the Creative Commons Attribution 4.0 License.
the Creative Commons Attribution 4.0 License.
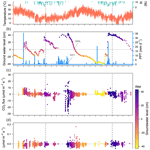
Drought conditions disrupt atmospheric carbon uptake in a Mediterranean saline lake
Ihab Alfadhel
Ignacio Peralta-Maraver
Isabel Reche
Enrique P. Sánchez-Cañete
Sergio Aranda-Barranco
Eva Rodríguez-Velasco
Andrew S. Kowalski
Penélope Serrano-Ortiz
Inland saline lakes play a key role in the global carbon cycle, acting as dynamic zones for atmospheric carbon exchange and storage. Given the global decline of saline lakes and the expected increase of periods of drought in a climate change scenario, changes in their potential capacity to uptake or emit atmospheric carbon are expected. Here, we conducted continuous measurements of CO2 and CH4 fluxes at the ecosystem scale in an endorheic saline lake of the Mediterranean region over nearly 2 years. Our focus was on determining net CO2 and CH4 exchanges with the atmosphere under both dry and flooded conditions, using the eddy covariance (EC) method. We coupled greenhouse gas flux measurements with water storage and analysed meteorological variables like air temperature and radiation, known to influence carbon fluxes in lakes. This extensive data integration enabled the projection of the net carbon flux over time, accounting for both dry and wet conditions on an interannual scale. We found that the system acts as a substantial carbon sink by absorbing atmospheric CO2 under wet conditions. In years with prolonged water storage, it is predicted that the lake's CO2 assimilation capacity can surpass 0.7 kg C m2 annually. Conversely, during extended drought years, a reduction in CO2 uptake capacity of more than 80 % is expected. Regarding CH4, we measured uptake rates that exceeded those of well-aerated soils such as forest soils or grasslands, reaching values of 0.2 µmol m−2 s−1. Additionally, we observed that CH4 uptake during dry conditions was nearly double that of wet conditions. However, the absence of continuous data prevented us from correlating CH4 uptake processes with potential environmental predictors. Our study challenges the widespread notion that wetlands are universally greenhouse gas emitters, highlighting the significant role that endorheic saline lakes can play as a natural sink of atmospheric carbon. However, our work also underscores the vulnerability of these ecosystem services in the current climate change scenario, where drought episodes are expected to become more frequent and intense in the coming years.
- Article
(5979 KB) - Full-text XML
-
Supplement
(3892 KB) - BibTeX
- EndNote
Inland saline lakes are diverse and play a crucial role in the global carbon cycle, serving as dynamic zones for carbon dioxide exchange with the atmosphere (Li et al., 2022; Liao et al., 2024) and long-term sinks of organic and inorganic carbon (Anderson and Stedmon, 2007; Song et al., 2013; Li et al., 2017). In limnology, however, the ecological importance of these systems has only recently been recognized, despite accounting for approximately 44 % of global lake volume and 23 % of lake surface area (Messager et al., 2016). Saline lakes vary in size from ephemeral ponds to extensive deep-water bodies, such as the Caspian Sea (Eugster and Hardie, 1978). These lakes are characterized by salinity levels that exceed 3 parts per thousand and are notably isolated from a direct marine influence (Williams, 2002; Wang et al., 2018). They are found in endorheic (hydrologically landlocked) basins across a wide array of climates, spanning cold to warm/hot arid regions in all continents, including Antarctica (Williams, 2002; Wang et al., 2018). As terminal points in many hydrological networks, they collect significant amounts of not only salts but also nutrients and organic and inorganic carbon (Anderson and Stedmon, 2007; Song et al., 2013; Batanero et al., 2017; Li et al., 2017; Liao et al., 2024).
Past estimations about the role of saline lakes on global carbon fluxes suggested that these lakes might function as hotspots for the CO2 emission (Duarte et al., 2008). However, more recent works point out that saline lakes have lower partial pressures of CO2 than freshwater lakes (Wen et al., 2017), and some systems appear to take up CO2 during the winter (Li et al., 2022) or even annually (Yang et al., 2021) due to physicochemical reactions and increased activity of primary producers. Therefore, more seasonal studies on CO2 fluxes in saline lakes are needed to understand the conditions when these systems behave as sinks or sources of CO2. Variations in CO2 and CH4 flux estimates across different studies of water bodies are primarily due to the highly variable data obtained from discrete sampling (Li et al., 2022) or because of differences in sampling seasons at the intra-annual scale (Liao et al., 2024). Meanwhile, gathering continuous time series data on CO2 and CH4 sequestration and emission fluxes over years is needed for accurate assessment of the net carbon balance in inland water systems (Martínez-García et al., 2024). Nevertheless, long-term, uninterrupted, and direct monitoring of greenhouse gas flux dynamics at the ecosystem level is relatively scarce in aquatic ecosystems, and this is particularly true for saline lakes. To the best of our knowledge, only a couple of studies have reported continuous year-round direct measurements at the ecosystem scale for CO2 fluxes (Yang et al., 2021; Li et al., 2022). However, saline lake characteristics differ with latitude (Hammer, 1986) and could have very different behaviours regarding carbon exchanges depending on climate conditions.
The carbon hydrochemistry in permanent saline lakes, especially in mountainous and Arctic latitudes such as the Tibetan Plateau or Svalbard, is largely influenced by surface ice formation (Anderson et al., 2024; Rysgaard et al., 2012, 2013; Wu et al., 2014; Yan and Zheng, 2015). In contrast, saline lakes in arid and semi-arid endorheic basins, including Mediterranean climates, are typically shallow, often ephemeral, and/or hypersaline due to evaporation exceeding precipitation (García et al., 1997; Batanero et al., 2017; Saccò et al., 2021). The lower depths and higher surface-to-volume ratio, driven by drought conditions, induce significant physicochemical fluctuations in these inland saline water bodies, spanning from diurnal to interannual scales (Comin et al., 1990; García and Niell, 1991; García et al., 1997; Batanero et al., 2017). Consequently, the precipitation regime and subsequent changes in groundwater levels determine the ecology of saline lakes in arid and semi-arid regions. However, research on the interannual variability of carbon fluxes in saline lakes affected by seasonal flooding and drought is lacking. This knowledge gap urgently requires focused research to elucidate the impacts of climatic variability on the carbon dynamics of these ecosystems, which have been identified as particularly vulnerable to climate fluctuations (Tweed et al., 2011). Furthermore, recent studies highlight a global decline in lake water storage in most endorheic basins and in the Sahara, Arabia, and the southern Europe basin in particular (Wang et al., 2018), a situation expected to worsen with more severe droughts in a climate change scenario, leading to lower water levels and prolonged desiccation periods (Wurtsbaugh et al., 2017; Hassani et al., 2020).
In this study, we carried out continuous and interannual measurements of CO2 and CH4 fluxes at the ecosystem level in Spain's saline lake Fuente de Piedra using the eddy covariance (EC) method. Serving as a model of a Mediterranean shallow saline lake, it is characterized by sporadic episodes of water retention but is predominantly dry during the summer. It is worth noting that Fuente de Piedra Lake was designated as a Ramsar site in 1983, ensuring a rich history of monitoring water storage and the various meteorological drivers of CO2 and CH4 fluxes. The objectives of our work are (a) to quantify carbon exchanges during the dry and the flooded conditions, determining its role as a carbon source or sink; (b) to evaluate main drivers promoting carbon exchange behaviours; and finally (c) to use available records of the time series for the main drivers of fluxes to model the annual net carbon flux of the system in the past. Our research aims to enhance our understanding of the carbon dynamics and the impacts of climate change on the net carbon balance in Mediterranean intermittent endorheic lakes.
