the Creative Commons Attribution 4.0 License.
the Creative Commons Attribution 4.0 License.
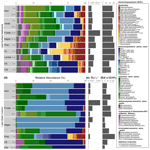
Distributions of bacteriohopanepolyols in lakes and coastal lagoons of the Azores Archipelago
Ellen C. Hopmans
Danica Mitrović
Pedro M. Raposeiro
Vítor Gonçalves
Ana C. Costa
Linda A. Amaral-Zettler
Laura Villanueva
Darci Rush
Bacteriohopanepolyols (BHPs) are a diverse class of lipids produced by bacteria across a wide range of environments. In this study, we aim to further identify BHPs related to ecological niches and/or specific bacteria by characterizing the distribution of BHPs in suspended particulate matter (SPM) of the water column and in sediments in a range of lakes and coastal lagoons from the Azores Archipelago, as well as in a co-culture enriched for methanotrophs. Sediment samples from Azorean lakes with low-oxygen conditions during the summer months (i.e., Azul, Verde, Funda, and Negra) contain relatively high abundances of BHPs that are typically associated with methane-oxidizing (methanotrophic) bacteria (i.e., aminotetrol, aminopentol, and methylcarbamate-aminopentol), as well as the ethenolamine-BHPs (i.e., ethenolamine-BHpentol and ethenolamine-BHhexol) and the N-formylated aminoBHPs. Both ethenolamine-BHPs and N-formylated aminoBHPs were also detected in a methanotroph–methylotroph co-culture that was enriched from a lake. In the SPM of all water columns, bacteriohopanetetrol (BHT), BHT cyclitol ether, and aminotriol are the dominant BHPs. In SPM from Lake Funda, nucleoside BHPs (i.e., Me-adenosylhopaneHG-diMe (where HG refers to head group), N1-methylinosylhopane, 2Me-N1-inosylhopane, and Me-N1-inosylhopane) are present in low abundance or absent under oxic conditions but increase in concentration near the chemocline, suggesting potential in situ production of these nucleoside BHPs rather than an allochthonous origin. In contrast, sediments from shallow, well-mixed lakes (i.e., Empadadas, São Jorge, and Lomba) contain higher abundances of adenosylhopane and N1-methylinosylhopane, which likely originate from bacteria living in nearby soils. Based on our current results we revised the existing Rsoil index, which was previously used to infer terrestrial inputs to aquatic environments, to exclude any potential nucleosides produced in the lake water column (Rsoil-lake). In the coastal lagoons, Cubres East and Cubres West, methoxylated BHTs were detected, and higher abundances of ethenolamine-BHT were observed. This study highlights the diversity of BHPs in lakes and coastal lagoons and their potential as taxonomic markers for bacteria associated with certain ecological niches, which can be preserved in sedimentary records.
- Article
(9595 KB) -
Supplement
(375 KB) - BibTeX
- EndNote
Bacteriohopanepolyols (BHPs) are pentacyclic triterpenoids found in the cell membrane of many gram-negative and gram-positive bacteria (Rohmer et al., 1984). Hopanoid derivatives of BHPs are considered to be some of the most abundant lipids on Earth and in the geologic record (Ourisson and Albrecht, 1992). BHPs are involved in various cell physiological processes (Welander et al., 2009; Sáenz, 2010; Doughty et al., 2011; Sáenz et al., 2012; Welander and Summons, 2012) and, due to their structural diversity, show potential as chemotaxonomic markers (Kusch and Rush, 2022). Functionalized BHPs were identified in sediment records that span 1.2 Myr (Talbot et al., 2014) as well as in Eocene Cobham Lignite (ca. 56 Ma; Talbot et al., 2016), highlighting their preservation potential as biomarkers in sedimentary records.
Recent studies and advancements in BHP analysis highlight the wide distribution and diversity of BHPs in both marine and terrestrial environments (Talbot and Farrimond, 2007; Pearson et al., 2009; Sáenz et al., 2011; Kusch et al., 2019; Hopmans et al., 2021). Bacteriohopanetetrol (BHT), BHT cyclitol ether (CE), and aminotriol, for instance, are ubiquitous in environmental samples and culture studies and are not associated with any specific organisms or environments (e.g., Talbot et al., 2003; Talbot and Farrimond, 2007; Zhu et al., 2011). Nucleoside BHPs (formerly known as adenosylhopanes) are typically associated with soils and are used to trace soil inputs to riverine and marine environments using a ratio of nucleosides to the more ubiquitous BHT, known as the Rsoil index (Taylor and Harvey, 2011; Zhu et al., 2011; De Jonge et al., 2016; Kusch et al., 2019). Recent studies, however, show that certain nucleoside BHPs can also be produced in the marine environment under low-oxygen conditions, complicating interpretations of the Rsoil index (Kusch et al., 2021b). Hopanoids methylated at the C-2 position are degradation products of 2-methylated BHPs (e.g., 2MeBHT) and are often considered diagnostic for cyanobacteria in the geologic record (Summons et al., 1999). 2Me-BHPs are produced by cyanobacteria (Talbot et al., 2008); however, the gene responsible for BHP methylation at the C-2 position was identified in a wide range of other bacterial phyla (Welander et al., 2010), suggesting multiple biological sources. This highlights the complexity of BHPs in the environment and the need for more studies to evaluate the potential of BHPs as biomarkers for specific bacterial groups.
Aerobic methane-oxidizing bacteria (MOB) regulate methane emissions in seasonally stratified lakes (Bastviken et al., 2008; Oswald et al., 2015; Guggenheim et al., 2020) and meromictic lakes (Oswald et al., 2016) by oxidizing methane before it reaches the atmosphere. MOB in lacustrine environments typically fall within the two phylogenetic groups in the phylum Proteobacteria: type I (members of Gammaproteobacteria) and type II (members of Alphaproteobacteria; Hanson and Hanson, 1996). They are known to produce a wide range of potentially diagnostic BHPs (Rohmer et al., 1984), including aminotetrol and aminopentol (Neunlist and Rohmer, 1985b, c; Cvejic et al., 2000; Talbot et al., 2001; van Winden et al., 2012), the recently identified methylcarbamate-aminoBHPs (Rush et al., 2016), and related 3β-methylated BHPs (Neunlist and Rohmer, 1985b; Zundel and Rohmer, 1985; Cvejic et al., 2000). Type I methanotrophs are typically associated with increased production of aminopentol, whereas type II methanotrophs are distinguished by the production of aminotetrol (Neunlist and Rohmer, 1985b, c; Cvejic et al., 2000; Talbot et al., 2001; van Winden et al., 2012). However, recent environmental studies, particularly in marine environments, suggest that this distinction is not as clear as previously proposed (Rush et al., 2016; Kusch and Rush, 2022). In addition, minor amounts of aminotetrol and aminopentol are also produced by sulfur-reducing bacteria and methylcarbamate-aminotriol was found in cultures of nitrite-oxidizing bacteria (Blumenberg et al., 2006; Elling et al., 2022). Few studies have evaluated the BHP composition of lacustrine methanotrophs or the utility of these biomarkers for tracing MOB in lakes.
In addition to their potential as taxonomic markers, BHP relative abundance was observed to change under certain environmental conditions. For instance, microcosm and mesocosm experiments that enriched for methanotrophs demonstrate that increasing temperatures led to increased concentrations of aminotriol, aminotetrol, and aminopentol (Osborne et al., 2017; van Winden et al., 2020), whereas a decrease in the relative abundance of unsaturated hopanoids was observed in culture experiments (Bale et al., 2019). Shifts in BHP compositions were also used to interpret changes in salinity (Coolen et al., 2008), redox conditions (Blumenberg et al., 2013; Matys et al., 2017; Rush et al., 2019; Zindorf et al., 2020), and soil inputs (Blumenberg et al., 2013) in sediment records. BHPs and BHP producers are particularly diverse in lakes (Farrimond et al., 2000; Watson and Farrimond, 2000; Talbot et al., 2003; Talbot and Farrimond, 2007; O'Beirne et al., 2022), yet few studies have associated BHPs with specific lacustrine settings.
The purpose of this study is to characterize the distribution of BHPs in lakes and coastal lagoons with the aim of identifying BHPs that are specific to lacustrine bacteria, with a focus on MOB and/or specific ecological niches, and of testing whether nucleoside BHPs are produced in situ in lakes. We also analyzed BHPs from a methanotroph–methylotroph co-culture enriched from a lake water column to identify potential novel BHPs related to lacustrine methanotrophy. We collected and analyzed sediment and water column suspended particulate matter (SPM) from eight lakes and two coastal lagoons on three different islands in the Azores Archipelago. The Azores Archipelago consists of 9 islands with 88 lakes that occur in either topographically depressed areas or volcanic depressions and range from severely impacted by humans to relatively “pristine” (Pereira et al., 2014). The diversity in lake types and comparison with marine-influenced sites, i.e., the coastal lagoons, in this study provide an ideal setting to investigate the partitioning of BHPs in the environment and their potential as biomarkers.
2.1 Sample collection
Samples were collected in lakes and lagoons on three different islands in the Azores Archipelago in June 2018 (Fig. 1 and Table 1): São Miguel (lakes Azul, Verde, and Empadadas), São Jorge (Lake São Jorge and lagoons Cubres East and Cubres West), and Flores (lakes Funda, Lomba, and Negra). June is considered part of the dry season in the Azores, which ranges from April to August. From September to March, the Azores Archipelago receives more rain and is exposed to high winds (Santos et al., 2004; Hernández et al., 2016). During the summer months, deeper lakes such as Azul, Verde, Funda, and Negra stratify and the bottom water becomes suboxic and, in some years, even fully anoxic (Gonçalves, 2008; Gonçalves et al., 2018). In contrast, shallow lakes are typically considered polymictic but might undergo short periods of stagnation during the summer months (Gonçalves, 2008). The lakes sampled for this study are either mesotrophic or eutrophic and range in water column depth from 2 to 115 m. During the summer months, lakes Verde and Funda experience large cyanobacterial blooms (Cordeiro et al., 2020). Both lakes Verde and Funda were experiencing a cyanobacterial bloom at the time of sampling.
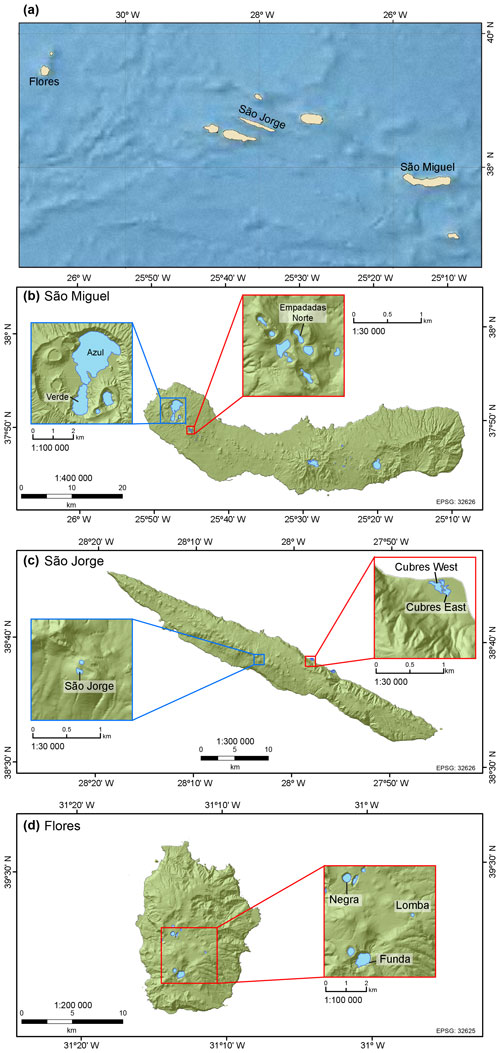
Figure 1(a) The Azores Archipelago with the location of lakes and coastal sites discussed in this study. Our study sites include (b) lakes Azul, Verde, and Empadadas Norte on São Miguel Island; (c) Lake São Jorge and coastal lagoons (i.e., Cubres East and Cubres West) on São Jorge Island; and (d) lakes Negra, Lomba, and Funda on Flores Island (maps generated in ArcGIS 10.3).
Table 1Location and characteristics of lakes and lagoons discussed in this study. The annual average water column properties are based on long-term monitoring data from 2003–2017 (Universidade dos Açores monitoring program). TP: total phosphorous, TN: total nitrogen.
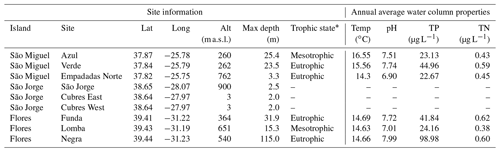
* Universidade dos Açores monitoring program (2003–2017) and Cordeiro et al. (2020).
Suspended particulate matter was collected from the surface water, near the oxycline and/or chemocline at the time of sampling and from the bottom water of the lake without disturbing the sediment–water interface. Water (5 to 10 L) was immediately filtered through Whatman GF/F 142 mm 0.7 µm pore size filters and frozen in liquid nitrogen. Surface sediment samples (0–1 cm) were also obtained at the same time using a gravity corer (UWITEC 90 mm, Austria). In Azul and Verde, surface sediments were collected near the lakeshore and in the deepest part of the lake. Replicate samples were collected for sediment cores from lakes Azul (n=2), Empadadas (n=4), and Negra (n=2) and analyzed for reproducibility. All samples were immediately frozen in a liquid nitrogen dewar and transported to the NIOZ Royal Netherlands Institute for Sea Research, where SPM and sediment samples were stored at −80 and −40 ∘C, respectively, until analysis. At the time of sample collection, water column profiles were obtained using a Hydrolab (Horiba U-50) for dissolved oxygen content (percent saturation and mg L−1), temperature, salinity, conductivity, pH, and turbidity (NTU, nephelometric turbidity unit). Additional long-term data were obtained from the Universidade dos Açores long-term monitoring program (data from 2003–2020).
2.2 Cultivation of a methanotroph–methylotroph co-culture
An enrichment co-culture consisting of a methanotroph (43 % Methylobacter sp.) and a methylotroph (21 % Methylotenera sp.) was previously isolated from a seasonally stratified and hypereutrophic lake (Lacamas Lake, WA, USA; van Grinsven et al., 2020). This culture was grown in triplicate under oxic conditions in the dark at 15 ∘C on a nitrate mineral salt (NMS) medium (Whittenbury et al., 1970). For lipid extractions, the cultures were grown on 250 mL NMS media in 580 mL acid-washed and autoclaved glass pressure bottles with butyl rubber stoppers with an addition of methane (16 mL, 99.99 % pure) to the headspace. After the methane gas was consumed, the cultures were filtered onto muffled Whatman GF/F 47 mm 0.3 µm pore size filters and frozen at −80 ∘C. The methylotroph identified in the co-culture is closely related to Methylotenera versatilis and Methylotenera mobilis (van Grinsven et al., 2020). Therefore, we obtained freeze-dried biomass of Methylotenera mobilis (DSM 17540) from the Deutsche Sammlung von Mikroorganismen und Zellkulturen (DSMZ) culture collection to investigate the presence of BHPs in this methylotroph.
2.3 Lipid extraction
All samples were freeze-dried and extracted using a modified Bligh–Dyer method (Bligh and Dyer, 1959; Bale et al., 2021). Samples were submerged in methanol (MeOH), dicholoromethane (DCM), and phosphate buffer (, ) and ultrasonically extracted twice. The solvent was collected in a separate flask after each extraction. DCM and phosphate buffer were added to the resulting solvent to obtain a new volume ratio of (). The DCM layer was collected, and the aqueous layer was washed two more times with DCM. The extraction was repeated using MeOH : DCM : aqueous trichloroacetic acid solution (, ) following the same procedure described above. The combined DCM layers were dried under N2 gas and stored at −20 ∘C until analysis. Prior to analysis, deuterated diacylglyceryltrimethylhomoserine (DGTS D-9; Avanti® Polar Lipids, USA) was added to the extracts as an internal standard, and the samples were redissolved in MeOH:DCM (9:1, v:v) before filtering the samples through a 0.45 µm regenerated cellulose syringe filter (4 mm diameter; Grace Alltech, Deerfield, IL).
