the Creative Commons Attribution 4.0 License.
the Creative Commons Attribution 4.0 License.
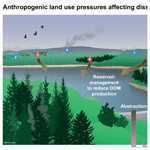
Reviews and syntheses: Understanding the impacts of peatland catchment management on dissolved organic matter concentration and treatability
Chris Evans
Bryan Spears
Amy Pickard
Pippa J. Chapman
Heidrun Feuchtmayr
Fraser Leith
Susan Waldron
Don Monteith
In the UK, most large reservoirs constructed for public water supply are in upland areas. Many are situated in catchments characterised by organic-rich soils, including peatlands. Although these soils naturally leach large amounts of dissolved organic matter (DOM) to water, the widespread degradation of upland peat in the UK is believed to have exacerbated rates of DOM loss. High and rising DOM concentrations in these regions raise treatment challenges for the water industry.
In the UK, water companies are increasingly considering whether upland-catchment peat restoration measures can slow down or even reverse rising source water DOM concentrations and, thus, reduce the need for more costly and complex engineering solutions. There remains considerable uncertainty around the effectiveness of such measures, and a comprehensive overview of the research in this area remains lacking. Here, we review the peer-reviewed evidence of the effectiveness of four catchment management options in controlling DOM release from peat soils: ditch blocking, revegetation, reducing forest cover and cessation of managed burning.
Results of plot-scale investigations into the effects of ditch blocking on DOM leaching are currently largely equivocal, while there is a paucity of information regarding impacts at spatial scales of more direct relevance to water managers. There is some, although limited, evidence that the terrestrial vegetation type may influence DOM concentrations and treatability. The presence of plantation forestry on peat soils is generally associated with elevated DOM concentrations, although reducing forest cover appears to have little short-term benefit, and associated disturbance may even increase concentrations further.
Catchment management measures have rarely been monitored with downstream water quality as the focus. To mitigate the uncertainty surrounding restoration effects on DOM, measures should be undertaken on a site-specific basis, where the scale, effect size and duration of the intervention are considered in relation to subsequent biogeochemical processing that occurs in the reservoir, the treatment capacity of the water treatment works and future projected DOM trends.
- Article
(2597 KB) - Full-text XML
- BibTeX
- EndNote
Dissolved organic matter (DOM) is ubiquitous across surface waters, with particularly high concentrations occurring in waters draining catchments with peat soils (e.g. Williamson et al., 2021). DOM originates from the decomposition of plant material and soil as well as from plant and algal production and microbial transformation within the water column (Tranvik et al., 2009). DOM in rivers and lakes is subject to both biotic and abiotic processing, and this processing changes both concentrations and the chemical structure (e.g. Algesten et al., 2004; Tranvik et al., 2009); thus, DOM concentrations at the point of abstraction from reservoirs represent the sum of these removal and generation processes (Fig. 1).
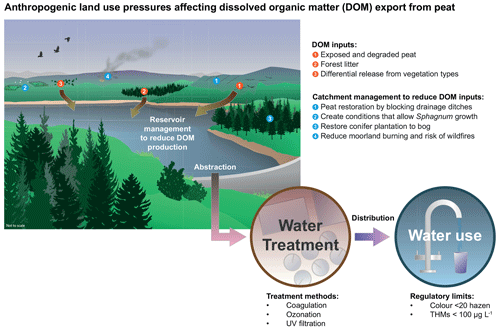
Figure 1Schematic showing the anthropogenic pressures on peatland catchments and the potential peatland management processes covered in this review.
Peatlands release particularly high amounts of organic matter as dissolved organic matter (DOM) into drainage waters, and DOM concentrations have been rising since the 1980s (e.g. Naden and Mcdonald, 1989; Robson and Neal, 1996; Harriman et al., 2001; Worrall et al., 2004; Evans et al., 2005). Mean DOM concentrations in UK Upland Waters Monitoring Network (UWMN) surface waters, most of which are dominated by organic-rich soils, have approximately doubled over the last 3 decades (Fig. 2). At the sub-catchment scale, Chapman et al. (2010) found that water colour increased by between 22 % and 155 % over a 20-year period between 1986 and 2006. This phenomenon has now been observed across much of industrialised North America and northern Europe, and it appears to result largely from a long-term increase in the solubility of terrestrial organic matter as soils recover from the effects of acid rain (Monteith et al., 2007; de Wit et al., 2021; Monteith et al., 2023). One consequence of these changes is that water treatment works in some regions are having to adjust to much higher source water DOM concentrations than they were originally designed to cope with, as most were built at a time of much higher atmospheric deposition and, hence, lower DOM. Atmospheric deposition of pollutants across the UK uplands has now declined to a very low level, and it is expected that changes in DOM export will be increasingly affected by other factors in future, including temperature, changes in precipitation seasonality and intensity, and marine ion deposition (Monteith et al., 2023). Rising levels of DOM in waters draining many of these peatland catchments pose considerable water treatment challenges, with respect to increasing treatment costs and risks of regulatory failure (see Fig. 1). It has been proposed that peatland restoration measures might help slow or even reverse these DOM trends, along with other important benefits including increased terrestrial carbon storage, water retention and improvements in upland biodiversity (e.g. Glenk and Martin-Ortega, 2018).
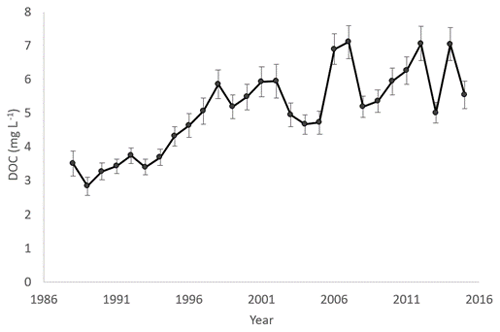
Figure 2Mean (± standard error) annual dissolved organic carbon (DOC) concentrations from the 23 UK Upland Waters Monitoring Network sites. These sites are predominately situated in the north and west of the UK; see https://www.uwmn.uk, last access: 10 October 2022 for more details.
Although consumption of DOM in drinking water is not directly harmful to people, coloured water reduces customer satisfaction (Ritson et al., 2014) and can be indicative of further problems. Indirectly, elevated DOM concentrations have implications for human health due to their potential influence on treatment processes and the production of carcinogenic disinfectant by-products (DBPs) such as trihalomethanes (THMs) during chemical disinfection, which are regulated by the Drinking Water Inspectorate due to their potential carcinogenic properties (Ding and Chu, 2017). DOM also may hamper the efficacy of chlorine as a disinfectant while simultaneously acting as a substrate for bacterial regrowth (Prest et al., 2016), thus increasing the risk of regulatory failure from bacterial contamination and the subsequent loss of customer trust.
The composition of DOM can have a large influence on the performance of the water treatment processes and the formation of DBPs upon chlorination (Matilainen et al., 2010). DOM in water draining peatland areas tends to be predominantly hydrophobic, relatively photoreactive and biologically recalcitrant (Anderson et al., 2019), and is relatively easily removed by conventional coagulation and filtration during drinking water treatment due to the presence of charged functional groups (Matilainen et al., 2010). Hydrophilic DOM, on the other hand, is mostly produced within the waterbodies by phytoplankton (Imai et al., 2002) and is biologically labile but less easily degraded by sunlight (Berggren and Del Giorgio, 2015; Berggren et al., 2018). The relative balance of hydrophobic to hydrophilic DOM in water is referred to as hydrophobicity and is conventionally assessed in the water treatment system using specific UV absorbance measurements at 254 nm (SUVA254), i.e. absorbance at 254 nm per unit dissolved organic carbon concentration (Weishaar et al., 2003). Values greater than 4 L mg−1 m−1 indicate hydrophobic dominance, whereas values less than 2 L mg−1 m−1 indicate that the DOM is primarily hydrophilic and will not be effectively removed using conventional coagulation and filtration alone (Matilainen et al., 2010).
Higher concentrations of DOM in raw water necessitate a greater amount of treatment to provide potable water to customers (Monteith et al., 2021). This may include larger coagulant dosages, shorter filter run times, and longer and more frequent cleaning of filtration units, and it may result in higher energy costs, higher sludge removal costs, and an increase in direct and indirect (energy-related) greenhouse gas (GHG) emissions from the treatment process (Jones et al., 2016). Major additional costs are incurred when capital investment is needed to upgrade treatment infrastructure designed for lower concentration ranges experienced in the past (Monteith et al., 2021).
Peatland restoration (physical interventions to return them to a more natural state, i.e. high water table and active peat-forming vegetation) has been suggested as a catchment-scale method for reducing DOM concentrations in water draining peatlands (IUCN Peatland Programme 2022). The primary restoration methods undertaken to date in the UK uplands are as follows: blocking of peatland drainage to raise the water table, revegetation of bare peat with peatland species, removal of plantation forestry to allow peatland species to recolonise and water tables to rise, and cessation of managed burning to encourage the growth of peatland plant species (Fig. 1) (IUCN Peatland Programme 2022). It is important, therefore, for water industry decision-makers to understand the extent to which peatland restoration could make a positive contribution to reducing DOM concentrations of raw water and, thus, relieve stresses on the treatment system and potentially remove the need for major additional capital investment in treatment plants. In this study, we review the available peer-reviewed literature relating to the impacts of peatland restoration on DOM concentrations and the treatability of raw drinking water. Finally, we consider the possible influence of catchment land use on in-reservoir DOM cycling and the impact that this may have had on drinking water treatability. We focus on the UK as a well-studied area in which peatlands make an important contribution to drinking water supplies (Xu et al., 2018) and in which rising DOM concentrations are having a significant impact on water treatment processes and costs, but the conclusions of the work are likely be relevant to other areas with peat-derived water supplies.