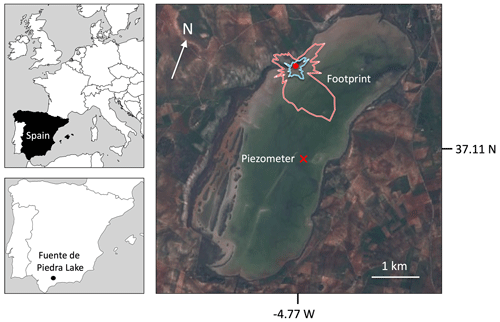
Figure 1Location of the Fuente de Piedra Lake (province of Málaga, southern Spain). Dot inside the polygon in the right panel shows the location of the eddy covariance tower. The areas within the footprint contributing the 90 % to measured fluxes are delimited inside polygons for daytime (blue) and night-time (red). The cross indicates the location of the piezometer used for measuring groundwater level. Source image: Sentinel-2. Wet image was taken on 17 February 2021.
2.1 Study site
Fuente de Piedra is a shallow and saline lake located in an endorheic basin in the province of Málaga, Andalusia, Spain (37.11° N, 4.77° W; elevation 410 m: Fig. 1). It spans approximately 17 km2, measuring 6.8 km in length and 2.5 km in width, with a maximum depth of 1.5 m. We take advantage of Fuente de Piedra Lake's inclusion in the Ramsar Convention in 1983. This designation ensures a rich history in the monitoring of water storage and the meteorological drivers discussed in this article. Such a comprehensive dataset allows for the back projection of the net carbon flux of the system over time, incorporating both dry and wet conditions at an interannual scale. This lake is recognized as a vital habitat within a protected wetland at various levels – regional (as a natural reserve), European (designated as a special bird protection area), and international (acknowledged as a Ramsar site) – and offers an exemplary nesting ground for the pink flamingo (Phoenicopterus roseus), largely due to its shallow waters. Among primary producers, diatoms constitute the largest fraction of primary producers of phytoplankton throughout the year, being dominated by Hantzschia amphioxys, Amphora coffeaeformis, Stauronensis amphioxys, Cocconeis placentula, and Entomoneis sp. and several species of Navicula and Nitzschia sp. (García and Niell, 1993).
Salinity levels in the lake vary significantly, ranging from oligosaline (5 ppt) to hypersaline conditions (>200 ppt), influenced by the annual hydrological cycle (Batanero et al., 2017). This cycle is delineated into two distinct phases: a pooling phase during autumn and winter (December to March) and an evaporative and drought phase spanning spring and summer (April to November). The lake primarily receives water from groundwater inflow (Rodríguez-Rodríguez et al., 2006), complemented by contributions from two streams (Fig. 1) and surface runoff from surrounding farmlands. Notably, the stream entering from the northeast adds nutrients. However, sediment samples distributed across the lake and analysed through combustion (Heiri et al., 2001) showed it to be homogeneous in organic carbon (0.21±0.07 mg C), nitrogen (0.015±0.004 mg N), and the C : N ratio (14.4±2.3).
2.2 Field measurements of greenhouse gas fluxes and meteorological drivers
We employed the eddy covariance method to quantify the exchanges of CO2, CH4, and energy (sensible and latent heat) every 30 min from August 2021 to May 2023. Thus, the eddy covariance system was operated for more than 21 months, including two dry periods in summer. An open-path eddy covariance (EC) system was strategically positioned atop a tower, 3.1 m above ground level, on the western bank of the lake (Fig. 1). This setup included two open-path infrared gas analysers: the LI7500 for CO2 and water vapour and the LI7700 for CH4 (LI-COR Inc., Lincoln, NE, USA). Wind vector components (u, v, w) and sonic temperature were accurately measured using a sonic anemometer (R. M. Young 81000V, Traverse City, MI, USA). These data were recorded on a data logger (CR1000, Campbell Scientific, Logan, UT, USA) at a frequency of 10 Hz.
In addition to gas measurements, we measured a comprehensive suite of environmental and soil state variables every 10 s to capture the conditions over a representative ground surface area and collected every 30 min average by a data logger (CR1000, Campbell Scientific, Logan, UT, USA). Air temperature (Ta) and relative humidity (RH) were monitored using a thermohygrometer (HMP 45C, Campbell Scientific, Logan, UT, USA). Net radiation (Rn) was quantified by a net radiometer (NR Lite, Kipp & Zonen, Delft, Netherlands). Soil heat flux (G) calculations, including the energy stored in the soil, were facilitated by one heat flux plate (HFP01SC, Hukseflux, Delft, Netherlands) placed at 8 cm depth, complemented by three pairs of soil temperature probes (TCAV, Campbell Scientific, Logan, UT, USA) situated at depths of 4 cm and lateral distances of 3.20, 6.34, and 8.90 m from the tower.
The groundwater level (GWL) was monitored daily using a piezometer situated within a well in the salt flats, approximately 2 km south of the EC tower and on the opposite side of the lake (37.1071° N, 4.7631° W; Fig. S1 in the Supplement). The location of the piezometer coincides with the central and deepest part of the lake when there is water. Negative GWL values indicate conditions when the lake is entirely lacking surface water. Positive values measured by the piezometer were used to determine the lake depth. Furthermore, data on daily precipitation (PPT), air temperature, and incident solar radiation (spanning wavelengths from 350 to 1100 nm) were acquired from a meteorological station located adjacent to Fuente de Piedra Lake, in Sierra de Yeguas (37.1383° N, 4.8358° W; 467 m a.s.l.). The tower setup and instruments were maintained (mainly cleaning lenses of the open-path sensors) every 2 weeks.
2.3 Greenhouse gas flux data processing, quality control, and partitioning
Half-hourly means (48 measurements per day), variances, and covariances of greenhouse gas fluxes, adhering to the principles of the Reynolds decomposition, were calculated using the EddyPro® 7.0.7 software (LI-COR), according to international standards and protocols (Sabbatini et al., 2018). The data processing protocol encompassed the following steps: (1) axis rotation for tilt correction using the double-rotation method (Wilczak et al., 2001), (2) turbulent fluctuations calculated using the block-averaging method, (3) time lag that was compensated by covariance maximization with default, (4) Webb–Pearman–Leuning (WPL) correction of air density fluctuation (Webb et al., 1980), (5) despiking and raw data statistical screening (Vickers and Mahrt, 1997), and (6) spectral corrections of high- and low-pass filtering effects. Regarding the latter, high-frequency loss due to path averaging, signal attenuation, and sensor separation was compensated according to Moncrieff et al. (1997), whereas low-frequency loss due to finite time-averaging length and detrending was corrected according to Moncrieff et al. (2004). Quality check flags were calculated for flux data according to the widely adopted methodology combining two tests: the steady-state test and the developed turbulent conditions test. Over the study period, we only selected high-quality fluxes (flag value =0) measured when the open-path sensors were totally clean according to their respective automatic gain control (AGC) values (AGC value equal to or above 56 for open-path LI-7500A CO2/H2O analyser and AGC value equal to or higher than 20 for LI-7700).
To quantify the sampling area of flux measurements, a footprint model was estimated using the method by Kljun et al. (2004; Fig. 1). Data periods when the wind came from the adjacent terrestrial environment (251 to 59°) were rejected, representing between 45 % and 70 % of the available daytime and night-time data respectively during the dry season (GWL ≤0) and 30 % of the available daytime and night-time data respectively during the wet seasons (GWL >0). Overall, for the nearly 2 years of measurements, 18 % and 8 % of the potential daytime data were of good quality for CO2 and CH4 fluxes respectively. For night-time, the available data were reduced to 10 % and 5 % respectively. In order to provide additional information regarding the turbulent flux quality (Moncrieff et al., 1997), we calculated the energy balance closure (ratio of the sum of sensible and latent turbulent fluxes, H+ LE, to the net radiation minus soil heat flux, Rn−G) using all the available data during the drought period. The obtained value was 76 % (R2=0.64; n=3117) and is within the range of most of the reported FLUXNET sites (Wilson et al., 2002; Stoy et al., 2013).
