the Creative Commons Attribution 4.0 License.
the Creative Commons Attribution 4.0 License.
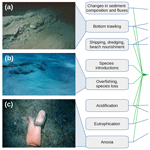
Ideas and perspectives: Human impacts alter the marine fossil record
Martin Zuschin
Adam Tomašových
Michał Kowalewski
Daniele Scarponi
The youngest fossil record is a crucial source of data documenting the recent history of marine ecosystems and their long-term alteration by humans. However, human activities that reshape communities and habitats also alter sedimentary and biological processes that control the formation of the sedimentary archives recording those impacts. These diverse physical, geochemical, and biological disturbances include changes in sediment fluxes due to the alteration of alluvial and coastal landscapes, seabed disturbance by bottom trawling and ship traffic, ocean acidification and deoxygenation, removal of native species, and introduction of invasive ecosystem engineers. These novel processes modify sedimentation rates, the depth and intensity of sediment mixing, the pore-water saturation state, and the preservation potential of skeletal remains – the parameters controlling the completeness and spatiotemporal resolution of the fossil record. We argue that humans have become a major force transforming the nature of the marine fossil record in ways that can both impede and improve our ability to reconstruct past ecological and climate dynamics. A better understanding of the feedback between human impacts on ecosystem processes and their preservation in the marine fossil record offers new research opportunities and novel tools for interpreting geohistorical archives of the ongoing anthropogenic transformation of the coastal ocean.
- Article
(2384 KB) - Full-text XML
- BibTeX
- EndNote
The fossil and sedimentary archives (geohistorical records) of the Anthropocene (sensu Gibbard et al., 2022; see Head et al., 2022, for an alternative view) are an indispensable source of data on ecosystem and climate states preceding the onset of ecological monitoring and instrumental records, allowing reconstruction of long-term human impacts on marine ecosystems (Dietl and Flessa, 2011; Kidwell, 2015; Yasuhara et al., 2020; Dillon et al., 2022). As with any historical record, the geohistorical data are most valuable when their information quality is well understood. However, humans are altering not only marine ecosystems but also the nature of the stratigraphic archives that record those changes (Wilkinson, 2005; Tyrrell, 2011; Oberle et al., 2016; Syvitski et al., 2022). In other words, humans affect not only what is preserved but also how it is preserved because key ecosystem processes affected by human impacts, such as bioturbation and remineralization of organic matter, also control the burial and preservation of skeletal remains. Although the resulting taphonomic and stratigraphic signatures may pose a challenge for accurately reconstructing past ecological and climate dynamics, they can also pinpoint historical shifts in ecosystem functioning (e.g., Gooday et al., 2009; Yasuhara et al., 2019; Tomašových et al., 2021) and thus improve our understanding of the consequences of global change.
Here, we propose a conceptual framework for understanding how human alteration of marine ecosystems changes the completeness and spatiotemporal resolution of geohistorical records that form in marginal marine, continental shelf, and slope environments, where human impacts are concentrated (Halpern et al., 2008). We highlight the implications of this phenomenon for marine paleoecology, conservation paleobiology, and paleoclimatic studies and suggest research strategies that can maximize the information value of the geohistorical data extracted from sediment cores and surface death assemblages (i.e., skeletal remains accumulating in the surface mixed layer of present-day seabeds). By providing an overview of mechanisms by which humans modify the incipient fossil record, we hope to encourage a more explicit consideration of these effects during interpretation of marine sedimentary and fossil archives.
The quality of geohistorical records formed by fossils embedded in sedimentary successions is determined by their spatiotemporal resolution and completeness. Spatiotemporal resolution corresponds to the extent of spatial mixing and time averaging of fossil assemblages, i.e., the co-occurrence of remains of organisms that lived at different times and/or places in a single sedimentary layer (depositional resolution sensu Kowalewski and Bambach, 2008). Completeness of the record can be understood both as completeness of fossil assemblages relative to their source communities (controlled by variability in preservation potential, both within and across taxa) and as stratigraphic completeness (Sadler, 1981) determined by the duration of hiatuses (stratigraphic resolution sensu Kowalewski and Bambach, 2008). Here, we will primarily focus on fossil assemblage completeness, which determines the fidelity of a given eco-environmental variable preserved in the geological record relative to its original signal. For example, a fossil sample limited to thick-shelled specimens varying in age by 3000 years has low completeness and coarse temporal resolution and thus low fidelity with respect to the original composition of the source living assemblage.
In the marine realm, the completeness and resolution of geohistorical records are primarily controlled by four parameters: (1) net sediment accumulation rate, (2) depth and intensity of sediment mixing below the seafloor, (3) disintegration rates determined mainly by the pore-water saturation state and bioerosion, and (4) skeletal production and durability (Kidwell, 1986; Olszewski, 2004; Kowalewski and Bambach, 2008; Tomašových et al., 2019). Skeletal production depends on community composition and population dynamics, which control the durability of skeletal remains and the rate at which they enter a death assemblage. Subsequently, sedimentation, sediment mixing, and pore-water chemistry determine whether the remains disintegrate near the sediment–water interface and if and at what rate they undergo burial to historical layers sealed from mixing, dissolution, or erosion (Olszewski, 2004). High sedimentation rates tend to increase both temporal resolution and completeness by reducing exposure time of skeletal remains to mixing and dissolution (Kidwell, 1986), while fast disintegration prevents the accumulation of older remains, resulting in low completeness but high temporal resolution (Kowalewski, 1997).
The net effects of these parameters on the quality of geohistorical archives often depends on complex interactions between them (Fig. 1). For example, changes in the sedimentation rate modulate benthic community composition (Thrush et al., 2004) but organisms can also modify sedimentation rates through sediment resuspension, stabilization, and production (Meadows et al., 2012). High input of skeletal carbonate, in turn, can buffer pore-water chemistry, reducing carbonate dissolution even in undersaturated conditions (Sulpis et al., 2022). Bioturbation and physical reworking generally reduce the temporal resolution, increase the physical wear of skeletal remains, and enhance carbonate dissolution by facilitating sulfide oxidation through pore-water irrigation (Aller, 2014). However, they can also lead to the rapid burial of skeletal remains, protecting them from higher skeletal disintegration rates near the sediment surface (Tomašových et al., 2019). Skeletal preservation is also affected by biotic interactions such as bioerosion, durophagous predation, and encrustation (Kidwell and Bosence, 1991).
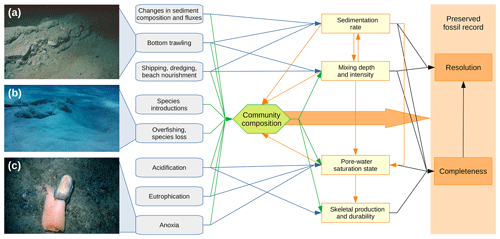
Figure 1A simplified conceptual framework for understanding how human impacts can affect the quality of the currently forming marine fossil record with examples of physical (a), biological (b), and geochemical (c) disturbances to marine ecosystems. The four parameters controlling the quality of the fossil record (yellow fields) can be affected by human impacts both directly (blue arrows) and indirectly through changes in community composition (green arrows). The four parameters are linked in turn by complex feedback loops (orange arrows) and together determine the resolution and completeness of the record (black arrows). The direction and strength of the effects of human activities are often context dependent (see examples in the main text). (a) Disturbance to the seabed (5–10 cm deep furrow) caused by trawling – Gulf of Trieste, northern Adriatic Sea (image courtesy of Michael Stachowitsch). (b) Foraging ray causing intensive bioturbation; an older feeding pit, several decimeters in diameter, is visible to the right – San Salvador, The Bahamas (image courtesy of Michał Kowalewski). (c) A deep infaunal bivalve Solecurtus sp., which emerged and died at the sediment surface during a benthic mass mortality event in September 1983 triggered by hypoxia – Gulf of Trieste, northern Adriatic Sea (image courtesy of Michael Stachowitsch).