2.4 UHPLC–HRMS analysis
Samples were analyzed following the methods described in Hopmans et al. (2021). Briefly, samples were analyzed on an Agilent 1290 Infinity I UHPLC (ultra-high-performance liquid chromatography) coupled to a Quadrupole-Orbitrap HRMS (high-resolution mass spectrometry) (Q Exactive, Thermo Fisher Scientific, Waltham, MA) equipped with an Ion Max source and heated electrospray ionization (HESI) probe (Thermo Fisher Scientific, Waltham, MA). Separation was achieved on an ACQUITY BEH C18 column (2.1×150 mm, 1.7 µm particle; Waters) and precolumn. The solvent system consisted of (A) MeOH : H2O (85:15) and (B) MeOH : isopropanol (1:1) with both containing 0.12 % () formic acid and 0.04 % () aqueous ammonia. Lipids were detected using positive ion monitoring of 350–2000 (resolution of 70 000 ppm at 200) with an inclusion list of calculated exact masses of BHPs: 171 for sediment samples and 357 for water column samples. Note that the difference in the number of exact masses in the inclusion lists reflects the addition of further novel BHPs after their identification. A Lake Verde sediment sample (Verde SS1) that included all the novel BHPs was rerun with an updated inclusion list (421 exact masses) for confirmation of novel BHPs. For untargeted BHP detection and identification, we used data-dependent MS2 (tandem mass spectrometry) (isolation window of 1 ; resolution of 17 500 ppm at 200) of the 10 most abundant ions for a total cycle of ca. 1.2 s and dynamic exclusion (6 s) with a 3 ppm mass tolerance. To obtain optimal fragmentation of BHPs, we used a stepped normalized collision energy of 22.5 and 40. Mass calibration was performed every 48 h using a Thermo Scientific Pierce LTQ Velos ESI Positive Ion Calibration Solution.
BHPs were identified based on their retention time, exact mass, and fragmentation spectra. Integrations were performed on (summed) mass chromatograms (within 3 ppm of mass accuracy) of relevant molecular ions ([M + H]+, [M + NH4]+, and [M + Na]+). We used an internal standard for normalization between sample runs by calculating the average peak area of the internal standard and correcting the peak areas to the average internal standard. However, we do not have an internal standard for BHP quantification; therefore all BHPs are reported as response units (RU). BHPs identified in water column and surface sediment samples are normalized to liters of water filtered (L) and grams of freeze-dried sediment (g), respectively.
2.5 Elemental analysis
Total organic carbon (TOC) and total nitrogen (TN) content was measured for the surface sediments (n=18) as described in Mitrović et al. (2023). Briefly, the samples were decalcified, powdered, and freeze-dried prior to analysis. All samples were analyzed using an Elementar vario ISOTOPE cube coupled to an isotope ratio mass spectrometer (IRMS) Elementar ISOPRIME visION. Prior to TOC analysis, carbonates were removed by pretreating the samples with excess 2 M hydrochloric acid (HCl) on a shaker overnight. The resulting samples were neutralized with bidistilled water and freeze-dried. All TOC samples were measured in duplicate with a reproducibility of <0.5 %.
δ13C and δ15N values of bulk organic matter were measured on decalcified samples of surface sediments (n=18) using an Elementar analyzer (Elementar vario ISOTOPE cube) coupled to an IRMS (Elementar ISOPRIME visION). Stable isotope ratios of 13C and 15N are expressed using the δ notation in units per mil, reported relative to Vienna Pee Dee Belemnite (VPDB) and atmospheric N2, respectively. The results were normalized to certified standards, and standard deviations were < 0.05 ‰.
2.6 Rsoil index
A soil index (Rsoil) was developed to trace inputs of terrestrially derived BHPs into the marine environment (Zhu et al., 2011). This index relies on the observations that BHT is present in higher abundance relative to nucleosides in the marine environment than in the soils (Pearson et al., 2009; Rethemeyer et al., 2010; Zhu et al., 2011). The index is calculated as follows:
Previously, “soil-marker BHPs” included only adenosylhopane, N1-methylinosylhopane (i.e., type-2 adenosylhopane after Hopmans et al., 2021), adenosylhopaneHG-Me (i.e., type-3 adenosylhopane after Hopmans et al., 2021), and their ring-methylated counterparts. BHT in the Rsoil equation only refers to the isomer with 17, 21(H), 22R, 32R, 33R, 34S stereochemistry. Previous high-latitude studies have revised this index, , to exclude methylated nucleosides (Doðrul Selver et al., 2012):
In this study, we tested both of these indices at our sample sites.
2.7 Data visualization and statistical analyses
Non-metric multidimensional scaling (NMDS) analysis was conducted using a Bray–Curtis dissimilarity matrix to visualize the similarities between BHP compositions at our sample sites. All analyses were performed and visualized in R (version R-4.2.1; R Core Team, 2022) using the vegan package (version 2.5–7; Oksanen et al., 2020) and ggplot2 (version 3.3.5; Wickham, 2016). NMDS plots were generated for both the water column and sediment samples. To test whether there was a significant difference between our sample sites and sample site types (for sediment samples: deep lakes, shallow lakes, and coastal environments; for water column: surface water, chemocline, and bottom water), we performed analysis of similarities (ANOSIM) tests in R using the vegan package for the sediment samples and water column samples. A Bray–Curtis dissimilarity matrix was used for these tests.
3.1 Diversity of BHPs in lake surface sediments and water columns
At our study sites, we identified 83 BHPs in total (Table A1). For the identification of novel BHPs, we discuss the carbon positions, rings, and modifications to the side chain of the core BHP structure as shown in Fig. B1. The novel BHPs are briefly discussed here and described in more detail in Appendix B. In the Azorean lakes, BHPs were more diverse in surface sediments (average of 51 BHPs per sediment sample) than in the water column SPM (average of 11 BHPs per SPM; Fig. 2).
Nucleoside BHPs were particularly diverse in the water column SPM and surface sediments in lakes Empadadas, São Jorge, and Lomba. We identified 14 nucleoside BHPs, including 3 inosylhopanes, described by Hopmans et al. (2021; Appendix B; Figs. B2, B3). Further, we identified five additional adenosyl-BHP isomers (Fig. B2), including early-eluting isomers (peaks b*, f*, g*, and k*). We also detected four additional inosylhopane isomers in surface sediments from Lake Empadadas with modifications in the position of the methyl group on the ring structure and/or head group (Fig. B3, peaks b*, c*, f*, and g*).
BHT (17, 21(H), 22R, 32R, 33R, 34S stereochemistry) was predominant in most samples, along with unsaturated and methylated versions of BHT. In the surface sediment from the coastal lagoons, Cubres East and Cubres West, we identified two compounds comparable to BHT; however, in the MS2 spectrum we observe the loss a methoxy moiety (32 Da (dalton), CH3OH), as well as three hydroxyl moieties (Appendix B2, Fig. B4). We tentatively identify these compounds as methoxylated BHTs. BHT cyclitol ether (CE) was also abundant in both water column SPM and surface sediments (Fig. 2). In addition, we detected MeBHT-CE in surface sediments from Lake Verde, as well as an isomer where, based on the MS2 spectrum, the methylation occurs on the cyclitol ether head group (Appendix B3, Fig. B5). We putatively identify this isomer as BHT-MeCE. BHpentol, BHpentol-CE, BHhexol, and BHhexol-CE were also identified at our sites but only in the lake surface sediments.
Aminotriol, as well as isomers of aminotriol and unsaturated aminotriol, was abundant in the water column SPM and surface sediment of the lakes (Fig. 2). Methylated and acylated versions of aminotriol were also identified but only in the surface sediment of the lakes. In contrast to a previous study in a hyper-euxinic and meromictic lake, we do not observe any diunsaturated aminotriols in the SPM or surface sediments at our study sites (O'Beirne et al., 2022).
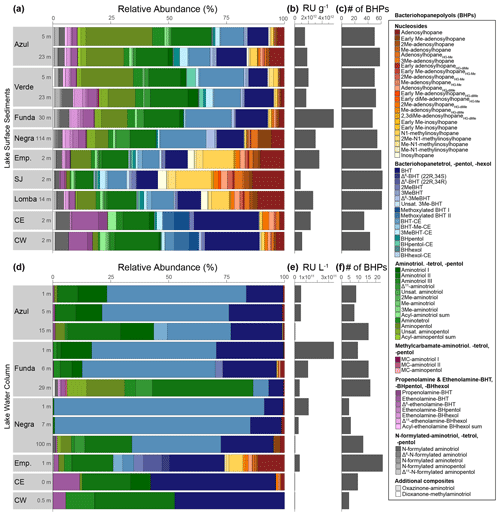
Figure 2(a) Relative abundance of all BHPs found in surface sediment samples labeled with the lake depth (meters) at which they were collected (all raw data are available in the Supplement). Emp: Empadadas, SJ: São Jorge, CE: Cubres East, CW: Cubres West. (b) The response units (RUs) of BHPs in the sediment samples normalized to grams of dry sediment and (c) the number of BHPs identified in each sample. (d) Relative abundance of BHPs identified in water column samples from the different lakes and lagoons with (e) the total response units per liters of water filtered and (f) the number of BHPs shown.
Aminotetrol and aminopentol, compounds commonly associated with methanotrophic activity (Neunlist and Rohmer, 1985b; Zundel and Rohmer, 1985; Cvejic et al., 2000), were found in almost all of the water column SPM and surface sediments. However, acylated versions of aminopentol (C14:0, C15:0, and C16:0) were only observed in the surface sediments of Azul and Verde. Two isomers of methylcarbamate-aminotriol (MC-aminotriol) and MC-aminopentol, compounds also associated with methanotrophs, were identified in water column SPM and surface sediment after Rush et al. (2016). The recently described propenolamine-BHT, ethenolamine-BHT, ethenolamine-BHpentol, and ethenolamine-BHhexol (Fig. 3) were identified in our samples after Hopmans et al. (2021). In addition, we putatively identified unsaturated versions of ethenolamine-BHT (Appendix B4, Fig. B6) and ethenolamine-BHhexol (Fig. 3e) with the double bond likely occurring at the Δ6 and Δ11 positions, respectively, based on the retention times. Acylated versions of ethenolamine-BHhexol (C15:0, C16:0, and C17:0) were also observed in lake surface sediments.
In sediment from Lake Verde (Fig. 3, peaks j and m), we identified several unknown composite BHPs previously described in Hopmans et al. (2021) with an assigned elemental composition (AEC) of C36H64O4N+ ( 574.483) and C36H64O5N+ ( 590.483), but no structure was assigned. In addition, we identified the same compound but with an additional hydroxy moiety (Fig. 3, peak o). We propose that these novel composite BHPs are a series of N-formylated aminoBHPs: N-formylated aminotriol (peak j), N-formylated aminotetrol (peak m), and N-formylated aminopentol (peak o). The proposed structure for N-formylated aminopentol is shown in Fig. 3f with the diagnostic fragmentation indicated. In addition, we observe unsaturated versions of N-formylated aminotriol (Fig. 3b and Appendix B5, Fig. B7), N-formylated aminotetrol (based on the MS1 and retention time), and N-formylated aminopentol with the double bond likely occurring at the Δ6 position for N-formylated aminotriol and Δ11 position for N-formylated aminotetrol and N-formylated aminopentol. In the mass chromatogram for 572.467 (Fig. 3b) from the Lake Verde sediment, we also observe a later-eluting peak (C36H62O4N+; Fig. 3b and d, peak l). Based on the MS2 spectrum, we propose that this is the same compound identified by Elling et al. (2022) in nitrite-oxidizing bacteria. This compound is likely a cyclized form of N-formylated aminotriol, and we putatively identify it as oxazinone-aminotriol (Appendix B6).
In addition to the discussed Δ6-ethenolamine-BHT, we observed a later-eluting peak at 21.88 min (Fig. 3a, peak c) in the mass chromatogram of 586.483 (C37H64O4N+; Δ ppm 0.72). We propose that one of the functionalities is part of a cyclized structure and this BHP is a formylated aminotriol, where the formic acid is part of a cyclic structure and the terminal amino group is methylated. The proposed structure with key fragmentation is shown in Fig. 3c. We tentatively identify this compound as a dioxanone-methylaminotriol (Appendix B7).
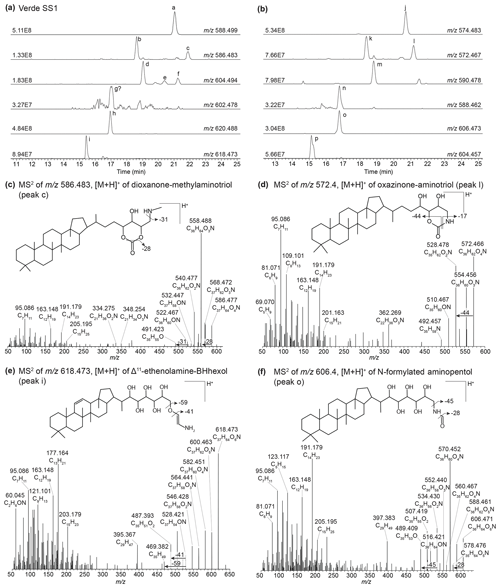
Figure 3(a) Partial mass chromatograms of ethenolamine-BHT (peak a), Δ6-ethenolamine-BHT (peak b), dioxanone-methylaminotriol (peak c), ethenolamine-BHpentol (peak d), isomers of methylcarbamate-aminotriol (peaks e and f), a potential peak for unsaturated ethenolamine-BHpentol (peak g), ethenolamine-BHhexol (peak h), and Δ11-ethenolamine-BHhexol (peak i). (b) Partial mass chromatograms of N-formylated aminotriol (peak j), Δ6-N-formylated aminotriol (peak k), oxazinone-aminotriol (peak l), N-formylated aminotetrol (peak m), Δ11-N-formylated aminotetrol (peak n), N-formylated aminopentol (peak o), and Δ11-N-formylated aminopentol (peak p). MS2 spectrum of (c) the dioxanone-methylaminotriol in 586.483 (peak c) and (d) the oxazinone-aminotriol composite (peak l) with tentative structures shown. MS2 spectrum of (e) [M + H]+Δ11-ethenolamine-BHhexol and (f) [M + H]+ N-formylated aminopentol with tentative structures shown. The chromatograms are from a surface sediment sample from Lake Verde.
3.2 Distribution of BHPs depends on environmental conditions in lakes and coastal lagoons
Based on the NMDS visualization, the more complex BHP distributions in the surface sediments allow for these sampling sites to be placed into three lake types (Fig. 4): deep lakes (i.e., Azul, Verde, Funda, and Negra; circles), shallow lakes (i.e., Empadadas, São Jorge, Lomba; squares), and coastal lagoons (i.e., Cubres East and Cubres West; stars). Using ANOSIM we find a significant difference both between the sample sites (R = 0.89, p<0.01) and the sample site types (R = 0.72, p<0.001). The variability between our sites, particularly in the surface sediments, is reflected in the overall differences in BHP distributions. In Azul and Verde surface sediments collected near the shore and in the deepest part of the lake show comparable BHP distributions. We also observe comparable BHP distributions in sample replicates for lakes Azul, Empadadas, and Negra; thus we will only discuss the average BHP values for the replicate sites. In the water column NMDS plot (Fig. 5) we observe the bottom water samples cluster closer together, whereas the samples from the surface water and chemocline overlap. Using the ANOSIM test, we find no significant difference in BHP distributions between the surface water, chemocline, and bottom water of these lakes (R = 0.04, p=0.32) or between the water columns of different sites (R = 0.06, p=0.35).