2.4 Predicting greenhouse gas fluxes as responses to meteorological drivers
We examine the relationship of CO2 and CH4 fluxes in response to groundwater level, serving as a proxy for long-term water storage, with air temperature as the main factor regulating respiration in the system and incident solar radiation modulating the photosynthetic rate. Since these variables were measured every 24 h, we processed half-hourly CO2 and CH4 fluxes collected to construct 24 h integrated values. It is important to note that gaps in long-term data records are inevitable when using eddy covariance, primarily due to instrument failure and insufficient turbulence (Falge et al., 2001; Baldocchi, 2003; Aubinet et al., 2012). Additionally, data coverage is often reduced when wind originates from undesirable sectors, though this does not necessarily compromise measurement quality (Goloub et al., 2023). Despite these limitations, most studies derive their primary results from annual or seasonal CO2 and CH4 balances using various gap-filling procedures. In contrast, we exercise greater caution with our limited data coverage, being very restrictive in quantifying daily fluxes. We selected dates with over 50 % of the anticipated data points, particularly those with more than 25 valid measurements well-distributed throughout the day, to calculate integrated daily flux. Before integrating daily flux values, we filled gaps in the half-hourly CO2 and CH4 flux data using linear interpolation for missing values. This selection criterion aimed to accurately represent the daily pattern in flux measurement distribution. Then, a trapezoidal integration of the values measured every 30 minutes was performed to calculate the daily flux.
To analyse the relationship between integrated daily fluxes and their potential environmental predictors, we employed a linear regression combined with a forward model selection technique (Aho et al., 2014). This method involved sequentially fitting a series of regression models, each incorporating different combinations of predictors and their interactions. The process began with simple models, each containing only one primary predictor, and gradually increased in complexity to include all possible interactions among predictors. We then used the Akaike information criterion (AIC) to evaluate and identify the most effective model from the set. The model with the lowest AIC was selected as the best fit, indicating it provides the most useful balance between model complexity and explanatory power. After selecting the model, we examined the influence of the predictors by analysing the slope β coefficients at a significance level of alpha =0.05, using a 95 % confidence interval to determine whether these coefficients were significantly different from zero. Additionally, while groundwater level (GWL) was measured daily, our model selection process also aimed to identify which daily measurements of air temperature and incident solar radiation (mean, minimum, or maximum) were the best predictors. This method ensured that the chosen model is robust and relevant to the ecological scales being studied.
Finally, the chosen candidate model for predicting the fluxes in the system was fitted using available records for the studied drivers, covering the period from 2001 to the present at Fuente de Piedra Lake. This approach aimed to retrospectively model the fluxes in the system. Detailed instructions on how to run these analyses are in the R script available in DRYAD (see details in the “Data availability” section).
3.1 Time series of greenhouse gas emissions and meteorological drivers
At Fuente de Piedra Lake, we observed significant seasonal variations in meteorological conditions, as illustrated in Fig. 2a. Air temperature (Ta) and incident solar radiation exhibited consistent trends throughout the study period, with mean daily values of 17±7 °C for Ta and 18±8 MJ m−2 d−1 for incident solar radiation. In contrast, these two environmental variables generally followed asynchronous patterns with groundwater level (GWL) and precipitation events, except for a brief peak in precipitation and an increase in GWL between August and September 2021 (Fig. 2a and b). Particularly during the summer months (July, August, September), the highest daily air temperature values coincided with GWLs beneath the surface and a lack of precipitation. Also, during dry conditions, a salt crust several centimetres thick developed on the sediment (see Fig. 3b). For instance, the minimum Ta recorded was 2 °C in January 2023, corresponding with a period of frequent precipitation and GWL above the surface. Conversely, the maximum Ta of 34 °C occurred in August 2021, during a period when the GWL was 24 cm below the surface.
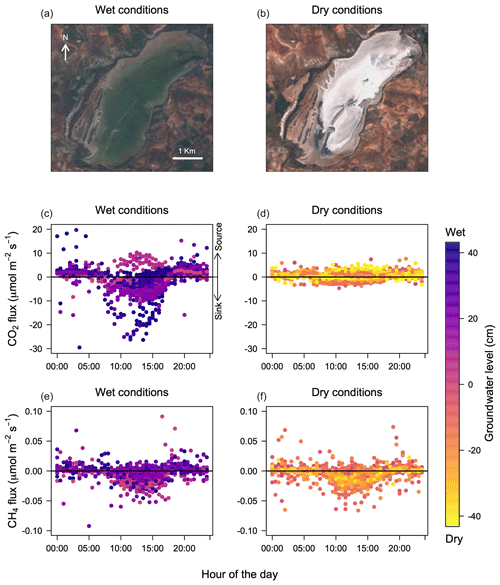
Figure 3Aerial photos of Fuente de Piedra Lake during a period of maximum water availability (wet, a) and during a typical dry episode (b). Note that for some periods during the dry episodes, a salt crust forms, covering practically the entire extent of the lake. The figure shows the daily pattern of CO2 and CH4 fluxes during wet conditions (c and e respectively) and dry conditions (d and f respectively). Water availability is measured in terms of groundwater level. Source image: Sentinel-2. Wet image was taken on 17 February 2021, and dry image was taken on 13 May 2021.
After the study period, we were able to collect 4128 measurements for CO2 fluxes and 2425 measurements for CH4 fluxes, which were relatively well-distributed over the study period. We observed a strong correspondence between water storage in Fuente de Piedra Lake and its capacity to assimilate atmospheric CO2. The CO2 flux patterns can be categorized into two distinct lake states: a flooded lake (groundwater level >0 cm, indicated from purple to blue colour in Fig. 2c and d) and a dry lake (groundwater level <0 cm, shown from orange to yellow colour). During flooding conditions, the lake acted as a CO2 sink, with fluxes ranging from 0 to −30 µmol m−2 s−1. The CO2 assimilation capacity increases with groundwater level and incident solar radiation, particularly from January to June.
Notably, the flooded conditions in the 2 years of the study showed marked differences in groundwater level. In 2022, we recorded the highest CO2 uptake of 30 µmol m−2 s−1 and an averaged value of −3.11 µmol m−2 s−1 during May, when the groundwater level was at its peak, 40 cm above the surface. This period was also characterized by high variability in CO2 flux, occasionally showing peaks of CO2 outgassing approaching 20 µmol m−2 s−1. The peak CO2 uptake in 2023 was approximately half of what was observed in 2022. Although this peak occurred in March rather than May, it followed a similar trend to the groundwater level (GWL), which was also about half of the peak level observed in 2022 (∼20 cm above the surface) resulting in averaged CO2 uptake values of −3.66 µmol m−2 s−1. In contrast, under dry conditions, Fuente de Piedra Lake ceases CO2 uptake, occasionally transitioning to minor CO2 emissions. Notice that during extreme rainfall pulses within dry conditions, when Fuente de Piedra Lake remained relatively dry with the groundwater level below the surface, we observed notable net CO2 emissions. For example, a heavy rainfall event in September 2021 (36 mm d−1) resulted in CO2 emissions reaching up to 10 µmol m−2 s−1, with a high elevation of the groundwater level above the surface (from −20 to near 20 cm). Also, in October of both 2021 and 2022, subsequent rainfall events (12 and 22 mm d−1, respectively) corresponded with CO2 emissions of 7 and 5.5 µmol m−2 s−1, respectively. In these cases, emissions occurred under negative GWL conditions.