The four parameters determining the completeness and resolution of marine geohistorical records are controlled by natural biological and geological processes driven by tectonics, climate, or biotic interactions. However, anthropogenic processes, which started to operate when humans became a major geological force, can exceed the spatial extent, frequency, rate, and magnitude of the natural drivers (Wilkinson, 2005; Tyrrell, 2011; Syvitski et al., 2022). Human activities can modify the four parameters that determine the quality of the record either directly (e.g., elevated sediment supply driven by deforestation can increase sedimentation rates) or indirectly by inducing changes in community composition and ecosystem functioning (e.g., the exclusion of burrowers by anoxia results in reduced sediment mixing; Fig. 1).
3.1 Physical disturbance
Humans alter the physical environment by changing rates and routes of sediment mobilization, transport, and deposition (Syvitski et al., 2022). On land, these changes are driven by river regulation, cropland and pasture expansion, deforestation, mining, urbanization, and other landscape alterations. In the sea, the direct physical impacts include the exploitation of living resources (trawling), the maintenance of global maritime trade (shipping), and interventions mitigating coastal erosion and sea-level rise. These activities affect the magnitude of sediment fluxes to the ocean, coastal geomorphology, sedimentation rates, and seabed properties, leaving clear signatures in stratigraphic sequences (e.g., Martín et al., 2014; Anthony et al., 2014; Handley et al., 2020).
Over the last 3000 years, periods of human population growth and intense landscape transformation have been associated with a significant increase in fluvial sediment discharge to marine basins and faster growth of river deltas (Maselli and Trincardi, 2013; Anthony et al., 2014; Syvitski et al., 2022). However, over the last century, despite the continuous increase in sediment production, its delivery to the oceans has declined rapidly due to river damming, reservoir construction, and soil conservation programs (Syvitski et al., 2022). For example, following the construction of the Three Gorges Dam, sediment supply to the Yangtze River Delta declined to its lowest level in its history, while coastal erosion increased (Wang et al., 2018), which should reduce the temporal resolution and completeness of the currently forming geological record (Fig. 2a).
Bottom trawl fishing, seabed excavation, and shipping are drivers of anthroturbation, i.e., anthropogenic sediment mixing (Zalasiewicz et al., 2014; Bunke et al., 2019), in marine ecosystems. Trawling is the most widespread source of physical disturbance to the seabed on continental shelves and slopes (Puig et al., 2012; Amoroso et al., 2018) (Fig. 1a). It can erode, mix, and resuspend large volumes of sediment; alter benthic community composition and functioning; and transform seascapes on a scale comparable to agriculture and deforestation on land (Martín et al., 2014; Paradis et al., 2021; Epstein et al., 2022). The impact of trawling on the seafloor ranges from minimal effects to intense winnowing and complete mixing of the top 30 cm (Oberle et al., 2016). For example, in the Baltic Sea, regularly trawled seabeds are thoroughly homogenized down to 25 cm, which significantly exceeds the depth of natural hydrodynamic mixing and bioturbation (<10 cm) and erases the chronological and geochemical signal in sediment cores (Bunke et al., 2019) (Fig. 2b). Trawling-induced fine-sediment resuspension and off-shelf transport can, in turn, lead to a significant increase in sedimentation rates and thus improve the temporal resolution of the sedimentary record forming on the continental slope below the fishing grounds (Martín et al., 2014).
Ship traffic and anchoring, dredging of navigation channels, and mining of marine sand and gravel can leave an even stronger physical footprint on the seabed, although their impact is restricted to shallower waters and more localized than trawling (Schoellhamer, 1996; Rapaglia et al., 2015; Mielck et al., 2021; de Schipper et al., 2021; Watson et al., 2022). For instance, ship wakes resuspend 1.2×106 t of sediments per year in the Venetian Lagoon and contribute to the significant erosion of shoals (Rapaglia et al., 2015). Land reclamation, beach nourishment, and the construction of coastal infrastructure to counteract erosion represent a direct large-scale human intervention into sedimentary dynamics both in the coastal zone and in offshore source areas where the sand for these projects is extracted (Mielck et al., 2021; de Schipper et al., 2021). Finally, the emerging industry of deep-seabed mining will extend the impacts of anthroturbation to abyssal plains (Levin et al., 2020).
In addition to modifying sediment fluxes, humans are also introducing novel materials to sedimentary systems, such as concrete, plastic, and other types of marine litter. The presence of micro- and macroplastic debris alters the physical properties of sediments (Russell et al., 2023; Wang and Hou, 2023), and the materials interact with marine biota (e.g., Galloway et al., 2017; Hope et al., 2021), affecting sediment stability, erosion, transport, and bioturbation rates.
All these physical disturbances directly impact the parameters controlling the temporal resolution of the geohistorical archives by significantly altering sedimentation rates and increasing the depth of the surface mixed layer. Moreover, mixing-induced oxygenation of surface sediments increases organic matter remineralization (Epstein et al., 2022) and thus skeletal disintegration rates (Aller, 2014), decreasing the completeness of the currently forming fossil record. Sediment erosion, transport, and redeposition triggered by human activities can also decrease spatial resolution through mixing of skeletal assemblages originating from different habitats (Benavente et al., 2005; Bizjack et al., 2017).
3.2 Geochemical disturbance
The decline in seawater pH and the saturation state of carbonate minerals triggered by rising atmospheric CO2 directly increase the carbonate dissolution rates (Tyrrell, 2011; Eyre et al., 2018; Fabricius et al., 2020). Consequently, as ocean acidification continues to escalate, the incipient fossil record forming at and directly below the seafloor will dissolve at an increasing pace. Some coral reefs are already undergoing net sediment loss due to dissolution, a pattern expected to accelerate globally (Eyre et al., 2018). More indirectly, acidification affects the ability of calcifying organisms to secrete their skeletons, which can become thinner, more porous, and fragile (Kroeker et al., 2013). For example, shells of gastropods living near submarine CO2 seeps – a natural system for studying biotic effects of acidification – show high levels of dissolution and reduced mechanical strength (Duquette et al., 2017), which impairs their preservation and long-term accumulation on the seabed (Fig. 2c). Deep-sea fossil archives are also affected due to impaired calcification of planktic foraminifera and pteropods (Bednaršek et al., 2012; Béjard et al., 2023) and shallowing of the calcite compensation depth (Sulpis et al., 2018).
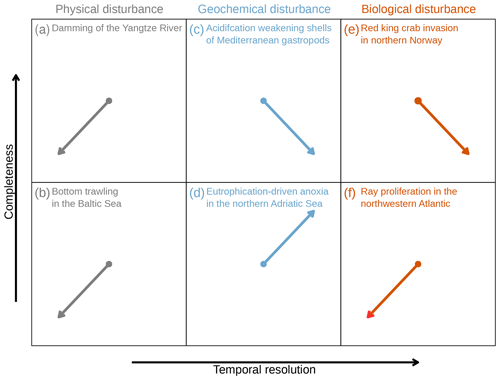
Figure 2Examples of expected shifts in the temporal resolution and completeness of the geohistorical record driven by human activities including physical (a–b), geochemical (c–d), and biological (e–f) disturbances. For most examples, quantitative estimates of changes in the resolution and completeness of the fossil record following a given disturbance are not available but the orientation of the arrows conveys the expected direction of the shifts in the nature of the geohistorical record. (a) Decrease in sedimentation rate on the Yangtze River Delta due to sediment retention by dams (Wang et al., 2018). (b) Mixing of surface sediments in regularly trawled areas of the southwestern Baltic Sea (Bunke et al., 2019). (c) Degradation of shell mechanical integrity in gastropods exposed to elevated pCO2 near submarine CO2 seeps north of Sicily, which represent a natural experiment on the long-term effects of ocean acidification (Duquette et al., 2017). (d) Decline in bioturbation and sediment ventilation due to seasonal anoxia in the northern Adriatic Sea (Tomašových et al., 2018). (e) Increase in shell-crushing predation and decrease in bioturbation caused by the red king crab invasion in northern Norway (Oug et al., 2018). (f) Increase in bioturbation and shell destruction due to the proliferation of cownose rays possibly triggered by a decline in shark abundance in the northwestern Atlantic Ocean (Myers et al., 2007).