To answer the question “Will peatland restoration reduce DOM concentrations in raw water?”, we explored the evidence within the peer-reviewed scientific literature with respect to catchment management approaches within peatland-dominated drinking water catchments influencing DOM concentrations in the soils and waters of peatland catchments. This was achieved by applying a standard set of Boolean search terms within Web of Science and Google Scholar. The terms were as follows: (“dissolved organic matter” OR “dissolved organic carbon” OR “DOM” OR “DOC” OR “colour”) AND (“peatland” OR “bog” OR “fen” OR “moor”) AND (“ditch blocking” OR “forest” OR “plantation” OR “managed burning”). Initial results, including titles and abstracts, were rapidly reviewed to determine whether the information within the papers was relevant, and relevant papers were then read in full and included in the review. Given the geographic focus of the project, we not only prioritised papers from the UK and Ireland where available but also drew on evidence from other temperate peatland regions where required. From the original searches, 272 papers were considered relevant enough for further reading and 104 were included in the review.
3.1 Ditch blocking
Extensive areas of upland peatlands across the UK uplands were drained in the mid-20th century in an attempt to increase agricultural productivity. Peatland drainage reduces water tables (Holden et al., 2011), resulting in a loss of peat-forming plant species. The consequent drying and cracking of peat surfaces exposes previously permanently saturated organic matter to oxidative processes, making them more vulnerable to erosion and dissolution into DOM (e.g. Clark et al., 2009). Extensive efforts have been made by the water industry and organisations concerned with peatland restoration to block ditches in an attempt to restore the hydrological, biogeochemical and ecological functions of these landscapes (Figs. 1, 4; IUCN Peatland Programme, 2023).
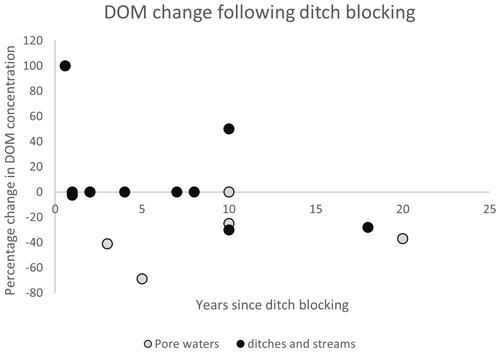
Figure 3Percentage change in the DOM concentration following ditch blocking. Grey circles show the DOM percentage change in peatland pore waters and black circles show the DOM percentage change in ditches and streams.
Search results of the scientific literature showed that the impact of ditch blocking on DOM concentrations had been assessed in pore waters, in ditches and in streams at the sites being restored. Of the five plot-scale studies of pore water identified during this review, four (Table 1) reported significant changes in DOM concentrations. The studies investigated effects between 5 and 20 years following ditch blocking and reported a cross-study mean 34 % reduction in the DOC concentration (range of 0 % to 69 %) (Wallage et al., 2006; Holl et al., 2009; Haapalehto et al., 2014; Strack et al., 2015; Menberu et al., 2017). Therefore, while suggesting a general tendency for ditch blocking to reduce pore water DOM concentrations, these studies do not necessarily imply that the effects will be translated through to surface waters and, ultimately, to the point of abstraction.
Table 1Summary of the impacts of drainage ditch blocking on DOM concentrations and fluxes from peatlands, reported in increasing time since ditch blocking. BA denotes before/after and CI represents control/intervention. Reference to “Chronosequence” in the “Experimental design” column refers to a sampling strategy whereby sites that had had interventions at different times were used as a proxy for control sites, while “Survey” refers to a short-term one-off sampling of multiple locations.
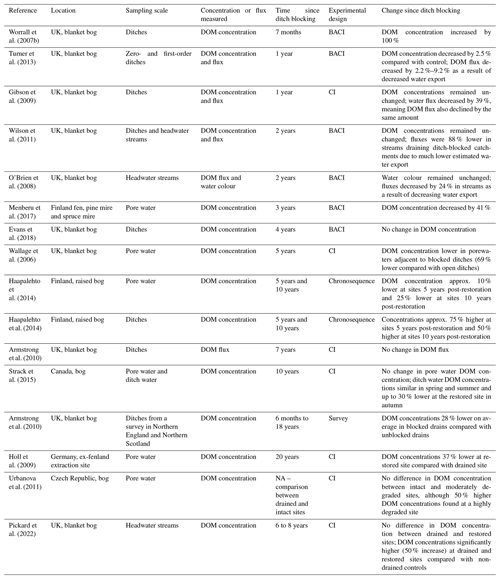
Changes observed in DOM concentrations at a drainage ditch scale are more variable than those for pore waters (Fig. 3, Table 1). The 11 studies reviewed showed a mean 8 % increase in DOM concentrations following ditch blocking, although this figure is skewed by the large increases reported by Worrall et al. (2007b) and Haapalehto et al. (2014) (100 % increase immediately following ditch blocking and 50 %–75 % increases after 10 and 5 years, respectively); the median change is zero. Importantly, no significant change in DOM concentration was reported in over half of these studies (O'Brien et al., 2008; Gibson et al., 2009; Armstrong et al., 2010; Wilson et al., 2011; Evans et al., 2018; Pickard et al., 2022). Likewise, a recent study found no reduction in DOM concentrations at a restored site compared to a ditched site 6 years after ditch blocking, while both the drained and restored site DOM concentrations remained elevated compared with the non-drained control (Pickard et al., 2022). Differences between studies with respect to the apparent effect size may in part be related to the experimental design, including whether the work included a simultaneous control, and the time period over which post-restoration monitoring was carried out.
Studies of DOM flux changes following ditch blocking report a mean 24 % reduction (range of 0 % to 88 %) in DOM flux, primarily attributed to decreased water fluxes from the restoration site. However, the measurement and reporting of water fluxes (and, hence, DOM fluxes) at a site or at the catchment scale require careful consideration of the potential for dominant water flow pathways to be altered following ditch blocking. For example, Holden et al. (2017) showed that the damming of drainage ditches in North Wales reduced discharge along the original ditch lines but that most, or all, of the displaced flow instead left the peatland via overland flow or near-surface throughflow. Subsequent reporting from the same experiment demonstrated that DOM concentrations in water displaced along these surficial pathways were approximately the same as those in water travelling along the ditches, with the result that ditch blocking was not found to have any clear effect on either DOM concentrations or fluxes at the catchment scale (Evans et al., 2018).
We identified nine studies that have assessed the potential impact of ditch blocking on DOM treatability and, hence, the ease of treatability within a conventional water treatment works. The majority of studies at UK and continental European ditch-blocking locations, along with results from their experimental work, showed little effect of ditch blocking on DOM treatability as measured by commonly reported metrics such as SUVA254, E2 : E3 ratios (ratio of light absorbance at 250 and 365 nm) and E4 : E6 ratios (ratio of light absorbance at 465 and 665 nm) (Glatzel et al., 2003; Strack et al., 2015; Gough et al., 2016; Lundin et al., 2017; Peacock et al., 2018). While none of the studies included direct measures of DOM hydrophobic and hydrophilic fractions, one measured THM formation potential and found no change between water samples taken from drained and rewetted blanket bog mesocosms (Gough et al., 2016), suggesting that ditch blocking may not reduce THM formation in the short term following water treatment.
More broadly, therefore, while the evidence suggests that ditch blocking may reduce DOM concentrations within pore waters (Fig. 3, Table 3), there is no published evidence that such activities have successfully influenced DOM concentrations in runoff at the catchment scale, and thus at a level of potential relevance for raw-water supply to treatment works. It is important to note, however, that catchment-scale studies are hugely challenging logistically and financially to design and maintain.
3.2 Revegetation of bare peat
Exposure of bare peat following anthropogenic disturbance has been an extensive problem in a number of UK peatland regions, most notably in the Peak District of Northern England (Pilkington et al., 2015). The subsequent erosion of the peat has caused significant problems for the water industry because of the high particulate loads from the catchment to the downstream reservoirs. There have been significant efforts in recent years to revegetate some of the most degraded upland peatland areas in order to stabilise these systems (Pilkington et al., 2015).
Published research on the impacts of the revegetation of peatland areas on DOM is limited, but Qassim et al. (2014) found that pore water DOM concentrations were higher at revegetated sites compared with bare peat areas and vegetated controls over a 5-year period. The initial revegetation mix in this work was a nurse crop of Agrostis sp., Deschampsia flexuosa and Festuca sp. applied in combination with additions of lime and fertiliser to ensure grass growth. Heather brash was also applied to stabilise the peat surface and provide a seed source of peatland species. The use of lime is likely to have increased DOM solubility through a reduction in the acidity of the peat (Evans et al., 2012), and the re-establishment of vegetation may have increased the production of “new” DOM via root leachate and fresh litter decomposition. Particulate losses from peatland systems decreased following stabilisation of the peat surface through revegetation irrespective of gully-blocking activities (Pilkington et al., 2015), as overland flow velocities are lower on vegetated peat than on bare peat (Holden et al., 2008). However, the same study (Pilkington et al., 2015), and more recent assessments of the effects of revegetation on DOM concentrations (Stimson et al., 2017; Alderson et al., 2019), found no long-term changes in DOM concentrations following revegetation at the headwater catchment scale.