In the case of CH4, the flux was relatively stable throughout the whole study period, generally acting as a sink, with values fluctuating between −0.2 and 0.1 µmol m−2 s−1 and an average of −0.05 µmol m−2 s−1 for the study period (Fig. 2d). Furthermore, the measured CH4 fluxes did not seem to align clearly with the meteorological variables examined (Fig. S1).
3.2 Fluxes of CO2 and CH4 at a daily scale
When examining the daily scale during wet conditions, it becomes evident that CO2 assimilation predominantly takes place during daylight hours, specifically between 09:00 and 14:00 (local time) (Fig. 3). It should be noted that the few emission values observed within this time frame correspond to the emission occurrences described earlier for the dry season, which promptly followed rainfall events. In the case of dry conditions, it is noteworthy that a salt crust forms over the lake, leading to a near cessation of CO2 fluxes. In the case of CH4, there is also a discernible increase in assimilation fluxes between 09:00 and 14:00 LT. This observed pattern is consistent in both wet and dry conditions.
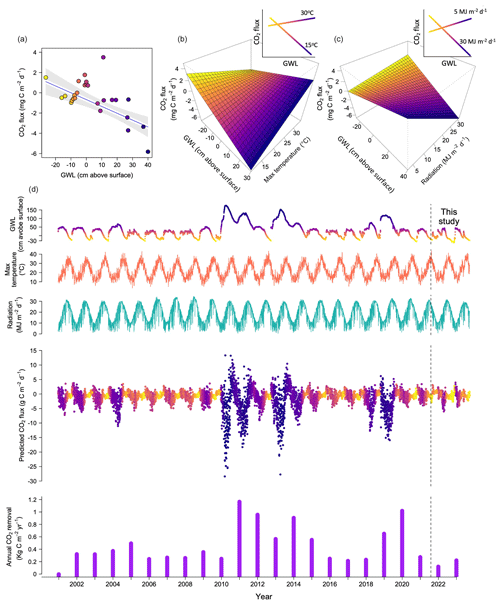
Figure 4Prediction of CO2 flux as a response to groundwater level with a slope of −0.115 (a), the interaction between groundwater level and daily maximum temperature (b), and the interaction between groundwater level and incident solar radiation (c). Model has an R2 of 0.73. Using existing time series for the model predictors, it has been possible to reconstruct the estimated CO2 fluxes, as well as the annual cumulative value of CO2 removal since 2001 for Fuente de Piedra Lake (d).
3.3 Model predictions of 24 h integrated flux values in the study system
No evident relationship between CH4 flux and the environmental predictors studied was found during the study period. Additionally, measurements obtained from the EC tower resulted in a substantial number of gaps in the CH4 time series, making it impossible to establish a predictive model for these fluxes. On the contrary, we were able to adjust a robust regression model for CO2 integrated daily flux. A total of 26 CO2 integrated daily flux values were obtained for the sampling period, analysing those dates when more than 25 valid measurements were available. After AIC model selection routine, the candidate predictive model for daily CO2 integrated flux was determined to include groundwater level, maximum daily air temperature, and mean incident solar radiation. Additionally, the model incorporated interactions between groundwater level and maximum daily air temperature, as well as between groundwater level and incident solar radiation (a summary table of the model is available in the Supplement). Indeed, the fitted statistical model has a relatively high explanatory capability (adjusted R2=0.73).
The model confirmed a positive effect of groundwater level on enhancing CO2 assimilation in the system (Fig. 4a; , 95 % CI to −0.016; note that assimilation corresponds with negative values of the CO2 flux). While the isolated impact of air temperature increase on CO2 assimilation could not be determined (, 95 %CI to +0.192), the model identified an antagonistic interaction between air temperature and groundwater level (, 95 %CI to +0.015). As air temperature increases, the positive effect of GWL on CO2 assimilation diminishes (Fig. 4b). Conversely, mean daily incident solar radiation was found to promote CO2 assimilation (2, 95 %CI to −0.03), with a pronounced synergy between mean daily incident solar radiation and the presence of water (CI to −0.003), notably enhancing the capacity for CO2 assimilation (Fig. 4c).
Using time series data for groundwater level, daily maximum air temperature, and mean daily incident solar radiation at Fuente de Piedra Lake, we generated retrospective predictions of CO2 assimilation capacity in the system dating back to 2001 (Fig. 4d). The model predictions closely aligned with the observed values for the study period when using the time series data for the predictors, supporting the robust predictive ability of our model (Fig. S2). Our estimates indicate a pronounced fluctuation in CO2 assimilation capacity according to hydrological variations. In years with higher groundwater level and prolonged water storage, the model predicted an exceptionally high capacity for atmospheric CO2 assimilation of the lake, with annual values surpassing 0.7 kg C m−2 yr−1 (e.g. in 2011, 2012, 2014, 2020). In contrast, during years marked by extended droughts, a substantial reduction in CO2 assimilation capacity was modelled, with predicted reductions exceeding 80 % compared to wetter years (Fig. 4d). These drought periods resulted in a reduction of the assimilation capacity to less than a third of the levels recorded in wet years (e.g. from 2006 to 2010).
In agreement with previous research on permanent saline lakes of the Tibetan Plateau (Li et al., 2022), we demonstrate that a model Mediterranean shallow saline lake serves as a substantial carbon sink, absorbing atmospheric CO2 when flooded. In particularly wet years, expected uptake values can exceed 1.2 kg C m−2 yr−1. Sporadic instances were also observed where the system acted as a CO2 emitter, resulting from rewetting events that followed rainfall during dry conditions. Conversely, CO2 uptake ceases during dry conditions, reducing the system's capacity in dry years to below 0.2 kg C m−2 yr−1. Longitudinal time series analysis reveals that prolonged droughts indeed hinder the ability of the system to assimilate atmospheric CO2 due to the lack of water, but we also observed that an increase in air temperature during wet conditions moderates the CO2 net assimilation capacity, a process likely related to the reduction of gas–water solubility with temperature. This underscores the pronounced impact of seasonal and interannual variability, ultimately dictated by drought and rainfall patterns, on the ability of the studied system to sequester atmospheric carbon. Moreover, this pattern also displayed considerable variability at the daily scale, closely correlating with fluctuations in incident solar radiation over daily cycles. In this regard, the CO2 assimilation capacity of the system peaked during those hours of maximum incident solar radiation. While measurement of CO2 (and CH4) fluxes at multiple scales is challenging and requires specialized equipment (i.e. eddy covariance sensors), our research proposes an alternative proxy. By integrating data from environmental predictors at various scales, we have been able to reconstruct the behaviour of CO2 exchanges between Fuente de Piedra Lake and the atmosphere. In essence, we estimate CO2 flux through the continuous measuring of accessible environmental variables (i.e. the amount of water, air temperature, and incident solar radiation).
In shallow, well-mixed, and oxygenated systems like Fuente de Piedra Lake, the photosynthetic capacity of the phytoplankton community is closely linked to the water column height (i.e. groundwater level; Batanero et al., 2017), promoting CO2 assimilation as the extent of the habitat for these communities expands (Wetzel, 2001). Related to the latter, a significant synergy exists between water storage in the ecosystem and incident radiation, serving as a proxy for the photosynthetically active radiation upon which photosynthesis depends. This interaction occurs on both a daily scale, associated with variations in light intensity following day–night cycles, and an annual seasonal scale, largely determined by changes in daylight hours throughout the year. Notably, during the night, the net exchange of CO2 between the water and the atmosphere in Fuente de Piedra Lake is negligible. This could be attributed to the absence of photosynthesis during night-time. Additionally, the high salinity inherent to these environments constrains methanogenesis, which is the least energy-efficient carbon mineralization process in the redox sequence (reviewed in Soued et al., 2024). Considering the above, it appears to offer a plausible explanation for why microbial respiration does not surpass inorganic carbon assimilation through photosynthesis in systems like Fuente de Piedra Lake during wet conditions, despite the high content of dissolved organic carbon ranging between 1.00 and 13.59 mmol C L−1 (Batanero et al., 2017).