Ocean acidification can interact with anthropogenic eutrophication, reducing carbonate production and preservation rates (Silbiger et al., 2018). Eutrophication increases primary productivity and reduces light levels, which can induce shifts in benthic community structure and increase the abundance of bioeroders (Lescinsky et al., 2002; Fabricius, 2005; Rice et al., 2020). In nearshore coral reef ecosystems, high input of land-derived nutrients reduces coral calcification rates and carbonate sediment production (Chazottes et al., 2008; Silbiger et al., 2018). In contrast, rates of bioerosion can be orders of magnitude higher than in oligotrophic locations, as documented in Hawaiian reefs affected by nutrient-rich submarine groundwater discharge (Prouty et al., 2017). Moreover, in habitats not subjected to bottom-water oxygen limitation, the eutrophication-driven proliferation of bioturbators can increase oxic–anoxic recycling in the sediment and, thus, carbonate dissolution.
Eutrophication, however, can also result in hypoxia and anoxia (Rabalais et al., 2014). Under low-oxygen conditions, the emergence of stressed infauna ceases particle reworking long before the actual mortality occurs (Riedel et al., 2014) (Fig. 1c), and the subsequent decline in abundance or extirpation of burrowers limits bioturbation and bioirrigation (Schaffner et al., 1992; Bianchi et al., 2021), modifying early diagenetic pathways (Middelburg and Levin, 2009). The resulting decline in sediment ventilation by O2 decreases carbonate dissolution by limiting reoxidation of reduced metabolites (Aller, 2014). Therefore, the increasing frequency of hypoxic events (Rabalais et al., 2014) improves both the completeness (increased shell preservation) and temporal resolution (reduced sediment mixing) of the Anthropocene record (Figs. 2d, 3).
3.3 Biological disturbance
Humans alter marine ecosystems by introducing non-native species, removing native taxa, or changing their relative abundances. Such widespread impacts often represent secondary consequences of physical and geochemical disturbances. If they affect ecosystem engineers or keystone species (Meadows et al., 2012; Guy-Haim et al., 2018; Bianchi et al., 2021), the resulting changes in bioturbation, sediment geochemistry, or even sedimentation rates (through biologically mediated sediment resuspension or stabilization) can rapidly alter the nature of the incipient fossil record.
The spread of non-native species can have profound sedimentological and taphonomic repercussions. For example, the invasion of the Barents Sea by red king crab (Paralithodes camtschaticus) feeding on burrowing invertebrates resulted not only in the intensification of shell-crushing predation but also in the decline of sediment reworking and bioirrigation, as indicated by the reduced thickness of the oxidized surface mixed layer (Oug et al., 2018). These functional changes should lead to a decrease in the preservation of skeletal benthos while simultaneously increasing the temporal resolution of their fossil record (Fig. 2e). In contrast, the proliferation in the Baltic Sea of the non-native polychaetes Marenzelleria spp., which burrow much deeper than native species, leads to more effective sediment reworking and bioirrigation (Kauppi et al., 2018), enhancing organic matter remineralization and facilitating the switch from a seasonally hypoxic system back to a normoxic one (Norkko et al., 2012).
Human activities can also result in the regional decline or extirpation of species that directly or indirectly influence sedimentary and taphonomic processes. For example, trophic cascades driven by overexploitation of large predators can have unexpected consequences for the biotic controls on skeletal preservation. In the northwestern Atlantic, a dramatic decline in shark abundance due to fishing pressure triggered a proliferation of cownose rays, Rhinoptera bonasus (Myers et al., 2007). As this durophagous species excavates large feeding pits (up to 1 m wide, 20–45 cm deep) to find its infaunal prey, increased ray abundance leads to intensive bioturbation and replacement of seagrass beds by unstable sands (Orth, 1975), thus affecting sediment mixing and shell fragmentation (Fig. 2f).
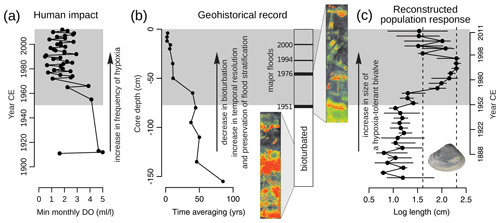
Figure 3An example of change in the quality of the post-1950 CE fossil record induced by anthropogenic alteration of a marine ecosystem (Tomašových et al., 2018). (a) The 20th-century eutrophication of the northern Adriatic Sea led to a decline in dissolved oxygen (DO) concentrations, an increase in the frequency of seasonal hypoxia, and loss of hypoxia-sensitive infauna. (b) In the stratigraphic record of the Po prodelta, this regime shift is associated with increased temporal resolution, as expressed by an upcore decrease in bioturbation intensity (strongly homogenized biofabric in the lower part of the core is replaced by discrete traces of echinoids in the upper part as visible on X-ray images); reduced time averaging of molluskan assemblages; and increased preservation of thin silty layers corresponding to decadal floods. Time averaging was measured as the interquartile range of post-mortem ages of bivalve shells dated with 14C-calibrated amino acid racemization methods (30 shells dated per core increment). (c) Fossil assemblages from sediment cores document a significant increase in the abundance and body size of the hypoxia-tolerant bivalve Varicorbula gibba, offering a window into pre-impact molluskan community dynamics extending back to the late 19th century – several decades before the first benthic surveys and over a century before the onset of systematic ecological monitoring in the northern Adriatic Sea (Tomašových et al., 2018, 2020).
These physical, geochemical, and biological disturbances often interact (e.g., Norkko et al., 2012; Silbiger et al., 2018; Handley et al., 2020; Geraldi et al., 2020), and their net effect on the quality of the youngest geohistorical archives is context-specific. For example, the negative impact of bottom trawling on the continental shelf records can be accompanied by improved temporal resolution on the adjacent continental slope due to a resuspension-driven increase in sedimentation rates. The intensity and spatial extent of human alteration of marine sedimentary sequences also vary significantly – from global or regional drivers (e.g., acidification, changes in sediment fluxes) to highly localized ones (e.g., anchoring) – producing a complex mosaic on the seascape, with strongly distorted portions of the continental shelf neighboring areas with a minimal impact (Oberle et al., 2016; Paradis et al., 2021). Moreover, the consequences of global warming and accelerating sea-level rise, such as increased denudation and coastal erosion (Syvitski et al., 2022; de Schipper et al., 2021), changes in the distribution of ecosystem engineers (Aronson et al., 2015; Bianchi et al., 2021; Weinert et al., 2022), the spread of non-native species (McKnight et al., 2021), or the potential impacts of ocean-based climate interventions (Levin et al., 2023), can intensify many anthropogenic processes that affect the quality of the fossil record.
On the one hand, the interactive effects of humans on both marine ecosystem processes and the nature of the sedimentary archives that record those human-driven shifts (Fig. 1) can represent a challenge for studies relying on geohistorical data. Changes in the quality of the fossil record coinciding with a major restructuring of ecosystems can mask or exaggerate the magnitude of ecological changes as reconstructed from geohistorical archives. Trawling-induced disturbance can distort the stratigraphic order of top sediment layers; destroy original sedimentary fabric; and truncate, flatten, or reverse near-surface geochemical concentration profiles, potentially affecting environmental interpretations based on sediment cores (Oberle et al., 2016; Martín et al., 2014; Bunke et al., 2019). However, the effects of other factors are more insidious, producing stratigraphic artifacts that can be mistaken for biological signals. For instance, increased time averaging due to low sedimentation rates not only reduces the temporal resolution but also can spuriously inflate the evenness and richness of fossil samples while decreasing compositional turnover between them (Kidwell and Tomasovych, 2013). Moreover, sediment mixing and time averaging can obliterate the stratigraphic expression of abrupt regime shifts, making them appear more gradual (Anderson, 2001; Tomašových et al., 2020), whereas breaks in deposition can turn gradual ecological transitions into abrupt changes in the stratigraphic record (Kidwell, 1986). Although such effects are most pronounced in the paleontological data, biogeochemical proxy records, including emerging ones such as biomarkers and sedimentary ancient DNA, are not immune to the human-induced changes in their preservation (Paradis et al., 2021) and the resolution of sedimentary archives. Skeletal remains are carriers of many key paleoenvironmental proxies (e.g., stable isotopes of foraminifera tests), while the interpretation of others ultimately relies on core age–depth models based on radioisotope profiles or radiocarbon dating of fossils and can be thus affected by changes in preservation, mixing, or sedimentation rates (Bunke et al., 2019; Kosnik et al., 2015; Lougheed et al., 2018).
