the Creative Commons Attribution 4.0 License.
the Creative Commons Attribution 4.0 License.
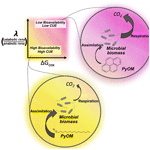
Potential bioavailability of representative pyrogenic organic matter compounds in comparison to natural dissolved organic matter pools
Hyun-Seob Song
Samantha Grieger
Vanessa A. Garayburu-Caruso
James C. Stegen
Kevin D. Bladon
Allison N. Myers-Pigg
Pyrogenic organic matter (PyOM) from wildfires impacts river corridors globally and is widely regarded as resistant to biological degradation. Though recent work suggests PyOM may be more bioavailable than historically perceived, estimating bioavailability across its chemical spectrum remains elusive. To address this knowledge gap, we assessed potential bioavailability of representative PyOM compounds relative to ubiquitous dissolved organic matter (DOM) with a substrate-explicit model. The range of potential bioavailability of PyOM was greater than natural DOM; however, the predicted thermodynamics, metabolic rates, and carbon use efficiencies (CUEs) overlapped significantly between all OM pools. Compound type (e.g., natural versus PyOM) had approximately 6-fold less impact on predicted respiration rates than simulated carbon and oxygen limitations. Within PyOM, the metabolism of specific chemistries differed strongly between unlimited and oxygen-limited conditions – degradations of anhydrosugars, phenols, and polycyclic aromatic hydrocarbons (PAHs) were more favorable under oxygen limitation than other molecules. Notably, amino sugar-like, protein-like, and lignin-like PyOM had lower carbon use efficiencies relative to natural DOM of the same classes, indicating potential impacts in process-based model representations. Overall, our work illustrates how similar PyOM bioavailability may be to that of natural DOM in the river corridor, furthering our understanding of how PyOM may influence riverine biogeochemical cycling.
- Article
(1329 KB) - Full-text XML
-
Supplement
(4434 KB) - BibTeX
- EndNote
Wildfires have burned an average of 1.8×106 ha yr−1 in the United States alone over the past 80 years, dramatically impacting terrestrial and aquatic ecosystems (Bladon et al., 2014; Shakesby and Doerr, 2006; Randerson et al., 2006; Verma and Jayakumar, 2012). As wildfire activity continues to increase in response to climate change (Pierce et al., 2004; Bowman et al., 2020; Flannigan et al., 2009), its impact on river corridor biogeochemistry is receiving significant attention (Wagner et al., 2018; Abney et al., 2019).
Pyrogenic organic matter (PyOM) generated by wildfires influences river corridor biogeochemistry due to the importance of organic matter as a carbon (C) and energy source. Though there is substantial uncertainty in the quantification of PyOM, in part due to its chemical diversity, estimates suggest that 116–385 Tg of condensed PyOM is generated per year. This amounts to 300 to 500 Tg C stored in sediments, soils, and waters (Jaffé et al., 2013; Dittmar et al., 2012; Hockaday et al., 2007; Santin et al., 2016) and ∼10 % of dissolved organic C pools in surface waters (Jaffé et al., 2013). Given that organic matter drives aquatic biogeochemical cycles, the loading of PyOM into river corridors has the potential to produce substantial impacts on ecosystem functions and downstream drinking water treatability (Emelko et al., 2011; Hohner et al., 2017).
While some estimates place aquatic residence times at thousands of years (Meyer and Wells, 1997; Elliott and Parker, 2001; Bigio et al., 2010; Kuzyakov et al., 2014), recent work has shown that PyOM may be more bioavailable (i.e., biolabile or able to be accessed and degraded by microorganisms) than previously thought (Myers-Pigg et al., 2015; Norwood et al., 2013; Zimmerman and Ouyang, 2019). This inference is also supported by research on biochars, highlighting the diverse reactivities of combustion byproducts (Sohi et al., 2010; Mia et al., 2017). PyOM bioavailability may, therefore, play an unrecognized role in global biogeochemical cycles and climate feedback. Yet, we are just beginning to understand its potential bioavailability (Zimmerman and Mitra, 2017; Wozniak et al., 2020; Masiello, 2004).
We used a substrate-explicit model to assess the potential bioavailability of PyOM in comparison to dissolved organic matter (DOM) from global surface waters and sediments. We derived 16 971 representative PyOM compounds from primary literature describing plant charcoals, biochar, smoke, burned soil, and their leachates (Table S1 in the Supplement). Natural DOM pool composition was derived from a recent high-resolution survey of river corridor sediments and surface waters (Garayburu-Caruso et al., 2020a; Fig. S1 in the Supplement). The substrate-explicit model used molecular formulae to predict energetic content, metabolic efficiency, and rates of aerobic metabolism for organic substrates. It has proven useful in linking DOM composition to aerobic metabolism in natural environments (Song et al., 2020; Graham et al., 2017; Garayburu-Caruso et al., 2020b). It provides a systematic way to formulate reaction kinetics and is agnostic of many factors that have complicated a universal understanding of organic matter bioavailability; these factors include molecular structure, chemical inhibition, mineral associations and physical protection, terminal electron acceptors, microbial community composition and accessibility, and abiotic reactions (reviewed in Arndt et al., 2013). We hypothesize that PyOM compounds have a greater similarity in their potential bioavailability to natural DOM pools than expected based on historical literature.