Radiocarbon (14C) measurements of DOM in UK upland waters indicate that the principal source of DOM in waters draining relatively undisturbed soils is recent primary production, probably formed within the last few years (Evans et al., 2014). It follows, therefore, that plant productivity and plant tissue composition and degradability, which depend both on ambient environmental conditions and species composition, may be important factors, both for DOM concentrations and the treatability of the DOM produced. In a laboratory-based extraction experiment, DOM leached from Sphagnum was more easily removed by a conventional coagulation process and decomposed more rapidly than DOM leached from Molinia caerulea or Calluna vulgaris litter. In addition, M. caerulea and C. vulgaris litter released more DOM per unit dry weight than Sphagnum litter (Ritson et al., 2016). At the field scale, published results are less clear cut: one study found that DOM concentrations in pore waters were higher in areas of blanket bog dominated by C. vulgaris compared with areas dominated by sedges or Sphagnum species (Armstrong et al., 2012). In contrast, Parry et al. (2015) found no correlation between the dominant vegetation type (differentiated into ericoids, grasses, sedges and bare peat) and stream water DOM concentrations in headwater catchments. This may reflect the greater biotic (as well as soil) heterogeneity of peatland environments at the catchment scale in comparison with single-species plot experiments.
The evidence available to date suggests that, while revegetation of peatland sites has stabilised bare peat surfaces (e.g. Pilkington et al., 2015) and is likely to have reduced particulate organic matter loss, revegetation may not have significantly changed DOM export from peat headwater catchments. Laboratory-based work has shown that the species present could impact DOM treatability, with Sphagnum-derived DOM being more easily treatable than M. caerulea or C. vulgaris litter (Ritson et al., 2016). This suggests that catchment management via revegetation should aim to achieve a high cover of Sphagnum species compared with vascular plants to maximise DOM treatability (Table 3).
3.3 Plantation forestry/deforestation
It has long been recognised that plantation forestry activities can have detrimental impacts on reservoir water quality and treatability. For example, in 1984 it was shown that drainage and deforestation resulted in large sedimentation issues at Crai Reservoir in South Wales (Stretton, 1984, cited in Hudson et al., 1997), and large pulses of nutrients (N and P) to upland streams were observed after forest felling (Neal, 2002). This review covers the impact of ground preparation and forest planting, in situ forest growth, and forest removal (including forest-to-bog restoration) on peat with respect to the DOM concentration and quality. Note that UK blanket bogs do not naturally support trees and that virtually all forestry activities on peat in the UK involve drainage and planting with non-native conifers.
To reduce the impacts of forest operations on sediment and nutrient loss and consequent raw-water quality in the UK, the Forest and Water Guidelines now state that no more than 20 % of a drinking water catchment should be felled in any 3-year period (Forestry Commission, 2017). In addition to this, although primarily to conserve soil carbon stocks rather than to improve water quality, the 2000 Forestry Commission Forest and Peatland Habitats guidance note (Patterson and Anderson, 2000) states that approval will no longer be given for forestry planting or regeneration on active raised bogs or inactive raised bogs that could be restored to active bogs nor for areas of active blanket bog greater than 25 ha area and peat with a depth of > 45–50 cm.
A recent review for Yorkshire Water (Chapman et al., 2017) noted that conventional conifer site preparation on Peat, Peaty Gley and Peaty Podzol soils would be expected to increase DOM concentrations. This would be largely due to the implemented drainage increasing the depth of the water table and, consequently, the production of DOM via increased aeration of the peat surface (Clark et al., 2009). In the absence of extensive primary data on the effects of forest establishment from the UK, research from Fennoscandia supports this conclusion; Jandl et al. (2007), in their review of studies on the effect of forest management on soil carbon sequestration, highlighted two Finnish studies in which DOM concentrations were found to increase following drainage ditch installation but returned to pre-drainage levels later in the forest cycle, while Schelker et al. (2012) observed increased colour at sites being prepared for forestry in northern Sweden. Furthermore, Rask et al. (1998) reported an increase in colour in streams draining peat-dominated catchments following afforestation in Finland, while afforestation has also been linked to long-term increases in water colour in Sweden (Skerlep et al., 2019). However, it should be noted that forest management in Fennoscandia often involves relatively limited levels of disturbance (e.g. ditching to accelerate growth of existing mixed native tree species), whereas it typically involves ditching, ploughing and active planting with non-native monocultures in the UK.
At a regional to national scale in the UK, recent work has suggested that the presence of plantation forestry on peat soils is associated with higher DOM concentrations in streams and rivers compared with peat soils supporting semi-natural vegetation (Williamson et al., 2021). The presence of conifers on peat soils in a UK and Irish context is associated with higher pore water DOM concentrations across the four studies covered in this review (Table 2), with a mean difference of approximately 130 %. The exception to this pattern was found in spruce plantations in North Wales where DOM concentrations in pore waters were 19 % lower than in adjacent blanket bog, although this pattern was not seen in pore water samples from under other plantation species (Gough et al., 2012). We found only one study (Gaffney et al., 2018) that compared DOM concentrations in drainage ditches between forested and blanket bog areas, with DOM concentrations approximately 100 % higher in the former. The presence of forestry on peat had less clear-cut impacts on stream water DOM concentrations: two out of three studies reported no significant difference between streams draining catchments with forestry and intact blanket bogs (Shah et al., 2021; Flynn et al., 2022), whereas the third study showed DOM concentrations approximately 25 % higher in a stream draining a forested catchment compared with a blanket bog catchment (Cummins and Farrell, 2003).
Table 2UK studies reporting DOM concentration monitoring of forestry activities on peat. Percentage differences preceded by “∼” denote cases in which concentrations were not explicitly listed in the text, figures and tables, or supplementary information; thus, in the aforementioned cases, this information was estimated from graphs.
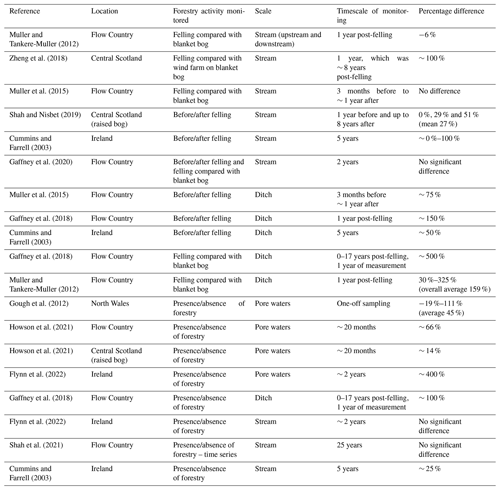
Clear felling tends to cause an increase in DOM, although the effects are not universal across studies and locations. Three of five studies of stream water DOM concentrations reported increases following felling (Cummins and Farrell, 2003; Zheng et al., 2018; Shah and Nisbet, 2019), with a mean increase of approximately 43 %, although the two studies in the Flow Country showed no change (Muller et al., 2015) and 6 % lower concentrations compared with the control site (Muller and Tankere-Muller, 2012), which was attributed to the success of buffer strips between the plantation and the monitored stream. The mean increase in DOM concentrations in ditches was nearly 200 % (ranging from a 50 % increase to a 500 % increase; see Table 2) (Cummins and Farrell, 2003; Muller and Tankere-Muller, 2012; Muller et al., 2015; Gaffney et al., 2018). Most studies measuring DOM concentrations from forestry on peat were relatively short term with respect to time frame, lasting 2 years or less. Only two studies monitored DOM concentrations for 5 years or longer.
Table 3Summary of the published impacts of catchment management activities on DOM concentrations and treatability, focussing on those studies relevant in a UK and Irish context. Numbers in parentheses refer to the number of studies showing that effect in each case, while the overall impacts on DOM concentration and treatability for water treatment are shown as +/=/− (positive/neutral/negative) for concentrations and treatability, respectively.
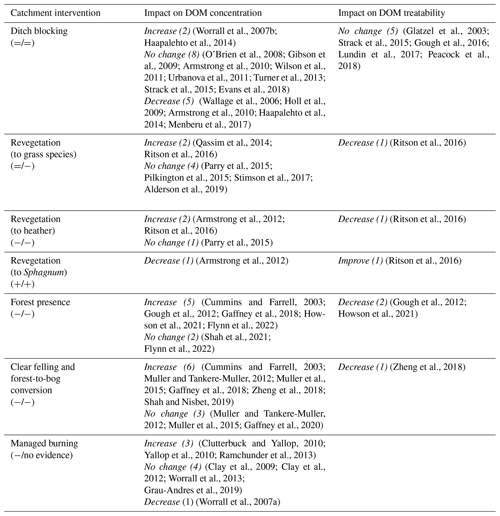
There has been comparatively little research on the effects of forest presence on the treatability of DOM, although Gough et al. (2012) evaluated DOM concentrations and SUVA254 values in waters draining catchments forested with different tree species. They found that pore water leachates from pine and larch plantations yielded particularly high DOM concentrations relative to a blanket bog control (19 and 13 mg L−1, respectively, compared with 9 mg L−1). Leachates also had lower SUVA254 values (1.2 and 2.4, respectively, compared with 3.3 L mg−1 m−1). This would suggest that DOM leaching from plantations dominated by these tree types may be less easily treatable than DOM from blanket bogs. Similarly, samples taken from Scottish blanket and raised bog sites (Howson et al., 2021) found that SUVA254 values were lower from forested sites, again suggesting that forestry on peat results in less aromatic, hydrophobic DOM that may be less easily removed via conventional coagulation, possibly because of additional DOM inputs from litter.
