Green, N. W., McInnis, D., Hertkorn, N., Maurice, P. A., and Perdue, E. M.: Suwannee River Natural Organic Matter: Isolation of the 2R101N Reference Sample by Reverse Osmosis, Environ. Eng. Sci., 32, 38–44, https://doi.org/10.1089/ees.2014.0284, 2015.
Guo, L. and Macdonald, R. W.: Source and transport of terrigenous organic matter in the upper Yukon River: Evidence from isotope (δ13C, Δ14C, and δ15N) composition of dissolved, colloidal, and particulate phases, Global Biogeochem. Cy., 20, GB2011, https://doi.org/10.1029/2005GB002593, 2006.
Harms, T. K., Edmonds, J. W., Genet, H., Creed, I. F., Aldred, D., Balser, A., and Jones, J. B.: Catchment influence on nitrate and dissolved organic matter in Alaskan streams across a latitudinal gradient, J. Geophys. Res.-Biogeo., 121, 350–369, 2016.
Harris, R. B.: Rangeland degradation on the Qinghai-Tibetan plateau: A review of the evidence of its magnitude and causes, J. Arid Environ., 74, 1–12, https://doi.org/10.1016/j.jaridenv.2009.06.014, 2010.
Hawkes, J. A., D'Andrilli, J., Agar, J. N., Barrow, M. P., Berg, S. M., Catalán, N., Chen, H., Chu, R. K., Cole, R. B., Dittmar, T., Gavard, R., Gleixner, G., Hatcher, P. G., He, C., Hess, N. J., Hutchins, R. H. S., Ijaz, A., Jones, H. E., Kew, W., Khaksari, M., Palacio Lozano, D. C., Lv, J., Mazzoleni, L. R., Noriega‐Ortega, B. E., Osterholz, H., Radoman, N., Remucal, C. K., Schmitt, N. D., Schum, S. K., Shi, Q., Simon, C., Singer, G., Sleighter, R. L., Stubbins, A., Thomas, M. J., Tolic, N., Zhang, S., Zito, P., and Podgorski, D. C.: An international laboratory comparison of dissolved organic matter composition by high resolution mass spectrometry: Are we getting the same answer?m Limnol. Oceanogr.-Meth., 18, 235–258, https://doi.org/10.1002/lom3.10364, 2020.
Helms, J. R., Mao, J., Stubbins, A., Schmidt-Rohr, K., Spencer, R.G. M., Hernes, P. J., and Mopper, K.: Loss of optical and molecular indicators of terrigenous dissolved organic matter during long-term photobleaching, Aquat. Sci., 76, 353–373, https://doi.org/10.1007/s00027-014-0340-0, 2014.
Hodson, A., Anesio, A. M., Tranter, M., Fountain, A., Osborn, M., Priscu, J., Laybourn-Parry, J., and Sattler, B.: GLACIAL ECOSYSTEMS, Ecol. Monogr., 78, 41–67, https://doi.org/10.1890/07-0187.1, 2008.
Hoikkala, L., Kortelainen, P., Soinne, H., and Kuosa, H.: Dissolved organic matter in the Baltic Sea, J. Marine Syst., 142, 47–61, https://doi.org/10.1016/j.jmarsys.2014.10.005, 2015.
Hood, E., Fellman, J., Spencer, R. G. M., Hernes, P. J., Edwards, R., D’Amore, D., and Scott, D.: Glaciers as a source of ancient and labile organic matter to the marine environment, Nature, 462, 1044–1047, https://doi.org/10.1038/nature08580, 2009.
Hopping, K. A., Knapp, A. K., Dorji, T., and Klein, J. A.: Warming and land use change concurrently erode ecosystem services in Tibet, Glob. Change Biol., 24, 5534–5548, https://doi.org/10.1111/gcb.14417, 2018.
Hu, E., He, H., Su, Y., Jeppesen, E., and Liu Z.: Use of Multi-Carbon Sources by Zooplankton in an Oligotrophic Lake in the Tibetan Plateau, Water, 8, 565, https://doi.org/10.3390/w8120565, 2016.