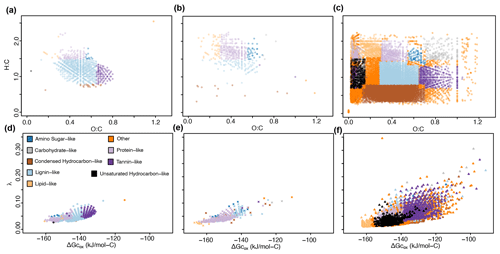
Figure 1Comparison of natural DOM and PyOM. (a–c) Van Krevelen diagrams of molecules that were present in at least 95 % of (a) surface water or (b) sediment samples versus (c) representative PyOM. (d–f) Distribution of energy content (ΔGCox) versus metabolic efficiency (λ) in (d) surface water and (e) sediment DOM versus (f) representative PyOM. Higher values on the x axis (ΔGCox) represent less energetically favorable molecules, while higher values on the y axis (λ) represent higher rates of catabolism relative to anabolism. Colors for all panels indicate inferred chemical classes.
Our model-based approach enabled us to directly compare known combustion products to thousands of ubiquitous DOM compounds, which would have been unfeasible to directly assess in a laboratory setting. Key outputs from the model include lambda (λ, the number of catabolic reactions that must occur to provide the energy required for the synthesis of 1 mol of biomass C), Gibbs free energy of C oxidation (ΔGCox) under standard conditions with a modification to pH 7, carbon use efficiency (CUE), and the rate of aerobic metabolism (as oxygen consumed per mole of C biomass produced) under three scenarios: (a) no limitations, (b) C limitation, and (c) oxygen (O) limitation. Though the relative magnitude of these metrics will vary based on the specific stoichiometry of a molecule, highly bioavailable compounds are indicated by low λ and ΔGCox coinciding with high CUE and metabolic rates. Low λ values denote efficient energetics of catabolism in producing biomass through anabolism. Low ΔGCox denotes high thermodynamic favorability in an electron donor half reaction associated with organic matter, and high CUE reflects more C assimilated into biomass per unit C respired.
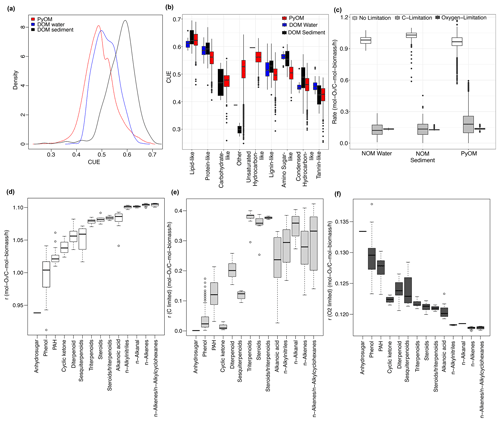
Figure 2Predicted CUE and metabolism of DOM and PyOM. Panel (a) shows the probability density function (PDF) of CUE in surface water DOM (blue), sediment DOM (black), and PyOM (red). The PDF reflects the relative likelihood that the value of a random sample drawn from a particular group would equal the value on the x axis. Panel (b) depicts CUE of surface water DOM, sediment DOM, and PyOM by inferred chemical class. Panel (c) depicts the predicted metabolism of surface water DOM, sediment DOM, and PyOM under no elemental limitations (no fill), C limitation (light gray fill), and oxygen limitation (dark gray fill). Panels (d–f) represent metabolic rates for PyOM molecules with known chemical classes under (d) no elemental limitations, (e) C limitation, and (f) oxygen limitation. For boxplots, median values are denoted by a bar, hinges correspond to the first and third quartiles, and whiskers extend to the largest/smallest value no further than 1.5 times the interquartile range from the hinge. Data beyond the end of the whiskers are plotted individually.
2.1 Potential bioavailability of pyrogenic organic matter
Previous work has shown that sediment DOM and surface water DOM are altered by wildfires (Cawley et al., 2018; Jaffé et al., 2013; Wagner et al., 2018), and our results suggest that these chemically distinct PyOM pools have similar potential bioavailability more natural to DOM. We found that the ranges of ΔGCox, λ, and CUE overlapped substantially between PyOM and DOM in sediments and surface waters (Figs. 1, 2a–b, S2). Predicted CUE of PyOM classes was also comparable to literature values reported by others (Saifuddin et al., 2019; Domeignoz-Horta et al., 2020; Pold et al., 2020). While λ, ΔGCox, and CUE were significantly different when comparing all three groups (ANOVA, P<0.001, F=16.12, 604.9, and 461.2, respectively), λ did not vary significantly between sediment DOM and PyOM (Tukey HSD (honestly significant difference), P=0.21) and was more similar between sediment DOM and PyOM than between surface water and sediment DOM pools (mean difference: surface water DOM–sediment DOM = 0.0025, sediment DOM − PyOM = 0.0019). The range of all PyOM metrics was greater than that of surface water DOM or sediment DOM, reflecting the known heterogenous nature of PyOM chemistries. Though λ of many PyOM compounds was higher than the maximum observed in either DOM pool, minimum values of λ were lowest for PyOM (surface water DOM = 0.026, sediment DOM = 0.015, PyOM = 0.010), indicating that a portion of wildfire-derived molecules may be more bioavailable than extant DOM pools. It is also interesting that surface water DOM and sediment DOM had greater dissimilarity in ΔGCox and CUE than any comparison involving PyOM. For example, the mean differences in ΔGCox and CUE between surface water and sediment DOM pools were 7.34 kJ mol C and 0.058, respectively. The differences between PyOM and surface water DOM were 4.28 kJ mol C for ΔGCox and 0.024 for CUE. Overall, statistical differences were not surprising, given an extremely large sample size (sediment n=398, surface water n=811, PyOM n=16 971), and the low effect sizes denote that differences in bioavailability between PyOM and DOM were minimal despite statistical separation.