On the other hand, alteration of sedimentary sequences induced by human-driven changes in ecosystem processes offers new opportunities for geohistorical research addressing different aspects of global change. Anthropogenic signatures in the completeness and resolution of geohistorical records can serve as essential proxies for major shifts in ecosystem functioning (Fig. 3). For example, the decrease in bioturbation, increase in organic carbon content, and presence of fine-scale sedimentary structures like lamination are commonly used as indicators of impacted ecosystems and anoxic conditions (Gooday et al., 2009; Tomašových et al., 2018), while the preservation state of skeletal remains observed in sediment cores can be used to track historical shifts in sediment bioirrigation efficiency (Tomašových et al., 2021) and aid in the identification of past coral mass mortality events (Wapnick et al., 2004).
Importantly, many human disturbances such as increased sediment supply or coastal hypoxia produce sedimentary records with higher temporal resolution than those that formed under the pre-impact conditions (Fig. 3), facilitating reconstructions of ecosystem response and recovery. Moreover, even when the quality of the record is reduced, a suite of novel multi-disciplinary approaches integrating ecological, taphonomic, sedimentological, biogeochemical, and stratigraphic data can provide robust interpretations of past ecosystem dynamics. Incorporating information on the preservation state, time averaging, and sedimentation rates into paleoecological reconstructions can help to pinpoint gaps in the record and facilitates disentangling the effects of major environmental perturbations from spurious patterns arising from changes in preservation or temporal resolution.
Recent advances in geochronological methods (e.g., Bush et al., 2013; Clark et al., 2014; Gottschalk et al., 2018) allow age dating of large numbers of individual fossil specimens, providing direct estimates of time averaging, stratigraphic disorder, and hiatus duration (e.g., Kosnik et al., 2007; Scarponi et al., 2013; Nawrot et al., 2022; Tomašových et al., 2022; reviewed by Tomašových et al., 2023) and thus more realistic assessment of the uncertainties associated with age–depth models (Kosnik et al., 2015; Lougheed et al., 2018; Dolman et al., 2021). Even more importantly, this approach can be used to unmix fossil assemblages and thus resolve temporal changes in production, body size, and community composition, which may not be evident in time-averaged records (e.g., Harnik et al., 2017; Tomašových and Kidwell, 2017; Tomašových et al., 2019, 2020; Clark et al., 2023). Radiocarbon dating of individual fossils (notwithstanding the alteration generated by nuclear testing; Dutta, 2016) can also be combined with analyses of geochemical proxies from the same specimens, allowing the extraction of well-resolved paleoenvironmental time series from highly time-averaged sedimentary successions (Lougheed et al., 2018).
Finally, numerical simulations that jointly model ecological, sedimentological, and taphonomic processes provide valuable insights into how the taphonomic and stratigraphic overprints can modify biotic and environmental signals. For instance, metacommunity models coupled with preservational processes were used to estimate the magnitude of change in community turnover expected under increasing loss in temporal resolution (Kidwell and Tomasovych, 2013), and the effect of time averaging on the detection of regime shifts in the stratigraphic record was explored by combining numerical simulation with evolutionary models (Tomašových et al., 2020). Contrasting the outputs of such models with the empirical data constrains the range of possible ecological scenarios that are consistent with the patterns observed in the stratigraphic record.
Somewhat analogously to the Heisenberg uncertainty principle, the very same human-driven processes that have been altering ecosystems also affect our ability to reconstruct these changes based on geohistorical data. The role of humans in transforming the currently forming paleobiological record is already substantial on land (Bennett et al., 2018; Plotnick and Koy, 2020) and will only intensify in the ocean as exploitation of its resources and other human stressors intensify (O'Hara and Halpern, 2022), representing a potential challenge for conservation paleobiology, historical ecology, and paleoclimatic research. At the same time, taking into account stratigraphic signatures left by past human-induced shifts in ecosystem functioning should further augment our ability to use fossils, geochemical proxies, ancient DNA (aDNA), and other geohistorical data to track the history of anthropogenic transformation of the coastal ocean. Therefore, we need a better understating of the effects of human actions on different parameters of the taphonomic equation that control the fidelity of the fossil record of the Anthropocene.
No primary data were used in this paper. Data used to create Fig. 3 are available as supplements to Tomašových et al. (2018, 2020).
RN conceived the original idea; RN, MZ, AT, MK, and DS performed the literature survey and contributed data; RN, MZ, AT, MK, and DS wrote the manuscript.
The contact author has declared that none of the authors has any competing interests.
Publisher’s note: Copernicus Publications remains neutral with regard to jurisdictional claims made in the text, published maps, institutional affiliations, or any other geographical representation in this paper. While Copernicus Publications makes every effort to include appropriate place names, the final responsibility lies with the authors.
We thank Michael Stachowitsch for sharing underwater photographs and Tadej Gračner for providing access to the Marine Biology Station Piran, Slovenia, where the initial outline of this paper was formulated.
This research has been supported by the National Science Foundation (grant nos. EAR-1559196 and EAR-1922562); the Agentúra na Podporu Výskumu a Vývoja (grant no. APVV-22-0523); the Vedecká Grantová Agentúra MŠVVaŠ SR a SAV (grant no. 2/0106/23); and the NextGenerationEU through the Italian Ministry of University and Research under PNRR – Mission 4 Component C2, Investment 1.1 “Conservation of life on Earth: the fossil record as an unparalleled archive of ecological and evolutionary responses to past warming events”.
This paper was edited by Olivier Sulpis and reviewed by Moriaki Yasuhara, Niklas Hohmann, and one anonymous referee.
Aller, R. C.: 8.11 – Sedimentary Diagenesis, Depositional Environments, and Benthic Fluxes, in: Treatise on Geochemistry (Second Edition), edited by: Holland, H. D. and Turekian, K. K., Elsevier, Oxford, 293–334, https://doi.org/10.1016/B978-0-08-095975-7.00611-2, 2014.
Amoroso, R. O., Pitcher, C. R., Rijnsdorp, A. D., McConnaughey, R. A., Parma, A. M., Suuronen, P., Eigaard, O. R., Bastardie, F., Hintzen, N. T., Althaus, F., Baird, S. J., Black, J., Buhl-Mortensen, L., Campbell, A. B., Catarino, R., Collie, J., Cowan, J. H., Durholtz, D., Engstrom, N., Fairweather, T. P., Fock, H. O., Ford, R., Gálvez, P. A., Gerritsen, H., Góngora, M. E., González, J. A., Hiddink, J. G., Hughes, K. M., Intelmann, S. S., Jenkins, C., Jonsson, P., Kainge, P., Kangas, M., Kathena, J. N., Kavadas, S., Leslie, R. W., Lewis, S. G., Lundy, M., Makin, D., Martin, J., Mazor, T., Gonzalez-Mirelis, G., Newman, S. J., Papadopoulou, N., Posen, P. E., Rochester, W., Russo, T., Sala, A., Semmens, J. M., Silva, C., Tsolos, A., Vanelslander, B., Wakefield, C. B., Wood, B. A., Hilborn, R., Kaiser, M. J., and Jennings, S.: Bottom trawl fishing footprints on the world's continental shelves, P. Natl. Acad. Sci. USA, 115, E10275–E10282, https://doi.org/10.1073/pnas.1802379115, 2018.
Anderson, D. M.: Attenuation of millennial-scale events by bioturbation in marine sediments, Paleoceanography, 16, 352–357, https://doi.org/10.1029/2000PA000530, 2001.