The deep lakes are monomictic and undergo stratification from June–October, with hypoxic and even anoxic conditions occurring during the summer months in the hypolimnion (Gonçalves, 2008; Raposeiro et al., 2018). These lakes contain a high abundance of BHT, BHT-CE, and aminotriol, particularly in the surface waters and sediment samples (Fig. 2 and Tables A3–A4). Previous studies that identified BHPs in lake sediments also described a high abundance of BHT, BHT-CE, and aminotriol (Farrimond et al., 2000; Talbot and Farrimond, 2007). Similarly, BHP distributions in the water column of a hypereutrophic, meromictic lake, Mahoney Lake (Canada), are dominated by BHT, unsaturated BHT, aminotriol, and BHT-CE in the surface waters and BHT, diunsaturated aminotriol, and aminotriol in the surface sediments (O'Beirne et al., 2022). In the Azores, the deep lakes (circles) are further distinguished by the presence of amino-containing BHPs in the surface sediments (Fig. 2a) that cluster together in the NMDS plot (Fig. 4), including aminotetrol, aminopentol, MC-aminopentol (green), N-formylated aminotetrol, N-formylated aminopentol (gray), ethenolamine-BHpentol, and ethenolamine-BHhexol (gold). This is also reflected in the BHP distributions of the bottom water SPM samples collected in June 2018 (Fig. 6), in which we observe amino-containing BHPs (i.e., aminopentol, ethenolamine-BHhexol, aminopentol, N-formylated aminopentol) in samples collected below the oxycline. A higher abundance of penta- and hexa-functionalized BHPs, including aminopentol, was previously described in eutrophic lakes relative to lakes with low primary productivity (Farrimond et al., 2000; Talbot and Farrimond, 2007).
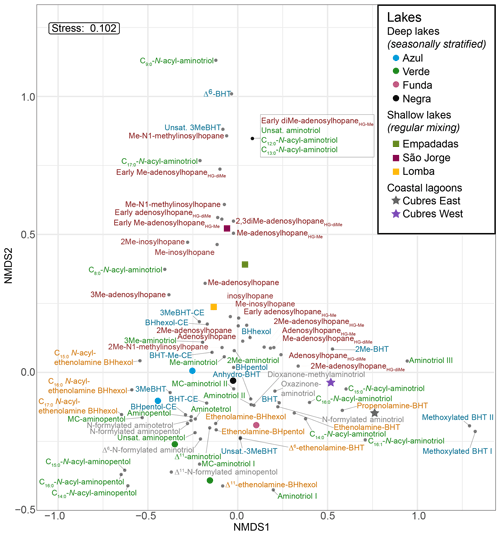
Figure 4NMDS visualization of BHP distributions in surface sediments from the deep lakes (circles), shallow lakes (squares), and lagoons (stars) analyzed in this study. The BHPs are colored by group as follows: nucleosides (brown), aminoBHPs (green), BHTs (blue), ethenolamine-BHPs (gold), and N-formylated aminoBHPs and additional novel BHPs (i.e., dioxanone-methylaminotriol and oxazinone-aminotriol; gray).
Although the shallower lakes might also undergo a period of stagnation, more regular mixing likely leads to oxygenation of the surface sediments. The shallow lakes are characterized by a higher abundance and diversity of nucleoside BHPs in both the surface sediment and water column SPM (Fig. 7). In addition, Me-inosylhopane, Me-N1-methylinosylhopane, and the early-eluting adenosylhopane type BHPs (brown) all cluster together near the shallow lakes, Empadadas, São Jorge, and Lomba (squares), in the NMDS plot (Fig. 4). Nucleoside BHPs (i.e., adenosylhopane, adenosylhopane type, and 2Me-adenosylhopane type) were previously reported in the sediment samples of two lakes, Loch Ness (Scotland) and Lake Nkunga (Kenya), that were associated with high levels of terrestrial input (Talbot and Farrimond, 2007). Loch Ness and Lake Nkunga also contained relatively high levels of BHT, aminotriol, BHT-CE, and even aminopentol (Talbot and Farrimond, 2007). Similar BHP distributions are observed in the sediments of Empadadas, São Jorge, and Lomba.
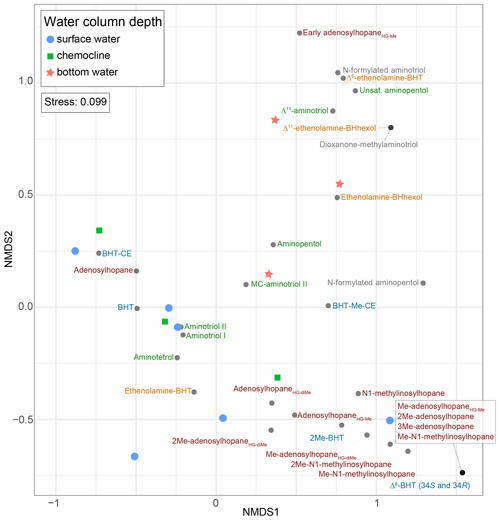
Figure 5NMDS visualization of BHP distributions between different water column samples from lakes Azul, Empadadas, Funda, and Negra, as well as Cubres East and Cubres West. Note that for Empadadas, Cubres East, and Cubres West we only have surface water column samples since the sampling locations were less than 3.5 m deep.
Finally, Cubres East and Cubres West are shallow, coastal lagoons that are influenced by both marine and freshwater inputs. Cubres East (salinity of 9.7 ppt) and Cubres West (salinity of 23.8 ppt) are defined by a high relative abundance of ethenolamine-BHT and propenolamine-BHT and by the presence of two methoxylated BHPs. In contrast to our freshwater sites, BHT-CE is mainly absent from the coastal lagoons (except for in the surface sediments of Cubres West). Similarly, in land-to-sea transects from the Yangtze (China) and Yenisei (Siberia) rivers, BHT-CE was more abundant in rivers, estuaries, and the coast relative to the open water (Zhu et al., 2011; De Jonge et al., 2016). Marine samples and fjord samples appear to predominantly consist of BHT and aminotriol (Farrimond et al., 2000; Zhu et al., 2011; Kusch et al., 2021a). In the estuary and coastal samples from the Yangtze River drainage basin, the BHP distributions consist of BHT, 2MeBHT, aminotriol, aminotetrol, aminopentol, nucleoside BHPs, and BHT-CE (Zhu et al., 2011). In general, we observe a similar distribution of BHPs in Cubres East and Cubres West.
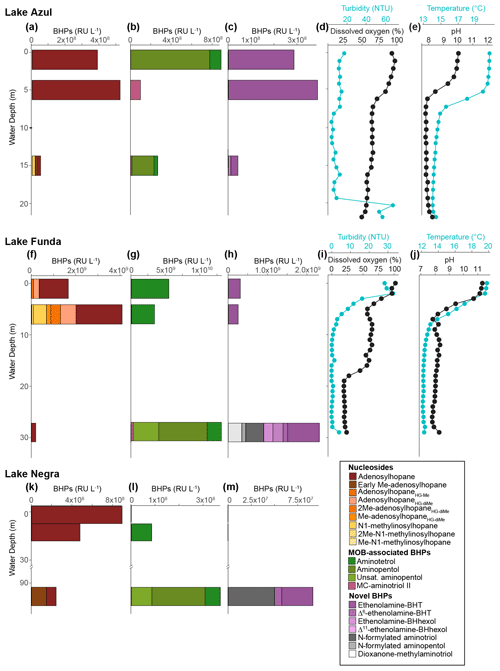
Figure 6Water column profiles for lakes Azul (a–e), Funda (f–j), and Negra (k–m). Panels (a), (f), and (k) show the response units per liter (RU L−1) of nucleoside BHPs identified in the water column. Panels (b), (g), and (l) show the response units per liter (RU L−1) of BHPs associated with methane-oxidizing bacteria found in the water column. Panels (c), (h), and (m) display the response units/liter (RU L−1) of ethenolamine-BHPs and recently identified composite BHPs (i.e., N-formylated aminoBHPs and dioxanone-methylaminotriol) identified in the water column. Panels (d) and (i) show the turbidity (blue) and dissolved oxygen (black) from the water column at the time of sampling in June 2018. Similarly, panels (e) and (j) display the temperature (blue) and pH (black) profiles of the water column at the time of sampling for both lakes Azul and Funda.
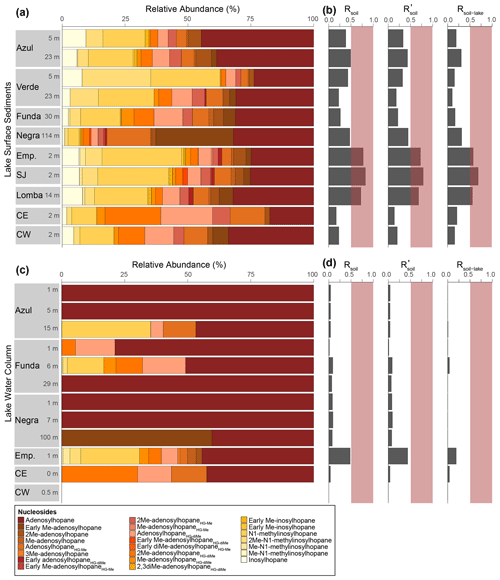
Figure 7(a) Relative abundance of nucleosides identified in the surface sediment samples for all Azorean lakes (Emp: Empadadas, SJ: São Jorge, CE: Cubres East, CW: Cubres West) with the (b) Rsoil (see Eq. 1), , and Rsoil-lake calculated for each sample. (c) The distribution of nucleosides in water column samples with the respective (d) Rsoil, , and Rsoil-lake values also shown. The red bars indicate the terrestrial end-member values. Note that nucleosides were not detected in the water column SPM of Cubres West.
Aminotriol and BHT were the most abundant BHPs at all of our sample sites. The presence of more diverse and abundant aminoBHPs in the deeper and seasonally stratified lakes allow us to distinguish them from the shallower lakes that contain a higher abundance of nucleoside BHPs. Finally, the lower diversity of BHPs and unique presence of methoxylated BHPs in Cubres East and Cubres West separates the marine influenced sites from the rest of the lakes in this study.
3.3 Diversity of BHPs in a methanotroph–methylotroph co-culture enriched from a lake water column
Previously, certain BHPs identified in MOB cultures were also detected in lake settings (Talbot et al., 2001; Talbot and Farrimond, 2007; Rush et al., 2016; Neunlist and Rohmer, 1985b, c; Cvejic et al., 2000; O'Beirne et al., 2022). However, neither methanotrophs nor lacustrine settings have been analyzed since recent methodological advancements have expanded BHP identification (Hopmans et al., 2021).
Here, we investigated the BHP composition of a lacustrine methanotroph–methylotroph (Methylobacter–Methylotenera) enrichment co-culture isolated from Lacamas Lake, WA, USA (van Grinsven et al., 2020). BHPs (i.e., aminotriol, 3Me-aminotriol, MC-aminotriol, aminotetrol, 3Me-aminotetrol, MC-aminotetrol, aminopentol, unsaturated aminopentol, 3Me-aminopentol, MC-aminopentol) have been previously detected in cultures of gammaproteobacterial type I MOB Methylobacter (Osborne, 2015; Rush et al., 2016). Our aim was to potentially identify novel BHPs from a lacustrine enrichment dominated by Methylobacter. This enrichment is also abundant in the methylotroph Methylotenera (van Grinsven et al., 2020). In order to rule out the synthesis of BHPs by this methylotroph, we investigated its genomic capacity to synthesize BHPs by searching for the key gene responsible for the formation of hopane and therefore BHP (i.e., squalene-hopene cyclase). A protein blast search (NCBI, National Center for Biotechnology Information) was performed with the sequence of the squalene-hopene cyclase gene of Bradyrhizobium japonicum as a query (accession no. WP_038942977.1), which did not lead to any hits in the genomes of Methylotenera species. In addition, we analyzed the BHP composition of Methylotenera mobilis, a strain closely related to the methylotroph also present in the co-culture, which was available in the DSMZ culture collection. Analysis of the M. mobilis biomass did not lead to the detection of BHPs. We acknowledge that other bacteria were also present in the enrichment culture (see van Grinsven et al., 2020); however, their BHP contribution is likely minor compared to the more abundant Methylobacter sp. present.
In the Methylobacter–Methylotenera co-culture we identified 22 BHPs (Table A4), with the most abundant being aminotriol (43 %) and aminopentol (43 %) (Fig. 8). Aminotetrol (6 %) was also observed, along with the unsaturated and acylated forms of aminotriol and aminopentol. Although present in low abundance, we identified MC-aminotriol, MC-aminopentol, ethenolamine-BHT, ethenolamine-BHhexol, N-formylated aminotriol, and N-formylated aminopentol (Fig. 8, Table A4). Low concentrations of nucleoside BHPs, i.e., adenosylhopane and 2Me-adenosylhopaneHG-diMe, were also present. Finally, we detected two novel BHPs ( 594; C35H64O6N+; Fig. 9), where the MS2 spectra show the consecutive loss of five hydroxyl moieties and an additional loss of 29 Da (CH3N). Based on the MS2 spectra and the elemental composition, we tentatively identify these compounds as aminohexol BHPs (Appendix B8). Notably, 3β-methylated BHPs are absent in the co-culture. Based on past culture studies, aminopentol and aminotetrol are associated with type I and type II MOB, respectively (Neunlist and Rohmer, 1985b, c; Cvejic et al., 2000; Talbot et al., 2001; van Winden et al., 2012). The high relative abundance of aminopentol relative to aminotetrol observed in the BHP distribution of the Methylobacter–Methylotenera co-culture analyzed in this study is similar to that of other type I MOB (Neunlist and Rohmer, 1985c; Talbot et al., 2001; van Winden et al., 2012; Rush et al., 2016; Kusch and Rush, 2022). The novel BHPs detected in the co-culture, however, are reported for the first time in this study. We conclude that the suite of BHPs identified in the co-culture, including the novel ethenolamine-BHPs, N-formylated aminoBHPs, and aminohexols, are attributed to the MOB Methylobacter present and thus can be potentially considered markers of aerobic methanotrophs in lake settings.
3.4 BHPs as biomarkers for aerobic methanotrophs in lakes
Aminotetrol is present in all of the Azorean samples, except for in the surface water of Negra (1 m depth), at 5 m depth in Azul, and in the water column and sediment of Cubres East (Fig. 2). Similarly, aminopentol is observed in the water column and sediment of Azul and Verde (except for at a depth of 5 m in the water column of Azul). In Funda and Negra, aminopentol only occurs in the deepest part of the water column and in the sediment samples. For Empadadas and Cubres East, aminopentol occurs both in the water column and the sediment, but it is only observed in the sediment sample from Cubres West. Similarly, aminopentol is found in both sediment samples from Lomba and São Jorge. Both aminopentol and aminotetrol are also produced in small amounts by sulfur-reducing bacteria (SRB; Blumenberg et al., 2006). As SRB also synthesize relatively higher amounts of aminotriol, ratios of aminopentol and aminotetrol to aminotriol have been used to determine the origin of the penta- and hexa-functionalized aminoBHPs (Blumenberg et al., 2006, 2009, 2012). The absence of anoxic conditions in the lake water column would rule out a major contribution of SRB-produced BHPs during the time of sampling. This is confirmed by the high aminopentol : aminotriol (water column of 0 (aminopentol absent) to 0.37 and sediment of 0.01 to 1.67) and aminotetrol : aminotriol (water column of 0 (aminotetrol absent) to 1.30 and sediment of 0 (aminotetrol absent) to 0.38) ratios in both water column and sediment samples. Recently two species of nitrite-oxidizing bacteria were shown to produce aminopentol in minor amounts under certain growth conditions (Elling et al., 2022), potentially contributing to the aminopentol observed in the surface waters of Azul, Empadadas, and Cubres East. However, in the oxygen-depleted bottom waters of Azul, Funda, and Negra, MOB are likely the primary producers of aminotetrol and aminopentol. A relatively higher abundance of aminopentol than aminotetrol in the water column and sediment samples would suggest a higher abundance of type I than type II MOB, in accordance with previous studies in both dimictic and meromictic lakes (Hanson and Hanson, 1996; Oswald et al., 2015, 2016; Guggenheim et al., 2020).