What is more, despite the limited CH4 flux data, our results position Fuente de Piedra Lake as a CH4 sink. Rough estimates determined that Fuente de Piedra could take up on average 1.83 and 3.70 mg C m−2 d−1 during wet and the dry conditions respectively (Fig. S3). Such values are even higher than those measured in typical well-aerated soils as in forests or grasslands, with average rates of 0.4–1.26 mg C m−2 d−1 (Murguia-Flores et al., 2021; Perez-Quezada et al., 2021). Twice the value of uptake during dry conditions compared to wet ones appears to be consistent with some proposed mechanisms promoting CH4 reduction according to the existing literature, since the increase of temperature together with gas diffusivity due to loss of water may increase methane oxidation in a similar way to terrestrial ecosystems (Chen et al., 2011; Rafalska et al., 2023). However, caution is needed when interpreting our results, as the dynamics of methane fluxes could become very complex in an intermittent system like Fuente de Piedra. On the one hand, just as methanogenic activity is inhibited by salinity (Herbert et al., 2015), methanotrophic activity has also been observed to be significantly reduced by salinity in terrestrial systems (Ho et al., 2018). However, methane oxidation processes associated with aquatic prokaryotes may be more resistant to salinity (Khmelenina et al., 2010; Deng et al., 2017), especially if the variation is gradual (Osudar et al., 2017). Thus, further measurements and analysis are needed to estimate the role of methane oxidation and the relevance of intermittent saline lakes as CH4 sinks in a climate change scenario.
Drought conditions are accompanied by an increase in air temperature, with the high air temperatures recorded immediately before the system completely dries out. We have found that this rise in air temperature leads to a reduction of the system's capacity to assimilate CO2, even during wet conditions. A direct consequence of climatic warming is the reduction of gas solubility accentuated in saline wetlands (Batanero et al., 2022). In addition, an increase in temperature can enhance microbial metabolic rates and, therefore, biomass-specific CO2 production (Smith et al., 2019). Given that endorheic saline lakes are fuelled by significant amounts of organic matter (Li et al., 2017; Batanero et al., 2017; Song et al., 2013), it is unsurprising that warming leads to a decrease in net primary production in the system as a result of enhanced microbial respiration and, consequently, a reduction in CO2 assimilation capacity. In addition, carbon emissions in inland waters could increase with warming, independently of organic carbon inputs, simply because the apparent activation energy is predicted to be higher for respiration than for photosynthesis (Yvon-Durocher et al., 2010, 2012). Finally, it has been recognized that photosynthesis is often the first process to be affected by environmental stressors, with photosynthetic capacity diminishing prior to other cellular functions (Feller, 2016; Cardona et al., 2018). Specifically, carbon assimilation through the Calvin–Benson cycle exhibits particular vulnerability to both drought and elevated temperatures, occurring even when photosynthetic electron transport continues to operate effectively (Sharkey, 2005). On the whole, we show the profound synergy between global warming and intensifying drought severity and frequency, disrupting the CO2 assimilation capacity of Mediterranean saline lakes and leading to negative feedback loops.
While the desiccation of saline lakes is not novel, with researchers highlighting the concerning increase in dry periods within many of these ecosystems over recent decades (Williams, 1993; Wurtsbaugh et al., 2017; Wang et al., 2018), our study underscores the significant implications this trend has for the ecosystem services they support. Our retrospective predictions show that in wet years, the system could exhibit a high CO2 assimilation rate. For instance, between 2010 and 2015, we estimated that Fuente de Piedra Lake had an average assimilation rate of 0.83 (SD 7) kg C m−2 yr−1, within the same range as the net assimilation observed in evergreen or deciduous forest systems worldwide (Pastorello et al., 2020) and marine salt marshes (Mayen et al., 2024). Considering the 13.6 km2 area of Fuente de Piedra and assuming a constant flux across the lake surface when flooded, we could estimate around 11 t of carbon sequestered during a wet and fully flooded year. Although this upscaling exercise is crude, it highlights that Mediterranean shallow saline lakes could be a substantial carbon sink and underline the potential keeping them inundated with water during their conservation policies. Therefore, further studies across different Mediterranean shallow saline lakes are necessary to refine these estimates and enhance our understanding of their role in the global-scale carbon budget.
Our result challenges the generalized belief that inland waters primarily act as sources of greenhouse gases (Raymond et al., 2013). Conversely, the system undergoes significant reductions in its annual atmospheric CO2 sequestration capacity during dry conditions. For instance, under severe drought conditions as observed in Fuente de Piedra from 2005 to 2009, the annual CO2 sequestration is estimated to have fallen to less than a quarter of what was observed in more humid conditions. Climate change projections, including even the most optimistic scenarios, forecast an increase in both the frequency and duration of heatwaves and droughts in the coming years (Trenberth, 2011; Perkins-Kirkpatrick, 2020). This implies that saline lake ecosystems in arid and semi-arid endorheic basins will remain dry for longer periods or may even vanish, resulting in the loss of a significant carbon sequestration pathway. Importantly, the disappearance of saline lakes due to water scarcity has been largely attributed to anthropogenic water overuse (i.e. agriculture) rather than to macroclimatic phenomena (Wurtsbaugh et al., 2017). This seems to be the case of Fuente de Piedra Lake, as the catchment area is dominated by agricultural land. Thus, a proper water system management during drought conditions seems to be the most plausible solution to preserve the ecosystem services provided by Mediterranean saline lakes.
The R script used to conduct the data analysis and the datasets are available in the Dryad Digital Repository: https://doi.org/10.5061/dryad.r4xgxd2nn (Alfadhel et al., 2024).
The supplement related to this article is available online at: https://doi.org/10.5194/bg-21-5117-2024-supplement.
PSO and IR conceived the study; all authors contributed to the installation and maintenance of the eddy covariance tower; IA led the fieldwork and the processing of the samples with the help of the rest of the authors; IPM carried out the analyses and the preparation of the results; IA, IPM, and PSO conducted the preparation of the first draft of the work; all authors participated in the drafting of the final draft.
The contact author has declared that none of the authors has any competing interests.
Publisher's note: Copernicus Publications remains neutral with regard to jurisdictional claims made in the text, published maps, institutional affiliations, or any other geographical representation in this paper. While Copernicus Publications makes every effort to include appropriate place names, the final responsibility lies with the authors.
We are thankful for the logistic support and groundwater level data provided by Manuel Rendón Martos and África Lupión Sánchez of the Natural Reserve of Laguna de Fuente de Piedra. The authors are also grateful for the reviews provided by the anonymous reviewers.
This research has been supported by the “Plan Propio” and “Unidades de Excelencia” program from the UGR; by grants BIOD22_001 and BIOD22_002, funded by Consejería de Universidad, Investigación e Innovación and Gobierno de España and Unión Europea–NextGenerationEU; by the project BAGAMET (P20_00016) funded by the Counseling of Economy, Innovation, Science and Employment from the Government of Andalucía, including European Union ERDF funds; and by projects PID2020-117825GB-C21 and PID2020-117825GB-C22 funded by MCIN/AEI/10.13039/501100011033. Ignacio Peralta Maraver developed his research as part of the eWARM project, supported by the Marie Skłodowska- Curie postdoctoral fellowship 2022 (project number 101110111).
This paper was edited by Perran Cook and reviewed by two anonymous referees.