Recently, there have been attempts to restore previously afforested fen and bog peatlands in parts of Europe and North America under what is often referred to as “forest-to-bog” restoration (Chimner et al., 2017; Andersen et al., 2017), and national policies on peat restoration may lead to its expansion in future. Some of the studies listed in Table 2 (Muller and Tankere-Muller, 2012; Muller et al., 2015; Gaffney et al., 2018; Shah and Nisbet, 2019; Gaffney et al., 2020; Howson et al., 2021; Shah et al., 2021) monitored the impacts of felling as part of ongoing forest-to-bog restoration monitoring, with the main differences in management being that the trees were felled to waste (the practice of leaving felled trees in situ to rot) and there was less ground disturbance at the site compared with the use of machinery to extract felled timber (Gaffney, 2017). However, the practice of felling trees to waste has been suggested to provide a potential additional DOM source as the trees slowly decompose (Muller et al., 2015), with mulched fallen trees providing a major source of water-soluble DOM (Howson et al., 2021).
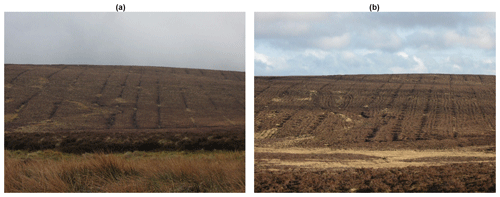
Figure 4Drainage ditches before (a) and after (b) blocking on a blanket bog in North Wales: the ditches run down the slope and individual dams can be seen crossing the ditches (photos: Chris Evans).
As bog vegetation regenerated after forest-to-bog restoration in the Flow Country, DOM concentrations decreased from elevated levels towards those seen in forest control areas. The time frame for complete recovery to pre-intervention levels is inconsistent to date, with some areas still showing elevated DOM at the restoration sites relative to the control sites after 17 years (Gaffney et al., 2018). At other sites, DOM concentrations had returned to those seen in intact blanket bog within the same time frame (Howson et al., 2021) or were showing inconsistent effects across sub-catchments (Pickard et al., 2022). Other studies have reported shorter-term perturbations in DOM (∼ 4–5 years) following forest-to-bog restoration, including within a lowland raised bog area in Scotland, Flanders Moss, where stream water baseline DOM levels were reached within 2 years at one site (Shah and Nisbet, 2019). In a Finnish study of the impacts of forest-to-mire restoration, a short-term peak in pore water DOM concentration following initial restoration activity was followed by a return to reference concentrations within 6 years (Menberu et al., 2017).
In summary, coniferous afforestation of peatlands increases DOM concentrations in pore waters and streams, both during site establishment, potentially during the forest growth, and again as the trees are felled (summarised in Table 3). Forest-to-bog restoration as a method of land management produces short-term increases in DOM concentrations while trees are felled and brash remaining on site decomposes. However, given a long enough time frame, DOM concentrations appear to decrease back towards levels seen at comparable control locations. From a water company perspective, it is important to note that this time frame can be up to 20 years in blanket bogs, i.e. considerably longer than the standard funding cycle. Removing felled timber and brash from the site, rather than felling to waste, would be expected to greatly reduce the magnitude and duration of any DOM peak.
3.4 Managed burning
Managed burning of peatland vegetation (Figs. 1, 5) (primarily the burning of Calluna sp. as part of grouse moor management) is a contentious issue within peatland conservation and management (e.g. Davies et al., 2016) and has been extensively reviewed and debated over the past decade, particularly in relation to the impacts on DOM (e.g. Worrall et al., 2010; Holden et al., 2012; Brown et al., 2015; Harper et al., 2018). There is little evidence within these reviews to suggest that DOM concentrations or colour increase within peat pore waters following managed burns. A recent study showed no change in DOM concentrations following low- and high-intensity burning (Grau-Andres et al., 2019), and pore water DOM concentrations were unchanged (Clay et al., 2009, 2012; Worrall et al., 2013) or decreased (Worrall et al., 2007a) in previous studies. At the catchment scale, positive correlations between the extent of burning and DOM concentrations and water colour have been interpreted as causal (Clutterbuck and Yallop, 2010; Yallop et al., 2010; Ramchunder et al., 2013), but this has been questioned in the literature (Holden et al., 2012). Burning as a management practice is designed to ensure that there is a mosaic of variously aged heather habitat, so it seems plausible that these effects are more linked to changes in vegetation cover. As previously discussed, C. vulgaris produced higher amounts of DOM than Sphagnum in the laboratory (Ritson et al., 2016) and at the plot scale (Armstrong et al., 2012). It is also worth noting that Evans et al. (2017b) found that a wildfire in Northern Ireland resulted in a temporary reduction in DOM concentrations in a downstream monitoring lake, which was attributed to re-acidification of catchment soils following the fire as well as the loss of DOM-producing vegetation cover.
4.1 The role of peatland catchment management
Table 3 summarises the range and extent of the current peer-reviewed evidence of the impacts of peatland restoration on DOM concentrations in raw water and the treatability of the DOM present. However, considerable knowledge gaps remain regarding the effects of peatland restoration on raw-water DOM concentrations and treatability. Our thorough screening of the literature revealed remarkably few published primary studies in this area, despite a widespread belief among UK conservation, policy and water industry groups that peatland degradation has driven increased DOM concentrations in upland water supplies (e.g. Anderson, 2012). This lack of evidence and the mixed findings of those studies that have been undertaken suggest that generalisations of the effects of most of the interventions examined must be taken with considerable caution.
The available literature does indicate that both revegetation of bare peat (particularly to Sphagnum-dominated bog) and ditch blocking is associated with decreased DOM concentrations within pore waters and ditches, at the location where restoration occurs. However, in contrast to reported positive impacts of these restoration actions with respect to carbon sequestration, soil particulate losses, flood management and upland biodiversity (Loisel and Gallego-Sala, 2022), evidence that such impacts may translate to quantitatively significant changes within the larger and more heterogeneous catchments that provide drinking water resources is generally lacking.
There is stronger evidence pointing to the risks posed by the afforestation of (naturally unforested) peatlands and the subsequent management of such plantations. Felling operations, including those associated with ongoing forest management and those associated with forest-to-bog restoration, tend to lead to increasing DOM concentrations and potentially reduced treatability of exported DOM. In the published literature, we have been unable to find experimental evidence incorporating local changes in water chemistry following interventions with changes in downstream DOM processing, to show whether water quality effects are detectable at the point of abstraction for water treatment works. This extension beyond the plot and hillslope scale represents a significant gap in current understanding, as DOM processing continues within the aquatic environment downstream of peatlands, and may be affected by upstream management.
Robust quantification of the impacts of catchment management on DOM concentration and treatability at the point of abstraction clearly represents a major current evidence gap. The spatial and temporal scale required to understand these impacts and the need for robust before–after control–impact (BACI) experiments entail a significant cost, which perhaps explains the current dearth of reliable information. This is particularly pertinent when changes in water chemistry may take a number of years to be seen, depending on catchment dynamics and possible in-reservoir processes. Our review has highlighted that studies of different land management approaches have not been followed downstream to monitor their impacts to the wider catchment.
4.2 DOM processing in drinking water catchments
The general paucity of evidence to support widespread (terrestrial) catchment-focussed interventions specifically to manage source water DOM concentrations and treatability leads then to the question as to whether there are other water quality management options that could be applied within reservoirs and whether these have been comparatively overlooked for DOM. DOM in rivers and lakes is subject to both biotic and abiotic processing, which affect both concentrations and the chemical structure (e.g. Tranvik et al., 2009) and, hence, treatability. For example DOM is lost to respiration (Koehler et al., 2012; Stets et al., 2010), sedimentation (Einola et al., 2011; von Wachenfeldt and Tranvik, 2008), photo-oxidisation (via UV radiation) (Moody et al., 2013; Koehler et al., 2014), and flocculation with naturally occurring aluminium and iron (McKnight et al., 1992; Koehler et al., 2014).
More importantly for treatability, however, DOM is generated within lakes and reservoirs via photosynthesis (production of algal exudates and release via cell lysis) and through the processing of particulate matter (Tranvik et al., 2009); thus, DOM concentrations at the point of abstraction from reservoirs represent the sum of these removal and generation processes. Consequently, the resulting DOM tends to be relatively transparent and hydrophilic compared with DOM generated by organic-rich soils, and thus presents different challenges for treatment, particularly as the hydrophilic DOM is not easily removed through coagulation (Matilainen et al., 2010) and may lead to the need for additional capital investment in order to effectively reduce residual DOM in drinking water.
Algal production, and hence within-reservoir generation of DOM, is often limited by the availability of phosphorus, nitrogen or both. Therefore, waterbodies with high concentrations of inorganic nutrients are likely to generate additional DOM within the water column (Feuchtmayr et al., 2019; Evans et al., 2017a). Further, evidence is growing with respect to the importance of lake and reservoir bed sediments as a direct source of DOM to the water column, with reducing conditions occurring during the stratification of lakes and reservoirs, causing the redissolution of previously sedimented organic matter (Peter et al., 2017).
In their assessment of DOM in lake and reservoir inflows and outflows, Evans et al. (2017a) concluded that any measures that can reduce N and P export from the catchment (e.g. Spears and May, 2015) or release from sediments or any measures that can strip nutrients from the water column (e.g. Spears et al., 2016) could provide effective mitigation for high DOM concentrations by reducing algal DOM production.
It is pertinent, therefore, to consider whether measures that reduce in-reservoir DOM production and/or favour in-reservoir DOM removal may be as – or perhaps more – effective than measures aimed at reducing DOM export from the terrestrial catchment. For lakes acting as DOM sources, management regimes that reduce nutrient (primarily N and P) inputs from catchments and/or internal loading of nutrients and DOM from sediment to the water column may be more effective than those focussed on reducing inflowing DOM concentrations directly. Restricting nutrient inputs is also likely to reduce organic nitrogen concentrations relative to organic carbon concentrations, which has the added benefit of reducing the formation potential of nitrogenous DBPs. In addition, Birk et al. (2020) suggest that increasing DOM loading from the catchment may act to dampen algal responses to nutrients via light limitation of primary production within some European lakes. If, by extension, this also limits in-reservoir DOM production, catchment interventions that relieve the DOM load but not the nutrient load may result in an increase in in-reservoir DOM production. Even in the case of less-nutrient-rich waterbodies, it appears that reducing N and P loadings would be beneficial for water treatment, as this is likely to restrict additional DOM formation.