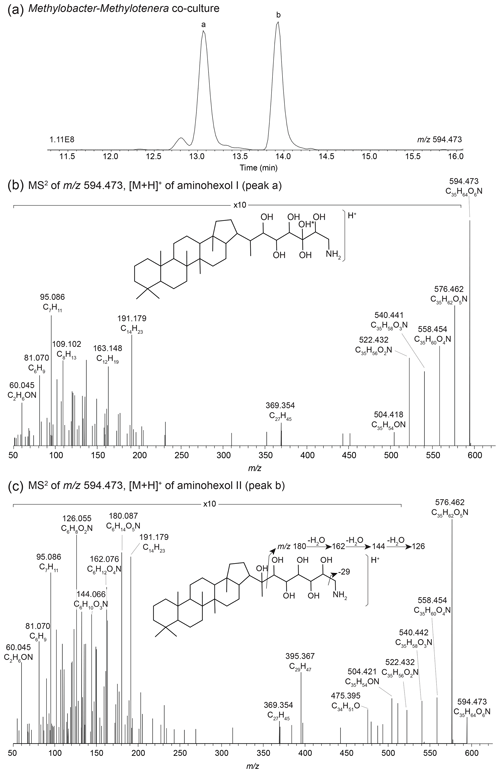
Figure 9(a) Partial mass chromatograms of aminohexol I (peak a) and II (peak b) BHPs from the Methylobacter–Methylotenera co-culture with MS2 spectra for (b) aminohexol I and (c) aminohexol II and the tentative structures shown. The asterisk (*) indicates arbitrary placement of the hydroxyl group on the side chain.
We observe unsaturated aminopentol (van Winden et al., 2012; Wagner et al., 2014) in the bottom water samples of lakes Funda and Negra (Fig. 6), which could be an adaptation by MOB to colder water temperatures (Bale et al., 2019). We also observe unsaturated aminotriol in the bottom water of Azul (15 m, 14 ∘C), Funda (29 m, 12 ∘C), and Negra (100 m, temperature data unavailable). C16:0N-acyl-aminopentol was reported in trace amounts near a terrestrial methane seep (Hopmans et al., 2021). C16:0N-acyl-aminopentol, as well as C14:0 and C15:0N-acyl-aminopentol, are present in sediment samples from Azul and Verde, whereas in Lomba and Empadadas we only observe C16:0N-acyl-aminopentol in the sediment (Fig. 2). In the NMDS plot of the surface sediments, the acylated aminopentols group closely with BHPs related to MOB, aminotetrol and aminopentol (Fig. 4). In addition, C14:0 and C16:0N-acyl-aminopentol are both detected in the Methylobacter–Methylotenera co-culture, confirming that MOB could be a potential source for these acyl-aminoBHPs in lakes Azul, Funda, Lomba, and Empadadas.
MC-aminotriol and MC-aminopentol were produced by the Methylobacter–Methylotenera co-culture (Fig. 8), albeit in low abundance. However, this is the first report of ethenolamine-BHT, ethenolamine-BHhexol, N-formylated aminotriol, and N-formylated aminopentol in culture. So far, ethenolamine-BHPs and N-formylated aminoBHPs were only identified near a terrestrial methane seep (Hopmans et al., 2021). Further environmental and culture studies are needed to identify whether these are robust biomarkers for methanotrophs. In all of our samples, except for the water column of Negra at 1 and 7 m depths (Fig. 2), we detected ethenolamine-BHPs, propenolamine, and/or N-formylated aminoBHPs (Hopmans et al., 2021). The widespread distribution of these compounds suggests they are derived from multiple producers. Although we cannot identify a direct source for these compounds, the NMDS analysis shows that ethenolamine-BHpentol, ethenolamine-BHhexol, acylated ethenolamine-BHhexols (C15:0, C16:0, C17:0), N-formylated aminotetrol, and N-formylated aminopentol cluster with MC-aminopentol, aminopentol, aminotetrol, and the acylated aminopentols near the surface sediments from Azul, Verde, Funda, and Negra (Fig. 4). In addition, ethenolamine-BHhexol and N-formylated aminopentol are detected in the bottom waters of Lake Funda (Fig. 6). Azul, Verde, Funda, and Negra all stratify during the summer months, and the bottom water in these lakes ranges from suboxic to anoxic conditions (Gonçalves et al., 2018). This would suggest that ethenolamine-BHpentol, ethenolamine-BHhexol, acylated ethenolamine-BHhexols, N-formylated aminotetrol, and N-formylated aminopentol are being produced under suboxic to anoxic conditions and/or could be associated with MOB.
BHPs with a 3β-methylation, particularly 3MeBHT and 3Me-aminotriol, are often linked to methane oxidation (Neunlist and Rohmer, 1985b; Zundel and Rohmer, 1985; Cvejic et al., 2000). However, 3β-methyl-BHPs are not exclusively produced by MOB (Zundel and Rohmer, 1985; Welander and Summons, 2012). BHPs with a 3β-methylation are also produced by other bacteria in the phyla Proteobacteria (classes Alpha-, Beta-, and Gammaproteobacteria), Cyanobacteria, Acidobacteriota, and Nitrospirota (Zundel and Rohmer, 1985; Sinninghe Damsté et al., 2017; Elling et al., 2022). In addition, 3β-methylation is crucial for bacterial cell survival in the late stationary phase and thus could have a broad taxonomic distribution (Welander and Summons, 2012). We observe 3MeBHT in all sediment samples except for Cubres East and Cubres West (Fig. 2); however, it was not detected in our water column samples. In contrast, 3Me-aminotriol only occurs in sediment samples from Lomba, Cubres West, São Jorge, Empadadas, Verde, and Azul. The absence of 3Me-BHPs in SPM samples suggests that at the time of sampling the bacteria responsible for 3β-methylation were not abundant in the water column. Future work will need to evaluate annual changes in both BHP distributions and bacterial communities in the water column to confirm the primary producers of 3Me-BHPs in lakes.
AminoBHPs with a methylcarbamate terminal group (MC-aminoBHPs) were proposed as biomarkers for type I MOB in marine settings based on culture samples (Rush et al., 2016), but, so far, the occurrence of MC-aminoBHPs in lakes has not been evaluated. MC-aminotriol has been observed in nitrite-oxidizing bacteria (Elling et al., 2022). The highest rates of methane oxidation in seasonally stratified lakes typically occurs at or directly below the chemocline (Rudd et al., 1976; Hanson, 1980; Harrits and Hanson, 1980; Oswald et al., 2015). In the Azorean lakes, MC-aminotriol was found in the oxycline and bottom water of both lakes Azul and Funda (Fig. 6). In Azul and Funda we observe MC-aminotriol near the oxycline, which could also be attributed to nitrite-oxidizing bacteria (Elling et al., 2022). We also observe MC-aminotriol in sediment samples from Verde, Negra, São Jorge, Lomba, Cubres East, and Empadadas. The co-occurrence of MC-aminopentol with 3MeBHT and/or 3Me-aminotriol in sediment samples from Verde, Negra, Lomba, and Empadadas could indicate a common MOB source. Both Lomba and Empadadas are relatively shallow lakes with a fully oxygenated water column, which means the primary site of methane oxidation occurs in the surface sediment and not in the water column (Hanson and Hanson, 1996). The different MC-aminoBHP distributions in the sediment samples could indicate that we were either not sampling during the peak period of methane oxidation in the lake, there was a shift in the MOB community, or methane oxidation was primarily occurring at the sediment surface.
Based on the distributions of aminoBHPs and MC-aminoBHPs, MOB are likely present, albeit in low abundance, at all Azorean sites. In the deeper lakes (i.e., Azul, Funda, and Negra), methane oxidation is likely occurring either at or below the chemocline, which would account for the diverse aminoBHPs observed in the water column (Fig. 6). Low δ13C values in bulk sediment samples from Lake Funda and primarily reducing conditions associated with the bottom water of the lake would suggest that methanogenesis is occurring in the bottom water and/or surface sediment of Lake Funda during the summer months (Richter et al., 2022). Similarly, proxy records suggest that eutrophication in Lake Azul contributed to hypoxic conditions in the bottom water (Raposeiro et al., 2017). In both lakes Funda and Azul, an increase in organic matter deposition combined with low-oxygen conditions in the bottom water during the summer months would support methane production and, therefore, a community of methane-oxidizing bacteria in the water column of these lakes. A similar distribution of BHPs associated with methane-oxidizing bacteria (i.e., aminopentol, unsaturated aminopentol, and aminotetrol) is found in high abundance in the methane-rich environments of floodplains in the Amazon basin and wetlands in the Congo River basin (Wagner et al., 2014; Spencer-Jones et al., 2015). In contrast, in the shallow lakes and the coastal lagoons methane oxidation might occur both in the water column and in the oxygenated sediment. Further molecular and culture studies, however, are needed to validate these conclusions.
3.5 Autochthonous vs. allochthonous source of nucleoside BHPs
A recent study suggests that some nucleoside BHPs may be in situ produced in the chemocline or under anoxic/euxinic conditions in marine water columns (Kusch et al., 2021b). To the best of our knowledge, no studies have investigated nucleoside BHP production in lacustrine environments. Nucleoside BHPs were detected in all water column and sediment samples except the water column of the lagoon Cubres West (salinity of 23.8 ppt; Fig. 7). The distribution of nucleoside BHPs in the sediment of Lake Empadadas is reflected in the BHP distribution of its surface water, suggesting that in shallow, well-mixed lakes nucleoside BHPs like adenosylhopane and N1-methylinosylhopane are either derived from production in the water column or surface water run-off (Cooke et al., 2008; Xu et al., 2009; Rethemeyer et al., 2010). In contrast, in Lake Funda we observe an increase in adenosylhopane, adensylhopaneHG-diMe, 2Me-adenosylhopaneHG-diMe, Me-adenosylhopaneHG-diMe, N1-methylinosylhopane, 2Me-N1-methylinosylhopane, and Me-N1-methylinosylhopane at the chemocline (6 m depth). Of these nucleoside BHPs, only adenosylhopane, adenosylhopaneHG-diMe, and 2Me-adenosylhopaneHG-diMe are present in the surface water (1 m) of Lake Funda. A pycnocline associated with the chemocline in the Azorean lakes could result in the accumulation of adenosylhopane, adenosylhopaneHG-diMe, and 2Me-adenosylhopaneHG-diMe; however, the lack of Me-adenosylhopaneHG-diMe, N1-methylinosylhopane, 2Me-N1-methylinosylhopane, and Me-N1-methylinosylhopane in the surface water of Lake Funda could also indicate in situ production of these compounds in the water column. Kusch et al. (2021b) found that adenosylhopane, N1-methylinosylhopane, and 2Me-N1-methylinosylhopane were produced within the chemocline and under anoxic or euxinic conditions in marine settings. In addition, adenosylhopane is separate from the rest of the nucleosides in the NMDS plot of only water column samples (Fig. 5), falling in between samples collected near the chemocline and the surface water. N1-methylinosylhopane is also observed in the bottom water of Lake Azul, in addition to adenosylhopaneHG-Me and adenosylhopaneHG-diMe, suggesting that these nucleosides are likely produced in situ. The primary producers of nucleoside BHPs, however, remain unconstrained and are likely associated with a wide range of bacteria. Adenosylhopane, in particular, is produced as an intermediate product in BHP synthesis (Bradley et al., 2010) and has the potential to be sourced from diverse bacteria, including purple non-sulfur bacteria (Neunlist and Rohmer, 1985a; Neunlist et al., 1988; Talbot et al., 2007), nitrifying bacteria (Seemann et al., 1999; Talbot et al., 2007; Elling et al., 2022), and nitrogen-fixing bacteria (Bravo et al., 2001; Talbot et al., 2007).
3.6 Implications for the Rsoil proxy
The ratio of nucleoside BHPs to BHT (Rsoil index, Eq. 1; Zhu et al., 2011) and the revised soil index for high latitudes (, Eq. 2; Doðrul Selver et al., 2012) have previously been used as an indication of soil input to riverine and marine environments (Cooke et al., 2009; Sáenz et al., 2011; Taylor and Harvey, 2011; Zhu et al., 2011; Doðrul Selver et al., 2012, 2015; De Jonge et al., 2016; Kusch et al., 2019). However, the recent indications that nucleoside BHPs may be in situ produced in marine (Kusch et al., 2021b) and lacustrine water columns (this study) could affect the application of the Rsoil proxy. Rsoil values of marine and lacustrine sediments from the tropics to Arctic range from 0.4–0.9 (Cooke et al., 2008; Pearson et al., 2009; Rethemeyer et al., 2010; Kusch et al., 2021a), and values between 0.5–0.8 have been employed as a tentative terrestrial end-member (Zhu et al., 2011). Here we revise the Rsoil index to exclude nucleoside BHPs that might be produced in the lake water column (i.e., adenosylhopane, early-eluting adenosylhopaneHG-Me, Me-adenosylhopaneHG-diMe, N1-methylinosylhopane, 2Me-N1-methylinosylhopane, Me-N1-methylinosylhopane). We call this new index Rsoil-lake. This ratio is significantly correlated with Rsoil (R = 0.94, p<0.0001) and (R = 0.60, p<0.0001). Further, this ratio is positively correlated with TOC TN (R = 0.81, p<0.005; Fig. C1), where TOC TN values between 4 and 10 reflect organic matter derived from lake algae and values higher than 20 indicate an increased source of vascular land plants (Meyers, 2003). The low sediment TOC TN values (7 to 11) and isotopically depleted δ13C values (−31 ‰ to −24 ‰; Fig. C2a) indicate predominantly in situ derived organic matter, which is in accordance with the high levels of primary productivity observed in all of the lakes (Cordeiro et al., 2020; Meyers, 2003). Similarly, low δ15N values (−1 ‰ to 2 ‰; Fig. C2b) are commonly associated with high levels of nitrogen fixation in accordance with the cyanobacterial blooms observed in the lakes during the summer months (Cordeiro et al., 2020; Meyers, 2003). The slightly more enriched δ13C values (−19 ‰ to −15 ‰) in Cubres East and Cubres West are typical of marine algae, also suggesting that the organic matter is mainly derived from in situ production (Meyers, 1994).
At all of the sites, we observe lower Rsoil-lake values in the water column than the sediment. As our water column SPM samples were collected in the dry season in the Azores (April–August; Hernández et al., 2016), we would anticipate low terrestrial inputs. In contrast, the BHPs detected in the sediments represent an integrated annual signal and likely include a larger input of terrestrial material during the wet season (September–March; Hernández et al., 2016). The Rsoil-lake values found in the sediments of the deeper lakes (i.e., Funda, Negra, and Azul) range from 0.17 to 0.32 and similarly have TOC TN values between 8.1 and 8.3. The sediments of the shallow lakes all contain relatively higher Rsoil-lake values (i.e., Empadadas of 0.57, São Jorge of 0.69, and Lomba of 0.56) and higher TOC TN values (ranging from 10 to 11.4), which could indicate slightly higher inputs of terrestrial organic matter, particularly during the wet season. In the coastal lagoons Cubres East and Cubres West, we observe low Rsoil-lake values both in the water column (Cubres East of 0.04 and Cubres West of 0) and sediment samples (Cubres East of 0.21 and Cubres West of 0.16). This could reflect fewer terrestrial inputs relative to the other lakes in this study. Low TOC TN values (Cubres East of 7.08 and Cubres West of 7.22) would also indicate a primarily in situ source of organic matter (Meyers, 2003); however, further work is needed to verify this.