These results signal a strong overlap between the potential bioavailabilities of PyOM and DOM pools; however, within PyOM compounds, there was variability in ΔGCox, λ, and CUE consistent with heterogeneous chemistries (Figs. 1 and 2). This is not surprising, given the diversity of PyOM molecules generated by wildfires of different burn severities and source materials (Wagner et al., 2015, 2018; Neary et al., 2005), some of which are chemically similar to natural DOM.
Interestingly, the large overlaps in predicted CUE across PyOM and DOM pools suggest that PyOM decomposition in rivers could emit proportionally similar amounts of CO2 to natural DOM (Fig. 2a–b). CUE is used in many microbially explicit decomposition models to constrain organic matter bioavailability (reviewed in Graham and Hofmockel, 2022). Therefore, predicted CUE offers a path for assimilating PyOM in microbially explicit models. Such an approach could be used to directly evaluate the impact of PyOM on global C cycles and help lead to better incorporation of PyOM impacts in models (Santin et al., 2020). We specifically highlight lower CUE of amino sugar-like, protein-like, and lignin-like PyOM in comparison to corresponding classes of natural DOM, pointing to potential shifts needed in the representation of these classes in process-based models in ecosystems impacted by wildfires.
2.2 Inferred metabolism of pyrogenic organic matter
Predicted rates of PyOM metabolism were also similar to DOM pools (Fig. 2c), reinforcing comparable bioavailability. Elemental limitations had a ∼6-fold larger impact on predicted metabolic rates than changes in compound type (e.g., surface water DOM, sediment DOM, and PyOM). Metabolic rates averaged across all compound types were 0.965, 0.179, and 0.136 mol O mol C−1 biomass produced for unlimited, C-limited, and O-limited conditions, respectively. In contrast, when metabolic rates were averaged across elemental limitations within each compound group, we found that surface water DOM, sediment DOM, and PyOM had nearly identical mean predicted rates of metabolism (ANOVA, P=0.14, F=1.922, all pairwise comparisons P>0.10). While metabolic rates within a given limitation scenario were statistically different across compound types (ANOVA, all P<0.001, F=197.4, 145.9, and 265.9), effect sizes were low in comparison to changes across elemental limitations. Still, PyOM displayed statistically elevated rates of metabolism under C and oxygen limitation versus both surface water and sediment DOM pools (Tukey HSD: P<0.001). Given that riverbed sediments can reach anoxia within millimeters of the sediment–water interface, elevated rates of PyOM metabolism under oxygen limitation may be ecologically relevant at ecosystem scales.
When considering the impact of elemental limitations on PyOM metabolism, predicted rates were strongly inhibited under low-C and low-oxygen conditions. Predicted PyOM metabolism was over 5 times lower when C or oxygen was scarce. Low decomposition rates under C and oxygen limitation could be one reason for the observed persistence of PyOM in depositional features that tend to be anoxic. It is worthwhile to note that the metabolism of all PyOM classes under low C or oxygen was predicted to be substantially slower than without elemental limitations, indicating that PyOM compounds may both actively cycle in well-oxygenated surface waters with fresh C inputs and persist over long periods of time in O-limited sediments.
When examining PyOM with known chemistries (e.g., instead of inferred chemical classes from Fourier transform ion cyclotron resonance mass spectrometry, (FTICR-MS)), anhydrosugars, phenols, and polycyclic aromatic hydrocarbons (PAHs) were less negatively impacted by oxygen limitation than any other group (Fig. 2d–f). Previous work has demonstrated that microorganisms are capable of decomposing chemically complex organic molecules, such as long-chained and/or aromatic hydrocarbons under low oxygen availability (Bushnell and Haas, 1941; Pozdnyakova, 2012; Rabus et al., 2016; Coates et al., 1997). Similar microbial metabolic pathways may also be capable of degrading pyrogenically modified phenols and PAHs in natural settings and could be investigated with future laboratory work.
Notably, our work also supports the notion that black nitrogen could be more bioavailable than other PyOM pools. While we only examined one class of PyOM molecules containing nitrogen in detail (n-alkylnitriles), it had among the highest predicted CUE and metabolic rates. We posit this may be due, in part, to its chemical structure that includes pyrrole-type moieties, which are relatively biodegradable (Knicker, 2010; De La Rosa and Knicker, 2011).
2.3 Correspondence to empirical investigations
While the substrate-explicit modeling approach used here has been validated in natural settings, we acknowledge that it does not account for all chemical and physical attributes influencing PyOM bioavailability. This includes DOM structure and size, abiotic reactions, and chemical complexation with minerals and particulates. Below, we note aspects of the model predictions that are inconsistent with experimental evidence and highlight the role of laboratory studies in evaluating PyOM bioavailability.
Compounds related to n-alkenes tended to have high modeled bioavailability despite being relatively stable in the environment (Wiesenberg et al., 2004; Smittenberg et al., 2004). These compounds are characterized by carbon–carbon double bonds, which are not considered by the model and may decrease bioavailability. However, n-alkanes generated through combustion tend to have reduced chain length in comparison to their unburned counterparts (Knicker et al., 2013), and they may thus be relatively bioavailable compared to unburned n-alkanes. Additionally, we note that previous work has shown fast degradation of combustion-derived lipids in soils (Knicker et al., 2013) as well as high n-alkene metabolism in anaerobic sediments and high lipid reactivities at the sediment–water interface (Grossi et al., 2008; Wilkes et al., 2016; Yongdong et al., 2015; Mbadinga et al., 2011; Canuel and Martens, 1996). While work on aerobic n-alkene metabolism is limited, the comparative bioavailability of n-alkenes and known degradation pathways suggests that sediment microbiomes may metabolize them as part of natural biogeochemical cycles.