In summary, our review demonstrates that catchment management initiatives, while providing clear overall restoration benefits for peatlands, have yet to deliver a generalised solution to the challenge of stabilising or reversing DOM increases in drinking water sources. There is some evidence that catchment interventions may provide benefits for DOM export in specific cases, but (with the possible exception of forest management activities) these have rarely been demonstrated consistently or at the whole-catchment scale. Furthermore, it now seems clear that the recent decadal-scale increase in surface water DOC concentrations was the result of an external driver (i.e. decreasing acid deposition), both in the UK and across large parts of Europe and North America, and cannot realistically be “managed away”. However, catchment management measures that reduce in-reservoir DOM production or favour in-reservoir DOM removal may be as or more effective, particularly with respect to more nutrient-rich systems. More generally, it seems clear that catchment management should be considered part of the response strategy to rising DOM levels and as part of a process to improve the resilience of source waters, not as a panacea. It is, therefore, important that research science and the water industry work together to measure variables at the temporal and spatial scales required and to develop effective tools to predict likely future DOM levels resulting from a combination of large-scale and catchment-scale drivers, to ensure that investments in both catchment management measures and DOM treatment infrastructure are correctly targeted, integrated, timely and cost-effective.
UWMN data are available upon request for non-commercial use from the website indicated in the legend of Fig. 2. No other datasets were used.
The work was conceptualised by DM and CE; funding acquisition was undertaken by DM, CE and BS; and JW carried out the initial review and wrote the manuscript with contribution from all authors.
The contact author has declared that none of the authors has any competing interests.
Publisher’s note: Copernicus Publications remains neutral with regard to jurisdictional claims in published maps and institutional affiliations.
We thank staff from Scottish Water, United Utilities, Yorkshire Water, Irish Water and Dŵr Cymru Welsh Water for their informative discussions and comments on early drafts of this paper. Discussions with Nadeem Shah and Tom Nisbet regarding work being undertaken by Forest Research are also gratefully acknowledged, as are the comments from two reviewers of an earlier draft of this paper. The artwork in Fig. 1 is by Andy Sier (UK Centre for Ecology & Hydrology).
This research has been supported by the Natural Environment Research Council (grant nos. NE/R009198/1, NE/S016937/2, and NE/N018087/1) and Scottish Water.
This paper was edited by Nicolas Brüggemann and reviewed by two anonymous referees.
Alderson, D. M., Evans, M. G., Shuttleworth, E. L., Pilkington, M., Spencer, T., Walker, J., and Allott, T. E. H.: Trajectories of ecosystem change in restored blanket peatlands, Sci. Total Environ., 665, 785–796, https://doi.org/10.1016/j.scitotenv.2019.02.095, 2019.
Algesten, G., Sobek, S., Bergstrom, A. K., Agren, A., Tranvik, L. J., and Jansson, M.: Role of lakes for organic carbon cycling in the boreal zone, Glob. Change Biol., 10, 141–147, https://doi.org/10.1111/j.1365-2486.2003.00721.x, 2004.
Andersen, R., Farrell, C., Graf, M., Muller, F., Calvar, E., Frankard, P., Caporn, S., and Anderson, P.: An overview of the progress and challenges of peatland restoration in Western Europe, Restor. Ecol., 25, 271–282, https://doi.org/10.1111/rec.12415, 2017.
Anderson, P.: United Utilities sustainable catchment management programme water quality monitoring results, Penny Anderson Associates Ltd., 2012.
Anderson, T. R., Rowe, E. C., Polimene, L., Tipping, E., Evans, C. D., Barry, C. D. G., Hansell, D. A., Kaiser, K., Kitidis, V., Lapworth, D. J., Mayor, D. J., Monteith, D. T., Pickard, A. E., Sanders, R. J., Spears, B. M., Torres, R., Tye, A. M., Wade, A. J., and Waska, H.: Unified concepts for understanding and modelling turnover of dissolved organic matter from freshwaters to the ocean: the UniDOM model, Biogeochemistry, 146, 105–123, https://doi.org/10.1007/s10533-019-00621-1, 2019.
Armstrong, A., Holden, J., Luxton, K., and Quinton, J. N.: Multi-scale relationship between peatland vegetation type and dissolved organic carbon concentration, Ecol. Eng., 47, 182–188, https://doi.org/10.1016/j.ecoleng.2012.06.027, 2012.
Armstrong, A., Holden, J., Kay, P., Francis, B., Foulger, M., Gledhill, S., McDonald, A. T., and Walker, A.: The impact of peatland drain-blocking on dissolved organic carbon loss and discolouration of water; results from a national survey, J. Hydrol., 381, 112–120, https://doi.org/10.1016/j.jhydrol.2009.11.031, 2010.
Berggren, M. and del Giorgio, P. A.: Distinct patterns of microbial metabolism associated to riverine dissolved organic carbon of different source and quality, J. Geophys. Res.-Biogeo., 120, 989–999, https://doi.org/10.1002/2015jg002963, 2015.
Berggren, M., Klaus, M., Selvam, B. P., Strom, L., Laudon, H., Jansson, M., and Karlsson, J.: Quality transformation of dissolved organic carbon during water transit through lakes: contrasting controls by photochemical and biological processes, Biogeosciences, 15, 457–470, https://doi.org/10.5194/bg-15-457-2018, 2018.
Birk, S., Chapman, D., Carvalho, L., Spears, B. M., Andersen, H. E., Argillier, C., Auer, S., Baattrup-Pedersen, A., Banin, L., Beklioğlu, M., Bondar-Kunze, E., Borja, A., Branco, P., Bucak, T., Buijse, A. D., Cardoso, A. C., Couture, R.-M., Cremona, F., de Zwart, D., Feld, C. K., Ferreira, M. T., Feuchtmayr, H., Gessner, M. O., Gieswein, A., Globevnik, L., Graeber, D., Graf, W., Gutiérrez-Cánovas, C., Hanganu, J., Işkın, U., Järvinen, M., Jeppesen, E., Kotamäki, N., Kuijper, M., Lemm, J. U., Lu, S., Solheim, A. L., Mischke, U., Moe, S. J., Nõges, P., Nõges, T., Ormerod, S. J., Panagopoulos, Y., Phillips, G., Posthuma, L., Pouso, S., Prudhomme, C., Rankinen, K., Rasmussen, J. J., Richardson, J., Sagouis, A., Santos, J. M., Schäfer, R. B., Schinegger, R., Schmutz, S., Schneider, S. C., Schülting, L., Segurado, P., Stefanidis, K., Sures, B., Thackeray, S. J., Turunen, J., Uyarra, M. C., Venohr, M., von der Ohe, P. C., Willby, N., and Hering, D.: Impacts of multiple stressors on freshwater biota across spatial scales and ecosystems, Nat. Ecol. Evol., 4, 1060–1068, https://doi.org/10.1038/s41559-020-1216-4, 2020.
Brown, L. E., Holden, J., Palmer, S. M., Johnston, K., Ramchunder, S. J., and Grayson, R.: Effects of fire on the hydrology, biogeochemistry, and ecology of peatland river systems, Freshw. Sci., 34, 1406–1425, https://doi.org/10.1086/683426, 2015.
Chapman, P. J., McDonald, A. T., Tyson, R., Palmer, S. M., Mitchell, G., and Irvine, B.: Changes in water colour between 1986 and 2006 in the headwaters of the River Nidd, Yorkshire, UK, Biogeochemistry, 101, 281–294, https://doi.org/10.1007/s10533-010-9474-x, 2010.
Chapman, P. J., Moody, C. S., Grayson, R., and Palmer, S. M.: Factors controlling water colour on the North York Moors, Part 1, University of Leeds, 2017.
Chimner, R. A., Cooper, D. J., Wurster, F. C., and Rochefort, L.: An overview of peatland restoration in North America: where are we after 25 years?, Restor. Ecol., 25, 283–292, https://doi.org/10.1111/rec.12434, 2017.
Clark, J. M., Ashley, D., Wagner, M., Chapman, P. J., Lane, S. N., Evans, C. D., and Heathwaite, A. L.: Increased temperature sensitivity of net DOC production from ombrotrophic peat due to water table draw-down, Glob. Change Biol., 15, 794–807, https://doi.org/10.1111/j.1365-2486.2008.01683.x, 2009.
Clay, G. D., Worrall, F., and Fraser, E. D. G.: Effects of managed burning upon dissolved organic carbon (DOC) in soil water and runoff water following a managed burn of a UK blanket bog, J. Hydrol., 367, 41–51, https://doi.org/10.1016/j.jhydrol.2008.12.022, 2009.
Clay, G. D., Worrall, F., and Aebischer, N. J.: Does prescribed burning on peat soils influence DOC concentrations in soil and runoff waters? Results from a 10 year chronosequence, J. Hydrol., 448, 139–148, https://doi.org/10.1016/j.jhydrol.2012.04.048, 2012.
Clutterbuck, B. and Yallop, A. R.: Land management as a factor controlling dissolved organic carbon release from upland peat soils 2 Changes in DOC productivity over four decades, Sci. Total Environ., 408, 6179–6191, https://doi.org/10.1016/j.scitotenv.2010.08.038, 2010.