Our results highlight that caution should be used to ensure that the nucleoside BHPs included in the Rsoil proxy are not produced in situ. Our current dataset, the Rsoil-lake index, which excludes nucleoside BHPs that likely originate from the water column, appears to work for all of our Azorean sites; however additional work is needed to distinguish the primary source of nucleoside BHPs and to expand the application of Rsoil-lake. More detailed water column and catchment studies are needed to confirm whether these nucleoside BHPs are actually being produced within the lakes. Furthermore, similar comparison studies should be performed in marine settings.
This study highlights the diversity and complexity of BHP distributions and their interpretations in the lacustrine and coastal environments. We identified several novel BHPs that are being described for the first time in lacustrine and coastal settings, including unsaturated ethenolamine-BHPs, N-formylated aminoBHPs, oxazinone-aminotriol, and dioxanone-methylaminotriol. Further, we identified several nucleoside BHPs that appear to be produced in the water column of lacustrine settings; however, further work is needed to verify the source of these BHPs. We propose a revised Rsoil index, Rsoil-lake, to distinguish between terrestrially and aquatically derived organic matter in lakes, by excluding any nucleosides that might be produced in situ. Within lakes, aminotetrol, aminopentol, and MC-aminopentol show potential as proxies for MOB. In addition, the recently identified ethenolamine-BHT, ethenolamine-BHhexol, N-formylated aminotriol, and N-formylated aminopentol are produced by a lacustrine methanotroph, Methylobacter sp., and within the Azorean lakes are associated with low-oxygen conditions. Additional studies focused on describing BHP distributions particularly in lacustrine settings and in culture studies, however, are needed to confirm whether certain BHPs can be used as biomarkers for biogeochemical cycles or environmental conditions.
Table A1BHPs identified in this study with the retention time (tr), assigned elemental composition (AEC), calculated exact mass (Mcalc), and Δ ppm (Δ ppm = ((Mcalc–Mmeasured) Mcalc) × 106).
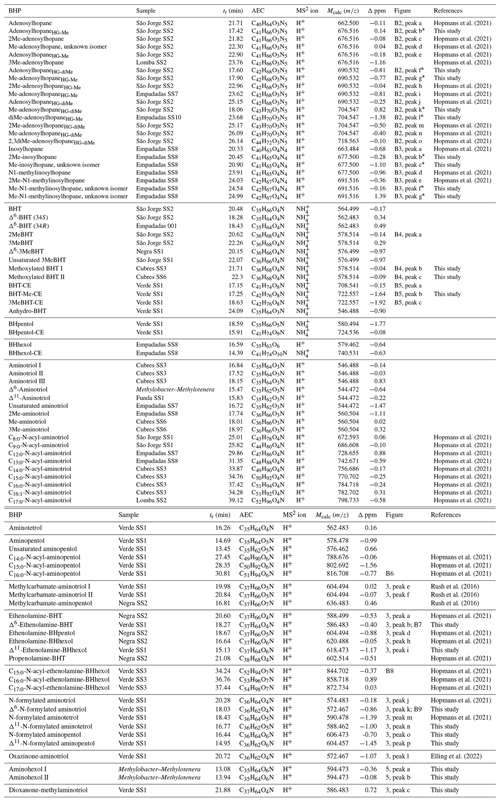
Table A2Water column samples and water column measurements from the time of sampling (June 2018) discussed in this study (D.O.: dissolved oxygen). The relative abundance of the major BHPs identified in each sample are also shown (BHT: bacteriohopanetetrol, BHT-CE: BHT cyclitol ether).
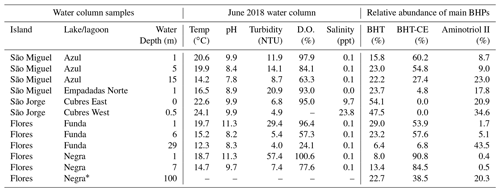
* Note that measurements in Lake Negra are only made up to ∼ 50 m water column depth.
Table A3Location and characteristics of the sediment sample sites discussed in this study (Z: maximum water depth, n: replicates, TN: total nitrogen, TOC: total organic carbon). The relative abundance of the major BHPs identified in each sample are also shown (Adeno.: adenosylhopane, N1-methylino.: N1-methylinosylhopane, BHT: bacteriohopanetetrol, and BHT-CE: BHT cyclitol ether).
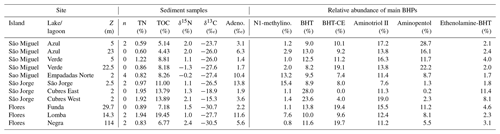
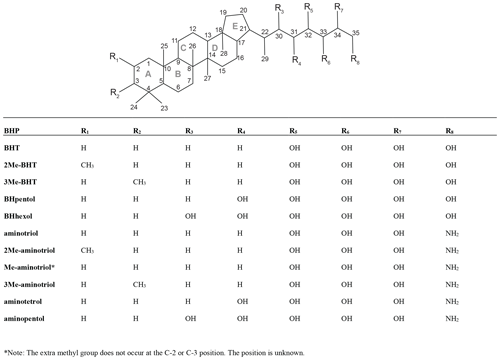
Figure B1Core structure of BHPs discussed in the text with the carbon positions and the rings labeled. More complex structures are shown in the figures.
B1 Novel nucleoside BHPs
We identified nucleoside BHPs based on Hopmans et al. (2021) in surface sediments and water column samples from the Azores Archipelago. Figure B2 shows the partial mass chromatograms of adenosyl-containing nucleoside BHPs with increasing degrees of methylation, found in surface sediment samples from São Jorge and Empadadas. In addition to the adenosyl-BHPs previously identified by Hopmans et al. (2021; peaks a, c–e, h–j, and m–o), we observe several additional peaks (b*, f*, g*, k*, and l*). In the mass chromatogram 676.516, an early-eluting peak (b*) occurs in both samples. The MS2 spectrum for peak b* contains one fragment ion related to the head group at 150.077 (C6H8N, Δ ppm −0.52), which suggests that the adenosyl head group is methylated. Therefore, we tentatively assign peak b* as early-eluting adenosylhopaneHG-Me (assigned elemental composition (AEC): C41H66O3N5, 676.516, Δ ppm 0.14; Fig. B2d). The mass chromatogram of 690.532, contains two early-eluting peaks in São Jorge (peaks f* and g*) and one early-eluting peak in Empadadas (peak g*). Peaks f* and g* are putatively identified as Me-adenosylhopaneHG-Me and adenosylhopaneHG-diMe based on the dominant fragment ions related to the head group at 150.077 (C6H8N, Δ ppm −0.52; Fig. B2e) and 164.093 (C7H10N, Δ ppm −1.09; Fig. B2f), respectively. In the sediment samples from Lake São Jorge, the mass chromatogram for 704.547 contains one early-eluting peak (peak k*). The MS2 spectrum of peak k* is characterized by a fragment at 164.093 (C7H10N, Δ ppm −0.78; Fig. B2g), which is associated with a dimethylated head group. Thus, we tentatively identify peak k* as Me-adenosylhopaneHG-diMe. In Empadadas, we observe another early-eluting peak (peak l*) in the mass chromatogram for 704.547 with an MS2 spectrum that contains two main mass fragments associated with the adenosyl head group: 150.077 (C6H8N, Δ ppm −0.85) and 164.093 (C7H10N, Δ ppm −1.27; Fig. B2h). Peak l* is likely another isomer of diMe-adenosylhopaneHG-Me but is co-eluting with Me-adenosylhopaneHG-Me (peak i) as suggested by the fragment 150.077 (C6H8N).
Inosylhopanes, described in Hopmans et al. (2021), were identified in surface sediments from Lake Empadadas (peaks a, d, and e; Fig. B3a), as well as several novel isomers of methylated inosylhopanes (peaks b*, c*, f*, and g*; Fig. B3a). The mass chromatogram of 691.516 contains two early-eluting peaks (peak b* and c*). Both mass spectra contain one primary fragment associated with the head group, 137.046 (C5H5ON, peak b*, Δ ppm −1.11, peak c*, Δ ppm −0.82; Fig. B3b), indicating an inosine head group. Thus, we identify these peaks as Me-inosylhopane with the methylation occurring on the core structure. The retention time difference between inosylhopane and peaks b* (0.12 min) and c* (0.57 min) is similar to the retention time difference between BHT and 2MeBHT (0.14 min) and MeBHT with a methylation at an unknown position in the ring system (0.58 min), respectively (Hopmans et al., 2021). Therefore, we tentatively assign peak b* as 2Me-inosylhopane and peak c* as Me-inosylhopane (unknown isomer). For peak f* we observe a fragment of 151.061 (C6H7ON, Δ ppm −1.14; Fig. B3c), suggesting a methylation on the inosine head group. We propose that peak f* is an isomer of methyl-N1-methylinosylhopane (elemental composition (EC): C42H67O4N4, 691.516). Based on the fragment 151.061 (C6H7ON, Δ ppm −1.08; Fig. B3d), peak g* is another isomer of Me-N1-methylinosylhopane with unknown positions of the methyl groups on the ring system and head group. However, we also observe an 164.093 (C7H10N, Δ ppm −1.15) fragment in the MS2 spectra of peak g*. We attribute this to a potential co-eluting peak that we are unable to distinguish.
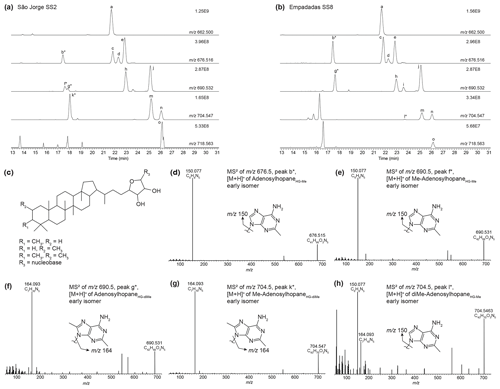
Figure B2Partial mass chromatograms of adenosyl-containing nucleoside BHPs in a sediment sample from (a) Lake São Jorge and (b) Lake Empadadas showing the exact mass and intensity of the highest peak in arbitrary units (AUs). Peaks identified as novel nucleoside BHP isomers are marked with an asterisk (*). (c) General structure of a nucleoside BHP. (d–h) MS2 of novel early-eluting nucleoside BHP isomers identified in Azorean lakes and a proposed structure for each nucleobase. Note that the mass spectra of (h) peak l* appear to be a mixed spectrum.
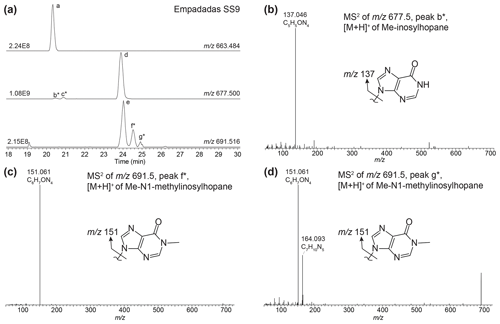
Figure B3(a) Partial mass chromatogram of inosylhopane (peak a), N1-methylinosylhopane (peak d), and 2-methyl-N1-methylinosylhopane (peak e) in a sediment sample from Lake Empadadas. (b) MS2 of a newly identified peak of putative Me-inosylhopane (peak b*) with the proposed structure for the nucleobase shown. MS2 of newly identified 2-methyl-N1-methylinosylhopane isomers for (c) peak f* and (d) peak g* with the structures of the proposed nucleobases. Note that the mass spectra for peak g* appear to be a mixed spectrum.
B2 Methoxylated bacteriohopanetetrol
In the mass chromatogram of 578.514 (C36H68O4N+) we identified two peaks that elute after the expected retention times of 2MeBHT (peak a) and 3MeBHT (Fig. B4a). The MS2 spectrum of peak b at 21.71 mins (Fig. B4b) shows a distinct loss of three hydroxyl moieties: 543.477 (C36H63O, Δ ppm −0.68), 525.467 (C36H61O, Δ ppm −0.48), and 507.459 (C36H59O+, Δ ppm −5.37) and a loss of 32 Da (CH3OH) from 525.466 (C36H61O, Δ ppm −0.48) to 493.441 (C35H57O+, Δ ppm −0.26). The lower mass range of the mass spectrum is comparable to that of BHT (Hopmans et al., 2021). We therefore tentatively identify this BHP as a methoxylated BHT. Peak c at 22.3 min shows a similar loss of three hydroxyl moieties and a loss of 32 Da. The later elution time of this peak could result from a different position of the methoxy group on the side chain. The position of the methoxy group in both isomers is unknown. We tentatively identify these peaks as methoxylated BHTs.
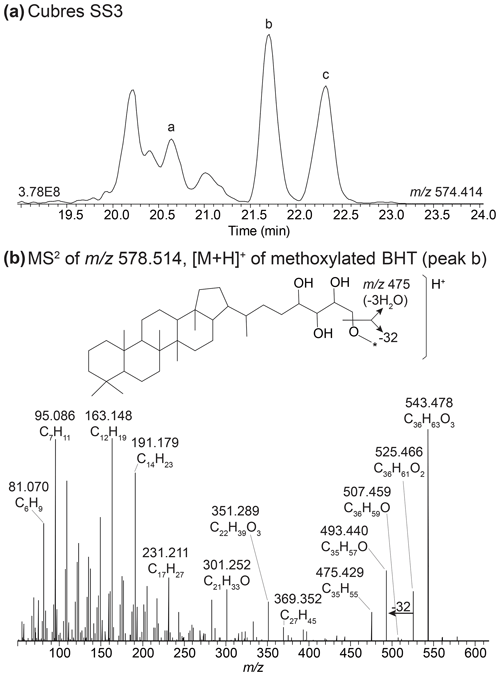
Figure B4(a) Partial mass chromatogram of 2MeBHT (peak a), methoxylated BHT I (peak b), and methoxylated BHT II in a sediment sample from Cubres East. (b) MS2 of the methoxylated BHT I (peak b) and the proposed structure for methoxylated BHT. The asterisk (*) indicates that the position of the methoxy moiety is unknown.
B3 Bacteriohopanetetrol-methyl cyclitol ether
In the mass chromatogram of 722.555, the calculated exact mass of MeBHT cyclitol ether (CE), from Verde SS1 we observe two peaks (Fig. B5a): a peak at 17.25 min (peak b) and 3MeBHT-CE (C42H76O8N+, peak c) at 18.63 min. The MS2 spectrum of peak b (Fig. B5b) shows the consecutive losses of two hydroxyl moieties producing fragment ions at 704.543 (C42H74O7N+, Δ ppm 3.85) and 686.529 (C42H72O6N+, Δ ppm 2.95); however, the expected loss of a third hydroxyl moiety (C42H70O5N+, 668.520) is not observed, likely due to the low intensity. In the lower mass range, the fragments follow the fragmentation pattern for a cyclitol ether head group described in Hopmans et al. (2021), but with an additional mass of 14 Da. The intact head group corresponds to the fragment 194.102 (C7H16O5N+, Δ ppm −0.31) followed by the loss of several hydroxyl moieties: 176.091 (C7H14O4N+, Δ ppm 1.05), 158.081 (C7H12O3N+, Δ ppm −2.47), and 140.070 (C7H10O2N+, Δ ppm 0.32). We also observe two fragments that correspond to the intact polar head group with two additional carbon atoms, originating from the side chain after fragmentation at the C-33 and C-34 bond: 236.113 (C9H18O6N+, Δ ppm 0.06) and 218.102 (C9H16O5N+, Δ ppm 0.09). Based on the MS2 spectrum, we tentatively identify this peak as a BHT cyclitol ether with a methylation on the head group: BHT-Me-CE (AEC: C42H76O8N+, 722.557, Δ ppm −1.64). Note that we do not observe a loss of 32 Da indicative of a methoxy moiety (CH4O+); therefore we propose that the methyl group occurs on the ring structure of the cyclitol ether.