Another notable discrepancy is the low potential bioavailability of anhydrosugars when compared to other PyOM compounds. Experimentally, anhydrosugars are highly bioavailable in oxic conditions, with a half-life of less than 7 d (Norwood et al., 2013). The model may therefore not adequately account for some enzyme-catalyzed reactions such as levoglucosan kinase or levoglucosan dehydrogenase that may be common enzymes in aquatic microorganisms (Bacik and Jarboe, 2016; Suciu et al., 2019).
Because of these nuances, the analysis presented here is best used as bounding estimates for experimental validation and as a holistic comparison to DOM bioavailability. Still, the span of compounds investigated here, and their comparison to DOM pools, provides a breadth of investigation that is unfeasible without model-based approaches.
Our work supports the hypothesis that PyOM may have similar overall bioavailability to natural sources of DOM and provides a foundation for targeted experiments investigating specific components of the PyOM continuum. Globally intensifying wildfires are increasing the production of PyOM with potential implications for water supplies, which are critical for domestic, industrial, agricultural, and ecological needs. Yet, many fundamental questions such as “how much” PyOM exists in ecosystems, “how fast” it cycles, and “how old” it is remain largely unknown (Abiven and Santin, 2019). Our work provides the first comprehensive computational assessment of the potential bioavailabilities of various PyOM chemistries in comparison to natural DOM pools. The comparable potential bioavailability to DOM revealed that PyOM may be actively transformed in aquatic ecosystems and may be an increasing source of C emissions to the atmosphere as the prevalence of wildfires increases.
An extended version of our methods is available in the Supplement.
To assess the potential bioavailability of PyOM, we searched primary literature for representative compounds of the PyOM continuum. Specifically, we targeted characteristic organic compounds from controlled burns of various fuel types representing a range of moisture, temperature, and oxygen conditions (Table S1). The chosen compounds focused on biomass burning alteration products derived from plant charcoals, biochars, smoke, burned soil, and their leachates, which are often used to characterize PyOM in the environment. This included compounds such as theoretical black-carbon-like compounds (defined here as condensed aromatic core structures polysubstituted with O-containing functionalities as per Wagner et al., 2017, anhydrosugars, and PAHs). The list also included compounds created and/or transformed from biomass burning, such as those derived from biopolymers like lignin (e.g., methoxyphenols), waxes (e.g., n-alkenes from thermal dehydration of n-alkanols), and resins (e.g., thermally oxidized diterpenoids) (Oros and Simoneit, 2001a, b). We also include molecular formulae assigned via FTICR-MS spectra collected on biochar leachates to increase data-type comparability with common methods used in natural organic matter studies. The selected set of compounds spans the chemical continuum of PyOM but is not intended to be exhaustive. In total, our literature search for PyOM chemistries yielded 16 971 unique molecular formulae. When a formula was found in more than one study or material type, this was noted in Table S1; however only unique formulae were considered for the model.
After generating a set of representative compounds, we used a substrate-explicit modeling framework developed by Song et al. (2020) to characterize the potential bioavailability of each compound and predict its rate of decomposition. The model uses molecular formulae to predict energetic content, metabolic efficiency, and rates of aerobic metabolism, while it does not account for structural components of organic molecules (e.g., double bonds, folding patterns, cross-linkages). This enables flexibility in application to high-throughput mass spectrometry techniques that yield chemical formulae but not structural information (e.g., FTICR-MS) for comparison to environmental DOM. Despite its limitations, the substrate-explicit model used here has proven useful in linking DOM composition to aerobic metabolism in natural environments (Song et al., 2020; Graham et al., 2017; Garayburu-Caruso et al., 2020b), and its structure is consistent with Harvey et al. (2016), who argued for the importance of thermodynamic estimates of PyOM bioavailability that underlie this model. It was chosen to allow for comparison of PyOM to the most comprehensive assessment of global aquatic DOM pools to date (Garayburu-Caruso et al., 2020a).
Briefly, the substrate-explicit model uses the elemental stoichiometry of organic molecules, based on molecular formulae, to predict the number of catabolic reactions that must occur to provide the energy required for the synthesis of 1 mol of biomass C. This quantity is described by the parameter lambda (λ) in which lower λ values denote more efficient energetics of catabolism in producing biomass through anabolism. The model also predicts the Gibbs free energy of C oxidation (ΔGCox) under standard conditions with a modification to pH 7 adjusted from LaRowe and Van Cappellen (2011) by Song et al. (2020), as well as carbon use efficiency (CUE) as defined by Saifuddin et al. (2019). Lower ΔGCox denotes higher thermodynamic favorability in an electron donor half reaction associated with organic matter, and higher CUE reflects more C assimilated into biomass per unit C respired. We also predicted the rate of aerobic metabolism (as oxygen consumed per mole C biomass produced) under three scenarios commonly observed in aquatic ecosystems: (a) C limitation, (b) oxygen (O) limitation, and (c) both C and O limitations. For more details of the substrate-explicit modeling approach used, please see Song et al. (2020). Each metric (λ, ΔGCox, CUE, metabolic rates) denotes a different aspect of potential bioavailability. Though the relative magnitude of the metrics in comparison to each other will vary based on the specific stoichiometry of a molecule, highly bioavailable compounds are indicated by low λ and ΔGCox coinciding with high CUE and metabolic rates.