Aho, K., Derryberry, D., and Peterson, T.: Model selection for ecologists: the worldviews of AIC and BIC, Ecology, 95, 631–636, https://doi.org/10.1890/13-1452.1, 2014.
Alfadhel, I., Peralta-Maraver, I., Reche, I., Sánchez-Cañete, E. P., Aranda-Barranco, S., Rodríguez-Velasco, E., Kowalski, A. S., and Serrano-Ortiz, P.: Data from: Drought disrupts atmospheric carbon uptake in a Mediterranean saline lake, Dryad [data set], https://doi.org/10.5061/dryad.r4xgxd2nn, 2024.
Anderson, L. G., Falck, E., Jones, E. P., Jutterström, S., and Swift, J. H.: Enhanced uptake of atmospheric CO2 during freezing of seawater: A field study in Storfjorden, Svalbard, J. Geophys. Res.-Oceans, 109, C06004, https://doi.org/10.1029/2003JC002120, 2024.
Anderson, N. J. and Stedmon, C. A.: The effect of evapoconcentration on dissolved organic carbon concentration and quality in lakes of SW Greenland, Freshwater Biol., 52, 280–289, https://doi.org/10.1111/j.1365-2427.2006.01688.x, 2007.
Aubinet, M., Vesala, T., and Papale, D.: Eddy covariance: A practical guide to measurement and data analysis, London, UK, Springer, 2012.
Baldocchi, D. D.: Assessing the eddy covariance technique for evaluating carbon dioxide exchange rates of ecosystems: past, present and future, Glob. Change Biol., 9, 479–492, https://doi.org/10.1046/j.1365-2486.2003.00629.x, 2003.
Batanero, G. L., León-Palmero, E., Li, L., Green, A. J., Rendón-Martos, M., Suttle, C. A., and Reche, I.: Flamingos and drought as drivers of nutrients and microbial dynamics in a saline lake, Sci. Rep., 7, 12173, https://doi.org/10.1038/s41598-017-12462-9, 2017.
Batanero, G. L., Green, A. J., Amat, J. A., Vittecoq, M., Suttle, C. A., and Reche, I.: Patterns of microbial abundance and heterotrophic activity along nitrogen and salinity gradients in coastal wetlands, Aquat. Sci., 84, 22, https://doi.org/10.1007/s00027-022-00855-6, 2022.
Cardona, T., Shao, S., and Nixon, P. J: Enhancing photosynthesis in plants: the light reactions, Essays Biochem., 62, 85–94, https://doi.org/10.1042/EBC20170015, 2018.
Chen, W., Wolf, B., Zheng, X., Yao, Z., Butterbach-bahl, K., Brueggemann, N., Liu, C., Han, S., and Han, X.: Annual methane uptake by temperate semiarid steppes as regulated by stocking rates, aboveground plant biomass and topsoil air permeability, Glob. Change Biol., 17, 2803–2816, https://doi.org/10.1111/j.1365-2486.2011.02444.x , 2011.
Comín, F. A., Julia, R., Comin, M. P., and Plana, F.: Hydrogeochemistry of Lake Gallocanta (Aragón, NE Spain), in: Saline Lakes: Proceedings of the Fourth International Symposium on Athalassic (inland) Saline Lakes, held at Banyoles, Spain, May 1988, Springer, Netherlands, 51–66, 1990.
Deng, Y., Liu, Y., Dumont, M., and Conrad, R.: Salinity affects the composition of the aerobic methanotroph community in alkaline lake sediments from the Tibetan Plateau, Microb. Ecol., 73, 101–110, https://doi.org/10.1007/s00248-016-0879-5, 2017.
Duarte, C. M., Prairie, Y. T., Montes, C., Cole, J. J., Striegl, R., Melack, J., and Downing, J. A.: CO2 emissions from saline lakes: A global estimate of a surprisingly large flux, J. Geophys. Res.-Biogeo., 113, G04041, https://doi.org/10.1029/2007JG000637, 2008.
Eugster, H. P. and Hardie, L. A.: Saline lakes, in: Lakes: chemistry, geology, physics, New York, NY, Springer, New York, 237–293, 1978.
Falge, E., Baldocchi, D., Olson, R., Anthoni, P., Aubinet, M., Bernhofer, C., Burbaf, G., Ceulemansg, R., Clementh, R., Dolman, H., Granier, A., Gross, P., Grünwald, T., Hollinger, D., Jensen, N-O., Katul, G., Keronen, P., Kowalski, A., Lai, C. T., Law, B. E., Meyers, T., Moncrieff, J., Moors, E., Munger, J. W., Pilegaard, K., Rannik, Ü., Rebmann, C., Suyker, A., Tenhunen, J., Tu, K., Verma, S., Vesala, T., Wilson, K., and Wofsy, S.: Gap filling strategies for long term energy flux data sets, Agr. Forest Meteorol., 107, 71–77, https://doi.org/10.1016/S0168-1923(00)00235-5, 2001.
Feller, U.: Drought stress and carbon assimilation in a warming climate: Reversible and irreversible impacts, J. Plant Physiol., 203, 84–94, https://doi.org/10.1016/j.jplph.2016.04.002, 2016.
Garcia, C. M. and Niell, F. X.: Burrowing beetles of the genus Bledius (Staphylinidae) as agents of bioturbation in the emergent areas and shores of an athalassic inland lake (Fuente de Piedra, southern of Spain), Hydrobiologia, 215, 163–173, https://doi.org/10.1007/BF00014719, 1991.
García, C. M. and Niell, F. X.: Seasonal change in a saline temporary lake (Fuente de Piedra, southern Spain), Hydrobiologia, 267, 211–223, https://doi.org/10.1007/BF00018803, 1993.
García, C. M., García-Ruiz, R., Rendón, M., Niell, F. X., and Lucena, J.: Hydrological cycle and interannual variability of the aquatic community in a temporary saline lake (Fuente de Piedra, Southern Spain), Hydrobiologia, 345, 131–141, https://doi.org/10.1023/A:1002983723725, 1997.
Golub, M., Koupaei-Abyazani, N., Vesala, T., Mammarella, I., Ojala, A., Bohrer, G., Weyhenmeyer, G. A., Blanken, P. D., Eugster, W., Koebsch, F., Chen, J., Czajkowski, K., Deshmukh, C., Guérin, F., Heiskanen, J., Humphreys, E., Jonsson, A., Karlsson, J., Kling, g., Lee, X., Liu, H., Lohila, A., Lundin, E., Morin, T., Podgrajsek, E., Provenzale, M., Rutgersson, A., Sachs, T., Sahlée, E., Serça, D., Shao, C., Spence, C., Strachan, I. B., Xiao, W., and Desai, A. R.: Diel, seasonal, and inter-annual variation in carbon dioxide effluxes from lakes and reservoirs, Environ. Res. Lett., 18, 034046, https://doi.org/10.1088/1748-9326/acb834, 2023.
Hammer, U. T: Saline lake ecosystems of the world, Springer Science and Business Media, 1986.
Hassani, A., Azapagic, A., D'Odorico, P., Keshmiri, A., and Shokri, N.: Desiccation crisis of saline lakes: A new decision-support framework for building resilience to climate change, Sci. Total Environ., 703, 134718, https://doi.org/10.1016/j.scitotenv.2019.134718, 2020.
Heiri, O., Lotter, A. F., and Lemcke, G.: Loss on ignition as a method for estimating organic and carbonate content in sediments: reproducibility and comparability of results, J. Paleolimnol., 25, 101–110, https://doi.org/10.1023/A:1008119611481, 2001.