Cummins, T. and Farrell, E. P.: Biogeochemical impacts of clearfelling and reforestation on blanket-peatland streams – II. major ions and dissolved organic carbon, Forest Ecol. Manag., 180, 557–570, https://doi.org/10.1016/s0378-1127(02)00649-7, 2003.
Davies, G. M., Kettridge, N., Stoof, C. R., Gray, A., Ascoli, D., Fernandes, P. M., Marrs, R., Allen, K. A., Doerr, S. H., Clay, G. D., McMorrow, J., and Vandvik, V.: The role of fire in UK peatland and moorland management: the need for informed, unbiased debate, Philos. T. R. Soc. B, 371, 20150342, https://doi.org/10.1098/rstb.2015.0342, 2016.
de Wit, H. A., Stoddard, J. L., Monteith, D. T., Sample, J. E., Austnes, K., Couture, S., Fölster, J., Higgins, S. N., Houle, D., Hruška, J., Krám, P., Kopáček, J., Paterson, A. M., Valinia, S., Van Dam, H., Vuorenmaa, J., and Evans, C. D.: Cleaner air reveals growing influence of climate on dissolved organic carbon trends in northern headwaters, Environ. Res. Lett., 16, 104009, https://doi.org/10.1088/1748-9326/ac2526, 2021.
Ding, S. and Chu, W.: Recent advances in the analysis of nitrogenous disinfection by-products, Trend. Environ. Anal. Chem., 14, 19–27, https://doi.org/10.1016/j.teac.2017.04.001, 2017.
Einola, E., Rantakari, M., Kankaala, P., Kortelainen, P., Ojala, A., Pajunen, H., Makela, S., and Arvola, L.: Carbon pools and fluxes in a chain of five boreal lakes: A dry and wet year comparison, J. Geophys. Res.-Biogeo., 116, G03009, https://doi.org/10.1029/2010jg001636, 2011.
Evans, C. D., Monteith, D. T., and Cooper, D. M.: Long-term increases in surface water dissolved organic carbon: Observations, possible causes and environmental impacts, Environ. Pollut., 137, 55–71, https://doi.org/10.1016/j.envpol.2004.12.031, 2005.
Evans, C. D., Jones, T. G., Burden, A., Ostle, N., Zielinski, P., Cooper, M. D. A., Peacock, M., Clark, J. M., Oulehle, F., Cooper, D., and Freeman, C.: Acidity controls on dissolved organic carbon mobility in organic soils, Glob. Change Biol., 18, 3317–3331, https://doi.org/10.1111/j.1365-2486.2012.02794.x, 2012.
Evans, C. D., Page, S. E., Jones, T., Moore, S., Gauci, V., Laiho, R., Hruska, J., Allott, T. E. H., Billett, M. F., Tipping, E., Freeman, C., and Garnett, M. H.: Contrasting vulnerability of drained tropical and high-latitude peatlands to fluvial loss of stored carbon, Global Biogeochem. Cy., 28, 1215–1234, https://doi.org/10.1002/2013gb004782, 2014.
Evans, C. D., Futter, M. N., Moldan, F., Valinia, S., Frogbrook, Z., and Kothawala, D. N.: Variability in organic carbon reactivity across lake residence time and trophic gradients, Nat. Geosci., 10, 832–835, https://doi.org/10.1038/ngeo3051, 2017a.
Evans, C. D., Malcolm, I. A., Shilland, E. M., Rose, N. L., Turner, S. D., Crilly, A., Norris, D., Granath, G., and Monteith, D. T.: Sustained Biogeochemical Impacts of Wildfire in a Mountain Lake Catchment, Ecosystems, 20, 813–829, https://doi.org/10.1007/s10021-016-0064-1, 2017b.
Evans, C. D., Peacock, M., Green, S. M., Holden, J., Chapman, P. J., Lebron, I., Callaghan, N., Grayson, R., and Baird, A.: The impact of ditch blocking on fluvial carbon export from a UK blanket bog, Hydrol. Process., 32, 2141–2154, 2018.
Feuchtmayr, H., Pottinger, T. G., Moore, A., De Ville, M. M., Caillouet, L., Carter, H. T., Pereira, M. G., and Maberly, S. C.: Effects of brownification and warming on algal blooms, metabolism and higher trophic levels in productive shallow lake mesocosms, Sci. Total Environ., 678, 227–238, https://doi.org/10.1016/j.scitotenv.2019.04.105, 2019.
Flynn, R., Mackin, F., McVeigh, C., and Renou-Wilson, F.: Impacts of a mature forestry plantation on blanket peatland runoff regime and water quality, Hydrol. Process., 36, e14494, https://doi.org/10.1002/hyp.14494, 2022.
Forestry Commission: The UK Forestry Standard, Edinburgh, Forestry Commission, ISBN: 978-0-85538-999-4, 2017.
Gaffney, P.: The effects of bog restoration in formerly afforested peatlands on water quality and aquatic carbon fluxes, PhD thesis, Environmental Research Institute, University of the Highlands and Islands, 2017.
Gaffney, P. P. J., Hancock, M. H., Taggart, M. A., and Andersen, R.: Measuring restoration progress using pore- and surface-water chemistry across a chronosequence of formerly afforested blanket bogs, J. Environ. Manag., 219, 239–251, https://doi.org/10.1016/j.jenvman.2018.04.106, 2018.
Gaffney, P. P. J., Hancock, M. H., Taggart, M. A., and Andersen, R.: Restoration of afforested peatland: Immediate effects on aquatic carbon loss, Sci. Total Environ., 742, 140594, https://doi.org/10.1016/j.scitotenv.2020.140594, 2020.
Gibson, H. S., Worrall, F., Burt, T. P., and Adamson, J. K.: DOC budgets of drained peat catchments: implications for DOC production in peat soils, Hydrol. Process., 23, 1901–1911, https://doi.org/10.1002/hyp.7296, 2009.
Glatzel, S., Kalbitz, K., Dalva, M., and Moore, T.: Dissolved organic matter properties and their relationship to carbon dioxide efflux from restored peat bogs, Geoderma, 113, 397–411, https://doi.org/10.1016/S0016-7061(02)00372-5, 2003.
Glenk, K. and Martin-Ortega, J.: The economics of peatland restoration, J. Environ. Econ. Policy, 7, 345–362, https://doi.org/10.1080/21606544.2018.1434562, 2018.
Gough, R., Holliman, P. J., Willis, N., Jones, T. G., and Freeman, C.: Influence of habitat on the quantity and composition of leachable carbon in the O2 horizon: Potential implications for potable water treatment, Lake Reserv. Manag., 28, 282–292, https://doi.org/10.1080/07438141.2012.741187, 2012.
Gough, R., Holliman, P. J., Fenner, N., Peacock, M., and Freeman, C.: Influence of Water Table Depth on Pore Water Chemistry and Trihalomethane Formation Potential in Peatlands, Water Environ. Res., 88, 107–117, https://doi.org/10.2175/106143015x14362865227878, 2016.
Grau-Andres, R., Davies, G. M., Waldron, S., Scott, E. M., and Gray, A.: Increased fire severity alters initial vegetation regeneration across Calluna-dominated ecosystems, J. Environ. Manag., 231, 1004–1011, https://doi.org/10.1016/j.jenvman.2018.10.113, 2019.
Haapalehto, T., Kotiaho, J. S., Matilainen, R., and Tahvanainen, T.: The effects of long-term drainage and subsequent restoration on water table level and pore water chemistry in boreal peatlands, J. Hydrol., 519, 1493–1505, https://doi.org/10.1016/j.jhydrol.2014.09.013, 2014.
Harper, A. R., Doerr, S. H., Santin, C., Froyd, C. A., and Sinnadurai, P.: Prescribed fire and its impacts on ecosystem services in the UK, Sci. Total Environ., 624, 691–703, https://doi.org/10.1016/j.scitotenv.2017.12.161, 2018.
Harriman, R., Watt, A. W., Christie, A. E. G., Collen, P., Moore, D. W., McCartney, A. G., Taylor, E. M., and Watson, J.: Interpretation of trends in acidic deposition and surface water chemistry in Scotland during the past three decades, Hydrol. Earth Syst. Sci., 5, 407–420, https://doi.org/10.5194/hess-5-407-2001, 2001.
Holden, J., Kirkby, M. J., Lane, S. N., Milledge, D. G., Brookes, C. J., Holden, V., and McDonald, A. T.: Overland flow velocity and roughness properties in peatlands, Water Resour. Res., 44, W06415, https://doi.org/10.1029/2007wr006052, 2008.
Holden, J., Wallage, Z. E., Lane, S. N., and McDonald, A. T.: Water table dynamics in undisturbed, drained and restored blanket peat, J. Hydrol., 402, 103–114, https://doi.org/10.1016/j.jhydrol.2011.03.010, 2011.
Holden, J., Chapman, P. J., Palmer, S. M., Kay, P., and Grayson, R.: The impacts of prescribed moorland burning on water colour and dissolved organic carbon: A critical synthesis, J. Environ. Manag., 101, 92–103, https://doi.org/10.1016/j.jenvman.2012.02.002, 2012.
Holden, J., Green, S. M., Baird, A. J., Grayson, R. P., Dooling, G. P., Chapman, P. J., Evans, C. D., Peacock, M., and Swindles, G.: The impact of ditch blocking on the hydrological functioning of blanket peatlands, Hydrol. Process., 31, 525–539, https://doi.org/10.1002/hyp.11031, 2017.