Jolliffe, I. T.: Principal Component Analysis, Springer-Verlag New York Inc, New York, NY, https://doi.org/10.1007/b98835, 2002.
Keil, A., Berking, J., Mügler, I., Schütt, B., Schwalb, A., and Steeb, P.: Hydrological and geomorphological basin and catchment characteristics of Lake Nam Co, South-Central Tibet, Quatern. Int., 218, 118–130, https://doi.org/10.1016/j.quaint.2009.02.022, 2010.
Kaiser, K., Miehe, G., Barthelmes, A., Ehrmann, O., Scharf, A., Schult, M., Schlütz, F., Adamczyk, S., and Frenzel, B.: Turf-bearing topsoils on the central Tibetan Plateau, China: Pedology, botany, geochronology, CATENA, 73, 300–311, 2008.
Koch, B. P. and Dittmar, T.: From mass to structure: an aromaticity index for high-resolution mass data of natural organic matter, Rapid Commun. Mass Sp., 20, 926–932, https://doi.org/10.1002/rcm.2386, 2006.
Koch, B. P. and Dittmar, T.: From mass to structure: an aromaticity index for high-resolution mass data of natural organic matter. Rapid Commun. Mass Sp., 30, 250–250, https://doi.org/10.1002/rcm.7433, 2016.
Lechtenfeld, O. J., Kattner, G., Flerus, R., McCallister, S. L., Schmitt-Kopplin, P., and Koch, B. P.: Molecular transformation and degradation of refractory dissolved organic matter in the Atlantic and Southern Ocean, Geochim. Cosmochim. Ac., 126, 321–37, 2014.
Leyva, D., Jaffe, R., and Fernandez-Lima, F.: Structural Characterization of Dissolved Organic Matter at the Chemical Formula Level Using TIMS-FT-ICR MS/MS, Anal. Chem., 92, 11960–11966, https://doi.org/10.1021/acs.analchem.0c02347, 2020.
Li, Y., Xiao, K., Du, J., Han, B., Liu, Q., Niu, H., Ren, W., Tan, J., and Wang, Y.: Spectroscopic fingerprints to track the fate of aquatic organic matter along an alpine headstream on the Tibetan Plateau, Sci. Total Environ., 792, 148376, https://doi.org/10.1016/j.scitotenv.2021.148376, 2021.
Liu, S., Schleuss, P.-M., and Kuzyakov, Y.: Carbon and Nitrogen Losses from Soil Depend on Degradation of Tibetan Kobresia Pastures, Land Degrad. Dev., 28, 1253–62, 2017.
Liu, S., He, Z., Tang, Z., Liu, L., Hou, J., Li, T., Zhang, Y., Shi, Q., Giesy, J. P., and Wu, F.: Linking the molecular composition of autochthonous dissolved organic matter to source identification for freshwater lake ecosystems by combination of optical spectroscopy and FT-ICR-MS analysis, Sci. Total Environ., 703, 134764, https://doi.org/10.1016/j.scitotenv.2019.134764, 2020.
Lu, Y., Li, X., Mesfioui, R., Bauer, J. E., Chambers, R. M., Canuel, E. A., and Hatcher, P.G.: Use of ESI-FTICR-MS to Characterize Dissolved Organic Matter in Headwater Streams Draining Forest-Dominated and Pasture-Dominated Watersheds, PLOS ONE, 10, e0145639, https://doi.org/10.1371/journal.pone.0145639, 2015.
Ma, W., Alhassan, A. R. M., Wang, Y., Li, G., Wang, H., and Zhao, J.: Greenhouse gas emissions as influenced by wetland vegetation degradation along a moisture gradient on the eastern Qinghai–Tibet Plateau of North-West China, Nutr. Cycl. Agroecosyst., 112, 335–354, https://doi.org/10.1007/s10705-018-9950-6, 2018.