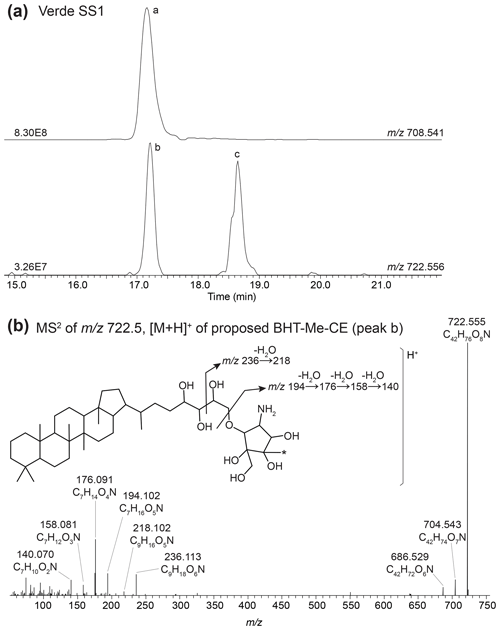
Figure B5(a) Partial mass chromatogram of BHT cyclitol ether (BHT-CE, peak a) and newly proposed BHT-Me-CE (peak b) in a sediment sample from Lake Verde. (b) MS2 spectrum of [M +,H]+ BHT-Me-CE (peak b) and a proposed structure for BHT-Me-CE with the diagnostic fragmentation of the cyclitol ether indicated. (Note that the asterisk (*) indicates that the position of the methyl group is unknown; the additional methylation could also occur at the C-39 or C-40 position.)
B4 Ethenolamine-BHPs
In our samples we identified ethenolamine-BHT (peak a), ethenolamine-BHpentol (peak d), and ethenolamine-BHhexol (peak h) after Hopmans et al. (2021; Fig. 3a). In addition, we identified unsaturated ethenolamine-BHT (peak b) and unsaturated ethenolamine-BHhexol (peak i) in the mass chromatograms of 586.483 (C37H64O4N+) and 618.474 (C37H64O6N+), respectively. The fragmentation spectra of both unsaturated ethenolamine-BHPs (Figs. 3e and B6, respectively) showed the characteristic loss of 41 Da (C2H3N) and 59 Da (C2H5ON) related to the loss of the ethenolamine moiety. In both fragmentation spectra we observe the expected fragment for unsaturation on the BHP core structure, i.e., 473.413 (C35H, Δ ppm 1.54) for an unsaturated BHT and 469.382 (C35H, Δ ppm 2.08) for an unsaturated BHhexol. The offset in retention time between ethenolamine-BHT and unsaturated ethenolamine-BHT (2.33 min) and ethenolamine-BHhexol and unsaturated ethenolamine-BHhexol (1.51 min), respectively, is similar to the offset between BHT and Δ6-BHT (2.34 min) and BHT and Δ11-BHT (1.38 min), respectively, as observed by Hopmans et al. (2021). Based on the retention time offset, we propose that the unsaturation in peaks b and i is at the Δ6 and Δ11 position, respectively. In the lower mass range of the MS2 spectrum of the proposed Δ6-ethenolamine-BHT we observe some deviations from the expected fragments for a Δ6-BHT, i.e., a relatively dominant 177 and 201 instead of 203, which is expected for a Δ6-BHT core (Hopmans et al., 2021). Perhaps the presence of a conjugation alters the fragmentation pattern of an unsaturated core to a small degree. The MS2 spectrum of the proposed Δ11-ethenolamine-BHhexol (peak i) also shows the dominant fragment ion at 177 but does show the expected fragment ion at 203 for unsaturation at the Δ11 position.
B5 N-formylated aminoBHPs
We detected two unknown composite BHPs previously described in Hopmans et al. (2021) in the partial mass chromatograms of 574.483 (C36H64O4N+) and 590.483 (C36H64O5N+). In addition to several consecutive losses of water representing three or four hydroxyl moieties on the side chain, the fragmentation spectra of both of these unknown composite BHPs is characterized by an initial loss of 28 Da (CO). Here, we detected these novel BHPs in sediment from Lake Verde (Fig. 3b, peaks j and m). In addition, we identified an additional BHP with 606.473 and an AEC of C36H64O6N+ (Fig. 3b and f, peak o) with a similar fragmentation: a loss of 28 Da (CO) from the original mass fragment produces the fragment at 578.484 (C35H64O5N+, Δ ppm −5.81), and a loss of a hydroxyl moiety (C36H62O5N+, 588.461, Δ ppm 1.29) followed by a loss of 28 Da (CO) results in the fragment at 560.468 (C35H62O4N+, Δ ppm −1.76). We also observe five sequential losses of hydroxyl moieties from the parent ion to produce 588.461 (C36H62O5N+, Δ ppm 2.96), 570.451 (C36H60O4N+, Δ ppm −0.17), 552.441 (C36H58O3N+, Δ ppm 2.03), 534.431 (C36H56O2N+, Δ ppm −1.92), and 516.420 (C36H54ON+, Δ ppm 1.09), respectively. In the fragmentation spectra of peaks j, m, and o we observe losses of 45 Da (CONH3), e.g., from 534 to 489 for peak o (Fig. 3f). Based on these losses we propose that these novel composite BHPs are a series of N-formylated aminoBHPs: N-formylated aminotriol (peak j), N-formylated aminotetrol (peak m), and N-formylated aminopentol (peak o). The proposed structure for N-formylated aminopentol is shown in Fig. 3f with the diagnostic fragmentation indicated.
We also observe unsaturated versions of N-formylated aminotriol, N-formylated aminotetrol, and N-formylated aminopentol based on the elemental composition C36H62O4N+ ( 572.467, peak k), C36H62O5N+ ( 588.462, peak n), and C36H62O6N 604.458, peak p), respectively. In the lower mass range of the 572.467 spectrum, we observe dominant 163.148 (C12H, Δ ppm −0.14), 177.163 (C13H, Δ ppm 0.10), 201.164 (C15H, Δ ppm 0.53), and 203.179 (C15H, Δ ppm −0.46) fragments and a notable absence of a dominant 191.179 (C14H). This is similar to the fragmentation patterns observed for unsaturated ethenolamine-BHT (Fig. B7) and unsaturated ethenolamine-BHhexol (Fig. 3e) as discussed in Sect. B4. We also observe the corresponding fragments of the D and E ring with a partially dehydroxylated side chain in the spectra: 350.271 (C21H36O3N+, Δ ppm −3.31) and after two additional hydroxyl losses, 314.249 (C21H32ON+, Δ ppm −5.95) and the loss of the hexane ring on the side chain to get 231.210 (C17H, Δ ppm 0.03). This indicates that unsaturation is not on the side chain or the D and E rings. Based on the presence of the 201.164 fragment and a similar offset in retention times between N-formylated aminotriol and unsaturated N-formylated aminotriol (2.25 min; Table A1) to that of ethenolamine-BHT and unsaturated ethenolamine-BHT (2.33 min; Table A1), we tentatively assign the double bond to the Δ6 position. In 588.462 (C36H62O5N+; Fig. 3b), we identify peak n as unsaturated N-formylated aminotetrol based on the MS1 spectrum. However, due to a low MS2 spectrum we cannot identify the position of the double bond. Based on the retention time, the double bond is likely at the Δ6 position. The difference in retention time between N-formylated aminopentol and unsaturated N-formylated aminopentol (1.49 min) is similar to that of the ethenolamine-BHhexol and unsaturated ethenolamine-BHhexol (1.51 min); therefore the double bond likely occurs in the same place for both compounds. We therefore tentatively identify peak p as Δ11-N-formylated aminopentol.
B6 Oxazinone-aminotriol
In the mass chromatogram for 572.467, in addition to the unsaturated N-formylated aminotriol (peak k), we observe a later-eluting peak (C36H62O4N+; Fig. 3b and d, peak l). The MS2 spectrum of this peak contains a loss of 44 Da (CO2) from the original mass fragment to produce 528.477 (C35H62O2N+, Δ ppm −1.33) followed by a loss of two hydroxyl moieties (C35H60ON+, 510.466, Δ ppm 1.36; C35H58N+, 492.457, Δ ppm −2.92). We propose that this is the same compound identified in Elling et al. (2022). The authors describe an acetylated compound (C40H66O6N+, 656.495), with the unique loss of 44 Da (CO2), the loss of two hydroxyl moieties, and an additional loss of 17 Da (NH). We indicate the loss of 17 Da (NH) in the proposed structure; however, we do not observe this loss in our MS2 spectrum, which could be attributed to low peak intensity. Based on the proposed structure, this appears to be the cyclized form of the N-formylated aminotriol. The International Union of Pure and Applied Chemistry (IUPAC) name for the cyclized part of the BHP is 1,3-oxazinan-2-one (National Center for Biotechnology Information, 2023a); therefore we refer to this compound as oxazinone-aminotriol.
B7 Dioxanone-methylaminotriol
In the mass chromatogram of 586.483 (C37H64O4N+, Δ ppm 0.72), an additional peak occurs at 21.88 min (Fig. 3a, peak c). Similar to the above-described N-formylated aminoBHPs, the MS2 spectrum is characterized by the distinct loss of 28 Da producing 558.488 (C36H64O3N+, Δ ppm −0.37; Fig. 3c). In addition, a loss of 31 Da (CNH5) is observed from 522.467 (C36H60ON+, Δ ppm 0.04) to produce the fragment at 491.424 (C35H55O+, Δ ppm 1.98), indicating a second conjugated moiety. Based on the elemental composition there appears to be an additional DBE (double-bond equivalent) in the structure, but there is no indication unsaturation is located in the core structure as the lower mass range of the fragmentation spectrum is similar to what is expected for BHT. We therefore assume one of the functionalities is part of a cyclized structure and propose this BHP is a type of N-formylated aminotriol, where the formic acid is part of a cyclic structure and the terminal amino group is methylated. The IUPAC name for the cyclic part of the structure is 1,3-dioxan-2-one (National Center for Biotechnology Information, 2023b); therefore we refer to this compound as a dioxanone-methylaminotriol. The proposed structure with key fragmentation is shown in Fig. 3c.
B8 Aminohexol BHPs
A search for additional BHPs in the 191.179 (C14H) fragment spectra revealed two early-eluting peaks in the mass chromatogram for 594.473 (C35H64O6N+) in the Methylobacter–Methylotenera co-culture (Fig. 9a). The MS2 spectrum for aminohexol I (Fig. 9b) shows the consecutive loss of five hydroxyl moieties to produce fragments at 594.473 (C35H64O6N+, Δ ppm −0.36), 576.462 (C35H62O5N+, Δ ppm 0.59), 558.454 (C35H60O4N+, Δ ppm −3.55), 540.441 (C35H58O3N+, Δ ppm 0.83), 522.432 (C35H56O2N+, Δ ppm −2.30), and 504.418 (C35H54ON+, Δ ppm 3.04). Based on the presence of 369.354 (C27H, Δ ppm −6.91) in the MS2 spectrum, the additional hydroxyl moiety is not located in the ring system. The position of the additional hydroxyl moiety on the tail is unknown. Similarly, the MS2 spectrum for aminohexol II (Fig. 9c) also shows a loss of five hydroxyl moieties and an additional loss of 29 Da (CH3N) from 504.422 (C35H54ON+, Δ ppm −3.01) to 475.393 (C34H51O+, Δ ppm 0.99). However, in the fragmentation spectrum of peak b, we observe several fragments that appear to represent the functionalized tail at 180.086 (C6H14O5N+, Δ ppm −0.12), 162.076 (C6H12O4N+, Δ ppm 0.95), 144.0655 (C6H10O3N+, Δ ppm −0.35), and 126.055 (C6H8O2N+, Δ ppm −1.55). The complementary ion to the tail fragments is observed at 395.368 (C29H, Δ ppm −0.06), indicating fragmentation at the C-22–C-30 bond. The presence of the 369.354 (C27H, Δ ppm −0.20) fragment indicates a hopanoid core without the addition of an extra hydroxyl group. We propose that in this isomer, the additional hydroxyl group is located at C-22 on the side chain, resulting in the initial fragmentation of the side chain and the progressive loss of the hydroxyl groups from the side chain fragment. Based on the MS2 spectra, we tentatively identify these compounds as aminohexol BHPs.
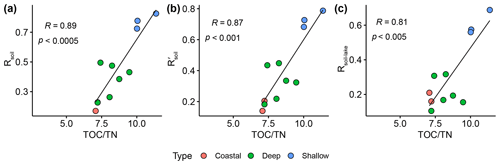
Figure C1Correlation between (a) Rsoil, (b) , and (c) Rsoil-lake with TOC TN. The different colors represent the different types of samples sites in this study: coastal (Cubres East and Cubres West), deep (Azul, Funda, and Negra), and shallow (Empadadas, São Jorge, and Lomba).
Mass spectra published in this paper will be added to the open-access Global Natural Products Social (GNPS) Molecular Networking library for public use (https://gnps.ucsd.edu/ProteoSAFe/static/gnps-splash.jsp, Wang et al., 2016). All additional data produced in this study are available in the Supplement.
The supplement related to this article is available online at: https://doi.org/10.5194/bg-20-2065-2023-supplement.
The study was conceptualized by NR, LAAZ, and DR. The investigation was carried out by all co-authors. Formal analysis was conducted by NR, ECH, and DM. ECH, PMR, VG, ACC, and LV contributed resources to make this project possible. Funding was acquired by LAAZ, PMR, LV, and DR. NR wrote the manuscript, and all co-authors contributed to the manuscript.
The contact author has declared that none of the authors has any competing interests.
Publisher’s note: Copernicus Publications remains neutral with regard to jurisdictional claims in published maps and institutional affiliations.
This work was supported by the Luso-American Development Foundation (“Crossing the Atlantic”) (Linda A. Amaral-Zettler), the national funds through the Foundation for Science and Technology (FCT; project no. UIDB/50027/2020), Pedro M. Raposeiro (grant no. DL57/2016/ICETA/EEC2018/25), and the Dutch Research Council (NWO) Gravitation grant for the Soehngen Institute of Anaerobic Microbiology (grant no. 024.002.002). We would like to thank everyone who participated and assisted with the field campaign to the Azores Archipelago in 2018, particularly Erik Zettler. Further, we would like to thank Sigrid van Grinsven for the co-culture and advice. We thank Nicole Bale, Anchelique Mets, Monique Verweij, Denise Dorhout, Jort Ossebaar, Ronald van Bommel, Marcel van der Meer, Ilsa Posthuma, Anna Nordeloos, and Michele Grego for technical support and advice.
This research has been supported by the Luso-American Development Foundation (“Crossing the Atlantic”), the Foundation for Science and Technology (grant nos. UIDB/50027/2020 and DL57/2016/ICETA/EEC2018/25), and the Soehngen Institute of Anaerobic Microbiology (grant no. 024.002.002).
This paper was edited by Sebastian Naeher and reviewed by Juliana Drozd and one anonymous referee.
Bale, N. J., Rijpstra, W. I. C., Sahonero-Canavesi, D. X., Oshkin, I. Y., Belova, S. E., Dedysh, S. N., and Sinninghe Damsté, J. S.: Fatty Acid and Hopanoid Adaption to Cold in the Methanotroph Methylovulum psychrotolerans, Front. Microbiol., 10, 589, https://doi.org/10.3389/fmicb.2019.00589, 2019.
Bale, N. J., Ding, S., Hopmans, E. C., Arts, M. G. I., Villanueva, L., Boschman, C., Haas, A. F., Schouten, S., and Sinninghe Damsté, J. S.: Lipidomics of Environmental Microbial Communities, I: Visualization of Component Distributions Using Untargeted Analysis of High-Resolution Mass Spectrometry Data, Front. Microbiol., 12, 659302, https://doi.org/10.3389/fmicb.2021.659302, 2021.
Bastviken, D., Cole, J. J., Pace, M. L., and Van de Bogert, M. C.: Fates of methane from different lake habitats: Connecting whole-lake budgets and CH4 emissions, J. Geophys. Res.-Biogeo., 113, G2, https://doi.org/10.1029/2007JG000608, 2008.