Holl, B. S., Fiedler, S., Jungkunst, H. F., Kalbitz, K., Freibauer, A., Drosler, M., and Stahr, K.: Characteristics of dissolved organic matter following 20 years of peatland restoration, Sci. Total Environ., 408, 78–83, https://doi.org/10.1016/j.scitotenv.2009.08.046, 2009.
Howson, T., Chapman, P. J., Shah, N., Anderson, R., and Holden, J.: A comparison of porewater chemistry between intact, afforested and restored raised and blanket bogs, Sci. Total Environ., 766, 144496, https://doi.org/10.1016/j.scitotenv.2020.144496, 2021.
Hudson, J. A., Gilman, K., and Calder, I. R.: Land use and water issues in the uplands with reference to the Plynlimon study, Hydrol. Earth Syst. Sci., 1, 389–397, https://doi.org/10.5194/hess-1-389-1997, 1997.
Imai, A., Fukushima, T., Matsushige, K., Kim, Y.-H., and Choi, K.: Characterization of dissolved organic matter in effluents from wastewater treatment plants, Water Res., 36, 859–870, https://doi.org/10.1016/S0043-1354(01)00283-4, 2002.
IUCN Peatland Programme: https://www.iucn-uk-peatlandprogramme.org/, last access: 5 January 2022.
Jandl, R., Lindner, M., Vesterdal, L., Bauwens, B., Baritz, R., Hagedorn, F., Johnson, D. W., Minkkinen, K., and Byrne, K. A.: How strongly can forest management influence soil carbon sequestration?, Geoderma, 137, 253–268, https://doi.org/10.1016/j.geoderma.2006.09.003, 2007.
Jones, T. G., Evans, C. D., and Freeman, C.: The greenhouse gas (GHG) emissions associated with aquatic carbon removal during drinking water treatment, Aquat. Sci., 78, 561–572, https://doi.org/10.1007/s00027-015-0458-8, 2016.
Koehler, B., von Wachenfeldt, E., Kothawala, D., and Tranvik, L. J.: Reactivity continuum of dissolved organic carbon decomposition in lake water, J. Geophys. Res.-Biogeo., 117, G01024, https://doi.org/10.1029/2011jg001793, 2012.
Koehler, B., Landelius, T., Weyhenmeyer, G. A., Machida, N., and Tranvik, L. J.: Sunlight-induced carbon dioxide emissions from inland waters, Global Biogeochem. Cy., 28, 696–711, https://doi.org/10.1002/2014gb004850, 2014.
Loisel, J. and Gallego-Sala, A.: Ecological resilience of restored peatlands to climate change, Commun. Earth Environ., 3, 208–216, https://doi.org/10.1038/s43247-022-00547-x, 2022.
Lundin, L., Nilsson, T., Jordan, S., Lode, E., and Strömgren, M.: Impacts of rewetting on peat, hydrology and water chemical composition over 15 years in two finished peat extraction areas in Sweden, Wetlands Ecol. Manag., 25, 405–419, https://doi.org/10.1007/s11273-016-9524-9, 2017.
Matilainen, A., Vepsäläinen, M., and Sillanpää, M.: Natural organic matter removal by coagulation during drinking water treatment: A review, Adv. Colloid Interfac., 159, 189–197, https://doi.org/10.1016/j.cis.2010.06.007, 2010.
McKnight, D. M., Bencala, K. E., Zellweger, G. W., Aiken, G. R., Feder, G. L., and Thorn, K. A.: Sorption of dissolved organic carbon by hydrous aluminum and iron oxides occurring at the confluence of Deer Creek with the Snake River, Summit County, Colorado, Environ. Sci. Technol., 26, 1388–1396, https://doi.org/10.1021/es00031a017, 1992.
Menberu, M., Mattila, H., Tahvanainen, T., Kotiaho, J. S., Hokkanen, R., Klove, B., and Ronkanen, A.: Changes in pore water quality after peatland restoration: assessment of a large scale replicated before-after-control-impact study in Finland, Water Resour. Res., 53, 8327–8343, 2017.
Monteith, D. T., Stoddard, J. L., Evans, C. D., de Wit, H. A., Forsius, M., Hogasen, T., Wilander, A., Skjelkvale, B. L., Jeffries, D. S., Vuorenmaa, J., Keller, B., Kopacek, J., and Vesely, J.: Dissolved organic carbon trends resulting from changes in atmospheric deposition chemistry, Nature, 450, 537–539, https://doi.org/10.1038/nature06316, 2007.
Monteith, D., Pickard, A. E., Spears, B. M., and Feuchtmayr, H.: An introduction to the FREEDOM-BCCR project: FREEDOM-BCCR briefing note 1 to the water industry, https://www.ceh.ac.uk/sites/default/files/2021-09/FREEDOM_BCCR_Project_Introduction_01.pdf (last access: 11 September 2023), 2021.
Monteith, D. T., Henrys, P. A., Hruška, J., de Wit, H. A., Krám, P., Moldan, F., Posch, M., Räike, A., Stoddard, J. L., Shilland, E. M., Pereira, M. G., and Evans, C. D.: Long-term rise in riverine dissolved organic carbon concentration is predicted by electrolyte solubility theory, Sci. Adv., 9, eade3491, https://doi.org/10.1126/sciadv.ade3491, 2023.
Moody, C. S., Worrall, F., Evans, C. D., and Jones, T. G.: The rate of loss of dissolved organic carbon (DOC) through a catchment, J. Hydrol., 492, 139–150, https://doi.org/10.1016/j.jhydrol.2013.03.016, 2013.
Muller, F. L. L. and Tankere-Muller, S. P. C.: Seasonal variations in surface water chemistry at disturbed and pristine peatland sites in the Flow Country of northern Scotland, Sci. Total Environ., 435, 351–362, https://doi.org/10.1016/j.scitotenv.2012.06.048, 2012.
Muller, F. L. L., Chang, K.-C., Lee, C.-L., and Chapman, S. J.: Effects of temperature, rainfall and conifer felling practices on the surface water chemistry of northern peatlands, Biogeochemistry, 126, 343–362, https://doi.org/10.1007/s10533-015-0162-8, 2015.
Naden, P. S. and McDonald, A. T.: Statistical modeling of water color in the uplands – the Upper Nidd catchment 1979–1987, Environ. Pollut., 60, 141–163, https://doi.org/10.1016/0269-7491(89)90224-8, 1989.
Neal, C.: Nutrient concentrations and fluxes for podzolic and gley soils at Plynlimon, mid-Wales: implications for modelling inorganic nitrogen and phosphorus in upland UK environments, Hydrol. Earth Syst. Sci., 6, 403–420, https://doi.org/10.5194/hess-6-403-2002, 2002.
O'Brien, H. E., Labadz, J. C., Butcher, D. P., Billett, M. F., and Midgley, N. G.: Impact of catchment management upon dissolved organic carbon and stream flows in the Peak District, Derbyshire, UK, 10th BHS National Hydrology Symposium, Exeter, 15–17 September 2008, Location Exeter, Publisher British Hydrological Society, ISBN: 1903741-16-5, 2008.
Parry, L. E., Chapman, P. J., Palmer, S. M., Wallage, Z. E., Wynne, H., and Holden, J.: The influence of slope and peatland vegetation type on riverine dissolved organic carbon and water colour at different scales, Sci. Total Environ., 527, 530–539, https://doi.org/10.1016/j.scitotenv.2015.03.036, 2015.
Patterson, G. and Anderson, R.: Forests and Peatland Habitats, Forestry Commission, Edinburgh, ISBN: 0-85538-528-6, 2000.
Peacock, M., Jones, T. G., Futter, M. N., Freeman, C., Gough, R., Baird, A. J., Green, S. M., Chapman, P. J., Holden, J., and Evans, C. D.: Peatland ditch blocking has no effect on dissolved organic matter (DOM) quality, Hydrol. Process., 32, 3891–3906, https://doi.org/10.1002/hyp.13297, 2018.
Peter, S., Agstam, O., and Sobek, S.: Widespread release of dissolved organic carbon from anoxic boreal lake sediments, Inland Waters, 7, 151–163, https://doi.org/10.1080/20442041.2017.1300226, 2017.
Pickard, A. E., Branagan, M., Billett, M. F., Andersen, R., and Dinsmore, K. J.: Effects of peatland management on aquatic carbon concentrations and fluxes, Biogeosciences, 19, 1321–1334, https://doi.org/10.5194/bg-19-1321-2022, 2022.
Pilkington, M., Walker, J., Maskill, R., Allott, T. E. H., and Evans, M.: Restoration of blanket bogs; flood risk reduction and other ecosystem benefits, Moors for the Future Partnership, Edale, 2015.
Prest, E. I., Hammes, F., van Loosdrecht, M. C. M., and Vrouwenvelder, J. S.: Biological Stability of Drinking Water: Controlling Factors, Methods, and Challenges, Front. Microbiol., 7, 45, https://doi.org/10.3389/fmicb.2016.00045, 2016.
Qassim, S. M., Dixon, S. D., Rowson, J. G., Worrall, F., Evans, M. G., and Bonn, A.: A 5-year study of the impact of peatland revegetation upon DOC concentrations, J. Hydrol., 519, 3578–3590, https://doi.org/10.1016/j.jhydrol.2014.11.014, 2014.
Ramchunder, S. J., Brown, L. E., and Holden, J.: Rotational vegetation burning effects on peatland stream ecosystems, J. Appl. Ecol., 50, 636–648, https://doi.org/10.1111/1365-2664.12082, 2013.
Rask, M., Nyberg, K., Markkanen, S.-L., and Ojala, A.: Forestry in catchments: effects on water quality, plankton, zoobenthos and fish in small lakes, Boreal Environ. Res., 3, 75–86, 1998.