Herbert, E. R., Boon, P., Burgin, A. J., Neubauer, S. C., Franklin, R. B., Ardón, M., Hopfensperger, K. N., Lamers, L. P. M., and Gell, P.: A global perspective on wetland salinization: ecological consequences of a growing threat to freshwater wetlands, Ecosphere, 6, 1–43, https://doi.org/10.1890/ES14-00534.1, 2015.
Ho, A., Mo, Y., Lee, H. J., Sauheitl, L., Jia, Z., and Horn, M. A.: Effect of salt stress on aerobic methane oxidation and associated methanotrophs; a microcosm study of a natural community from a non-saline environment, Soil Biol. Biochem., 125, 210–214, https://doi.org/10.1016/j.soilbio.2018.07.013, 2018.
Khmelenina, V. N., Shchukin, V. N., Reshetnikov, A. S., Mustakhimov, I. I., Suzina, N. E., Eshinimaev, B. T., and Trotsenko, Y. A.: Structural and functional features of methanotrophs from hypersaline and alkaline lakes, Microbiology, 79, 472–482, https://doi.org/10.1134/S0026261710040090, 2010.
Kljun, N., Calanca, P., Rotach, M. W., and Schmid, H. P.: A simple parameterisation for flux footprint predictions, Bound.-Lay. Meteorol., 112, 503–523, https://doi.org/10.1023/B:BOUN.0000030653.71031.96, 2004.
Li, X. Y., Shi, F. Z., Ma, Y. J., Zhao, S. J., and Wei, J. Q.: Significant winter CO2 uptake by saline lakes on the Qinghai-Tibet Plateau, Glob. Change Biol., 28, 2041–2052, https://doi.org/10.1111/gcb.16054, 2022.
Li, Y., Zhang, C., Wang, N., Han, Q., Zhang, X., Liu, Y., Xu, L., and Ye, W.: Substantial inorganic carbon sink in closed drainage basins globally, Nat. Geosci., 10, 501–506, https://doi.org/10.1038/ngeo2972, 2017.
Liao, Y., Xiao, Q., Li, Y., Yang, C., Li, J., and Duan, H.: Salinity is an important factor in carbon emissions from an inland lake in arid region, Sci. Total Environ., 906, 167721, https://doi.org/10.1016/j.scitotenv.2023.167721, 2024.
Martínez-García, A., Peralta-Maraver, I., Rodríguez-Velasco, E., Batanero, G. L., García-Alguacil, M., Picazo, F., Calvo, J., Morales-Baquero, R., Rueda, F. J., and Reche, R.: Particulate organic carbon sedimentation triggers lagged methane emissions in a eutrophic reservoir, Limnol. Oceanogr. Lett., 9, 247–257, https://doi.org/10.1002/lol2.10379, 2024.
Mayen, J., Polsenaere, P., Lamaud, É., Arnaud, M., Kostyrka, P., Bonnefond, J.-M., Geairon, P., Gernigon, J., Chassagne, R., Lacoue-Labarthe, T., Regaudie de Gioux, A., and Souchu, P.: Atmospheric CO2 exchanges measured by eddy covariance over a temperate salt marsh and influence of environmental controlling factors, Biogeosciences, 21, 993–1016, https://doi.org/10.5194/bg-21-993-2024, 2024.
Messager, M. L., Lehner, B., Grill, G., Nedeva, I., and Schmitt, O.: Estimating the volume and age of water stored in global lakes using a geo-statistical approach, Nat. Commun., 7, 13603, https://doi.org/10.1038/ncomms13603, 2016.
Moncrieff, J., Clement, R., Finnigan, J., and Meyers, T: Averaging, Detrending, and Filtering of Eddy Covariance Time Series, in: Handbook of Micrometeorology, 7–31, https://doi.org/10.1007/1-4020-2265-4_2, 2004.
Moncrieff, J. B., Massheder, J. M., de Bruin, H., Elbers, J., Friborg, T., Heusinkveld, B., Kabat, P., Scott, S., Soegaard, H., and Verhoef, A.: A system to measure surface fluxes of momentum, sensible heat, water vapour and carbon dioxide, J. Hydrol., 188–189, 589–611, https://doi.org/10.1016/S0022-1694(96)03194-0, 1997.
Murguia-Flores, F., Ganesan, A. L., Arndt, S., and Hornibrook, E. R.: Global uptake of atmospheric methane by soil from 1900 to 2100, Global Biogeochem. Cy., 35, e2020GB006774, https://doi.org/10.1029/2020GB006774, 2021.
Osudar, R., Klings, K. W., Wagner, D., and Bussmann, I.: Effect of salinity on microbial methane oxidation in freshwater and marine environments, Aquat. Microb. Ecol., 80, 181–192, https://doi.org/10.3354/ame01845, 2017.
Pastorello, G., Trotta, C., Canfora, E., et al.: The FLUXNET2015 dataset and the ONEFlux processing pipeline for eddy covariance data, Sci. Data, 7, 225, https://doi.org/10.1038/s41597-020-0534-3, 2020.
Perez-Quezada, J. F., Urrutia, P., Olivares-Rojas, J., Meijide, A., Sánchez-Cañete, E. P., and Gaxiola, A.: Long term effects of fire on the soil greenhouse gas balance of an old-growth temperate rainforest, Sci. Total Environ., 755, 142442, https://doi.org/10.1016/j.scitotenv.2020.142442, 2021.
Perkins-Kirkpatrick, S. E. and Lewis, S. C.: Increasing trends in regional heatwaves, Nat. Commun., 11, 3357, https://doi.org/10.1038/s41467-020-16970-7, 2020.
Rafalska, A., Walkiewicz, A., Osborne, B., Klumpp, K., and Bieganowski, A.: Variation in methane uptake by grassland soils in the context of climate change–A review of effects and mechanisms, Sci. Total Environ., 871, 162127, https://doi.org/10.1016/j.scitotenv.2023.162127, 2023.
Raymond, P. A., Hartmann, J., Lauerwald, R., Sobek, S., McDonald, C., Hoover, M., Butman, D., Strieg, R., Mayorga, E, Humborg, C., Kortelainen, P., Durr, H., Meybeck, M., Ciais, P., and Guth, P.: Global carbon dioxide emissions from inland waters, Nature, 503, 355–359, https://doi.org/10.1038/nature12760, 2013.
Rodríguez- Rodríguez, M., Benavente, J., and Moral, F.: High density ground-water flow, major-ion chemistry and field experiments in a closed basin: Fuente de Piedra Playa Lake (Spain), American J. Environ. Sciences, 1, 164–171, 2006.
Rysgaard, S., Glud, R. N., Lennert, K., Cooper, M., Halden, N., Leakey, R. J. G., Hawthorne, F. C., and Barber, D.: Ikaite crystals in melting sea ice – implications for pCO2 and pH levels in Arctic surface waters, The Cryosphere, 6, 901–908, https://doi.org/10.5194/tc-6-901-2012, 2012.
Rysgaard, S., Søgaard, D. H., Cooper, M., Pućko, M., Lennert, K., Papakyriakou, T. N., Wang, F., Geilfus, N. X., Glud, R. N., Ehn, J., McGinnis, D. F., Attard, K., Sievers, J., Deming, J. W., and Barber, D.: Ikaite crystal distribution in winter sea ice and implications for CO2 system dynamics, The Cryosphere, 7, 707–718, https://doi.org/10.5194/tc-7-707-2013, 2013.
Saccò, M., White, N. E., Harrod, C., Salazar, G., Aguilar, P., Cubillos, C. F., Meredith, K., Baxter, B. K., Oren, A., Anufriieva, E., Shadrin, N., Marambio- Alfaro, Y., Bravo-Naranjo, V., and Allentoft, M. E.: Salt to conserve: A review on the ecology and preservation of hypersaline ecosystems, Biol. Rev., 96, 2828–2850, https://doi.org/10.1111/brv.12780, 2021.