Bligh, E. G. and Dyer, W. J.: A rapid method of total lipid extraction and purification, Can. J. Biochem. Physiol., 37, 911–917, https://doi.org/10.1139/o59-099, 1959.
Blumenberg, M., Krüger, M., Nauhaus, K., Talbot, H. M., Oppermann, B. I., Seifert, R., Pape, T., and Michaelis, W.: Biosynthesis of hopanoids by sulfate-reducing bacteria (genus Desulfovibrio), Environ. Microbiol., 8, 1220–1227, https://doi.org/10.1111/j.1462-2920.2006.01014.x, 2006.
Blumenberg, M., Seifert, R., Kasten, S., Bahlmann, E., and Michaelis, W.: Euphotic zone bacterioplankton sources major sedimentary bacteriohopanepolyols in the Holocene Black Sea, Geochim. Cosmochim. Ac., 73, 750–766, https://doi.org/10.1016/j.gca.2008.11.005, 2009.
Blumenberg, M., Hoppert, M., Krüger, M., Dreier, A., and Thiel, V.: Novel findings on hopanoid occurrences among sulfate reducing bacteria: Is there a direct link to nitrogen fixation?, Org. Geochem., 49, 1–5, https://doi.org/10.1016/j.orggeochem.2012.05.003, 2012.
Blumenberg, M., Berndmeyer, C., Moros, M., Muschalla, M., Schmale, O., and Thiel, V.: Bacteriohopanepolyols record stratification, nitrogen fixation and other biogeochemical perturbations in Holocene sediments of the central Baltic Sea, Biogeosciences, 10, 2725–2735, https://doi.org/10.5194/bg-10-2725-2013, 2013.
Bradley, A. S., Pearson, A., Sáenz, J. P., and Marx, C. J.: Adenosylhopane: The first intermediate in hopanoid side chain biosynthesis, Org. Geochem., 41, 1075–1081, https://doi.org/10.1016/j.orggeochem.2010.07.003, 2010.
Bravo, J.-M., Perzl, M., Härtner, T., Kannenberg, E. L., and Rohmer, M.: Novel methylated triterpenoids of the gammacerane series from the nitrogen-fixing bacterium Bradyrhizobium japonicum USDA 110, Eur. J. Biochem., 268, 1323–1331, https://doi.org/10.1046/j.1432-1327.2001.01998.x, 2001.
Cooke, M. P., Talbot, H. M., and Farrimond, P.: Bacterial populations recorded in bacteriohopanepolyol distributions in soils from Northern England, Org. Geochem., 39, 1347–1358, https://doi.org/10.1016/j.orggeochem.2008.05.003, 2008.
Cooke, M. P., van Dongen, B. E., Talbot, H. M., Semiletov, I., Shakhova, N., Guo, L., and Gustafsson, Ö.: Bacteriohopanepolyol biomarker composition of organic matter exported to the Arctic Ocean by seven of the major Arctic rivers, Org. Geochem., 40, 1151–1159, https://doi.org/10.1016/j.orggeochem.2009.07.014, 2009.
Coolen, M. J. L., Talbot, H. M., Abbas, B. A., Ward, C., Schouten, S., Volkman, J. K., and Sinninghe Damsté, J. S.: Sources for sedimentary bacteriohopanepolyols as revealed by 16S rDNA stratigraphy, Environ. Microbiol., 10, 1783–1803, https://doi.org/10.1111/j.1462-2920.2008.01601.x, 2008.
Cordeiro, R., Luz, R., Vilaverde, J., Vasconcelos, V., Fonseca, A., and Gonçalves, V.: Distribution of Toxic Cyanobacteria in Volcanic Lakes of the Azores Islands, Water, 12, 3385, https://doi.org/10.3390/w12123385, 2020.
Cvejic, J. H., Bodrossy, L., Kovács, K. L., and Rohmer, M.: Bacterial triterpenoids of the hopane series from the methanotrophic bacteria Methylocaldum spp.: phylogenetic implications and first evidence for an unsaturated aminobacteriohopanepolyol, FEMS Microbiol. Lett., 182, 361–365, https://doi.org/10.1111/j.1574-6968.2000.tb08922.x, 2000.
De Jonge, C., Talbot, H. M., Bischoff, J., Stadnitskaia, A., Cherkashov, G., and Sinninghe Damsté, J. S.: Bacteriohopanepolyol distribution in Yenisei River and Kara Sea suspended particulate matter and sediments traces terrigenous organic matter input, Geochim. Cosmochim. Ac., 174, 85–101, https://doi.org/10.1016/j.gca.2015.11.008, 2016.
Doðrul Selver, A., Talbot, H. M., Gustafsson, Ö., Boult, S., and van Dongen, B. E.: Soil organic matter transport along an sub-Arctic river–sea transect, Org. Geochem., 51, 63–72, https://doi.org/10.1016/j.orggeochem.2012.08.002, 2012.
Doðrul Selver, A., Sparkes, R. B., Bischoff, J., Talbot, H. M., Gustafsson, Ö., Semiletov, I. P., Dudarev, O. V., Boult, S., and van Dongen, B. E.: Distributions of bacterial and archaeal membrane lipids in surface sediments reflect differences in input and loss of terrestrial organic carbon along a cross-shelf Arctic transect, Org. Geochem., 83/84, 16–26, https://doi.org/10.1016/j.orggeochem.2015.01.005, 2015.
Doughty, D. M., Coleman, M. L., Hunter, R. C., Sessions, A. L., Summons, R. E., and Newman, D. K.: The RND-family transporter, HpnN, is required for hopanoid localization to the outer membrane of Rhodopseudomonas palustris TIE-1, P. Natl. Acad. Sci. USA, 108, E1045–E1051, https://doi.org/10.1073/pnas.1104209108, 2011.
Elling, F. J., Evans, T. W., Nathan, V., Hemingway, J. D., Kharbush, J. J., Bayer, B., Spieck, E., Husain, F., Summons, R. E., and Pearson, A.: Marine and terrestrial nitrifying bacteria are sources of diverse bacteriohopanepolyols, Geobiology, 20, 399–420, https://doi.org/10.1111/gbi.12484, 2022.
Farrimond, P., Head, I. M., and Innes, H. E.: Environmental influence on the biohopanoid composition of recent sediments, Geochim. Cosmochim. Ac., 64, 2985–2992, https://doi.org/10.1016/S0016-7037(00)00404-X, 2000.
Gonçalves, V.: Contribuição do estudo das microalgas para a avaliação da qualidade ecológica das lagoas dos Açores: fitoplâncton e diatomáceas bentónicas, PhD thesis, University of the Azores, Ponta Delgada, 343 pp., 2008.
Gonçalves, V., Raposeiro, P. M., Marques, H. S., Vilaverde, J., Balibrea, A., Rosa, F., Sixto, M., and Costa, A. C.: Monitorização das Massas de Água Interiores e de Transição da Região Hidrográfica dos Açores. Relatório Anual do Ano 3 (R5/Ano 3) Ponta Delgada, University of the Azores, 2018.
Guggenheim, C., Freimann, R., Mayr, M. J., Beck, K., Wehrli, B., and Bürgmann, H.: Environmental and Microbial Interactions Shape Methane-Oxidizing Bacterial Communities in a Stratified Lake, Front. Microbiol., 11, 579427, https://doi.org/10.3389/fmicb.2020.579427, 2020.
Hanson, R. S.: Ecology and Diversity of Methylotrophic Organisms, in: Advances in Applied Microbiology, vol. 26, edited by: Perlman, D., Academic Press, 3–39, https://doi.org/10.1016/S0065-2164(08)70328-9, 1980.
Hanson, R. S. and Hanson, T. E.: Methanotrophic bacteria, Microbiol. Rev., 60, 439–471, https://doi.org/10.1128/mr.60.2.439-471.1996, 1996.
Harrits, S. M. and Hanson, R. S.: Stratification of aerobic methane-oxidizing organisms in Lake Mendota, Madison, Wisconsin, Limnol. Oceanogr., 25, 412–421, https://doi.org/10.4319/lo.1980.25.3.0412, 1980.
Hernández, A., Kutiel, H., Trigo, R. M., Valente, M. A., Sigró, J., Cropper, T., and Santo, F. E.: New Azores archipelago daily precipitation dataset and its links with large-scale modes of climate variability, Int. J. Climatol., 36, 4439–4454, https://doi.org/10.1002/joc.4642, 2016.
Hopmans, E. C., Smit, N. T., Schwartz-Narbonne, R., Sinninghe Damsté, J. S., and Rush, D.: Analysis of non-derivatized bacteriohopanepolyols using UHPLC-HRMS reveals great structural diversity in environmental lipid assemblages, Org. Geochem., 160, 104285, https://doi.org/10.1016/j.orggeochem.2021.104285, 2021.
Kusch, S. and Rush, D.: Revisiting the precursors of the most abundant natural products on Earth: A look back at 30+ years of bacteriohopanepolyol (BHP) research and ahead to new frontiers, Org. Geochem., 172, 104469, https://doi.org/10.1016/j.orggeochem.2022.104469, 2022.
Kusch, S., Sepúlveda, J., and Wakeham, S. G.: Origin of Sedimentary BHPs Along a Mississippi River–Gulf of Mexico Export Transect: Insights From Spatial and Density Distributions, Front. Mar. Sci., 6, 729, https://doi.org/10.3389/fmars.2019.00729, 2019.
Kusch, S., Wakeham, S. G., Dildar, N., Zhu, C., and Sepúlveda, J.: Bacterial and archaeal lipids trace chemo(auto)trophy along the redoxcline in Vancouver Island fjords, Geobiology, 19, 521–541, https://doi.org/10.1111/gbi.12446, 2021a.
Kusch, S., Wakeham, S. G., and Sepúlveda, J.: Diverse origins of “soil marker” bacteriohopanepolyols in marine oxygen deficient zones, Org. Geochem., 151, 104150, https://doi.org/10.1016/j.orggeochem.2020.104150, 2021b.
Matys, E. D., Sepúlveda, J., Pantoja, S., Lange, C. B., Caniupán, M., Lamy, F., and Summons, R. E.: Bacteriohopanepolyols along redox gradients in the Humboldt Current System off northern Chile, Geobiology, 15, 844–857, https://doi.org/10.1111/gbi.12250, 2017.
Meyers, P. A.: Preservation of elemental and isotopic source identification of sedimentary organic matter, Chem. Geol., 114, 289–302, https://doi.org/10.1016/0009-2541(94)90059-0, 1994.
Meyers, P. A.: Applications of organic geochemistry to paleolimnological reconstructions: a summary of examples from the Laurentian Great Lakes, Org. Geochem., 34, 261–289, https://doi.org/10.1016/S0146-6380(02)00168-7, 2003.
Mitrović, D., Hopmans, E. C., Bale, N. J., Richter, N., Amaral-Zettler, L. A., Baxter, A. J., Peterse, F., Miguel Raposeiro, P., Gonçalves, V., Cristina Costa, A., and Schouten, S.: Isoprenoidal GDGTs and GDDs associated with anoxic lacustrine environments, Org. Geochem., 178, 104582, https://doi.org/10.1016/j.orggeochem.2023.104582, 2023.
National Center for Biotechnology Information: PubChem Compound Summary for CID 641496, 1,3-Oxazinan-2-one, https://pubchem.ncbi.nlm.nih.gov/compound/1_3-Oxazinan-2-one, last access: 26 January 2023a.
National Center for Biotechnology Information: PubChem Compound Summary for CID 123834, 1,3-Dioxan-2-one, https://pubchem.ncbi.nlm.nih.gov/compound/1_3-Dioxan-2-one, last access: 26 January 2023b.
Neunlist, S. and Rohmer, M.: A novel hopanoid, 30-(5′-adenosyl)hopane, from the purple non-sulphur bacterium Rhodopseudomonas acidophila, with possible DNA interactions, Biochem. J., 228, 769–771, 1985a.
Neunlist, S. and Rohmer, M.: Novel hopanoids from the methylotrophic bacteria Methylococcus capsulatus and Methylomonas methanica. (22S)-35-aminobacteriohopane-30,31,32,33,34-pentol and (22S)-35-amino-3β-methylbacteriohopane-30,31,32,33,34-pentol, Biochem. J., 231, 635–639, https://doi.org/10.1042/bj2310635, 1985b.
Neunlist, S. and Rohmer, M.: The Hopanoids of “Methylosinus trichosporium”: Aminobacteriohopanetriol and Aminobacteriohopanetetrol, Microbiology, 131, 1363–1367, https://doi.org/10.1099/00221287-131-6-1363, 1985c.
Neunlist, S., Bisseret, P., and Rohmer, M.: The hopanoids of the purple non-sulfur bacteria Rhodopseudomonas palustris and Rhodopseudomonas acidophila and the absolute configuration of bacteriohopanetetrol, Eur. J. Biochem., 171, 245–252, https://doi.org/10.1111/j.1432-1033.1988.tb13783.x, 1988.
O'Beirne, M. D., Sparkes, R., Hamilton, T. L., van Dongen, B. E., Gilhooly, W. P., and Werne, J. P.: Characterization of diverse bacteriohopanepolyols in a permanently stratified, hyper-euxinic lake, Org. Geochem., 168, 104431, https://doi.org/10.1016/j.orggeochem.2022.104431, 2022.
Oksanen, J., Simpson, G., Blanchet, F., Kindt, R., Legendre, P., Minchin, P., O'Hara, R., Solymos, P., Stevens, M., Szoecs, E., Wagner, H., Barbour, M., Bedward, M., Bolker, B., Borcard, D., Carvalho, G., Chirico, M., De Caceres, M., Durand, S., Evangelista, H., FitzJohn, R., Friendly, M., Furneaux, B., Hannigan, G., Hill, M., Lahti, L., McGlinn, D., Ouellette, M., Ribeiro Cunha, E., Smith, T., Stier, A., Ter Braak C., and Weedon J.: vegan: Community Ecology Package R package version 2.6-4, https://CRAN.R-project.org/package=vegan (last access: 4 May 2023), 2022.
Osborne, K. A.: Environmental controls on bacteriohopanepolyol signatures in estuarine sediments, Newcastle University, UK, PhD thesis, Newcastle University, http://theses.ncl.ac.uk/jspui/handle/10443/3214 (last access: 4 May 2023), 2015.
Osborne, K. A., Gray, N. D., Sherry, A., Leary, P., Mejeha, O., Bischoff, J., Rush, D., Sidgwick, F. R., Birgel, D., Kalyuzhnaya, M. G., and Talbot, H. M.: Methanotroph-derived bacteriohopanepolyol signatures as a function of temperature related growth, survival, cell death and preservation in the geological record, Environ. Microbiol. Rep., 9, 492–500, https://doi.org/10.1111/1758-2229.12570, 2017.
Oswald, K., Milucka, J., Brand, A., Littmann, S., Wehrli, B., Kuypers, M. M. M., and Schubert, C. J.: Light-Dependent Aerobic Methane Oxidation Reduces Methane Emissions from Seasonally Stratified Lakes, PLOS ONE, 10, e0132574, https://doi.org/10.1371/journal.pone.0132574, 2015.
Oswald, K., Milucka, J., Brand, A., Hach, P., Littmann, S., Wehrli, B., Kuypers, M. M. M., and Schubert, C. J.: Aerobic gammaproteobacterial methanotrophs mitigate methane emissions from oxic and anoxic lake waters, Limnol. Oceanogr., 61, S101–S118, https://doi.org/10.1002/lno.10312, 2016.
Ourisson, G. and Albrecht, P.: Hopanoids, 1. Geohopanoids: the most abundant natural products on Earth?, Acc. Chem. Res., 25, 398–402, https://doi.org/10.1021/ar00021a003, 1992.
Pearson, A., Leavitt, W. D., Sáenz, J. P., Summons, R. E., Tam, M. C.-M., and Close, H. G.: Diversity of hopanoids and squalene-hopene cyclases across a tropical land-sea gradient, Environ. Microbiol., 11, 1208–1223, https://doi.org/10.1111/j.1462-2920.2008.01817.x, 2009.