Three sets of organic molecules were used as model inputs: measured global dissolved (1) surface water and (2) sediment DOM pools, extracted in H2O and analyzed by FTICR-MS as per Garayburu-Caruso et al. (2020a), and (3) literature-derived PyOM compounds as described above. Inputs to the model from the PyOM compounds were unique molecular formulae, grouped in subsequent analysis by their corresponding compound classes (Table S1). If one molecular formula was represented by several PyOM compounds (e.g., C10H16O2, which corresponds to the sesquiterpenoid cis-chrysanthemic acid and 3-, 4-substituted methylcatechol phenols), we assigned multiple compound classes to that molecular formula. Surface water and sediment DOM pools were filtered to compounds occurring in 95 % of samples to yield a dataset of globally ubiquitous DOM (surface water n=811 molecules, sediment n=398 molecules). The purpose of this filtering was to focus on widespread natural organic matter chemistries by removing compounds that were specific to subsets of rivers. Formulae assignment and inferred chemical classes via van Krevelen diagrams in DOM pools are described by Garayburu-Caruso et al. (2020a). As a whole, 16 332 compounds were found only in PyOM, 197 were found only in surface water DOM, and 167 were found only in sediment DOM. We compared modeling outputs from representative PyOM to outputs of ubiquitous DOM pools to infer relative bioavailability using ANOVA and Tukey HSD statistical tests with R software v 4.1.0 (R Core Team, 2021). Visualizations were produced using base R and “ggplot2” (Wickham, 2011). All model outputs are available in Tables S2–S4.
Code is available at https://github.com/hyunseobsong/lambda (last access: 7 June 2023, Song et al., 2023). Data describing DOM pool chemistry are published as data packages (Goldman et al., 2020; Toyoda et al., 2020) (https://doi.org/10.15485/1603775) and are discussed in more detail by Garayburu-Caruso et al. (2020a).
The supplement related to this article is available online at: https://doi.org/10.5194/bg-20-3449-2023-supplement.
EBG conceived of the paper and was responsible for writing the paper and generating all figures. HSS performed all modeling. SG determined PyOM compounds for modeling based on extensive literature review, with guidance from AMP. VAGC and JCS contributed data on and insight on DOM pool chemistry. All authors contributed to revisions.
The contact author has declared that none of the authors has any competing interests.
Publisher’s note: Copernicus Publications remains neutral with regard to jurisdictional claims in published maps and institutional affiliations.
This article is part of the special issue “The role of fire in the Earth system: understanding interactions with the land, atmosphere, and society (ESD/ACP/BG/GMD/NHESS inter-journal SI)”. It is a result of the EGU General Assembly 2020, 4–8 May 2020.
This research was supported by the US Department of Energy (DOE), Office of Biological and Environmental Research (BER), Environmental System Science (ESS) Program as part of the River Corridor Science Focus Area (SFA) at the Pacific Northwest National Laboratory (PNNL). PNNL is operated by Battelle Memorial Institute for the US Department of Energy under contract no. DE-AC05-76RL01830. This study used data from the Worldwide Hydrobiogeochemistry Observation Network for Dynamic River Systems (WHONDRS) under the River Corridor SFA at PNNL and facilitated by the US Department of Energy Environmental Molecular Sciences Laboratory User Facility.
This research has been supported by the Office of Science (grant no. DE-AC05-76RL01830).
This paper was edited by Renata Libonati and Kirsten Thonicke and reviewed by two anonymous referees.
Abiven, S. and Santin, C.: From Fires to Oceans: Dynamics of Fire-Derived Organic Matter in Terrestrial and Aquatic Ecosystems, Front. Earth Sci., 7, 31, https://doi.org/10.3389/feart.2019.00031, 2019.
Abney, R. B., Kuhn, T. J., Chow, A., Hockaday, W., Fogel, M. L., and Berhe, A. A.: Pyrogenic carbon erosion after the Rim Fire, Yosemite National Park: The role of burn severity and slope, J. Geophys. Res.-Biogeo., 124, 432–449, 2019.
Arndt, S., Jørgensen, B. B., LaRowe, D. E., Middelburg, J., Pancost, R., and Regnier, P.: Quantifying the degradation of organic matter in marine sediments: a review and synthesis, Earth Sci. Rev., 123, 53–86, 2013.
Bacik, J. P. and Jarboe, L. R.: Bioconversion of anhydrosugars: emerging concepts and strategies, IUBMB Life, 68, 700–708, 2016.
Bigio, E., Swetnam, T. W., and Baisan, C. H.: A comparison and integration of tree-ring and alluvial records of fire history at the Missionary Ridge Fire, Durango, Colorado, USA, Holocene, 20, 1047–1061, 2010.
Bladon, K. D., Emelko, M. B., Silins, U., and Stone, M.: Wildfire and the future of water supply, Environ. Sci. Technol., 48, 8936–8943, https://doi.org/10.1021/es500130g, 2014.
Bowman, D. M., Kolden, C. A., Abatzoglou, J. T., Johnston, F. H., van der Werf, G. R., and Flannigan, M.: Vegetation fires in the Anthropocene, Nat. Rev. Earth Environ., 1, 500–515, 2020.
Bushnell, L. and Haas, H.: The utilization of certain hydrocarbons by microorganisms, J. Bacteriol., 41, 653, https://doi.org/10.1128/jb.41.5.653-673.1941, 1941.