Mann, B. F., Chen, H., Herndon, E. M., Chu, R. K., Tolic, N., Portier, E. F., Roy Chowdhury, T., Robinson, E. W., Callister, S. J., Wullschleger, S. D., Graham, D. E., Liang, L., and Gu, B.: Indexing Permafrost Soil Organic Matter Degradation Using High-Resolution Mass Spectrometry, PLOS ONE, 10, e0130557, https://doi.org/10.1371/journal.pone.0130557, 2015
Maurischat, P., Lehnert, L., Zerres, V. H. D., Tran, T. V., Kalbitz, K., Rinnan, Å., Li, X. G., Dorji, T., and Guggenberger, G.: The glacial–terrestrial–fluvial pathway: A multiparametrical analysis of spatiotemporal dissolved organic matter variation in three catchments of Lake Nam Co, Tibetan Plateau, Sci. Total Environ., 838, 156542, https://doi.org/10.1016/j.scitotenv.2022.156542, 2022a.
Maurischat, P., Seidel, M., Dittmar, T., and Guggenberger, G.: Dataset – Dissolved organic matter sources and processing in the endorheic Lake Nam Co catchment (Tibet) as assessed by ultra-high resolution Fourier transform ion cyclotron resonance mass spectrometry (FT-ICR MS), Zenodo [data set], https://doi.org/10.5281/zenodo.5816680, 2022b.
Medeiros, P. M., Seidel, M., Niggemann, J., Spencer, R. G. M., Hernes, P. J., Yager, P. L., Miller, W. L., Dittmar, T., and Hansell, D. A.: A novel molecular approach for tracing terrigenous dissolved organic matter into the deep ocean, Global Biogeochem. Cy., 30, 689–699, https://doi.org/10.1002/2015GB005320, 2016.
Merder, J., Freund, J. A., Feudel, U., Hansen, C. T., Hawkes, J. A., Jacob, B., Klaproth, K., Niggemann, J., Noriega-Ortega, B. E., Osterholz, H., Rossel, P. E., Seidel, M., Singer, G., Stubbins, A., Waska, H., and Dittmar, T.: ICBM-OCEAN: Processing Ultrahigh-Resolution Mass Spectrometry Data of Complex Molecular Mixtures, Anal. Chem. 92, 6832–6838, https://doi.org/10.1021/acs.analchem.9b05659, 2020.
Miehe, G., Miehe, S., Kaiser, K., Jianquan, L., and Zhao, X.: Status and Dynamics of the Kobresia pygmaea Ecosystem on the Tibetan Plateau, AMBIO, 37, 272–279, https://doi.org/10.1579/0044-7447(2008)37[272:SADOTK]2.0.CO;2, 2008.
Miehe, G., Schleuss, P.-M., Seeber, E., Babel, W., Biermann, T., Braendle, M., Chen, F., Coners, H., Foken, T., Gerken, T., Graf, H.-F., Guggenberger, G., Hafner, S., Holzapfel, M., Ingrisch, J., Kuzyakov, Y., Lai, Z., Lehnert, L., Leuschner, C., Li, X., Liu, J., Liu, S., Ma, Y., Miehe, S., Mosbrugger, V., Noltie, H. J., Schmidt, J., Spielvogel, S., Unteregelsbacher, S., Wang, Y., Willinghöfer, S., Xu, X., Yang, Y., Zhang, S., Opgenoorth, L., and Wesche, K.: The Kobresia pygmaea ecosystem of the Tibetan highlands – Origin, functioning and degradation of the world's largest pastoral alpine ecosystem: Kobresia pastures of Tibet. Sci. Total Environ., 648, 754–71, https://doi.org/10.1016/j.scitotenv.2018.08.164, 2019.
Nieberding, F., Wille, C., Ma, Y., Wang, Y., Maurischat, P., Lehnert, L., and Sachs, T.: Winter daytime warming and shift in summer monsoon increase plant cover and net CO 2 uptake in a central Tibetan alpine steppe ecosystem, J. Geophys. Res.-Biogeo., 126, e2021JG006441, https://doi.org/10.1029/2021JG006441, 2021.