Anthony, E. J., Marriner, N., and Morhange, C.: Human influence and the changing geomorphology of Mediterranean deltas and coasts over the last 6000 years: From progradation to destruction phase?, Earth-Sci. Rev., 139, 336–361, https://doi.org/10.1016/j.earscirev.2014.10.003, 2014.
Aronson, R. B., Smith, K. E., Vos, S. C., McClintock, J. B., Amsler, M. O., Moksnes, P.-O., Ellis, D. S., Kaeli, J., Singh, H., Bailey, J. W., Schiferl, J. C., van Woesik, R., Martin, M. A., Steffel, B. V., Deal, M. E., Lazarus, S. M., Havenhand, J. N., Swalethorp, R., Kjellerup, S., and Thatje, S.: No barrier to emergence of bathyal king crabs on the Antarctic shelf, P. Natl. Acad. Sci. USA, 112, 12997–13002, https://doi.org/10.1073/pnas.1513962112, 2015.
Bednaršek, N., Tarling, G. A., Bakker, D. C. E., Fielding, S., Jones, E. M., Venables, H. J., Ward, P., Kuzirian, A., Lézé, B., Feely, R. A., and Murphy, E. J.: Extensive dissolution of live pteropods in the Southern Ocean, Nat. Geosci., 5, 881–885, https://doi.org/10.1038/ngeo1635, 2012.
Béjard, T. M., Rigual-Hernández, A. S., Flores, J. A., Tarruella, J. P., Durrieu de Madron, X., Cacho, I., Haghipour, N., Hunter, A., and Sierro, F. J.: Calcification response of planktic foraminifera to environmental change in the western Mediterranean Sea during the industrial era, Biogeosciences, 20, 1505–1528, https://doi.org/10.5194/bg-20-1505-2023, 2023.
Benavente, J., Gracia, F.-J., Anfuso, G., and Lopez-Aguayo, F.: Temporal assessment of sediment transport from beach nourishments by using foraminifera as natural tracers, Coast. Eng., 52, 205–219, https://doi.org/10.1016/j.coastaleng.2004.12.002, 2005.
Bennett, C. E., Thomas, R., Williams, M., Zalasiewicz, J., Edgeworth, M., Miller, H., Coles, B., Foster, A., Burton, E. J., and Marume, U.: The broiler chicken as a signal of a human reconfigured biosphere, R. Soc. Open Sci., 5, 180325, https://doi.org/10.1098/rsos.180325, 2018.
Bianchi, T. S., Aller, R. C., Atwood, T. B., Brown, C. J., Buatois, L. A., Levin, L. A., Levinton, J. S., Middelburg, J. J., Morrison, E. S., Regnier, P., Shields, M. R., Snelgrove, P. V. R., Sotka, E. E., and Stanley, R. R. E.: What global biogeochemical consequences will marine animal–sediment interactions have during climate change?, Elem. Sci. Anthr., 9, 00180, https://doi.org/10.1525/elementa.2020.00180, 2021.
Bizjack, M. T., Kidwell, S. M., Velarde, R. G., Leonard-Pingel, J., and Tomašových, A.: Detecting, sourcing, and age-dating dredged sediments on the open shelf, southern California, using dead mollusk shells, Mar. Pollut. Bull., 114, 448–465, https://doi.org/10.1016/j.marpolbul.2016.10.010, 2017.
Bunke, D., Leipe, T., Moros, M., Morys, C., Tauber, F., Virtasalo, J. J., Forster, S., and Arz, H. W.: Natural and anthropogenic sediment mixing processes in the south-western Baltic Sea, Front. Mar. Sci., 6, 677, https://doi.org/10.3389/fmars.2019.00677, 2019.
Bush, S. L., Santos, G. M., Xu, X., Southon, J. R., Thiagarajan, N., Hines, S. K., and Adkins, J. F.: Simple, rapid, and cost effective: a screening method for 14C analysis of small carbonate samples, Radiocarbon, 55, 631–640, 2013.
Chazottes, V., Reijmer, J. J. G., and Cordier, E.: Sediment characteristics in reef areas influenced by eutrophication-related alterations of benthic communities and bioerosion processes, Mar. Geol., 250, 114–127, https://doi.org/10.1016/j.margeo.2008.01.002, 2008.
Clark, T. R., Roff, G., Zhao, J., Feng, Y., Done, T. J., and Pandolfi, J. M.: Testing the precision and accuracy of the U–Th chronometer for dating coral mortality events in the last 100 years, Quat. Geochronol., 23, 35–45, https://doi.org/10.1016/j.quageo.2014.05.002, 2014.
Clark, T. R., Roff, G., Chapman, T., Markham-Summers, H., Hammerman, N. M., Liu, F., Feng, Y., Pandolfi, J. M., and Zhao, J.: Reconstructing past disturbance in coral communities using U-Th dating of dead coral skeletons, Geology, 51, 983–987, https://doi.org/10.1130/G51419.1, 2023.
de Schipper, M. A., Ludka, B. C., Raubenheimer, B., Luijendijk, A. P., and Schlacher, T. A.: Beach nourishment has complex implications for the future of sandy shores, Nat. Rev. Earth Environ., 2, 70–84, https://doi.org/10.1038/s43017-020-00109-9, 2021.
Dietl, G. P. and Flessa, K. W.: Conservation paleobiology: putting the dead to work, Trends Ecol. Evol., 26, 30–37, https://doi.org/10.1016/j.tree.2010.09.010, 2011.
Dillon, E. M., Pier, J. Q., Smith, J. A., Raja, N. B., Dimitrijević, D., Austin, E. L., Cybulski, J. D., De Entrambasaguas, J., Durham, S. R., Grether, C. M., Haldar, H. S., Kocáková, K., Lin, C.-H., Mazzini, I., Mychajliw, A. M., Ollendorf, A. L., Pimiento, C., Regalado Fernández, O. R., Smith, I. E., and Dietl, G. P.: What is conservation paleobiology? Tracking 20 years of research and development, Front. Ecol. Evol., 10, 1031483, https://doi.org/10.3389/fevo.2022.1031483, 2022.
Dolman, A. M., Groeneveld, J., Mollenhauer, G., Ho, S. L., and Laepple, T.: Estimating Bioturbation From Replicated Small-Sample Radiocarbon Ages, Paleoceanogr. Paleocl., 36, e2020PA004142, https://doi.org/10.1029/2020PA004142, 2021.
Duquette, A., McClintock, J. B., Amsler, C. D., Pérez-Huerta, A., Milazzo, M., and Hall-Spencer, J. M.: Effects of ocean acidification on the shells of four Mediterranean gastropod species near a CO2 seep, Mar. Pollut. Bull., 124, 917–928, https://doi.org/10.1016/j.marpolbul.2017.08.007, 2017.
Dutta, K.: Sun, Ocean, Nuclear Bombs, and Fossil Fuels: Radiocarbon Variations and Implications for High-Resolution Dating, Annu. Rev. Earth Planet. Sci., 44, 239–275, https://doi.org/10.1146/annurev-earth-060115-012333, 2016.
Epstein, G., Middelburg, J. J., Hawkins, J. P., Norris, C. R., and Roberts, C. M.: The impact of mobile demersal fishing on carbon storage in seabed sediments, Glob. Change Biol., 28, 2875–2894, https://doi.org/10.1111/gcb.16105, 2022.
Eyre, B. D., Cyronak, T., Drupp, P., Carlo, E. H. D., Sachs, J. P., and Andersson, A. J.: Coral reefs will transition to net dissolving before end of century, Science, 359, 908–911, https://doi.org/10.1126/science.aao1118, 2018.
Fabricius, K. E.: Effects of terrestrial runoff on the ecology of corals and coral reefs: review and synthesis, Mar. Pollut. Bull., 50, 125–146, https://doi.org/10.1016/j.marpolbul.2004.11.028, 2005.
Fabricius, K. E., Neill, C., Van Ooijen, E., Smith, J. N., and Tilbrook, B.: Progressive seawater acidification on the Great Barrier Reef continental shelf, Sci. Rep., 10, 18602, https://doi.org/10.1038/s41598-020-75293-1, 2020.