Canuel, E. A. and Martens, C. S.: Reactivity of recently deposited organic matter: Degradation of lipid compounds near the sediment-water interface, Geochim. Cosmochim. Ac., 60, 1793–1806, 1996.
Cawley, K. M., Hohner, A. K., McKee, G. A., Borch, T., Omur-Ozbek, P., Oropeza, J., and Rosario-Ortiz, F. L.: Characterization and spatial distribution of particulate and soluble carbon and nitrogen from wildfire-impacted sediments, J. Soil. Sediment., 18, 1314–1326, 2018.
Coates, J. D., Woodward, J., Allen, J., Philp, P., and Lovley, D. R.: Anaerobic degradation of polycyclic aromatic hydrocarbons and alkanes in petroleum-contaminated marine harbor sediments, Appl. Environ. Microb., 63, 3589–3593, 1997.
de la Rosa, J. M. and Knicker, H.: Bioavailability of N released from N-rich pyrogenic organic matter: an incubation study, Soil Biol. Biochem., 43, 2368–2373, 2011.
Dittmar, T., De Rezende, C. E., Manecki, M., Niggemann, J., Ovalle, A. R. C., Stubbins, A., and Bernardes, M. C.: Continuous flux of dissolved black carbon from a vanished tropical forest biome, Nat. Geosci., 5, 618–622, 2012.
Domeignoz-Horta, L. A., Pold, G., Liu, X.-J. A., Frey, S. D., Melillo, J. M., and DeAngelis, K. M.: Microbial diversity drives carbon use efficiency in a model soil, Nat. Commun., 11, 1–10, 2020.
Elliott, J. G. and Parker, R.: Developing a post-fire flood chronology and recurrence probability from alluvial stratigraphy in the Buffalo Creek watershed, Colorado, USA, Hydrol. Proc., 15, 3039–3051, 2001.
Emelko, M. B., Silins, U., Bladon, K. D., and Stone, M.: Implications of land disturbance on drinking water treatability in a changing climate: Demonstrating the need for “source water supply and protection” strategies, Water Res., 45, 461–472, 2011.
Flannigan, M. D., Krawchuk, M. A., de Groot, W. J., Wotton, B. M., and Gowman, L. M.: Implications of changing climate for global wildland fire, Int. J Wildland Fire, 18, 483–507, 2009.
Garayburu-Caruso, V. A., Danczak, R. E., Stegen, J. C., Renteria, L., Mccall, M., Goldman, A. E., Chu, R. K., Toyoda, J., Resch, C. T., and Torgeson, J. M.: Using Community Science to Reveal 85 the Global Chemogeography of River Metabolomes, Metabolites, 10, 518, https://doi.org/10.3390/metabo10120518, 2020a.
Garayburu-Caruso, V. A., Stegen, J. C., Song, H.-S., Renteria, L., Wells, J., Garcia, W., Resch, C. T., Goldman, A. E., Chu, R. K., Toyoda, J., and Graham, E. B.: Carbon limitation leads to 90 thermodynamic regulation of aerobic metabolism, Environ. Sci. Technol., 2020, 517–524, 2020b.
Goldman, A. E., Chu, R. K., Danczak, R. E., Daly, R. A., Fansler, S., Garayburu-Caruso, V. A., Graham, E. B., McCall, M. L., Ren, H., and Renteria, L.: WHONDRS Summer 2019 Sampling Campaign: Global River Corridor Sediment FTICR-MS, Dissolved Organic Carbon, Aerobic Respiration, Elemental Composition, Grain Size, Total Nitrogen and Organic Carbon Content, Bacterial Abundance, and Stable Isotopes. River Corridor and Watershed Biogeochemistry SFA, ESS-DIVE repository, [data set], https://doi.org/10.15485/1729719, 2020.
Graham, E. B. and Hofmockel, K.: Ecological stoichiometry as a foundation for omics-enabled biogeochemical models of soil organic matter decomposition, Biogeochemistry, 157, 31–50, 2022.
Graham, E. B., Tfaily, M. M., Crump, A. R., Goldman, A. E., Bramer, L. M., Arntzen, E., Romero, E., Resch, C. T., Kennedy, D. W., and Stegen, J. C.: Carbon inputs from riparian vegetation limit oxidation of physically bound organic carbon via biochemical and thermodynamic processes, J. Geophys. Res.-Biogeo., 122, 3188–3205, 2017.
Grossi, V., Cravo-Laureau, C., Guyoneaud, R., Ranchou-Peyruse, A., and Hirschler-Réa, A.: Metabolism of n-alkanes and n-alkenes by anaerobic bacteria: A summary, Org. Geochem., 39, 1197–1203, 2008.
Harvey, O. R., Myers-Pigg, A. N., Kuo, L.-J., Singh, B. P., Kuehn, K. A., and Louchouarn, P.: Discrimination in degradability of soil pyrogenic organic matter follows a return-on-energy-investment principle, Environ. Sci. Technol., 50, 8578–8585, 2016.
Hockaday, W. C., Grannas, A. M., Kim, S., and Hatcher, P. G.: The transformation and mobility of charcoal in a fire-impacted watershed, Geochim. Cosmochim. Ac., 71, 3432–3445, 2007.
Hohner, A. K., Terry, L. G., Townsend, E. B., Summers, R. S., and Rosario-Ortiz, F. L.: Water treatment process evaluation of wildfire-affected sediment leachates, Environ. Sci. Water Res. Tech, 3, 352–365, 2017.