Osterholz, H., Turner, S., Alakangas, L. J., Tullborg, E.-L., Dittmar, T., Kalinowski, B. E., and Dopson, M.: Terrigenous dissolved organic matter persists in the energy-limited deep groundwaters of the Fennoscandian Shield, Nat. Commun., 13, 4837, https://doi.org/10.1038/s41467-022-32457-z, 2022.
Pohlabeln, A. M., Gomez-Saez, G. V., Noriega-Ortega, B. E., and Dittmar, T.: Experimental Evidence for Abiotic Sulfurization of Marine Dissolved Organic Matter, Frontiers in Marine Science, 4, 364, https://doi.org/10.3389/fmars.2017.00364, 2017.
Qiu, J.: China: The third pole, Nature, 454, 393–396, https://doi.org/10.1038/454393a, 2008.
Qu, B., Zhang, Y., Kang, S., and Sillanpää, M.: Water quality in the Tibetan Plateau: Major ions and trace elements in rivers of the “Water Tower of Asia”. Sci. Total Environ., 649, 571–81, 2019.
R Core Team: R: A language and environment for statistical computing, R Foundation for Statistical Computing, Vienna, Austria, 2013.
Riedel, T. and Dittmar, T.: A method detection limit for the analysis of natural organic matter via Fourier transform ion cyclotron resonance mass spectrometry, Anal. Chem., 86, 8376–8382, https://doi.org/10.1021/ac501946m, 2014.
Roebuck, J. A., Seidel, M., Dittmar, T., and Jaffé, R.: Land Use Controls on the Spatial Variability of Dissolved Black Carbon in a Subtropical Watershed, Environ. Sci. Technol., 52, 8104–8114, https://doi.org/10.1021/acs.est.8b00190, 2018.
Roebuck, J. A., Seidel, M., Dittmar, T., and Jaffé, R.: Controls of Land Use and the River Continuum Concept on Dissolved Organic Matter Composition in an Anthropogenically Disturbed Subtropical Watershed. Environ. Sci. Technol., 54, 198–206, https://doi.org/10.1021/acs.est.9b04605, 2020.
Ruediger, S.: Siberian River Run-Off in the Kara Sea: Characterisation, Quantification, Variability and Environmental Significance, Elsevier, Amsterdam, ISBN13 978-0444513656, 2003.
Sankar, M. S., Dash, P., Lu, Y., Mercer, A. E., Turnage, G., Shoemaker, C. M., Chen, S., and Moorhead, R. J.: Land use and land cover control on the spatial variation of dissolved organic matter across 41 lakes in Mississippi, USA, Hydrobiologia, 847, 1159–1176, 2020.
šantl-Temkiv, T., Finster, K., Dittmar, T., Hansen, B. M., Thyrhaug, R., Nielsen, N. W., and Karlson, U. G.: Hailstones: a window into the microbial and chemical inventory of a storm cloud, PLOS ONE, 8, e53550, https://doi.org/10.1371/journal.pone.0053550, 2013.
Seidel, M., Yager, P. L., Ward, N. D., Carpenter, E. J., Gomes, H. R., Krusche, A. V., Richey, J. E., Dittmar, T., and Medeiros, P. M.: Molecular-level changes of dissolved organic matter along the Amazon River-to-ocean continuum, Mar. Chem., 177, 218–231, https://doi.org/10.1016/j.marchem.2015.06.019, 2015.
Seidel, M., Manecki, M., Herlemann, D. P. R., Deutsch, B., Schulz-Bull, D., Jürgens, K., and Dittmar, T.: Composition and Transformation of Dissolved Organic Matter in the Baltic Sea, Front. Earth Sci., 5, G01004, https://doi.org/10.3389/feart.2017.00031, 2017.
Seifert, A.-G., Roth, V.-N., Dittmar, T., Gleixner, G., Breuer, L., Houska, T., and Marxsen, J.: Comparing molecular composition of dissolved organic matter in soil and stream water: Influence of land use and chemical characteristics. Sci. Total Environ., 571, 145–152, https://doi.org/10.1016/j.scitotenv.2016.07.033, 2016.