Pereira, C. L., Raposeiro, P. M., Costa, A. C., Bao, R., Giralt, S., and Gonçalves, V.: Biogeography and lake morphometry drive diatom and chironomid assemblages' composition in lacustrine surface sediments of oceanic islands, Hydrobiologia, 730, 93–112, https://doi.org/10.1007/s10750-014-1824-6, 2014.
R Core Team: R: A language and environment for statistical computing, R Foundation for Statistical Computing, Vienna, Austria, URL https://www.R-project.org/ (last access: 4 May 2023), 2023.
Raposeiro, P. M., Rubio, M. J., González, A., Hernández, A., Sánchez-López, G., Vázquez-Loureiro, D., Rull, V., Bao, R., Costa, A. C., Gonçalves, V., Sáez, A., and Giralt, S.: Impact of the historical introduction of exotic fishes on the chironomid community of Lake Azul (Azores Islands), Palaeogeogr. Palaeocl., 466, 77–88, https://doi.org/10.1016/j.palaeo.2016.11.015, 2017.
Raposeiro, P. M., Saez, A., Giralt, S., Costa, A. C., and Gonçalves, V.: Causes of spatial distribution of subfossil diatom and chironomid assemblages in surface sediments of a remote deep island lake, Hydrobiologia, 815, 141–163, https://doi.org/10.1007/s10750-018-3557-4, 2018.
Rethemeyer, J., Schubotz, F., Talbot, H. M., Cooke, M. P., Hinrichs, K.-U., and Mollenhauer, G.: Distribution of polar membrane lipids in permafrost soils and sediments of a small high Arctic catchment, Org. Geochem., 41, 1130–1145, https://doi.org/10.1016/j.orggeochem.2010.06.004, 2010.
Richter, N., Russell, J. M., Amaral-Zettler, L., DeGroff, W., Raposeiro, P. M., Gonçalves, V., de Boer, E. J., Pla-Rabes, S., Hernández, A., Benavente, M., Ritter, C., Sáez, A., Bao, R., Trigo, R. M., Prego, R., and Giralt, S.: Long-term hydroclimate variability in the sub-tropical North Atlantic and anthropogenic impacts on lake ecosystems: A case study from Flores Island, the Azores, Quaternary Sci. Rev., 285, 107525, https://doi.org/10.1016/j.quascirev.2022.107525, 2022.
Rohmer, M., Bouvier-Nave, P., and Ourisson, G. 1984: Distribution of Hopanoid Triterpenes in Prokaryotes, Microbiology, 130, 1137–1150, https://doi.org/10.1099/00221287-130-5-1137, 1984.
Rudd, J. W. M., Furutani, A., Flett, R. J., and Hamilton, R. D.: Factors controlling methane oxidation in shield lakes: The role of nitrogen fixation and oxygen concentration1, Limnol. Oceanogr., 21, 357–364, https://doi.org/10.4319/lo.1976.21.3.0357, 1976.
Rush, D., Osborne, K. A., Birgel, D., Kappler, A., Hirayama, H., Peckmann, J., Poulton, S. W., Nickel, J. C., Mangelsdorf, K., Kalyuzhnaya, M., Sidgwick, F. R., and Talbot, H. M.: The Bacteriohopanepolyol Inventory of Novel Aerobic Methane Oxidising Bacteria Reveals New Biomarker Signatures of Aerobic Methanotrophy in Marine Systems, PLOS ONE, 11, e0165635, https://doi.org/10.1371/journal.pone.0165635, 2016.
Rush, D., Talbot, H. M., van der Meer, M. T. J., Hopmans, E. C., Douglas, B., and Sinninghe Damsté, J. S.: Biomarker evidence for the occurrence of anaerobic ammonium oxidation in the eastern Mediterranean Sea during Quaternary and Pliocene sapropel formation, Biogeosciences, 16, 2467–2479, https://doi.org/10.5194/bg-16-2467-2019, 2019.
Sáenz, J. P.: Hopanoid enrichment in a detergent resistant membrane fraction of Crocosphaera watsonii: Implications for bacterial lipid raft formation, Org. Geochem., 41, 853–856, https://doi.org/10.1016/j.orggeochem.2010.05.005, 2010.
Sáenz, J. P., Eglinton, T. I., and Summons, R. E.: Abundance and structural diversity of bacteriohopanepolyols in suspended particulate matter along a river to ocean transect, Org. Geochem., 42, 774–780, https://doi.org/10.1016/j.orggeochem.2011.05.006, 2011.
Sáenz, J. P., Sezgin, E., Schwille, P., and Simons, K.: Functional convergence of hopanoids and sterols in membrane ordering, P. Natl. Acad. Sci. USA, 109, 14236–14240, https://doi.org/10.1073/pnas.1212141109, 2012.
Santos, F. D., Valente, M. A., Miranda, P. M. A., Aguiar, A., Azevedo, E. B., Tomé, A. R., and Coelho, F.: Climate change scenarios in the Azores and Madeira Islands, World Resour. Rev., 16, 473–491, 2004.
Seemann, M., Bisseret, P., Tritz, J.-P., Hooper, A. B., and Rohmer, M.: Novel bacterial triterpenoids of the hopane series from Nitrosomonas europaea and their significance for the formation of the C35 bacteriohopane skeleton, Tetrahedron Lett., 40, 1681–1684, https://doi.org/10.1016/S0040-4039(99)00064-7, 1999.
Sinninghe Damsté, J. S., Rijpstra, W. I. C., Dedysh, S. N., Foesel, B. U., and Villanueva, L.: Pheno- and Genotyping of Hopanoid Production in Acidobacteria, Front. Microbiol., 8, 968, https://doi.org/10.3389/fmicb.2017.00968, 2017.
Spencer-Jones, C. L., Wagner, T., Dinga, B. J., Schefuß, E., Mann, P. J., Poulsen, J. R., Spencer, R. G. M., Wabakanghanzi, J. N., and Talbot, H. M.: Bacteriohopanepolyols in tropical soils and sediments from the Congo River catchment area, Org. Geochem., 89–90, 1–13, https://doi.org/10.1016/j.orggeochem.2015.09.003, 2015.
Summons, R. E., Jahnke, L. L., Hope, J. M., and Logan, G. A.: 2-Methylhopanoids as biomarkers for cyanobacterial oxygenic photosynthesis, Nature, 400, 554–557, https://doi.org/10.1038/23005, 1999.
Talbot, H. M. and Farrimond, P.: Bacterial populations recorded in diverse sedimentary biohopanoid distributions, Org. Geochem., 38, 1212–1225, https://doi.org/10.1016/j.orggeochem.2007.04.006, 2007.
Talbot, H. M., Watson, D. F., Murrell, J. C., Carter, J. F., and Farrimond, P.: Analysis of intact bacteriohopanepolyols from methanotrophic bacteria by reversed-phase high-performance liquid chromatography–atmospheric pressure chemical ionisation mass spectrometry, J. Chromatogr. A, 921, 175–185, https://doi.org/10.1016/S0021-9673(01)00871-8, 2001.
Talbot, H. M., Watson, D. F., Pearson, E. J., and Farrimond, P.: Diverse biohopanoid compositions of non-marine sediments, Org. Geochem., 34, 1353–1371, https://doi.org/10.1016/S0146-6380(03)00159-1, 2003.
Talbot, H. M., Rohmer, M., and Farrimond, P.: Rapid structural elucidation of composite bacterial hopanoids by atmospheric pressure chemical ionisation liquid chromatography/ion trap mass spectrometry, Rapid Commun. Mass Sp., 21, 880–892, https://doi.org/10.1002/rcm.2911, 2007.
Talbot, H. M., Summons, R. E., Jahnke, L. L., Cockell, C. S., Rohmer, M., and Farrimond, P.: Cyanobacterial bacteriohopanepolyol signatures from cultures and natural environmental settings, Org. Geochem., 39, 232–263, https://doi.org/10.1016/j.orggeochem.2007.08.006, 2008.
Talbot, H. M., Handley, L., Spencer-Jones, C. L., Dinga, B. J., Schefuß, E., Mann, P. J., Poulsen, J. R., Spencer, R. G. M., Wabakanghanzi, J. N., and Wagner, T.: Variability in aerobic methane oxidation over the past 1.2Myrs recorded in microbial biomarker signatures from Congo fan sediments, Geochim. Cosmochim. Ac., 133, 387–401, https://doi.org/10.1016/j.gca.2014.02.035, 2014.
Talbot, H. M., Bischoff, J., Inglis, G. N., Collinson, M. E., and Pancost, R. D.: Polyfunctionalised bio- and geohopanoids in the Eocene Cobham Lignite, Org. Geochem., 96, 77–92, https://doi.org/10.1016/j.orggeochem.2016.03.006, 2016.
Taylor, K. A. and Harvey, H. R.: Bacterial hopanoids as tracers of organic carbon sources and processing across the western Arctic continental shelf, Org. Geochem., 42, 487–497, https://doi.org/10.1016/j.orggeochem.2011.03.012, 2011.
Wagner, T., Kallweit, W., Talbot, H. M., Mollenhauer, G., Boom, A., and Zabel, M.: Microbial biomarkers support organic carbon transport from methane-rich Amazon wetlands to the shelf and deep sea fan during recent and glacial climate conditions, Org. Geochem., 67, 85–98, https://doi.org/10.1016/j.orggeochem.2013.12.003, 2014.
Watson, D. F. and Farrimond, P.: Novel polyfunctionalised geohopanoids in a recent lacustrine sediment (Priest Pot, UK), Org. Geochem., 31, 1247–1252, https://doi.org/10.1016/S0146-6380(00)00148-0, 2000.
Welander, P. V. and Summons, R. E.: Discovery, taxonomic distribution, and phenotypic characterization of a gene required for 3-methylhopanoid production, P. Natl. Acad. Sci. USA, 109, 12905–12910, https://doi.org/10.1073/pnas.1208255109, 2012.
Welander, P. V., Hunter, R. C., Zhang, L., Sessions, A. L., Summons, R. E., and Newman, D. K.: Hopanoids Play a Role in Membrane Integrity and pH Homeostasis in Rhodopseudomonas palustris TIE-1, J. Bacteriol., 191, 6145–6156, https://doi.org/10.1128/JB.00460-09, 2009.
Welander, P. V., Coleman, M. L., Sessions, A. L., Summons, R. E., and Newman, D. K.: Identification of a methylase required for 2-methylhopanoid production and implications for the interpretation of sedimentary hopanes, P. Natl. Acad. Sci. USA, 107, 8537–8542, https://doi.org/10.1073/pnas.0912949107, 2010.
Whittenbury, R., Phillips, K. C., and Wilkinson, J. F.: Enrichment, Isolation and Some Properties of Methane-utilizing Bacteria, J. General Microbiol., 61, 205–218, https://doi.org/10.1099/00221287-61-2-205, 1970.
Wickham, H.: ggplot2: Elegant Graphics for Data Analysis, Springer-Verlag New York, ISBN 978-3-319-24277-4, https://ggplot2.tidyverse.org (last access: 4 May 2023), 2016.
van Grinsven, S., Sinninghe Damsté, J. S., Harrison, J., and Villanueva, L.: Impact of Electron Acceptor Availability on Methane-Influenced Microorganisms in an Enrichment Culture Obtained From a Stratified Lake, Front. Microbiol., 11, 715, https://doi.org/10.3389/fmicb.2020.00715, 2020.
van Winden, J. F., Talbot, H. M., Kip, N., Reichart, G.-J., Pol, A., McNamara, N. P., Jetten, M. S. M., Op den Camp, H. J. M., and Sinninghe Damsté, J. S.: Bacteriohopanepolyol signatures as markers for methanotrophic bacteria in peat moss, Geochim. Cosmochim. Ac., 77, 52–61, https://doi.org/10.1016/j.gca.2011.10.026, 2012.
van Winden, J. F., Talbot, H. M., Reichart, G.-J., McNamara, N. P., Benthien, A., and Sinninghe Damsté, J. S.: Influence of temperature on the 13C values and distribution of methanotroph-related hopanoids in Sphagnum-dominated peat bogs, Geobiology, 18, 497–507, https://doi.org/10.1111/gbi.12389, 2020.
Wang, M., Carver, J. J., Phelan, V. V., Sanchez, L. M., Garg, N., Peng, Y., Nguyen, D. D., Watrous, J., Kapono, C. A., Luzzatto-Knaan, T., Porto, C., Bouslimani, A., Melnik, A. V., Meehan, M. J., Liu, W.-T., Crüsemann, M., Boudreau, P. D., Esquenazi, E., Sandoval-Calderón, M., Kersten, R. D., Pace, L. A., Quinn, R. A., Duncan, K. R., Hsu, C.-C., Floros, D. J., Gavilan, R. G., Kleigrewe, K., Northen, T., Dutton, R. J., Parrot, D., Carlson, E. E., Aigle, B., Michelsen, C. F., Jelsbak, L., Sohlenkamp, C., Pevzner, P., Edlund, A., McLean, J., Piel, J., Murphy, B. T., Gerwick, L., Liaw, C.-C., Yang, Y.-L., Humpf, H.-U., Maansson, M., Keyzers, R. A., Sims, A. C., Johnson, A. R., Sidebottom, A. M., Sedio, B. E., Klitgaard, A., Larson, C. B., Boya P, C. A., Torres-Mendoza, D., Gonzalez, D. J., Silva, D. B., Marques, L. M., Demarque, D. P., Pociute, E., O’Neill, E. C., Briand, E., Helfrich, E. J. N., Granatosky, E. A., Glukhov, E., Ryffel, F., Houson, H., Mohimani, H., Kharbush, J. J., Zeng, Y., Vorholt, J. A., Kurita, K. L., Charusanti, P., McPhail, K. L., Nielsen, K. F., Vuong, L., Elfeki, M., Traxler, M. F., Engene, N., Koyama, N., Vining, O. B., Baric, R., Silva, R. R., Mascuch, S. J., Tomasi, S., Jenkins, S., Macherla, V., Hoffman, T., Agarwal, V., Williams, P. G., Dai, J., Neupane, R., Gurr, J., Rodríguez, A. M. C., Lamsa, A., Zhang, C., Dorrestein, K., Duggan, B. M., Almaliti, J., Allard, P.-M., et al.: Sharing and community curation of mass spectrometry data with Global Natural Products Social Molecular Networking, Nat Biotechnol, 34, 828–837, https://doi.org/10.1038/nbt.3597, 2016 (data available at https://gnps.ucsd.edu/ProteoSAFe/static/gnps-splash.jsp, last access: 4 May 2023).
Xu, Y., Cooke, M. P., Talbot, H. M., and Simpson, M. J.: Bacteriohopanepolyol signatures of bacterial populations in Western Canadian soils, Org. Geochem., 40, 79–86, https://doi.org/10.1016/j.orggeochem.2008.09.003, 2009.
Zhu, C., Talbot, H. M., Wagner, T., Pan, J.-M., and Pancost, R. D.: Distribution of hopanoids along a land to sea transect: Implications for microbial ecology and the use of hopanoids in environmental studies, Limnol. Oceanogr., 56, 1850–1865, https://doi.org/10.4319/lo.2011.56.5.1850, 2011.
Zindorf, M., Rush, D., Jaeger, J., Mix, A., Penkrot, M. L., Schnetger, B., Sidgwick, F. R., Talbot, H. M., van der Land, C., Wagner, T., Walczak, M., and März, C.: Reconstructing oxygen deficiency in the glacial Gulf of Alaska: Combining biomarkers and trace metals as paleo-redox proxies, Chem. Geol., 558, 119864, https://doi.org/10.1016/j.chemgeo.2020.119864, 2020.
Zundel, M. and Rohmer, M.: Prokaryotic triterpenoids, Eur. J. Biochem., 150, 23–27, https://doi.org/10.1111/j.1432-1033.1985.tb08980.x, 1985.