Ritson, J. P., Graham, N. J. D., Templeton, M. R., Clark, J. M., Gough, R., and Freeman, C.: The impact of climate change on the treatability of dissolved organic matter (DOM) in upland water supplies: A UK perspective, Sci. Total Environ., 473/474, 714–730, https://doi.org/10.1016/j.scitotenv.2013.12.095, 2014.
Ritson, J. P., Bell, M., Brazier, R. E., Grand-Clement, E., Graham, N. J. D., Freeman, C., Smith, D., Templeton, M. R., and Clark, J. M.: Managing peatland vegetation for drinking water treatment, Sci. Rep., 6, 36751, https://doi.org/10.1038/srep36751, 2016.
Robson, A. J. and Neal, C.: Water quality trends at an upland site in Wales, UK, 1983–1993, Hydrol. Process., 10, 183–203, https://doi.org/10.1002/(sici)1099-1085(199602)10:2<183::Aid-hyp356>3.0.Co;2-8, 1996.
Schelker, J., Eklof, K., Bishop, K., and Laudon, H.: Effects of forestry operations on dissolved organic carbon concentrations and export in boreal first-order streams, J. Geophys. Res.-Biogeo., 117, G01011, https://doi.org/10.1029/2011jg001827, 2012.
Shah, N. and Nisbet, T.: The effects of forest clearance for peatland restoration on water quality, Sci. Total Environ., 693, 133617, https://doi.org/10.1016/j.scitotenv.2019.133617, 2019.
Shah, N. W., Nisbet, T. R., and Broadmeadow, S. B.: The impacts of conifer afforestation and climate on water quality and freshwater ecology in a sensitive peaty catchment: A 25 year study in the upper River Halladale in North Scotland, Forest Ecol. Manag., 502, 119616, https://doi.org/10.1016/j.foreco.2021.119616, 2021.
Skerlep, M., Steiner, E., Axelsson, A. L., and Kritzberg, E. S.: Afforestation driving long-term surface water browning, Glob. Change Biol., 26, 1390–1399, https://doi.org/10.1111/gcb.14891, 2019.
Spears, B. M. and May, L.: Long-term homeostasis of filterable un-reactive phosphorus in a shallow eutrophic lake following a significant reduction in catchment load, Geoderma, 257/258, 78–85, https://doi.org/10.1016/j.geoderma.2015.01.005, 2015.
Spears, B. M., Mackay, E. B., Yasseri, S., Gunn, I. D. M., Waters, K. E., Andrews, C., Cole, S., De Ville, M., Kelly, A., Meis, S., Moore, A. L., Nürnberg, G. K., van Oosterhout, F., Pitt, J.-A., Madgwick, G., Woods, H. J., and Lürling, M.: A meta-analysis of water quality and aquatic macrophyte responses in 18 lakes treated with lanthanum modified bentonite (Phoslock®), Water Res., 97, 111–121, https://doi.org/10.1016/j.watres.2015.08.020, 2016.
Stets, E. G., Striegl, R. G., and Aiken, G. R.: Dissolved organic carbon export and internal cycling in small, headwater lakes, Global Biogeochem. Cy., 24, Gb4008, https://doi.org/10.1029/2010gb003815, 2010.
Stimson, A. G., Allott, T. E. H., Boult, S., and Evans, M. G.: Fluvial organic carbon composition and concentration variability within a peatland catchment-Implications for carbon cycling and water treatment, Hydrol. Process., 31, 4183–4194, https://doi.org/10.1002/hyp.11352, 2017.
Strack, M., Zuback, Y., McCarter, C., and Price, J.: Changes in dissolved organic carbon quality in soils and discharge 10 years after peatland restoration, J. Hydrol., 527, 345–354, https://doi.org/10.1016/j.jhydrol.2015.04.061, 2015.
Stretton, C.: Water supply and forestry – a conflict of interests: Cray reservoir, a case study, J. Inst. Water Eng. Sci., 38, 323–330, 1984.
Tranvik, L. J., Downing, J. A., Cotner, J. B., Loiselle, S. A., Striegl, R. G., Ballatore, T. J., Dillon, P., Finlay, K., Fortino, K., Knoll, L. B., Kortelainen, P. L., Kutser, T., Larsen, S., Laurion, I., Leech, D. M., McCallister, S. L., McKnight, D. M., Melack, J. M., Overholt, E., Porter, J. A., Prairie, Y., Renwick, W. H., Roland, F., Sherman, B. S., Schindler, D. W., Sobek, S., Tremblay, A., Vanni, M. J., Verschoor, A. M., von Wachenfeldt, E., and Weyhenmeyer, G. A.: Lakes and reservoirs as regulators of carbon cycling and climate, Limnol. Oceanogr., 54, 2298–2314, 2009.
Turner, E. K., Worrall, F., and Burt, T. P.: The effect of drain blocking on the dissolved organic carbon (DOC) budget of an upland peat catchment in the UK, J. Hydrol., 479, 169–179, https://doi.org/10.1016/j.jhydrol.2012.11.059, 2013.
Urbanova, Z., Picek, T., and Barta, J.: Effect of peat re-wetting on carbon and nutrient fluxes, greenhouse gas production and diversity of methanogenic archaeal community, Ecol. Eng., 37, 1017–1026, https://doi.org/10.1016/j.ecoleng.2010.07.012, 2011.
von Wachenfeldt, E. and Tranvik, L. J.: Sedimentation in boreal lakes – The role of flocculation of allochthonous dissolved organic matter in the water column, Ecosystems, 11, 803–814, https://doi.org/10.1007/s10021-008-9162-z, 2008.
Wallage, Z. E., Holden, J., and McDonald, A. T.: Drain blocking: An effective treatment for reducing dissolved organic carbon loss and water discolouration in a drained peatland, Sci. Total Environ., 367, 811–821, https://doi.org/10.1016/j.scitotenv.2006.02.010, 2006.
Weishaar, J. L., Aiken, G. R., Bergamaschi, B. A., Fram, M. S., Fujii, R., and Mopper, K.: Evaluation of specific ultraviolet absorbance as an indicator of the chemical composition and reactivity of dissolved organic carbon, Environ. Sci. Technol., 37, 4702–4708, https://doi.org/10.1021/es030360x, 2003.
Williamson, J. L., Tye, A., Lapworth, D. J., Monteith, D., Sanders, R., Mayor, D. J., Barry, C., Bowes, M., Bowes, M., Burden, A., Callaghan, N., Farr, G., Felgate, S., Fitch, A., Gibb, S., Gilbert, P., Hargreaves, G., Keenan, P., Kitidis, V., Juergens, M., Martin, A., Mounteney, I., Nightingale, P. D., Pereira, M. G., Olszewska, J., Pickard, A., Rees, A. P., Spears, B., Stinchcombe, M., White, D., Williams, P., Worrall, F., and Evans, C.: Landscape controls on riverine export of dissolved organic carbon from Great Britain, Biogeochemistry, 163–184, https://doi.org/10.1007/s10533-021-00762-2, 2021.
Wilson, L., Wilson, J., Holden, J., Johnstone, I., Armstrong, A., and Morris, M.: Ditch blocking, water chemistry and organic carbon flux: Evidence that blanket bog restoration reduces erosion and fluvial carbon loss, Sci. Total Environ., 409, 2010–2018, https://doi.org/10.1016/j.scitotenv.2011.02.036, 2011.
Worrall, F., Harriman, R., Evans, C. D., Watts, C. D., Adamson, J., Neal, C., Tipping, E., Burt, T., Grieve, I., Monteith, D., Naden, P. S., Nisbet, T., Reynolds, B., and Stevens, P.: Trends in dissolved organic carbon in UK rivers and lakes, Biogeochemistry, 70, 369–402, https://doi.org/10.1007/s10533-004-8131-7, 2004.
Worrall, F., Armstrong, A., and Adamson, J. K.: The effects of burning and sheep-grazing on water table depth and soil water quality in a upland peat, J. Hydrol., 339, 1–14, https://doi.org/10.1016/j.jhydrol.2006.12.025, 2007a.
Worrall, F., Armstrong, A., and Holden, J.: Short-term impact of peat drain-blocking on water colour, dissolved organic carbon concentration, and water table depth, J. Hydrol., 337, 315–325, https://doi.org/10.1016/j.jhydrol.2007.01.046, 2007b.
Worrall, F., Clay, G. D., Marrs, R., and Reed, M.: Impacts of burning management on peatlands, IUCN technical report, 2010.
Worrall, F., Rowson, J., and Dixon, S.: Effects of managed burning in comparison with vegetation cutting on dissolved organic carbon concentrations in peat soils, Hydrol. Process., 27, 3994–4003, https://doi.org/10.1002/hyp.9474, 2013.
Xu, J., Morris, P. J., Liu, J., and Holden, J.: Hotspots of peatland-derived potable water use identified by global analysis, Nat. Sustain., 1, 246–253, https://doi.org/10.1038/s41893-018-0064-6, 2018.
Yallop, A. R., Clutterbuck, B., and Thacker, J.: Increases in humic dissolved organic carbon export from upland peat catchments: the role of temperature, declining sulphur deposition and changes in land management, Clim. Res., 45, 43–56, https://doi.org/10.3354/cr00884, 2010.
Zheng, Y., Waldron, S., and Flowers, H.: Fluvial dissolved organic carbon composition varies spatially and seasonally in a small catchment draining a wind farm and felled forestry, Sci. Total Environ., 626, 785–794, https://doi.org/10.1016/j.scitotenv.2018.01.001, 2018.