Galloway, T. S., Cole, M., and Lewis, C.: Interactions of microplastic debris throughout the marine ecosystem, Nat. Ecol. Evol., 1, 1–8, https://doi.org/10.1038/s41559-017-0116, 2017.
Geraldi, N. R., Anton, A., Santana-Garcon, J., Bennett, S., Marbà, N., Lovelock, C. E., Apostolaki, E. T., Cebrian, J., Krause-Jensen, D., Martinetto, P., Pandolfi, J. M., and Duarte, C. M.: Ecological effects of non-native species in marine ecosystems relate to co-occurring anthropogenic pressures, Glob. Change Biol., 26, 1248–1258, https://doi.org/10.1111/gcb.14930, 2020.
Gibbard, P., Walker, M., Bauer, A., Edgeworth, M., Edwards, L., Ellis, E., Finney, S., Gill, J. L., Maslin, M., Merritts, D., and Ruddiman, W.: The Anthropocene as an Event, not an Epoch, J. Quaternary Sci., 37, 395–399, https://doi.org/10.1002/jqs.3416, 2022.
Gooday, A. J., Jorissen, F., Levin, L. A., Middelburg, J. J., Naqvi, S. W. A., Rabalais, N. N., Scranton, M., and Zhang, J.: Historical records of coastal eutrophication-induced hypoxia, Biogeosciences, 6, 1707–1745, https://doi.org/10.5194/bg-6-1707-2009, 2009.
Gottschalk, J., Szidat, S., Michel, E., Mazaud, A., Salazar, G., Battaglia, M., Lippold, J., and Jaccard, S. L.: Radiocarbon measurements of small-size foraminiferal samples with the Mini Carbon Dating System (MICADAS) at the University of Bern: Implications for paleoclimate reconstructions, Radiocarbon, 60, 469–491, https://doi.org/10.1017/RDC.2018.3, 2018.
Guy-Haim, T., Lyons, D. A., Kotta, J., Ojaveer, H., Queirós, A. M., Chatzinikolaou, E., Arvanitidis, C., Como, S., Magni, P., Blight, A. J., Orav-Kotta, H., Somerfield, P. J., Crowe, T. P., and Rilov, G.: Diverse effects of invasive ecosystem engineers on marine biodiversity and ecosystem functions: A global review and meta-analysis, Glob. Change Biol., 24, 906–924, https://doi.org/10.1111/gcb.14007, 2018.
Head, M. J., Zalasiewicz, J. A., Waters, C. N., Turner, S. D., Williams, M., Barnosky, A. D., Steffen, W., Wagreich, M., Haff, P. K., Syvitski, J., Leinfelder, R., Mccarthy, F. M. G., Rose, N. L., Wing, S. L., An, Z., Cearreta, A., Cundy, A. B., Fairchild, I. J., Han, Y., Ivar Do Sul, J. A., Jeandel, C., Mcneill, J. R., and Summerhayes, C. P.: The proposed Anthropocene Epoch/Series is underpinned by an extensive array of mid-20th century stratigraphic event signals, J. Quaternary Sci., 37, 1181–1187, https://doi.org/10.1002/jqs.3467, 2022.
Halpern, B. S., Walbridge, S., Selkoe, K. A., Kappel, C. V., Micheli, F., D'Agrosa, C., Bruno, J. F., Casey, K. S., Ebert, C., Fox, H. E., Fujita, R., Heinemann, D., Lenihan, H. S., Madin, E. M. P., Perry, M. T., Selig, E. R., Spalding, M., Steneck, R., and Watson, R.: A global map of human impact on marine ecosystems, Science, 319, 948–952, https://doi.org/10.1126/science.1149345, 2008.
Handley, S. J., Swales, A., Horrocks, M., Gibbs, M., Carter, M., Ovenden, R., and Stead, J.: Historic and contemporary anthropogenic effects on granulometry and species composition detected from sediment cores and death assemblages, Nelson Bays, Aotearoa-New Zealand, Cont. Shelf Res., 202, 104147, https://doi.org/10.1016/j.csr.2020.104147, 2020.
Harnik, P. G., Torstenson, M. L., and Williams, M. A.: Assessing the effects of anthropogenic eutrophication on marine bivalve life history in the Northern Gulf of Mexico, Palaios, 32, 678–688, https://doi.org/10.2110/palo.2017.033, 2017.
Hope, J. A., Coco, G., Parsons, D. R., and Thrush, S. F.: Microplastics interact with benthic biostabilization processes, Environ. Res. Lett., 16, 124058, https://doi.org/10.1088/1748-9326/ac3bfd, 2021.
Kauppi, L., Bernard, G., Bastrop, R., Norkko, A., and Norkko, J.: Increasing densities of an invasive polychaete enhance bioturbation with variable effects on solute fluxes, Sci. Rep., 8, 7619, https://doi.org/10.1038/s41598-018-25989-2, 2018.
Kidwell, S. M.: Models for fossil concentrations: paleobiologic implications, Paleobiology, 12, 6–24, https://doi.org/10.1017/S0094837300002943, 1986.
Kidwell, S. M.: Biology in the Anthropocene: Challenges and insights from young fossil records, P. Natl. Acad. Sci. USA, 112, 4922–4929, https://doi.org/10.1073/pnas.1403660112, 2015.
Kidwell, S. M. and Bosence, D. W. J.: Taphonomy and time-averaging of marine shelly faunas, in: Taphonomy, Releasing the Data Locked in the Fossil Record, edited by: Allison, P. A. and Briggs, D. E. G., Plenum Press, New York, 115–209, ISBN 030648763, 1991.
Kidwell, S. M. and Tomasovych, A.: Implications of time-averaged death assemblages for ecology and conservation biology, Annu. Rev. Ecol. Evol. Syst., 44, 539–563, https://doi.org/10.1146/annurev-ecolsys-110512-135838, 2013.
Kosnik, M. A., Hua, Q., Jacobsen, G. E., Kaufman, D. S., and Wüst, R. A.: Sediment mixing and stratigraphic disorder revealed by the age-structure of Tellina shells in Great Barrier Reef sediment, Geology, 35, 811–814, https://doi.org/10.1130/G23722A.1, 2007.
Kosnik, M. A., Hua, Q., Kaufman, D. S., and Zawadzki, A.: Sediment accumulation, stratigraphic order, and the extent of time-averaging in lagoonal sediments: a comparison of 210Pb and 14C/amino acid racemization chronologies, Coral Reefs, 34, 215–229, https://doi.org/10.1007/s00338-014-1234-2, 2015.
Kowalewski, M.: The reciprocal taphonomic model, Lethaia, 30, 86–88, https://doi.org/10.1111/j.1502-3931.1997.tb00447.x, 1997.
Kowalewski, M. and Bambach, R. K.: The limits of paleontological resolution, in: High-Resolution Approaches in Stratigraphic Paleontology, edited by: Harries, P. J., Springer Netherlands, Dordrecht, 1–48, https://doi.org/10.1007/978-1-4020-9053-0_1, 2008.
Kroeker, K. J., Kordas, R. L., Crim, R., Hendriks, I. E., Ramajo, L., Singh, G. S., Duarte, C. M., and Gattuso, J.-P.: Impacts of ocean acidification on marine organisms: quantifying sensitivities and interaction with warming, Glob. Change Biol., 19, 1884–1896, https://doi.org/10.1111/gcb.12179, 2013.
Lescinsky, H. L., Edinger, E., and Risk, M. J.: Mollusc shell encrustation and bioerosion rates in a modern epeiric sea: Taphonomy experiments in the Java Sea, Indonesia, Palaios, 17, 171–191, https://doi.org/10.1669/0883-1351(2002)017<0171:MSEABR>2.0.CO;2, 2002.
Levin, L. A., Amon, D. J., and Lily, H.: Challenges to the sustainability of deep-seabed mining, Nat. Sustain., 3, 784–794, https://doi.org/10.1038/s41893-020-0558-x, 2020.