Singer, G. A., Fasching, C., Wilhelm, L., Niggemann, J., Steier, P., Dittmar, T., and Battin, T. J.: Biogeochemically diverse organic matter in Alpine glaciers and its downstream fate, Nat. Geosci., 5, 710–714, https://doi.org/10.1038/ngeo1581, 2012.
Spencer, R. G. M., Stubbins, A., Hernes, P. J., Baker, A., Mopper, K., Aufdenkampe, A. K., Dyda, R. Y., Mwamba, V. L., Mangangu, A. M., Wabakanghanzi, J. N., and Six, J.: Photochemical degradation of dissolved organic matter and dissolved lignin phenols from the Congo River, J. Geophys. Res., 114, G03010, https://doi.org/10.1029/2009JG000968, 2009.
Spencer, R. G. M., Guo, W., Raymond, P. A., Dittmar, T., Hood, E., Fellman, J., and Stubbins, A.: Source and biolability of ancient dissolved organic matter in glacier and lake ecosystems on the Tibetan Plateau, Geochim. Cosmochim. Ac., 142, 64–74, https://doi.org/10.1016/j.gca.2014.08.006, 2014.
Takeuchi, N.: Optical characteristics of cryoconite (surface dust) on glaciers: the relationship between light absorbency and the property of organic matter contained in the cryoconite, Ann. Glaciol., 34, 409–414, https://doi.org/10.3189/172756402781817743, 2002.
Telling, J., Anesio, A. M., Tranter, M., Irvine-Fynn, T., Hodson, A., Butler, C., and Wadham, J.: Nitrogen fixation on Arctic glaciers, Svalbard, J. Geophys. Res., 116, G03039, https://doi.org/10.1029/2010JG001632, 2011.
Thukral, A. K.: A review on measurement of Alpha diversity in biology, Int. J. Contemp. Microbiol., 54, 1–10, https://doi.org/10.5958/2395-146X.2017.00001.1, 2017.
Tran, T. V., Buckel, J., Maurischat, P., Tang, H., Yu, Z., Hördt, A., Guggenberger, G., Zhang, F., Schwalb, A., and Graf, T.: Delineation of a Quaternary Aquifer Using Integrated Hydrogeological and Geophysical Estimation of Hydraulic Conductivity on the Tibetan Plateau, China, Water, 13, 1412, https://doi.org/10.3390/w13101412, 2021.
van Dongen, B. E., Zencak, Z., and Gustafsson, Ö.: Differential transport and degradation of bulk organic carbon and specific terrestrial biomarkers in the surface waters of a sub-arctic brackish bay mixing zone, Mar. Chem., 112, 203–214, https://doi.org/10.1016/j.marchem.2008.08.002, 2008.
Vähätalo, A. V. and Wetzel, R. G.: Photochemical and microbial decomposition of chromophoric dissolved organic matter during long (months–years) exposures, Mar. Chem., 89, 313–326, 2004.
Vega, E. N., Bastidas Navarro, M., and Modenutti, B.: Goose and hare faeces as a source of nutrients and dissolved organic matter for bacterial communities in the newly formed proglacial lake Ventisquero Negro (Patagonia, Argentina), Hydrobiologia, 847, 1479–1489, https://doi.org/10.1007/s10750-020-04202-4, 2020.
Wang, H., Zhou, X., Wan, C., Fu, H., Zhang, F., and Ren, J.: Eco-environmental degradation in the northeastern margin of the Qinghai–Tibetan Plateau and comprehensive ecological protection planning, Environ. Geol., 55, 1135–1147, 2008.
Wang, J., Huang, L., Ju, J., Daut, G., Ma, Q., Zhu, L., Haberzettl, T., Baade, J., Mäusbacher, R., Hamilton, A., Graves, K., Olsthoorn, J., and Laval, B. E.: Seasonal stratification of a deep, high-altitude, dimictic lake: Nam Co, Tibetan Plateau, J. Hydrol., 584, 124668, https://doi.org/10.1016/j.jhydrol.2020.124668, 2020.