Jaffé, R., Ding, Y., Niggemann, J., Vähätalo, A. V., Stubbins, A., Spencer, R. G., Campbell, J., and Dittmar, T.: Global charcoal mobilization from soils via dissolution and riverine transport to the oceans, Science, 340, 345–347, 2013.
Knicker, H.: “Black nitrogen” – an important fraction in determining the recalcitrance of charcoal, Org. Geochem., 41, 947–950, 2010.
Knicker, H., Hilscher, A., De la Rosa, J., González-Pérez, J. A., and González-Vila, F. J.: Modification of biomarkers in pyrogenic organic matter during the initial phase of charcoal biodegradation in soils, Geoderma, 197, 43–50, 2013.
Kuzyakov, Y., Bogomolova, I., and Glaser, B.: Biochar stability in soil: decomposition during eight years and transformation as assessed by compound-specific 14C analysis, Soil Biol. Biochem., 70, 229–236, 2014.
LaRowe, D. E. and Van Cappellen, P.: Degradation of natural organic matter: a thermodynamic analysis, Geochim. Cosmochim. Ac., 75, 2030–2042, 2011.
Masiello, C. A.: New directions in black carbon organic geochemistry, Mar. Chem., 92, 201–213, 2004.
Mbadinga, S. M., Wang, L.-Y., Zhou, L., Liu, J.-F., Gu, J.-D., and Mu, B.-Z.: Microbial communities involved in anaerobic degradation of alkanes, Int. Biodeterior., 65, 1–13, 2011.
Meyer, G. A. and Wells, S. G.: Fire-related sedimentation events on alluvial fans, Yellowstone National Park, USA, J. Sediment. Res., 67, 776–791, 1997.
Mia, S., Dijkstra, F. A., and Singh, B.: Long-term aging of biochar: a molecular understanding with agricultural and environmental implications, Adv. Agron., 141, 1–51, 2017.
Myers-Pigg, A. N., Louchouarn, P., Amon, R. M., Prokushkin, A., Pierce, K., and Rubtsov, A.: Labile pyrogenic dissolved organic carbon in major Siberian Arctic rivers: Implications for wildfire-stream metabolic linkages, Geophys. Res. Lett., 42, 377–385, 2015.
Neary, D. G., Ryan, K. C., and DeBano, L. F.: Wildland fire in ecosystems: effects of fire on soils and water, Gen. Tech. Rep. RMRS-GTR-42-vol. 4, Ogden, UT: US Department of Agriculture, Forest Service, Rocky Mountain Research Station, 42, 250 pp., 2005.
Norwood, M. J., Louchouarn, P., Kuo, L.-J., and Harvey, O. R.: Characterization and biodegradation of water-soluble biomarkers and organic carbon extracted from low temperature chars, Org. Geochem., 56, 111–119, 2013.
Oros, D. R. and Simoneit, B. R.: Identification and emission factors of molecular tracers in organic aerosols from biomass burning Part 2. Deciduous trees, Appl. Geochem., 16, 1545–1565, 2001a.
Oros, D. R. and Simoneit, B. R.: Identification and emission factors of molecular tracers in organic aerosols from biomass burning Part 1. Temperate climate conifers, Appl. Geochem., 16, 1513–1544, 2001b.
Pierce, J. L., Meyer, G. A., and Jull, A. T.: Fire-induced erosion and millennial-scale climate change in northern ponderosa pine forests, Nature 432, 87–90, https://doi.org/10.1038/nature03058, 2004.
Pold, G., Domeignoz-Horta, L. A., Morrison, E. W., Frey, S. D., Sistla, S. A., and DeAngelis, K. M.: Carbon use efficiency and its temperature sensitivity covary in soil bacteria, Mbio, 11, e02293-19, https://doi.org/10.1128/mBio.02293-19, 2020.
Pozdnyakova, N. N.: Involvement of the ligninolytic system of white-rot and litter-decomposing fungi in the degradation of polycyclic aromatic hydrocarbons, Biotech. Res. Int., 2012, 243217, https://doi.org/10.1155/2012/243217 2012.
Rabus, R., Boll, M., Heider, J., Meckenstock, R. U., Buckel, W., Einsle, O., Ermler, U., Golding, B. T., Gunsalus, R. P., and Kroneck, P. M.: Anaerobic microbial degradation of hydrocarbons: from enzymatic reactions to the environment, J. Molec. Microb. Biotech., 26, 5–28, 2016.
Randerson, J. T., Liu, H., Flanner, M. G., Chambers, S. D., Jin, Y., Hess, P. G., Pfister, G., Mack, M., Treseder, K., and Welp, L.: The impact of boreal forest fire on climate warming, Science, 314, 1130–1132, 2006.
Saifuddin, M., Bhatnagar, J. M., Segrè, D., and Finzi, A. C.: Microbial carbon use efficiency predicted from genome-scale metabolic models, Nat. Commun., 10, 1–10, 2019.
Santin, C., Doerr, S. H., Jones, M. W., Merino, A., Warneke, C., and Roberts, J. M.: The relevance of pyrogenic carbon for carbon budgets from fires: insights from the FIREX experiment, Global Biogeochem. Cy., 34, e2020GB006647, https://doi.org/10.1029/2020GB006647, 2020.
Santin, C., Doerr, S. H., Kane, E. S., Masiello, C. A., Ohlson, M., de la Rosa, J. M., Preston, C. M., and Dittmar, T.: Towards a global assessment of pyrogenic carbon from vegetation fires, Global Change Biol., 22, 76–91, 2016.