Levin, L. A., Alfaro-Lucas, J. M., Colaço, A., Cordes, E. E., Craik, N., Danovaro, R., Hoving, H.-J., Ingels, J., Mestre, N. C., Seabrook, S., Thurber, A. R., Vivian, C., and Yasuhara, M.: Deep-sea impacts of climate interventions, Science, 379, 978–981, https://doi.org/10.1126/science.ade7521, 2023.
Lougheed, B. C., Metcalfe, B., Ninnemann, U. S., and Wacker, L.: Moving beyond the age–depth model paradigm in deep-sea palaeoclimate archives: dual radiocarbon and stable isotope analysis on single foraminifera, Clim. Past, 14, 515–526, https://doi.org/10.5194/cp-14-515-2018, 2018.
Martín, J., Puig, P., Palanques, A., and Giamportone, A.: Commercial bottom trawling as a driver of sediment dynamics and deep seascape evolution in the Anthropocene, Anthropocene, 7, 1–15, https://doi.org/10.1016/j.ancene.2015.01.002, 2014.
Maselli, V. and Trincardi, F.: Man made deltas, Sci. Rep., 3, 1926, https://doi.org/10.1038/srep01926, 2013.
McKnight, E., Spake, R., Bates, A., Smale, D. A., and Rius, M.: Non-native species outperform natives in coastal marine ecosystems subjected to warming and freshening events, Glob. Ecol. Biogeogr., 30, 1698–1712, https://doi.org/10.1111/geb.13318, 2021.
Meadows, P. S., Meadows, A., and Murray, J. M. H.: Biological modifiers of marine benthic seascapes: Their role as ecosystem engineers, Geomorphology, 157–158, 31–48, https://doi.org/10.1016/j.geomorph.2011.07.007, 2012.
Middelburg, J. J. and Levin, L. A.: Coastal hypoxia and sediment biogeochemistry, Biogeosciences, 6, 1273–1293, https://doi.org/10.5194/bg-6-1273-2009, 2009.
Mielck, F., Michaelis, R., Hass, H. C., Hertel, S., Ganal, C., and Armonies, W.: Persistent effects of sand extraction on habitats and associated benthic communities in the German Bight, Biogeosciences, 18, 3565–3577, https://doi.org/10.5194/bg-18-3565-2021, 2021.
Myers, R. A., Baum, J. K., Shepherd, T. D., Powers, S. P., and Peterson, C. H.: Cascading Effects of the Loss of Apex Predatory Sharks from a Coastal Ocean, Science, 315, 1846–1850, https://doi.org/10.1126/science.1138657, 2007.
Nawrot, R., Berensmeier, M., Gallmetzer, I., Haselmair, A., Tomašových, A., and Zuschin, M.: Multiple phyla, one time resolution? Similar time averaging in benthic foraminifera, mollusk, echinoid, crustacean, and otolith fossil assemblages, Geology, 50, 902–906, https://doi.org/10.1130/G49970.1, 2022.
Norkko, J., Reed, D. C., Timmermann, K., Norkko, A., Gustafsson, B. G., Bonsdorff, E., Slomp, C. P., Carstensen, J., and Conley, D. J.: A welcome can of worms? Hypoxia mitigation by an invasive species, Glob. Change Biol., 18, 422–434, https://doi.org/10.1111/j.1365-2486.2011.02513.x, 2012.
Oberle, F. K. J., Swarzenski, P. W., Reddy, C. M., Nelson, R. K., Baasch, B., and Hanebuth, T. J. J.: Deciphering the lithological consequences of bottom trawling to sedimentary habitats on the shelf, J. Mar. Syst., 159, 120–131, https://doi.org/10.1016/j.jmarsys.2015.12.008, 2016.
O'Hara, C. C. and Halpern, B. S.: Anticipating the future of the world's ocean, Annu. Rev. Environ. Resour., 47, 291–315, https://doi.org/10.1146/annurev-environ-120120-053645, 2022.
Olszewski, T. D.: Modeling the influence of taphonomic destruction, reworking, and burial on time-averaging in fossil accumulations, Palaios, 19, 39–50, https://doi.org/10.1669/0883-1351(2004)019<0039:MTIOTD>2.0.CO;2, 2004.
Orth, R. J.: Destruction of Eelgrass, Zostera marina, by the Cownose Ray, Rhinoptera bonasus, in the Chesapeake Bay, Chesap. Sci., 16, 205–208, https://doi.org/10.2307/1350896, 1975.
Oug, E., Sundet, J. H., and Cochrane, S. K. J.: Structural and functional changes of soft-bottom ecosystems in northern fjords invaded by the red king crab (Paralithodes camtschaticus), J. Mar. Syst., 180, 255–264, https://doi.org/10.1016/j.jmarsys.2017.07.005, 2018.
Paradis, S., Goñi, M., Masqué, P., Durán, R., Arjona-Camas, M., Palanques, A., and Puig, P.: Persistence of biogeochemical alterations of deep-sea sediments by bottom trawling, Geophys. Res. Lett., 48, e2020GL091279, https://doi.org/10.1029/2020GL091279, 2021.
Plotnick, R. E. and Koy, K. A.: The Anthropocene fossil record of terrestrial mammals, Anthropocene, 29, 100233, https://doi.org/10.1016/j.ancene.2019.100233, 2020.
Prouty, N. G., Cohen, A., Yates, K. K., Storlazzi, C. D., Swarzenski, P. W., and White, D.: Vulnerability of Coral Reefs to Bioerosion From Land-Based Sources of Pollution, J. Geophys. Res.-Oceans, 122, 9319–9331, https://doi.org/10.1002/2017JC013264, 2017.
Puig, P., Canals, M., Company, J. B., Martín, J., Amblas, D., Lastras, G., Palanques, A., and Calafat, A. M.: Ploughing the deep sea floor, Nature, 489, 286–289, https://doi.org/10.1038/nature11410, 2012.
Rabalais, N. N., Cai, W.-J., Carstensen, J., Conley, D. J., Fry, B., Hu, X., Quinones-Rivera, Z., Rosenberg, R., Slomp, C. P., and Turner, R. E.: Eutrophication-driven deoxygenation in the coastal ocean, Oceanography, 27, 172–183, https://doi.org/10.5670/oceanog.2014.21, 2014.
Rapaglia, J., Zaggia, L., Parnell, K., Lorenzetti, G., and Vafeidis, A. T.: Ship-wake induced sediment remobilization: Effects and proposed management strategies for the Venice Lagoon, Ocean Coast. Manage., 110, 1–11, https://doi.org/10.1016/j.ocecoaman.2015.03.002, 2015.
Rice, M. M., Maher, R. L., Correa, A. M. S., Moeller, H. V., Lemoine, N. P., Shantz, A. A., Burkepile, D. E., and Silbiger, N. J.: Macroborer presence on corals increases with nutrient input and promotes parrotfish bioerosion, Coral Reefs, 39, 409–418, https://doi.org/10.1007/s00338-020-01904-y, 2020.
Riedel, B., Pados, T., Pretterebner, K., Schiemer, L., Steckbauer, A., Haselmair, A., Zuschin, M., and Stachowitsch, M.: Effect of hypoxia and anoxia on invertebrate behaviour: ecological perspectives from species to community level, Biogeosciences, 11, 1491–1518, https://doi.org/10.5194/bg-11-1491-2014, 2014.
Russell, C. E., Fernández, R., Parsons, D. R., and Gabbott, S. E.: Plastic pollution in riverbeds fundamentally affects natural sand transport processes, Commun. Earth Environ., 4, 1–10, https://doi.org/10.1038/s43247-023-00820-7, 2023.
Sadler, P. M.: Sediment accumulation rates and the completeness of stratigraphic sections, J. Geol., 89, 569–584, 1981.
Scarponi, D., Kaufman, D., Amorosi, A., and Kowalewski, M.: Sequence stratigraphy and the resolution of the fossil record, Geology, 41, 239–242, https://doi.org/10.1130/G33849.1, 2013.