Wang, L., Zhang, L., Cai, W.-J., Wang, B., and Yu, Z.: Consumption of atmospheric CO2 via chemical weathering in the Yellow River basin: The Qinghai–Tibet Plateau is the main contributor to the high dissolved inorganic carbon in the Yellow River, Chem. Geol., 430, 34–44, 2016.
Wickham, H., Averick, M., Bryan, J., Chang, W., McGowan, L., François, R., Grolemund, G., Hayes, A., Henry, L., Hester, J., Kuhn, M., Pedersen, T., Miller, E., Bache, S., Müller, K., Ooms, J., Robinson, D., Seidel, D., Spinu, V., Takahashi, K., Vaughan, D., Wilke, C., Woo, K., and Yutani, H.: Welcome to the Tidyverse, JOSS, 4, 1686, https://doi.org/10.21105/joss.01686, 2019.
Wilson, H. F. and Xenopoulos, M. A.: Effects of agricultural land use on the composition of fluvial dissolved organic matter, Nat. Geosci., 2, 37–41, https://doi.org/10.1038/ngeo391, 2009.
Wu, X., Zhang, W., Liu, G., Yang, X., Hu, P., Chen, T., Zhang, G., and Li, Z.: Bacterial diversity in the foreland of the Tianshan No. 1 glacier, China, Ann. Glaciol., 7, 14038, https://doi.org/10.1088/1748-9326/7/1/014038, 2012.
Yao, T., Thompson, L., Yang, W., Yu, W., Gao, Y., Guo, X., Yang, X., Duan, K., Zhao, H., Xu, B., Pu, J., Lu, A., Xiang, Y., Kattel, D. B., and Joswiak, D.: Different glacier status with atmospheric circulations in Tibetan Plateau and surroundings, Nat. Clim. Change, 2, 663–667, https://doi.org/10.1038/nclimate1580, 2012.
Yao, T., Xue, Y., Chen, D., Chen, F., Thompson, L., Cui, P., Koike, T., Lau, W. K.-M., Lettenmaier, D., Mosbrugger, V., Zhang, R., Xu, B., Dozier, J., Gillespie, T., Gu, Y., Kang, S., Piao, S., Sugimoto, S., Ueno, K., Wang, L., Wang, W., Zhang, F., Sheng, Y., Guo, W., Ailikun, Yang, X., Ma, Y., Shen, S. S. P., Su, Z., Chen, F., Liang, S., Liu, Y., Singh, V. P., Yang, K., Yang, D., Zhao, X., Qian, Y., Zhang, Y., and Li, Q.: Recent Third Pole’s Rapid Warming Accompanies Cryospheric Melt and Water Cycle Intensification and Interactions between Monsoon and Environment: Multidisciplinary Approach with Observations, Modeling, and Analysis, B. Am. Meteorol. Soc., 100, 423–444, https://doi.org/10.1175/BAMS-D-17-0057.1, 2019.
Yoo, H.-J., Choi, Y.-J., and Cho, K.: Characterization of Natural Organic Matter in Spring Water, Mass Spectrometry Letters, 11, 90–94, https://doi.org/10.5478/MSL.2020.11.4.90, 2020.
Yu, Z., Wu, G., Li, F., Chen, M., Vi Tran, T., Liu, X., and Gao, S.: Glaciation enhanced chemical weathering in a cold glacial catchment, western Nyaingêntanglha Mountains, central Tibetan Plateau, J. Hydrol., 597, 126197, https://doi.org/10.1016/j.jhydrol.2021.126197, 2021.
Zhang, Y., Wang, G., and Wang, Y.: Changes in alpine wetland ecosystems of the Qinghai–Tibetan plateau from 1967 to 2004, Environ. Monit. Assess., 180, 189–199, 2011.
Zhang, Z., Qin, J., Sun, H., Yang, J., and Liu, Y.: Spatiotemporal Dynamics of Dissolved Organic Carbon and Freshwater Browning in the Zoige Alpine Wetland, Northeastern Qinghai–Tibetan Plateau, Water, 12, 2453, https://doi.org/10.3390/w12092453, 2020.