Shakesby, R. and Doerr, S.: Wildfire as a hydrological and geomorphological agent, Earth Sci. Rev., 74, 269–307, 2006.
Smittenberg, R. H., Hopmans, E. C., Schouten, S., Hayes, J. M., Eglinton, T. I., and Sinninghe Damsté, J.: Compound-specific radiocarbon dating of the varved Holocene sedimentary record of Saanich Inlet, Canada, Paleoceanography, 19, https://doi.org/10.1029/2003PA000927, 2004.
Sohi, S. P., Krull, E., Lopez-Capel, E., and Bol, R.: A review of biochar and its use and function in soil, Adv. Agron., 105, 47–82, 2010.
Song, H.-S., Stegen, J. C., Graham, E. B., Lee, J.-Y., Garayburu-Caruso, V., Nelson, W. C., Chen, X., Moulton, J. D., and Scheibe, T. D.: Representing Organic Matter Thermodynamics in Biogeochemical Reactions via Substrate-Explicit Modeling, Front. Microbiol., 11, 531756, https://doi.org/10.3389/fmicb.2020.531756, 2020.
Song, H.-S., Stegen, J. C., Graham, E. B., Lee, J.-Y., Garayburu-Caruso, V. A., Nelson, W. C., Chen, X., Moulton, J. D., and Scheibe, T. D.: Representing Organic Matter Thermodynamics in Biogeochemical Reactions via Substrate-Explicit Modeling, [code], https://github.com/hyunseobsong/lambda (last access: 13 June 2023), 2023.
Suciu, L. G., Masiello, C. A., and Griffin, R. J.: Anhydrosugars as tracers in the Earth system, Biogeochemistry, 146, 209–256, 2019.
Team, R. C.: R: A language and environment for statistical computing, R Foundation for Statistical Computing [code], https://www.r-project.org/ (last access: 30 May 2023), 2021.
Toyoda, J. G., Goldman, A. E., Arnon, S., Bar-Zeev, E., Chu, R. K., Danczak, R. E., Daly, R. A., Forbes, B., Garayburu-Caruso, V. A., Graham, E. B., Lin, X., Moran, J. J., Ren, H., Renteria, L., Resch, C. T., Tfaily, M., Tolic, N., Torgeson, J. M., Wells, J., Wrighton, K. C., and Stegen, J. C.: WHONDRS Consortium T (2020): WHONDRS Summer 2019 Sampling Campaign: Global River Corridor Surface Water FTICR-MS, NPOC, TN, Anions, Stable Isotopes, Bacterial Abundance, and Dissolved Inorganic Carbon. River Corridor and Watershed Biogeochemistry SFA, ESS-DIVE repository, [data set], https://doi.org/10.15485/1603775 accessed via https://data.ess-dive.lbl.gov/datasets/doi:10.15485/1603775 (last access: 13 June 2023), 2023.
Verma, S. and Jayakumar, S.: Impact of forest fire on physical, chemical and biological properties of soil: A review, Proceedings of the International Academy of Ecology and Environmental Sciences, 2, 168–176, 2012.
Wagner, S., Ding, Y., and Jaffé, R.: A new perspective on the apparent solubility of dissolved black carbon, Front. Earth Sci., 5, https://doi.org/10.3389/feart.2017.00075, 2017.
Wagner, S., Dittmar, T., and Jaffé, R.: Molecular characterization of dissolved black nitrogen via electrospray ionization Fourier transform ion cyclotron resonance mass spectrometry, Organ. Geochem., 79, 21–30, 2015.
Wagner, S., Jaffé, R., and Stubbins, A.: Dissolved black carbon in aquatic ecosystems, Limnol. Oceanogr. Lett., 3, 168–185, 2018.
Wickham, H.: ggplot2, Wiley interdisciplinary reviews: computational statistics, 3, 180–185, 2011.
Wiesenberg, G. L., Schwarzbauer, J., Schmidt, M. W., and Schwark, L.: Source and turnover of organic matter in agricultural soils derived from n-alkane/n-carboxylic acid compositions and C-isotope signatures, Org. Geochem., 35, 1371–1393, 2004.
Wilkes, H., Buckel, W., Golding, B. T., and Rabus, R.: Metabolism of hydrocarbons in n-alkane-utilizing anaerobic bacteria, J. Molec. Microb. Biotech., 26, 138–151, 2016.
Wozniak, A. S., Goranov, A. I., Mitra, S., Bostick, K. W., Zimmerman, A. R., Schlesinger, D. R., Myneni, S., and Hatcher, P. G.: Molecular heterogeneity in pyrogenic dissolved organic matter from a thermal series of oak and grass chars, Org. Geochem., 148, 104065, https://doi.org/10.1016/j.orggeochem.2020.104065, 2020.
Yongdong, Z., Yaling, S., Zhengwen, L., Xiangchao, C., Jinlei, Y., Xiaodan, D., and Miao, J.: Long-chain n-alkenes in recent sediment of Lake Lugu (SW China) and their ecological implications, Limnologica, 52, 30–40, 2015.
Zimmerman, A. R. and Mitra, S.: Trial by Fire: On the Terminology and Methods Used in Pyrogenic Organic Carbon Research, Front. Earth Sci., 5, 95, https://doi.org/10.3389/feart.2017.00095, 2017.
Zimmerman, A. R. and Ouyang, L.: Priming of pyrogenic C (biochar) mineralization by dissolved organic matter and vice versa, Soil Biol. Biochem., 130, 105–112, 2019.