Schaffner, L. C., Jonsson, P., Diaz, R. J., Rosenberg, R., and Gapcynski, P.: Benthic communities and bioturbation history of estuarine and coastal systems: effects of hypoxia and anoxia, in: Marine Coastal Eutrophication, edited by: Vollenweider, R. A., Marchetti, R., and Viviani, R., Elsevier, Amsterdam, 1001–1016, https://doi.org/10.1016/B978-0-444-89990-3.50087-0, 1992.
Schoellhamer, D. H.: Anthropogenic sediment resuspension mechanisms in a shallow microtidal estuary, Estuar. Coast. Shelf Sci., 43, 533–548, https://doi.org/10.1006/ecss.1996.0086, 1996.
Silbiger, N. J., Nelson, C. E., Remple, K., Sevilla, J. K., Quinlan, Z. A., Putnam, H. M., Fox, M. D., and Donahue, M. J.: Nutrient pollution disrupts key ecosystem functions on coral reefs, P. R. Soc. B, 285, 20172718, https://doi.org/10.1098/rspb.2017.2718, 2018.
Sulpis, O., Boudreau, B. P., Mucci, A., Jenkins, C., Trossman, D. S., Arbic, B. K., and Key, R. M.: Current CaCO3 dissolution at the seafloor caused by anthropogenic CO2, P. Natl. Acad. Sci. USA, 115, 11700–11705, https://doi.org/10.1073/pnas.1804250115, 2018.
Sulpis, O., Agrawal, P., Wolthers, M., Munhoven, G., Walker, M., and Middelburg, J. J.: Aragonite dissolution protects calcite at the seafloor, Nat. Commun., 13, 1104, https://doi.org/10.1038/s41467-022-28711-z, 2022.
Syvitski, J., Ángel, J. R., Saito, Y., Overeem, I., Vörösmarty, C. J., Wang, H., and Olago, D.: Earth's sediment cycle during the Anthropocene, Nat. Rev. Earth Environ., 3, 179–196, https://doi.org/10.1038/s43017-021-00253-w, 2022.
Thrush, S., Hewitt, J., Cummings, V., Ellis, J., Hatton, C., Lohrer, A., and Norkko, A.: Muddy waters: elevating sediment input to coastal and estuarine habitats, Front. Ecol. Environ., 2, 299–306, https://doi.org/10.1890/1540-9295(2004)002[0299:MWESIT]2.0.CO;2, 2004.
Tomašových, A. and Kidwell, S. M.: Nineteenth-century collapse of a benthic marine ecosystem on the open continental shelf, P. R. Soc. B, 284, 20170328, https://doi.org/10.1098/rspb.2017.0328, 2017.
Tomašových, A., Gallmetzer, I., Haselmair, A., Kaufman, D. S., Kralj, M., Cassin, D., Zonta, R., and Zuschin, M.: Tracing the effects of eutrophication on molluscan communities in sediment cores: outbreaks of an opportunistic species coincide with reduced bioturbation and high frequency of hypoxia in the Adriatic Sea, Paleobiology, 44, 575–602, https://doi.org/10.1017/pab.2018.22, 2018.
Tomašových, A., Kidwell, S. M., Alexander, C. R., and Kaufman, D. S.: Millennial-scale age offsets within fossil assemblages: result of bioturbation below the taphonomic active zone and out-of-phase production, Paleoceanogr. Paleocl., 34, 954–977, https://doi.org/10.1029/2018PA003553, 2019.
Tomašových, A., Albano, P. G., Fuksi, T., Gallmetzer, I., Haselmair, A., Kowalewski, M., Nawrot, R., Nerlović, V., Scarponi, D., and Zuschin, M.: Ecological regime shift preserved in the Anthropocene stratigraphic record, P. R. Soc. B., 287, 20200695, https://doi.org/10.1098/rspb.2020.0695, 2020.
Tomašových, A., Berensmeier, M., Gallmetzer, I., Haselmair, A., and Zuschin, M.: Pyrite-lined shells as indicators of inefficient bioirrigation in the Holocene–Anthropocene stratigraphic record, Biogeosciences, 18, 5929–5965, https://doi.org/10.5194/bg-18-5929-2021, 2021.
Tomašových, A., Gallmetzer, I., Haselmair, A., and Zuschin, M.: Inferring time averaging and hiatus durations in the stratigraphic record of high-frequency depositional sequences, Sedimentology, 69, 1083–1118, https://doi.org/10.1111/sed.12936, 2022.
Tomašových, A., Dominici, S., Nawrot, R., and Zuschin, M.: Temporal scales, sampling designs and age distributions in marine conservation palaeobiology, Geol. Soc. Lond. Spec. Publ., 529, 1–39, https://doi.org/10.1144/SP529-2022-361, 2023.
Tyrrell, T.: Anthropogenic modification of the oceans, Philos. T. Roy. Soc. A, 369, 887–908, https://doi.org/10.1098/rsta.2010.0334, 2011.
Wang, L. and Hou, D.: Plastistone: An emerging type of sedimentary rock, Earth-Sci. Rev., 247, 104620, https://doi.org/10.1016/j.earscirev.2023.104620, 2023.
Wang, Z., Saito, Y., Zhan, Q., Nian, X., Pan, D., Wang, L., Chen, T., Xie, J., Li, X., and Jiang, X.: Three-dimensional evolution of the Yangtze River mouth, China during the Holocene: impacts of sea level, climate and human activity, Earth-Sci. Rev., 185, 938–955, https://doi.org/10.1016/j.earscirev.2018.08.012, 2018.
Wapnick, C. M., Precht, W. F., and Aronson, R. B.: Millennial-scale dynamics of staghorn coral in Discovery Bay, Jamaica, Ecol. Lett., 7, 354–361, https://doi.org/10.1111/j.1461-0248.2004.00586.x, 2004.
Watson, S. J., Ribó, M., Seabrook, S., Strachan, L. J., Hale, R., and Lamarche, G.: The footprint of ship anchoring on the seafloor, Sci. Rep., 12, 7500, https://doi.org/10.1038/s41598-022-11627-5, 2022.
Weinert, M., Kröncke, I., Meyer, J., Mathis, M., Pohlmann, T., and Reiss, H.: Benthic ecosystem functioning under climate change: modelling the bioturbation potential for benthic key species in the southern North Sea, PeerJ, 10, e14105, https://doi.org/10.7717/peerj.14105, 2022.
Wilkinson, B. H.: Humans as geologic agents: A deep-time perspective, Geology, 33, 161–164, https://doi.org/10.1130/G21108.1, 2005.
Yasuhara, M., Rabalais, N. N., Conley, D. J., and Gutierrez, D.: Palaeo-records of histories of deoxygenation and its ecosystem impact, in: Ocean Deoxygenation: Everyone's Problem – Causes, Impacts, Consequences and Solutions, edited by: Laffoley, B. and Baxter, J. M., IUCN, Gland, Switzerland, 213–224, 2019.
Yasuhara, M., Huang, H.-H. M., Hull, P., Rillo, M. C., Condamine, F. L., Tittensor, D. P., Kučera, M., Costello, M. J., Finnegan, S., and O'Dea, A.: Time machine biology: Cross-timescale integration of ecology, evolution, and oceanography, Oceanography, 33, 16–28, https://doi.org/10.5670/oceanog.2020.225, 2020.
Zalasiewicz, J., Waters, C. N., and Williams, M.: Human bioturbation, and the subterranean landscape of the Anthropocene, Anthropocene, 6, 3–9, https://doi.org/10.1016/j.ancene.2014.07.002, 2014.
- Abstract
- Introduction
- What determines the quality of geohistorical records?
- Anthropogenic processes altering geohistorical records
- Interpreting marine geohistorical records of the Anthropocene
- Conclusions
- Data availability
- Author contributions
- Competing interests
- Disclaimer
- Acknowledgements
- Financial support
- Review statement
- References
- Abstract
- Introduction
- What determines the quality of geohistorical records?
- Anthropogenic processes altering geohistorical records
- Interpreting marine geohistorical records of the Anthropocene
- Conclusions
- Data availability
- Author contributions
- Competing interests
- Disclaimer
- Acknowledgements
- Financial support
- Review statement
- References