Sabbatini, S., Mammarella, I., Arriga, N., Fratini, G., Graf, A., Hörtnagl, L., Ibrom, A., Longdoz, B., Mauder, M., Merbold, L., Metzger, S., Montagnani, L., Pitacco, A., Rebmann, C., Sedlák, P., Šigut, L., Vitale, D., and Papale, D.: Eddy covariance raw data processing for CO2 and energy fluxes calculation at ICOS ecosystem stations, Int. Agrophys., 32, 495–515, https://doi.org/10.1515/intag-2017-0043, 2018.
Sharkey, T. D.: Effects of moderate heat stress on photosynthesis: importance of thylakoid reactions, rubisco deactivation, reactive oxygen species, and thermotolerance provided by isoprene, Plant Cell Environ., 28, 269–277, https://doi.org/10.1111/j.1365-3040.2005.01324.x, 2005.
Smith, T. P., Thomas, T. J., García-Carreras, B., Sal, S., Yvon-Durocher, G., Bell, T., and Pawar, S.: Community-level respiration of prokaryotic microbes may rise with global warming, Nat. commUN., 10, 5124, https://doi.org/10.1038/s41467-019-13109-1, 2019.
Song, K. S., Zang, S. Y., Zhao, Y., Li, L., Du, J., Zhang, N. N., Wang, X. D., Shao, T. T., Guan, Y., and Liu, L.: Spatiotemporal characterization of dissolved carbon for inland waters in semi-humid/semi-arid region, China, Hydrol. Earth Syst. Sci., 17, 4269–4281, https://doi.org/10.5194/hess-17-4269-2013, 2013.
Soued, C., Bogard, M. J., Finlay, K., Bortolotti, L. E., Leavitt, P. R, Badiou, P., Knox, S.H., Jensen, S., Mueller, P., Lee, S. C., Ng, D., Wissel, B., Chan, C. N., Page, B., and Kowal, P.: Salinity causes widespread restriction of methane emissions from small inland waters, Nat. CommUN., 15, 717, https://doi.org/10.1038/s41467-024-44715-3, 2024.
Stoy, P. C., Mauder, M., Foken, T., Marcolla, B., Boegh, E., Ibrom, A., Arain, M. A., Arneth, A., Aurela, M., Bernhofer, C., Cescatti, A., Dellwik, E., Duce, P., Gianelle, D., van Gorsel, E., Kiely, G., Knohl, A., Margolis, H., Mccaughey, H., Merbold, L., Montagnani, L., Papale, D., Reichstein, M., Saunders, M., Serrano-Ortiz, P., Sottocornola, M., Spano, D., Vaccari, F., and Varlagin, A.: A data-driven analysis of energy balance closure across FLUXNET research sites: The role of landscape scale heterogeneity, Agr. ForEST Meteorol., 171–172, 137–152, https://doi.org/10.1016/j.agrformet.2012.11.004, 2013.
Trenberth, K. E.: Changes in precipitation with climate change, Clim. Res., 47, 123–138, https://doi.org/10.3354/cr00953, 2011.
Tweed, S., Grace, M., Leblanc, M., Cartwright, I., and Smithyman, D.: The individual response of saline lakes to a severe drought, Sci. Total Environ., 409, 3919–3933, https://doi.org/10.1016/j.scitotenv.2011.06.023, 2011.
Vickers, D. and Mahrt, L.: Quality control and flux sampling problems for tower and aircraft data, J. Atmos. Ocean. Tech., 14, 512–526, https://doi.org/10.1175/1520-0426(1997)014<0512:QCAFSP>2.0.CO;2, 1997.
Wang, J., Song, C., Reager, J. T., Yao, F., Famiglietti, J. S., Sheng, Y., MacDonald, G. M., Brun, F., Schmied, H. M., Marston, A. R., and Wada, Y.: Recent global decline in endorheic basin water storages, Nat. Geosci., 11, 926–932, https://doi.org/10.1038/s41561-018-0265-7, 2018.
Webb, E. K., Pearman, G. I., and Leuning, R. Correction of flux measurements for density effects due to heat and water vapour transfer, Q. J. Roy. Meteor. Soc., 106, 85–100, https://doi.org/10.1002/qj.49710644707, 1980.
Wen, Z., Song, K., Shang, Y., Fang, C., Li, L., Lv, L., Lv, X., and Chen, L.: Carbon dioxide emissions from lakes and reservoirs of China: a regional estimate based on the calculated pCO2, Atmos. Environ., 170, 71–81, https://doi.org/10.1016/j.atmosenv.2017.09.032, 2017.
Wilczak, J. M., Oncley, S. P., and Stage, S. A.: Sonic anemometer tilt correction algorithms, Bound.-Lay. Meteorol., 99, 127–150, https://doi.org/10.1023/A:1018966204465, 2001.
Williams, W. D.: Conservation of salt lakes, Hydrobiologia, 267, 291–306, https://doi.org/10.1007/BF00018809, 1993.
Williams, W. D.: Environmental threats to salt lakes and the likely status of inland saline ecosystems in 2025, Environ. Conserv., 29, 154–167, https://doi.org/10.1017/S0376892902000103, 2002.
Wilson, K., Goldstein, A., Falge, E., Aubinet, M., Baldocchi, D., Berbigier, P., Bernhofer, C., Ceulemans, R., Dolman, H., Field, C., Grelle, A., Ibrom, A., Law, B.E., Kowalski, A., Meyers, T., Moncrieff, J., Monson, R., Oechel, W., Tenhunen, J., Valentini, R., and Verma, S.: Energy balance closure at FLUXNET sites, Agr. Forest Meteorol., 113, 223–243, https://doi.org/10.1016/S0168-1923(02)00109-0, 2002.
Wu, Y., Wang, N., Zhao, L., Zhang, Z., Chen, L. I., Lu, Y., Lü, X., and Chang, J.: Hydrochemical characteristics and recharge sources of Lake Nuoertu in the Badain Jaran Desert, Sci. Bull., 59, 886–895, https://doi.org/10.1007/s11434-013-0102-8, 2014.
Wurtsbaugh, W. A., Miller, C., Null, S. E., DeRose, R. J., Wilcock, P., Hahnenberger, M., Howe, F., and Moore, J.: Decline of the world's saline lakes. Nat. Geosci., 10, 816–821, https://doi.org/10.1038/ngeo3052, 2017.
Yan, L. and Zheng, M.: Influence of climate change on saline lakes of the Tibet Plateau, 1973–2010, Geomorphology, 246, 68–78, https://doi.org/10.1016/j.geomorph.2015.06.006, 2015.
Yang, P., Wang, N. A., Zhao, L., Zhang, D., Zhao, H., Niu, Z., and Fan, G.: Variation characteristics and influencing mechanism of CO2 flux from lakes in the Badain Jaran Desert: A case study of Yindeer Lake, Ecol. Ind., 127, 107731, https://doi.org/10.1016/j.ecolind.2021.107731, 2021.
Yvon-Durocher, G., Jones, J. I., Trimmer, M., Woodward, G., and Montoya, J. M.: Warming alters the metabolic balance of ecosystems, Phil. T. R. Soc. B, 365, 2117–2126, https://doi.org/10.1098/rstb.2010.0038, 2010.
Yvon-Durocher, G., Caffrey, J. M., Cescatti, A., Dossena, M., Giorgio, P. D., Gasol, J. M., Montoya, J. M., Pumpanen, J., Staehr, P. A., Trimmer, M., Woodwards, G., and Allen, A. P.: Reconciling the temperature dependence of respiration across timescales and ecosystem types, Nature, 487, 472–476, https://doi.org/10.1038/nature11205, 2012.